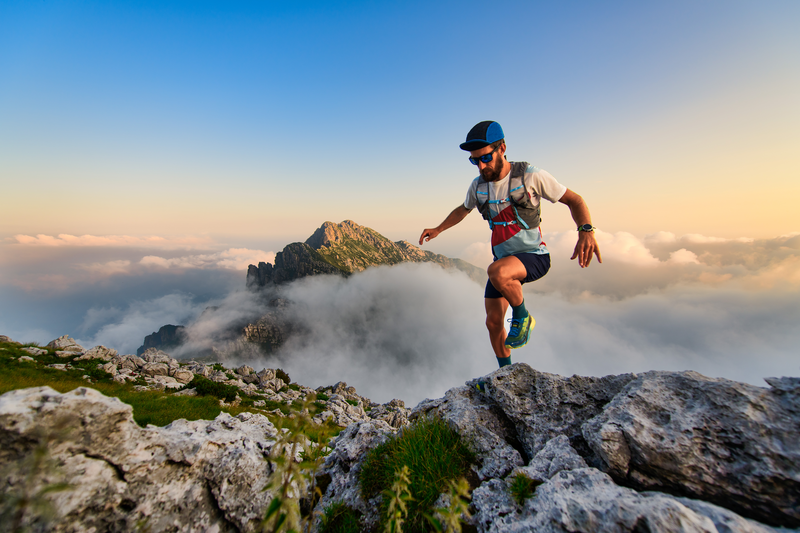
94% of researchers rate our articles as excellent or good
Learn more about the work of our research integrity team to safeguard the quality of each article we publish.
Find out more
ORIGINAL RESEARCH article
Front. Conserv. Sci. , 14 April 2021
Sec. Human-Wildlife Interactions
Volume 2 - 2021 | https://doi.org/10.3389/fcosc.2021.652415
This article is part of the Research Topic Frontiers in Conservation Science – Highlights From Its First Year. View all 10 articles
Agriculture is an essential production system used to feed the growing human population, but at the same time has become a major driver of biodiversity loss and environmental degradation. Employing production methods that restore degraded landscapes can have a positive impact on biodiversity, whilst improving food production. We assessed how mammalian biodiversity, specifically richness and their relative abundances varied on five Karoo farms in South Africa that had been amalgamated and subjected to a transition from traditional livestock grazing techniques (sporadic rotational grazing and lethal predator control) to wildlife-friendly non-lethal predator management, using human shepherding of livestock under a high-density short-duration grazing regime. We used camera trap data collected over a 4-year period, to measure mammalian species richness, distribution and relative abundance on the wildlife-friendly farm to investigate temporal changes throughout the conversion from traditional farming practices. In the last year of the study (2019) additional cameras were used to provide a spatial comparison of mammalian species on the wildlife-friendly farm to two neighboring farms, a traditional livestock farm using lethal predator controls, and a game farm. We found that mammalian species richness increased year on year resulting in a significant increase of 24% over the duration of the study. Herbivores showed an increase of 33% in the number of species detected over the years, while predator species increased by 8%. The relative abundance and distribution of most species also showed increases as the conversion process took place. For example, 73% of the herbivore species detected throughout the study increased in their relative abundance. Similarly, 67% of all species showed an increase in the number of sites occupied over the years. In the final year of the study the wildlife-friendly farm had more mammalian species compared to the game farm and traditional livestock farm, with the latter two sites having a similar number of species when compared to the commencement of the conversion of the wildlife-friendly site. These broad improvements in mammalian biodiversity demonstrate that livestock production can benefit local mammalian biodiversity through a combination of herder grazing management and wildlife-friendly farming.
Conserving and restoring biodiversity has become a rising priority globally. Over the last two decades, ambitious targets set by the United Nations Convention on Biological Diversity (CBD) to curb the rate of biodiversity loss have failed (Butchart et al., 2010; Kok et al., 2018). While society increasingly realizes the value of biodiversity to human well-being (Hill et al., 2015), responses have been insufficient in achieving short-term goals and cast doubt on the ability of society to achieve the long-term goals of the CBD. These ongoing losses of biodiversity are predicted to result in ecosystem collapse by the turn of the century (Díaz et al., 2019; IPBES, 2019). Natural ecosystems around the world are shrinking, and with approximately a third of the world's land surface used for agriculture; either livestock or crop production (UN Food Agriculture Organization, 2017), these production lands and their impact on biodiversity is becoming increasingly important to conservation efforts (Kok et al., 2018). In South Africa, 69% of the land surface is reported to be suitable for livestock farming (Goldblatt, 2010), making this sector an important component of efforts to promote biodiversity conservation regionally.
However, agricultural landscapes are notorious for negative impacts on wildlife and ecosystems, and are attributed to causing the largest impact on terrestrial biodiversity loss (Kok et al., 2018). Livestock farming has been shown to negatively affect animal diversity (Filazzola et al., 2020), plant biodiversity (Nenzhelele et al., 2018), primary productivity, carbon sequestration, soil erosion, and water retention (du Toit et al., 2009, Stavi et al., 2016). In South Africa, livestock production practices have degraded landscapes through poor grazing practices (Milton et al., 1994; Hoffman, 2014), hunting and trapping to control predators and competing wildlife (Nattrass and Conradie, 2015) and erecting fences to control livestock movements (Roche, 2004; Dean et al., 2018). Apex predators that once roamed vast areas, including lions (Panthera leo), cheetah (Acinonyx jubatus), and spotted hyena (Crocuta crocuta), have long been extirpated from the landscape, leaving only the leopard (Panthera pardus), and meso-predators such as black-backed jackal (Canis mesomelas) and caracal (Caracal caracal) as the last major free-roaming mammalian predators in South Africa. Furthermore, the historical herds of ungulates that occasionally migrated across the Karoo in their millions have also been removed through hunting and fencing (Roche, 2004; Harris et al., 2009). The long-term implications of extensive networks of fences are far reaching (Dean et al., 2018); they cut off vital migratory routes of animals, restricting dispersal patterns and genetic exchange (Hayward and Kerley, 2009) and continue to cause mortalities of faunal species, through entanglement or electrocution (Boone and Hobbs, 2004). These migrations would have played a vital role in nutrient cycles and healthy soils which ensured landscapes remained resilient during harsh climatic conditions. These compounding factors, together with hunting and lethal predator controls and associated by-catch, have resulted in brittle ecosystems in semi-arid regions which are more vulnerable to prolonged droughts and changing climate conditions.
Changing how we manage livestock rangelands to increase biodiversity and ecological function could have a profound impact on global ecological health. Alternative rangeland management strategies, such as using traditional grazing regimes, have been shown to increase biodiversity and ecosystem functioning (Mu et al., 2016) as opposed to commercial livestock farming. Using livestock to mimic the effect of historical mass migrations of ungulates can aid in restoring degraded landscapes (Savory and Parsons, 1980, Esler et al., 2010; McManus et al., 2018). These techniques have shown some positive effects on veld (uncultivated open scrub or grassland) production, particularly in degraded semi-arid landscapes, as they allow for longer resting and veld recovery periods and more nutritious grazing (McManus et al., 2018). Furthermore, the constant attendance of human shepherds who oversee livestock, manage herd health concerns, and direct their grazing routes, reduces predation threats and the need for fencing on rangelands. These impacts should reduce human-wildlife conflict as livestock are protected and rangelands are allowed time to recover in semi-arid landscapes and thus offer improved forage for both livestock and wildlife (McManus et al., 2018). The removal of fences facilitates the reconnection of previously isolated animal populations as species can disperse further, which will aid genetic resilience to disease and effects related to climate change (Woodroffe et al., 2014). Crucial to these efforts is the shift from indiscriminate lethal control methods aimed at eradicating predators to mitigation via non-lethal methods, focusing instead on livestock management such as human shepherding, kraaling (enclosing at night in pens) or using guardian animals (Hazzah et al., 2014, McManus et al., 2014; Van Eeden et al., 2017).
The semi-arid Karoo in South Africa is an ecologically sensitive region notorious for slow vegetation recovery rates of degraded landscapes (Seymour et al., 2010), and a key region for livestock production in the country. We use the conversion of the five amalgamated farms as a case study to determine if human shepherding of livestock under a high-density, short duration grazing regime, combined with wildlife coexistence and fence removal, and a cessation of hunting wildlife could play a positive role in ecosystem restoration, i.e., reversing land degradation, improving the quantity and quality of palatable grasses (Savory and Parsons, 1980) and improving biodiversity on livestock production landscapes (Landmark Foundation, 2017). Human shepherding also provides additional benefits for local economic development and employment, whilst improving herd health and productivity. These latter effects were not however evaluated in this study but were concurrent benefits.
We expected that changes at all levels of biodiversity, would occur as a result of the conversion to wildlife-friendly management practices. Although data was collected on the biodiversity of birds, insects and plants we elected initially to focus on the changes observed in mammalian species since a number of the new management practices (stopping all hunting and trapping, and fence removals) would directly impact these species. Mammals would additionally be less impacted by severe short term climatic conditions, such as droughts compared to birds and insects. Results of the changes of biodiversity of birds, and plants are being prepared for separate publication.
Three complementary metrics were chosen to document changes in mammalian biodiversity: (1) species richness was chosen as the most basic indicator of mammalian biodiversity of the local environment (Duelli and Obrist, 2003) and was predicted to increase during conversion, (2) species distribution was chosen to get a measure of whether changes in species richness were also reflected in the distribution of species in response to removal of fencing constraints and the concentration of livestock and associated human activity to only a small proportion of the farm at any on time as a result of moving to shepherding, (3) relative abundance index (RAI) of a species was chosen to provide an indication of the size of the population of each mammalian species.
In this study, we evaluated the mammalian biodiversity changes (mammalian species richness, distribution, and abundance) that took place over a 4-year period on a Karoo farm that was undergoing a transition from a traditional farming practice to wildlife-friendly farming. This Karoo farm was an amalgamation of five individual farms into a single management unit. The extensive size of this farm was an attempt to mimic the effect of the adoption of non-lethal shepherding at a landscape level and to limit edge-effects on the management treatment introduced, since it was embedded in a landscape dominated by lethal control. Mammalian species richness was also used to evaluate the biodiversity associated with three different land uses; (1) the wildlife-friendly system, (2) a traditional commercial livestock production strategy, and (3) a fenced game farm. The comparison across land uses was evaluated at a single point in time, when the conversion to wildlife friendly farming was completed on the experimental site. We predicted that after 4 years of wildlife-friendly conversion and management, the wildlife-friendly farm would have a higher mammalian species richness (number of species), higher mammalian species distribution (spatial extent), and would have seen an increase in abundance within mammalian species (frequency of occurrence). We predicted that this would hold true when compared to either the traditional livestock farm or the game farm.
The study was conducted on the Nuweveld Mountain range, ~45 km north east of Beaufort West in South Africa, between 2015 and 2019 (Figure 1). Three sites were compared; the first site is a wildlife-friendly livestock farm (22,111 ha). The second site lies on the northern boundary and is a game farm that excludes domestic livestock (5,435 ha), and the third site is a traditional livestock farm (11,244 ha) ~20 km to the north of the wildlife-friendly farm (Figure 1).
Figure 1. Location of the three study sites, which included the wildlife-friendly farm (black), a game farm (red), and a traditional livestock farm (blue), on a hillshade projection of the Nuweveld Mountain range (Van Niekerk, 2015). Camera stations retained in the analysis are indicated by black dots. The inset shows the location of the study region (green) within South Africa.
The study region falls within the semi-arid Nama Karoo biome and includes two dominant vegetation types: the Upper Karoo Hardeveld and the Eastern Upper Karoo (Mucina et al., 2006). The Upper Karoo Hardeveld is typically present on steep, hilly topography covered with large boulders, and comprises sparse dwarf Karoo scrub with drought-tolerant grasses. The Eastern Upper Karoo is present on flat or gently-sloping landscapes and characterized by a higher proportion of grasses among the low shrubs (Mucina et al., 2006).
The region is in the summer rainfall zone classification (Roffe et al., 2019) and receives an average annual rainfall of 270 mm, with peak rainfall occurring from October to April. During the 4-year study period a drought resulted in stochastic rainfall events, with lower-than-average rainfall measured on the wildlife-friendly farm of 186 mm per year (2016: 235.5 mm, 2017: 303 mm, 2018: 66 mm, 2019: 138 mm). Topographically the region varies from flat open plains to steep riverine gullies (Figure 1). The altitude above sea-level varies across the three sites from ~1,100 m in the lower lying ravines to 1,700 m at the highest peaks.
Prior to mid-2015 the individual farms (that later became the wildlife-friendly farm) were managed in a traditional rotational grazing system and employed lethal control methods such as leg-hold traps (gin-traps), jackal-proof fencing and hunting (inclusive of night-time call and shoot hunting, and helicopter hunting), to limit the numbers of predators and damage causing animals, mainly black-backed jackal, caracal and chacma baboon (Papio ursinus), as well as other non-target species. Since July 2015 livestock (sheep and cattle) farming, as well as the ecological restoration of the consolidated properties, were considered as complimentary management goals. This conversion has entailed using (1) high-density, short duration grazing with a single herd of sheep and cattle and night-time kraaling of sheep and cattle using human shepherds, (2) non-lethal predator management, and (3) no hunting of wildlife, and (4) a cumulative removal of internal farm fencing allowing wildlife migrations.
The first phase of the management conversion started in July 2015 when all lethal predator control ceased, and the farm continued to operate under conventional rotational paddocking. The livestock were monitored sporadically by direct observation from a suitable distance that would not influence the livestock or wild animal behavior. All deaths and their associated cause were recorded. Since January 2017 the livestock where herded throughout the day by human shepherds employing a high-density, short duration grazing technique. Additionally, the shepherds protect the livestock at night by using mobile kraal sites (as part of non-lethal livestock protection from carnivores). These kraal sites are relocated every 7 days. Kraal sites were placed on severely degraded locations and the dung, urine and hoof actions were rehabilitative on these sites. Shepherds maintain 24-h contact with the livestock 365 days per year which allows for herd-health interventions as well as preventing livestock depredation (McManus et al., 2018). In the 4 years of this management practice the number of adult sheep fluctuated between 1,500 and 1,650, while cattle varied between 200 and 350 (they were incorporated into the herd in May 2017). The livestock are checked and counted daily as they are released from the kraals, allowing heath issues to be attended to as well as deaths to be identified. Since January 2017 (the start of shepherding) only one lamb has been lost to predation, compared with 116 (12.3% of the herd) predation losses during 2016. Lamb survival at weaning also improved from 70 to 100%. These production and financial effects are part of other research projects.
Beginning in 2017 internal fences were sporadically and ongoingly removed from the wildlife-friendly farm. Due to labor constraints, key fence lines were targeted for initial removal, followed by short sections in strategic locations. This allowed the landscape to be efficiently opened up. Gates were also opened between paddocks and along roads (except a few that were still used to contain bulls and rams in non-breeding seasons). By the completion of the study a total of 28 km, out of a total 230 km, had been removed, allowing free migration of wildlife throughout the farm.
The traditional livestock farm was stocked with 1,800 sheep resulting in a carrying capacity of 0.16 livestock units per hectare. The rainfall was 127 mm in 2019, and historically has experienced the same rainfall regime as the wildlife-friendly farm as it is only 10 km away and is at the same altitude. It utilizes rotational paddocking, where the livestock are moved from one camp to another at one−3 months intervals, depending on veld conditions. Livestock roamed freely in paddocks ranging between 200 and 600 hectares in size, and lethal methods are used to reduce predator numbers. Predator controls include hunting via night-time call and shoot culling, helicopter predator culls, as well as trapping with cages, leg-hold traps and neck-snapping traps. The use of poison and hunting dogs on the property is denied. The total annual lamb losses for 2019 (due to predation, health issues, still birth etc.) amounted to 276 lambs. Both the outer boundary and internal fences are strictly maintained, and most are jackal proof (wire mesh up to 1.2 m high). Gates are typically kept closed to ensure livestock are kept in specific paddocks. This farming enterprise was located in a region of extensive livestock farming utilizing similar paddock rotations, lethal controls, and jackal proof fencing.
The game farm was converted from a traditional livestock farm in 1997. It is fully enclosed by a 2.2 m high non-electrified game fence which is jackal proof, as regulated by the provincial conservation authorities to contain all herbivores on the farm, allowing game ownership in South African laws. No lethal predator control reportedly takes place, but the farm is subject to very occasional game hunting. The game farm is well-established and is situated adjacent to a similarly managed game farm of twice its spatial extent.
Since its inception, Cape mountain zebra (Equus zebra zebra), eland (Taurotragus oryx), gray rhebok (Pelea capreolus), red hartebeest (Alcelaphus buselaphus caama), blue wildebeest (Connochaetes taurinus), waterbuck (Kobus ellipsiprymnus), mountain reedbuck (Redunca fulvorufula), and giraffe (Giraffa camelopardalis giraffa) were all introduced by 2017, thus the site contains indigenous and extralimital species. There are approximately 800 total game animals, with their numbers managed through live sales (~50 individuals per year are removed), and the occasional introduction of new individuals and species (S. Lovemore, personal communication, 25 November 2020). There has been no direct evidence of predation on any game species. However, the game species are only occasionally monitored so predation events may be missed. In 2019 they also received a lower than average rainfall for the region of 170 mm, and would have had historically experienced the same rainfall regime as the farm is immediately adjacent to the wildlife-friendly farm and at the same altitude.
We deployed 48 camera stations across the three sites (Supplementary Table 1) to detect terrestrial mammals. We used three different camera types; Cuddeback™ AttackIR, Cuddeback™ E2 (Wisconsin, 115 USA; www.cuddeback.com), and Bushnell® 119436 Trophy Cameras (Overland Park, KS, USA; www.bushnell.com). Each camera is triggered by passive infrared sensors and were set to 3-min intervals between triggers. All night-time images were taken with an infrared flash. Twenty-nine camera stations (762 ha per camera) were active on the wildlife-friendly farm since late 2015. These cameras operated throughout the 4-year study period to enable temporal variations within the wildlife-friendly study site to be evaluated as the conversion to new management practices unfolded. Two cameras were removed from the study due to malfunctions. Access to the traditional livestock and game farms, and camera resources, dictated that camera stations could only be deployed on the comparison sites in 2019, which was to evaluate the comparison after the wildlife friendly conversion was completed on the experimental farm. We placed thirteen camera stations (865 ha per camera) on the traditional livestock farm, and six (906 ha per camera) on the game farm. Camera stations were placed with the aim to cover all areas and habitat types across the three farms in order to capture the full mammalian biodiversity of the farms. The straight-line distance between a camera station and its nearest neighbor averaged 1,834 m (range 591–3,834 m, Figure 1) on the wildlife-friendly farm, 2,333 m on the traditional livestock farm (1,754–3,134 m) and 1,321 m on the game farm (894–2,161 m). All camera stations consisted of a single camera fixed ~40 cm from the ground at locations where evidence of diverse animal species had been found (e.g., spoor), or was expected to occur, such as along low usage gravel roads, game trails and ephemeral river courses.
We used four study periods running for 6 months each year (July–December) to assess the mammalian species richness, distribution, and RAI of species during the surveyed years (2016–2019). The same 6-month period was analyzed for all three study sites in 2019. These periods were chosen to allow the management changes (initiated prior to, or at the start of years) to have an impact on the wildlife-friendly farm, and to ensure independence between measures. Camera images were processed using digiKam, version 5.9.0 (www.digikam.org). Camera station performance (camera effort) was assessed using the camtrapR package (Niedballa et al., 2016) run in the R statistical software version 3.6.2 (R Core Team, 2017). Cameras stations with a camera effort of <90 days were removed from the analysis to ensure adequate sampling took place. The mean (±SE) camera effort was 170.8 ± 3.0 days for the wildlife friendly farm (over the 4 study periods), 153.9 ± 10.6 days for the traditional farm, and 99.0 ± 6.5 days for the game farm. To ensure independence of photographic events, additional photographs of the same species captured within a 60-min timeframe were excluded from the analyses (Tobler et al., 2008). All domestic species, human and vehicle records were excluded.
The three measures used to evaluate changes to the mammalian population within the wildlife-friendly site were (1) species richness, a measure of the number of species observed at a camera station, (2) the distribution of a species being the number of camera stations within a study site where it was detected, and (3) RAI which is a measure of a species' capture frequency (i.e., number of independent captures of a species divided by the camera station effort) at each camera station (Wearn and Glover-Kapfer, 2017). The RAIs were standardized to 183 active capture nights (the 6-month survey period) to allow for reliable comparisons. Whilst the RAI is susceptible to various biases (behavioral activity being the most prominent), and it does not include species detection probability in the formulation (e.g., Sollmann, 2018), it contributes to understanding the community assemblage in relation to commonness and rarity of different species. Furthermore, this index is the best alternative when it is not possible to identify individuals (e.g., Wearn and Glover-Kapfer, 2017; Pardo et al., 2018). RAI values derived from large scale camera trapping have been shown to correlate strongly with independent density estimates for a range of species (Palmer et al., 2018). Variations in species richness over time at the wildlife-friendly farm were evaluated by a repeated measures ANOVA and paired-T test analysis using the rstatix package (Kassambara, 2020). Species accumulation curves were calculated using the iNEXT package (Hsieh et al., 2016). Only species richness and composition were compared between the three study sites due to no repeat measures during different years on the two comparison sites.
The detected species were grouped according to their foraging guild, either herbivore or predator. Baboons were placed with the predators due to their predatory role on livestock farms (killing lambs; Muriuki et al., 2017). The detected species were additionally grouped into two weight categories calculated from Stuart and Stuart (2015); herbivores with an average mass below 18 kg were classed as small and above as large. Predators below 5 kg were classed as small and above as medium.
We retained data from 16 camera stations on the wildlife-friendly farm site (n = 16). These were active for a total of 10,932 camera trap nights, ranging from 2,619 to 2,806 nights per year (Supplementary Table 1) and captured a total 10,206 independent mammalian species events. Data from ten camera stations (n = 10) at the traditional farm was retained in 2019, for a total 1,539 camera trap nights, capturing 1,372 independent records. The game farm retained only three camera stations (n = 3) tallying 297 camera trap nights, and capturing 695 independent records. Each 6-month analysis period, at all three sites, reached an asymptote for total species richness detected indicating that the sampling effort was adequate. Rainfall was measured on the wildlife-friendly study site and totalled for the year. During the period from 2016 to 2019 the total yearly rainfall decreased by 42% indicating the presence of a worsening drought. Rainfall values at each camera location were not measured and so no direct analysis of the local effects of rainfall on species richness or distribution could be made.
The species accumulation curves for the wildlife-friendly farm (Figure 2) indicate that species richness had reached an asymptote for each year, despite differing number of capture events, indicating that these are reliable measures of species richness and that they represent a steady improvement in species richness over the 4-year study at the wildlife-friendly farm.
The number of observed species at the wildlife-friendly farm increased year on year from 28 in 2016 to 30 in 2017, 32 in 2018 and 34 species in 2019 (Figure 3, Table 1). The total number of species detected over the entire study period, increased by nine species (24%) to a total of 37 species, of which 24 were consistently detected in all four study periods (Table 1). Only two species which were present in the 2016 survey, meerkat (Suricata suricatta) and Cape clawless otter (Aonyx capensis), were not detected in 2019. The pattern of year on year increasing species richness held for herbivores and for predators (Figure 3), although the slope was steeper for herbivores (1.6, R2 = 0.98, p < 0.01) than predators (0.4, R2 = 0.80, p = 0.1), and it held for all size classes except medium predators which remained the same.
Figure 3. Species richness detected on the wildlife-friendly farm per sample period and broken down by guild and body size.
Table 1. Species detected per sample period (July to December of each year) on the wildlife-friendly farm.
Over the 4 years of the study the species richness on the wildlife-friendly site was found to vary significantly [ANOVA, F(3/45) = 12.52, p < 0.0001]. There is a clear indication of an increased species richness each year between 2016 and 2019 (Figure 4), with 2019 having significantly more species than 2016 (p < 0.001), 2017 (p < 0.01) and 2018 (p < 0.05). However, there was no significant difference detected between the years 2016 and 2017 or 2017 and 2018.
Figure 4. Species richness (SR) detected over four consecutive years from the 16 camera sites retained on the wildlife-friendly farm. The median value, interquartile range and range are shown for each year. The letters above each plot indicate significant differences (ns-not significant, *'s indicate the degree of significance).
There was a general increase in the number of camera stations where a given species were detected. In total, 68% of species (25 of 37) were detected at more camera stations in 2019 than in 2016 (Table 2). Six species were detected at the same number of camera stations and six were present on fewer camera stations. The largest increases were seen with blue wildebeest and gemsbok (Oryx gazella), which were both captured at seven new camera stations. Other large increases were seen with ungulate species, namely, red hartebeest, waterbuck, common duiker (Sylvicapra grimmia), eland and plains zebra (Equus quagga). However, kudu (Tragelaphus strepsiceros) presented a slight decrease and was seen on one fewer camera station in 2019 compared to 2016. African wildcat (Felis lybica) exhibited the largest increase in distribution (three additional camera stations) in the predator guild. The larger of the predators detected on the farm, black-backed jackal and caracal, had smaller increases (two and one additional camera station, respectively) in their detections. Herbivores show the most notable improvements, with 16 out of 21 (76%) species appearing at more stations, compared to 9 out of 16 (56%) predator species showing increased distribution.
Table 2. Number of cameras at which each species was detected, ordered by absolute (Δ) increase and then percentage increase.
The RAI values for the common species detected in every year (Table 3) indicates that 67% (16 of 24) increased their relative abundance. Ten species more than doubled their RAI (blue wildebeest, eland, water mongoose (Atilax paludinosus), blesbok (Damaliscus pygargus phillipsi), red hartebeest, baboon, common duiker, gemsbok, vervet monkey (Chlorocebus pygerythrus), and porcupine), while only six [gray rhebok, steenbok (Raphicerus campestris), small-spotted genet (Genetta genetta), aardwolf, klipspringer (Oreotragus oreotragus), and dassie] decreased. Both the caracal and small-gray mongoose maintained an approximately constant relative abundance (<5% variation).
Table 3. Relative Abundance Index (RAI) of species that occurred in all 4 years on all 16 cameras retained on the wildlife-friendly farm.
Species accumulation curves for the three different study sites from 2019 (Figure 5) show that at asymptote the game farm and traditional livestock farm presented a similar species richness (23 and 24, respectively). They were both significantly lower (outside of the 95% confidence interval) than that detected on the wildlife-friendly farm which recorded 34 species during the same 6-month period in 2019. Only 14 species were detected on all three study sites (Supplementary Table 2). Three species (giraffe, Cape clawless otter, and Cape mountain zebra) were not detected on the wildlife-friendly farm but were present on the game farm. The traditional farm detected a single species (leopard) that was not present on the wildlife-friendly farm. All three sites were experiencing the same drought conditions and received approximately the same rainfall.
Figure 5. Species accumulation curves for wildlife-friendly, traditional livestock and game farms for 2019, created using the individually corrected camera event histories (RAI values).
Despite the importance of livestock rangeland management to global biodiversity conservation few studies have evaluated the effect of different management practices at a farm scale. This study of a working Karoo farm is the only farm scale test we know of that investigates the effect of a change in management from traditional farming to wildlife-friendly farming. Our main findings suggest that the combination of stopping hunting and lethal predator control, the removal of internal fences, and replacing a camp grazing system with focused herding and night-time kraaling to produce a high intensity, short-duration, long rest grazing regime can increase mammalian biodiversity, distribution and relative abundance, even during an extreme and worsening drought period.
We found that species richness increased year-on-year on the wildlife-friendly farm since 2016, with a significant increase occurring in 2019 compared to previous years (Figure 4). We found that in comparison to the traditional livestock and game farm species richness was significantly higher on the wildlife-friendly farm (Figure 5). Species appeared to become increasingly distributed among camera stations between 2016 and 2019 (Table 2), whilst the majority (67%) of species that were detected in all 4 years showed increases in their relative abundance (Table 3). These positive outcomes were evident despite a prolonged period of drought.
The lack of increase in medium size predator species numbers is at odds with the general pattern of increase in mammalian biodiversity. This can partly be explained by the fact that 85% of the medium sized predators that could be detected in this area were already present throughout the wildlife-friendly farm in the 2016 survey. This leaves only a small margin for potential recruitment into the guild, unlike the herbivore guild where many species were undetected in 2016. Additionally, non-lethal predator management commenced 1 year prior to the initial survey period which may have allowed early species recovery and population shifts to occur by the time of the first sampling. The social structures and territorial behavior of these species may also have resulted in early territorial establishment. In the final survey year, the Cape clawless otter disappeared, likely due to the intensified drought in the region at this time, causing a reduction in both the length of streams and quantity of water bodies such as dams and natural pools. At the same time, the honey badger (Mellivora capensis) was first detected on the wildlife-friendly site, possibly due to more favorable conditions in this arid environment. The swapping of these species explains the lack of change in the number species.
Only one large predator species, the leopard, was detected across all three study sites. It was identified from a single capture event on the traditional livestock farm during the 2019 survey. However, two independent captures of leopard were made on the wildlife-friendly farm by camera traps not used in this study. It is suspected that all these captures are of the same individual leopard that was moving through the area. Wildlife-friendly farming has the potential to enable the coexistence of resident large predators, like the leopard, on rangelands where there is an abundant natural prey base without the danger of livestock losses.
The comparison of a wildlife-friendly farm to both a traditional livestock farm and game farm in 2019 yielded a notable difference in species richness and composition. Both the game farm and lethal control livestock farm presented a similar species richness, which was lower than that detected on the wildlife-friendly farm (Supplementary Table 2, Figure 5). It should be noted that the species richness for the wildlife-friendly farm in 2016 (~12 months after lethal control was stopped) was similar to the 2019 value for both the comparison properties. This suggests a common baseline in species richness across the region in the absence of shepherding. However, this should be interpreted cautiously due to the worsening drought and the comparison of different years. Follow up studies are needed to conclusively confirm the stability of the regional baseline. The direct comparison of the wildlife-friendly farm to the traditional farm reveals two groups of species absent from the latter: large game (blesbok, eland, red hartebeest, waterbuck, and blue wildebeest), and small predators [yellow mongoose (Cynictis penicillate), water mongoose, striped polecat (Ictonyx striatus), and small-spotted genet]. The large game species are easily controlled by fences and so their presence on any farm is generally due to reintroductions or retaining existing populations when fencing, conversely, their absence is largely due to human management. A traditional livestock production farm gains no direct financial value from the presence of these species, they may even be perceived as a problem in the form of increased competition for grazing, and as a result these species may have been traded or hunted out entirely. The lack of small predators on rangelands has been linked to changes in vegetation structure (shrub encroachment) as a result of overgrazing (Blaum et al., 2007). The high-density, short duration grazing strategy implemented on the wildlife-friendly farm impacts the vegetation in a non-selective manner, reducing overall shrub cover and promoting grass re-growth (S. Goets, personal communication, 10 October 2020). This increase in grass cover may provide additional food resources for small carnivore prey species, which in turn provide an increase in resource availability for small predators. Conversely, the high general stocking density and selective grazing occurring on both the game and the traditional livestock farms, could result in an increase in shrub cover, causing a decline in small carnivore prey availability, and thus small predators. Additionally, some of these small predators may be removed as by-catch during periods of indiscriminate lethal predator control (gin traps, foot hold, and cage traps and poison usage), inclusive of neighboring properties that were not sampled.
Conversion of the farm from traditional management to wildlife-friendly management had a widespread positive effect on mammalian biodiversity. Identifying the particular mechanisms underpinning the recovery is however difficult, since many changes occurred simultaneously as part of the conversion of the farm: (1) all hunting was stopped; (2) all lethal control of damage causing animals (and by-catch) was stopped, including setting of indiscriminate gin-traps, as well as targeted shooting and trapping of black-backed jackals, caracals, porcupine, baboons, Cape foxes and otters; (3) fences were removed and gates left open allowing all species access to the entire farm; (4) human and livestock activity was concentrated in a single area at any one time leaving the rest of the farm undisturbed by production activities and rested; (5) high-density grazing was limited to small areas, increasing soil disturbance, and defecation with potentially higher water infiltration and concentrated nutrient enrichment; (6) animals were kraaled at high densities in small areas for consecutive nights resulting in even a higher magnitude of transformation of these kraaling sites through trampling, grazing and defecation with beneficial vegetation recovery on these sites (McManus et al., 2018).
Any of these changes or combinations of them might have contributed to the observed increase in biodiversity richness, distribution and abundance of mammal species, and most likely different factors affected different species.
1) Ceasing hunting would have the potential to increase the number of large ungulates who would have been the target of recreational hunting. We see evidence of this increase in that four of the five species that showed the greatest increase in abundance were popular hunting species (blue wildebeest - 31-fold increase, eland - 11-fold increase, blesbok - 5-fold increase, red hartebeest - 4-fold increase) (Table 3). All other traditionally hunted species, gemsbok, springbok and kudu also increased in abundance (Table 3). The increase of ungulates that were traditionally hunted would also be affected by the removal of fences (4) which would allow a larger area to sustain larger populations that were formally accidentally, or intentionally confined to certain camps.
2) Ceasing targeted lethal control of “problem animals” should have increased the distribution and occurrence of black-backed jackals, caracals, baboons, porcupines, aardvark, Cape Fox and honey badgers all of which have been recorded as problem animal targets either for “assumed” killing of livestock or causing damage to fencing and water infrastructure. We have evidence of increases in distribution and for all of these species (Tables 2, 3, Cape fox and honey badger do not appear in Table 3 as the former was only recorded from 2017 onward and the latter only in the final year of the study Table 1).
3) Stopping lethal control by trapping also has the potential to affect mammal species by-catch, potentially increasing the presence of aardvark, aardwolf, bat-eared fox, duiker, small-spotted genet, small gray mongoose, water mongoose, yellow mongoose, striped polecat, scrub hare, African wildcat, and ground squirrel all of which have been recorded as by catch of gin traps (Bothma, 2012). All of these species (duiker, small gray mongoose, water mongoose, scrub hare, African wildcat) that occurred in all years of the study and therefore qualified for analysis of distribution and abundance (RAI) increased in both distribution and relative abundance.
4) Opening of fences and gates would have potentially increased the presence of all large ungulates on camera stations which would have been previously confined by fences. By concentrating livestock and humans (shepherds and managers) in a single area where the single large mixed herd occurred essentially allowed that at any one time 95% of the farm to be totally natural and undisturbed by livestock farming operations (on any single week livestock were always confined to <1,000 ha of the 22,111 ha farm), this would have benefitted all wild species. There is strong evidence that this benefitted the species previously enclosed by these fences – blue wildebeest, gemsbok, red hartebeest, eland, mountain reedbuck, springbok all showing large increase in distribution and relative abundance, while kudu who are known to jump fences and the smaller antelope that go through the fence like steenbok (Lindsey et al., 2012) showed small or negative changes.
Changes to the vegetation structure in the arid Karoo due to grazing is known to take place over long timescales (Wiegand and Milton, 1996), thus the response of mammals to the changes brought about by kraaling and high-density grazing would only become evident over time scales longer than this study (a decade or more). The stopping of hunting, and lethal control with its direct effects on target species and by-catch, as well as opening the entire farm's resources to the large ungulates by removing fences and barriers to movement, while only operating on <5% of the farm at any one time while leaving the rest of the area undisturbed were immediate and had large implications for wildlife, yet it is impossible to separate the direct effects of each of these new management actions. In essence the combination of these practices turns a commercial livestock farm into a more natural landscape akin to a nature reserve or conservation area.
The results of this case study are important as this farm scale conversion illustrates the potential of changing a large part of the world's area (livestock rangelands) to a situation where wildlife coexists on productive rangelands providing the resources to feed a growing population while maintaining employment (the shepherds) and economic opportunities in rural areas. The key to this is conceiving rangelands as primarily conservation areas where the dividends of natural capital (palatable grazing and forage) produced in a biodiverse naturally functioning ecosystem is used to produce food and livelihoods. Whilst these findings are based on the conversion of a single Karoo farm, although itself being a combination of five farms, the range and size of the improvements observed in mammalian biodiversity should be seen as motivation to encourage further farms, both in the Karoo and in other regions, to adopt some or all of these farming methods.
Globally, ecosystems are in decline and in order to reverse this trend, novel approaches to farming must be considered so that species diversity and ecosystem function can be restored. Samhouri et al. (2017) evaluated the implications of different approaches to facilitating wildlife recovery in a variety of scenarios. They identified that the fastest way to restore both predators and prey in an ecosystem was through a synchronized approach. Here, we have provided a case study demonstrating a significant and extensive improvement to mammalian biodiversity at a farm scale, achieved through a combination of wildlife-friendly management practices implemented on a commercial production farm, where both predator and prey species can recover simultaneously. Mammalian species have generally increased in variety, distribution, and density and the change was rapid occurring over a period of only 4 years. The recovery also demonstrated a resilience occurring as it did during a period of drought. Higher rainfall is known to increase species richness (Yarnell et al., 2007) while droughts result in reduced species diversity (Seabrook et al., 2011). However, we found that substantially lower rainfall occurred in 2018 and 2019 when the species richness was highest, demonstrating that even through the harshest conditions ecological recovery is possible. Whilst this study is limited by the constraints of not having replicate sites and the practicalities of sampling different sized farms, the trends observed are clear and informative. Going forward, we have implemented a modified monitoring protocol to ensure a more even sampling of the environments, and to allow an ongoing temporal analysis at all three study sites and we plan to expand farm scale studies to determine if these results are broadly applicable to similar conversions. This will provide for a future long-term evaluation of the implications of converting to wildlife-friendly farming.
This study has shed light onto the complex mammalian species responses to landscape modification and sustainable agriculture. We found that a continuing implementation of wildlife-friendly livestock practices has a positive effect on mammalian biodiversity by increasing species richness, as well as the number of sites occupied and the relative abundance of the majority of species. Although some changes where not evident on a yearly basis, the accumulated improvements suggest that a recovery time of at least 1 years is needed to start seeing the effect of restoration processes through better management practices. Should this restorative farming practice be applied to global rangelands, the implications for mammalian biodiversity recovery, and long-term security, are considerable.
The raw data supporting the conclusions of this article will be made available by the authors, without undue reservation.
BS and JM conceptualized and designed the study. MS, SG, and JM carried out the field work. MS, LP, and JM performed the statistical analysis. MS and DG drafted the manuscript. IS, CC, and VC provided input into the data interpretation. All authors contributed to the article and approved the submitted version.
Global Environmental Facility project managed by the United Nations Environmental Program: GEF Project identity number: 9382; Umoja No. 01333. GEF project: The project funding supported a multiple funded program to mainstream biodiversity conservation and sustainable use into production landscapes and production sectors. The project facilitated the support to re-establish human herding as management practice, to monitor and evaluate biodiversity response, production and financial impacts, to establish payment for ecosystem services and the running of a commercial farming enterprise with these principles. Green Fund, administered by the Development Bank of Southern Africa under contract dated 14 January 2015. The Green Fund set out to redevelop human herding as a conservation and green employment initiative, research into its efficacy in terms of employment, biodiversity conservation, production efficiency and financial impacts, and the facilitation of green production incentives. Woolworths Trust donation. The Woolworths Trust supported non-lethal predator control efforts in rangeland livestock production and the evaluation of such efforts. National Lotteries Distribution Trust Fund, Project Number 73027. The NLDTF, now called National Lotteries Commission, supported the training of herders in implementation of human herding in the research. Tamarisk Trust, Timothy Allsop, Brad Banducci, Henry and Iris Englund Foundation, Mones Michaels Trust, and Arne Hanson were private Trusts or persons that supported the research studies through private donations.
The authors declare that the research was conducted in the absence of any commercial or financial relationships that could be construed as a potential conflict of interest.
We sincerely thank the farmers who participated. We would like to extend our gratitude to the funders of this study: Timothy Allsop, Tamarisk Trust, National Department of Environment, Forestry and Fisheries, Development Bank of Southern Africa, Global Environmental Facility, Green Fund, United Nations Environmental Program, United Nations Development Program, Landmark Foundation Trust, Henry and Iris Englund Foundation, National Lotteries Distribution Trust Fund, Mones Michaels Trust, Brad Banducci and Arne Hanson. We would also like to thank Harry Lewis, Tamar Kendon and Candice Denner for assistance with the data processing.
The Supplementary Material for this article can be found online at: https://www.frontiersin.org/articles/10.3389/fcosc.2021.652415/full#supplementary-material
Blaum, N., Rossmanith, E., Schwager, M., and Jeltsch, F. (2007). Responses of mammalian carnivores to land use in arid savanna rangelands. Basic Appl. Ecol. 8, 552–564. doi: 10.1016/j.baae.2006.09.003
Boone, R. B., and Hobbs, N. T. (2004). Lines around fragments: effects of fencing on large herbivore. Afr. J. Range Forage Sci. 21, 147–158. doi: 10.2989/10220110409485847
Bothma, J. P. (2012). Literature Review of the Ecology .and Control of the Black-backed Jackal and Caracal in South Africa. Cape Town: CapeNature.
Butchart, S. H. M., Walpole, M., Collen, B., van Strien, A., Scharlemann, J. P. W., Almond, R. E. A., et al. (2010). Global Biodiversity: indicators of recent declines. Science 328, 1164–1168. doi: 10.1126/science.1187512
Dean, W. R. J., Seymour, C. L., and Joseph, G. S. (2018). Linear structures in the Karoo, South Africa, and their impacts on biota. Afr. J. Range Forage Sci. 35, 223–232. doi: 10.2989/10220119.2018.1514530
Díaz, S., Settele, J., Brondízio, E. S., Ngo, H. T., Agard, J., Arneth, A., et al. (2019). Pervasive human-driven decline of life on Earth points to the need for transformative change. Science 366:eaax3100. doi: 10.1126/science.aax3100
du Toit, G. N., Snyman, H. A., and Malan, P. J. (2009). Physical impact of grazing by sheep on soil parameters in the Nama Karoo subshrub/grass rangeland of South Africa. J. Arid Environ. 73, 0–810. doi: 10.1016/j.jaridenv.2009.03.013
Duelli, P., and Obrist, M. (2003). Biodiversity indicators: the choice of values and measures. Agric. Ecosyst. Environ. 98, 87–98. doi: 10.1016/S0167-8809(03)00072-0
Esler, K. J., Milton, S. J., and Dean, W. R. J. (2010). Karoo Veld: Ecology and Management. Pretoria: Briza Publications.
Filazzola, A., Brown, C., Dettlaff, M. A., Batbaatar, A., Grenke, J., Bao, T., Peetoom Heida, I., and Cahill, J. F. Jr (2020). The effects of livestock grazing on biodiversity are multi-trophic: a meta-analysis. Ecol Lett. 23, 1298–1309. doi: 10.1111/ele.13527
Goldblatt, A. (2010). Agriculture: Facts & Trends, South Africa. WWF-SA. Available online at: http://awsassets.wwf.org.za/downloads/facts_brochure_mockup_04_b.pdf (accessed December 24, 2020).
Harris, G., Thirgood, S., Hopcraft, J. G. C., Cromsigt, J. P. G. M., and Berger, J. (2009). Global decline in aggregated migrations of large terrestrial mammals. Endanger. Species Res. 7, 55–76. doi: 10.3354/esr00173
Hayward, M. W., and Kerley, G. I. H. (2009). Fencing for conservation: restriction of evolutionary potential or a riposte to threatening processes? Biol. Conserv. 142, 1–13. doi: 10.1016/j.biocon.2008.09.022
Hazzah, L., Dolrenry, S., Naughton, L., Edwards, C. T. T., Mwebi, O., Kearney, F., et al. (2014). Efficacy of two lion conservation programs in Maasailand, Kenya. Conserv. Biol. 28, 851–860. doi: 10.1111/cobi.12244
Hill, R., Dyer, G. A., Lozada-Ellison, L. M., Gimona, A., Martin-Ortega, J., Munoz-Rojas, J., et al. (2015). A social–ecological systems analysis of impediments to delivery of the Aichi 2020 Targets and potentially more effective pathways to the conservation of biodiversity. Glob. Environ. Change 34, 22–34. doi: 10.1016/j.gloenvcha.2015.04.005
Hoffman, M. T. (2014). Changing patterns of rural land use and land cover in South Africa and their implications for land reform. J. South. Afr. Stud. 40, 707–725. doi: 10.1080/03057070.2014.943525
Hsieh, T. C., Ma, K. H., and Chao, A. (2016). iNEXT: an R package for rarefaction and extrapolation of species diversity (Hill numbers). Methods Ecol. Evol. 7, 1451–1456. doi: 10.1111/2041-210X.12613
IPBES (2019). “Summary for policymakers of the global assessment report on biodiversity and ecosystem services of the intergovernmental science-policy platform on biodiversity and ecosystem services,” in eds S. Díaz, J. Settele, E. S. Brondízio, H. T. Ngo, M. Guèze, J. Agard, A. Arneth, P. Balvanera, K. A. Brauman, S. H. M. Butchart, K. M. A. Chan, L. A. Garibaldi, K. Ichii, J. Liu, S. M. Subramanian, G. F. Midgley, P. Miloslavich, Z. Molnár, D. Obura, A. Pfaff, S. Polasky, A. Purvis, J. Razzaque, B. Reyers, R. Roy Chowdhury, Y. J. Shin, I. J. Visseren-Hamakers, K. J. Willis, and C. N. Zayas (Bonn, Germany: IPBES secretariat), 56.
Kassambara, A. (2020). rstatix: Pipe-Friendly Framework for Basic Statistical Tests. R package version 0.6.0. Available online at: https://CRAN.R-project.org/package=rstatix (accessed June 18, 2020).
Kok, M. T., Alkemade, R., Bakkenes, M., van Eerdt, M., Janse, J., Mandryk, M., et al. (2018). Pathways for agriculture and forestry to contribute to terrestrial biodiversity conservation: a global scenario-study. Biol. Conserv. 221, 137–150. doi: 10.1016/j.biocon.2018.03.003
Landmark Foundation (2017). Shepherding Back Our Biodiversity – Mainstreaming Biodiversity Conservation on Agriculturally Productive Landscapes. Landmark Foundation. Available online at: https://www.landmarkfoundation.org.za/past-and-present-projects/shepherding-biodiversity/ (accessed April 21, 2016).
Lindsey, P. A., Masterson, C. L., Beck, A. L., and Romañach, S. S. (2012). “Ecological, social and financial issues related to fencing as a conservation tool in Africa,” In: Fencing for Conservation, eds M. Somers, and M. Hayward (New York, NY: Springer). Vol. 840, Chapter 12.
McManus, J., Goets, S. A., Bond, W. J., Henschel, J. R., Smuts, B., and Milton, S. J. (2018). Effects of short-term intensive trampling on Karoo vegetation. Afr. J. Range Forage Sci. 35, 311–318. doi: 10.2989/10220119.2018.1529706
McManus, J. S., Dickman, A. J., Gaynor, D., Smuts, B. H., and Macdonald, D. W. (2014). Dead or alive? Comparing costs and benefits of lethal and non-lethal human–wildlife conflict mitigation on livestock farms. Oryx 49, 687–695. doi: 10.1017/S0030605313001610
Milton, S. J., du Plessis, M. A., and Siegfried, W. R. (1994). A conceptual model of arid rangeland degradation. Bioscience 44, 70–76. doi: 10.2307/1312204
Mu, J., Zeng, Y., Wu, Q., Niklas, K. J., and Niu, K. (2016). Traditional grazing regimes promote biodiversity and increase nectar production in Tibetan alpine meadows. Agric. Ecosyst. Environ. 233, 336–342. doi: 10.1016/j.agee.2016.09.030
Mucina, L., Rutherford, M. C., Palmer, A. R., Milton, S. J., Scott, L., Lloyd, J. W., et al. (2006). “Nama-Karoo biome,” in: The Vegetation of South Africa, Lesotho and Swaziland. Strelitzia 19, eds L. Mucina, M. C. Rutherford (Pretoria: South African National Biodiversity Institute), 324–347.
Muriuki, M. W., Ipara, H., and Kiringe, J. W. (2017). The cost of livestock lost to lions and other wildlife species in the Amboseli ecosystem, Kenya. Eur. J. Wildl. Res. 63:60. doi: 10.1007/s10344-017-1117-2
Nattrass, N., and Conradie, B. (2015). Jackal narratives: predator control and contested ecologies in the Karoo, South Africa. J. South. Afr. Stud. 41, 753–771. doi: 10.1080/03057070.2015.1049484
Nenzhelele, E., Todd, S. W., and Hoffman, M. T. (2018). Long-term impacts of livestock grazing and browsing in the Succulent Karoo: a 20-year study of vegetation change under different grazing regimes in Namaqualand. Afr. J. Range Forage Sci. 35, 277–287. doi: 10.2989/10220119.2018.1519640
Niedballa, J., Sollmann, R., Courtiol, A., and Wilting, A. (2016). camtrapR: an R package for efficient camera trap data management. Methods Ecol. Evol. 7, 1457–1462. doi: 10.1111/2041-210X.12600
Palmer, M. S., Swanson, A., Kosmala, M., Arnold, T., and Packer, C. (2018). Evaluating relative abundance indices for terrestrial herbivores from large-scale camera trap surveys. Afr. J. Ecol. 56, 791–803. doi: 10.1111/aje.12566
Pardo, L. E., Campbell, M. J., Edwards, W., Clements, G. R., and Laurance, W. F. (2018). Terrestrial mammal responses to oil palm dominated landscapes in Colombia. PLoS ONE. 13:e0197539. doi: 10.1371/journal.pone.0197539
R Core Team (2017). R: A Language and Environment for Statistical Computing. Vienna: R Foundation for Statistical Computing.
Roche, C. (2004). “Ornaments of the Desert”: springbok treks in the Cape Colony, 1774–1908 (Unpublished MA thesis). University of Cape Town.
Roffe, S. J., Fitchett, J. M., and Curtis, C. J. (2019). Classifying and mapping rainfall seasonality in South Africa: a review. South Afr. Geogr. J. 101, 158–174. doi: 10.1080/03736245.2019.1573151
Samhouri, J., Stier, A., Hennessey, S., Novak, M., Halpern, B., and Levin, P. (2017). Rapid and direct recoveries of predators and prey through synchronized ecosystem management. Nat. Ecol. Evol. 1:0068. doi: 10.1038/s41559-016-0068
Seabrook, L., McAlpine, C., Baxter, G., Rhodes, J., Bradley, A., and Lunney, D. (2011). Drought-driven change in wildlife distribution and numbers: a case study of koalas in south west Queensland. Wildl. Res. 38, 509–524. doi: 10.1071/WR11064
Seymour, C. L., Milton, S. J., Joseph, G. S., Dean, W. R. J., Ditlhobolo, T., and Cumming, G. S. (2010). Twenty years of rest returns grazing potential, but not palatable plant diversity, to Karoo rangeland, South Africa. J. Appl. Ecol. 47, 859–867. doi: 10.1111/j.1365-2664.2010.01833.x
Sollmann, R. (2018). A gentle introduction to camera-trap data analysis. Afr. J. Ecol. 56, 740–749. doi: 10.1111/aje.12557
Stavi, I., Barkai, D., Knoll, Y. M., and Zaady, E. (2016). Livestock grazing impact on soil wettability and erosion risk in post-fire agricultural lands. Sci. Total Environ. 573, 1203–1208. doi: 10.1016/j.scitotenv.2016.03.126
Stuart, C., and Stuart, T. (2015). Stuarts' Field Guide to Mammals of Southern Africa, Including Angola, Zambia & Malawi. Cape Town: Struik Nature.
Tobler, M. W., Carrillo-Percastegui, S. E., Pitman, R. L., Mares, R., and Powell, G. (2008). An evaluation of camera traps for inventorying large- and medium-sized terrestrial rainforest Mammals 11, 169–178. doi: 10.1111/j.1469-1795.2008.00169.x
UN Food Agriculture Organization (2017). The State of Food and Agriculture: Leveraging Food Systems for Inclusive Rural Transformation. Available online at: http://www.fao.org/3/a-i7658e.pdf (accessed August 21, 2019).
Van Eeden, L. M., Crowther, M. S., Dickman, C. R., Macdonald, D. W., Ripple, W. J., Ritchie, E. G., et al. (2017). Managing conflict between large carnivores and livestock. Conserv. Biol. 32, 26–34. doi: 10.1111/cobi.12959
Van Niekerk, A. (2015). Stellenbosch University Digital Elevation Model (SUDEM) Product Description v15.15. Stellenbosch: Stellenbosch University.
Wiegand, T., and Milton, S. J. (1996). Vegetation change in semiarid communities. Vegetatio 125, 169–183. doi: 10.1007/BF00044649
Woodroffe, R., Hedges, S., and Durant, S. M. (2014). To fence or not to fence. Science 344, 46–48. doi: 10.1126/science.1246251
Keywords: farming, predator, shepherding, abundance, herbivore, rangelands, fences, richness
Citation: Schurch MPE, McManus J, Goets S, Pardo LE, Gaynor D, Samuels I, Cupido C, Couldridge V and Smuts B (2021) Wildlife-Friendly Livestock Management Promotes Mammalian Biodiversity Recovery on a Semi-Arid Karoo Farm in South Africa. Front. Conserv. Sci. 2:652415. doi: 10.3389/fcosc.2021.652415
Received: 12 January 2021; Accepted: 09 March 2021;
Published: 14 April 2021.
Edited by:
Silvio Marchini, University of São Paulo, BrazilReviewed by:
Julie Young, Animal and Plant Health Inspection Service (USDA), United StatesCopyright © 2021 Schurch, McManus, Goets, Pardo, Gaynor, Samuels, Cupido, Couldridge and Smuts. This is an open-access article distributed under the terms of the Creative Commons Attribution License (CC BY). The use, distribution or reproduction in other forums is permitted, provided the original author(s) and the copyright owner(s) are credited and that the original publication in this journal is cited, in accordance with accepted academic practice. No use, distribution or reproduction is permitted which does not comply with these terms.
*Correspondence: Matthew P. E. Schurch, bWF0dGhld0BsYW5kbWFya2ZvdW5kYXRpb24ub3JnLnph
Disclaimer: All claims expressed in this article are solely those of the authors and do not necessarily represent those of their affiliated organizations, or those of the publisher, the editors and the reviewers. Any product that may be evaluated in this article or claim that may be made by its manufacturer is not guaranteed or endorsed by the publisher.
Research integrity at Frontiers
Learn more about the work of our research integrity team to safeguard the quality of each article we publish.