- 1Department of Art and Design, University of Minnesota Duluth, Duluth, MN, United States
- 2Stazione Zoologica Anton Dohrn, Naples, Italy
- 3Center for Synaptic Neuroscience and Technology (NSYN), Istituto Italiano di Tecnologia, Genova, Italy
- 4Department of the History, Philosophy and Ethics of Medicine, Centre for Health and Society, Medical Faculty, Heinrich Heine Universität Düsseldorf, Düsseldorf, Germany
- 5Biofaction KG, Vienna, Austria
Cephalopods are outstanding animals. For centuries, they have provided a rich source of inspiration to many aspects of human cultures, from art, history, media, and spiritual beliefs to the most exquisite scientific curiosity. Given their high esthetical value and “mysteriously” rich behavioral repertoire they have functioned as boundary objects (or subjects) connecting seemingly distinct thematic fields. Interesting aspects of their being span from the rapid camouflaging ability inspiring contemporary art practices, to their soft and fully muscular body that curiously enough inspired both gastronomy and (soft) robotics. The areas influenced by cephalopods include ancient mythology, art, behavioral science, neuroscience, genomics, camouflage technology, and bespoken robotics. Although these might seem far related fields, in this manuscript we want to show how the increasing scientific and popular interest in this heterogeneous class of animals have indeed prompted a high level of integration between scientific, artistic, and sub-popular culture. We will present an overview of the birth and life of cephalopod investigations from the traditional study of ethology, neuroscience, and biodiversity to the more recent and emerging field of genomics, material industry, and soft robotics. Within this framework, we will attempt to capture the current interest and progress in cephalopod scientific research that lately met both the public interest and the “liberal arts” curiosity.
Introduction
Cephalopods are the molluscan class including octopus, squid, cuttlefish, and nautilus. There are over 800 species found in the oceans around the world ranging from shallow tropical water to deep sea at more than 5,000 m (Hanlon and Messenger, 2018). Their body size can vary from mere 1 cm to over 18 m in its total length and their brain to body mass ratio can be higher than that of some vertebrates (Packard, 1972). They can rapidly change their body pattern and shape to avoid predation and for inter, and intraspecific communication. Many can glow in the dark using bioluminescent ink to create their body double, cross-dress to deceive rivals during the mating season, move through the water column using jet Propulsion, etc. (Nixon and Young, 2003). The list of cephalopods' unique abilities and features continues on and on, not to mention that they also provide the essential protein source for many marine animals and humans alike (section Modern Cephalopod Science). Sperm whales are estimated to consume equal biomass of squid each year as the total annual catch of the world fishing industries (Vidal, 2014). Each one of these diverse abilities and attributes of cephalopods has fascinated people from diverse range of fields and disciplines for centuries.
In recent years, with the help of social media and Internet providing access to specialized information and growing interest in interdisciplinary academic collaboration fields, there has been increasing attention to cephalopods not only as model animals but also as a boundary object/subject connecting fields together. As examples, we show the organization of art exhibitions in conjunction with scientific conferences, the establishment of side-by-side collaboration between cephalopod behavioral scientist and art schools and military departments on topics such as camouflage technology, to end with sports fishermen working with scientists in a citizen science project. Although these fields are still in their infancy of interdisciplinary collaboration, the slow but assertive new developments in cephalopod research and culture have certainly began to transform the traditional paradigm of the cephalopod research. In this study, we attempt to capture this moment of transformation by revisiting the scientific development as well as to list and analyze some of the significant progress in the respective fields. In this way, we wish to capture the energy that will drive cephalopod research and culture in the twenty-first century.
History
Scientific Development
Messenger (1988) wrote that animals could be studied for two reasons: because of their inherent beauty or because they provide especially suitable conditions for tackling one particular problem of general interest. This issue was addressed in 1929 by the Nobel laureate August Krogh (1929) who formulated the following principle: “for such a large number of problems there will be some animal of choice, or a few such animals, on which it can be most conveniently studied” (later known as Krogh's Principle—see Krebs, 1975).
Cephalopods have played both roles in the history of biology and medicine; they provided answers and (more often) questions that have kept generations of researchers busy. Systematic observation of cephalopod structure and behavior can be traced back to Aristotle's The History of Animals, Book IV (ca. 350 BC) and, after a rather long eclipse, this knowledge was further developed during the Renaissance (Schmitt, 1965). In the early nineteenth century, cephalopods had a prominent place in the famous Cuvier-Geoffroy debate on comparative anatomy. This landmark confrontation in the history of zoology was spurred by Geoffroy's comparison of the cephalopods' internal structure to that of a vertebrate, the body of which is bent so that the pelvis touches the head (see Flourens, 1865; Packard, 1972; Appel, 1987).
By the end of the nineteenth century, the development of aquaria and marine stations, places where these animals could be kept alive for long, started the age of cephalopods as experimental animals. Inevitably, the period in which this development took place, and its specific, dominant “scientific atmosphere” have strongly influenced the way researchers have looked at them. Most cephalopods were subjected, thanks to the new infrastructure, to the professional gaze of the most diverse experimenters, psychologists, physiologists, zoologists, and, later, biophysicists. Whereas a few efforts were made (most notably by Jatta, Lo Bianco and Naef at the Naples Zoological Station) to expand our knowledge of these animals in their natural environment, it was the use-value of cephalopods in the laboratory that first defined them—notably, as “Guinea pigs of the sea” (Grimpe, 1928). This identity, duly separated from that of mythical monsters or allegorical representations that characterized their earlier relations with humans (see, e.g., Hugo, 1866; Lee, 1875), stuck for quite some time. Early in the twentieth century, cephalopods started to leave fairy-tales to massively colonize laboratory manuals for physiologists and physicians (Grimpe being a case in point, but see also von Uexküll, 1905). Octopuses, in particular, became a much sought-after preparation for the study of the effect of poisons, thanks to their excellent capacity for acclimatization, resilience to surgical interventions in comparison with sepia and squids and the long survival of their organs after extirpation. The study of regeneration of nerve and tissue also greatly profited from the “contribution” of cuttlefish and octopuses (Sereni and Young, 1932), as did the physiology of vision (Dröscher, 2016). In 1913, Wilhelm Fröhlich reportedly obtained the first electroretinograms ever from the eyes of Eledone and Octopus, which proved to be particularly suitable to the task thanks to their relative structural simplicity and the easy access of the recording locations. On the other hand, cephalopods turned out to be an excellent source of questions also in this domain: the debate on color vision in cephalopods spanned more than half a century (Messenger et al., 1973) before the necessary consensus was reached on their color-blindness (but see Gagnon et al., 2016; Stubbs and Stubbs, 2016). Physiologists and psychologists were also fascinated by the camouflage abilities especially of cuttlefish and the chances the chromatophore system afforded to study reflex responses following visual input. The reason behind can be found in the extremely fast response of the chromatophore organs following an eye-directed visual stimuli. This is due to the existence of a direct input connection between specific brain centers (the chromatophore lobes) and the chromatophores located overall the animal body.
Then, in the late 1930s, the Age of the Squid began. Their so-called giant axon—a syncytium, and as such an exception to the strict Cajalian rule of anatomical independence of nerve cells (Young, 1938)—was famously re-discovered by the zoologist John Zachary Young in 1936. The Marine Stations of Plymouth and the Woods Hole (Massachusetts, US) became hothouses for the introduction and development of this model, which was soon adopted by axonologists worldwide. In particular, the giant axon became the experimental model of the Cambridge biophysical school thanks to the work of Alan Hodgkin, Andrew Huxley (Hodgkin and Huxley, 1939), and Bernhard Katz, all of whom it helped to win the Nobel Prize. As Hodgkin later mused: “It is arguable that the introduction of the squid giant nerve fiber […] did more for axonology than any other single advance in technique during the last 40 years. Indeed a distinguished neurophysiologist remarked recently at a congress dinner (not, I thought, with the utmost tact) ‘It's the squid they really ought to give the Nobel Prize to” (Hodgkin, 1975, p. 16).
Young was also responsible for another significant turn in cephalopod laboratory history. After the war, and, initially, with the sole help of his assistant Brian B. Boycott, he started at the Naples Zoological Station an ambitious research program on the comparative study of memory. The idea was to set up a comparative study of the neural correlates of learning and memory in different classes. Octopus vulgaris is abundant in Naples and—as Young knew from his previous collaboration with the physician and physiologist Enrico Sereni—is a suitable animal for the laboratory experiments. Among its virtues were an enormous appetite (essential for behavioral experiments), a reportedly excellent learning ability, exploratory and aggressive behavior and a non-compact, “compartmentalized” brain. Moreover, it lacked any hard component, which made it easily accessible for the kind of research Young had in mind. The idea, following the standard defined by Karl Lashley in his work with mice, was to teach the animals a task (discrimination, or even problem-solving), then cut portions of their higher ganglia (purportedly controlling the more complex functions) and repeat the learning paradigm, with the hope of thus establishing correlations among the missing parts and the lost abilities.
It took Boycott 3 years to fine-tune the experimental system, and basically to single-handedly discover the needs, potentials, and problems of a laboratory octopus. By 1950, however, the system was in place, complete with a learning paradigm (a discrimination task: crab alone vs. crab + lead plate and shock), the preliminary functional anatomy of the higher ganglia and a vague but promising theoretical framework, based on vertebrate research (Boycott, 1954). The first full experimental report was published only in 1955 (Boycott and Young, 1955) but was encouraging. The octopus not only had kept all of its promises, (it learned fast, and a tentative association between learning and specific ganglia above the esophagus was presented)—it also had provided a few surprises. Most notably, Boycott and Young reported of a probable bi-partition of the memory storage, with two distinct (putative) neuronal circuits for long- and short-term memories, which at the time resonated incredibly well with the first studies of Brenda Milner on subjects. Boycott and Young were also the first to define the concept of a “memory system” (Buckner, 2007), a dedicated “neuronal net” devoted to the storage of memories. So did Octopus vulgaris begin a new career, that of “model of the brain” (De Sio, 2011). The early successes attracted new collaborators, who in turn helped to improve and diversify the learning paradigm and added to the growing complexity of the anatomical picture. Meanwhile, since the early 1950s Young was falling more and more under the influences of cybernetics. The comparative project became the octopus project, and the octopus progressively became a mechanical model—a living computer containing, rather than being characterized by, a memory.
The natural end-point of such a development was the attempt at building a learning machine based on what the researchers had learned about the performances and structure of the animal. In 1953, an electrical engineer by the name of Wilfrid Kenelm Taylor was hired and started the design and construction of a “feature detector” simplified retina made out of nine photocells, randomly connected to a whole wall full of electrical synapses, mimicking a part of an optic lobe. By 1956 the machine was in operation, or perhaps one should rather say it was undergoing education. It was, in fact, a wholly analog device, extremely plastic and fast-learning, but it required long training sessions, in which it was “shown” different pictures of human faces. In a relatively short time, it proved able to tell a female from a male face. It was also possible to have it “forget” things, by re-setting the electrical neurons, and then “re-learn” them. This machine later came to be subsumed under the wider category of “perceptron,” or neural networks and is rather part of the pre-history of artificial intelligence (AI) than of its history proper. The analog phase of AI research was in fact very short, with heavy, expensive and cumbersome machines being soon superseded by more efficient and economic purpose-developed software being run on all-purpose computers. It would take more than three decades for this avenue to be re-opened. At that time, however, the cephalopod research was about to take a different, more promising avenue: that of robotics and biomimicry, which has today reached its first staggering results (see section Fisheries, Conservation and Biodiversity and Camouflage Technology). The Octopus-perceptron was dismantled in the late 1960s and consigned to oblivion, but it had served its function. Despite the promises to his patrons (developing a learning computer) what Young had in mind was a comparative study of animal and machine learning, in which not only the animal could provide a blueprint for the machine, but the machine could also help in the interpretation of the structure-function nexus in the octopus. It was from this unlikely resonance that Young's famous model of the “mnemon” or memory cell, was born—the first selective model for memory formation (Young, 1964; Edelman, 1987; Changeux, 2006).
Modern Cephalopod Science
In the 1980's, cephalopod science took a big step. Cephalopods, which have rapid growth rates are abundant in the sea, were considered to be a vital source of protein to feed the increasing world population (Vidal, 2014). In order to create a more accurate stock assessment of cephalopods in the world ocean, seven scientists were gathered to investigate life cycle, population distribution, and species identification in 1983. This meeting, then, became the first the Cephalopod International Advisory Council (CIAC) meeting in 1985. Since then, CIAC meetings have grown to over 250 participants from diverse scientific fields including robotics, AI, neuroscience, behavior, and more (Fiorito et al., 2014). This meeting enlightened the multidimensionality of cephalopod research and provided a valuable platform to create a synergy of multiple fields. Among these, the most current and immediate frontier seems to lie in neuroscience, behavioral biology, and conservation. The rich and flexible behavioral repertoire supported by the well-developed brain, muscular structure, and circulatory systems drives and offers multivalent research opportunities to be explored.
Neuroscience and Cellular Biology
Cephalopods have proven excellent experimental models for a number of general problems in physiology, cell biology, and neuroscience (Abbott et al., 1995; Fiorito et al., 2014), including synaptic transmission (Bullock, 1948; Katz and Miledi, 1970; Armstrong and Bezanilla, 1973) and neural control of behavior (Nixon and Young, 2003). They have evolved a specialty in cognition among invertebrates and even more interestingly a “different” type of brain centers and decentralized decision making areas at many levels of their body including the arms (Hochner et al., 2006; Zullo and Hochner, 2011; Mather and Dickel, 2017). Despite the utterly unique brain organization, Edelman et al. (2005), for example, emphasized that the brains composed of about 170 million neurons, complex sensory receptors, and motor control systems are the obviously comparable situation to its vertebrate counterparts. As a representative case, the cephalopod brain analogous to the reentrant loops of the thalamo-cortical system will be a landmark as a conscious system of the mammalian and birds.
Furthermore, the possibility that they might have personality has been suggested by a series of investigations where the general behavior of animals held in captivity and their reaction to the environmental threats have been carefully observed. The neural basis of personality is largely unknown, but these studies have nicely shown that animals respond to the threats differently (for example interacting, hiding, or escaping the stimulus) following what seems to be an exclusive “personal” variability (Mather and Anderson, 1993, 1999; Mather and Carere, 2012). In addition to this, cephalopods seem to have a simple form of consciousness adapted to their behavioral abilities such as environment navigation, requiring a form of self-awareness possibly similar to that vertebrates and insects, motor control of highly flexible arms and, eventually, to their potential social interaction (Figure 1). In light of this, a test such as the mirror self-recognition test (MSR) has been conducted with cephalopods to measure their ability to visual self-recognize (Ikeda, 2009; Figure 1). Taken together, this makes them interesting animals with big brains in comparison and contrast to vertebrates for the study of evolution (Mather and Kuba, 2013).
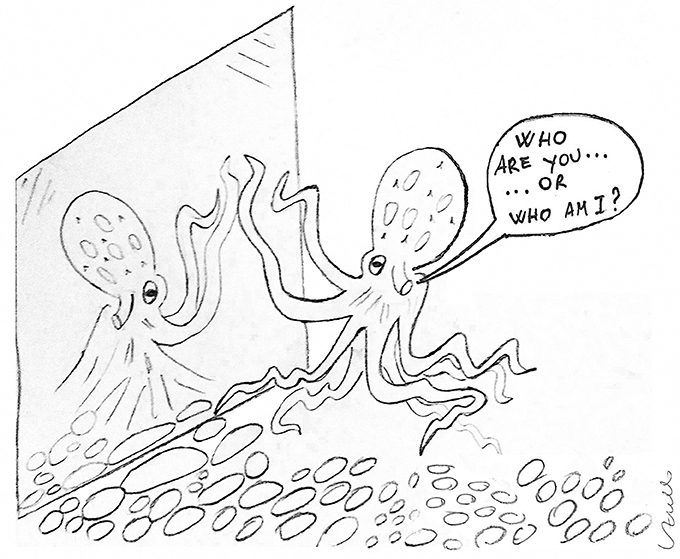
Figure 1. What consciousness in Cephalopods? Sketch representing the Gallup's mirror test technique applied to an octopus to assess its visual self-recognition abilities (drawing by L. Zullo).
Behavioral Ecology and Biology
Beyond the traditional knowledge of cephalopod neuroscience and behaviors as summarized in Wells (1978), Mangold (1989), Abbott et al. (1995), Hanlon and Messenger (2018), and Borrelli et al. (2006), recent cephalopod behavioral studies continue to provide many interesting discoveries. Researchers have found new cues for cephalopod novelty or intelligent behaviors, including new body chromatophore coloration, light sensing skin, observational learning, human-like arm use, mimicking, developmental cognition, sociality, and possible tool use and play (Hochner et al., 2006; Zullo and Hochner, 2011; Darmaillacq et al., 2012; Mather and Dickel, 2017 for reviews). These behaviors are supported by a complex and well-developed sensory system that possibly integrates a variety of information coming from different sources such as visual system, motor system, etc. (Budelmann, 1995; Zullo et al., 2009; Hanke and Osorio, 2018).
Moreover, we have to consider that cephalopods are worldwide-distributed animals and can occupy almost all kind of marine habitats, an aspect that is reflected in the incredible number and diversification of existent species. Given this ecosystem diversity, any researcher studying cephalopods can face a wide variety of problems connected to the animal collection and lifestyle along with having access to a number of different, and fascinating, scientific questions.
As an example, exploring the behavioral ecology of deep-sea species has long been challenging, but Kubodera and his team became the first to capture photos of the live giant squid and to observe its active pre-capture behaviors (Kubodera and Mori, 2005). The mysterious vampire squid, Vampyroteuthis infernalis lives in extreme deep sea conditions and, unlike most cephalopods, it has been shown to have multiple reproductive cycles (Hoving et al., 2015). Furthermore, a deep-sea incirrate octopus has been shown to breed big eggs almost for 4 years, the longest in any known animal (Barratt et al., 2007; Robison et al., 2014). Another interesting aspect of mesopelagic and deep-sea species that live across a broad range of depths ~1,200 m is their adaptability to aphotic (lightless) depths and a new strategy of visual adaptation has been recently revealed (Chung and Marshall, 2017).
Fisheries, Conservation, and Biodiversity
Differences in the behavioral ecology of cephalopods are well represented also in other fields such as that of fisheries. Cephalopod fishery science has been continuing to focus on life history, population dynamics, and stock assessment of commercially important species in the ever-changing state of world oceans. Since Malcolm Clarke estimated that sperm whales consume 100 million tons of squid per year, this task has been challenging to accomplish due to oceanic nature of the target species. In light of this, currently, an ecosystem approach to fisheries combining biological information, taxonomy, biogeography, systematics, annual sampling, and oceanographic data has become the desired method for stock assessment and monitoring (Rodhouse et al., 2014). In addition to interdisciplinary data analysis, various tagging methods have been used to track migration pattern, movement and distribution in species such as Caribbean Reef squid, Humboldt Squid, Short finned squid, Arrow squid, Chokka squid, Japanese flying squid, and more. Furthermore, creating economically viable aquaculture for the Common octopus, Octopus vulgaris has also been a significant goal of fishery science, ecology, and conservation (Iglesias et al., 2004; Navarro et al., 2014).
The extensive commercial fishery for cephalopods started in 1950. According to FAO, total annual world catch of cephalopods was 750,000 tons in 1961 and has increased to 4 million tons in 2013. Although the total number is growing due to jumbo flying squid, Dosidicus gigas, harvest in the East Pacific, cuttlefishes are showing steady to slight decrease (FAO, 2016). More significantly, the annual catch of commercially important species as Japanese flying squid, Todarodes pacificus has plummeted from 444,000 tons in 1996 to 67,800 tons in 2016 (MAFF, 2017). With increasing threats due to ocean acidification and climate changes related to human activities, monitoring marine food chains is now an urgent task. Cephalopods exhibit rapid growth, short lifespans, and strong life-history plasticity, allowing them to adapt quickly to changing environments. Also, related to these fields, cephalopod fisheries and aquaculture have traditional importance as a food source, and recent advancements were reviewed in Iglesias et al. (2014) and Vidal (2014). Hence, studying cephalopod ecology, biodiversity and conservation occupy a unique position in marine science (Boyle and Rodhouse, 2008; Doubleday et al., 2016).
There has been a rapid technological advancement of molecular tools and powerful next-generation sequencers to perform species identification. DNA barcoding, mitochondrial and 16S rRNA sequences, for example, offered rapid species assignment and provided significant potential for species identification and biodiversity (Dai et al., 2012). Analysis of environmental DNA is also a powerful tool to estimate large-scale biomass and ecological niche from a limited seawater sample by using a highly sensitive quantified PCR technology (Mauvisseau et al., 2017). Also, natural history studies of cephalopods have been based on the molecular methodological tools available. After the first publication of a cephalopod DNA in 1983 (Walker and Doolittle, 1983), understanding of the cephalopod phylogeny and classification has advanced through the comprehensive approaches of mitochondrial and nuclear genomics, and transcriptomic multi-gene sequence analyses (Allcock et al., 2015; Lindgren and Anderson, 2017; Uribe and Zardoya, 2017).
In addition to the technical advancement of molecular tools and sequencers, the rapid growth of information technologies and related infrastructures has changed the methodology employed in cephalopod studies over the past 20 years. Advanced research vessels, submersibles, physical sensors, acoustic transmitters, and observing systems are now mutually combined with computer networks to investigate deep-sea cephalopods (Hoving et al., 2014). For example, monitoring seasonal habitat changes of deep-sea benthic cephalopods, a novel Internet Operation Vesicle, a benthic crawler was used. This vesicle was connected to the NEPTUNE cabled infrastructure operated by Ocean Networks Canada (Doya et al., 2017).
The worldwide global networks are now dramatically changing our communication tools through the website and multi-institute administered databases, i.e., the World Register of Marine Species (WoRMS) has provided the most authoritative data since its launch in 2007. Not only the specialists in the fields, scuba divers, nature enthusiasts, sports fishermen, and many others can now mutually share the cephalopod information via social network systems such as Facebook, WhatsApp, Instagram, Line, Twitter, Flickr, and Youtube. As users of social network systems and their daily activities have blurred the boundary between social media and the traditional web, the phenomenon has become a great influence over scientific communities. Indeed, scientists can significantly benefit from the prompt availability of a massive amount of data in the form of images, videos and data records of cephalopods in the wild.
Genomics and Molecular Biology
Twenty years ago, our ability to study cephalopod genes and proteins homologous to other model animals were limited, but recent advances in high-throughput techniques including next-generation sequencing, as stated above, enable us to profile molecular data from a number of species rapidly (Albertin et al., 2012; Liscovitch-Brauer et al., 2017). Indeed, molecular data in cephalopod transcriptomes indicate that key neurotransmitters and regulatory genes are present similarly in the tissues of vertebrates, insects, worms, and other marine invertebrates. There is a minor variation, but no obvious evidence exists for systematic expansion of neurotransmission gene families between octopus and vertebrate genomes (Albertin et al., 2015). These similarities are interpreted as evidence for evolutionary conservation inherited from a common ancestor. For some examples, studies of DNA-binding homeobox domain Pax6 (Tomarev et al., 1997) and Hox genes (Lee et al., 2003), each of which showed evolutionary conservation with those of vertebrates and insects for the evolution of eyes or whole body. By analyzing such evolutionary conserved developmental control genes, biologists are now challenged to understand how cephalopod brain and body are organized.
In a paper on the octopus draft genome, Albertin et al. (2015) provided evidence that the genome size of Octopus bimaculoides was comparable to the 3 billion base pair human genome and there was no evidence for whole genome duplication as in vertebrates. As notable cephalopod novelties, they found dramatic diversification of kinds of genes including the C2H2 superfamily of zinc finger transcription factors, protocadherins, interleukin 17-like genes, RNA editing, and elevated transposon expression in the neural tissues that may produce a genome rearrangement as seen in Hox gene complement unusually splitting into nine clusters in the octopus. Interestingly, Garrett and Rosenthal (2012) found that transcribed messenger RNAs of K+ channel gene are extensively edited in Antarctic and Arctic octopuses compared to those of tropical species, creating the functional diversity of ion channels to accelerate gating kinetics greatly. Namely, they showed that adenosine-to-inosine RNA editing can respond to the cold-water environment. Furthermore, Yoshida et al. (2014) showed splicing variants of developmental genes that display unique features in cephalopod eye evolution, and Liscovitch-Brauer et al. (2017) discovered a cephalopod specific novelty, exhibiting extensive RNA editing of squids, cuttlefish, and octopuses. Cephalopods may have transcriptome plasticity via RNA editing in evolution beyond genome.
Cephalopods genomic complexity has to be seen not only as a strategy developed to allow survival in different habitats and various lifestyle. As highlighted in the previous section genome together with body shape and environment co-evolved to generate animals that are unique also in their nervous system and the control architecture of body and behaviors. It is indeed the flexibility that they demonstrate at several levels of their biological organization that in the last decades fed another far-related discipline, that of engineering and in particular of soft robotics.
Soft Robotics
Soft robotics is a booming field that has attracted significant research interest in the last decade, because of the potential of soft robots to better interact with real-world environments. When, in March 2014, the scientific journal “Soft Robotics” was launched, the editor commented: “By building soft materials into the fundamental design of machines, or by building them completely from soft materials, we add a new dimension for design and create an untapped resource for entirely new types of machinery” (Trimmer, 2014).
Soft robots are devoid of rigid components and have several mechanical advantages over classically structured robots such as the ability to squeeze, stretch, and stiff (Laschi et al., 2016). They can operate in unstructured environments and, due to their inherent and modifiable compliance, they can perform operations across a wide variety of substrates and environments (Wang et al., 2015). Soft-robotics stands on “a completely different way of building robots,” and instead of vertebrate, the octopus (and generally cephalopods) can be used as a natural template to learn from. Cephalopods are explicitly and repeatedly mentioned as a natural template for soft robotics. Indeed, cephalopods are currently an important source of inspiration for many bio-roboticists and material scientists due to the interesting characteristics of softness, robustness, environmental adaptability and control mechanisms of their body (Figure 2). Rus and Tolley (2015), for example, wrote in their review paper on soft robotics: “Cephalopods, for example, achieve amazing feats of manipulation and locomotion without a skeleton […]. Inspired by nature, engineers have begun to explore the design and control of soft-bodied robots composed of compliant materials.”
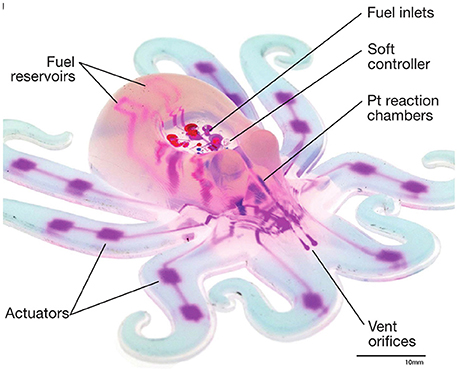
Figure 2. The design of the so-called octobot is taken from the octopus. It is a fully soft, autonomous robot that is controlled via the embedded microfluidic soft controller and powered by monopropellant decomposition. Scale bar, 10 mm, Source: Wehner et al. (2016). The figure is reproduced with the permission of the copyright holder [Springer Nature].
Cephalopod limbs are muscle hydrostats; they are almost entirely composed of muscle and connective tissue used both for force production and as structural support. They can bend in any direction and change the stiffness at any point of the entire arm length. Hence, they offer a valuable model to study two fundamental properties (and challenges) in soft-robotics that are: (1) the modulation of stiffness and (2) the position control (Figure 3). In one word, they are “hyper-redundant” structure whose control and coordination present a dramatic computational complexity. To date, several aspects of their behavioral repertoire have been addressed both for computational modeling and Artificial Intelligence (AI) and for the development of robotic prototypes (Guglielmino et al., 2012, 2013; Nakajima et al., 2015). Among others, propulsion swimming, single and multiple arm manipulation, crawling and exploratory behaviors and sucker attachment have been studied in more detail.
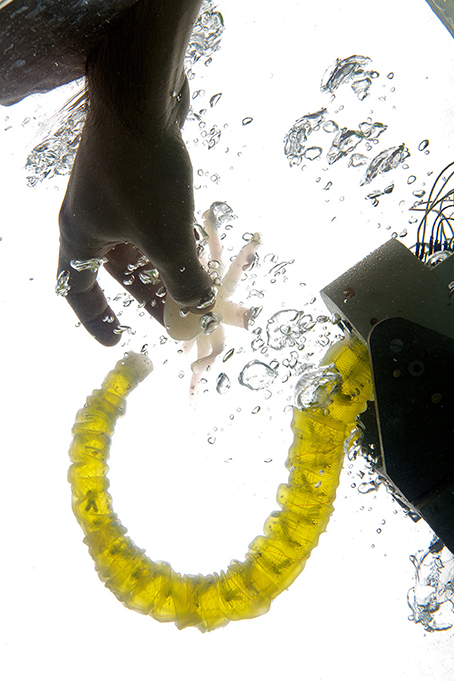
Figure 3. The Lighthouse: prototype of an artificial bio-inspired arm developed during the EU OCTOPUS Integrating project, 231608 (photograph by Massimo Brega).
Recently developed soft-robots prototypes have a wide variety of functions and application spanning from robotic gripper for minimally invasive surgery, to soft-robot underwater exploration and even soft manipulators for assistive human care (Calisti et al., 2015; Krieg et al., 2015; Shen, 2016). The last application takes advantages of the high compliance and dexterity of soft manipulators, which ensures safe human-robot interaction (Ansari et al., 2017). Given the complexity of soft-robotics, results have been possible only through the cross-integration of diverse expertise coming from neuroscientists, engineers, material scientists, and computational biologists, thus setting the bases for a “melting pot” between biology and soft-robotics engineering. Altogether, these investigations have highlighted the potential for soft machines as well as the drawbacks of the available technologies and the limitation in the current knowledge of cephalopods intelligence and motor control strategies. In particular, it has clearly emerged the need for new materials that embed together softness and robustness and of new control strategies for these deformable materials.
Most importantly, they provided a proof of concept of the existence and modes of operation of an “embodied intelligence.” This term was originally conceived to describe autonomous robots to explain how their efficiency derives from the interaction between the controller (the brain or actuation system), the mechanical system (the body artifact) and the testing environment (Pfeifer et al., 2007). Whilst deriving from a robotic field, this terminology has been lately adopted by biologists to underpin the existence of a self-contained intelligence within each animal body. In this case, embodied intelligence stands for the co-evolution of animal body/nervous system and environment as a result of natural selection (Hochner, 2013).
Octopus and more generally (shell-less) coleoid cephalopods are living examples of this concept.
Camouflage Technology
Cephalopods have been a source of inspiration in the robotic field also for another important behavioral capability, their amazing capacity of modifying their appearance and their body pattern in response to a variety of different stimuli (Osorio, 2014; How et al., 2017). This aspect has drawn the attention of engineers and material scientists aiming at developing biomimetic artificial skin able not only to match its background but also to fast adapt to a changing environment, all this, without losing flexibility. Few interesting prototypes based on electroluminescent material have been developed taking inspiration from cephalopods skin. For example, researchers from Cornell University have recently produced a synthetic skin able to emit light while undergoing large stretching and surface area modifications. In the latest version, this stretchable surface has been provided with the ability to change “on demand” both color and texture thus transforming from 2D to 3D shape just like it happens in cephalopod skin following environmental stimuli or communication needs (Larson et al., 2016; Pikul et al., 2017).
Although these prototypes are remarkable in their ability to change their appearance, we believe we are still far from reaching an active cephalopod-like camouflage system. This should not surprise as camouflage is a feature of immense complexity and, despite many decades of investigation, we are just at the start of our comprehension of the biology behind cephalopod visual-spatial perception and accustomization to the surrounding environment. To make things even simpler, recent investigations started disclosing the existence of independent mechanisms of control of the skin pigmentation based on solely “skin perception” of environmental illumination (Kingston et al., 2015; Ramirez and Oakley, 2015). These studies showed that the primary elements of pigmentation in cephalopods, the chromatophore organs, can be light-activated in a manner completely independent of the central nervous system. Interestingly enough this process seems to be based on a common and conserved molecular mechanism of light photo-transduction between the eye and the skin. But, despite the mechanisms underlying the formation of pattern and texture the unique and exquisite endpoint of the chromatophore marvelous machinery is the generation of ordered images and eventually the arousal of “beauty.” We can definitely state that these animals carry a high aesthetic value, and this has been caught early by our ancient predecessors.
Art
Historical Representations of Cephalopods
Cephalopods are present in the art of many a coastal culture around the world. During New-Place Phase II (LM IB) to Post-Palace Phase II (LM IIIA) of late Minoan civilization (c. 1550–1100 BCE), representations of Cephalopods in pots, coins, thumbs, pendants, etc., are ubiquitous (Figure 4, octopus pendant and a large octopus pot). Such Minoan representation of cephalopods is roughly categorized into two different styles separated by the era, Marine Design style of around 1500 BC and Palace Style of between 1450 BCE and Mycenaean invasion (Betancourt, 1985). At first glance, Marine style octopus vessels seem formally and technically primitive with simplified and abstracted features such as googly eyes over an exaggerated mantle, very long and extended arms. These abstracted features create somewhat of a comical impression and familiarity of infantile art. However, with careful examination, one would realize that these images are not a product of nonchalant and causal relationship to the animals rather they are a product of careful and deliberate observation of both form and behavior of the animals. For instance, An octopus represented on a large vessel depicts regenerated arms, a biological attribute used as a symbol of regeneration and rebirth. In another example, a hectocotylus, a specialized arm to transfer spermatophores to the reproductive tract of a female's mantle cavity has been described in a small gold pendant top as a symbol of fertility. These careful observations of morphology and behavior, seem to have supported the symbolic use of octopus in Abstract Design style where octopus design was not a mere representation of an animal but a codified cultural signifier.
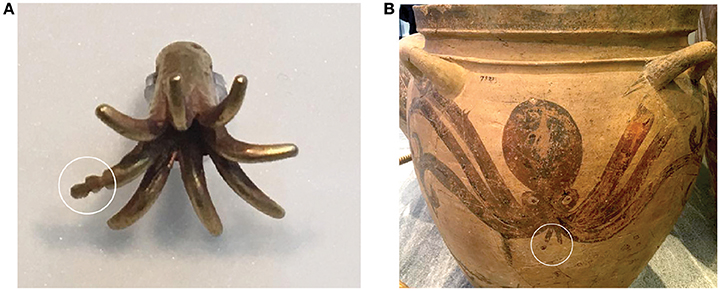
Figure 4. A gold octopus pendant is showing a hectocotylus (A), and a large octopus ceramic pot is describing two regenerated arms (B). Collections of Heraklion Archaeological Museum, Crete Greece (photographs by R. Nakajima).
While there are many different manifestations of cephalopod motifs, there is one distinctive feature that is shared among many, the gaze. These octopus designs look straight at the viewer with two large eyes simultaneously recognizing both viewers and its existence. This exchange builds psychological dimension that includes both a sense of mutual recognition and self-awareness. The Ancient Greek artisans have successfully represented this rather abstract and enigmatic character of cephalopods and our metaphysical relationship with them.
In addition to the historical representation of cephalopods through arts and crafts across the cultures from Roman mosaic to Katsushika Hokusai's woodcut print, there are many notable accomplishments in scientific illustrations that sparked people's imagination. Cephalopoda is the volume 18 of Wissenschaftliche Ergebnisse der Deutschen Tiefsee-Expedition auf dem Dampfer “Valdivia” 1898–1899 written by Carl Chun. The book contains over 100 color and black and white plates visually describing many deep-sea cephalopods including the first image of the vampire squid, Vampyroteuthis infernalis. The illustrations provided in the book are not only scientifically accurate descriptions of taxonomically essential details but also show aesthetic and formal considerations. In contrast to Chun, the work of Ernst Haeckel is biologically less accurate but highly designed with an acute sense of formal concerns such as form, line, and color. Haeckel with much fascination for symmetry organized composition and design based on the overall page layout with overtly elongated tentacles and perfectly composed arms of octopuses with symmetry and counter symmetry. In addition to the 2-dimensional biological illustrations, glass models by Leopold and Rudolf Blaschka are products of extraordinary craftsmanship and care. Using transparent properties of the glass, Blaschkas were able to represent more than 700 different species including squid and octopus, which are collected at institutions around the world. Although these late nineteenth to early twentieth century scientific artifacts do not share the level of conceptual and philosophical foundation of their contemporary artists, the level of craftsmanship and aesthetic considerations produced a vital foundation in cephalopod visualization culture.
Japanese had a different take on octopuses representation. In 1814, Hokusai, a Japanese woodcut printer produced one of the most famous Shunga (erotic prints) titled The Dream of the Fisherman's Wife. Unlike his more mainstream series such as Thirty-six Views of Mount Fuji, this is an illegal and underground operation depicting an Ama diver sexually entangled with two octopuses. This rather odd sexual fantasy has made such a profound impact on the Japanese psyche that such notable contemporary Japanese artists as Makoto Aida, Masami Teraoka, Namada, Yuji Moriguchi have made homages to it. In addition to these fine art homages, the print has also affected Japanese pornographic anime. Toshio Maeda was the first to introduce in 1987 this type of representation to avoid the strict censorship law in Japan which banned all representation of genitalia. Maeda used tentacles to replace both male genitalia and bondage expression. Urotsukidoji, which was produced initially as a two-volume comic book, has expanded into 15 video series, two featured movies, video games, and special feature books. Since then the release of the original series, “Shokushu (tentacle)” has become one of the dominant genres in Japanese pornographic and non-pornographic anime alike.
Cephalopods in Contemporary Art
Cephalopods remain popular motifs in contemporary art and continue to draw public attention. Takashi Murakami's solo exhibition entitled The Octopus Eats Its Own Leg at the Museum of Contemporary Art Chicago, which featured over 50 sculptures and large-scale paintings, has broken attendance record in the MCA's 50-year history. More than 193,000 people attending the exhibition came to see Murakami's “Superflat” Japanese pop anime, subculture combined with traditional imageries, including many Octopus-inspired characters. The exhibition at Qatar Museum “What About the Art?” curated by Chinese artist Cai Guo-Qiang featured work by Huang Yong Ping's six-ton giant “sea monster” hanging from the museum ceiling wrapping its arms on the large 20-foot Column overpowering the audiences walking underneath. A Japanese painter, Yutaka Mukoyama who paints various marine animals, has been creating incredibly detailed photorealistic oil paintings of squids, which are stunning and mesmerizing. All of these are just fragments of cephalopod inspired art that is produced in the recent years that can be found in the ever expansive artistic realm from artworks in high profile art museums and galleries to the street of San Francisco bringing and nurturing people's curiosity and interests toward cephalopods (Figure 5).
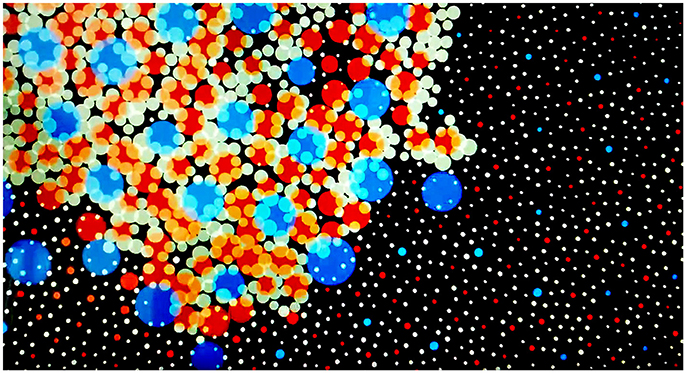
Figure 5. This is a still image of an interactive video installation “Chromatophore simulator” by Todd Anderson. The image is provided at courtesy of the artist.
In addition to plastic art such as paintings, drawings sculptures and other traditional mediums, cephalopod motifs have been used in many diverse modes of representation, from an underwater site-specific installation of an 80-foot Kraken sank to the British Virgin Island Seafloor (BVI ART Reef, http://www.divethebviartreef.com) to a rideable large-scale kinetic squid sculpture at Les Machines de l'Île de Nantes (http://www.lesmachines-nantes.fr/en/). While many cephalopods themed artworks have been produced since the time of the ancient Greeks, there are three notable artworks that may represent possible future direction in Cephalopod art as synergy of art and science, Insane in the Chromatophores by Backyard Brains, Chromatophores simulation system (Figure 6) by Todd Anderson and Octopus Brainstorming: Empathy by Victoria Vesna and Mark Cohen. Insane in the Chromatophores was produced in collaboration with Dr. Roger Hanlon's laboratory at Woods Hole Marine Biological Laboratory. This project connects iPhone music to living tissue of a Longfin Inshore Squid (Doryteuthis pealeii) by electrodes. As a result, electric signals of the music contract radial muscle fiber surrounding chromatophores allowing a rhythmical change in the visual appearance of the squid synchronized with the music. In Anderson's Chromatophores simulation system, is an interactive digital simulator of chromatophore movement and change in colors. Anderson's simulator detects the movement of spectators and moves color dots based on an algorithm of cephalopod chromatophores. Finally, Octopus Brainstorming: Empathy is a performance/installation that allows viewers to visually detect performer's thoughts through octopus-like contraptions worn by them. Although these three projects are technically and conceptually different, all of these projects focus on multiple characteristics of cephalopods and represent an innovative fusion of cell biology, neuroscience, computer programing, video, sculpture, performance, and more, thereby expanding the share notion on artistic and creative practice and scientific investigations alike.
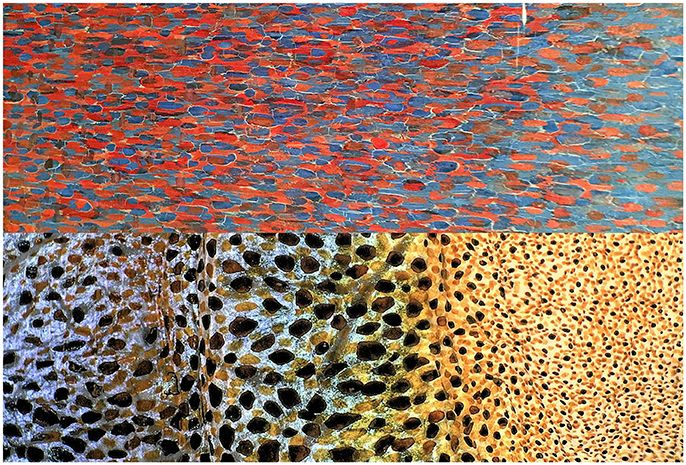
Figure 6. Image comparison of detail close up of painted frame of Georges Seurat's painting View of Le Crotoy from Upstream (1889), oil on canvas, collection of Detroit Institute of Arts (Top) and octopus skin (Bottom) showing the dense distribution of chromatophores and iridophores. Note: (1) A momentary flash of an anesthetized octopus multi-color skin that was taken with a next-generation KEYENCE digital VHX900F microscope and a 20 Å~ greater depth-of-field VH-Z00R lens under a multi-angle observation. Photographed by S. Shigeno. (2) The detail of Seurat's painting frame was photographed by R. Nakajima at Detroit Institute of Arts in 2017.
The synergy between science and art has been a part of artistic practice, perhaps, since Leonardo Da Vinci's extensive notes on art, biology, and engineering. Other examples may include the use of camera obscura by Johannes Vermeer, hyper-detailed animal illustration paintings of Jakuchu Ito, highly aestheticized biological illustrations of Ernst Haeckel. In 1960's the rise of American Avant-garde movement that explored many avenues of non-traditional art making has drastically opened the door for more conscious and deliberate use of scientific practices in art. Artists such as Harold Cohen (http://www.aaronshome.com/) who pioneered in integrating AI “AARON” system to study the process of painting, Helen Mayer and Newton Harrison (http://theharrisonstudio.net/) who have combined environmental science, agriculture, activism and art, Nam June Paik (https://americanart.si.edu/artist/nam-june-paik-3670) who explored video texture, have all helped firm down the foundation for the interdisciplinary approaches to art making that evolved into current New Media Arts including Biology Inspired Art practices. These multiplicities not only gather independent fields together but also generate interests and discovery in an unexpected category of audiences and help expand the possibility of each area. As more traditional creative modes such as paintings, sculptures, ceramics, prints, photographs will continue to be active, these contemporary interdisciplinary approach truly removes many boundaries not only between arts and sciences, but also help create deeper mutual understanding between the two areas that provides a tangible platform for intellectual exchange expanding imagination, creativity, and vision (Figure 7).
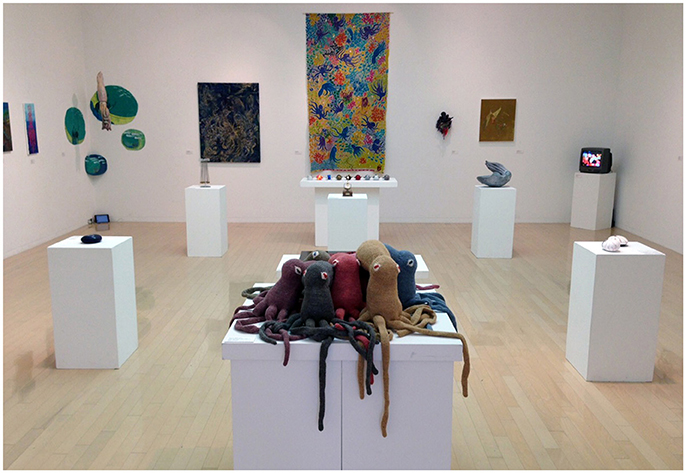
Figure 7. A cephalopod themed art exhibition Cephalopod Interface was organized at the Okinawa Prefectural Art Museum in conjunction with an interdisciplinary cephalopod symposium held at the same location (photographs by R. Nakajima).
Cephalopods in Popular Culture and Media
High art is not the only place for representations of cephalopods. They can be found in various corners of popular culture including movies, animes, illustrations, toys, video game characters, and more. In 1981, Nintendo released Octopus on a line up of their Game Watch that sold estimate of 250,000 to 1 million copies worldwide. In 2015, Nintendo released Splatoon, which sold 4.87 million copies in just 2 years, and is till now the top-selling video game designed for home console. Cephalopod figures and toys are sold at most aquariums and seaside resort gift shops to be collected (Figure 8). In movies, the five versions of 20,000 Leagues Under the Sea (1907, 1916, 1954, 1985, and 1997), might be one of the most extensive series with a giant cephalopod, which has been adapted from a novel by Jules Verne. More recent films such as Finding Dory (2016), Pirates of the Caribbean, At World's End (2007), SHARKTOPUS (2010), Mega Shark vs. Giant Octopus (2009), and Leviathan (2016) also feature cephalopod as a dominant element in their narrative and many others allude to it.
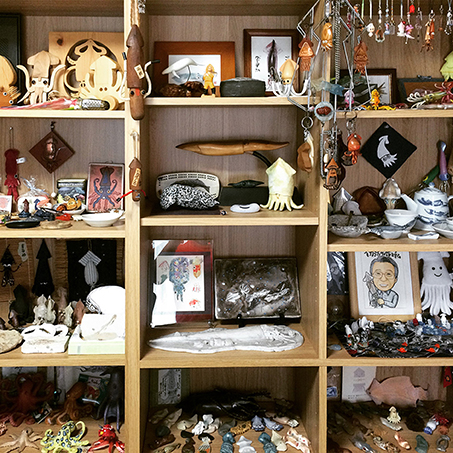
Figure 8. A part of cephalopod figure collection of Dr. Yasunori Sakurai at his home office showing incredible variations in cephalopod character designs (photographs by R. Nakajima).
The original Star Wars (1977) invested 6 min sequence where Luke Skywalker, Han Solo, Princess Leia, and Chewbacca are trapped inside a garbage chamber battling with Dianoga, an octopus-like monster. The sequence starts with a statement by Han, “I am beginning to like her (Princess Leia)” to Luke who is his rival over the princess and the sequence ends with the first embrace between Han and Leia. George Lucas cleverly sets up this intergalactic romance by locking up two knights and a prince in a dungeon with a giant octopus. While Luke busies himself with his drone friend C3PO, Han continues to fondle Leia as trash compressor push them closer to each other. This, one of the most memorable and cinematic love scenes of the first Star Wars trilogy, references the legacy of the myth of Kraken as an embodiment of sublime forces of nature but also carefully fuse the story of Saint George and the Dragon with it in the narrative structure.
One other contemporary example of the legacy between myth and public interest can be found in the following happening that in 2010 took the appearance of a modern fairytale. During the FIFA world cup Germany 2010, a common octopus, Octopus vulgaris exhibited at Sea Life Center in Oberhausen, Germany was stealing the show as an animal oracle. During the tournament, Paul the octopus predicted the winning results of 10 out of 12 matches. Scientifically, an octopus selecting or not selecting one over the other is an interesting issue in terms of pattern recognition and color discrimination. However, here the interest is more metaphysical. Despite all religious differences in the world, people were mesmerized by the fact that this little sea creature was exercising its' “supernatural power” that is beyond natural human capability. The Octopus with the name of one of the most important patrons Saint Paul is prophesying the outcome of an important sports event that impacts the social, economic, cultural, and emotional well-being for millions of people. Paul, with much higher success rate than the best bookies in the world, was transformed from an invertebrate to a prophet. This happening together with the international impact and debate arising from it, has been wisely narrated in the much enjoyable documentary “The Life and Times of Paul the Psychic Octopus” (2012) by Philippe (2012).
The concept that the uncertainty of nature can only be accessible and comprehensible by counter parting it with its own natural force is very similar to that of ancient Greek or Chinese oracle and other Paganistic and shamanistic practices. The only difference here is that without any shared religious and social foundation, the media frenzy has recontextualized a marine invertebrate into an autonomous being with a superior consciousness that is directly communicating its own thought with its own logic. By stepping on the podium of predictor animals, the octopus was the prophet and not an instrument of ritual that required an interpreter and many have accepted it even with slight hesitation. Through news media, music, dance, movies, photographs, illustrations, Internet, articles, and all the other traditional and modern information dissemination methods, the octopus spoke and we listened. This seeming absence of mediator between the octopus and the people made an ordinary hunting behavior into a modern Totemism creating a temporary yet significant universality.
Cephalopod science has been active in the mass media increasing public interest in cephalopods. In 2012, a group of scientists led by NHK (Japan Broadcasting Corporations), and Discovery Channel successfully filmed a giant squid, Architeuthis dux in its natural environment. Combining social network systems and the major mass media sources, the news reached millions of viewers worldwide offering a sense of natural wonder, a joy of discovery and entertainment. The special exhibition “Deep-Sea” organized in conjunction with the release of the footage at The National Museum of Nature and Science Tokyo became the most visited exhibition in Japan in 2013 well exceeding Raphael, El Greco and J.W Turner bringing over 600,000 visitors in 86 days. Not as catchy as the news of giant squid, media coverage of the first complete sequencing of octopus genome published in 2015 was unique. The story was covered in 136 news articles following the initial press release by Okinawa Institute of Science and Technology Graduate University 64 out of 136 articles alluded to the idea of intelligence and/or octopus being an alien promoting the idea of octopus as an intelligent being comparable to human. By bringing Kraken to reality or by promoting the existence of extraterrestrial being and its potential intelligence, cephalopods science, and the media seems to be able to draw and trigger public attention. This unique characteristic of cephalopod helps build a useful information dissemination platform that brings public closer to nature, science, and culture promoting interdisciplinary and multivalent understanding.
Current Challenges
With ever-expanding horizons of cephalopod research brought by increasing numbers of direct and indirect associations to cephalopods and their characteristics, the relational dynamics between different fields is facing new challenges and opportunities. The current academic atmosphere is still carrying many aspects of later twentieth century necessity to subdivide academia into the smallest possible units without many lateral interactions with other disciplines (see Fiorito et al., 2014 for example). Due to this structural and philosophical division, interdisciplinary is still in its infancy. It is not so easy to find the time, place and occasion to have in-depth discussions with others. Moreover, the difference of financial standing between science and humanities is so large that it might represent a difficulty for researchers in art and scientists in forming an equal partnership. The development of an interdisciplinary mindset, in particular within the scientific community, might overcome some of these practical limitations. This might account for, as an example, support and easier access to cephalopod aquaculture facilities and marine stations to non-scientific investigators where they could meet live cephalopods and encounter investigators from various disciplines.
This aspect is particularly important if we consider that there are only very few laboratories in the world that regularly house cephalopod species that can extensively serve both science and art community. Cephalopod aquaculture is extremely time-consuming and costly, and only a few species are currently cultured on a small-scale due to several bottlenecks in their culturing system (Iglesias et al., 2014). Limiting factors are represented by, to mention a few, their low reproduction performance and fecundity in captivity (for some species), the broodstock management, the need for optimization of hatching efficiency and the absence of appropriate diets for each life stage and the consequent occurrence of cannibalism or massive death, etc…. These traits are unfortunately accompanied by the elevated cost of maintenance of the reproductive and spawning tanks in term of infrastructure, manpower, space, and even running costs. Taken together the biological and economic aspects represent a relevant obstacle for the expansion of cephalopod aquaculture over the world especially when the outcome does not directly represent a profitable end-product as it is the case in cephalopod open-sea aquaculture.
To maximize the potential of the interdisciplinary, it is imperative to create a stronger lateral relationship between the fields. By combining art and science, it will present a certain solution to raise awareness about cephalopod science. The urge for scientists to communicate and to engage with the public about their research, and the relevance and social implication of their findings both increase. Also, to explore further understanding and stimulate inspiration, it will become increasingly important to bring art and science together. Considering the current trends in cephalopod research, it may become a useful model for other fields to achieve its full creative, emotional and intellectual potential.
Conclusion and Perspective
Cephalopods, especially the octopus, have fascinated humanity since the ancient Minoan, Greek and old Chinese high cultures. With their large eyes and seemingly smart behavior, capable of learning and planning into the future, cephalopods trigger a strong fascination in scientists and lay people alike. Their complex brain and behavioral repertoire have evolved independently of all vertebrates including humans and show how evolution can independently lead to comparable central nervous systems. In this review, we found that such unique cephalopod features have provided a strong influence on scientists and engineers. Cephalopods are animals endowed with a well-developed brain that controls highly flexible appendages. This unique control strategy has been used by bio-roboticists as templates for the design of a new type of adaptable machines able to dynamically interact with a changing environment and “learn” from it just like animals do in the natural world.
The similarities and differences between cephalopods and humans are a rich source of wonder, fascination, and inspiration. Nowhere else has this sentiment been better captures than in Flusser and Bec's (1987) book “Vampyroteuthis infernalis,” that is a part scientific treaty, part spoof, part philosophical discourse, and part fable. Vampyroteuthis infernalis, the vampire squid, lives in the ocean's abyss, a dark, cold space far away from the habitats that humans populate. Flusser and Bec explore the real and philosophical ocean that separates Vampirotheutis from humans and eventually attempt to explore the metaphysical foundation that encompasses both the vampire squid and humans. They write, “The abyss that separates us [from the vampire squid] is incomparably smaller than that which separates us from extraterrestrial life as imagined by science fiction and astrobiology,” thus proclaiming the cephalopod as the ultimate biological, technological, philosophical, and spiritual challenge that planet Earth has to offer. The quest for such an encompassing metaphysics stands in stark contrast to the fact that the main role cephalopods had in human history, is an involuntary contribution as tasty seafood.
A first step toward the acknowledgment of cephalopods was set by the European Union Directive 2010/63/EU, coming into force on 1 January 2013 across all Europe and regulating the research of any of the about 700 extant species of “live cephalopods.” The Directive establishes measures for the “protection of animals used for scientific or educational purposes.” Cephalopods are the sole invertebrate taxon included in this Directive following Canada in 1991, New Zealand in 1999, Australia in 2004, Switzerland 2008, and Norway in 2009. This marked a paradigm shift for invertebrates in EU, by covering the use of an entire class of Mollusks, namely “live cephalopods” (i.e., hatched juveniles and adults) in the legislation covering experimental procedures likely to cause pain, suffering, distress, or lasting harm. This means that, under the Directive and transposed national laws, cephalopods have the same legal status as vertebrates, in relation to their experimental use in research and testing.
The EU funded COST Action FA 1301 “A network for improvement of cephalopod welfare husbandry in research, aquaculture and fisheries (CephsInAction)” (2013–2017) was the first international network initiative to advance the understanding, methods, and dissemination of cephalopod research (see: http://www.cephsinaction.org/). CephsInAction was supported by scientists from 19 European countries, Israel, Australia, and the US, and could well be the starting point for a future and more intense interdisciplinary debate about cephalopods and how they could propel our understanding of evolution, natural and artificial intelligence, emotions, consciousness, and future technological innovations.
What is the goal of cephalopod research in the twenty-first century? As the squid giant axon provided a generalized model that pioneered modern neuronal studies in any animals including humans, we may expect to reach a complete molecular understanding of cephalopod cells, organs, and behaviors via post-genome approaches. This would, in turn, allow exploring a universal molecular basis of emotion, pain, sleep, and even consciousness. We may find a healthy stock of deep-sea cephalopod that would enlighten us on the evolutionary development of the extreme environment adaptability strategies. We would be able to create a viable aqua culturing method, and we may even create a deep neural network-driving soft robot that communicates to us by changing its body pattern. Are these far-fetched ideas that only belong to science fiction movies? The answer is No. These are the ideas that many scientists are currently working on, however independent and fragmented they might appear. Through this investigation, we found in many instances the possibilities and high potentials to interweave many existing thoughts, disciplines, practices and fields that already possess shared goals. In conclusion, the complexity and varieties provided by cephalopods and their biological properties, cultural symbolism, and history, appear to function as an ideal boundary object that offers great potential to accelerate development of truly innovative interdisciplinary platforms for science, art, and engineering alike.
Author Contributions
Each author worked on their area of speciality. RN worked on Art and media. FD worked on the history. SS and LZ worked collaboratively on modern cephalopod science section. RN and MS worked on the introduction and conclusion sections. All of us worked on editing the entire text together.
Funding Information
SS has been supported by the Stazione Zoologica Anton Dohrn Fellowship (BEOM Department, Stazione Zoologica 548 Anton Dohrn). The initial panel discussion was supported by COST Action FA 1301 and CephsInAction.
Conflict of Interest Statement
The authors declare that the research was conducted in the absence of any commercial or financial relationships that could be construed as a potential conflict of interest.
References
Abbott, N. J., Williamson, R., and Maddock, L. (1995). Cephalopod Neurobiology: Neuroscience Studies in Squid, Octopus and Cuttlefish. Oxford: Oxford University Press.
Albertin, C. B., Bonnaud, L., Brown, C. T., Crookes-Goodson, W. J., da Fonseca, R. R., Di Cristo, C., et al. (2012). Cephalopod genomics: a plan of strategies and organization. Stand. Genomic Sci. 7, 175–188. doi: 10.4056/sigs.3136559
Albertin, C. B., Simakov, O., Mitros, T., Wang, Y. Z., Pungor, J. R., Edsinger-Gonzales, E., et al. (2015). The octopus genome and the evolution of cephalopod neural and morphological novelties. Nature 524, 220–224. doi: 10.1038/nature14668
Allcock, A. L., Lindgren, A., and Strugnell, J. M. (2015). The contribution of molecular data to our understanding of cephalopod evolution and systematics: a review. J. Nat. Hist. 49, 1373–1421. doi: 10.1080/00222933.2013.825342
Ansari, Y., Manti, M., Falotico, E., Mollard, Y., Cianchetti, M., and Laschi, C. (2017). Towards the development of a soft manipulator as an assistive robot for personal care of elderly people. Int. J. Adv. Robot. Syst. 14:1729881416687132. doi: 10.1177/1729881416687132
Appel, T. A. (1987). The Cuvier-Geoffrey Debate. French Biology in the Decades before Darwin. Oxford: Oxford University Press.
Armstrong, C. M., and Bezanilla, F. (1973). Currents related to movement of the gating particles of the sodium channels. Nature 242, 459–461. doi: 10.1038/242459a0
Barratt, M., Johnson, M. P., and Allcock, A. L. (2007). Fecundity and reproductive strategies in deep-sea incirrate octopuses (Cephalopoda: Octopoda). Mar. Biol. 150, 387–398. doi: 10.1007/s00227-006-0365-6
Borrelli, L., Gherardi, F., and Fiorito, G. (2006). A Catalogue of Body Patterning in Cephalopoda. Firenze: Firenze University Press.
Boycott, B. B. (1954). Learning in Octopus vulgaris and other cephalopods. Pubbl. della Staz. Zool. Napoli. 25, 67–93.
Boycott, B. B., and Young, J. Z. (1955). A memory system in Octopus vulgaris Lamarck. Proc. R. Soc. Lond. B. 143, 449–480. doi: 10.1098/rspb.1955.0024
Boyle, P., and Rodhouse, P. (2008). Cephalopods: Ecology and Fisheries. New York, NY: John Wiley and Sons.
Buckner, R. L. (2007). “Memory systems: an incentive, not an endpoint,” in Science of Memory: Concepts, eds H. L. Roediger, Y. D. Dudai, and S. M. Fitzpatrick (Oxford: Oxford University Press), 359–364.
Budelmann, B. U. (1995). Cephalopod sense organs, nerves and the brain: adaptations for high performance and life style. Mar. Freshw. Behav. Physiol. 25, 13–33. doi: 10.1080/10236249409378905
Bullock, T. H. (1948). Properties of a single synapse in the stellate ganglion of squid. J. Neurophysiol. 11, 343–364. doi: 10.1152/jn.1948.11.4.343
Calisti, M., Corucci, F., Arienti, A., and Laschi, C. (2015). Dynamics of underwater legged locomotion: modeling and experiments on an octopus-inspired robot. Bioinspir Biomim. 10:046012. doi: 10.1088/1748-3190/10/4/046012
Chung, W.-S., and Marshall, N. J. (2017). Complex visual adaptations in squid for specific tasks in different environments. Front. Physiol. 8:105. doi: 10.3389/fphys.2017.00105
Dai, L., Zheng, X., Kong, L., and Li, Q. (2012). DNA barcoding analysis of Coleoidea (Mollusca: Cephalopoda) from Chinese waters. Mol. Ecol. Resour. 12, 437–447. doi: 10.1111/j.1755-0998.2012.03118.x
Darmaillacq, A. S., Dickel, L., and Mather, J. A. (2012). Cephalopod Cognition. Cambridge: Cambridge University Press.
De Sio, F. (2011). Leviathan and the soft animal: medical humanism and the invertebrate models for higher nervous functions, 1950s−90s. Med. Hist. 55, 369–374. doi: 10.1017/S0025727300005421
Doubleday, Z. A., Prowse, T. A., Arkhipkin, A., Pierce, G. J., Semmens, J., Steer, M., et al. (2016). Global proliferation of cephalopods. Cur. Biol. 26, R406–R407. doi: 10.1016/j.cub.2016.04.002
Doya, C., Chatzievangelou, D., Bahamon, N., Purser, A., Leo, F. C. D., Juniper, S. K., et al. (2017). Seasonal monitoring of deep-sea megabenthos in Barkley Canyon cold seep by internet operated vehicle (IOV). PLoS ONE 12:e0176917doi: 10.1371/journal.pone.0176917
Dröscher, A. (2016). Pioneering studies on cephalopod's eye and vision at the Stazione Zoologica Anton Dohrn (1883-1977). Front. Physiol. 7:618. doi: 10.3389/fphys.2016.00618
Edelman, D. B., Baars, B. J., and Seth, A. K. (2005). Identifying hallmarks of consciousness in non-mammalian species. Consci. Cognit. 14, 169–187. doi: 10.1016/j.concog.2004.09.001
Edelman, G. M. (1987). Neural Darwinism: The Theory of Neuronal Group Selection. New York, NY: Basic books.
FAO Fisheries and Aquaculture Department (2016). The State of World Fisheries and Aquaculture 2010. Rome: Food and Agriculture Organization of the United Nations.
Fiorito, G., Affuso, A., Anderson, D. B., Basil, J., Bonnaud, L., Botta, G., et al. (2014). Cephalopods in neuroscience: regulations, research and the 3Rs. Invert Neurosci. 14, 13–36. doi: 10.1007/s10158-013-0165-x
Flusser, V., and Bec, L. (1987). Vampyroteuthis Infernalis. Eine Abhandlung samt Befund des Institut Scientifique de Recherche Paranaturaliste. Göttingen: Immatrix Publications.
Gagnon, Y. L., Osorio, D. C., Wardill, T. J., Marshall, N. J., Chung, W. S., and Temple, S. E. (2016). Can chromatic aberration enable color vision in natural environments? Proc. Natl. Acad. Sci. U.S.A. 113, 6908–6909. doi: 10.1073/pnas.1612239113
Garrett, S., and Rosenthal, J. J. C. (2012). RNA editing underlies temperature adaptation in K+ channels from polar octopuses. Science 17, 848–851. doi: 10.1126/science.1212795
Grimpe, G. (1928). Pflege, Behandlung und Zucht der Cephalopoden fur zoologische und physiologische Zweeke. Abderhalden Handbuch der biologischen Arbeitsmethoden, Abt. IX. Teil 5, 331–402
Guglielmino, E., Godage, I., Zullo, L., and Caldwell, D. G. (2013). “A pragmatic bioinspired approach to the design of octopus-inspired arms,” in Proceedings of the IEEE/RSJ International Conference on Intelligent Robots and Systems (IROS 2013) (Tokyo), 4577–4582.
Guglielmino, E., Zullo, L., Cianchetti, M., Follador, M., Branson, D., and Caldwell, D. G. (2012). “The application of embodiment theory to the design and control of an octopus-like robotic arm,” in Proceedings IEEE International Conference on Robotics and Automation (ICRA) (Saint Paul, MN), 5277–5282.
Hanke, F. D., and Osorio, D. C. (2018). Vision in Cephalopods. Front. Physiol. 9:18. doi: 10.3389/978-2-88945-430-3
Hanlon, R. T., and Messenger, J. B. (2018). Cephalopod Behaviour. 2nd Edn. Cambridge: Cambridge University Press.
Hochner, B. (2013). How nervous systems evolve in relation to their embodiment: what we can learn from octopuses and other molluscs. Brain Behav. Evol. 82, 19–30. doi: 10.1159/000353419
Hochner, B., Shomrat, T., and Fiorito, G. (2006). The octopus: a model for a comparative analysis of the evolution of learning and memory mechanisms. Biol. Bull. 210, 308–317. doi: 10.2307/4134567
Hodgkin, A. L., and Huxley, A. F. (1939). Action potentials recorded from inside a nerve fibre. Nature 144, 710–711. doi: 10.1038/144710a0
Hodgkin, A. O. M. (1975). Address of the president sir Alan Hodgkin, OM at the Anniversary Meeting, 30 November 1974. Proc. R. Soc. Lond. B 188, 103–119. doi: 10.1098/rspb.1975.0006
Hoving, H. J. T., Laptikhovsky, V. V., and Robison, B. H. (2015). Vampire squid reproductive strategy is unique among coleoid cephalopods. Curr. Biol. 25, R322–R323. doi: 10.1016/j.cub.2015.02.018
Hoving, H. J. T., Perez, J. A., Bolstad, K. S., Braid, H. E., Evans, A. B., Fuchs, D., et al. (2014). The study of deep-Sea cephalopods. Adv. Mar. Biol. 67, 235–359. doi: 10.1016/B978-0-12-800287-2.00003-2
How, M. J., Norman, M. D., Finn, J., Chung, W. S., and Marshall, N. J. (2017). Dynamic skin patterns in cephalopods. Front. Physiol. 8:393. doi: 10.3389/fphys.2017.00393
Iglesias, J., Otero, J. J., Moxica, C., Fuentes, L., and Sánchez, F. J. (2004). The completed life cycle of the octopus (Octopus vulgaris, Cuvier) under culture conditions: paralarval rearing using Artemia and zoeae, and first data on juvenile growth up to 8 months of age. Aquac. Int. 12, 481–487. doi: 10.1023/B:AQUI.0000042142.88449.bc
Ikeda, Y. (2009). A perspective on the study of cognition and sociality of cephalopod mollusks, a group of intelligent marine invertebrates. Jpn. Psychol. Res. 51, 146–153. doi: 10.1111/j.1468-5884.2009.00401.x
Katz, B., and Miledi, R. (1970). Further study of the role of calcium in synaptic transmission. J. Physiol. 207, 789–801. doi: 10.1113/jphysiol.1970.sp009095
Kingston, A. C., Kuzirian, A. M., Hanlon, R. T., and Cronin, T. W. (2015). Visual phototransduction components in cephalopod chromatophores suggest dermal photoreception. J. Exp. Biol. 218, 1596–1602. doi: 10.1242/jeb.117945
Krebs, H. A. (1975). The August Krogh principle: “For many problems there is an animal on which it can be most conveniently studied”. J. Exp. Zool. 194, 221–226. doi: 10.1002/jez.1401940115
Krieg, M., Sledge, I., and Mohseni, K. (2015). Design considerations for an underwater soft-robot inspired from marine invertebrates. Bioinspir. Biomim. 10:065004. doi: 10.1088/1748-3190/10/6/065004
Kubodera, T., and Mori, K. (2005). First-ever observations of a live giant squid in the wild. Proc. R. Soc. B 272, 2583–2586. doi: 10.1098/rspb.2005.3158
Larson, C., Peele, B., Li, S., Robinson, S., Totaro, M., Beccai, L., et al. (2016). Highly stretchable electroluminescent skin for optical signaling and tactile sensing. Science 351, 1071–1074. doi: 10.1126/science.aac5082
Laschi, C., Mazzolai, B., and Cianchetti, M. (2016). Soft robotics: technologies and systems pushing the boundaries of robot abilities. Sci. Robotics 1:3690. doi: 10.1126/scirobotics.aah3690
Lee, P. N., Callaerts, P., De Couet, H. G., and Martindale, M. Q. (2003). Cephalopod Hox genes and the origin of morphological novelties. Nature 424, 1061–1065. doi: 10.1038/nature01872
Lindgren, A. R., and Anderson, F. E. (2017). Assessing the utility of transcriptome data for inferring phylogenetic relationships among coleoid cephalopods. Mol. Phylogenet. Evol. 118, 330–342. doi: 10.1016/j.ympev.2017.10.004
Liscovitch-Brauer, N., Alon, S., Porath, H. T., Elstein, B., Unger, R., Ziv, T., et al. (2017). Trade-off between transcriptome plasticity and genome evolution in cephalopods. Cell 169, 191–202. doi: 10.1016/j.cell.2017.03.025
MAFF (2017). FY2016 Trends in Fisheries FY2017 Fisheries Policy White Paper on Fisheries: Summary (PDF). Available online at: http://www.maff.go.jp/e/data/publish/
Mangold, K. (1989). Céphalopodes. Traité de Zoologie. Anatomie, Systématique, Biologie, Tome 5, Fascicule 4. Paris: Masson.
Mather, J. A., and Anderson, R. C. (1993). Personalities of octopuses (Octopus rubescens) J. Comp. Psychol. 107, 336–340. doi: 10.1037/0735-7036.107.3.336
Mather, J. A., and Anderson, R. C. (1999). Exploration, play, and habituation in octopuses (Octopus dofleini) J. Comp. Psychol. 113, 333–338. doi: 10.1037/0735-7036.113.3.333
Mather, J. A., and Carere, C. (2012). Editorial: why (and how) personalities in invertebrates? Curr. Zool. 58:566. doi: 10.1093/czoolo/58.4.566
Mather, J. A., and Dickel, L. (2017). Cephalopod complex cognition. Cur. Opin. Behav. Sci. 16, 131–137. doi: 10.1016/j.cobeha.2017.06.008
Mather, J. A., and Kuba, M. (2013). The cephalopod specialties: complex nervous system, learning, and cognition. Can. J. Zool. 91, 431–449 doi: 10.1139/cjz-2013-0009
Mauvisseau, Q., Parrondo, M., Fernández, M. P., García, L., Martínez, J. L., García-Vázqueza, E., et al. (2017). On the way for detecting and quantifying elusive species in the sea: the Octopus vulgaris case study. Fish. Res. 191, 41–48. doi: 10.1016/j.fishres.2017.02.023
Messenger, J. B. (1988). Why octopuses? Have we learned anything from studying their brains? Sci. Prog. 72, 297–320.
Messenger, J. B., Wilson, A. P., and Hedge, A. (1973). Some evidence for colour-blindness in Octopus. J. Exp. Biol. 59, 77–94.
Nakajima, K., Hauser, H., Li, T., and Pfeifer, R. (2015). Information processing via physical soft body. Sci. Rep. 5:10487. doi: 10.1038/srep10487
Navarro, J., Monroig, Ó., and Sykes, A. (2014). “Chapter: 5. Nutrition as a key factor for cephalopod aquaculture,” in Cephalopod Culture, eds J. Iglesias, L. Fuentes, and R. Villanueva (Dordrecht: Springer), 77–95.
Nixon, M., and Young, J. Z. (2003). The Brain and Lives of Cephalopods. Oxford: Oxford University Press.
Osorio, D. (2014). Cephalopod behaviour: skin flicks. Curr. Biol. 24, 684–685. doi: 10.1016/j.cub.2014.06.066
Packard, A. (1972). Cephalopods and fish: the limits of convergence. Biol. Rev. 47, 241–307. doi: 10.1111/j.1469-185X.1972.tb00975.x
Pfeifer, R., Lungarella, M., and Iida, F. (2007). Self-organization, embodiment, and biologically inspired robotics. Science 318, 1088–1093. doi: 10.1126/science.1145803
Pikul, J. H., Li, S., Bai, H., Hanlon, R. T., Cohen, I., and Shepherd, R. F. (2017). Stretchable surfaces with programmable 3D texture morphing for synthetic camouflaging skins. Science 6360, 210–214. doi: 10.1126/science.aan5627
Ramirez, M. D., and Oakley, T. H. (2015). Eye-independent, light-activated chromatophore expansion (LACE) and expression of phototransduction genes in the skin of Octopus bimaculoides. J. Exp. Biol. 218, 1513–1520. doi: 10.1242/jeb.110908
Robison, B., Seibel, B., and Drazen, J. (2014). Deep-sea octopus (Graneledone boreopacifica) conducts the longest-known egg-brooding period of any animal. PLoS ONE 9:e103437. doi: 10.1371/journal.pone.0103437
Rodhouse, P. G., Pierce, G. J., Nichols, O. C., Sauer, W. H., Arkhipkin, A. I., Laptikhovsky, V. V., et al. (2014). Environmental effects on cephalopod population dynamics: implications for management of fisheries. in Adv. Mar. Biol. 67, 99–233. doi: 10.1016/B978-0-12-800287-2.00002-0
Rus, D., and Tolley, M. T. (2015). Design, fabrication and control of soft robots. Nature 521, 467–475. doi: 10.1038/nature14543
Schmitt, C. B. (1965). Aristotle as a cuttlefish: the origin and development of a Renaissance image. Stud. Renaiss. 12, 60–72. doi: 10.2307/2857069
Sereni, E., and Young, J. Z. (1932). Nervous degeneration and regeneration in cephalopods. Pubbl. Staz. Zool. Napoli, 12, 173–208.
Shen, H. (2016). Meet the soft, cuddly robots of the future. Nature 530, 24–26. doi: 10.1038/530024a
Stubbs, A. L., and Stubbs, C. V. T. (2016). Color vision via chromatic blur and pupil shape. Proc. Nat. Acad. Sci. U.S.A. 113, 8206–8211. doi: 10.1073/pnas.1524578113
Tomarev, S. I., Callaerts, P., Kos, L., Zinovieva, R., Halder, G., Gehring, W., et al. (1997). Squid Pax-6 and eye development. Proc. Natl. Acad. Sci. U.S.A. 94, 2421–2426. doi: 10.1073/pnas.94.6.2421
Trimmer, B. (2014). A journal of soft robotics: why now? Soft Robot. 1, 1–4. doi: 10.1089/soro.2013.0003
Uribe, J. E., and Zardoya, R. (2017). Revisiting the phylogeny of Cephalopoda using complete mitochondrial genomes. J. Moll. St. 83, 133–144. doi: 10.1093/mollus/eyw052
Vidal, E. (2014). Advances in Cephalopod Science: Biology, Ecology, Cultivation and Fisheries. Vol. 67, Boston, MA: Academic Press.
von Uexküll, J. (1905). Leitfaden in das Studium der Experimentellen Biologie der Wassertiere. Wiesbaden: J. F. Bergmann.
Walker, W. F., and Doolittle, W. F. (1983). 5S ribosomal-RNA sequences from four marine-invertebrates and implications for base-pairing models of metazoan sequences. Nucleic Acids Res. 11, 5159–5164. doi: 10.1093/nar/11.15.5159
Wang, Z., Chen, M. Z., and Yi, J. (2015). Soft robotics for engineers. HKIE Trans. 22, 88–97. doi: 10.1080/1023697X.2015.1038321
Wehner, M., Truby, R. L., Fitzgerald, D. J., Mosadegh, B., Whitesides, G. M., Lewis, J. A., et al. (2016). An integrated design and fabrication strategy for entirely soft, autonomous robots. Nature 536:451. doi: 10.1038/nature19100
Wells, M. J. (1978). Octopus. Physiology and Behaviour of an Advanced Invertebrate. London: Chapman and Hall.
Yoshida, M., Yura, K., and Ogura, A. (2014). Cephalopod eye evolution was modulated by the acquisition of Pax-6 splicing variants. Sci. Rep. 4:4256. doi: 10.1038/srep04256
Young, J. Z. (1938). The functioning of the giant nerve fibres of the squid. J. Exp. Biol. 15, 170–185.
Zullo, L., and Hochner, B. (2011). A new perspective on the organization of an invertebrate brain. Comm. Integ. Biol. 4, 26–29. doi: 10.4161/cib.13804
Keywords: cephalopod, interdisciplinary, culture, art, science, communication
Citation: Nakajima R, Shigeno S, Zullo L, De Sio F and Schmidt MR (2018) Cephalopods Between Science, Art, and Engineering: A Contemporary Synthesis. Front. Commun. 3:20. doi: 10.3389/fcomm.2018.00020
Received: 27 February 2018; Accepted: 02 May 2018;
Published: 13 June 2018.
Edited by:
Tarla Rai Peterson, The University of Texas at El Paso, United StatesReviewed by:
Karen M. Taylor, University of Alaska Fairbanks, United StatesEmily Plec, Western Oregon University, United States
Copyright © 2018 Nakajima, Shigeno, Zullo, De Sio and Schmidt. This is an open-access article distributed under the terms of the Creative Commons Attribution License (CC BY). The use, distribution or reproduction in other forums is permitted, provided the original author(s) and the copyright owner are credited and that the original publication in this journal is cited, in accordance with accepted academic practice. No use, distribution or reproduction is permitted which does not comply with these terms.
*Correspondence: Ryuta Nakajima, cm5ha2FqaW1AZC51bW4uZWR1
Markus R. Schmidt, c2NobWlkdEBiaW9mYWN0aW9uLmNvbQ==
†Present Address: Shuichi Shigeno, Department of Neurobiology, University of Chicago, Chicago, IL, United States