- 1Department of Biological Sciences, Macquarie University, Sydney, NSW, Australia
- 2Taronga Conservation Society Australia, Mosman, NSW, Australia
Translocation programmes implying the movement of animals from one place to another aim to sustain endangered populations in the wild. However, their success varies greatly, with predation being a major contributing factor. This is particularly prevalent in released captive-raised individuals which have a reduced or lost awareness of predators. Alarm calls are an immediate response made toward a predator, mostly studied in highly predated, social vertebrates. These warning vocalizations are a vital part of a prey species' anti-predator behavior, enhancing the individuals' and surrounding listeners' survival. To date, most translocation programmes have not considered this behavior for release success. Here we review the literature summarizing alarm communication systems of wild and captive vertebrates, aiming to establish recommendations and actions which could encourage alarm communication behavior in captive vertebrate species. Observations of wild animals show that alarm-call understanding is gained through the experience of predation pressure from a young age, amongst conspecific and heterospecific social groups, which captive individuals can lack. This information, combined with consideration of a programme's accessible resources and captive individual's developmental history, is pivotal to efficiently guide appropriate actions. Focusing on preserving behaviors in captivity, we provide a list of recommendations and actions to guide the reinforcement of alarm communication throughout the translocation process. Ensuring predator awareness and the maintenance of alarm communication in translocated individuals may greatly improve the likelihood of release success.
Introduction
Changes in the Earth's environment through overexploitation and habitat destruction are threatening many species across the world (De Vos et al., 2015). Globally more than 5,300 vertebrates (10%) are considered to be endangered or critically endangered (IUCN, 2020). To date, a myriad of direct conservation actions have been employed to temper the pace of species declines. These include, but are not limited to, the creation of protected areas, the control of invasive species, and establishment of insurance populations in captivity or the wild (Berger-Tal and Saltz, 2016). In particular, translocation programmes are an important component, with 16% of critically endangered vertebrates dependent on these for survival (IUCN, 2020). Captive breeding programmes underpin conservation translocation programmes (from now on referred to as translocations), providing sufficient individuals for intentional release into former or new habitats, as a means of establishing or supplementing wild populations (Wallace, 1994; Thévenin, 2019; Berger-Tal et al., 2020).
Translocations have successfully prevented the extinction of a number of species. For example, Brazil's golden lion tamarin (Leontopithecus rosalia) was almost extinct in 1984 but increased to an estimated wild population of 1,600 individuals by 2006 (Kierulff et al., 2012). Similarly, a successful translocation programme for Western Australia's noisy scrub-bird (Atrichornis clamosus) increased its population size from 100 individuals to 770 over a five-decade period (Sooare, 2010). In both cases, success was attributed to a range of measures including long-term constant monitoring and adaptation of the programmes, habitat modification, and focusing on the socio-ecology and behavior of the species. The success of translocation programmes depends on numerous factors, including: the number of released individuals; the species' reproductive rate; the pertinent threat; active management; long-term post-monitoring commitment; location of release sites; and quality of habitat (Griffith et al., 1989; Fischer and Lindenmayer, 2000). The success of release programmes for threatened and endangered species is markedly lower (40% or less) than those for game species (Griffith et al., 1989). Overlooking a species' behavioral ecology is a likely reason for relatively low success of released threatened and endangered species (Griffith et al., 1989). On assessment of 293 case studies collated by the IUCN Conservation Translocation Specialist Group, the most reported issues limiting translocation success were associated with animal behavior (Berger-Tal et al., 2020). Programmes using wild source individuals have found success rates to be 14% higher than those using captive-born individuals (Fischer and Lindenmayer, 2000). For example, in carnivores, Jule et al. (2008) found projects to be three times more likely to succeed when releasing wild-caught individuals compared to captive-born. This highlights that the source of the released population can strongly influence the success of a translocation programme (Griffith et al., 1989).
Predation is a dominant limiting factor in translocation success, especially for released captive-born individuals, due to predator naivety (Berger-Tal et al., 2020). Without prior exposure to predators, released prey species have much lower odds of survival (Griffin et al., 2000; Jule et al., 2008). Within 3 months, up to 89% of captive-reared malleefowl (Leipoa ocellata) survived when released at sites without foxes (Vulpes vulpes) whereas 0–25% survived when released at locations with abundant foxes (Short, 2004). Prior exposure to predators can help. A study showed that 67% of greater bilbies (Macrotis lagotis) survived when they were exposed to a low density of feral cats (Felis catus) before release in comparison to only 29% of those with no prior exposure (Ross et al., 2019). Predation risk may have been reduced for cat-exposed bilbies as they took longer to emerge from cover and showed signs of increased social cooperation by sharing burrows in high predator pressure contexts (Ross et al., 2019). This emphasizes that predation can undermine a translocation programme's efforts if anti-predator behavior is not considered.
Sociality is an integral part of successful anti-predator behavior (Goldenberg et al., 2019). Alarm calls are a vital mechanism for many social prey species to quickly alert others to dangers within a group, ultimately increasing survival and fitness (Gill and Bierema, 2013; Kalb et al., 2019). “Alarm communication” entails production of calls, using calls in the right context, recognition of calls, and responding appropriately given the type of call. Variations in calls alert listeners to react in certain ways. For example, meerkats (Suricata suricatta) have acoustically distinct recruitment, aerial and terrestrial calls made in specific predator contexts, though the structure of each of these call types also varies within contexts, becoming noisier in high urgency situations (Manser, 2001). Japanese great tits (Parus major minor) use two distinct calls to code avian vs. snake nest predators, with physical mobbing occurring at a much closer distance to snakes to deter them from entering their nest cavities (Suzuki, 2014). Ensuring the maintenance of social (Goldenberg et al., 2019; Armansin et al., 2020) and predator awareness (West et al., 2018) behaviors throughout a captive-breeding programme is likely to be a key factor in minimizing predation, and therefore could increase the likelihood of a successful translocation programme (Berger-Tal et al., 2020).
By reviewing the literature on alarm communication in the wild and in captivity, and on current methods of addressing the impact of predation on translocation programmes, the objective of this paper is to provide recommendations and actions to preserve and reinforce alarm communication in captive species, to improve their chances of survival on release.
Alarm Communication in the Wild
Alarm calls are short and repetitive vocalizations evolved to enable distinct and fast communication when a predator is present (Klump and Shalter, 1984; Magrath et al., 2015a). These vocalizations are studied across a variety of highly predated and social vertebrate species such as noisy miners (Manorina melanocephala) (Cunningham and Magrath, 2017), black-fronted titi monkeys (Callicebus nigrifrons) (Cäsar et al., 2012), meerkats (Hollén and Manser, 2007), and Gunnison's prairie dogs (Cynomys gunnisoni) (Slobodchikoff and Placer, 2006). Different calls can encode different levels of risk, influencing an appropriate behavioral response by listeners to minimize their chances of being attacked. Risk can be communicated by varying acoustic properties, such as temporal and spectral structural differences (e.g. pitch or call length), which are associated with the function of the call (Marler, 1955; Klump and Shalter, 1984). Calls made at a lower frequency and slower speed are often classified as mobbing, recruitment or terrestrial calls (Kalb et al., 2019), because they are easily detectable to recruit others to the location of the predator to harass and deter it (Consla and Mumme, 2012). Upon detecting a high-risk predator, a number of fast and sometimes longer and higher frequency calls are made (Marler, 1955; Jurisevic and Sanderson, 1994; McLachlan and Magrath, 2020), which are classified as flee, aerial, or warning calls because they urge the listeners to hide and become difficult to be located by predators (Klump and Shalter, 1984; Igic and Magrath, 2014; Maziarz et al., 2018).
Individuals in social groups benefit from alarm calls, both as callers and as receivers (Brilot et al., 2012; Gill and Bierema, 2013). Even though vocalizing is likely to increase the caller's risk of predation by drawing attention to itself, calling improves the individual's odds of survival in a group context (Klump and Shalter, 1984). Sound travels, so acoustic information can influence a number of surrounding individuals at once and is advantageous in the context of terrestrial and aerial calls. The more individuals that respond to terrestrial calls by mobbing a predator [which elevates stress levels and discourages predators from hunting in the same area again (Consla and Mumme, 2012)], the more successful the group will be at driving the predator away. The more individuals that flee and hide in response to an aerial call, the less likely the high-risk predator will quickly approach the area and the more likely that more potential mates will survive (Smith, 1965). A predator's search area is also minimized when fewer prey are in the area after fleeing, which potentially reduces future visits (Klump and Shalter, 1984). Smaller populations of cooperative species have a higher predation risk (Krause and Ruxton, 2002) as larger groups help improve survival through increased vigilance (Sridhar et al., 2009) and better mobbing outcomes (Morand-Ferron and Quinn, 2011; Crates et al., 2017). A larger conspecific group is important to improve population survival when facing predation, a concept referred to as the Allee effect (Stephens et al., 1999). This means that predation is an imminent challenge for species that are close to extinction.
Vocalizations can be learned or inherited behaviors exhibited by both sexes (Seyfarth and Cheney, 2010). Captive-bred animals do not always have the same repertoire as wild individuals (Campbell and Snowdon, 2009; Ouattara et al., 2009) and are often naïve toward predators (McLean et al., 1999; Blumstein et al., 2019), which indicates that predator awareness and alarm communication are not purely instinctive. Learning plays a valuable role in effectively responding to predators because of the variability in predator encounters, such as predation risk, context, surrounding environment and social groups or networks (Griffin et al., 2000). Wild Diana monkeys (Cercopithecus diana) demonstrate that the vocal repertoire can develop in response to specific pressures in the immediate environment. One site-specific group of monkeys made complex call sequences specifically toward leopards (Panthera pardus), whereas a different site-specific group, where leopards were not present, did not (Stephan and Zuberbühler, 2008). Both monkey groups were exposed to the same leopard growl playbacks. The monkeys without leopard interaction used the same acoustically-structured call as used toward other general disturbances rather than a distinct call. Considering the value of alarm calls, social learning likely plays an important role in effectively understanding dangerous situations (Hollén and Radford, 2009; Greggor et al., 2014; Potvin et al., 2018). This can be seen amongst co-habiting species which can learn to identify unfamiliar calls through experience (Magrath et al., 2009a, 2015b). Superb fairy-wrens (Malurus cyaneus) which were not in the same geographical area as noisy-miners did not respond to noisy-miner alarm-call playbacks, highlighting that local interactions are required to recognize calls, particularly if they are dissimilar to their own (Magrath and Bennett, 2012). “Eavesdropping” on heterospecific calls provides a survival advantage by increasing the social response group size locally and across the wider community (Marler, 1957; Magrath et al., 2007, 2009b; Haff and Magrath, 2013). This commonly occurs in wild communities of small, sympatric passerines (Fallow et al., 2013) and is likely difficult to mimic in captive settings.
Studies of juveniles indicate the periods of time during which animals become able to learn calls and respond to them. Wild white-browed scrubwren (Sericornis frontalis) fledglings respond to conspecific alarm calls but not to heterospecific calls, presenting a “gradual learning response” (Haff and Magrath, 2013). Juveniles were found to have the same full-response repertoire as adults, including heterospecific calls, just 2 weeks post-fledging, highlighting a pivotal learning timeframe. An exposure to calls is also important as scrubwren fledglings only responded to heterospecific calls if brought up in the same geographical area as the heterospecific species (Haff and Magrath, 2013). Young mammals have also been shown to require an exposure to alarm calls in order to be able to rapidly understand them (Hollén and Manser, 2007). Juvenile Belding's ground squirrels (Urocitellus beldingi) tend to freeze when first leaving their burrows, not discriminating between calls, but quickly learn within just 5 days (Mateo, 2010). How experience with predators exactly influences this process is largely unknown, but learning through the direct experience, maturation (Stephan and Zuberbühler, 2008; Magrath et al., 2015b), and repeated exposure to predators (Hollén and Manser, 2007; Hollén and Radford, 2009) is likely to play an important role.
Alarm Communication in Captivity
Captive species may or may not have the same alarm-call repertoire, or acoustic structure, as their wild counterparts. Captive meerkats with little predator experience had the same vocal repertoire as wild meerkats (Hollén and Manser, 2007). However, low-urgency recruitment calls had a marginally different acoustic structure which was closer to medium-urgency terrestrial calls made in the wild, potentially caused by limited predator experience and captive habitats dissimilar to the wild. An investigation of whether a difference in acoustic structure has an impact on anti-predator behavior success once released into the wild would be valuable. Hollén and Manser (2007) suggest that meerkats maintained their wild repertoire through an exposure to similar predatory cues whilst in their outdoor enclosure (from overhead buzzards, airplanes, and animals in nearby enclosures), or because they were only up to five generations from wild-caught and retained their innate calling ability. Campbell's monkeys (Cercopithecus campbelli) provide an example where captive individuals are not described as having as an extensive alarm-call repertoire as wild individuals, likely due to limited predator exposure in general or during infant development (Ouattara et al., 2009). Exposing captive monkeys to predators would help uncover whether the behavioral difference is due to captive individuals' not having particular calls in their repertoire or not having an occasion to use particular alarm calls. As calls are considered a costly behavior, the ‘use it or lose it’ analogy may explain why captive animals do not call or have the same repertoire as wild counterparts (Ouattara et al., 2009). It is possible that being raised in a static, resource-rich environment with routines and boundaries may explain why captive-bred individuals do not succeed in translocation programmes in comparison with wild individuals.
Inability of released individuals to respond appropriately to predators (Fischer and Lindenmayer, 2000; Jule et al., 2008) is highlighted as the main cause of low translocation success (Heezik et al., 1999; Griffin et al., 2000; Grueber et al., 2017). The length of time in captivity and a lack of diverse experience during an animal's ontogeny are both likely to contribute to behavioral loss as shown in the following examples. Long-term captive breeding of Tasmanian devils (Sarcophilus harrisii) resulted in a reduction of behaviors necessary to survive in the wild (Grueber et al., 2017) and predator awareness behaviors were rapidly lost in northern quolls (Dasyurus hallucatus) within 13 generations of living in a predator-free environment (Jolly et al., 2018). Carrete and Tella (2015) compared the anti-predator response of captive-bred vs. wild-caught parrots and songbirds. They found that wild-caught individuals are more likely to sustain wild populations because they are more likely to respond to predators and reproduce. Consla and Mumme (2012) studied the response of raptors to mobbing events, comparing the individuals which were brought into captivity as juveniles and adults. Adults that had grown up in the wild had a heightened stress response to mobbing calls, whereas juveniles that had been in captivity from a young age showed a reduced physiological response to alarm cues (Consla and Mumme, 2012). Captive-bred individuals are limited in learning opportunities, adaptation, and selective pressures which are significantly controlled in captive environments (Jule et al., 2008). Integral behavioral knowledge is less likely to be passed on over generations in captivity as there is no reason to do so.
Translocation Success: Addressing Predation
Predation is a problem for the release of captive-bred individuals, but many of the issues associated with this can be attenuated and/or resolved through ex-situ (in captivity) or in-situ (in the wild) management actions of a translocation programme, as discussed in detail below.
Ex-situ management focuses on training captive animals to avoid predators. A variety of conditioning methods are used to encourage predator awareness and post-release survival, although they have mixed results (Blumstein et al., 2019; Rowell et al., 2020). Using live predators has been documented as an effective training method (Klump and Shalter, 1984; McLean et al., 1999). For example, post-release survival of captive houbara bustards (Chlamydotis undulata) improved for those exposed to real foxes in comparison to using model foxes or having little contact with humans (Heezik et al., 1999). However, using real predators requires a consideration of ethical and welfare implications. Other examples of training were unsuccessful, such as training naïve cotton-top tamarins (Saguinus oedipus) to mob a snake by coupling a moving, live, or novel predator with mobbing calls (Campbell and Snowdon, 2009). Interestingly, similar methods proved successful at training wild fairy-wrens to respond to a previously unknown alarm call (Magrath et al., 2015b), suggesting that training methods need to be specific (actual predator experience vs. call playbacks) or require more time to work with captive groups. Wild individuals have developed under high pressures and in changing environments, so they are likely to adapt quicker, unlike those in captive contexts which have had limited experiences. Greggor et al. (2014) highlighted the implementation of appropriate training methods based on cues and cognitive mechanisms of the behavior. For example, to promote continued avoidance frequent alteration of cues and reduction of their predictability is recommended to effectively manipulate a behavior (Greggor et al., 2014). Determining suitable methods to successfully train captive individuals how to defend themselves would likely require species-specific research and depend on captivity parameters (Griffin et al., 2000). Ideally, providing housing and upbringing that are similar to wild conditions, such as outdoor (Campbell and Snowdon, 2009) and mixed-species enclosures (Maziarz et al., 2018), can encourage alarm communication and may help sustain the diversity of anti-predator responses (Blumstein et al., 2019). Measuring release success after training individuals is most important for improving conservation outcomes (Rowell et al., 2020).
The most successful case studies of releases of captive-born individuals are associated with suitable predator control in-situ (Short, 2004). Appropriate methods aim to slow down or reduce the intensity of predation by releasing individuals into predator-free areas, predator-proof habitats, or areas affected by predator removal (Griffin et al., 2000; Canessa et al., 2019). Many examples are seen in Australia, a country with particularly high rates of reintroduction failure (56%) compared to the rest of the world, linked to a high number of feral predators (Fischer and Lindenmayer, 2000). Reducing numbers of cats and foxes resulted in the successful reintroduction of brush-tailed bettongs (Bettongia penicillata) and parma wallabies (Macropus parma), whereas insufficient lethal control resulted in failed reintroductions of golden bandicoots (Isoodon auratus) and burrowing bettongs (Bettongia lesueur) (Short, 2004). Although promising, predator management can be difficult to implement and maintain for ongoing success. Large amounts of land, funds and continued maintenance (e.g. fences and monitoring) are required to keep areas predator-free. On top of that, strategies to maintain predator awareness behaviors of released populations in predator-free areas must be implemented (Jolly et al., 2018). Predator-proofing of specific sites, for example using artificial nests or burrows, may target only one predator type and released individuals may ignore designated nests or burrows (Canessa et al., 2019). Culling predators can improve release survival outcomes in the short term (Fulton and Ford, 2001) but requires multiple considerations including: the protected status of the predator(s); compliance to ethical standards; communicating methods publicly for community understanding and approval; culling a justified number of predator individuals to secure the effectiveness of translocation; assessing opportunities for other predators in response to culling; and estimating the time for immigration and re-population of predators in the area. Effective implementation of any of these methods requires project-specific planning and application.
Recommendations
Here we provide recommendations and suggest actions to help preserve and reinforce the alarm communication systems of captive species that naturally have an alarm-call repertoire. These are divided into four stages of a translocation programme: (1) initial feasibility; (2) pre-release planning and preparation; (3) release; and (4) post-release monitoring (IUCN/SSC, 2013). Each recommendation has a brief justification, followed by bullet-pointed action options. Figure 1 provides a flow diagram showing the decision-making process of the provided recommendations.
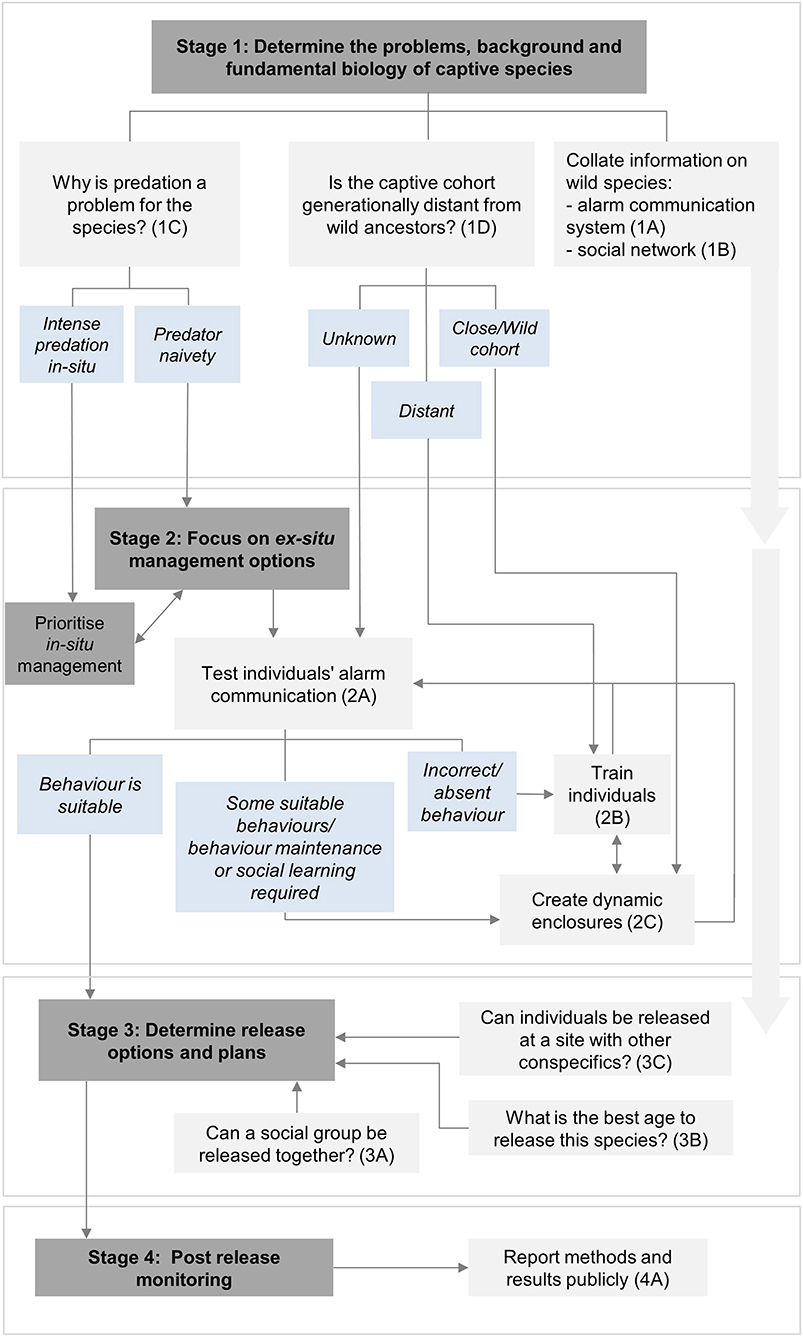
Figure 1. The decision-making flowchart showing the key considerations and actions to preserve and reinforce alarm communication systems of captive species during the translocation process. Dark gray boxes indicate relevant stages of the translocation programme, light gray boxes indicate important recommendations to consider and light blue boxes provide outcomes to help guide the most useful recommendations to proceed with. Further details about specific recommendations can be found next to the corresponding number and letter (e.g. 1A) within the text. Double-headed arrows found in Stage 2 mean that both recommendations can be useful and applied together if sufficient resources are available for the programme.
Stage 1: Initial Feasibility
1A Collate available information on the species' wild alarm communication system:
This information is helpful to determine whether the behavior/repertoire of captive individuals is similar or dissimilar to wild individuals.
• Determine the minimum age at which individuals can perceive and respond to alarm calls.
• If the wild call repertoire of the species is unknown, determine the feasibility of obtaining this data over the course of the breeding programme, or examine closely related species.
1B Collate a thorough understanding of the species' social network:
Understanding social interactions of a species, particularly whether groups live among interspecific networks, can help determine appropriate enclosure dynamics to match wild conditions in the best way. This is also an important factor in encouraging natural learning of conspecific and heterospecific calls, which is advantageous for survival in the wild.
• Ensure that enough individuals are brought into the breeding programme to be able to form a sufficient conspecific group.
• Provide enclosures which match the species' natural networks, both conspecific and heterospecific, to encourage natural learning. For example, ensure that a group of conspecifics are kept together with a number of individuals matching wild group sizes, or create an outdoor enclosure with multiple heterospecific species which would naturally co-habit in the wild.
1C Determine the primary reason why predation is a problem for the released species:
Identifying the main concerns regarding predation (naivety, wild predation pressure or a combination of both) will help guide the most efficient actions during captive breeding of the species. For example, if predation intensity is high, translocation success may be low even if released species have sufficient anti-predator behavior.
• Review past research and survey results of predation impact on the declining species or undertake surveys if required.
• If naivety is likely the main concern, focus resources on pre-release planning and preparation methods. Determine the main predator in the wild and focus on training appropriate alarm calling and alarm calling responses to this predator in particular.
• If predation pressure is the main concern, focus on addressing this threat in-situ.
1D Determine generation distance from the wild:
The generation distance from wild ancestors impacts behavioral traits of captive individuals (Grueber et al., 2017; Jolly et al., 2018). Those closer to wild generations are more likely to innately express alarm communication behaviors (Hollén and Manser, 2007).
• If individuals are generationally close to individuals originated from the wild or wild-born sourced individuals, then the programme could focus predominately on maintaining behaviors through varied threat exposure methods or dynamic enclosures (see recommendation 2C for more details). Testing for alarm communication behaviors is still recommended (recommendation 2A).
• Conversely if individuals are generationally distant to individuals originated from the wild or wild-born sourced individuals, training and developing anti-predator behavior will likely be important to prioritize through appropriate training and/or through dynamic enclosures (see recommendation 2C for more details).
Stage 2: Pre-release Planning and Preparation
2A Ex-situ—test for suitable alarm communication behavior to determine whether the captive-cohort has suitable predator awareness behaviors:
Determining whether the captive group/individuals utter suitable alarm calls to the appropriate threats and respond suitably assists in targeting management actions.
• If call and response repertoire is similar to wild individuals, focus on maintaining this behavior for the captive lifetime of the individuals through appropriate continued exposure, enclosure management, social group interactions and releasing at suitable ages. Also, focus on implementing other steps of the translocation process (such as in-situ methods) to minimize predation risk on release.
• If call and response repertoire is minimal/dissimilar from wild individuals, focus on appropriate behavioral conditioning of animals prior to release through training and/or enclosure management.
2B Ex-situ—establish suitable alarm communication training methods:
Training can help minimize predator naivety by promoting suitable alarm communication behaviors. Methods will depend on the species, their current behavioral representation, context, ethics and feasibility.
• Know where the species will be released so training can be targeted on predators found in the release site or only on the main predator (e.g. nest or aerial predators with alarm calls being unique for each predator type).
• Target conditioning training at an earlier stage of development (Mateo, 2010).
• Have regular predator exposure, taking habituation into consideration and using different cues throughout the captive life to maintain behaviors (Greggor et al., 2014).
• Set a specific goal for behavioral change (Rowell et al., 2020).
2C Ex-situ—Create dynamic enclosures (i.e. large, outdoor enclosures with mixed species) as similar to natural habitats as possible:
Enclosure environments can assist in maintaining or encouraging alarm communication behaviors. Also, development in an environment that mimics wild environments may help reduce adaptation time (Berger-Tal and Saltz, 2016).
• Outdoor enclosures allow for interactions with cues which may be the same or similar to predator cues in the wild.
• Mixed-species enclosures, similar to wild communities, may encourage learning of interspecific networks such as understanding heterospecific calls (Hollén and Radford, 2009).
• Moving groups between different enclosures and providing them with new environments and different species to interact with and learn from may increase the call diversity and the speed at which animals learn to react in new and different environments.
• Ensure that there are enough individuals to support group dynamics and social learning. Different age ranges may be required to support learning skills (Hollén and Radford, 2009).
• Supplement captive groups with wild animals over time to assist with training alarm calls.
• If required and possible, implement this recommendation in conjunction with training.
• Consider controlled predator exposure by including a low density of predators within a suitable enclosure or release-pen at the intended location of release (Ross et al., 2019). This may happen in place of other forms of training (recommendation 2B) or could be performed as a multi-stage part of pre-release preparation, occurring after training and/or time in a dynamic enclosure but prior to release into the wild.
Stage 3: Release
3A Release social groups together:
A group may have formed social bonds, hierarchy and reliability of communication, and this can be beneficial for survival success (Goldenberg et al., 2019).
• If a social group has bonded in captivity, releasing together may improve the odds of survival through alarm-call reliability.
• Ensure that captive husbandry can support training, learning and social dynamics of new individuals/groups brought into captivity if a whole group (likely at different ages) is intended to be released together.
3B Release individuals at suitable ages:
Age can impact learning ability.
• Learning to recognize and respond to alarm calls in the wild by being released at an earlier age may improve long-term ability of the behavior. Although juveniles are at a higher risk of predation due to limited knowledge and skills, it is the crucial time for learning. A risk-based ethical approach should be considered.
3C Consider population dynamics in release sites:
Knowing precisely where a captive cohort will be released can help determine if integration with wild or prior-released conspecifics is possible.
• Releasing captives with a current group of conspecifics of wild origin may provide an enhanced opportunity for learning alarm calls from wild groups.
Stage 4: Post-release Monitoring
4A Report results publicly:
Any insight and experience of incorporating maintenance and reinforcement of species' alarm communication systems into a programme, successful or not, is useful, practical and worth sharing.
• Clarify the methods used throughout the translocation process to encourage alarm calls, providing generation details on the species and how success was measured on release.
• Determine suitable measure of improved survivorship.
Conclusion
Translocations are an important conservation tool to assist the global biodiversity loss, but their success is variable. Predation is a significant inhibitor of success in translocation programmes. Alarm communication is vital for survival (Anthony and Blumstein, 2000) and its development and maintenance should be encouraged, potentially improving the translocation outcomes. Identifying the key factors of a captive population such as the distance from the wild source and testing the naivety of captive animals are important to efficiently address actions which shape appropriate behaviors. We believe that actions encouraging learning and development in captivity, such as direct training and enclosure management, can increase alarm communication behavior. Identifying and appropriately implementing these actions will improve the success rates of translocation programmes and the species they aim to conserve.
Data Availability Statement
The original contributions presented in the study are included in the article, further inquiries can be directed to the corresponding author/s.
Author Contributions
VM developed the concept and wrote the manuscript with significant contribution from BP and AC. All authors contributed to the article and approved the submitted version.
Funding
This research was funded by Macquarie University's scholarships for Higher Degree Research (HDR). BP is partially funded by a Macquarie University Research Fellowship. Macquarie University's Department of Biological Sciences has assisted with funds toward publication fees.
Conflict of Interest
The authors declare that the research was conducted in the absence of any commercial or financial relationships that could be construed as a potential conflict of interest.
Acknowledgments
We would like to thank G. Chariton for editing the manuscript and R. Furnell and E. Dodson for assistance in preparation of the decision-making flowchart.
References
Anthony, L. L., and Blumstein, D. T. (2000). Integrating behaviour into wildlife conservation: the multiple ways that behaviour can reduce Ne. Biol. Conserv. 95, 303–315. doi: 10.1016/S0006-3207(00)00037-9
Armansin, N. C., Stow, A. J., Cantor, M., Leu, S. T., Klarevas-Irby, J. A., Chariton, A. A., et al. (2020). Social barriers in ecological landscapes: the social resistance hypothesis. Trends Ecol. Evol. 35, 137–148. doi: 10.1016/j.tree.2019.10.001
Berger-Tal, O., Blumstein, D. T., and Swaisgood, R. R. (2020). Conservation translocations: a review of common difficulties and promising directions. Anim. Conserv. 23, 121–131. doi: 10.1111/acv.12534
Berger-Tal, O., and Saltz, D. (2016). Conservation Behavior: Applying Behavioral Ecology to Wildlife Conservation and Management, Vol. 21. New York, NY: Cambridge University Press. doi: 10.1017/CBO9781139627078
Blumstein, D. T., Letnic, M., and Moseby, K. E. (2019). In situ predator conditioning of naive prey prior to reintroduction. Philos. Trans. R. Soc. B Biol. Sci. 374:20180058. doi: 10.1098/rstb.2018.0058
Brilot, B. O., Bateson, M., Nettle, D., Whittingham, M. J., and Read, J. C. A. (2012). When is general wariness favored in avoiding multiple predator types? Am. Nat. 179, E180–E195. doi: 10.1086/665648
Campbell, M. W., and Snowdon, C. T. (2009). Can auditory playback condition predator mobbing in captive-reared Saguinus oedipus? Int. J. Primatol. 30, 93–102. doi: 10.1007/s10764-008-9331-0
Canessa, S., Taylor, G., Clarke, R. H., Ingwersen, D., Vandersteen, J., and Ewen, J. G. (2019). Risk aversion and uncertainty create a conundrum for planning recovery of a critically endangered species. Conserv. Sci. Pract. n/a(n/a). e138. doi: 10.1111/csp2.138
Carrete, M., and Tella, J. L. (2015). Rapid loss of antipredatory behaviour in captive-bred birds is linked to current avian invasions. Sci. Rep. 5, 1–8. doi: 10.1038/srep18274
Cäsar, C., Byrne, R. W., Hoppitt, W., Young, R. J., and Zuberbühler, K. (2012). Evidence for semantic communication in titi monkey alarm calls. Anim. Behav. 84, 405–411. doi: 10.1016/j.anbehav.2012.05.010
Consla, D. J., and Mumme, R. L. (2012). Response of captive raptors to avian mobbing calls: the roles of mobber size and raptor experience. Ethology 118, 1063–1071. doi: 10.1111/eth.12007
Crates, R., Rayner, L., Stojanovic, D., Webb, M., and Heinsohn, R. (2017). Undetected Allee effects in Australia's threatened birds: implications for conservation. Emu 117, 207–221. doi: 10.1080/01584197.2017.1333392
Cunningham, S., and Magrath, R. D. (2017). Functionally referential alarm calls in noisy miners communicate about predator behaviour. Anim. Behav. 129, 171–179. doi: 10.1016/j.anbehav.2017.05.021
De Vos, J. M., Joppa, L. N., Gittleman, J. L., Stephens, P. R., and Pimm, S. L. (2015). Estimating the normal background rate of species extinction. Conserv. Biol. 29, 452–462. doi: 10.1111/cobi.12380
Fallow, P. M., Pitcher, B. J., and Magrath, R. D. (2013). Alarming features: birds use specific acoustic properties to identify heterospecific alarm calls. Proc. R. Soc. B Biol. Sci. 280:20122539. doi: 10.1098/rspb.2012.2539
Fischer, J., and Lindenmayer, D. B. (2000). An assessment of the published results of animal relocations. Biol. Conserv. 96, 1–11. doi: 10.1016/S0006-3207(00)00048-3
Fulton, G. R., and Ford, H. A. (2001). The Pied Currawong's role in avian nest predation: a predator removal experiment. Pacific Conserv. Biol. 7:154. doi: 10.1071/PC010154
Gill, S. A., and Bierema, A. M.-K. (2013). On the meaning of alarm calls: a review of functional reference in avian alarm calling. Ethology 119, 449–461. doi: 10.1111/eth.12097
Goldenberg, S. Z., Owen, M. A., Brown, J. L., Wittemyer, G., Oo, Z. M., and Leimgruber, P. (2019). Increasing conservation translocation success by building social functionality in released populations. Glob. Ecol. Conserv. 18:e00604. doi: 10.1016/j.gecco.2019.e00604
Greggor, A. L., Clayton, N. S., Phalan, B., and Thornton, A. (2014). Comparative cognition for conservationists. Trends Ecol. Evol. 29, 489–495. doi: 10.1016/j.tree.2014.06.004
Griffin, A. S., Blumstein, D. T., and Evans. (2000). Review: training captive-bred or translocated animals to avoid predators. Conserv. Biol. 14:11. doi: 10.1046/j.1523-1739.2000.99326.x
Griffith, B., Scott, J. M., Carpenter, J. W., and Reed, C. (1989). Translocation as a species conservation tool: status and strategy. Science 245, 477–480. doi: 10.1126/science.245.4917.477
Grueber, C. E., Reid-Wainscoat, E. E., Fox, S., Belov, K., Shier, D. M., Hogg, C. J., et al. (2017). Increasing generations in captivity is associated with increased vulnerability of Tasmanian devils to vehicle strike following release to the wild. Sci. Rep. 7, 1–7. doi: 10.1038/s41598-017-02273-3
Haff, T. M., and Magrath, R. D. (2013). Eavesdropping on the neighbours: fledglings learn to respond to heterospecific alarm calls. Anim. Behav. 85, 411–418. doi: 10.1016/j.anbehav.2012.11.016
Heezik, Y., Seddon, P. J., and Maloney, R. F. (1999). Helping reintroduced houbara bustards avoid predation: effective anti-predator training and the predictive value of pre-release behaviour. Anim. Conserv. 2, 155–163. doi: 10.1111/j.1469-1795.1999.tb00061.x
Hollén, L. I., and Manser, M. B. (2007). Persistence of alarm-call behaviour in the absence of predators: a comparison between wild and captive-born meerkats (Suricata Suricatta). Ethology 113, 1038–1047. doi: 10.1111/j.1439-0310.2007.01409.x
Hollén, L. I., and Radford, A. N. (2009). The development of alarm call behaviour in mammals and birds. Anim. Behav. 78, 791–800. doi: 10.1016/j.anbehav.2009.07.021
Igic, B., and Magrath, R. D. (2014). A songbird mimics different heterospecific alarm calls in response to different types of threat. Behav. Ecol. 25, 538–548. doi: 10.1093/beheco/aru018
IUCN (2020). The IUCN Red List of Threatened Species. IUCN Red List of Threatened Species. Available online at: https://www.iucnredlist.org/en (accessed March 26, 2020).
IUCN/SSC (2013). Guidelines for Reintroductions and Other Conservation Translocations. Gland: IUCN Species Survival Commission, viiii + 57pp.
Jolly, C. J., Webb, J. K., and Phillips, B. L. (2018). The perils of paradise: an endangered species conserved on an island loses antipredator behaviours within 13 generations. Biol. Lett. 14:20180222. doi: 10.1098/rsbl.2018.0222
Jule, K. R., Leaver, L. A., and Lea, S. E. G. (2008). The effects of captive experience on reintroduction survival in carnivores: a review and analysis. Biol. Conserv. 141, 355–363. doi: 10.1016/j.biocon.2007.11.007
Jurisevic, M. A., and Sanderson, K. J. (1994). The vocal repertoires of six honeyeater (Meliphagidae) species from Adelaide, South Australia. Emu, 94, 141–148. doi: 10.1071/MU9940141
Kalb, N., Anger, F., and Randler, C. (2019). Subtle variations in mobbing calls are predator-specific in great tits (Parus major). Sci. Rep. 9:6572. doi: 10.1038/s41598-019-43087-9
Kierulff, M. C. M., Ruiz-Miranda, C. R., Oliveira, P. P., Beck, B. B., Martins, A., Dietz, J. M., et al. (2012). The Golden lion tamarin Leontopithecus rosalia: a conservation success story. Int. Zoo. Yb. 46, 36–45. doi: 10.1111/j.1748-1090.2012.00170.x
Klump, G., and Shalter, M. (1984). Acoustic behaviour of birds and mammals in the predator context; I. Factors affecting the structure of alarm signals. II. The functional significance and evolution of alarm signals. Z. Tierpsychol. 66, 189–226. doi: 10.1111/j.1439-0310.1984.tb01365.x
Magrath, R. D., and Bennett, T. H. (2012). A micro-geography of fear: learning to eavesdrop on alarm calls of neighbouring heterospecifics. Proc. R. Soc. B: Biol. Sci. 279, 902–909 doi: 10.1098/rspb.2011.1362
Magrath, R. D., Haff, T. M., Fallow, P. M., and Radford, A. N. (2015a). Eavesdropping on heterospecific alarm calls: from mechanisms to consequences. Biol. Rev. 90, 560–586. doi: 10.1111/brv.12122
Magrath, R. D., Haff, T. M., McLachlan, J. R., and Igic, B. (2015b). Wild birds learn to eavesdrop on heterospecific alarm calls. Curr. Biol. 25, 2047–2050. doi: 10.1016/j.cub.2015.06.028
Magrath, R. D., Pitcher, B. J., and Gardner, J. L. (2007). A mutual understanding? Interspecific responses by birds to each other's aerial alarm calls. Behav. Ecol. 18, 944–951. doi: 10.1093/beheco/arm063
Magrath, R. D., Pitcher, B. J., and Gardner, J. L. (2009a). Recognition of other species' aerial alarm calls: speaking the same language or learning another? Proc. R. Soc. B Biol. Sci. 276, 769–774. doi: 10.1098/rspb.2008.1368
Magrath, R. D., Pitcher, B. J., and Gardner, J. L. (2009b). An avian eavesdropping network: alarm signal reliability and heterospecific response. Behav. Ecol. 20, 745–752. doi: 10.1093/beheco/arp055
Manser, M. B. (2001). The acoustic structure of suricates' alarm calls varies with predator type and the level of response urgency. Proc. R. Soc. London Ser. B 268, 2315–2324. doi: 10.1098/rspb.2001.1773
Marler, P. (1957). Specific distinctiveness in the communication signals of birds. Behaviour 11, 13–39. doi: 10.1163/156853956X00066
Mateo, J. M. (2010). Alarm calls elicit predator-specific physiological responses. Biol. Lett. 6, 623–625. doi: 10.1098/rsbl.2010.0118
Maziarz, M., Piggott, C., and Burgess, M. (2018). Predator recognition and differential behavioural responses of adult wood warblers Phylloscopus sibilatrix. Acta Ethol. 21, 13–20. doi: 10.1007/s10211-017-0275-2
McLachlan, J. R., and Magrath, R. D. (2020). Speedy revelations: how alarm calls can convey rapid, reliable information about urgent danger. Proc. R. Soc. B Biol. Sci. 287:20192772. doi: 10.1098/rspb.2019.2772
McLean, I. G., Hölzer, C., and Studholme, B. J. (1999). Teaching predator-recognition to a naive bird: implications for management. Biol. Conserv. 87, 123–130. doi: 10.1016/S0006-3207(98)00024-X
Morand-Ferron, J., and Quinn, J. L. (2011). Larger groups of passerines are more efficient problem solvers in the wild. Proc. Natl. Acad. Sci. 108, 15898–15903. doi: 10.1073/pnas.1111560108
Ouattara, K., Zuberbühler, K., N'goran, E. K., Gombert, J.-E., and Lemasson, A. (2009). The alarm call system of female Campbell's monkeys. Anim. Behav. 78, 35–44. doi: 10.1016/j.anbehav.2009.03.014
Potvin, D. A., Ratnayake, C. P., Radford, A. N., and Magrath, R. D. (2018). Birds learn socially to recognize heterospecific alarm calls by acoustic association. Curr. Biol. 28, 2632–2637. doi: 10.1016/j.cub.2018.06.013
Ross, A. K., Letnic, M., Blumstein, D. T., and Moseby, K. E. (2019). Reversing the effects of evolutionary prey naiveté through controlled predator exposure. J. Appl. Ecol. 56, 1761–1769. doi: 10.1111/1365-2664.13406
Rowell, T. A., Magrath, M. J., and Magrath, R. D. (2020). Predator-awareness training in terrestrial vertebrates: progress, problems and possibilities. Biol. Conserv. 252:108740. doi: 10.1016/j.biocon.2020.108740
Seyfarth, R. M., and Cheney, D. L. (2010). Production, usage, and comprehension in animal vocalizations. Brain Lang. 115, 92–100. doi: 10.1016/j.bandl.2009.10.003
Short, J. (2004). “Conservation of the Malleefowl: are there lessons from the successful conservation of native mammals by intensive fox control?” in Proceedings of the National Malleefowl Forum (Dubbo, Australia), 54–68.
Slobodchikoff, C. N., and Placer, J. (2006). Acoustic structures in the alarm calls of Gunnison's prairie dogs. J. Acoust. Soc. Am. 119, 3153–3160. doi: 10.1121/1.2185489
Sooare, P. S. (2010). Global Re-Introduction Perspectives: 2010. Abu Dahbi: IUCN/SSC Re-introduction Specialist Group.
Sridhar, H., Beauchamp, G., and Shanker, K. (2009). Why do birds participate in mixed-species foraging flocks? A large-scale synthesis. Anim. Behav. 78, 337–347. doi: 10.1016/j.anbehav.2009.05.008
Stephan, C., and Zuberbühler, K. (2008). Predation increases acoustic complexity in primate alarm calls. Biol. Lett. 4, 641–644 doi: 10.1098/rsbl.2008.0488
Stephens, P. A., Sutherland, W. J., and Freckleton, R. P. (1999). What is the Allee effect? Oikos 87, 185–190. doi: 10.2307/3547011
Suzuki, T. N. (2014). Communication about predator type by a bird using discrete, graded and combinatorial variation in alarm calls. Anim. Behav. 87, 59–65. doi: 10.1016/j.anbehav.2013.10.009
Thévenin, C. (2019). Reintroduction efficiency: a stepping stone approach to reintroduction success? Anim. Conserv. 22, 116–117. doi: 10.1111/acv.12501
Wallace, M. P. (1994). Control of behavioral development in the context of reintroduction programs for birds. Zoo Biol. 13, 491–499. doi: 10.1002/zoo.1430130511
Keywords: anti-predation, reintroductions, communication, conservation, animal behavior, social species
Citation: Morris V, Pitcher BJ and Chariton A (2021) A Cause for Alarm: Increasing Translocation Success of Captive Individuals Through Alarm Communication. Front. Conserv. Sci. 2:626311. doi: 10.3389/fcosc.2021.626311
Received: 05 November 2020; Accepted: 08 February 2021;
Published: 26 February 2021.
Edited by:
Katherine Moseby, University of New South Wales, AustraliaReviewed by:
Robert Magrath, Australian National University, AustraliaIgor Khorozyan, University of Göttingen, Germany
Copyright © 2021 Morris, Pitcher and Chariton. This is an open-access article distributed under the terms of the Creative Commons Attribution License (CC BY). The use, distribution or reproduction in other forums is permitted, provided the original author(s) and the copyright owner(s) are credited and that the original publication in this journal is cited, in accordance with accepted academic practice. No use, distribution or reproduction is permitted which does not comply with these terms.
*Correspondence: Vanessa Morris, dmFuZXNzYS5tb3JyaXNAbXEuZWR1LmF1