- 1Department of Zoology, University of Otago, Dunedin, New Zealand
- 2Otago Regional Council, Dunedin, New Zealand
- 3Takahē Recovery Programme, Department of Conservation, Te Anau, New Zealand
- 4School of Geography, University of Otago, Dunedin, New Zealand
- 5Unit of Biodiversity and Conservation, Department of Biology and Geology, Physics and Inorganic Chemistry, Rey Juan Carlos University, Madrid, Spain
- 6Department of Ecology, Swedish University of Agricultural Sciences, Uppsala, Sweden
In response to anthropogenic threats, conservation translocations are increasingly used to combat species' population and range declines. However, moving animals outside of their current distribution can mean introducing them to novel conditions, even in the case of reintroductions to formerly inhabited areas due to ecosystem changes following extirpation. This exposure to novel conditions introduces uncertainty that can undermine decision making for species conservation. Here we propose two strategies, which we define as conservative and extrapolative, for approaching and managing novelty and the resulting uncertainty in conservation translocations. Conservative strategies are characterised by the avoidance and removal of novel conditions as much as possible, whereas extrapolative strategies are more experimental, allowing exposure to novel conditions and monitoring outcomes to increase understanding of a species' ecology. As each strategy carries specific risks and opportunities, they will be applicable in different scenarios. Extrapolative strategies suit species in recovery which can afford some experimental management, or species facing novel and emerging threats which require less traditional translocations, such as assisted colonisations. We provide examples, applying our framework to two endemic New Zealand species with long histories of translocation management: tuatara (Sphenodon punctatus), a reptile and takahē (Porphyrio hochstetteri), a flightless bird.
Introduction
Anthropogenic ecosystem degradation has occurred throughout human history (Waters et al., 2016), resulting in species declines and extinctions. There has been an estimated 68% decrease in population sizes of mammals, birds, amphibians, reptiles, and fish between 1970 and 2016 alone (WWF, 2020). These population declines often go hand in hand with dramatic range contractions for many species (Faurby and Araújo, 2018). Conceptually this can be viewed as shrinkage of a species' realised niche as human activity reduces the portion of their fundamental niche space that is accessible (Scheele et al., 2017). Species are often excluded from core niche spaces and restricted to a peripheral realised niche representing marginally tolerable conditions for the species (Crooks et al., 2017). Such species typically persist in a relict distribution, representing areas of low habitat quality, but often with little human activity (Kerley et al., 2012, 2020).
Species that occupy only a fraction of their former geographical distribution are often conservation priorities, as without the factors driving their range contractions being halted and/or reversed, they remain at high risk of extinction (White et al., 2014; Hunter et al., 2016). The restoration of such range-reduced species increasingly includes conservation translocations, or the human-mediated movement of living organisms from one area, with release in another where the primary objective is conservation benefit. Reintroductions, to re-establish populations in areas of the species' indigenous range from which they had previously been extirpated, and reinforcements, the release of individuals into an existing population of conspecifics to increase population viability, are important forms of conservation translocations (IUCN/SSC, 2013; Seddon et al., 2014). There is inherent uncertainty in translocating range-reduced species, as most direct information of species' behaviour and environmental preferences will come from observations in their relict distribution (Mihoub et al., 2014). While reintroductions will typically aim to release animals into areas of their indigenous range, such areas will rarely be directly analogous to the relict distribution (Osborne and Seddon, 2012; White et al., 2014; Taylor et al., 2017). As such, there will always be a degree of novelty inherent in conservation translocations.
We suggest that strategies for dealing with novelty, and resulting uncertainty, can be conceptually classified into “conservative” and “extrapolative” at the two ends of a continuous spectrum of approaches. For translocations following a conservative strategy, novel conditions would be avoided as much as possible, with release sites selected to be as similar as possible to areas within the current (relict) distribution of the translocated species. In contrast, under an extrapolative strategy, more exploratory or experimental translocations would be considered, allowing species to encounter novel conditions. Here choices would be based on predicting species' behaviour through extrapolation from observed patterns. While we primarily propose these strategies for species restricted to a relict distribution, we note that they might also have relevance for other species and translocation objectives. For example, in translocations where individuals are relocated due to displacement by infrastructure, animal welfare concerns could promote a conservative strategy, as this could minimise stress for relocated animals (Teixeira et al., 2007). Alternatively extrapolative strategies in such translocations could be used as an opportunity to pursue more active ecosytem engineering objectives (Perring et al., 2015).
Multiple options for species conservation management could also be compared using a similar classification system. For example, threat management within a species relict distribution could be considered a conservative option compared with the alternatives of either translocating individuals or taking them into captivity (Snyder et al., 1996). However, here we are specifically referring to management following the decision to translocate animals, rather than considering translocations relative to alternative actions.
We identify the challenges posed by the novelty inherent in conservation translocations in relation to release-site selection and post-release habitat use, and provide a conceptual framework to explore how this novelty can be approached following either a conservative or an extrapolative strategy. We illustrate this framework using case studies of the translocation management of two endemic New Zealand species that have suffered dramatic anthropogenic range contractions; a reptile, the tuatara (Sphenodon punctatus), and a flightless bird, the South Island takahē (Porphyrio hochstetteri).
Novelty
We define novelty at two levels: the individual level due to a founder animal's initial unfamiliarity with the specific features of the release area, and the species level when a release area differs considerably from the extant range of a species (Thatcher et al., 2006; Yott et al., 2011; Attum and Cutshall, 2015). These sources of novelty create uncertainty that can undermine the decision-making process for conservation translocations (Seddon et al., 2007). This uncertainty is manifest in two key areas:
• Reintroduction site selection, where choices based on relict distributions should identify areas where a translocated cohort is able to persist, but might potentially miss sites with conditions best able to support population growth and persistence (Kerley et al., 2012; Osborne and Seddon, 2012).
• Post-release resource use, where expectations based only on relict populations are unlikely to anticipate the full range of potential responses of the reintroduced population under novel conditions (Mihoub et al., 2014; Massaro et al., 2018).
These sources of novelty and consequent uncertainty are represented in two of the consequences for reintroductions listed in Osborne and Seddon (2012): “present day locations might not indicate currently suitable habitat,” and “present day locations where a species is absent might not indicate unsuitable habitat.” Here we provide a framework for how each of these uncertainties can be approached, through making either relatively conservative or extrapolative inferences.
Conservative Strategy
Adopting a conservative strategy, candidate release sites are given priority based on their similarity to conditions within the current distribution of the species, regardless of past population declines and range contractions. As such, selected translocation sites will have conditions similar to those in which the species is known to survive, which should reduce the number of novel or unknown factors. Reinforcements may be considered a conservative translocation strategy, as the presence or proximity of conspecifics provides clear evidence that the species can survive in a release area. A conservative approach can increase confidence that the reintroduced population will be able to survive in selected release areas. However, this conservatism will inevitably reduce the number of potential release sites to be considered, and is likely to miss suitable, or even preferred, release sites that are not represented within a species relict distribution (Kerley et al., 2012, 2020).
Similarly, following a conservative strategy, predictions of resource selection and population growth for conservation translocations would be made and evaluated based on patterns observed within the focal species' current range. This would have the advantage of limiting novelty, and thus uncertainty, as the founding cohort would be expected to require the same resources as are available in their relict range. Where not all resources are available, management could focus on providing these. This could be through the provision of supplementary feed and/or breeding shelter, such as nest boxes (Panfylova et al., 2016), removal of pests and predators (Hegg et al., 2012; Taylor et al., 2018), vegetation management (Lloyd and Powlesland, 1994) and/or controlling human activity to reduce harvest or persecution (Chapron et al., 2014). This has the advantage of reducing uncertainty in post-release outcomes as the environment is managed to provide conditions known to be at least minimally adequate for species persistence (Seddon et al., 2014). Similarly, and particularly in the case of reinforcements, decisions relating to population carrying capacities and appropriate social and demographic structures would be based on and evaluated with reference to observed patterns in relict populations.
In addition to potential additional management costs associated with release site modifications, there are potential missed opportunities associated with a conservative post-release strategy. Reducing novel conditions in a reintroduction area will remove opportunities for adaptation, whether through behavioural plasticity or genetic mechanisms, which could benefit the survival and management of the species in the long term (Zeisset and Beebee, 2013). There will likely be cases where removing or reducing resource provision such as feeding or nest sites, while reducing population growth in the immediate term, could provide the impetus for animals to explore previously unexploited resources and might eventually lead to greater population growth and stability over subsequent generations (Mertes et al., 2019). Furthermore, conditions in a species' relict range might lie on the fringes of a species' niche if the relict range represents refugia from anthropogenic threats (Kerley et al., 2012). If these are used as a benchmark for identifying population growth and persistence potential, then it will be difficult to identify sub-optimal performance in reintroduced populations (Beauchamp and Worthy, 1988). Management practises could also inadvertently reinforce or perpetuate these sub-optimal conditions, thus limiting population growth. For example, a population growth rate at least as strong as that observed within the relict range might be considered satisfactory by managers, despite this potentially being well below the maximum biological rate for the species (Morris and Doak, 2002; Kerley et al., 2012, 2020).
Extrapolative Strategy
In order to make predictions beyond currently observable conditions, extrapolative translocation management could consider additional data sources from outside the relict population. This could include indigenous distributions prior to range contractions (Lentini et al., 2017) or biophysical and behavioural information from captive animals (Mitchell et al., 2012). Alternatively, evidence could be sought from other species, e.g., from sister-species (Hunter-Ayad and Hassall, 2020), or trophically analogous species (Andelman and Fagan, 2000). However, while additional data sources can inform extrapolative translocations, they are not always available, or might not be considered reliable due to temporal, spatial, environmental, ecological, and/or taxonomic distance from the relevant management conditions (Osborne and Seddon, 2012; Svenning et al., 2016). Additional data are not always necessary to enable extrapolation, as trends within a species' relict distribution can be extended beyond observed conditions by expert inference (Beauchamp and Worthy, 1988; Kerley et al., 2012, 2020) or through statistical or biophysical models (Elith et al., 2010; Gallien et al., 2012). Regardless of the methods of extrapolation, confidence in predictions regarding translocation outcomes will be proportional to the differences between conditions at release sites relative to those in the input data, as more separation will necessitate a higher degree of extrapolation to generate predictions. As such, post-release establishment and persistence will tend to be less assured than under more conservative strategies.
Tolerating a higher degree of novelty when selecting release sites would mean that candidate sites would not be rejected simply because conditions differ from those in a species' relict distribution. This has the advantage of expanding the number and type of release sites that can be considered by conservation managers (Kerley et al., 2020). For reinforcements, while site selection is clearly based on the presence of conspecifics, extrapolative choices could be those that are considered more experimental with regards to the populations chosen for reinforcement. For example, rather than reinforcements being used as a tool to “save” struggling or declining populations, a common use (Hegg et al., 2012), they could be used to promote rapid growth in stable or increasing populations under extrapolative strategies. However, a key challenge under extrapolative strategies is determining what novelty is acceptable. Many novel conditions will render a release site clearly unsuitable, whereas other types of novelty could be suitable for a species. Ecological habitat models (e.g., correlative and mechanistic niche models) are a valuable tool in addressing this challenge as they can be used to estimate potential release site suitability even under novel conditions (Mitchell et al., 2012; Chauvenet et al., 2013; Lentini et al., 2017; Hunter-Ayad et al., 2020).
When predicting and evaluating post-release resource selection, extrapolative management would consider a broad array of resources as potentially usable in a release area. However, there might be considerable uncertainty in the degree to which any or all novel resources will be exploited, and when this might be. There might be temporal latency arising from the necessity for animals to explore novel resources and to adapt their behaviour appropriately (Osborne and Seddon, 2012). For example ‘Alalā, or Hawaiian crow (Corvus hawaiiensis) reintroduced to the island of Hawai’i steadily transitioned from reliance on familiar areas and supplementary feed, towards exploring novel areas and natural food sources over a 200 day post-release tracking period (Smetzer et al., 2021). Such adaptions to make use of novel resources could be useful in distinguishing whether certain resource uses observed in refugee populations are facultative or obligate in nature. For instance, apparent dietary specialisation in giant pandas (Ailuropoda melanoleuca) is potentially a consequence of a restricted breadth of suitable food in their relict distribution and, speculatively, they could adapt to make use of novel food sources were they translocated outside of this distribution (Kerley et al., 2020).
However, the risk with such conservation translocations is that the species is unable to adapt and exploit novel resources, and consequent high post-release mortality might increase the likelihood of the extinction of the founder cohort (White et al., 2014). Additionally, population, social, and demographic structures are likely to vary upon exposure to novel environments, or be altered via reinforcements, and should not be judged directly against patterns in extant populations. As such, reintroductions conducted following an extrapolative strategy will tend to have more open-ended predictions of post-release performance. This will also change the nature of any evaluation of the translocation; as expected goals and outcomes are less certain, post-release monitoring could be an opportunity to reveal new data regarding the species and to inform their conservation into the future, rather than checking whether focused goals are being met per se. The greater underlying uncertainty arising from the translocated species reaction to novel conditions will mean that such reintroductions carry a higher risk of failure and unintended or unforeseen outcomes.
Applying The Strategies
Both conservative and extrapolative strategies have specific strengths and limitations, and thus will be suitable in different contexts (Table 1). For instance, the inflexibility of conservative strategies, basing management on observations only from a relict population, can hamper adaptive management and reduce the ability for species management to respond to emerging threats (Corlett, 2016). However, this is a long-term concern and there are many instances where maximising confidence in rapid actions is required to save a species from immediate extinction (Lloyd and Powlesland, 1994; Massaro et al., 2018; Mukhlisi et al., 2020). In contrast, extrapolative strategies can often fail to provide certainty or concrete recommendations, making them a less palatable option to inform high stakes conservation decisions. It would be more appropriate to consider extrapolative predictions of release site suitability as hypotheses which can be tested via a translocation and subsequent monitoring (Armstrong and Seddon, 2008). As such, extrapolative translocations can be useful in an adaptive management setting as effective monitoring of post-release performance can provide evidence and information for the long-term conservation management of the reintroduced species (Seddon et al., 2014). However, the scope to experiment with extrapolative translocations is often not a luxury afforded in conservation plans for many threatened species.
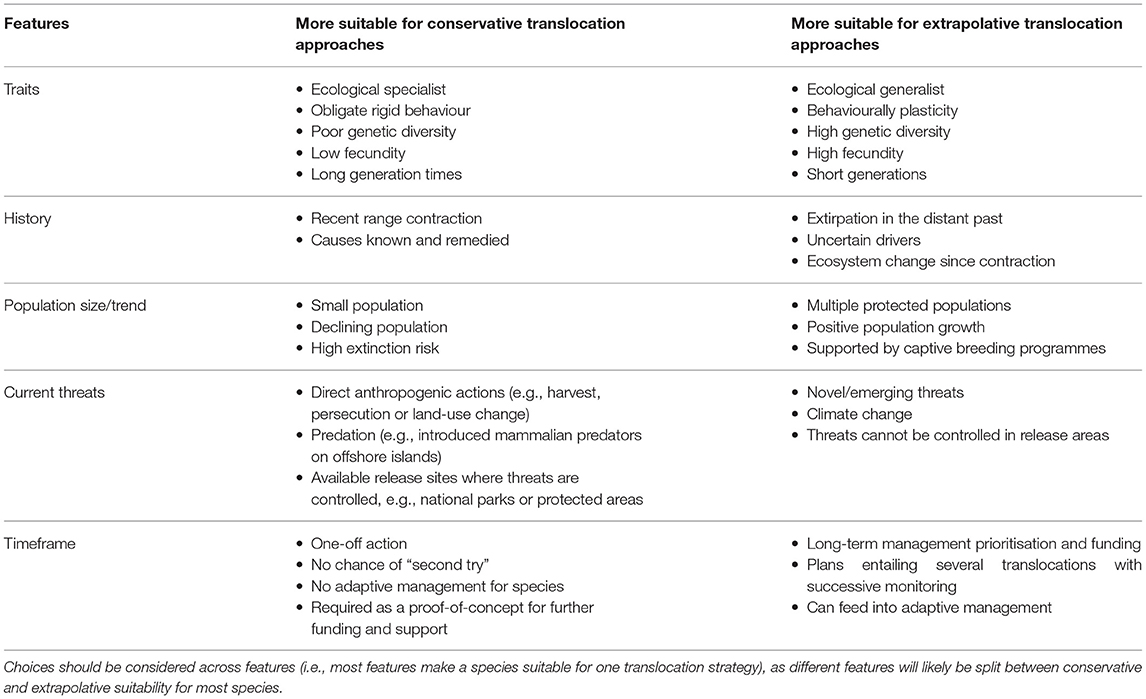
Table 1. Summary of conditions lending themselves to conservative and extrapolative translocation management.
In addition to the conservation context of a species' being translocated, consideration should be given to the life-history traits of that species, as some species will be inherently more suited to conservative or extrapolative translocations, respectively. For instance, species with generally low adaptive potential (i.e., behavioural rigidity and/or genetic restriction) are likely to have little tolerance for novel stresses (Gillies and St. Clair, 2008; Heikkinen et al., 2015). Therefore, more adaptive species are inherently suited to more conservative translocation strategies. Whereas, species with high adaptive potential (i.e., behavioural plasticity and/or genetic diversity) are much more likely to be able to adapt to novel stresses encountered following a translocation (Gillies and St. Clair, 2010), so are inherently better candidates for extrapolative translocations. We note, however, that the inherent suitability of a translocated species must still be balanced with other factors that influence the suitability of a given strategy for each specific setting. The strategic challenge for conservation managers then becomes determining how to balance several conditions, which might produce conflicting views over which strategy should be pursued.
Our framework can also be considered at multiple levels (i.e., community, clade, genus, species, population, individual, and life-stage), as strategies are relevant both to developing both broad strategies (ecosystem management) and detailed decision making (make-up of release cohorts). In this way, these rules-of-thumb can guide practitioners in considering from the widest scope, ecosystem function and services (Hale and Koprowski, 2018), to the finest detail, individual personality and traits (Koolhaas et al., 2007; Boyer et al., 2010).
For species facing a high risk of extinction in the short-term due to a combination of species' traits and sustained or increasing threats within their relict range, a conservative approach is likely to be the most suitable. This would focus on securing stable populations to save the species in the short-term, which must be a management priority, albeit while perhaps limiting long-term population growth potential. However, species not under immediate risk of extinction, with stable or growing populations might be better served by an extrapolative strategy where novelty need not be avoided. Although this might seem counter intuitive, this situation does occur. For instance, species might maintain stable or growing populations over a large, but still reduced, range. An example are Eurasian cranes (Grus grus), which were reintroduced to South-West England in 2010 in order to restore and enhance ecosystems in this region. Cranes were extirpated from the British Isles in the sixteenth century, though large populations have persisted on the Eurasian mainland. As such, the species has never been threatened and is listed as least-concern on the International Union for Conservation of Nature (IUCN) Red List for threatened species (Birdlife International., 2016; Soriano-Redondo et al., 2019). Alternatively, species could have stable or growing populations due to active management in captivity and/or in the wild (such as the tuatara and takahē case studies given below). In this case, growing populations provide opportunities for extrapolative translocations to be considered, even though species survival remains dependent on ongoing conservation efforts.
In cases where extinction is not an imminent concern there is scope to view conservation translocations as ecological experiments, enabling researchers and conservationists to learn more about the niche-breadth of the species, as well as aspects of species ecology such as dietary and behavioural plasticity (Sarrazin and Barbault, 1996). In such cases even a failed translocation attempt can provide valuable information for the conservation of the species (Taylor et al., 2017). For example, a “failed” translocation could perhaps confirm that at least one condition in the release area was outside of the species niche, or that the release procedure was unsuitable, without jeopardising species' survival. We expect the conservative and extrapolative strategies laid out above to be useful in clarifying decision-making processes for conservation managers and scientists. For instance, the choice of a conservative vs. extrapolative strategy can inform several key decisions in the building of ecological models (i.e., how models are set up, including what data used as model input and the kinds of relationships fitted to those data), a key tool in supporting conservation management (Hunter-Ayad et al., 2020).
To examine our conservative-extrapolative translocation framework, we use two case studies for species where conservation translocations have formed an integral part of their rehabilitation: the tuatara and the takahē. Both of these species are endemic to New Zealand (also known as Aotearoa New Zealand, hereafter NZ), which was discovered by humans only in ~AD 1280 (Wilmshurst et al., 2008). Tuatara and takahē were extirpated from the majority of their indigenous range following the arrival of humans to NZ (Figures 1, 3), with this largely attributed to predation by introduced mammals and habitat loss (Beauchamp and Worthy, 1988; Cree, 2014). Both species are of cultural significance in NZ to the indigenous Māori people as taonga (treasures), as well as to European communities (Lee and Jamieson, 2001; Cree, 2014). For several decades, conservation management of tuatara and takahē has included conservation translocations, making them suitable candidate species to examine our conservative-extrapolative framework. Tuatara management has been extensively documented in the published literature. Whereas, for takahē recent developments are largely unpublished outside of the grey literature. Ongoing takahē research will draw from and emulate the work to date with tuatara, developing quantitative models to identify potential impacts of climate change.
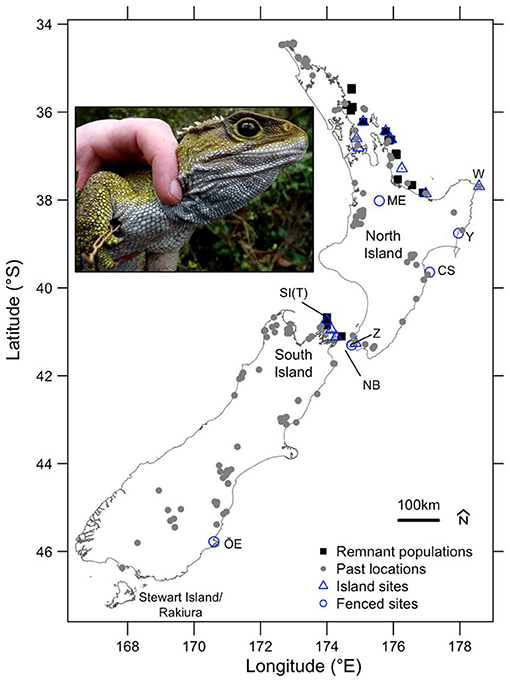
Figure 1. Map: Tuatara remnant and past locations as well as translocation release sites. Past locations (grey circles) from Holocene-aged fossil deposits (last 11,650 cal years BP, as determined by biochronological reasoning, namely the dominance of the moa Anomalopteryx didiformis; Worthy and Holdaway, 2002) and other known or probable extinctions from offshore islands (Cree, 2014). The remnant populations (black squares) and translocation sites (blue outlines) are split into groups of island and fenced sites, as these entail more conservative and extrapolative translocations, respectively. Locations of key places mentioned in the text are listed from north to south: W, East Island/Whangaokeno; ME, Maungatautiri Ecological Island; Y, Young Nick's Head; CS, Te Matau a Maui—Cape Sanctuary; SI(T), Stephen Island (Takapourewa); Z, ZEALANDIA; NB, North Brother Island; OE, Orokonui Ecosanctuary; Inset, An adult male tuatara about to be reintroduced to OE; photo by SJ. Adapted from Cree (2014) and Jarvie et al. (2021).
Tuatara Case Study
Tuatara (Sphenodon punctatus) are a lizard-like reptile endemic to NZ and of evolutionary significance as the sole living representative of Rhynchocephalia, the sister group to Squamata (Cree, 2014). Tuatara are diurno-nocturnal and adapted to cooler climates, being active at body temperatures from ~5 to 30°C (Barwick, 1982; Thompson and Daugherty, 1998). Compared to most other reptiles tuatara have a long maturation time (~13–20 years), lengthy inter-clutch intervals (~2–9 years), a small clutch size (~8 eggs; range 1–17), and a long life span of at least 100 years (Cree, 2014).
Once widespread across NZ's North and South Islands, as well as many offshore islands (Figure 1), tuatara became restricted to a relict distribution, being only found naturally on 32 offshore islands. Although taxonomy remained uncertain for living tuatara until recently (Hay et al., 2010), all living populations of tuatara are currently considered to be S. punctatus (Hay et al., 2010; Gemmell et al., 2020).
Conservation translocations to islands from which introduced mammals have been eradicated (Gaze, 2001), and to mainland predator-fenced sanctuaries have been used to create insurance populations and to restore ecosystem function (Miller et al., 2012; Jarvie et al., 2021). Beginning in 1995, tuatara have now been translocated to 14 islands and 5 mainland fenced sanctuaries, increasing the number of populations to 47 (Figure 1; see Jarvie et al., 2021). Most conservation translocations of tuatara arose out of well-documented recovery planning during the 1990s and early 2000s (Cree and Butler, 1993, Gaze, 2001), although these recovery plans are now out of date. The long-term goal for tuatara from the most recent Recovery Plan is to preserve genetic diversity of all existing populations and to restore new populations throughout their pre-human range, including locations with increased accessibility to the general public (Gaze, 2001).
Following a conservative strategy, earlier conservation translocations of tuatara focused mainly on restoring populations within the same ecological region as the source population (Cree, 2014; Jarvie et al., 2014). These population restorations included both reintroductions and reinforcements. Initial reintroductions included establishing new populations with at-risk genetic stocks (Cree and Butler, 1993), such as the release of tuatara previously identified as a separate species (S. guntheri from North Brothers Island, which is now considered to also be S. punctatus; Hay et al., 2010).
With encouraging provisional results, a more extrapolative strategy was adopted for the reintroduction of tuatara to the predator-fenced mainland sanctuary of ZEALANDIA (formerly known as Karori Wildlife Sanctuary) in 2005 (Figure 1; McKenzie, 2007). The tuatara were released in the sanctuary, which was mostly free of introduced mammals, except for the house mouse (Mus musculus). Because of the possible impacts of mice, most of the translocated tuatara (60 of the 70) were released within a small (1 ha) mouse-proof enclosure within the larger sanctuary. The remaining 10 tuatara were released into the outer sanctuary (225 ha) and were monitored using radio-transmitters to assess the possible impacts of mice before further releases (McKenzie, 2007). Following high rates of survival of the released tuatara in both the mouse-proof enclosure and outer sanctuary, the population was reinforced in 2007 (Cree, 2014; Jarvie et al., 2021). In 2012, tuatara were translocated following an extrapolative strategy to considerably outside of the ecological region of the source population, to four predator-fenced mainland sanctuaries and an island (Figure 1; Cree, 2014; Jarvie et al., 2021). While mice are periodically detected at some mainland sites, their effects on tuatara remain uncertain. Several mainland locations have also had occasional incursions of larger introduced mammals such as Norway rats (Rattus norvegicus), ship rats (R. rattus), and stoats (Mustela erminea), but control of these predators has been needed to minimise the threat to tuatara. Preliminary survivorship of tuatara at monitored mainland locations is encouraging (McKenzie, 2007; Cree, 2014; Jarvie et al., 2015, 2016, 2021; Price et al., 2020), although continued vigilance and monitoring for introduced mammals remains necessary.
Prior to previous conservation translocations for tuatara, climatic suitability of release sites was considered on the basis of laboratory studies, field studies, and expert inference, and have been informed by knowledge of the Holocene fossil record (Cree, 2014; Jarvie et al., 2014, 2021). A recent study built correlative species distribution models (SDMs) using occurrence records from remnant populations and past locations from the Holocene (Figure 1) as well as paleoclimates, to estimate climatically suitable areas (Figure 2; Jarvie et al., 2021). By incorporating locations of Holocene deposits and/or knowledge of past locations in SDMs, larger areas of suitable climate were identified compared to SDMs derived from remnant populations only. These results highlight the need to consider data from outside relict distributions when assessing climate suitability for future conservation translocations for tuatara, and other relict species.
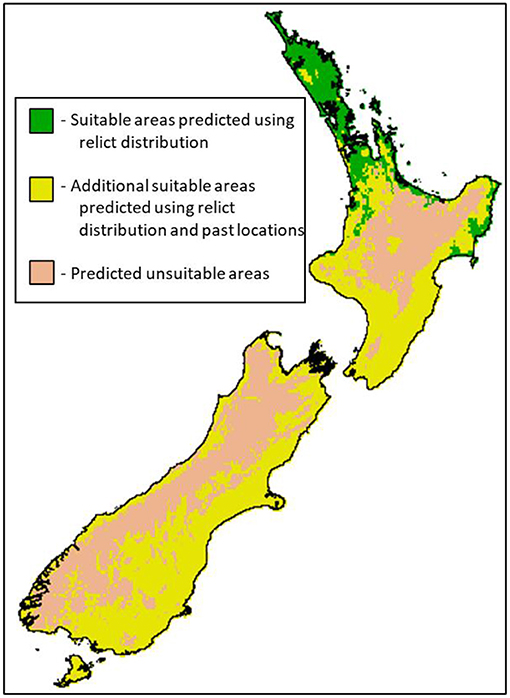
Figure 2. Map of current climatic suitability from a maximum entropy model (Phillips et al., 2006) trained with remnant populations and past locations for tuatara (Sphenodon punctatus) across New Zealand. Green areas were identified as suitable by models using only data from remnant populations of tuatara (Figure 1), whereas the yellow areas show the additional space predicted to be suitable when remnant populations and past locations (Figure 1; radio-carbon dated deposits, Holocene-age deposits, and known or probable extinctions) were also used as model inputs. For full methodological details see Jarvie et al. (2021), including with figures showing projections under climate change and with model uncertainty.
To support future conservation management of tuatara, research should be undertaken to inform translocations. Comparisons of remnant populations with translocated populations are useful to understand changes in demography (e.g., sex ratios, mating systems, and populations trends), phenotypic plasticity and phenology (e.g., Mitchell et al., 2008; Miller et al., 2012; Rout et al., 2013). Further development of mechanistic models for tuatara should be undertaken to explore responses of populations to climate change in existing and novel environments (e.g., Carter et al., 2018). The resulting mechanistic models could also be compared to results from correlative SDMs, potentially providing key insights into processes shaping the species' range limits (Tingley et al., 2014; Briscoe et al., 2016). Because the evolutionary consequences of translocations in long-lived species might not be apparent for centuries, confirming the accuracy of predictions based on correlative SDMs and mechanistic models could take decades. The continued measurement of responses of extant populations to climate change will be key for model validation.
Population viability analyses and gene retention modelling could be used to inform management of source and translocated populations, including investigating demographic responses to ambient temperature and population density. In some instances, the choice of founders from more than one source population might also be considered to maximise genetic diversity (Weeks et al., 2011; Miller et al., 2012). For example, where release sites are between remnant populations, founder populations could comprise individuals from adjacent genetic groups (Hay et al., 2010) to increase the adaptive population of any new population.
However, more extrapolative translocations might have benefits and unintended drawbacks (Miller et al., 2012). On one hand, there might be an increase in fitness due to greater genetic variation and hybrid vigour, and there could be microevolutionary responses because of adaptation to new local conditions (Jamieson et al., 2006). On the other, the resulting hybridisation could cause outbreeding depression or maladapted phenotypes, and expose founders from different populations to novel diseases (Miller et al., 2012). A mixed-stock approach to future translocations of tuatara would require careful monitoring, for example, of short-term survival and growth of founders, recruitment, and performance of hybrids relative to founder stock (Weeks et al., 2011). Intensive consultation with stakeholders and iwi (Māori tribes) partners prior to translocations would also be necessary (Miller et al., 2012).
For selection of future release sites, an increasingly extrapolative approach could be considered if remnant populations remain secure and translocated populations become established within the indigenous range. While reintroductions and reinforcements should still be used for future translocations to establish populations within the indigenous range, consideration could also be given to introductions of tuatara to islands or mainland sites where habitat is predicted to be suitable under climate change. The combined use of correlative SDMs and mechanistic models could inform such translocations. Proposed release sites could include assisted colonisation (Brodie et al., 2021) of tuatara to locations such as Stewart Island/Rakiura, which is outside the indigenous range of the species.
South Island Takahē Case Study
The South Island takahē (Pophyrio hochstetteri) is the world's largest extant rail species, characterised by their size and flightlessness. Takahē, along with the kākāpo (Strigops habroptila), represent the last vestige of NZ's unique pre-human guild of flightless herbivorous birds. They were presumed extinct in the early twentieth century and a high-profile rediscovery in 1948 instigated over half a century of conservation effort to preserve and restore the species (Lee and Jamieson, 2001).
Prior to the arrival of humans, the takahē's indigenous range was widespread across the South Island, with a distinct species, the North Island takahē (Porphyrio mantelli), on the North Island. However, following the arrival of humans NZ and the associated introduction of mammalian predators the North Island species was driven to extinction, while the South Island takahē was largely restricted to a single mountain range (the Murchison Mountains, Fiordland) by the early 1940s (Hegg et al., 2012, 2013; Figure 3).
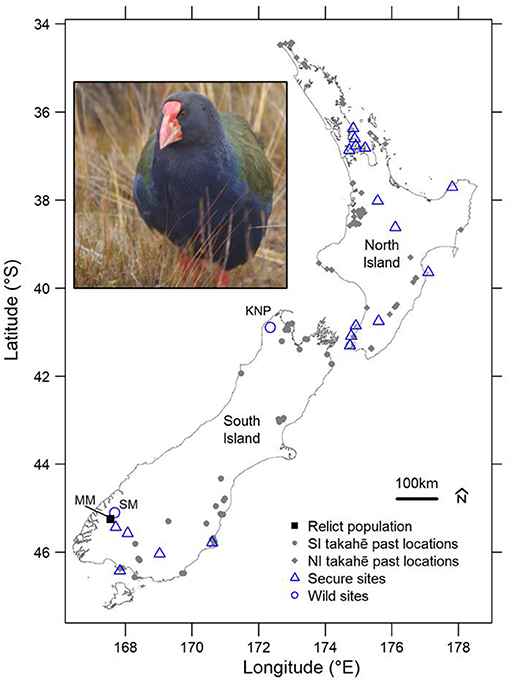
Figure 3. Map: Takahē remnant and past locations as well as translocation release sites. Past locations (grey circles) from Holocene-aged fossil deposits (last 11,650 cal years BP, as determined by biochronological reasoning, namely the dominance of the moa Anomalopteryx didiformis; Worthy and Holdaway, 2002) are used to indicate of the pre/early human distribution of both North Island (NI) takahē (Porphyrio mantelli) and South Island (SI) takahē (Porphyrio hochstetteri). The relict population (Murchison Mountains, MM), wild sites (blue-outlined circles, SM, Stuart Mountains; KNP, Kahurangi National Park), and secure sites (blue-outlined triangles) are also shown. Inset: A South Island takahē following reintroduction to KNP; photo by JH-A.
The location of the relict population of takahē has been designated a special protected area since the 1950s. Despite this, the species remained vulnerable to extinction as it persisted only as a single population (Hegg et al., 2012). Consequently, conservation translocations began in the 1970s to create secondary, secure populations in predator-free reserves and small islands (hereafter “secure sites”) in case of extirpation of the single relict population (Hegg et al., 2013). The primary recovery goal for the takahē is to establish sustainable wild populations of takahē within their natural range. The secure site populations play a critical role for genetic insurance and population growth purposes, and the use and extent of secures sites continues to be reviewed as wild population security grows (DOC Unpublished Strategies).
Between 1987 and 1992 a reintroduction of takahē was attempted to an open mainland site (hereafter “wild site”). This was to establish a population in the Stuart Mountains, neighbouring the relict population in the Murchison Mountains (Hegg et al., 2013). However, this reintroduction was ultimately unsuccessful, despite conservative site selection, as high mortality and dispersal prevented establishment of a population. This failure was attributed to the small size of annual release cohorts, unusually harsh winters in the early 1990s and high densities of mammalian predators (Lee and Jamieson, 2001; DOC Unpublished Records).
Following the failure of the Stuart Mountains reintroduction, focus shifted to conservation efforts within the Murchison Mountains relict population, as well as a continued establishment and management of small populations at secure sites. The most important criteria for selecting a secure site were the absence of introduced mammalian predators and operational access (as the remoteness of the Stuart Mountains hampered monitoring and management actions there). All secure sites represent novel conditions outside of the relict distribution in the Murchison Mountains, as they are different in terms of climate, ecological community and human activity. As a result, this site selection can be viewed as extrapolative. However, rather than being a preference of management this was due to the necessary focus on the major threat to takahē persistence, invasive predators, and the lack of conservative sites in which these predators could be effectively controlled. Takahē have now been established in 18 secure sites, removing immediate risks of extinction and beginning to reverse the decline of the species, such that today there are ~500 living takahē, up from a low of 124 birds in 1981 (Hegg et al., 2012; DOC Unpublished Data). This resulted in a downgrading of the NZ species threat level, Nationally Critical to Nationally Vulnerable in 2016 (Robertson et al., 2016).
While selection of secure sites has been necessarily extrapolative, management of post-release resource selection has followed a more conservative approach. Habitat management for takahē has been employed when necessary at secure sites, particularly to prevent vegetation succession. Prior eradication and on-going exclusion of invasive mammalian predators has been essential, while supplementary food is also provided at some sites. Genetic resources and population densities are also strictly managed, with breeding pairs established to maintain current levels and patterns of genetic diversity (Greaves et al., 2020). This population admixture serves the purpose of avoiding inbreeding and genetic bottlenecks, but might also limit the ability of takahē to adapt to conditions in any one secure site as continual translocations between secure, relict and wild sites are used to maintain genetic diversity across the global takahē population (Lees et al., 2014; Zavodna et al., 2015; Greaves et al., 2020). However, despite this control, some local behavioural adaptations have been observed as takahē at secure sites expand their use of resources that have limited availability in the Murchison Mountains such as fallen fruits, exotic grasses, small reptiles, and the chicks of other bird species (particularly ducks). This has demonstrated that takahē are a more adaptable and generalist species than is evident from their behaviour and ecology within the relict population (Beauchamp and Worthy, 1988; Mills et al., 1991).
From the mid-late 2010s mainland reintroductions of takahē were again planned to establish new wild sites supporting larger populations and with less intensive management than at secure sites. This followed population growth across multiple secure sites and increased ecological understanding and refinement of management methods, enabling policy to become more ambitious in scope. The first reintroduction has been to Kahurangi National Park in 2018, in the north-west of the South Island. This site was chosen relatively conservatively as it bears similarities to takahē-inhabited areas in the Murchison Mountains. However, post-release management (particularly a lack of fencing/containment) allowed takahē to establish and roam as they pleased (except into farmland), making this aspect of the reintroduction more extrapolative than at secure sites. Ongoing research is building SDMs using occurrence records from the Murchison Mountain takahē population (Figure 4), to estimate environmentally suitable areas in Kahurangi for the species (Figure 4; Supplementary Data Sheets).
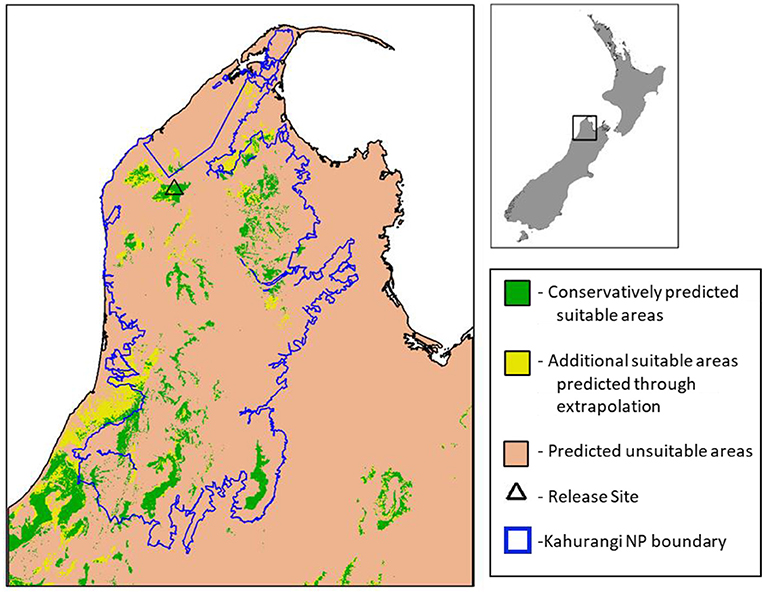
Figure 4. Map of current environmental suitability from a maximum entropy model (Phillips et al., 2006) trained with records from South Island takahē (Porphyrio hochstetteri) relict population (Figure 3). Green areas were identified as suitable by a conservatively fitted model, whereas the yellow areas show the additional space predicted to be suitable when the model fitting was relaxed to allow a greater degree of extrapolation. The conservative model only identifies habitat in environments analogous to those occupied in the models' input data, whereas the extrapolative model focuses on excluding areas only because they are analogous to unused areas in the species training data. For full details of the methods used to generate these predictions see Supplementary Data Sheet 1.
Although mammalian predators are controlled to low densities within Kahurangi, eradication was not considered essential for the release of birds. Thus far, no supplementary feed has been provided as the extensive tussock grasslands at the release site are intended to provide sufficient food for released takahē, though post-release monitoring will be crucial to confirming if this is the case. Additionally, having a relatively large release cohort of 30 individuals allowed for greater complexity in social structures and interactions to arise post-release than in previous translocations.
Early post-release monitoring of this population seems to indicate similar patterns of resource-use, population growth, and social structure to that of the relict population (DOC Unpublished Data). However, this was initially disrupted, possibly in part due to a female gender bias in the release cohort. Single females were observed to disturb established breeding groups, causing them to disband prior to and during the first post-release breeding season, potentially contributing to low population growth. Dispersal away from the release site was also observed predominantly in unpaired birds, rather than in stable pairs (DOC Unpublished Data). Further investigation of post-release resource use in Kahurangi is planned, using methods such as faecal analyses, stable isotope analysis, and eDNA to provide more information on dietary composition and changes over time (DOC Unpublished Proposals).
The conservation management plan for takahē considers that future reintroductions will be essential to maintain and restore the species, with an increasingly extrapolative attitude being considered as the species becomes more secure from historic threats while encountering new threats from climate change. The Takahē Recovery Programme is continuing to use existing sites to improve in-situ population performance and to predict likelihood of success at new sites. These new locations will be more extrapolative as takahē appear to prefer low-mid elevation and benign topography and climate. The primary focus is on likely predation pressure from introduced mammals and the feasibility of mitigation, ease of access and minimising excess dispersal through natural barriers.
Discussion
In this paper we have sought to develop a strategic framework to inform translocation strategies in terms of environmental novelty, highlighting the risks and rewards of opposing attitudes. We separate strategies into two broad categories based on defining novel conditions as those not represented within a species relict distribution. We define these as conservative (novelty avoidance) and extrapolative (novelty exposure) strategies, suggesting that each strategy has specific advantages and drawbacks, so will be suited to different species and conservation contexts. Our case studies demonstrate how previous translocation decisions can be viewed through the lens of our conservative-extrapolative framework. Management of both tuatara and takahē has shown a general trend of moving from initial conservative, cautious translocations, and towards generally more extrapolative, experimental manipulations. For tuatara this progress has followed this trend relatively clearly, with more recent translocations establishing populations further outside of their relict distribution than ever before. Whereas, for takahē, specific setbacks and practical restrictions meant that earlier site-selection decisions were extrapolative by necessity while population management was conservative, and more recent translocations have become more conservative in terms of site-selection while post-release management has become more extrapolative. We hope that these case-studies will demonstrate how our proposed framework can provide a lens through which both historic and future translocations decisions can be viewed.
As each of our developed strategies create specific opportunities and risks, they are intrinsically better suited to certain species, situations, and management objectives. It will remain a challenge to assess and balance numerous factors that will suggest a more conservative or a more extrapolative approach is suitable (Table 1). These factors are likely to counteract one another in many instances. For example, a species that has been through a population bottleneck, such as the alpine ibex (Capra ibex ibex), might have low genetic diversity and adaptive potential, suggesting suitability for conservative translocations, despite having a large and growing population, suggesting they could be candidates for extrapolative reintroductions (Stüwe and Nievergelt, 1991). Balancing such conflicting conditions will remain a significant challenge for translocation management, and we emphasise two key aspects to bear in mind: firstly, these strategies can be considered as “rules-of-thumb” or guiding principles, and secondly that, although we have defined the strategies categorically for convenience, they in fact characterise the ends of a continuum of attitudes to novelty exposure. Based on these considerations we propose that our strategies are best applied at the discretion of management when opportunities and conditions allow. Furthermore application of the strategies in the face of conflicting factors can be flexible. Choices here would be based either on a judgement call from conservation practitioners based on their priorities or be a compromise where applied strategies are somewhat intermediate, conservative in some respects but more extrapolative in others. This ability to be flexibly applied should make our framework potentially useful in many settings and given many constraints.
Historically, reintroduction has mostly been the limit of ambition for ecosystem management and restoration by managers and scientists, although there have been key exceptions (Lloyd and Powlesland, 1994; Greuber et al., 2012; Carter et al., 2017). We expect that strategies for dealing with novelty in translocations will become much more extrapolative in the future, as the scope and ambition of emerging management goals will allow less room for the strict control of resources and conditions that conservative strategies require. Thus, our framework provides a structure for the development of adaptive management strategies for conservation translocations (Osborne and Seddon, 2012; Smith et al., 2012). We expect this to facilitate the development of strategies where the exposure to risk from novel conditions is explicitly considered in terms of species traits, and population conservation status (Kennedy et al., 2013). However, a cautious, conservative approach will likely be most appropriate for the early stages in the restoration of highly threatened species, as securing a stable population and removing the imminent threat of extinction must be a priority in such cases. Conservative translocations will also be viewed more favourably by the public, indigenous peoples, and other stakeholders, whereas extrapolative translocations are likely be more controversial (e.g., Ricciardi and Simberloff, 2009).
Extrapolative strategies have particular importance in the context of ongoing anthropogenic climate and land-use change. To combat emerging threats, increasingly more extensive ecosystem manipulations are suggested, including assisted colonisation (Brodie et al., 2021), the translocation of individuals outside of their established range as a response to threats such as climate change (IUCN/SSC, 2013). These sorts of translocations, moving organisms outside of both their current and indigenous ranges are inherently extrapolative, as managers are knowingly creating unprecedented conditions and ecological interactions (Rendall et al., 2018; Bouma et al., 2020).
We urge caution in the application of extrapolative translocation strategies, particularly with regard to assisted colonisation and rewilding projects that seek to create novel ecosystem conditions (Corlett, 2016). In these cases, where there is so much inherent uncertainty, we suggest that decisions should always be informed by what data are available, and that even the most experimental translocations should be based on evidence-based hypotheses regarding the potential success and risks of the translocation (Armstrong and Seddon, 2008; Bouma et al., 2020). This is due to the cautionary tales from the field of invasive species management, in which many species that have been introduced outside of their native range, whether accidentally or intentionally, are a major driver of ecosystem degradation and biodiversity decline, costing conservation management vast resources to reduce and reverse the resulting damage (Brook et al., 2008; O'Donnell et al., 2017). We strongly suggest that a minimum requirement for conservation introductions be that there is evidence that the focal species will not only survive, but will also have (at least) no negative impact on the overall biodiversity and ecosystem functioning of the release area.
We further suggest that the principles developed in our framework can be applied to other areas of conservation practise, beyond conservation translocations. For example, distinguishing a similar continuum of conservative to extrapolative measures could usefully refine and clarify decision making for predator/pest control (Recio et al., 2015; Roy et al., 2016; O'Donnell et al., 2017), resource extraction (Peres et al., 2016) and tourism volume (Daily et al., 2009). Relating these choices to specific ecological evidence and considerations. These strategies can be framed generally as an application of cost-benefit analysis to conservation management (Chee, 2004; Fletcher et al., 2011) and could usefully enhance the clarity and transparency of risk management in decision making processes (Sutherland et al., 2004).
Inherent risks and uncertainties emphasise the need for caution in planning conservation translocations. We add to previous calls to view translocations as ecological experiments, following a mindset whereby explicit a priori predictions are made and then tested, even in cases where scientific enquiry is not the primary objective of management (Seddon et al., 2007; Taylor et al., 2017). We believe the conservative-extrapolative framework presented here will help with the application of this concept. Following this framework can help to ensure that predictions are appropriately framed in terms of the confidence that can be placed on them and that “tests” from post-release monitoring are also viewed and incorporated into adaptive management plans in a suitable way. We expect a conservative-extrapolative framework will have increasing relevance as conservation practise is developing away from traditional, preservationist approaches, so called “fortress” conservation, towards goals with increasing ambition and scope to include ecosystem services, ecological restoration, and rewilding as key objectives (Seddon et al., 2014; Corlett, 2016).
Data Availability Statement
The original contributions presented in the study are included in the article/Supplementary Material, further inquiries can be directed to the corresponding author/s.
Author Contributions
JH-A, PS, MR, and RO conceived the idea and scope of the work. JH-A reviewed the literature and led the writing of the manuscript. SJ led the writing of the tuatara case-study, while AD and GG provided crucial comments towards the takahē case study, as well as the manuscript as a whole. All authors contributed critically to the draughts and gave final approval for publication.
Funding
Funding support was provided to JH-A by a University of Otago Doctoral Scholarship. Monitoring of takahē in the Murchison Mountains provided species data for corelative models presented in the takahē case study. This population monitoring was supported by Fulton Hogan, commercial partner of the Takahē Recovery Programme, who provided funding for operational costs.
Conflict of Interest
The authors declare that the research was conducted in the absence of any commercial or financial relationships that could be construed as a potential conflict of interest.
Acknowledgments
For prior consultation that helped to clarify our ideas, we thank New Zealand's Department of Conservation Te Papa Atawhai, Ngāti Koata [kaitiaki or guardians of tuatara from Stephens Island (Takapourewa)] and Kāti Huirapa Runaka ki Puketeraki (mana whenua or indigenous people of Orokonui Ecosanctuary). We would also like to thank Alison Cree and Lynn Adams for discussions on conservation translocations of tuatara, and Trevor Worthy and Paul Scofield for providing Holocene occurrence records for tuatara and takahē. Finally we would like to thank our reviewers, CC and TW for their help in improving a previous draft of the paper.
Supplementary Material
The Supplementary Material for this article can be found online at: https://www.frontiersin.org/articles/10.3389/fcosc.2021.691714/full#supplementary-material
Supplementary Data Sheet 1. Takahe models.
Supplementary Data Sheet 2. Takahe environmental data.
Supplementary Data Sheets 3–10. ENMeval replicate data.
References
Andelman, S. J., and Fagan, W. F. (2000). Umbrellas and flagships: efficient conservation surrogates or expensive mistakes? Proc. Natl. Acad. Sci. U.S.A. 97, 5954–5959. doi: 10.1073/pnas.100126797
Armstrong, D. P., and Seddon, P. J. (2008). Directions in reintroduction biology. Trends Ecol. Evol. 23, 20–25. doi: 10.1016/j.tree.2007.10.003
Attum, O., and Cutshall, C. D. (2015). Movement of translocated turtles according to translocation method and habitat structure. Restor. Ecol. 23, 588–594. doi: 10.1111/rec.12233
Barwick, R. E. (1982). “Observation on active thermoregulation in the tuatara, Sphenodon punctatus (Reptilia: Rhynchocephalia),” in New Zealand Herpetology, ed D. G. Newman (Wellington: New Zealand Wildlife Service Occasional Publication No. 2), 225–236.
Beauchamp, A. J., and Worthy, T. H. (1988). Decline in distribution of the takahe Porphyrio (= notornis) mantelli: a re-examination. J. R. Soc. New Zeal. 18, 103–118. doi: 10.1080/03036758.1988.10421698
Birdlife International. (2016). Grus grus. IUCN Red List Threat. Species 2016 e.T2269214. Available online at: https://dx.doi.org/10.2305/IUCN.UK.2016-6623.RLTS.T22692146A86219168.en (accessed February 18, 2021).
Bouma, A., Kuchling, G., Zhai, S. Y., and Mitchell, N. (2020). Assisted colonisation trials for the western swamp turtle show that juveniles can grow in cooler and wetter climates. Endanger. Species Res. 43, 75–88. doi: 10.3354/esr01053
Boyer, N., Réale, D., Marmet, J., Pisanu, B., and Chapuis, J. L. (2010). Personality, space use and tick load in an introduced population of Siberian chipmunks Tamias sibiricus. J. Anim. Ecol. 79, 538–547. doi: 10.1111/j.1365-2656.2010.01659.x
Briscoe, N. J., Kearney, M. R., Taylor, C. A., and Wintle, B. A. (2016). Unpacking the mechanisms captured by a correlative species distribution model to improve predictions of climate refugia. Glob. Chang. Biol. 22, 2425–2439. doi: 10.1111/gcb.13280
Brodie, B. J. F., Lieberman, S., Moehrenschlager, A., Redford, K. H., Rodríguez, J. P., and Schwartz, M. (2021). Global policy for assisted colonization of species. Science 372, 456–458. doi: 10.1126/science.abg0532
Brook, B. W., Sodhi, N. S., and Bradshaw, C. J. A. (2008). Synergies among extinction drivers under global change. Trends Ecol. Evol. 23, 453–460. doi: 10.1016/j.tree.2008.03.011
Carter, A. L., Kearney, M. R., Hartley, S., Porter, W. P., and Nelson, N. J. (2018). Geostatistical interpolation can reliably extend coverage of a very high-resolution model of temperature-dependent sex determination. J. Biogeogr. 45, 652–663. doi: 10.1111/jbi.13152
Carter, I., Foster, J., and Lock, L. (2017). The role of animal translocations in conserving british wildlife: an overview of recent work and prospects for the future. Ecohealth 14, 7–15. doi: 10.1007/s10393-015-1097-1
Chapron, A. G., Kaczensky, P., Linnell, J. D. C., Von, M., Fuxjäger, C., and Groff, C. (2014). Recovery of large carnivores in Europe' s modern human- dominated landscapes. Science 346, 1517–1519. doi: 10.1126/science.1257553
Chauvenet, A. L. M., Ewen, J. G., Armstrong, D., and Pettorelli, N. (2013). Saving the hihi under climate change: a case for assisted colonization. J. Appl. Ecol. 50, 1330–1340. doi: 10.1111/1365-2664.12150
Chee, Y. E. (2004). An ecological perspective on the valuation of ecosystem services. Biol. Conserv. 120, 549–565. doi: 10.1016/j.biocon.2004.03.028
Corlett, R. T. (2016). Restoration, reintroduction, and rewilding in a changing world. Trends Ecol. Evol. 31, 453–462. doi: 10.1016/j.tree.2016.02.017
Cree, A. (2014). Tuatara: Biology and Conservation of a Venerable Survivor. Christchurch: University Press.
Cree, A., and Butler, D. (1993). Tuatara Recovery Plan (Sphenodon spp.). Threatened Species Recovery Plan 9. Wellington: Threatened Species Unit; Department of Conservation. Available online at: https://www.doc.govt.nz/globalassets/documents/science-and-technical/tsrp09.pdf
Crooks, K. R., Burdett, C. L., Theobald, D. M., King, S. R. B., Di Marco, M., Rondinini, C., et al. (2017). Quantification of habitat fragmentation reveals extinction risk in terrestrial mammals. Proc. Natl. Acad. Sci. U.S.A. 114, 7635–7640. doi: 10.1073/pnas.1705769114
Daily, G. C., Polasky, S., Goldstein, J., Kareiva, P. M., Mooney, H. A., Pejchar, L., et al. (2009). Ecosystem services in decision making: time to deliver. Front. Ecol. Environ. 7, 21–28. doi: 10.1890/080025
Elith, J., Kearney, M., and Phillips, S. (2010). The art of modelling range-shifting species. Methods Ecol. Evol. 1, 330–342. doi: 10.1111/j.2041-210X.2010.00036.x
Faurby, S., and Araújo, M. B. (2018). Anthropogenic range contractions bias species climate change forecasts. Nat. Clim. Chang. 8, 252–256. doi: 10.1038/s41558-018-0089-x
Fletcher, R. J., Robertson, B. A., Evans, J., Doran, P. J., Alavalapati, J. R. R., and Schemske, D. W. (2011). Biodiversity conservation in the era of biofuels: risks and opportunities. Front. Ecol. Environ. 9, 161–168. doi: 10.1890/090091
Gallien, L., Douzet, R., Pratte, S., Zimmermann, N. E., and Thuiller, W. (2012). Invasive species distribution models - how violating the equilibrium assumption can create new insights. Glob. Ecol. Biogeogr. 21, 1126–1136. doi: 10.1111/j.1466-8238.2012.00768.x
Gemmell, N. J., Rutherford, K., Prost, S., Tollis, M., Winter, D., Macey, J. R., et al. (2020). The tuatara genome reveals ancient features of amniote evolution. Nature 584, 403–409. doi: 10.1038/s41586-020-2561-9
Gillies, C. S., and St. Clair, C. C. (2008). Riparian corridors enhance movement of a forest specialist bird in fragmented tropical forest. Proc. Natl. Acad. Sci. U.S.A. 105, 19774–19779. doi: 10.1073/pnas.0803530105
Gillies, C. S., and St. Clair, C. C. (2010). Functional responses in habitat selection by tropical birds moving through fragmented forest. J. Appl. Ecol. 47, 182–190. doi: 10.1111/j.1365-2664.2009.01756.x
Greaves, G., Rutschmann, A., Joustra, T., Marsh, P., and Lees, C. M. (2020). South Island Takahē Meta-population: 2020 Modelling Report. Apple Valley, MN: IUCN SSC Conservation Planning Specialist Group.
Greuber, C. E., Maxwell, J. M., and Jamieson, I. G. (2012). Are introduced takahe populations on offshore islands at carrying capacity? Implications for genetic management. N. Z. J. Ecol. 36, 223–227. Available online at: https://www.jstor.org/stable/24060849
Hale, S. L., and Koprowski, J. L. (2018). Ecosystem-level effects of keystone species reintroduction: a literature review. Restor. Ecol. 26, 439–445. doi: 10.1111/rec.12684
Hay, J. M., Sarre, S. D., Lambert, D. M., Allendorf, F. W., and Daugherty, C. H. (2010). Genetic diversity and taxonomy: a reassessment of species designation in tuatara (Sphenodon: Reptilia). Conserv. Genet. 11, 1063–1081. doi: 10.1007/s10592-009-9952-7
Hegg, D., Greaves, G., Maxwell, J. M., Mackenzie, D. I., and Jamieson, I. G. (2012). Demography of takahe (Porphyrio hochstetteri) in Fiordland: environmental factors and management affect survival and breeding success. N. Z. J. Ecol. 36, 75–89. Available online at: https://www.jstor.org/stable/24060877
Hegg, D., Mackenzie, D. I., and Jamieson, I. G. (2013). Use of Bayesian population viability analysis to assess multiple management decisions in the recovery programme for the Endangered takahe Porphyrio hochstetteri. Oryx 47, 144–152. doi: 10.1017/S0030605311001736
Heikkinen, R. K., Pöyry, J., Virkkala, R., Bocedi, G., Kuussaari, M., Schweiger, O., et al. (2015). Modelling potential success of conservation translocations of a specialist grassland butterfly. Biol. Conserv. 192, 200–206. doi: 10.1016/j.biocon.2015.09.028
Hunter, M., Westgate, M., Barton, P., Calhoun, A., Pierson, J., Tulloch, A., et al. (2016). Two roles for ecological surrogacy: indicator surrogates and management surrogates. Ecol. Indic. 63, 121–125. doi: 10.1016/j.ecolind.2015.11.049
Hunter-Ayad, J., and Hassall, C. (2020). An empirical, cross-taxon evaluation of landscape-scale connectivity. Biodivers. Conserv. 29, 1339–1359. doi: 10.1007/s10531-020-01938-2
Hunter-Ayad, J., Ohlemüller, R., Recio, M. R., and Seddon, P. J. (2020). Reintroduction modelling: a guide to choosing and combining models for species reintroductions. J. Appl. Ecol. 57, 1233–1243. doi: 10.1111/1365-2664.13629
IUCN/SSC. (2013). Guidelines for Reintroductions and Other Conservation Translacations. IUCN/SSC Re-introduction Specialist Group, IUCN, Gland, Switzerland and Cambridge, United Kingdom. Available online at: http://www.iucnsscrsg.org/
Jamieson, I. G., Wallis, G. P., and Briskie, J. V. (2006). Inbreeding and endangered species management: is New Zealand out of step with the rest of the world? Conserv. Biol. 20, 38–47. doi: 10.1111/j.1523-1739.2005.00282.x
Jarvie, S., Besson, A. A., Seddon, P. J., and Cree, A. (2014). Assessing thermal suitability of translocation release sites for egg-laying reptiles with temperature-dependent sex determination: a case study with tuatara. Anim. Conserv. 17, 48–55. doi: 10.1111/acv.12152
Jarvie, S., Recio, M. R., Adolph, S. C., Seddon, P. J., and Cree, A. (2016). Resource selection by tuatara following translocation: a comparison of wild-caught and captive-reared juveniles. N. Z. J. Ecol. 40, 334–341. doi: 10.20417/nzjecol.40.35
Jarvie, S., Senior, A. M., Adolph, S. C., Seddon, P. J., and Cree, A. (2015). Captive rearing affects growth but not survival in translocated juvenile tuatara. J. Zool. 297, 184–193. doi: 10.1111/jzo.12263
Jarvie, S., Worthy, T. H., Saltré, F., Scofield, R. P., Seddon, P. J., and Cree, A. (2021). Using Holocene fossils to model the future: Distribution of climate suitability for tuatara, the last rhynchocephalian. J. Biogeogr. 48, 1489–1502. doi: 10.1111/jbi.14092
Kennedy, P., Lach, L., Lugo, A., and Hobbs, R. (2013). “Fauna and novel ecosystems,” in Novel Ecosystems, eds. R. J. Hobbs, E. S. Higgs, and C. M. Hall (Chichester: Wiley-Blackwell), 127–141. doi: 10.1002/9781118354186
Kerley, G. I. H., Beest, M., Cromsigt, J. P. G. M., Pauly, D., and Shultz, S. (2020). The Protected Area Paradox and refugee species: the giant panda and baselines shifted towards conserving species in marginal habitats. Conserv. Sci. Pract. 2:e203. doi: 10.1111/csp2.203
Kerley, G. I. H., Kowalczyk, R., and Cromsigt, J. P. G. M. (2012). Conservation implications of the refugee species concept and the European bison: king of the forest or refugee in a marginal habitat? Ecography 35, 519–529. doi: 10.1111/j.1600-0587.2011.07146.x
Koolhaas, J. M., De Boer, S. F., Buwalda, B., and Van Reenen, K. (2007). Individual variation in coping with stress: a multidimensional approach of ultimate and proximate mechanisms. Brain. Behav. Evol. 70, 218–226. doi: 10.1159/000105485
Lee, W. G., and Jamieson, I. G. (2001). The Takahe: Fifty Years of Conservation Management and Research. Dunedin: University of Otago Press.
Lees, C. M., Greaves, G., Joustra, T., Eason, D., and Jamieson, I. (2014). 2014 Plan for a North Island Meta-Population of Takahē. Available online at: file:///Users/andrew/MyDocs/Conservation/papers/Papers2/Reports/2014/2014Lees_Plan_for_a_North_Island_meta-population_of_takahē.pdf%5Cnpapers2://publication/uuid/D47A5F25-8938-4B93-AF27-217B4B1CF77D
Lentini, P. E., Stirnemann, I. A., Stojanovic, D., Worthy, T. H., and Stein, J. A. (2017). Using fossil records to inform reintroduction of the kakapo as a refugee species. Biol. Conserv. 217, 157–165. doi: 10.1016/j.biocon.2017.10.027
Lloyd, B. D., and Powlesland, R. G. (1994). The decline of kakapo Strigops habroptilus and attempts at conservation by translocation. Biol. Conserv. 69, 75–85. doi: 10.1016/0006-3207(94)90330-1
Massaro, M., Chick, A., Kennedy, E. S., and Whitsed, R. (2018). Post-reintroduction distribution and habitat preferences of a spatially limited island bird species. Anim. Conserv. 21, 54–64. doi: 10.1111/acv.12364
McKenzie, K. L. (2007). Returning tuatara (Sphenodon punctatus) to the New Zealand mainland (M.Sc. dissertation). Victoria University of Wellington, Wellington, New Zealand.
Mertes, K., Stabach, J. A., Songer, M., Wacher, T., Newby, J., Chuven, J., et al. (2019). Management background and release conditions structure post-release movements in reintroduced ungulates. Front. Ecol. Evol. 7:470. doi: 10.3389/fevo.2019.00470
Mihoub, J. B., Jiguet, F., Lécuyer, P., Eliotout, B., and Sarrazin, F. (2014). Modelling nesting site suitability in a population of reintroduced Eurasian black vultures Aegypius monachus in the Grands Causses, France. Oryx 48, 116–124. doi: 10.1017/S0030605312000634
Miller, K. A., Miller, H. C., Moore, J. A., Mitchell, N. J., Cree, A., Allendorf, F. W., et al. (2012). Securing the demographic and genetic future of tuatara through assisted colonization. Conserv. Biol. 26, 790–798. doi: 10.1111/j.1523-1739.2012.01902.x
Mills, J. A., Lavers, R. B., Lee, W. G., and Mara, M. K. (1991). Food selection by Takahe Notornis mantelli in relation to chemical composition. ORNIS Scand. 22, 111–128. doi: 10.2307/3676542
Mitchell, N., Hipsey, M. R., Arnall, S., McGrath, G., Tareque, H., Bin, K. G., et al. (2012). Linking eco-energetics and eco-hydrology to select sites for the assisted colonization of Australia's rarest reptile. Biology 2, 1–25. doi: 10.3390/biology2010001
Mitchell, N. J., Kearney, M. R., Nelson, N. J., and Porter, W. P. (2008). Predicting the fate of a living fossil: how will global warming affect sex determination and hatching phenology in tuatara? Proc. R. Soc. B Biol. Sci. 275, 2185–2193. doi: 10.1098/rspb.2008.0438
Morris, W. F., and Doak, D. F. (2002). Quantitative Conservation Biology : Theory and Practice of Population Viability Analysis. Mass, MI: Sunderland; Sinauer Associates.
Mukhlisi, S. M., Yanuar, A., and Sayektiningsih, T. (2020). Wildlife diversity and identification of potential zoonosis around the Sumatran Rhino (Dicerorhinus sumatrensis harrissoni) sanctuary, East Kalimantan, Indonesia. IOP Conf. Ser. Earth Environ. Sci. 457:012029. doi: 10.1088/1755-1315/457/1/012029
O'Donnell, C. F. J., Weston, K. A., and Monks, J. M. (2017). Impacts of introduced mammalian predators on New Zealand's alpine fauna. N. Z. J. Ecol. 41, 1–22. doi: 10.20417/nzjecol.41.18
Osborne, P. E., and Seddon, P. J. (2012). “Selecting suitable habitats for reintroductions: variation, change and the role of species distribution modelling,” in Reintroduction Biology: Integrating Science and Management, eds J. G. Ewen, D. P. Armstrong, K. A. Parker, and P. J. Seddon (Chichester: Blackwell Publishing Ltd.), 73–104. doi: 10.1002/9781444355833.ch3
Panfylova, J., Bemelmans, E., Devine, C., Frost, P., and Armstrong, D. (2016). Post-release effects on reintroduced populations of hihi. J. Wildl. Manage. 80, 970–977. doi: 10.1002/jwmg.21090
Peres, C. A., Emilio, T., Schietti, J., Desmoulière, S. J. M., and Levi, T. (2016). Dispersal limitation induces long-term biomass collapse in overhunted Amazonian forests. Proc. Natl. Acad. Sci. U.S.A. 113, 892–897. doi: 10.1073/pnas.1516525113
Perring, M. P., Standish, R. J., Price, J. N., Craig, M. D., Erickson, T. E., Ruthrof, K. X., et al. (2015). Advances in restoration ecology: rising to the challenges of the coming decades. Ecosphere 6:art131. doi: 10.1890/ES15-00121.1
Phillips, S. J., Anderson, R. P., and Schapire, R. E. (2006). Maximum entropy modeling of species geographic distributions. Ecol. Modell. 190, 231–259. doi: 10.1016/j.ecolmodel.2005.03.026
Price, S. J., Grayson, K. L., Gartrell, B. D., and Nelson, N. J. (2020). Survival and growth of tuatara Sphenodon punctatus following translocation from the cook strait to warmer locations in their historic range. Oryx 54, 222–233. doi: 10.1017/S003060531800008X
Recio, M. R., Seddon, P. J., and Moore, A. B. (2015). Niche and movement models identify corridors of introduced feral cats infringing ecologically sensitive areas in New Zealand. Biol. Conserv. 192, 48–56. doi: 10.1016/j.biocon.2015.09.004
Rendall, A., Coetsee, A., and Sutherland, D. (2018). Predicting suitable release sites for assisted colonisations: a case study of eastern barred bandicoots. Endanger. Species Res. 36, 137–148. doi: 10.3354/esr00893
Ricciardi, A., and Simberloff, D. (2009). Assisted colonization is not a viable conservation strategy. Trends Ecol. Evol. 24, 248–253. doi: 10.1016/j.tree.2008.12.006
Robertson, H. A., Baird, K., Dowding, J. E., Elliott, G. P., Hitchmough, R. A., Miskelly, C. M., et al. (2016). Conservation Status of New Zealand Birds. Available online at: https://www.doc.govt.nz/Documents/science-and-technical/nztcs19entire.pdf
Rout, T. M., McDonald-Madden, E., Martin, T. G., Mitchell, N. J., Possingham, H. P., and Armstrong, D. P. (2013). How to decide whether to move species threatened by climate change. PLoS ONE 8:e75814. doi: 10.1371/journal.pone.0075814
Roy, H. E., Brown, P. M. J., Adriaens, T., Berkvens, N., Borges, I., Clusella-Trullas, S., et al. (2016). The harlequin ladybird, Harmonia axyridis: global perspectives on invasion history and ecology. Biol. Invasions 18, 997–1044. doi: 10.1007/s10530-016-1077-6
Sarrazin, F., and Barbault, R. (1996). Reintroduction: challenges and lessons for basic ecology. Trends Ecol. Evol. 11, 474–478. doi: 10.1016/0169-5347(96)20092-8
Scheele, B. C., Foster, C. N., Banks, S. C., and Lindenmayer, D. B. (2017). Niche contractions in declining species: mechanisms and consequences. Trends Ecol. Evol. 32, 346–355. doi: 10.1016/j.tree.2017.02.013
Seddon, P. J., Armstrong, D. P., and Maloney, R. F. (2007). Developing the science of reintroduction biology. Conserv. Biol. 21, 303–312. doi: 10.1111/j.1523-1739.2006.00627.x
Seddon, P. J., Griffiths, C. J., Soorae, P. S., and Armstrong, D. P. (2014). Reversing defaunation: restoring species in a changing world. Science 354, 406–412. doi: 10.1126/science.1251818
Smetzer, J. R., Greggor, A. L., Paxton, K. L., Masuda, B., and Paxton, E. H. (2021). Automated telemetry reveals post-reintroduction exploratory behavior and movement patterns of an endangered corvid, ‘Alalā (Corvus hawaiiensis) in Hawai‘i, USA. Glob. Ecol. Conserv. 26:e01522. doi: 10.1016/j.gecco.2021.e01522
Smith, R. J., Werner, P., and Kelcey, J. G. (2012). Reintroduction Biology Conservation Science and Practice Series. Chichester: Blackwell Publishing Ltd.
Snyder, N. F. R., Derrickson, S. R., Beissinger, S. R., Wiley, J. W., Smith, T. B., Toone, W. D., et al. (1996). Limitations of captive breeding in endangered species recovery. Conserv. Biol. 10, 338–348. doi: 10.1046/j.1523-1739.1996.10020338.x
Soriano-Redondo, A., Jones-Todd, C. M., Bearhop, S., Hilton, G. M., Lock, L., Stanbury, A., et al. (2019). Understanding species distribution in dynamic populations: a new approach using spatio-temporal point process models. Ecography 42, 1092–1102. doi: 10.1111/ecog.03771
Stüwe, M., and Nievergelt, B. (1991). Recovery of alpine ibex from near extinction: the result of effective protection, captive breeding, and reintroductions. Appl. Anim. Behav. Sci. 29, 379–387. doi: 10.1016/0168-1591(91)90262-V
Sutherland, W. J., Pullin, A. S., Dolman, P. M., and Knight, T. M. (2004). The need for evidence-based conservation. Trends Ecol. Evol. 19, 305–308. doi: 10.1016/j.tree.2004.03.018
Svenning, J. C., Pedersen, P. B. M., Donlan, C. J., Ejrnæs, R., Faurby, S., Galetti, M., et al. (2016). Science for a wilder anthropocene: synthesis and future directions for trophic rewilding research. Proc. Natl. Acad. Sci. U.S.A. 113, 898–906. doi: 10.1073/pnas.1502556112
Taylor, G., Canessa, S., Clarke, R. H., Ingwersen, D., Armstrong, D. P., Seddon, P. J., et al. (2017). Is Reintroduction biology an effective applied science? Trends Ecol. Evol. 32, 873–880. doi: 10.1016/j.tree.2017.08.002
Taylor, H., Nelson, N., and Ramstad, K. (2018). The first recorded interaction between two species separated for centuries suggests they were ecological competitors. N. Z. J. Ecol. 43, 1–6. doi: 10.20417/nzjecol.43.11
Teixeira, C. P., de Azevedo, C. S., Mendl, M., Cipreste, C. F., and Young, R. J. (2007). Revisiting translocation and reintroduction programmes: the importance of considering stress. Anim. Behav. 73, 1–13. doi: 10.1016/j.anbehav.2006.06.002
Thatcher, C. A., Manen, F. T., and Van Clark, J. D. (2006). Identifying suitable sites for florida panther reintroduction. J. Wildl. Manage. 70, 752–763. doi: 10.2193/0022-541X(2006)70752:ISSFFP2.0.CO;2
Thompson, M. B., and Daugherty, C. H. (1998). Metabolism of tuatara, Sphenodon punctatus. Comp. Biochem. Physiol. A Mol. Integr. Physiol. 119, 519–522. doi: 10.1016/S1095-6433(97)00459-5
Tingley, R., Vallinoto, M., Sequeira, F., and Kearney, M. R. (2014). Realized niche shift during a global biological invasion. Proc. Natl. Acad. Sci. U.S.A. 111, 10233–10238. doi: 10.1073/pnas.1405766111
Waters, C. N., Zalasiewicz, J., Summerhayes, C., Barnosky, A. D., Poirier, C., Gałuszka, A., et al. (2016). The Anthropocene is functionally and stratigraphically distinct from the Holocene. Science 351:aad2622. doi: 10.1126/science.aad2622
Weeks, A. R., Sgro, C. M., Young, A. G., Frankham, R., Mitchell, N. J., Miller, K. A., et al. (2011). Assessing the benefits and risks of translocations in changing environments: a genetic perspective. Evol. Appl. 4, 709–725. doi: 10.1111/j.1752-4571.2011.00192.x
White, T. H., Collazo, J. A., Dinsmore, S. J., and Llerandi-Román, I. (2014). “Niche restriction and conservatism in a neotropical Psittacine: the case of the Puerto Rican parrot,” in Habitat Loss: Causes, Impacts on Biodiversity and Reduction Strategies, ed B. Devore (New York, NY: Nova Science Publishers, Inc.), 1–83.
Wilmshurst, J. M., Anderson, A. J., Higham, T. F. G., and Worthy, T. H. (2008). Dating the late prehistoric dispersal of Polynesians to New Zealand using the commensal Pacific rat. Proc. Natl. Acad. Sci. U.S.A. 105, 7676–7680. doi: 10.1073/pnas.0801507105
Worthy, T. H., and Holdaway, R. N. (2002). The Lost World of the Moa: Prehistoric life of New Zealand. Indiana University Press.
WWF (2020). Living Planet Report 2020 - Bending the Curve of Biodiversity Loss. Gland: WWF. Available online at: http://www.ecoguinea.org/papers-development.html
Yott, A., Rosatte, R., Schaefer, J. A., Hamr, J., and Fryxell, J. (2011). Movement and spread of a founding population of reintroduced elk (Cervus elaphus) in Ontario, Canada. Restor. Ecol. 19, 70–77. doi: 10.1111/j.1526-100X.2009.00639.x
Zavodna, M., Abdelkrim, J., Pellissier, V., and Machon, N. (2015). A long-term genetic study reveals complex population dynamics of multiple-source plant reintroductions. Biol. Conserv. 192, 1–9. doi: 10.1016/j.biocon.2015.08.025
Keywords: translocation, restoration, novelty, ecological conservation, strategy, wildlife management, evidence-based conservation, adaptive management
Citation: Hunter-Ayad J, Jarvie S, Greaves G, Digby A, Ohlemüller R, Recio MR and Seddon PJ (2021) Novel Conditions in Conservation Translocations: A Conservative-Extrapolative Strategic Framework. Front. Conserv. Sci. 2:691714. doi: 10.3389/fcosc.2021.691714
Received: 06 April 2021; Accepted: 09 June 2021;
Published: 08 July 2021.
Edited by:
Rob Hale, Arthur Rylah Institute for Environmental Research (ARI), AustraliaReviewed by:
Thomas H. White, United States Fish and Wildlife Service, Puerto RicoColleen Cassady St. Clair, University of Alberta, Canada
Copyright © 2021 Hunter-Ayad, Jarvie, Greaves, Digby, Ohlemüller, Recio and Seddon. This is an open-access article distributed under the terms of the Creative Commons Attribution License (CC BY). The use, distribution or reproduction in other forums is permitted, provided the original author(s) and the copyright owner(s) are credited and that the original publication in this journal is cited, in accordance with accepted academic practice. No use, distribution or reproduction is permitted which does not comply with these terms.
*Correspondence: James Hunter-Ayad, jameshunter.ayad@gmail.com