- 1Department of Biological Sciences, University of Texas at El Paso, El Paso, TX, United States
- 2Department of Vertebrate Zoology, National Museum of Natural History, Washington, DC, United States
- 3Ocean and Earth Science, University of Southampton, Southampton, United Kingdom
- 4Museum Conservation Institute, Smithsonian Institution, Suitland-Silver Hill, MD, United States
- 5Department of Ecology and Evolutionary Biology, Cornell University, Ithaca, NY, United States
- 6Scottish Marine Animal Stranding Scheme, Invernesst, United Kingdom
- 7Institute of Biodiversity, Animal Health & Comparative Medicine, University of Glasgow, Glasgow, United Kingdom
- 8German Oceanographic Museum Foundation, Stralsund, Germany
- 9Icelandic Institute of Natural History, Gardabaer, Iceland
- 10Canadian Museum of Nature, Ottawa, ON, Canada
- 11Department of Biology, Laurentian University, Greater Sudbury, ON, Canada
- 12Department of Natural Sciences, National Museums Scotland, Edinburgh, United Kingdom
- 13Natural History Museum Rotterdam, Rotterdam, Netherlands
- 14Maurice Lamontagne Institute, Fisheries and Oceans Canada, Mont-Joli, QC, Canada
- 15University Museum of Bergen, Bergen, Norway
- 16Human Origins Program, National Museum of Natural History, Washington, DC, United States
- 17Department of Life Sciences, Natural History Museum, London, United Kingdom
- 18Marine and Freshwater Research Institute, Reykjavik, Iceland
- 19Protected Species Branch, Northeast Fisheries Science Center, Falmouth, MA, United States
Elusive wildlife are challenging to study, manage, or conserve, as the difficulty of obtaining specimens or conducting direct observations leads to major data deficiencies. Specimens of opportunity, such as salvaged carcasses or museum specimens, are a valuable source of fundamental biological and ecological information on data-deficient, elusive species, increasing knowledge of biodiversity, habitat and range, and population structure. Stable isotope analysis is a powerful indirect tool that can be used to infer foraging behavior and habitat use retrospectively from archived specimens. Beaked whales are a speciose group of cetaceans that are challenging to study in situ, and although Sowerby's beaked whale (Mesoplodon bidens) was discovered >200 years ago, little is known about its biology. We measured δ13C and δ15N stable isotope composition in bone, muscle, and skin tissue from 102 Sowerby's beaked whale specimens of opportunity collected throughout the North Atlantic Ocean to infer movement ecology and spatial population structure. Median δ13C and δ15N values in Sowerby's beaked whale bone, muscle, and skin tissues significantly differed between whales sampled from the east and west North Atlantic Ocean. Quadratic discriminant analysis that simultaneously considered δ13C and δ15N values correctly assigned >85% of the specimens to their collection region for all tissue types. These findings demonstrate Sowerby's beaked whale exhibits both short- and long-term site fidelity to the region from which the specimens were collected, suggest that this species is composed of two or more populations or exhibits a metapopulation structure, and have implications for conservation and management policy. Stable isotope analysis of specimens of opportunity proved a highly successful means of generating new spatial ecology data for this elusive species and is a method that can be effectively applied to other elusive species.
Introduction
Knowledge of a species' population structure and spatial ecology is essential for effective wildlife conservation, particularly in potentially highly migratory marine species. Species of concern are often by definition rare or elusive, resulting in large gaps in knowledge regarding their biology and ecology (Cunningham and Lindenmayer, 2005). As a result, the conservation needs of these species are often unknown, presenting challenges to those tasked with developing conservation and management plans. Traditional field research techniques may be ineffective when species are rarely encountered, live in remote or inaccessible habitats, or actively avoid human researchers and equipment (Green and Young, 1993; Breck, 2006; Meek et al., 2014). In such cases, alternative, often indirect, approaches to the generation of basic ecological and biological knowledge may be needed to fill essential knowledge gaps (Piggott and Taylor, 2003; Joseph et al., 2006).
Specimens of opportunity provide sources of ecological and biological information that may be particularly valuable for rare and elusive species (Roberts et al., 2016). These sources include museum specimens, salvaged carcasses, or specimens collected in the wildlife trade or for human consumption and sold in the marketplace. Museums are critically important repositories of biological data, and museum specimens have been used in comparative studies, to identify new species and to understand historical biodiversity (Newbold, 2010; Holmes et al., 2016; MacLean et al., 2019). Salvaged carcasses, such as roadkill or stranded marine mammals, have provided new information on range and population structure (Coombs et al., 2019; Schwartz et al., 2020). Similarly, animals collected for the pet trade or human consumption have yielded new species and information on hybridization events (Erdmann, 1999; Baker et al., 2007; Ebert et al., 2019). As research tools develop, the quantity and quality of information that can be gained from these specimens of opportunity grow, providing an invaluable resource to investigate the biology and ecology of elusive species.
Stable isotope analysis is a powerful and efficient tool that can be used on specimens of opportunity for addressing biological and ecological questions that may otherwise be challenging or impossible to answer (McKechnie, 2004; West et al., 2006; MacKenzie et al., 2011). Using a combination of tissues synthesized at different times or at different rates, researchers can infer spatial origin and diet across time (Phillips and Eldridge, 2006; Vander Zanden et al., 2015). The rate that stable isotopes are incorporated into a specific tissue is determined by that tissue's growth and replacement rates. Some tissues grow rapidly and are replaced within months, while others are incrementally grown and replaced depending on environmental and physiological pressures (Fry and Arnold, 1982). Stable isotope analysis conducted on specimens of opportunity has been used to infer animal migrations, the spatial origin of wildlife products, and even historical trophic structures (Hobson, 1999; Chasar et al., 2005; Hopkins and Ferguson, 2012). Two of the most commonly used isotopes for wildlife studies are carbon (expressed as δ13C values), used to evaluate habitat range and latitudinal shifts, and nitrogen (expressed as δ15N values), used for obtaining foraging and trophic information (Ben-David and Flaherty, 2012). As the body of literature on the application of stable isotope analysis to wildlife studies grows, in conjunction with more high-resolution maps documenting the spatial relationship of stable isotope abundance (i.e., isoscapes), this analytical approach creates more opportunities to utilize specimens of opportunity to increase knowledge regarding elusive species (Vander Zanden et al., 2018; Trueman et al., 2019).
Although beaked whales comprise more than 25% of extant whale and dolphin species, they are poorly understood and elusive, with most questions regarding their basic biology unanswered (Dalebout et al., 2004; Mead, 2009). This paucity of data is largely attributable to the challenge of locating beaked whales and distinguishing among species due to their elusive behavior and similar appearance (MacLeod et al., 2005). Beaked whales generally inhabit deep ocean, off-shelf habitats, and their relatively small size, limited time spent at the surface, and deep-diving behavior protected most species from being targeted by commercial whaling operations and contribute to a lack of observational data. Consequently, visually identifying and studying beaked whales in situ is challenging, and many field sightings of beaked whales can only be reliably identified to genus, with possibly a suggestion of species. Specimens of opportunity already have proven critical to increasing our understanding of beaked whale diversity and ecology (Feyrer et al., 2020; Landrau-Giovannetti et al., 2020). For example, three species (Mesoplodon bowdoini, M. traversii, and M. hotaula) have never been observed alive and are known only from stranded carcasses, and several other species are known from stranded carcasses and a few unconfirmed sightings (van Helden et al., 2002; Dalebout et al., 2014). Similarly, during the last 20 years, four new species were discovered by re-examining museum specimens (Dalebout et al., 2002, 2014; Yamada et al., 2019).
Sowerby's beaked whale (Mesoplodon bidens) was first described in 1804, yet in more than 200 years, little has been learned about its life history (Sowerby, 1804; MacLeod et al., 2005; Ellis et al., 2017). Most information on the species' basic biology, such as its spatial and foraging ecology, is still largely unknown, explaining why it is considered “data deficient” by the IUCN and a species of special concern by the Committee on the Status of Endangered Wildlife in Canada (Taylor et al., 2008; COSEWIC, 2019). The species' range encompasses much of the North Atlantic Ocean, and although individuals of this species have been observed and collected from both North American and European waters, it is unknown if this is one continuous and highly mobile population, or if the species is structured into spatially distinct subpopulations.
Based on the lack of data on the population structure and spatial ecology of Sowerby's beaked whales, management needs of this species are unclear and effective conservation plans cannot be developed across this species' range. Site fidelity has been recorded in other beaked whales, such as Cuvier's (Ziphius cavirostris) and Blainville's (M. densirostris), but this has not been investigated or documented in Sowerby's beaked whale (McSweeney et al., 2007). Analysis of seven cranial elements from 112 Sowerby's beaked whale museum specimens identified significant morphological differences between specimens collected in the east and west Atlantic Ocean, suggesting there may be distinct populations of Sowerby's beaked whale (Smith et al., 2021). However, mitochondrial DNA analysis of 14 individuals identified both regionally distinct and shared haplotypes between animals collected from both sides of the Atlantic Ocean (COSEWIC, 2006). Thus, the spatial ecology and population connectivity of this species are largely unknown. Additional data regarding the spatial ecology of Sowerby's beaked whale are needed to identify conservation threats and aid in the development of management plans.
In this study, we measured δ13C and δ15N compositions of three tissues with distinct growth and isotopic turnover rates from Sowerby's beaked whale specimens of opportunity from the east and west Atlantic. Our research brings together tissues from museum specimens, stranded carcasses, and bycaught animals to create a robust and diverse collection of specimens of opportunity. Our objectives were to (i) evaluate the general efficacy of specimens of opportunity in spatial ecology studies, (ii) identify and characterize regional patterns in isotopic values among Sowerby's beaked whale individuals, and (iii) determine if isotope values from specimens of opportunity can be used to illuminate the spatial ecology of Sowerby's beaked whales across months and years.
Methods
Sampling
We sampled 102 opportunistically collected Sowerby's beaked whale specimens from museums, stranding programs, and research centers for bone (n = 71), muscle (n = 40), and skin (n = 50) tissue samples (Supplementary Table 1). For 46 specimens, we acquired more than one tissue type: bone, muscle, and skin from 13; bone and muscle from 4; bone and skin from 12; and muscle and skin from 17. Original specimen collection locations were from the east and west North Atlantic Ocean, here defined as being on either side of the 35th meridian west (Figure 1). East Atlantic specimens (n = 64) were stranded or recovered in dredging operations, whereas west Atlantic specimens (n = 38) were stranded or bycaught in the former swordfish (Xiphias gladius) pelagic drift gillnet fishery of the western North Atlantic (Wenzel et al., 2013). Original specimen collection dates ranged across all months and seasons from 1980 to 2019; included male, female, and unknown sex individuals; and spanned age classes (Supplementary Table 1).
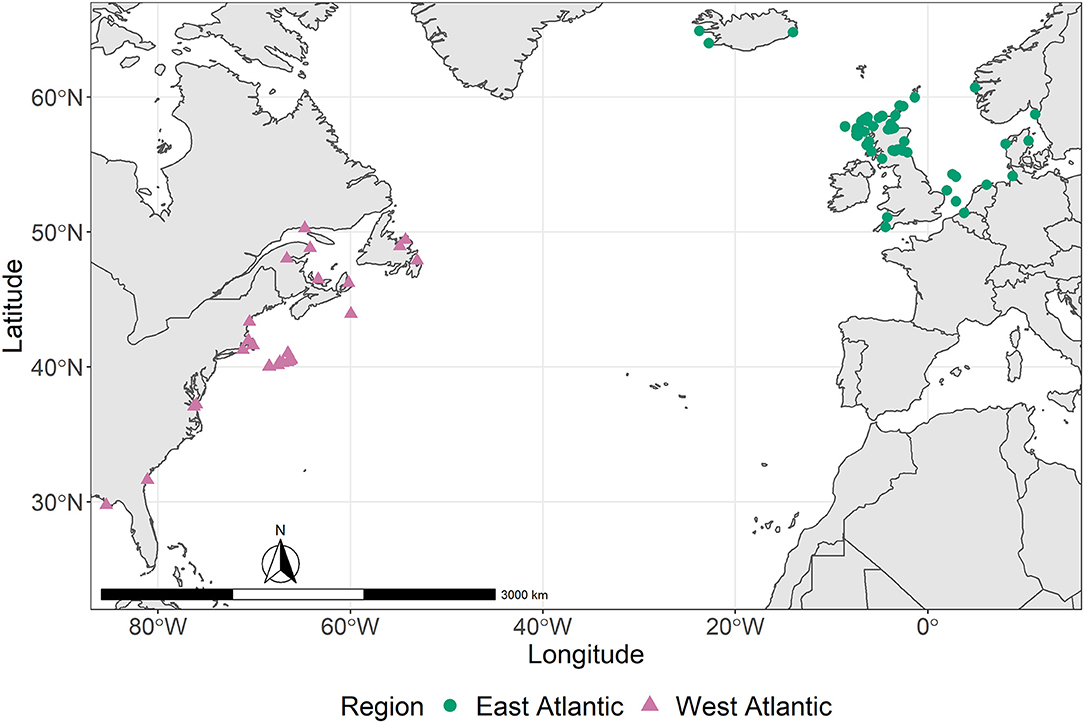
Figure 1. Collection locations for 102 opportunistically collected Sowerby's beaked whale specimens housed in museums, stranding programs, and research centers collected in the east (n = 64) and west (n = 38) Atlantic Ocean basin, defined here as being on either side of the 35th meridian west.
We used a handheld drill to remove 1 g of bone tissue from the occipital bone, when available. In 17 specimens, this bone was not available, and we sampled an alternate location. We sampled 0.5 g from soft tissues and stored them in 95% ethanol for transportation. Soft tissues are commonly preserved in ethanol, which can contribute to lipid removal but has insignificant effects on δ13C and δ15N values (Sarakinos et al., 2002; Javornik et al., 2019).
Stable Isotope Analysis
We subsampled ~200 mg of bone tissue for collagen extraction and followed the protocol outlined by Smith et al. (2020), including lipid extraction and HCl and NaOH baths to remove the mineral component. Analysis was completed at the Cornell Isotope Laboratory at Cornell University using a Thermo Delta V isotope mass spectrometer interfaced with a NC2500 elemental analyzer (Thermo Fisher Scientific, 168 Third Avenue Waltham, MA, USA 02451). We calibrated our sample values using two in-house protein standards with known δ13C and δ15N values relative to Vienna Pee Dee Belemnite (V-PDB) for δ13C and Atmospheric Air for δ15N. An in-house animal tissue standard was included between every 10 samples, with an analytical precision of ±0.1‰ (1σ) for δ13C and δ15N.
Soft tissue samples were freeze-dried, finely ground, and lipid extracted with 2:1 chloroform:methanol for 30 min, manually agitating every 5 min; additional lipid extractions were performed as necessary if the supernatant was not clear. We dried samples at 60°C after extraction. Analysis was completed at the Smithsonian Museum Conservation Institute Stable Isotope Mass Spectrometry Laboratory using a Thermo Delta V Advantage mass spectrometer coupled to an Elementar vario ISOTOPE Cube Elemental Analyzer via a Thermo Conflo IV (Thermo Fisher Scientific, 168 Third Avenue Waltham, MA, USA 02451). We calibrated our sample values to V-PDB and Air via two standards, an in-house Costech Acetanilide (Costech Analytical, 26074 Avenue Hall, Suite 14 Valencia, CA, USA 91355) and Urea-UIN3, calibrated to USGS40 and USGS41 L-glutamic acid (Schimmelmann et al., 2009). Standards were included between every 10 samples, with an analytical precision of ±0.2‰ (1σ) for δ13C and δ15N.
We use delta notation (δ) to express our stable isotope results. This is the parts per thousand difference between the sample and international standards, expressed as δyX = [(Rsample – Rstandard) / (Rstandard)], where X is the element, y is the atomic mass of the stable isotope, and R is the ratio of heavy to light isotopes. In order to account for the Suess effect (i.e., changing atmospheric carbon isotope ratios due to fossil fuel input) (Keeling, 1979), we Suess-corrected the data by adding 0.015‰ to the δ13C value for each year since 1980 to the date the sample was collected (Sonnerup et al., 1999; Young et al., 2013). Because the cumulative change in δ13C values caused by the Suess effect across the time the samples in this study were collected exceeded the analytical precision of our standards, failure to account for this variation could result in the mischaracterization of δ13C values for this species.
Data Analysis
We first explored differences in isotopic values between samples collected from the east and west Atlantic graphically and using descriptive statistics. We performed Mann–Whitney U-tests to explore differences in median tissue isotope values between regions and considered p ≤ 0.05 significant. This test was selected because some data categories were non-normally distributed. We then assessed the ability to assign samples to their collection location in the east or west Atlantic based on stable isotope values using jackknife quadratic discriminant analysis of δ13C values alone, δ15N values alone, or δ13C and δ15N values simultaneously. Quadratic discriminant analysis was used because it is appropriate for analyzing data which are unequally sampled across regions and have unequal variance. We performed all analyses using R (R Core Team, 2018) with RStudio (RStudio Team, 2016) and JMP (SAS, 2019).
Results
δ13C and δ15N biplots demonstrated that stable isotope values differed between tissues from Sowerby's beaked whales collected from the east and west Atlantic (Figure 2). Of the three tissues sampled, bone had the most overlap in isotope values between regions, whereas the regional groups for both skin and muscle samples were more distinct. Box and whisker plots demonstrated differences in median isotope values in all three tissues between collection regions, and that specimens collected in the west Atlantic consistently displayed higher median δ13C and δ15N values (Figure 3). East Atlantic specimens exhibited a larger range in δ13C and δ15N values than west Atlantic specimens except for muscle δ15N, which was the same between regions (Table 1). In both regions and for both isotopes, bone was more enriched than muscle and skin. Median δ13C values in muscle were higher than those in skin in both regions. Median δ15N values were higher in skin compared with muscle in specimens from the east Atlantic but lower than muscle in specimens from the west Atlantic. Mann–Whitney U-tests demonstrated significant differences in median δ13C and δ15N values between east and west Atlantic samples in all three tissue types (Table 1).
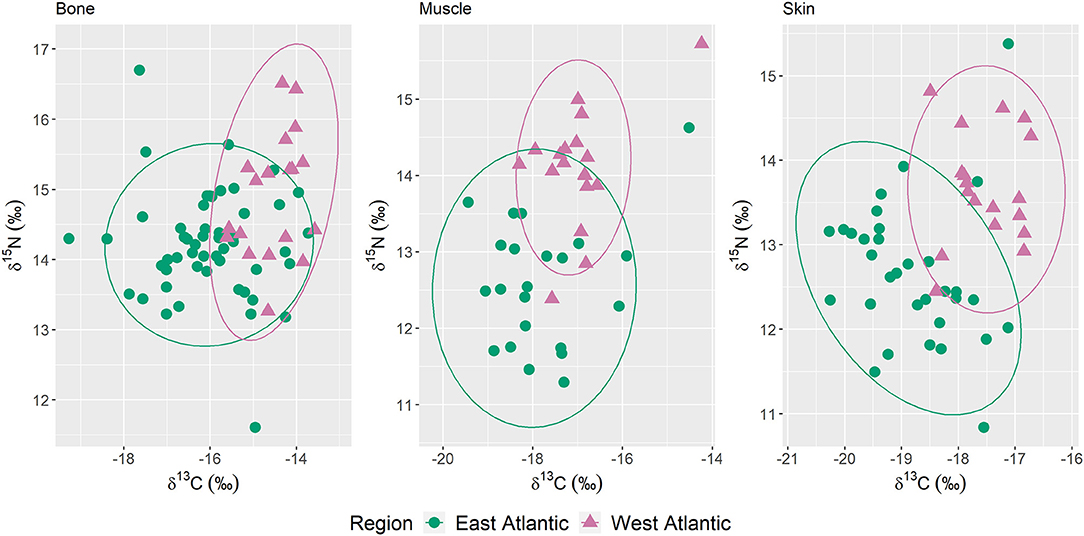
Figure 2. δ13C and δ15N of bone (n = 71), muscle (n = 40), and skin (n = 50) samples from Sowerby's beaked whale specimens of opportunity collected 1980–2019. Ellipses are 95% normal confidence ellipses.
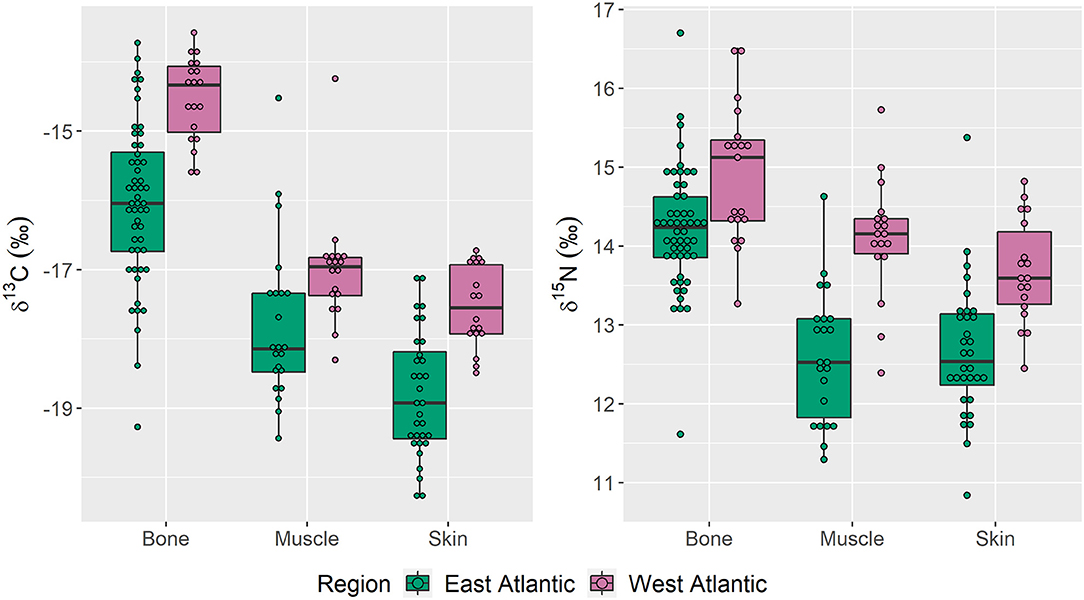
Figure 3. δ13C and δ15N values from Sowerby's beaked whale specimens of opportunity collected in the east (n = 64) and west (n = 38) Atlantic Ocean basin, 1980–2019. Boxes present median and interquartile range and whiskers represent 95% confidence intervals.
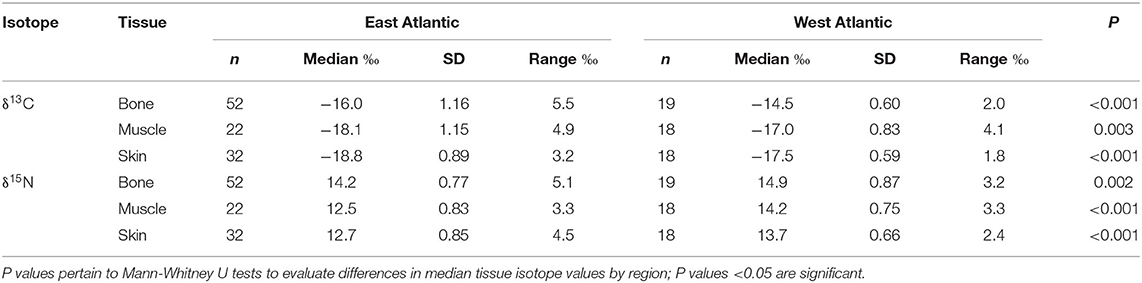
Table 1. δ13C and δ15N values of Sowerby's beaked whale specimens of opportunity collected in the east and west Atlantic Ocean basin, 1980–2019.
Quadratic discriminant analysis assigned specimens to their collection location with a high degree of success (Table 2A). Analysis that simultaneously considered both δ13C and δ15N values was more successful at correctly assigning specimens than analysis of either isotope separately. Skin, muscle, and bone samples analyzed simultaneously for δ13C and δ15N were correctly assigned for 92.0, 90.0, and 84.5% of the samples, respectively. Single isotope assignment percent probabilities for all tissue types were >70.0% (70.4–82.5%; Table 2A). We found no consistent trends in sex, age, or collection location among misassigned samples. Of the 46 specimens sampled for more than one tissue type, simultaneous δ13C and δ15N quadratic discriminant analysis correctly assigned 80.4% (n = 37) to their collection location across all tissues (Table 2B).
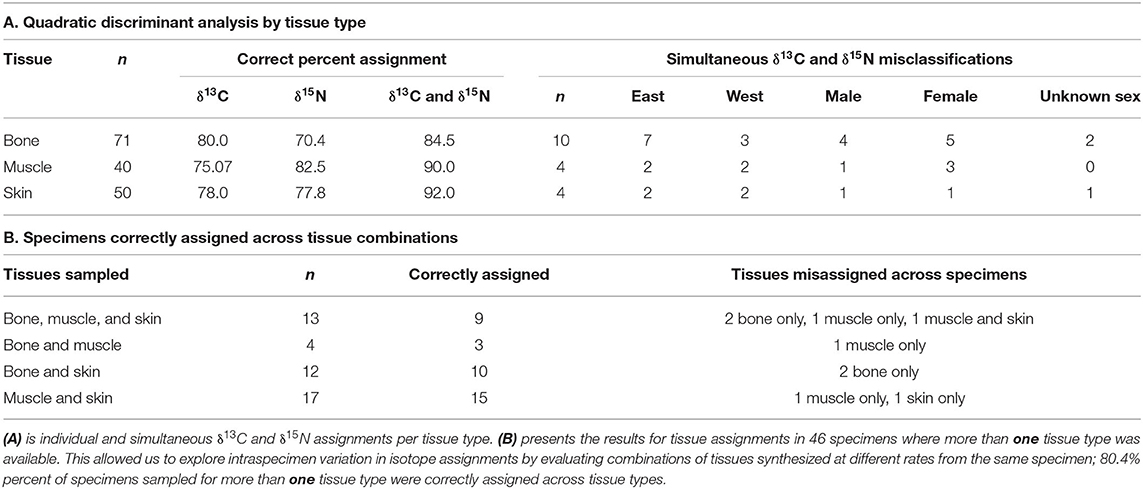
Table 2. Quadratic discriminant analysis assignment percent probabilities for δ13C and δ15N values of Sowerby's beaked whale specimens of opportunity collected in the east and west Atlantic Ocean basin, 1980–2019.
Discussion
Our results suggest that Sowerby's beaked whales exhibit short- and long-term regional site fidelity. The regional differences in δ13C and δ15N values across three tissue types with different growth and turnover rates imply these Sowerby's beaked whales were not only present in the region from which they were collected during the final months of their lives, but over a long-term, possibly decadal, scale. Exact tissue growth and turnover times are species-dependent and influenced by animal health and body condition, where the sample was taken from the carcass, and environmental factors such as temperature. These values are not known for Sowerby's beaked whales, or indeed for most cetaceans; however, we can make broad approximations based on other marine mammals, which experience similar ecophysiological pressures, and large terrestrial mammals (Newsome et al., 2010; Vander Zanden et al., 2015).
Stable Isotope Values by Tissue Type
Skin is the fastest growing tissue we evaluated, and the isotopic composition of skin proteins represent short-term movement and foraging behavior. Skin can be relatively easily sampled in wild cetacean populations using biopsy darts, its growth rate has been studied in bottlenose dolphins (Tursiops truncatus) and beluga whales (Delphinapterus leucas), and skin isotope incorporation rate has been studied in captive bottlenose dolphins and killer whales (Orcinus orca). Hicks et al. (1985) estimated complete skin turnover in bottlenose dolphins at 73 days, while Aubin et al. (1990) found similar results (70–75 days) in beluga whales. Williams et al. (2008) found that captive bottlenose dolphins and killer whales fed controlled diets for 5–7 months had reached isotopic equilibrium in their skin and had isotope values that reflected their diets. Thus, we estimate the skin isotope signatures in the Sowerby's beaked whales in our study reflect habitat and foraging behavior ~3–7 months prior to sampling.
Skin samples from the east Atlantic had lower mean δ13C values than those from the west Atlantic, a pattern which parallels the distribution of δ13C values in Atlantic Ocean isoscape models (Magozzi et al., 2017). Nitrogen isotope values followed the same pattern, with east Atlantic samples showing lower δ15N values than west Atlantic samples. The clear distinction in median δ13C and δ15N isotope values between east and west Atlantic specimens and the high assignment percent probabilities for simultaneous δ13C and δ15N values suggest that the animals in our study were living and foraging in the region from which they were collected several months prior to their deaths (Tables 1, 2, Figure 3). This indicates these animals were not moving between the east and west Atlantic during the months prior to their collection, suggesting regional site fidelity on the order of months at a time.
Muscle is a more challenging tissue to study than skin due to the invasive nature required to collect samples, which is often limited to animals that have died and been opportunistically sampled, or to non-cetacean animals that have been sacrificed in feeding studies. As a result, there is a lack of information on cetacean muscle growth and isotope turnover time. Vander Zanden et al. (2015) found a positive correlation between body mass and isotope half-life in mammal muscle tissue, and muscle isotope turnover rate has been studied in cattle, which provide the best current approximation to Sowerby's beaked whale due to similar body mass (i.e., ~700 kg). Bahar et al. (2009) switched diets of beef cattle 5 months before slaughter and found that carbon and nitrogen isotopic equilibrium was not reached in that time, implying that muscle turnover time and isotopic integration in mammals of this size likely takes more than 5 months. They suggested it may take a year or more for sampled muscle tissue to reflect diet. For these reasons, we estimate that muscle isotope signatures in Sowerby's beaked whales reflect foraging and habitat use from about 1 year prior to sampling.
Muscle samples followed the same isotopic patterns as skin, with lower δ13C and δ15N values in whales from the east Atlantic compared with those from the west Atlantic (Table 1, Figure 3). These results suggest that animals were in the region of collection about 1 year prior to sampling. Combined with the shorter temporal snapshot of skin, muscle values strongly suggest that Sowerby's beaked whales are not frequently moving between regions, instead demonstrating regional site fidelity for 1 year or more.
No data are available on cetacean bone growth and turnover rates. However, in other large mammals, bone can represent a decade or more of growth and has a turnover rate of 3–10% per year in adults (Clarke, 2008; Charapata et al., 2018). Thus, bone tissue presents a consolidated isotopic signature from several years, making this the most complex tissue to analyze in our study. Despite this complexity, bone tissue followed the same patterns as skin and muscle, with lower δ13C and δ15N values in east Atlantic specimens and with distinct isotopic median values between regions (Table 1, Figure 3). Bone had the lowest simultaneous assignment percent probability; however, with an assignment percent probability of 84.5%, isotopic variation among specimens from the same region may reflect changing ecosystem isotope values rather than trans-Atlantic movement patterns (Table 2A). Ecosystem variables, such as the Atlantic meridional mode, which contributes to interannual and decadal variation in Atlantic Ocean sea surface temperature, the confluence of shallow and deep-water currents particularly in the western Atlantic, and globally changing δ13C values due to the Suess effect, may drive within-region bone isotope variation (Reverdin et al., 2003; Hakkinen and Rhines, 2009; Doi et al., 2010; Lorrain et al., 2020). Additionally, Smith et al. (2020) found that Sowerby's beaked whale skeletons exhibit median intraskeletal δ13C variation of ~4‰, which may explain some of the isotope variation and misassigned specimens in our study because we could not sample the occipital bone in 17 specimens. Thus, the bone isotopic values in our study demonstrate that bone tissue is largely being grown in a single geographic region, and even in this complex and slow-growing tissue, we see long-term east and west Atlantic site fidelity, with the possibility of infrequent broader movements.
Spatial Population Structuring
Distinct median δ15N values were observed between east and west Atlantic specimens across tissue types, suggesting long-term differences in foraging locations between these groups (Table 1, Figure 3). Few data are available regarding Sowerby's beaked whale foraging, as most specimens strand without stomach contents. In the east Atlantic, stomach contents have been analyzed from specimens that were stranded in the Azores and the Bay of Biscay, where both studies found that small- to medium-sized mid-water fish species, such as hake and cod (e.g., Micromesistius poutassou, Trisopterus spp., and Merluccius merluccius), comprised the majority of stomach contents (Pereira et al., 2011; Spitz et al., 2011). In the west Atlantic, stomach contents from healthy Sowerby's beaked whales bycaught in the former pelagic driftnet fishery revealed similarities in prey items: fish comprised the majority of stomach contents, with short beard codling (Laemonema barbatulum), Cocco's lanternfish (Lobianchia gemellarii), marlin-spike grenadier (Nezumia bairdii), lanternfishes (Lampanyctus spp.), and longfin hake (Phycis chesteri) being the most abundant (Wenzel et al., 2013). Despite the similarities in types of prey items between east and west Atlantic specimens, the differences we observed in δ15N values indicate that east and west Atlantic Sowerby's beaked whales demonstrate spatial variation in their foraging behavior and long-term fidelity in their foraging locations.
Distinct median δ13C values in our specimens indicate long-term regional fidelity rather than continuous or seasonal movement throughout the Atlantic Ocean basin (Table 1, Figure 3). We observed a pattern of lower δ13C values in whales sampled from the east Atlantic compared with the west Atlantic across tissue types; this trend is consistent with δ13C isoscape models of the Atlantic Ocean basin (Magozzi et al., 2017; Trueman and St John Glew, 2019). Although we Suess-corrected our samples to account for the long-term increase in isotopically light carbon being incorporated into marine ecosystems due to fossil fuel use (Keeling, 1979; Sonnerup et al., 1999), recent studies have shown more extreme declines in some marine ecosystem δ13C values than previously recorded (Lorrain et al., 2020). These changing regional δ13C values could account for some of the δ13C variation we observed among specimens collected from the same region; however, δ13C values alone still successfully assigned >70% of the specimens across all tissue types to their collection region (Table 2A). The trends in δ13C values in our samples aligned with trends in regional δ13C isoscape values, suggesting that if it we had sufficient ecosystem data to account for environmental fluctuations in δ13C values for these samples, assignment percent probability would increase.
Among the specimens sampled for more than one tissue type, simultaneous δ13C and δ15N discriminant analysis correctly assigned a high degree (80.4%, Table 2B) of specimens to their collection location across tissues. Analysis of combinations of tissues synthesized at different times from the same specimens provided an opportunity to explore intraspecimen variation in isotope assignments. Of the 46 specimens sampled for a combination of tissues, 37 were correctly assigned to their collection region across all tissue types. This indicates that these specimens were continuously living in the region of their collection prior to their deaths and that trans-Atlantic movements may be rare in this species, providing further insight into the long-term site fidelity patterns of these specimens. The results of this study provide the first stable isotope evidence for spatial structuring in Sowerby's beaked whale. Coupled with previously identified morphological differences in skull measurements (Smith et al., 2021), our results suggest that Sowerby's beaked whale exhibits a metapopulation structure of two or more populations with limited movement of individuals between regions. However, genetic analysis is also needed to further explore whether these are distinct population segments or if this is a panmictic species with habitat preference among individuals and regional mixing for mating.
The Atlantic Ocean basin is a complex ecosystem, and environmental factors such as seasonal productivity, temperature, and ocean currents likely influence Sowerby's beaked whale spatial distribution. Future studies focused on exploring the nuances of these factors, and on evaluating how Sowerby's beaked whale isotope values align with seasonally changing Atlantic isoscapes, are needed. East Atlantic specimens are better represented than west Atlantic specimens in our dataset; this may be due to multiple oceanic currents in the west Atlantic acting to carry distressed animals and carcasses away from shore. For example, the Gulf Stream may be carrying specimens east and out to sea, resulting in less stranded carcasses in the west Atlantic. We do not think that west Atlantic carcasses are being carried to strand in the east Atlantic, as the level of decomposition in many strandings had not progressed sufficiently to suggest long-term drift and the isotopic data suggest that it is unlikely. Similarly, in the east Atlantic, the North Atlantic Drift Current may explain why Sowerby's beaked whales strand in the British Isles, particularly in Scotland, with such a high frequency as compared with other locations.
Conclusions
Our results provide critical data regarding spatial structuring in Sowerby's beaked whale populations, demonstrate the value of specimens of opportunity for conservation science, and illustrate the usefulness of stable isotope analysis for elusive species research. The methods we used can be applied to other beaked whales, providing much needed information about this enigmatic group of animals. Due to the paucity of data on beaked whales in general, analysis of specimens of opportunity for some species may be the only way to garner sufficient baseline data to reliably inform future research and conservation plans for beaked whales. For beaked whales assumed to have large distributions, stable isotope analysis of specimens of opportunity can provide an efficient and inexpensive means to test this assumption and thus provide insight into population units or regional fidelity among groups or individuals.
Specimens of opportunity are vital sources of biological information regarding elusive species, and stable isotope analysis is an efficacious means of quickly generating data to address ecological questions. The methods used in this study can be applied to an array of other marine or terrestrial animals, narrowing the knowledge gap for elusive species and aiding in the development of conservation plans. Museum and research institutions often store multiple tissues from specimens of opportunity, and with the increase in frozen tissue repositories, researchers have access to multiple temporal snapshots and can reconstruct short- and long-term foraging and movement behavior. Our results demonstrate the usefulness of these samples to elusive species research and provide a framework to apply these methods to other species.
Data Availability Statement
The original contributions presented in the study are included in the article/Supplementary Material, further inquiries can be directed to the corresponding author/s.
Author Contributions
KS, CT, and MP conceived the study. CF and JS advised on the study design. AB, MD, ND, GG, KK, AK, BL, VL, HM, JO, RS, ZT, GV, and FW contributed the samples. KS, CF, and JS processed the samples for analysis. KS and MP analyzed the data. KS led the writing of the manuscript. All authors contributed to the drafts and gave final approval for publication.
Funding
This work was supported by the National Museum of Natural History Peter Buck Fellowship Program, Smithsonian's Museum Conservation Institute Federal and Trust Funds, the Inter-university Training for Continental Scale Ecology (ITCE) program sponsored by the National Science Foundation (EF-1241286), and the University of Texas at El Paso.
Conflict of Interest
The authors declare that the research was conducted in the absence of any commercial or financial relationships that could be construed as a potential conflict of interest.
Acknowledgments
We thank Darrin Lunde, Charley Potter, James Mead, and Michael McGowen at the National Museum of Natural History, USA; Jerry Herman at the National Museums Scotland, UK; University Museum of Bergen, Norway; Mariel ten Doeschate at Scottish Marine Animal Stranding Scheme, which is supported by Marine Scotland and the Department for Environment, Food and Rural Affairs as part of the UK government's commitment to a number of international conservation agreements; Marine and Freshwater Research Institute, Iceland; Icelandic Institute of Natural History, Iceland; Halldór Gíslason at the Húsavík Whale Museum, Iceland; Natural History Museum Rotterdam, Netherlands; Friederike Johansson at Gothenburg Museum of Natural History, Sweden; Pepijn Kamminga at Naturalis Biodiversity Center, Netherlands; Morten Tange Olsen at the Natural History Museum of Denmark, Denmark; Donald McAlpine and Mary Sollows at the New Brunswick Museum, Canada; German Oceanographic Museum, Germany; Natural History Museum, UK; Canadian Museum of Nature, Canada; Northeast Fisheries Science Center, USA; and Yves Morin at the Fisheries and Oceans Canada, Canada. Kim Sparks of the Cornell Isotope Laboratory provided invaluable support and guidance. We also thank the editor (YN) and two reviewers for their helpful feedback and recommendations, which improved an earlier version of this manuscript.
Supplementary Material
The Supplementary Material for this article can be found online at: https://www.frontiersin.org/articles/10.3389/fcosc.2021.653766/full#supplementary-material
References
Aubin, D. J. S., Smith, T. G., and Geraci, J. R. (1990). Seasonal epidermal molt in beluga whales, Delphinapterus leucas. Can. J. Zool. 68, 359–367. doi: 10.1139/z90-051
Bahar, B., Moloney, A. P., Monahan, F. J., Harrison, S. M., Zazzo, A., Scrimgeour, C. M., et al. (2009). Turnover of carbon, nitrogen, and sulfur in bovine longissimus dorsi and psoas major muscles: implications for isotopic authentication of meat. J. Anim. Sci. 87, 905–913. doi: 10.2527/jas.2008-1360
Baker, C. S., Cooke, J. G., Lavery, S., Dalebout, M. L., Ma, Y.-U., Funahashi, N., et al. (2007). Estimating the number of whales entering trade using DNA profiling and capture-recapture analysis of market products. Mol. Ecol. 16, 2617–2626. doi: 10.1111/j.1365-294X.2007.03317.x
Ben-David, M., and Flaherty, E. A. (2012). Stable isotopes in mammalian research: a beginner's guide. J. Mammal. 93, 312–328. doi: 10.1644/11-MAMM-S-166.1
Breck, S. W. (2006). Sampling rare or elusive species: concepts, designs, and techniques for estimating population parameters, edited by William L. Thompson. Wildlife Society Bull. 34, 897–898. doi: 10.2193/0091-7648(2006)34897:BRROES2.0.CO
Charapata, P., Horstmann, L., Jannasch, A., and Misarti, N. (2018). A novel method to measure steroid hormone concentrations in walrus bone from archaeological, historical, and modern time periods using liquid chromatography/tandem mass spectrometry. Rapid Commun. Mass Spectrom. 32, 1999–2023. doi: 10.1002/rcm.8272
Chasar, L. C., Chanton, J. P., Koenig, C. C., and Coleman, F. C. (2005). Evaluating the effect of environmental disturbance on the trophic structure of Florida Bay, U.S.A.: multiple stable isotope analyses of contemporary and historical specimens. Limnol. Oceanogr. 50, 1059–1072. doi: 10.4319/lo.2005.50.4.1059
Clarke, B. (2008). Normal bone anatomy and physiology. Clin. J. Am. Soc. Nephrol. 3, S131–S139. doi: 10.2215/CJN.04151206
Coombs, E. J., Deaville, R., Sabin, R. C., Allan, L., O'Connell, M., Berrow, S., et al. (2019). What can cetacean stranding records tell us? A study of UK and Irish cetacean diversity over the past 100 years. Mar. Mamm. Sci. 35, 1527–1555. doi: 10.1111/mms.12610
COSEWIC. (2006). COSEWIC Assessment and Update Status Report on the Sowerby's Beaked Whale Mesoplodon Bidens in Canada. Ottawa, ON: CotSoEWi.
COSEWIC. (2019). COSEWIC Assessment and Status Report on the Sowerby's Beaked Whale Mesoplodon bidens in Canada. Ottawa, ON: CotSoEWi.
Cunningham, R. B., and Lindenmayer, D. B. (2005). Modeling count data of rare species: some statistical issues. Ecology 86, 1135–1142. doi: 10.1890/04-0589
Dalebout, M. L., Baker, C. S., Mead, J. G., Cockcroft, V. G., and Yamada, T. K. (2004). A comprehensive and validated molecular taxonomy of beaked whales, family Ziphiidae. J. Heredity 95, 459–473. doi: 10.1093/jhered/esh054
Dalebout, M. L., Baker, C. S., Steel, S., Thompson, K., Robertson, K. M., Chivers, S. J., et al. (2014). Resurrection of Mesoplodon hotaula Deraniyagala 1963: a new species of beaked whale in the tropical Indo-Pacific. Mar. Mamm. Sci. 30, 1081–1108. doi: 10.1111/mms.12113
Dalebout, M. L., Mead, J. G., Baker, C. S., Baker, A. N., and van Helden, A. L. (2002). A new species of beaked whale Mesoplodon Perrini sp. n. (cetacea: Ziphiidae) discovered through phylogenetic analyses of mitochondrial DNA sequences. Mar. Mamm. Sci. 18, 577–608. doi: 10.1111/j.1748-7692.2002.tb01061.x
Doi, T., Tozuka, T., and Yamagata, T. (2010). The atlantic meridional mode and its coupled variability with the guinea dome. J. Clim. 23, 455–475. doi: 10.1175/2009JCLI3198.1
Ebert, D. A., Akhilesh, K. V., and Weigmann, S. (2019). Planonasus indicus sp. n., a new species of pygmy false catshark (Chondrichthyes: Carcharhiniformes: Pseudotriakidae), with a revised diagnosis of the genus and key to the family. Mar. Biodivers. 49, 1321–1341. doi: 10.1007/s12526-018-0915-4
Ellis, R., Mead, J. G., Ellis, R., and Mead, J. G. (2017). Sowerby's Beaked Whale Mesoplodon Bidens (Sowerby, 1804). Baltimore, MD: Johns Hopkins Univ Press.
Erdmann, M. V. (1999). An account of the first living coelacanth known to scientists from Indonesian waters. Environ. Biol. Fishes 54, 439–443. doi: 10.1023/A:1007584227315
Feyrer, L. J., Zhao, S.t, Whitehead, H., and Matthews, C. J. D. (2020). Prolonged maternal investment in northern bottlenose whales alters our understanding of beaked whale reproductive life history. PLoS ONE 15:e0235114. doi: 10.1371/journal.pone.0235114
Fry, B., and Arnold, C. (1982). Rapid 13C/12C turnover during growth of brown shrimp (Penaeus aztecus). Oecologia 54, 200–204. doi: 10.1007/BF00378393
Green, R. H., and Young, R. C. (1993). Sampling to detect rare species. Ecol. Appl. 3, 351–356. doi: 10.2307/1941837
Hakkinen, S., and Rhines, P. B. (2009). Shifting surface currents in the northern North Atlantic Ocean. J. Geophys. Res. 114:12. doi: 10.1029/2008JC004883
Hicks, B. D., Staubin, D. J., Geraci, J. R., and Brown, W. R. (1985). Epidermal growth in the bottlenose dolphin, tursiops truncatus. J. Invest. Dermatol. 85, 60–63. doi: 10.1111/1523-1747.ep12275348
Hobson, K. A. (1999). Tracing origins and migration of wildlife using stable isotopes: a review. Oecologia 120, 314–326. doi: 10.1007/s004420050865
Holmes, M. W., Hammond, T. T., Wogan, G. O., Walsh, R. E, LaBarbera, K., Wommack, E. A., et al. (2016). Natural history collections as windows on evolutionary processes. Mol. Ecol. 25, 864–881. doi: 10.1111/mec.13529
Hopkins, J. B., and Ferguson, J. M. (2012). Estimating the diets of animals using stable isotopes and a comprehensive bayesian mixing model. PLoS ONE 7:e28478. doi: 10.1371/annotation/d222580b-4f36-4403-bb1f-cfd449a5ed74
Javornik, J., Hopkins, J. B. I. I. I, Zavadlav, S., Levanič, T, Lojen, S., Polak, T., et al. (2019). Effects of ethanol storage and lipids on stable isotope values in a large mammalian omnivore. J. Mammal. 100, 150–157. doi: 10.1093/jmammal/gyy187
Joseph, L. N., Field, S. A., Wilcox, C., and Possingham, H. P. (2006). Presence-absence versus abundance data for monitoring threatened species. Conserv. Biol. 20, 1679–1687. doi: 10.1111/j.15231739.2006.00529.x
Keeling, C. D. (1979). The suess effect: 13Carbon-14Carbon interrelations. Environ. Int. 2, 229–300. doi: 10.1016/0160-4120(79)90005-9
Landrau-Giovannetti, N., Subramaniam, K., Brown, M. A., Ng, T. F. F., Rotstein, D. S., West, K., et al. (2020). Genomic characterization of a novel circovirus from a stranded Longman's beaked whale (Indopacetus pacificus). Virus Res. 277:197826. doi: 10.1016/j.virusres.2019.197826
Lorrain, A., Pethybridge, H., Cassar, N., Receveur, A., Allain, V., Bodin, N., et al. (2020). Trends in tuna carbon isotopes suggest global changes in pelagic phytoplankton communities. Global Change Biol. 26, 458–470. doi: 10.1111/gcb.14858
MacKenzie, K. M., Palmer, M. R., Moore, A., Ibbotson, A. T., Beaumont, W. R. C., Poulter, D. J. S., et al. (2011). Locations of marine animals revealed by carbon isotopes. Sci. Rep. 1:21. doi: 10.1038/srep00021
MacLean, H. J., Nielsen, M. E., Kingsolver, J. G., and Buckley, L. B. (2019). Using museum specimens to track morphological shifts through climate change. Philos. Trans. R. Soc. B 374:20170404. doi: 10.1098/rstb.2017.0404
MacLeod, C. D., Errin, W. F. P., Pitman, R. L., Barlow, J., Ballance, L. T., Gerrodette, T., et al. (2005). Known and inferred distributions of beaked whale species (Cetacea: Ziphiidae). J. Cetacean Res. Manage. 7, 271–286.
Magozzi, S., Yool, A., Vander Zanden, H. B., Wunder, M. B., and Trueman, C. N. (2017). Using ocean models to predict spatial and temporal variation in marine carbon isotopes. Ecosphere 8:e01763. doi: 10.1002/ecs2.1763
McKechnie, A. E. (2004). Stable isotopes: powerful new tools for animal ecologists. South Afr. J. Sci. 100, 131–134.
McSweeney, D. J., Baird, R. W., and Mahaffy, S. D. (2007). Site fidelity, associations, and movements of Cuvier's (Ziphius cavirostris) and Blainville's (Mesoplodon densirostris) beaked whales off the island of Hawai'i. Mar. Mamm. Sci. 23, 666–687. doi: 10.1111/j.1748-7692.2007.00135.x
Mead, J. G. (2009). “Beaked whales, overview: Ziphiidae,” in Encyclopedia of Marine Mammals (Second Edition) eds W. F. Perrin, B. Würsig, and J. G. M. Thewissen JGM (London: Academic Press), 94–97. doi: 10.1016/B978-0-12-373553-9.00027-4
Meek, P. D., Ballard, G. A., Fleming, P. J. S., Schaefer, M., Williams, W., and Falzon, G. (2014). Camera traps can be heard and seen by animals. PLoS ONE 9:e110832. doi: 10.1371/journal.pone.0110832
Newbold, T. (2010). Applications and limitations of museum data for conservation and ecology, with particular attention to species distribution models. Prog. Phys. Geography. 34, 3–22. doi: 10.1177/0309133309355630
Newsome, S. D., Clementz, M. T., and Koch, P. L. (2010). Using stable isotope biogeochemistry to study marine mammal ecology. Mar. Mamm. Sci. 26, 509–572. doi: 10.1111/j.1748-7692.2009.00354.x
Pereira, J. N., Neves, V. C., Prieto, R., Silva, M. A., Cascão, I., Oliveira, C., et al. (2011). Diet of mid-Atlantic Sowerby's beaked whales Mesoplondon bidens. Deep-Sea Res. Part I-Oceanographic Res. Papers 58, 1084–1090. doi: 10.1016/j.dsr.2011.08.004
Phillips, D. L., and Eldridge, P. M. (2006). Estimating the timing of diet shifts using stable isotopes. Oecologia 147, 195–203. doi: 10.1007/s00442-005-0292-0
Piggott, M. P., and Taylor, A. C. (2003). Remote collection of animal DNA and its applications in conservation management and understanding the population biology of rare and cryptic species. Wildlife Re. 30, 1–13. doi: 10.1071/W.R.02077
R Core Team (2018). R: A Language and Environment for Statistical Computing. Vienna: R Foundation for Statistical Computing.
Reverdin, G., Niiler, P. P., and Valdimarsson, H. (2003). North Atlantic Ocean surface currents. J. Geophys. Res. 108, 2-1-2-21. doi: 10.1029/2001JC001020
Roberts, D. L., Taylor, L., and Joppa, L. N. (2016). Threatened or data deficient: assessing the conservation status of poorly known species. Divers. Distribut. 22, 558–565. doi: 10.1111/ddi.12418
Sarakinos, H. C., Johnson, M. L., and Zanden, M. J. V. (2002). A synthesis of tissue-preservation effects on carbon and nitrogen stable isotope signatures. Can. J. Zool. 80, 381–387. doi: 10.1139/z02-007
Schimmelmann, A., Albertino, A., Sauer, P. E., Qi, H., Molinie, R., and Mesnard, F. (2009). Nicotine, acetanilide and urea multi-level 2H-, 13C- and 15N-abundance reference materials for continuous-flow isotope ratio mass spectrometry. Rapid Commun. Mass Spectrom. 23, 3513–3521. doi: 10.1002/rcm.4277
Schwartz, A. L. W., Shilling, F. M., and Perkins, S. E. (2020). The value of monitoring wildlife roadkill. Europ. J. Wildlife Res. 66:12. doi: 10.1007/s10344-019-1357-4
Smith, K. J., Mead, J. G., and Peterson, M. J. (2021). Specimens of opportunity provide vital information for research and conservation regarding elusive whale species. Environ. Conserv. 48, 84–92. doi: 10.1017/S0376892920000521
Smith, K. J., Sparks, J. P., Timmons, Z. L., and Peterson, M. J. (2020). Cetacean skeletons demonstrate ecologically relevant variation in intraskeletal stable isotopic values. Front. Mar. Sci. 7:388. doi: 10.3389/fmars.2020.00388
Sonnerup, R. E., Quay, P. D., McNichol, A. P., Bullister, J. L., Westby, T. A., and Anderson, H. L. (1999). Reconstructing the oceanic 13C Suess Effect. Global Biogeochem. Cycles 13, 857–872. doi: 10.1029/1999GB900027
Sowerby, J. (1804). The British Miscellany, or, Coloured Figures of New, Rare, or Little Known Animal Subjects: Many Not Before Ascertained to be Inhabitants of the British Isles: and Chiefly in the Possession of the Author, James Sowerby. London: R. Taylor & Co. doi: 10.5962/bhl.title.120056
Spitz, J., Cherel, Y., Bertin, S., Kiszka, J., Dewez, A., and Ridoux, V. (2011). Prey preferences among the community of deep-diving odontocetes from the Bay of Biscay, Northeast Atlantic. Deep Sea Res. I Oceanographic Res. Papers 58:273–282. doi: 10.1016/j.dsr.2010.12.009
Taylor, B. L., Baird, R., Barlow, J., Dawson, S. M., Ford, J., Mead, J. G., et al. (2008). Mesoplodon Bidens. The IUCN Red List of Threatened Species. Available online at: www.iucnredlist.org (accessed November 18, 2020).
Trueman, C. N., Jackson, A. L., Chadwick, K. S., Coombs, E. J., Feyrer, L. J., Magozzi, S., et al. (2019). Combining simulation modeling and stable isotope analyses to reconstruct the last known movements of one of Nature's giants. PeerJ 7:e7912. doi: 10.7717/peerj.7912
Trueman, C. N., and St John Glew, K. (2019). “Isotopic tracking of marine animal movement,” in Tracking Animal Migration with Stable Isotopes (Second Edition), eds K. A. Hobson and L. I. Wassenaar (Academic Press), 137–172. doi: 10.1016/B978-0-12-814723-8.00006-4
van Helden, A. L., Baker, A. N., Dalebout, M. L., Reyes, J. C., Waerebeek, K. V., and Baker, C. S. (2002). Resurrection of Mesoplodon Traversii (Gray, 1874), senior synonym of M. Bahamondi Reyes, Van Waerebeek, Cárdenas and Yáñez, (1995). (Cetacea: Ziphiidae). Mari. Mam. Sci. 18, 609–621. doi: 10.1111/j.1748-7692.2002.tb01062.x
Vander Zanden, H. B., Nelson, D. M., Wunder, M. B., Conkling, T. J., and Katzner, T. (2018). Application of isoscapes to determine geographic origin of terrestrial wildlife for conservation and management. Biol. Conserv. 228, 268–280. doi: 10.1016/j.biocon.2018.10.019
Vander Zanden, M. J., Clayton, M. K., Moody, E. K., Solomon, C. T., and Weidel, B. C. (2015). Stable isotope turnover and half-life in animal tissues: a literature synthesis. PLoS ONE 10:e0116182. doi: 10.1371/journal.pone.0116182
Wenzel, F. W., Polloni, P. T., Craddock, J. E., Gannon, D. P., Nicolas, J. R., Read, A. J., et al. (2013). Food habits of Sowerby's beaked whales (Mesoplodon bidens) taken in the pelagic drift gillnet fishery of the western North Atlantic. Fishery Bull. 44, 381–389. doi: 10.7755/FB.111.4.7
West, J. B., Bowen, G. J., Cerling, T. E., and Ehleringer, J. R. (2006). Stable isotopes as one of nature's ecological recorders. Trends Ecol. Evolut. 21, 408–414. doi: 10.1016/j.tree.2006.04.002
Williams, T., Robin, C., Dunkin, P., Yochem, J., McBain, K., Fox-Dobbs, H., Mostman-Liwanag, et al. (2008). Assessing stable isotope signature variation in cetaceans: an evaluation of skin sampling techniques and correlations with diet for bottlenose dolphins and killer whales. NWFSC Contract Report. doi: 10.1017/CBO9781107415324.004
Yamada, T. K., Kitamura, S., Abe, S., Tajima, Y., Matsuda, A., Mead, J. G., et al. (2019). Description of a new species of beaked whale (Berardius) found in the North Pacific. Sci. Rep. 9:14. doi: 10.1038/s41598-019-46703-w
Keywords: bone, muscle, skin, Sowerby's beaked whale, Mesoplodon bidens, δ13 C and δ15 N, museum collections
Citation: Smith KJ, Trueman CN, France CAM, Sparks JP, Brownlow AC, Dähne M, Davison NJ, Guðmundsson G, Khidas K, Kitchener AC, Langeveld BW, Lesage V, Meijer HJM, Ososky JJ, Sabin RC, Timmons ZL, Víkingsson GA, Wenzel FW and Peterson MJ (2021) Stable Isotope Analysis of Specimens of Opportunity Reveals Ocean-Scale Site Fidelity in an Elusive Whale Species. Front. Conserv. Sci. 2:653766. doi: 10.3389/fcosc.2021.653766
Received: 15 January 2021; Accepted: 16 April 2021;
Published: 25 May 2021.
Edited by:
Yonggang Nie, Chinese Academy of Sciences (CAS), ChinaReviewed by:
Amy Wallace, University of Florida, United StatesHan Han, China West Normal University, China
Copyright © 2021 Smith, Trueman, France, Sparks, Brownlow, Dähne, Davison, Guðmundsson, Khidas, Kitchener, Langeveld, Lesage, Meijer, Ososky, Sabin, Timmons, Víkingsson, Wenzel and Peterson. This is an open-access article distributed under the terms of the Creative Commons Attribution License (CC BY). The use, distribution or reproduction in other forums is permitted, provided the original author(s) and the copyright owner(s) are credited and that the original publication in this journal is cited, in accordance with accepted academic practice. No use, distribution or reproduction is permitted which does not comply with these terms.
*Correspondence: Kerri J. Smith, c21pdGhrZXJyaWomI3gwMDA0MDtnbWFpbC5jb20=