- 1School of Health and Welfare, Halmstad University, Halmstad, Sweden
- 2Real Salt Lake, Salt Lake City, UT, United States
- 3Centre for Cognitive Neuroscience, Division of Sport, Health and Exercise Sciences, College of Health, Medicine and Life Sciences, Brunel University London, Uxbridge, United Kingdom
- 4Centre for Sport Research, Institute of Physical Activity and Nutrition, School of Exercise and Nutrition Sciences, Deakin University, Burwood, VIC, Australia
- 5U.S. Army Medical Research Directorate-West, Walter Reed Army Institute of Research, Joint Base Lewis-McChord, WA, United States
- 6Institute of Human and Machine Cognition, Pensacola, FL, United States
Introduction: We examined skilled-based differences in the impact of exposure to an opponent with action tendencies that were either independent of, dependent on, or both independent of and dependent on evolving environmental information during anticipation.
Methods: A video-based two-vs.-two soccer task was employed, where 14 expert and 14 novice soccer players had to predict an attacking opponent's imminent actions, before and after exposure to the preceding actions of the opponent.
Results: Anticipation accuracy, number of responses congruent with the opponent's action tendencies, response confidence, and visual dwell time on the opponent in possession increased following opponent exposure, both in experts and novices. When compared to novices, experts demonstrated higher anticipation accuracy, more congruent responses, and greater response confidence. Novices performed at their best when the opponent exhibited action tendencies that were independent of the environment, whereas experts demonstrated their highest performance when the opponent had action tendencies that were both independent of and dependent on unfolding environmental information.
Discussion: Our findings provide novel insights into the role of context-environment dependency and support the notion that experts are superior to novices in detecting and utilizing opponents' action tendencies and integrating this information with unfolding environmental information during anticipation.
Introduction
In dynamic and highly time-constrained performance environments, the ability to anticipate the outcome of unfolding events is a significant marker of domain-specific expertise (Williams and Jackson, 2019). Historically, anticipation has primarily been examined by focusing on skill-based differences in the way performers acquire and use kinematic sources of information (i.e., biological motion emanating from the movements of an opponent). This body of research has consistently demonstrated that experts utilize more reliable kinematic sources of information, which enable them to anticipate faster and more accurately than their less-expert counterparts (see Mann et al., 2007). It is suggested that the more effective processing skills exhibited by experts are due to prolonged accumulation of domain-specific experience resulting in a greater attunement to task-relevant information (Williams and Ford, 2008). However, over the past decade, researchers have highlighted the important role of non-kinematic—contextual—sources of information when trying to explain expert anticipation (see Gredin et al., 2020a). In sport, such contextual sources of information may be applicable across competitive settings and different opponents, such as the positioning of players on the field of play (e.g., Loffing and Hagemann, 2014; Murphy et al., 2016; Runswick et al., 2017), the risk and rewards associated with different game scenarios (e.g., Canãl-Bruland et al., 2015; Gredin et al., 2019), and the footedness/handedness of opponents (e.g., McMorris and Colenso, 1996; Loffing et al., 2016). Alternatively, these contextual sources can be more specific to the action tendencies of an individual opponent, such as a certain action pattern that the opponent exhibits (e.g., Farrow and Reid, 2012; Loffing et al., 2015; Milazzo et al., 2016), or the opponent's proportional distribution of different actions in each situation (e.g., Mann et al., 2014; Gredin et al., 2018; Thomas et al., 2022).
Advances in technology have enabled sophisticated analyses of competitors sporting behavior, providing athletes with contextual information pertaining to the action tendencies of forthcoming opponents (Memmert et al., 2017; Marković et al., 2020). This information may be provided in the form of explicit probabilistic information about the opponent's action tendencies (e.g., Navia et al., 2013; Broadbent et al., 2018; Murta et al., 2021) or by exposing the athletes to the preceding actions of the opponent during simulated scenarios (e.g., McRobert et al., 2011; Mann et al., 2014; Thomas et al., 2022). Experts have consistently demonstrated a superior ability to use contextual information about opponent-specific action tendencies when compared to less-expert counterparts, whether it is explicitly provided or accrued through opponent exposure (for a review, see McRobert et al., 2011; Gredin et al., 2018, 2023; Thomas et al., 2022). However, less is known about how the nature of the action tendencies may influence this process. It has been suggested that the dependency between contextual information and evolving environmental information (i.e., if the usefulness of the contextual information is independent of or dependent on unfolding features in the environment) may influence perceptual and cognitive requirements during anticipation. Contextual information, such as opponent action tendencies, that is independent of environmental information is informative about the to-be-anticipated event, without having to account for unfolding features in the environment (e.g., positioning of players off the ball). In contrast, the informativeness of contextual information that is dependent on environmental information is dictated by the nature of evolving environmental information (e.g., information about opponent action tendencies must be integrated with the positioning of players off the ball). However, the impact of this context-environment dependency during anticipation has yet to be tested empirically (Gredin et al., 2020a). In this study, we examine the impact of exposure to an opponent with action tendencies that were either independent of, dependent on, or both independent of and dependent on, evolving environmental information on anticipation performance and associated perceptual-cognitive processes.
Gray (2002) demonstrated that expert baseball batters seemed to change their expectations about upcoming pitch types based on their previous exposure to pitches from the same opponent. Measures of temporal errors in bat-ball contact on a simulated baseball-batting task, revealed that the batters performed with 47 ms lower error when a fast pitch was preceded by three consecutive fast pitches, compared to when a fast pitch was preceded by three consecutive slow pitches. The performance difference was interpreted as substantial, because the temporal margin for error in hitting has been estimated at 9 ms (see Watts and Bahill, 1990). The findings suggest that the batters formed a-priori expectations that the speed of impending pitches would be congruent with the speed of the preceding pitches from the opponent. The biasing effect of being exposed to the preceding action of an opponent has subsequently been demonstrated across a wide range of sport tasks, including tennis (Farrow and Reid, 2012), handball (Mann et al., 2014), volleyball (Loffing et al., 2015), karate (Milazzo et al., 2016), and soccer (Thomas et al., 2022). Furthermore, expert, or more experienced, athletes seem to be better able to detect an opponent's preceding action patterns or action distributions and use this information to inform their predictions of the opponent's upcoming actions, compared to novice, or less experienced, athletes (Farrow and Reid, 2012; Loffing et al., 2015; Milazzo et al., 2016; Thomas et al., 2022).
Thomas et al. (2022) used a temporally occluded video-based anticipation task to examine the role of expertise in using an opponent's action tendencies and kinematic information during increasing exposure to the preceding actions of the opponent. Video-based occlusion techniques, where the availability of unfolding environmental information (e.g., kinematic information) is removed (i.e., occluded) by stopping the video before a to-be-anticipated event (e.g., an opponent's action) is fully disclosed, are commonly used to examine the pick-up and use of information during anticipation tasks (see Mann and Savelsbergh, 2015). The task simulated a two-vs.-two defensive soccer scenario where expert and novice players had to predict the ball direction (left or right) of the final action from an opponent in possession of the ball. In each trial, there was one opponent in possession of the ball, a second opponent off the ball and one defender marking the second attacker throughout the trial. Halfway through each trial, the opponent off the ball made a direction change toward either the left or the right. At the end of the trial, the opponent in possession could either pass the ball to his teammate or dribble the ball in the opposite direction from his teammate (e.g., if the opponent off the ball was on the right side of the opponent in possession at the end of the trial, the opponent in possession could either pass the ball to the right or dribble the ball to the left). Anticipation accuracy, response confidence, and visual-search behaviors were recorded across two experimental phases, where each experimental phase comprised two blocks of 18 non-occluded exposure trials. The opponent in possession exhibited skewed action tendencies (dribble = 67%, pass = 33% or pass = 67%, dribble = 33%) during both exposure and testing and, in the second experimental phase, the opponent exhibited action tendencies that were skewed oppositely to those in the initial phase.
Following each of the two exposure blocks in the first experimental phase, moderate to large, and small to moderate, increases in anticipation accuracy were found for experts and novices, respectively. However, when the opponent's action tendencies were flipped between experimental phases, a large decline in anticipation accuracy was found in experts, but no significant effect was documented in novices. Over the following two exposure blocks in the second experimental phase, the anticipation accuracy of novice players remained stable, whereas expert players recovered to the performance level they had before the opponent changed his action tendencies. The authors concluded that increasing exposure to the preceding actions of an opponent affects expert and novice soccer players differently. They argued that expert players seem to better utilize and adapt to the action tendencies of the opponent, whereas the adaptations in novice players seem to be driven by an enhanced attunement to the kinematic information of the opponent.
In support of this notion, Thomas et al. (2022) reported that, when compared to novices, expert players exhibited a moderately higher number of visual fixations on the opponent off the ball in the two-vs.-two defensive soccer scenarios. It was inferred that the experts checked more so for positional information from the opponent off the ball to “translate” information about the opponent's action tendencies (i.e., dribble or pass) into the most likely to-be-anticipated ball direction (i.e., left or right). For experts, there was a moderate increase in the number of visual fixations on the opponent off the ball over the initial experimental phase, which may reflect an exposure-induced increased awareness that the opponent exhibited skewed action tendencies that were dependent on this environmental source of information (see also Gredin et al., 2018). Thomas et al. (2022) also recorded retrospective self-reports of response confidence which revealed that both experts and novices became more confident in their responses following opponent exposure. However, overall, experts were more confident in their responses than novices, which the authors regarded to reflect a greater awareness of opponent action tendencies (cf. Murphy et al., 2018).
An important feature of the task design used in the studies by Gredin et al. (2018) and Thomas et al. (2022) was that, unlike most other studies examining the impact of contextual information in the form of opponent exposure (e.g., Farrow and Reid, 2012; Mann et al., 2014; Loffing et al., 2015) or explicit instruction (e.g., Navia et al., 2013; Broadbent et al., 2018; Murta et al., 2021), information about the opponent's action tendencies had to be integrated with evolving environmental information to be useful. Specifically, as the task for the participants was to predict ball direction (i.e., left or right), whereas the action tendencies referred to action type (i.e., dribble or pass), the participants had to take into account the positional information about the opponent off the ball (i.e., evolving environmental information), to convert information about the most likely action type to information about the most likely ball direction. As the positioning of the opponent off the ball unfolded during the trial, this conversion process had to governed by the participant during task performance. This process may place higher requirements on perceptual and cognitive systems, as it promotes a top-down selection of visual attention (i.e., context-driven acquisition of environmental information) and requires conditional reasoning (i.e., conversion of contextual information based on acquired environmental information; see Gredin et al., 2020b). However, this proposition, and the way it influences anticipation performance among expert and novice athletes, is yet to be examined in a systematic manner (Gredin et al., 2020a). Such insight may provide valuable knowledge that can be used to explain the nature of expertise in the multifaceted and ever-changing performance settings encountered in many professional domains.
In the present study, we employed the video-based anticipation task used by Thomas et al. (2022) to examine anticipation performance among expert and novice soccer players following exposure to an opponent with skewed action tendencies. However, in contrast to previous research examining the impact of action tendency information on anticipation (e.g., Broadbent et al., 2018; Gredin et al., 2018; Thomas et al., 2022), we systematically manipulated the dependency between action tendency information and evolving environmental information. Specifically, the players were exposed to an opponent that exhibited action tendencies that were either independent of (i.e., referred to ball direction; e.g., left pass or dribble), dependent on (i.e., referred to action type; e.g., pass left or right), or both independent of and dependent (i.e., referred to both ball direction and action type; e.g., predominantly pass left) on evolving environmental information (i.e., the positioning of the opponent off the ball). Response accuracy, eye-tacking data, and retrospective self-reports of response confidence were collected to gain insights into the perceptual and cognitive processes employed during task performance.
Due to their prolonged accumulation of domain-specific experience, we predicted that expert players would outperform novices, regardless of the action tendencies the opponent exhibited (Williams and Ford, 2008; Gredin et al., 2018; Thomas et al., 2022). We hypothesized that both experts and novices would demonstrate improved performance on the task following opponent exposure (cf. Thomas et al., 2022). These improvements were expected to be driven by an increased attunement to the opponent's action tendencies, manifest as a higher number of congruent responses and higher levels of response confidence, and be more profound among experts than novices (cf. Thomas et al., 2022). Given the task design, directional tendencies, unlike action type tendencies, did not have to be integrated with unfolding environmental information to become useful and therefore were predicted to be less demanding on the perceptual and cognitive processes (see Gredin et al., 2020a); thus, both skill-groups were predicted to perform better when the opponent exhibited directional, compared to action type, tendencies. As the action tendencies that referred to both ball direction and action type provided the most reliable information with regard to the to-be-anticipated ball direction, we expected that both experts and novices would perform at their best when the opponent exhibited these combined action tendencies (cf. Gredin et al., 2021). With respect to gaze behavior, we predicted that experts and novices would spend more time looking at the player in possession, compared to the opponent off the ball, as the opponent in possession would be considered as the most threatening player and the main responsibility of the participant during the task (cf. Gredin et al., 2018; Thomas et al., 2022). However, based on the assumption that experts would be better able to integrate contextual information that is dependent on unfolding environmental information (see Gredin et al., 2020a), we predicted that opponent exposure would bias how experts, but not novices, allocated visual attention toward the opponent off the ball, when the opponent exhibited action type tendencies. However, due to the lack of such integration requirements, this effect was not predicted under conditions where the opponent exhibited directional tendencies. As participants could choose to rely on either the information about ball direction or the information about action type when the opponent exhibited combined action tendencies, we did not forward hypotheses related to gaze behavior and opponent exposure under these conditions.
Methods
Participants
Altogether, 14 expert (Mage = 23.2; SD = 2.01) and 14 novice (Mage = 22.14; SD = 1.99) soccer players were recruited. A statistical power analysis was performed for sample size estimation using G*Power (version 3.1). Based on the effect of video-based exposure to opponents with skewed action tendencies on anticipation accuracy reported in the study by Mann et al. (2014), the analysis revealed that a total sample size of 28 participants (i.e., 14 in each skill group) would be sufficient to detect a medium effect size with an alpha of 0.05 and a power of 0.80. The expert group had accumulated an average of 15.1 years (SD = 2.85) of experience playing competitive soccer. All expert players reported playing competitively at a varsity high-school level, with 10 players additionally playing for independent competitive clubs outside of school. Five experts had played at least 1 year at the collegiate level, whereas all individuals within the novice group reported playing <1 year of soccer at a recreational level. All experimental procedures were approved by the lead university's Institutional Review Board, and written informed consent was obtained from each participant.
Test stimuli
Video sequences were filmed on an artificial soccer pitch using a wide-angle converter lens (Canon WD-H72 0.8x, Tokyo, Japan) that was attached to a high-definition digital video camera (Canon XF 100, Tokyo, Japan). The camera was mounted to a mobile trolley situated at a height of 1.7 m (~ 5′7”), replicating the first-person perspective of a central defender in a typical match situation (cf. Gredin et al., 2018, 2019, 2020b, 2021; Thomas et al., 2022). Three soccer players with similar levels of experience as the expert sample in this study were recruited to construct the test stimuli. These players followed a script to create standardized sequences depicting 2-vs.-2 counterattacking scenarios. Each sequence contained an opponent in possession of the ball, a secondary opponent off the ball, and a defender marked the secondary opponent off the ball situating the participant to be the primary defender of the opponent in possession. Sequences were constructed to portray a rapidly unfolding scenario that presented high levels of perceived threat, requiring the athletes to make accurate anticipatory judgements to stop the attack (Triolet et al., 2013), as well as promote the use of prior expectancies (Roca et al., 2013).
Sequences began with the opponent in possession situated 3 m inside the halfway line, roughly 7 m in front of the participant. Both the opponent off the ball and the marking defender started 3 m behind the opponent in possession, either on the left or right side. To better replicate the environmental information that the participant would have been aware of in a real match scenario, sequences began with a 1-s freeze-frame which allowed participants the opportunity to gather positional information from the opponent in possession, the opponent off the ball, and the other defender (Roca et al., 2011). Following the freeze-frame, the opponent in possession started to dribble toward the participant. As the opponent in possession closed in on the participant, both the opponent off the ball and the marking defender would maintain their run on the same side as they began the sequence or would cross behind the primary opponent in possession ~1½ s into the sequence, ultimately ending on the opposite side. At the end of each dynamic counterattacking scenario, the opponent in possession was ~3 m in front of the participant with the opponent off the ball level on his right or left side. At the end of the trial, the opponent in possession could either pass the ball to the opponent off the ball or dribble the ball in the opposite direction from the opponent off the ball. That is, to be able to control the dependency between action tendency information and the positioning of the opponent off the ball across action tendency conditions (see Procedure) and to standardize the response options (i.e., left or right) across action types (i.e., dribble or pass), the opponent in possession never dribbled the ball in the same direction as the opponent off the ball. Thus, there were four possible outcomes to the counterattack: the opponent in possession dribbled to the (1) right side or the (2) left side of the participant, or he passed the ball to the opponent off the ball on the (3) right or (4) left side (see Figure 1). Accordingly, the final position of the opponent off the ball relative to the opponent in possession, would dictate the possible event that could occur (i.e., if the opponent off the ball ended on the left side, the opponent in possession could only dribble right or pass to the left). Each sequence lasted ~5 s. In total, 130 sequences were created. Altogether, 48 video sequences (12x videos per counterattacking outcome) were independently selected by two qualified soccer coaches (UEFA A license holders) who predicated their selection on identifying the clips that were most representative of actual game play. This sub-selection of clips was used to generate the sequences used in this study. The footage was constructed using Adobe Premier Pro 2020 (Adobe Inc., San Jose, CA) and was projected onto a 3.3 × 1.8 m screen (Elite Screens Inc., Garden Grove, CA) using a 1030 3LCD Projector (Epson, Nagano, Japan). The screen was located 3.7 m in front of the participant during testing, providing a horizontal and vertical viewing angle of ~48° and 27°, respectively (North et al., 2009; Roca et al., 2011; cf. Gredin et al., 2018).
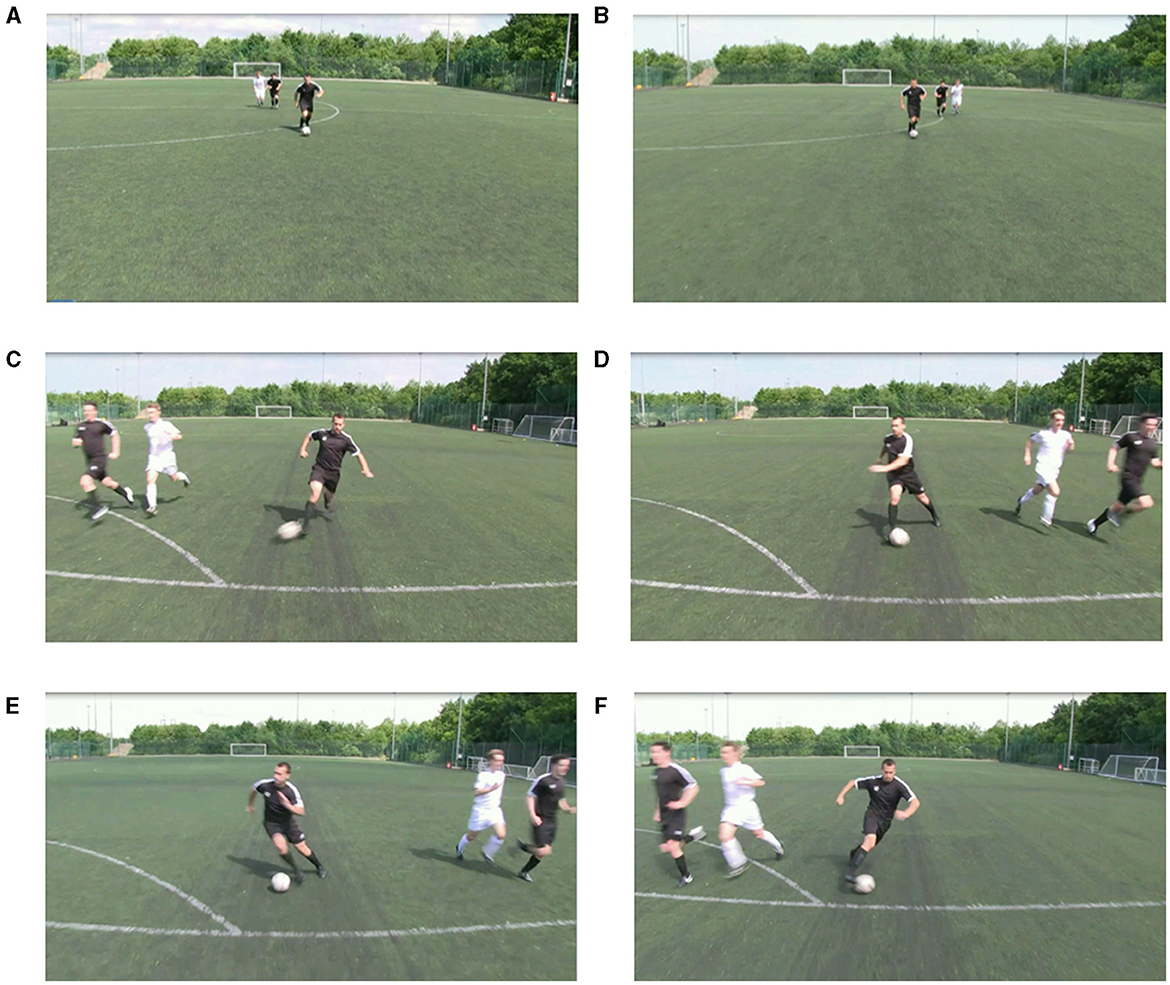
Figure 1. Test stimuli. The two different positions that the opponent off the ball and the defender could have at the start of each sequence: to the left (A) or the right (B) side of the opponent in possession. The four different actions that the opponent in possession could carry out at the end of each sequence: pass left (C), pass right (D), dribble left (E), and dribble right (F). Figure adapted from Gredin et al. (2019) (CC-BY 4.0).
Procedure
Upon arrival at the laboratory, each participant completed a brief questionnaire that consisted of demographic information and prior experience of playing soccer. Participants were fitted with a mobile head-mounted eye-tracking system [ETG 2.0; Sensorimotor Instruments (SMI), Teltow, Germany]. The eye-tracking system connected to a mobile phone that was used to calibrate the system and record gaze data. Participants began with eight trials that provided familiarity with the stimulus presentation, the nature of the counterattacking scenarios and response requirements (i.e., verbally predict the direction of the ball—left or right—following the final action of the opponent in possession). Directional response options were chosen, rather than action type response options, as the effect of the opponent's final action (i.e., left or right ball direction) was considered as more threatening, and therefore a more relevant source of information, than the nature of the action per se (i.e., dribble or pass). The familiarly trials consisted of two clips from each of the four possible trial outcomes were presented: pass left; pass right; dribble left; dribble right. Prior to beginning the pre-test, participants confirmed appropriate understanding of the counterattacking scenarios and the response requirements.
Participants performed the anticipation task under eight different action tendency conditions, which differed in terms the proportional distribution of trials that concluded with a certain action type or ball direction: Left (left = 67%, right = 33%); Right (right = 67%, left = 33%); Dribble (dribble = 67%, pass = 33%); Pass (pass = 67%, dribble = 33%); Dribble Left (dribble left = 67%, dribble right = 11%, pass left = 11%, pass right = 11%); Dribble Right (dribble right = 67%, dribble left = 11%, pass left = 11%, pass right = 11%); Pass Left (pass left = 67%, dribble left = 11%, dribble right = 11%, pass right = 11%); and Pass Right (pass right = 67%, dribble left = 11%, dribble right = 11%, pass left = 11%). Each action tendency condition comprised a pre-test block, an exposure block, and a post-test block; each test and exposure block consisted of 18 trials that reflected the opponent's condition-specific action tendencies. That is, in each of the eight action tendency conditions, there were 18 pre-test trials, 18 exposure trials, and 18 post-test trials where the proportional distribution of action outcomes in each block of 18 trials aligned with the opponent's condition-specific action tendencies. Using the same exposure stimuli and test task as in the current study, previous research has shown that a predominant action outcome of 67% during 18 exposure trials is sufficient to elicit response bias among expert soccer players (Thomas et al., 2022).
Each of the eight action tendency conditions were assigned to one of three categories based on the nature of the action tendencies the opponent exhibited: directional (Left and Right); action type (Dribble and Pass); or combined (Dribble left, Dribble right, Pass left, and Pass right) tendencies. Directional tendencies were independent of evolving environmental information, as both action tendency information and response options referred to possible ball directions (i.e., left and right). As such, directional tendencies were informative about the to-be-anticipated ball direction in themselves, without having to account for whether the opponent off the ball was on the left or the right side of the opponent in possession at the end of the trial (cf. Broadbent et al., 2018). Action type tendencies were dependent on evolving environmental information, as the action tendency information referred to action type (i.e., dribble and pass), but the response options referred to the possible ball directions (i.e., left and right). Thus, the participant had to account for the unfolding positioning of the opponent off the ball (i.e., left or right side of the opponent in possession) to convert information about the most likely action type to information about the most likely ball direction (cf. Gredin et al., 2018). The combined tendencies contained information that were both independent of (directional tendencies) and dependent on (action type tendencies) the evolving positioning of the opponent off the ball (see Table 1). In the two conditions with directional action tendencies, the opponent completed an equal number of passes and dribbles. Similarly, in the two conditions with action type tendencies, the proportional distribution of left and right ball directions was balanced.
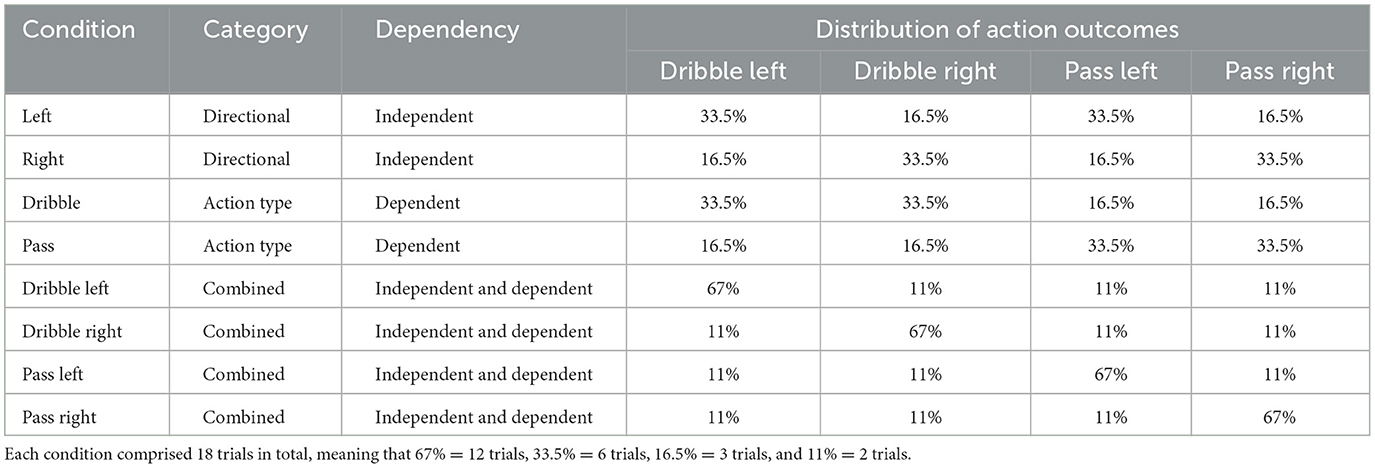
Table 1. The eight action tendency conditions, the three action tendency categories, the dependency between action tendencies and environmental information, and the distribution of action outcomes.
The accuracy of the eye tracker was checked after each test block, providing the participants ~3-min periods to rest. To avoid ordering effects, conditions were pseudorandomized into three separate stimulus videos to ensure two conditions within the category were not presented consecutively. To minimize the influence of potential learning and carryover effects across action tendency conditions, the order in which the different action tendency conditions were presented was pseudorandomized (i.e., two action tendency conditions from the same action tendency category were not presented consecutively) and counterbalanced across participants (cf. Thomas et al., 2022).
Test trials that concluded with a pass were occluded 120 ms prior to the last foot-ball contact from the opponent in possession, whereas test trials that concluded with a dribble were occluded 240 ms before the opponent's final foot-ball contact (cf. Thomas et al., 2022). Occlusion points were predicated on prior work that deduced the time between the emergence of relevant kinematic information and the last moment of foot-ball contact was shorter when the final action was a pass vs. a dribble (Gredin et al., 2021). To make the temporal demands more representative of those under match conditions, participants were instructed to respond quickly and accurately once the occlusion point occurred. Audio responses were recorded through the microphone on the eye-tracking system. Responses made 2.5 s after the occlusion point were considered as incorrect. Participants responded with their prediction of what direction the ball would go following the final action of the opponent in possession (e.g., if they predicted the opponent would pass the ball to his teammate on the right side of the visual display, the participant would verbally respond right). Following their verbal response, participants provided a response regarding their perceived level of confidence in their prediction on a scale of 1–10 (cf. Thomas et al., 2022).
The 18 exposure trials, that were presented in between the pre- and post-tests of each condition, were stopped 240 ms after the final foot-ball contact from the opponent, such that the outcome of the opponent's final action was fully revealed to the participants and no response was required. Due to the limited quantity of available clips, the clips used in the exposure phase were the same used in the test blocks. To mitigate the potential confounding effects of participants remembering the trial order or the outcome of specific trials in the exposure phase, the order in which trials were presented in each exposure phase and test block was randomized (cf. Thomas et al., 2022). The experimental session took between 75 and 90 min to complete for each participant.
Dependent measures
Anticipation accuracy
A binary metric of whether each response was congruent to the ball direction of the final action (cf. Thomas et al., 2022).
Congruent responses
The number of responses that were congruent with the most likely outcome, given the action tendencies of the opponent (e.g., number of “dribble responses” in the condition Dribble). This measure has been suggested to reflect the participant's reliance on the opponent's action tendencies during task performance (e.g., Gredin et al., 2019, 2021; Thomas et al., 2022).
Response confidence
Self-reported confidence ratings were obtained after each test trial to assess the level of confidence participants felt in their response on the preceding trial. Response confidence was measured on a 10-point scale with 1 indicating the least confidence, and 10 indicating feeling very confident in their response (cf. Thomas et al., 2022).
Gaze data
The eye-tracking data were uploaded into SMI BeGaze analysis software (v3.7) where gaze fixations were mapped onto key areas of interest. The two primary areas of interest were the opponent in possession and the opponent off the ball (cf. Gredin et al., 2018; Thomas et al., 2022). Fixations onto these areas of interest were quantified post-processing using BeGazeTM. The fixations spanning each ~5 s trial in the pre-test and post-test were manually mapped during this process (n = 6,912 trials). Trials in which fixations were missing, or disrupted due to poor calibration, were removed from the analysis (<4.2%). Skill-based changes in the allocation of visual attention between pre- and post-test, as well as across action tendency categories, was measured primarily through visual dwell time on each area of interest (cf. Gredin et al., 2018). Thus, the average dwell time spent on each area of interest was averaged across test blocks initially, and further across groups for each action tendency category.
Data analysis
Anticipation accuracy, number of congruent responses, and response confidence data were averaged across the test block level for each participant during the experiment and across each of the eight action tendency conditions. Group level averages were calculated among both expert and novice groups. Dependent measures were analyzed using separate three-way mixed design ANOVAs that were completed in two steps. First, an initial 2 (Group: Expert, Novice) × 2 (Test Block: Pre-test, Post-test) × 3 (Category: Directional, Action Type, Combined) mixed model ANOVA was conducted to assess broader differences in the dependent measures at the category level. Next, mixed model ANOVAs were conducted to test for within-category differences. Separate three-way mixed design ANOVAs were accordingly run for each action tendency category. These included 2 (Group: Expert, Novice) × 2 (Test Block: Pre-test, Post-test) × 2 and 4 Condition (Directional: Left, Right; Action Type: Dribble, Pass; Combined: Dribble left, Dribble right, Pass left, Pass right).
Visual search measures were aggregated at the trial level to derive average gaze behaviors for each counterattacking sequence. Specifically, the percentage (%) of each case that participants fixated on the opponent in possession vs. the opponent off the ball was averaged across test blocks, as well as at the group level. To initially assess differences in fixations relative to the two areas of interest within group, separate ANOVAs were conducted on both the expert and novice groups: 3 (Category: Directional, Action Type, Combined) × 2 (Test Block: Pre-test, Post-test) × 2 (Area of Interest: Opponent in Possession, Opponent Off the Ball). Furthermore, separate 2 (Group: Expert, Novice) × 3 (Category: Directional, Action Type, Combined) × 2 (Test Block: Pre-test, Post-test) three-way ANOVAs were conducted for dwell % within each area of interest.
For all statistical tests, the statistical significance level was set to α = 0.05, and the Greenhouse-Geisser correction was used when assumptions of sphericity were violated. Partial eta-squared values () are reported as effect size for ANOVA models. Bonferroni corrected independent t-tests were further conducted to evaluate skill-based differences, or changes across blocks, when two or three-way interaction occurred. Data were analyzed using R (v3.4.1; R Core Team, 2017), and R-Studio 1.1.463 (Boston, MA).
Results
Anticipation accuracy
The mixed-factorial ANOVA revealed significant main effects for Group, F(1,78) = 38.50, p < 0.001, = 0.242 and Test Block, F(1,78) = 22.73, p = <0.001, = 0.092. Compared to the novice group, expert participants recorded higher accuracy scores across all three action type categories, while both groups improved their performance following a period opponent exposure. These main effects were superseded by a significant Group × Test Block × Category interaction, F(1,78) = 2.51, p = 0.01, = 0.039. Post-hoc tests revealed that during the post-test, the expert group were better at anticipating when the opponent had combined action tendencies (M = 77.9% ± 4.3) than they were at anticipating when the opponent exhibited action type (M = 69.3% ± 3.9, p = 0.01) or directional tendencies (M = 67.5% ± 4.1, p = 0.008). In contrast, the novice group anticipated more accurately against the opponent when he exhibited directional tendencies (M = 57.3% ± 5.1), compared to action type (M = 50.4% ± 3.7, p = 0.005), or combined tendencies (M = 53.0% ± 4.9, p = 0.01). The findings are highlighted in Figure 2.
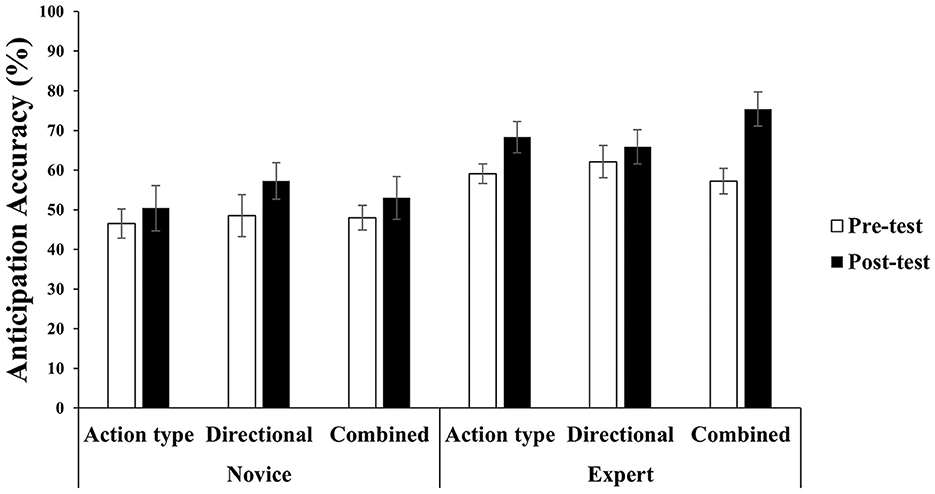
Figure 2. The means and SDs of anticipation accuracy across test blocks for novices and experts within each action tendency category.
The mixed-factor ANOVA assessing performance differences between conditions within the action type category, revealed a main effect for Group, F(1,52) = 15.90, p < 0.001, = 0.187 and Test Block, F(1,52) = 13.86, p < 0.001, = 0.065. While both groups increased their performance following the exposure block, experts outperformed novices, both under conditions where the opponent had a greater tendency to dribble and under conditions where he had a greater tendency to pass. This main effect was superseded by a significant Group × Test Block × Condition interaction, F(1,52) = 5.43, p = 0.009, = 0.071. Post-hoc tests revealed that during the pretest, experts were better at anticipating under conditions were the opponent exhibited a propensity to dribble (M = 64.5% ± 3.7), compared to when he had a greater tendency to pass the ball (M = 54.0% ± 5.3, p = 0.006).
When the opponent exhibited directional tendencies, the mixed-factor ANOVA showed a main effect for Group, F(1,52) = 14.65, p < 0.001, = 0.126 and Test Block, F(1,52) = 6.43 p = 0.021, = 0.063. Experts could more correctly predict the ball direction under both directional conditions (i.e., Left and Right) when compared to novices. However, both groups were able to improve anticipation performance following the exposure block. There was a significant Group × Condition interaction, F(1,52) = 4.32, p = 0.017, = 0.102. Follow-ups indicated that experts displayed better anticipation of the ball direction in the Left, compared to the Right, condition.
The mixed-factorial ANOVA for the category with combined action tendencies revealed main effects of Group, F(1,104) = 24.42, p < 0.001, = 0.118, Test Block, F(1,104) = 19.10, p < 0.001, = 0.073, and Condition, F(1,104) = 14.49, p < 0.001, = 0.17. The expert group outperformed the novice participants across each condition, though both groups improved anticipation performance between the pre- and post-test. In addition, the condition in which the opponent preferred to dribble left was the easiest to anticipate for both the expert and the novice group. A significant Group × Condition interaction, F(1,104) = 8.43, p < 0.001, = 0.068 further revealed that the condition in which the opponent preferred to pass to the right proved to be the most difficult condition for experts (see Table 2).
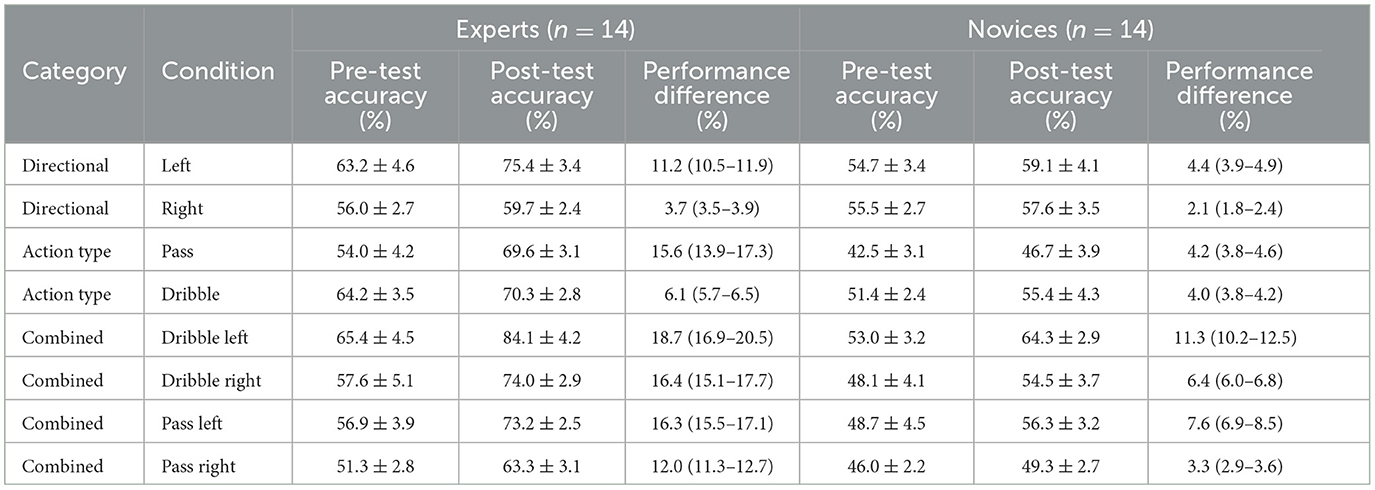
Table 2. The means ± SDs for anticipation accuracy by expertise group within each action tendency condition, as well as means and 95% CL for within-group differences between test blocks.
Congruent responses
For congruent responses, there were main effects for Group, F(1,78) = 40.35, p < 0.001, = 0.150, and Test Block, F(1,78) = 14.07, p < 0.001, = 0.193. The expert group made more anticipation responses that aligned with the opponent's actual tendencies, and both groups increased their number of congruent responses following exposure.
Within the action type category, main effects of Group, F(1,52) = 17.46, p < 0.001, = 0.113, and Test Block, F(1,52) = 15.54, p < 0.001, = 0.193 were reported. Experts provided more responses congruent with the opponent's true tendencies, compared to novices, but both groups increased their congruent responses following the exposure block.
The analysis for directional tendencies revealed main effects of Group, F(1,52) = 8.84, p < 0.004, = 0.063, Test Block, F(1,52) = 7.27, p = 0.013, = 0.074, and Condition, F(1,52) = 8.14, p = 0.006, = 0.124. Experts provided more congruent responses than novices, with both groups increasing the number of congruent responses from pre- to post-test. Both groups provided more congruent responses in the Left condition, compared to in the Right condition.
The final analysis assessing the combined tendency category resulted in main effects of Group, F(1,104) = 26.21, p < 0.001, = 0.193, Test Block, F(1,104) = 43.31, p < 0.001, = 0.273, and Condition, F(1,104) = 11.43, p = 0.003, = 0.093. Under conditions of combined action tendencies, participants exhibited a higher number of congruent responses following the exposure block, but the expert group responded with a greater number of congruent responses regardless of block or condition. Main effects for Block and Condition were superseded by a significant Test Block × Condition interaction, F(1,104) = 6.88, p < 0.001, = 0.031. Post-hoc decomposition showed that in the post-test, both groups responded with more congruent responses when the opponent preferred to dribble left (pre-test, experts: M = 8.9 ± 2.1, post-test, experts: M = 10.4 ± 2.5, p = 0.012; pre-test, novices; M = 5.8 ± 1.8, post-test, novices: M = 7.3 ± 1.9, p = 0.008) and pass left (pre-test, experts: M = 7.5 ± 2.0, post-test, experts: M = 9.1 ± 1.7, p = 0.018; pre-test, novices: M = 7.2 ± 1.9, post-test, novices: M = 8.5 ± 1.5, p = 0.021).
Response confidence
The three-way mixed factorial ANOVA for self-reported confidence resulted in significant main effects for Group, F(1,78) = 24.74, p < 0.001, = 0.214, and Test Block, F(1,78) = 18.43, p < 0.001, = 0.085, indicating that experts were more confident than novices across conditions, but both groups felt more confident following the exposure block.
Within the action type category, the mixed model ANOVA revealed a main effect of Group, F(1,52) = 17.05, p < 0.001, = 0.222, and Test Block, F(1,52) = 23.48, p < 0.001, = 0.054. Experts were more confident in their responses than novices, but both groups increased their response confidence following the exposure block. With respect to the category with directional tendencies, similar main effects of Group, F(1,52) = 5.12, p = 0.028, = 0.079, and Test Block, F(1,52) = 14.32, p = <0.001, = 0.044, were documented. That is, experts felt more confident in their responses than novices overall, but both groups increased their response confidence from pre- to post-test. A Group × Test Block × Condition interaction was also documented, F(1,52) = 6.32, p = 0.008, = 0.083, indicating that during the posttest, experts were more confident in their responses in the Left condition (M = 8.43 ± 1.3) compared to in the Right condition (M = 7.03 ± 1.9, p = 0.003).
When the opponent exhibited combined action tendencies, significant main effects of Group, F(1,104) = 19.87 p < 0.001, = 0.134, and Test Block, F(1,104) = 12.81, p < 0.001, = 0.059, were found, indicating that experts felt more confident than novices and both groups felt more confident following exposure.
Gaze behaviors
The three-way mixed model ANOVA for the expert players led to a significant main effect of Area of Interest, F(1,162) = 142.76, p < 0.001, = 0.64. Experts fixated more on the opponent in possession than the opponent off the ball. There was a significant interaction between, Area of Interest and Test Block, F(1,162) = 8.42, p = 0.004, = 0.131. Post-hoc analysis revealed experts fixated more on the opponent in possession, and accordingly less on the opponent off the ball, in the post-test. Lastly, there was a Category × Area of Interest × Test Block interaction, F(2,162) = 14.33 p < 0.001, = 0.227. Further post-hoc tests showed that the experts spent more time fixating on the opponent off the ball during the pre-test when the opponent exhibited directional (M = 43.2% ± 5.3, p = 0.014) and action type tendencies (M = 34.3% ± 3.7, p = 0.023), compared to when the opponent exhibited combined tendencies (M = 22.1% ± 3.1). For novices, there was a main effect of Area of Interest, F(1,162) = 100.18, p < 0.001, = 0.56. The novice players fixated more on the opponent in possession than the opponent off the ball. Additionally, there was a Category × Area if Interest × Test Block interaction, F(2,162) = 9.41, p < 0.001, = 0.094. Novices significantly increased the fixation time on the opponent in possession, and accordingly decreased the time spent fixating on the opponent off the ball, when the opponent had action type tendencies (see Figure 3).
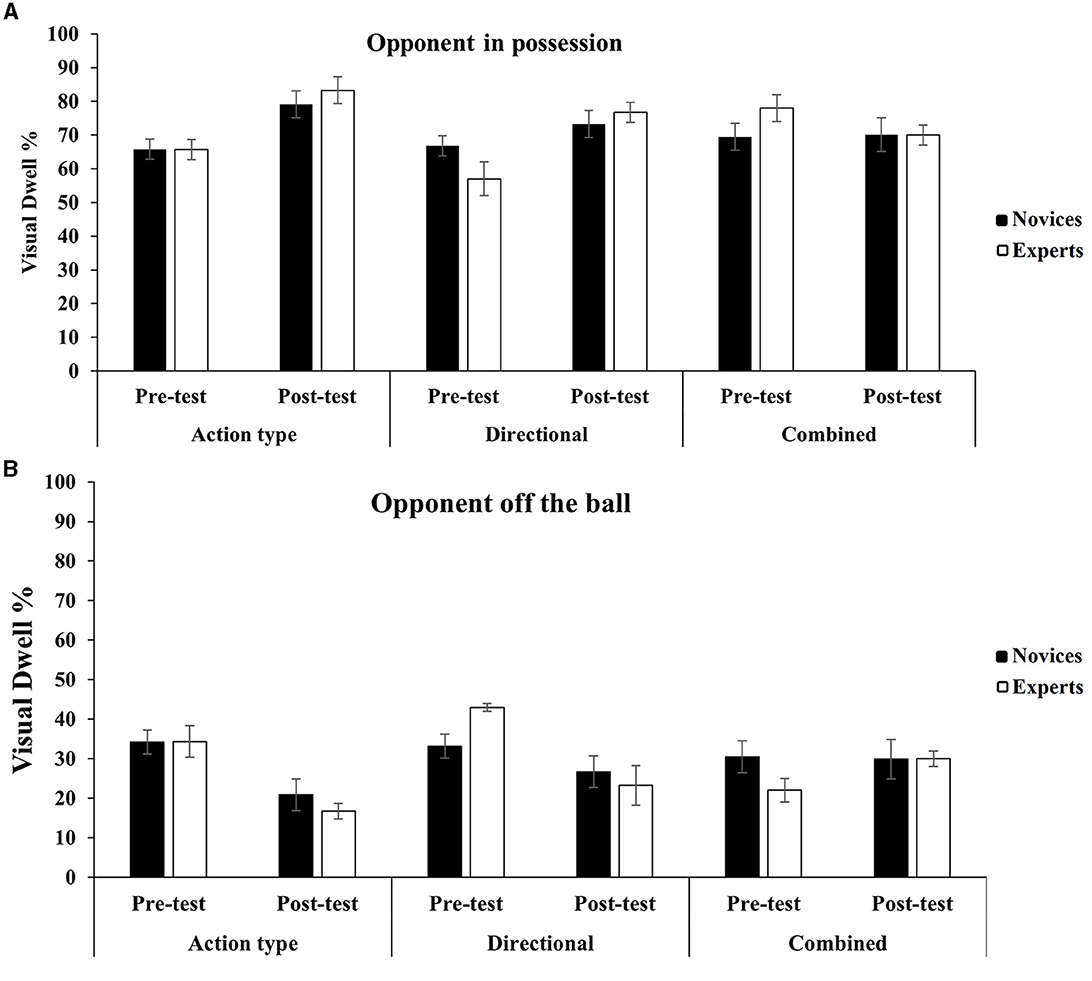
Figure 3. The average visual dwell time (%) on the opponent in possession (A) and opponent off the ball (B) for novices and experts across action tendency categories and test blocks.
Regarding between-group differences, the three-way mixed model for dwell % on the opponent in possession did not result in a main effect of Group (p = 0.65). However, a Group × Category × Test Block, F(2,78) = 11.71 p < 0.001, = 0.24 interaction was revealed. Post-hoc analysis demonstrated that the novice group spent more time fixating on the opponent in possession during the pre-test when the opponent exhibited directional tendencies. A similar interaction was found, in that the expert group spent more time viewing the opponent off the ball during the pre-test when the opponent had directional tendencies.
Discussion
We examined the impact of exposing experts and novices to an opponent's preceding actions on their ability to predict the opponent's imminent actions. We employed a representative video-based anticipation task simulating two-vs.-two defensive soccer scenarios. We recorded anticipation accuracy, number of congruent responses, overt visual attention, and response confidence before and after a period of exposure to the opponent's preceding actions. We systematically manipulated the nature of the opponent's action tendencies, in that they were either independent of (i.e., directional), dependent on (i.e., action type), or both independent of and dependent on (i.e., directional and action type) the unfolding positioning of the opponent off the ball. We predicted that expert soccer players would outperform novices on the anticipation task, both before and after the exposure period, regardless of whether the opponent exhibited directional, action type, or combined action tendencies (Williams and Ford, 2008; Gredin et al., 2018; Thomas et al., 2022). This hypothesis was supported, as experts exhibited superior anticipation accuracy under all test conditions. This finding adds to the extensive body of existing literature demonstrating expertise-based differences in anticipation performance on representative video-based sport tasks (see Mann et al., 2007; Williams and Jackson, 2019; Gredin et al., 2020a).
We expected that both experts and novices would improve performance following the exposure block (cf. Thomas et al., 2022). In support of this hypothesis, both skill-groups anticipated with greater accuracy in post-tests compared to in pre-tests; this effect was found in all action tendency categories. Collectively, the findings reported in the study by Thomas et al. (2022) and in the current study, suggest that both experts and novices seem to be able to utilize task-relevant information from an opponent's preceding actions to better anticipate the opponent's proceeding actions. In line with our predictions, response data of the number of congruent responses exhibited by the players in the current study suggest that the experts' superior performance, and the performance-enhancing effects observed in both skill-groups, were underpinned by a higher/increased number of responses that aligned with the opponent's action tendencies. These findings concur with those reported in the study by Thomas et al. (2022), in which the authors argued that the number of congruent responses may reflect the athlete's reliance on opponent action tendencies during anticipation. As such, experts exhibiting a higher number of congruent responses compared to novices in the current study provides further support for the notion that experts are more receptive to, or at least cognizant of, opponent-specific action tendencies (cf. Farrow and Reid, 2012; Loffing et al., 2015; Milazzo et al., 2016; Thomas et al., 2022). Thomas et al. (2022) flipped opponent's action tendences across two experimental phases, concluding that expert soccer players appear to adapt to changes in the opponent's behavioral preferences, whereas novices showed no such adaptation and instead only improved their anticipation performance during the initial experimental phase once becoming attuned to opponents' kinematic information. While no such conclusions can be drawn from the current data, it is reasonable to assume that the observed effects of opponent exposure on experts and novices were predominantly driven by adaptations to action tendency information and kinematic information, respectively.
Similar interpretations can be drawn from the response confidence data. Higher levels of response confidence on anticipation tasks have been associated with greater awareness of the contextual information, such as opponent action tendencies, used during task performance (Murphy et al., 2018; Thomas et al., 2022). In line with our predictions, and previous research (Thomas et al., 2022), both skill-groups reported increasing levels of response confidence following exposure, and experts reported higher response confidence levels than novices, in all action tendency categories.
With respect to the performance effects of the different action tendency categories, we predicted that the players would exhibit superior performance in the category with directional tendencies than with action type tendencies. This effect was hypothesized based on the notion that contextual information that is dependent on evolving environmental information (i.e., action type tendencies in our study) comes with higher requirements on the individual's perceptual and cognitive systems, compared with contextual information that is independent of evolving environmental information (i.e., directional tendencies in our study; Gredin et al., 2020a). However, this predicted effect was only observed for novice players. A possible explanation is that due to greater domain-specific experience, experts were better equipped to deal with the requirements of integrating information about action type tendencies with unfolding information about the positioning of the opponent off the ball, to convert information about the opponent's tendency to dribble or pass to likely directional outcomes. The experts advantage over novices in this regard has been shown in previous research (Gredin et al., 2018) and, as mirrored in our results, the performance benefit of not having to deal with those requirements when the opponent exhibited directional action tendencies seemed to be less among experts than novices.
When the opponent exhibited directional action tendencies, experts were both more accurate and confident in their responses when left was the most prevalent directional outcome, compared to under conditions where the ball was directed toward right more often; this effect was observed both before and after the exposure block. These effects may be attributed to the impact of other sources of contextual information that have proven to influence performance during sport-anticipation tasks, such as field positioning (Loffing and Hagemann, 2014; Murphy et al., 2016; Runswick et al., 2017) and judgment risks and rewards (Canãl-Bruland et al., 2015; Gredin et al., 2019). In soccer, possession of the ball in a more central position near the penalty area (i.e., to the participant's left in the task of this study) is more frequently associated with positive attacking outcomes, compared to possession in a wider position (i.e., to the participant's right in the task of this study; Brooks et al., 2016). Thus, it is likely that the expert players in the current study associated the left side with greater goal threat and, from their defender perspective, they were therefore more inclined to opt “left,” which resulted in both greater response accuracy and greater response confidence when the ball was directed to the left more often than the right. Due to their lack of experience from actual gameplay, it is not surprising that the corresponding effects were not found for novice players.
Both expert and novice players were predicted to anticipate with the highest accuracy under conditions where the opponent exhibited combined directional and action type tendencies. This was hypothesized since, when combined, directional and action type tendencies conveyed more reliable information regarding the to-be-anticipated ball direction, compared to on their own. More specifically, in 78% of the trials, the ball was directed in the direction that corresponded to most likely one given the combined action tendencies (e.g., if the opponent had the propensity to dribble left, the ball would be directed toward the left in 14 of the 18 trials within the test/expose block; 12 trials in which the opponent dribbled left and two trials in which the opponent passed the ball to his teammate on the lefthand side); the corresponding figure for directional and action type tendencies on their own was 67%. However, this prediction was only supported by the performance data within the expert group, in which the players exhibited higher anticipation accuracy, compared to when the opponent exhibited either directional tendencies or action type tendencies. Novices, on the other hand, performed better under conditions with only directional tendencies, compared to when directional and action type tendencies were combined. It has been proposed that not only the relative reliabilities of the available sources of contextual information influence performance during anticipation, but also the individual's ability to infer these reliabilities. That is, due to greater domain-specific experience and attunement to relevant information sources, expert can estimate the reliability of the contextual information at hand more accurately than novices and are better able to integrate various sources of information on the basis of their comparative reliabilities (Gredin et al., 2020a). This phenomenon has previously been demonstrated when examining the impact of providing explicit information about opponent action tendencies to expert and novice soccer players during anticipation (Gredin et al., 2018) and may explain why experts, but not novices, in the current study exhibited their best performance when the opponent's action tendencies conveyed the most reliable information (i.e., under conditions of combined action tendencies).
When the opponent was most likely to dribble left, both experts and novices performed better than in the other combined action tendency conditions. This finding may be because the opponent only controlled the ball with his right foot, both in the run-up to the concluding action and in the execution of the final action. Opponent footedness has been identified as source of contextual information that influences the ability to anticipate an opponent's forthcoming actions. McMorris and Colenso (1996) demonstrated that expert soccer goalkeepers were more accurate in predicting the directions of penalty kicks from right-footed penalty takers (i.e., reflecting the preferred foot of the most soccer players), compared to left-footed players. Thus, it is possible that the players in our study inferred the “dribble left” as the most likely option, as that would put the right-footed opponent in the most favorable position on their strongest foot for an attempt on goal, resulting in greater response accuracy when this belief concurred with the actual action tendencies of the opponent. Another potential reason why players exhibited greater accuracy when the opponent dribbled the ball to the left, compared to when the ball was passed to the left, could be that they considered the opponent in possession as their primary defensive responsibility, given that the opponent off the ball was marked by the other defender. As would be expected due to discrepancies in domain-specific experience, superior performance in the condition where the opponent dribbled to the left most often was more profound in the expert than in the novice group. In future, researchers should examine how various sources of contextual information, such as opponent action tendencies, footedness, and risks and rewards associated with field positioning, are weighted, and integrated together by experts to anticipate an opponent's behavior.
In line with our predictions and previous reports (Gredin et al., 2018; Thomas et al., 2022), both expert and novice players spent more time looking at the opponent in possession compared to the opponent off the ball in all test blocks. This finding is not surprising given the nature of the scenarios used, where the opponent off the ball was being marked by a secondary defender throughout the sequence. Consequently, participants were likely to consider the opponent in possession as the most threatening player and their main responsibility during task performance. However, in contrast to our prediction, both experts and novices decreased the time spent looking at the opponent off the ball following exposure, both when the opponent exhibited directional and action type tendencies. This finding contradicts previous work which showed that providing opponent action type tendencies biased expert, but not novice, players to allocate visual attention toward the opponent off the ball as they attempt to integrate the dependent contextual information with context-relevant features of the unfolding environment (Gredin et al., 2018; Thomas et al., 2022). In future, researchers need to further explore the impact of dependent and independent contextual information on the processing priorities performers employ during anticipation.
We did not make any specific hypotheses about the impact of exposure under conditions where the opponent exhibited combined action tendencies. The reason for this was that the participants could choose to rely on either the directional component (which did not require integration with evolving environmental information) or the action type component (which had to be integrated with the unfolding positioning of the opponent off the ball) of the combined tendencies. Under these conditions, the experts, but not novices, increased the time they spent looking at the opponent off the ball between pre- and post-tests, which suggest that they were more receptive to the action type component of the opponent's combined tendencies during exposure (Gredin et al., 2020a).
While we were able to systematically manipulate the dependency between contextual and environmental information through various conditions related to the opponent action tendencies, a limitation is that the response requirements for the participants were the same in all conditions (i.e., directional). Therefore, we cannot rule out the possibility that the results obtained across action tendency categories were due to the features of the tendencies themselves (i.e., whether they related to ball direction, action type, or both ball direction and action type), rather than their dependency with unfolding environmental information. To control for the potential confounding impact of the tendency feature itself and obtain a more valid measure of the hypothesized dependency effect, in future researchers could modify the current study design by altering the response requirements (i.e., responding with ball direction or action type) within each condition. For example, if using the same test stimuli as in the current study, the response requirements could be manipulated so that they concurred with either directional tendencies (which was the case under the conditions of directional tendencies in the current study) or action type tendencies (which was not tested in the current study). Another interesting avenue for future research would be to adjust the nature of the combined action tendencies employed. In the current study, the directional and action type components of the combined action tendencies were allied in that 67% of the trials concluded with the same direction and action, and the outcome in the remaining 33% of the trials were distributed evenly across the other three possible direction-action combinations. To gain a more sensitive measure of the relative reliance on the different tendency components of the combined tendencies, and to enable exploration of how the congruency between components may influence performance, the information associated with each component should be independent of the information associated with the other component (e.g., dribble = 67% and left = 67%, rather than dribble left = 67%). Finally, it is worth highlighting that the opponent in possession was always right-footed, and all scenarios took place slightly right of the central line of the pitch. These other sources of contextual information could have influenced the detection of, and reliance on, action tendency information. As previously discussed, participants may have used other sources of contextual information (i.e., judgment risk and rewards associated with opponent footedness and field positioning) to inform their judgments, which may have reduced their reliance on information about the opponent's action tendencies (Gredin et al., 2019). In future, researchers can mitigate these potential confounding factors by randomizing pitch location and opponent footedness within stimuli sets.
In summary, the findings align with previous reports of experts outperforming their less skilled counterparts on representative video-based anticipation tasks (see Mann et al., 2007; Williams and Jackson, 2019; Gredin et al., 2020a). In line with previous findings (Thomas et al., 2022), the performance data in the current study suggest that exposure to an opponent's actions enhances the ability to predict the forthcoming actions of an opponent. Our findings, with regard to congruent responses and response confidence, provide support for the notion that experts are superior to novices in utilizing opponent-specific action tendencies in the opponent's preceding actions (cf. Farrow and Reid, 2012; Loffing et al., 2015; Milazzo et al., 2016; Thomas et al., 2022). By systematically manipulating the dependency between action tendency information and evolving environmental information, our study provides novel support for the suggestion that the nature of the opponent's action tendencies, and the associated dependency between action tendency information and evolving environmental information, influences anticipation (cf. Gredin et al., 2020a). Furthermore, the expertise effects revealed in this study provide novel evidence that this effect is moderated by the level of domain-specific expertise. Namely, experts, but not novices, performed at their best when then the opponent's action tendencies related to both direction and action type. Novices, on the other hand, performed better when the opponent exhibited directional action tendencies, compared to when the opponent exhibited action type tendencies. These findings support the notion that experts are superior in inferring the reliability of the contextual information at hand and better able to integrate this information with unfolding environmental information, compared to their less-expert counterparts (cf. Gredin et al., 2018). However, the proposed integration requirements associated with different action tendencies, and the suggested integration behaviors across skill-groups, were not represented in the gaze data collected. The impact of opponent exposure on the ability to predict the opponent's imminent actions may be contingent upon the nature of the opponent's action tendencies; this is possibly due to different perceptual and cognitive requirements associated with different types of action tendencies. However, future research is needed to further verify this latter suggestion.
Data availability statement
The raw data supporting the conclusions of this article will be made available by the authors, without undue reservation.
Ethics statement
The studies involving human participants were reviewed and approved by the Institutional Review Board of University of Utah. The patients/participants provided their written informed consent to participate in this study.
Author contributions
NG: conceptualization and design, data interpretation, drafting the article, and final approval of the article to be published. JT: conceptualization and design, data collection, data analysis, data interpretation, critical revision of draft, and final approval of the article to be published. DB, BF, and AW: conceptualization and design, data interpretation, critical revision of draft, and final approval of the article to be published. All authors contributed to the article and approved the submitted version.
Conflict of interest
JT was employed by company Real Salt Lake.
The remaining authors declare that the research was conducted in the absence of any commercial or financial relationships that could be construed as a potential conflict of interest.
Publisher's note
All claims expressed in this article are solely those of the authors and do not necessarily represent those of their affiliated organizations, or those of the publisher, the editors and the reviewers. Any product that may be evaluated in this article, or claim that may be made by its manufacturer, is not guaranteed or endorsed by the publisher.
Author disclaimer
The authors consent to publish these data. Material has been reviewed by the Walter Reed Army Institute of Research. There is no objection to its presentation and/or publication. The opinions or assertions contained herein are the private views of the author, and are not to be construed as official, or as reflecting true views of the Department of the Army or the Department of Defense. The investigators have adhered to the policies for protection of human subjects as prescribed in AR 70–25.
References
Broadbent, D. P., Gredin, N. V., Rye, J. L., Williams, A. M., and Bishop, D. T. (2018). The impact of contextual priors and anxiety on performance effectiveness and processing efficiency in anticipation. Cogn. Emot. 33, 589–596. doi: 10.1080/02699931.2018.1464434
Brooks, J., Kerr, M., and Guttag, J. (2016). “Developing a data-driven player ranking in soccer using predictive model weights,” in Proceedings of the 22nd ACM SIGKDD International Conference on Knowledge Discovery and Data Mining - KDD '16 (New York, NY: ACM Press), 49–55. doi: 10.1145/2939672.2939695
Canãl-Bruland, R., Filius, M. A., and Oudejans, R. R. D. (2015). Sitting on a fastball. J. Motor Behav. 47, 267–270. doi: 10.1080/00222895.2014.976167
Farrow, D., and Reid, M. (2012). The contribution of situational probability information to anticipatory skill. J. Sci. Med. Sport 15, 368–373. doi: 10.1016/j.jsams.2011.12.007
Gray, R. (2002). “Markov at the bat”: a model of cognitive processing in baseball batters. Psychol. Sci. 13, 542–547. doi: 10.1111/1467-9280.00495
Gredin, N. V., Bishop, D. T., Broadbent, D. P., Tucker, A., and Williams, A. M. (2018). Experts integrate explicit contextual priors and environmental information to improve anticipation efficiency. J. Exp. Psychol. 24, 509–520. doi: 10.1037/xap0000174
Gredin, N. V., Bishop, D. T., Williams, A. M., and Broadbent, D. P. (2020a). The use of contextual priors and kinematic information during anticipation in sport: toward a Bayesian integration framework. Int. Rev. Sport Exerc. Psychol. 2020, 1–25. doi: 10.1080/1750984X.2020.1855667
Gredin, N. V., Bishop, D. T., Williams, A. M., and Broadbent, D. P. (2021). Integrating explicit contextual priors and kinematic information during anticipation. J. Sports Sci. 39, 783–791. doi: 10.1080/02640414.2020.1845494
Gredin, N. V., Broadbent, D. P., Findon, J. L., Williams, A. M., and Bishop, D. T. (2020b). The impact of task load on the integration of explicit contextual priors and visual information during anticipation. Psychophysiology 57, 1–13. doi: 10.1111/psyp.13578
Gredin, N. V., Broadbent, D. P., Thomas, J. L., and Williams, A. M. (2023). The role of action tendencies in expert anticipation. Asian J. Sport Exerc. Psychol. 2, 1. doi: 10.1016/j.ajsep.2023.02.001
Gredin, N. V., Broadbent, D. P., Williams, A. M., and Bishop, D. T. (2019). Judgement utility modulates the use of explicit contextual priors and visual information during anticipation. Psychol. Sport Exerc. 45, 101578. doi: 10.1016/j.psychsport.2019.101578
Loffing, F., and Hagemann, N. (2014). On-court position influences skilled tennis players' anticipation of shot outcome. J. Sport Exerc. Psychol. 36, 14–26. doi: 10.1123/jsep.2013-0082
Loffing, F., Sölter, F., Hagemann, N., and Strauss, B. (2016). On-court position and handedness in visual anticipation of stroke direction in tennis. Psychol. Sport Exerc. 27, 195–204. doi: 10.1016/j.psychsport.2016.08.014
Loffing, F., Stern, R., and Hagemann, N. (2015). Pattern-induced expectation bias in visual anticipation of action outcomes. Acta Psychol. 161, 45–53. doi: 10.1016/j.actpsy.2015.08.007
Mann, D. L., and Savelsbergh, G. J. P. (2015). “Issues in the measurement of anticipation,” in Routledge Handbook of Sport Expertise, eds J. Baker and D. Farrow (London: Routledge), 166–175. doi: 10.4324/9781315776675-15
Mann, D. L., Schaefers, T., and Cañal-Bruland, R. (2014). Action preferences and the anticipation of action outcomes. Acta Psychol. 152, 1–9. doi: 10.1016/j.actpsy.2014.07.004
Mann, D. T., Williams, A. M., Ward, P., and Janelle, C. M. (2007). Perceptual-cognitive expertise in sport : a meta-analysis. J. Sport Exerc. Psychol. 29, 457–478. doi: 10.1123/jsep.29.4.457
Marković, S., Cuk, I., and Živković, A. (2020). The impact of information technologies on the scouting process in sports games. Proc. Int. Sci. Conf. Sinteza 2020, 240–245. doi: 10.15308/Sinteza-2020-240-245
McMorris, T., and Colenso, S. (1996). Anticipation of professional soccer goalkeepers when facing right- and left-footed penalty kicks. Percept. Motor Skills 84, 931–934. doi: 10.2466/pms.1996.82.3.931
McRobert, A. P., Ward, P., Eccles, D. W., and Williams, A. M. (2011). The effect of manipulating context-specific information on perceptual-cognitive processes during a simulated anticipation task. Br. J. Psychol. 102, 519–534. doi: 10.1111/j.2044-8295.2010.02013.x
Memmert, D., Lemmink, K. A. P. M., and Sampaio, J. (2017). Current approaches to tactical performance analyses in soccer using position data. Sports Med. 47, 1–10. doi: 10.1007/s40279-016-0562-5
Milazzo, N., Farrow, D., Ruffault, A., and Fournier, J. F. (2016). Do karate fighters use situational probability information to improve decision-making performance during On-Mat tasks? J. Sports Sci. 34, 1547–1556. doi: 10.1080/02640414.2015.1122824
Murphy, C. P., Jackson, R. C., Cooke, K., Roca, A., Benguigui, N., and Williams, A. M. (2016). Contextual information and perceptual-cognitive expertise in a dynamic, temporally-constrained task. J. Exp. Psychol. 22, 455–470. doi: 10.1037/xap0000094
Murphy, C. P., Jackson, R. C., and Williams, A. M. (2018). The role of contextual information during skilled anticipation. Quart. J. Exp. Psychol. 71, 2070–2087. doi: 10.1177/1747021817739201
Murta, C. C. F., Albuquerque, M. R., Greco, P. J., Raab, M., and Praça, G. M. (2021). Goalkeepers put their money where the coach's mouth is: knowing kickers' preferences enhances anticipation of football goalkeepers. Int. J. Sport Exerc. Psychol. 2021, 1–16. doi: 10.1080/1612197X.2021.1987962
Navia, J. A., Van der Kamp, J., and Ruiz, L. M. (2013). On the use of situation and body information in goalkeer actions during a soccer penalty kick. Int. J. Sport Psychol. 44, 234–251.
North, J. S., Williams, A. M., Hodges, N., Ward, P., and Ericsson, K. A. (2009). Perceiving patterns in dynamic action sequences: investigating the processes underpinning stimulus recognition and anticipation skill. Appl. Cogn. Psychol. 23, 878–894. doi: 10.1002/acp,.1581
R Core Team (2017). R: A Language and Environment for Statistical Com- Puting. Vienna: R Foundation for Statistical Computing.
Roca, A., Ford, P. R., McRobert, A. P., and Williams, A. M. (2011). Identifying the processes underpinning anticipation and decision-making in a dynamic time-constrained task. Cogn. Process. 12, 301–310. doi: 10.1007/s10339-011-0392-1
Roca, A., Ford, P. R., McRobert, A. P., and Williams, A. M. (2013). Perceptual-cognitive skills and their interaction as a function of task constraints in soccer. J. Sport Exerc. Psychol. 35, 144–155. doi: 10.1123/jsep.35.2.144
Runswick, O. R., Roca, A., Williams, A. M., Bezodis, N. E., and North, J. S. (2017). The effects of anxiety and situation-specific context on perceptual–motor skill: a multi-level investigation. Psychol. Res. 17, 8. doi: 10.1007/s00426-017-0856-8
Thomas, J. L., Broadbent, D. P., Gredin, N. V., Fawver, B. J., and Williams, A. M. (2022). Skill-based differences in the detection and utilization of opponent action preferences following increasing exposure and changes in tendencies. J. Sport Exerc. Psychol. 44, 370–381. doi: 10.1123/jsep.2021-0244
Triolet, C., Benguigui, N., Le Runigo, C., and Williams, A. M. (2013). Quantifying the nature of anticipation in professional tennis. J. Sports Sci. 31, 820–830. doi: 10.1080/02640414.2012.759658
Watts, R. G., and Bahill, A. (1990). Keep Your Eye on the Ball: The Science and Folklore of Baseball. New York, NY: W.H. Freeman.
Williams, A. M., and Ford, P. R. (2008). Expertise and expert performance in sport. Int. Rev. Sport Exerc. Psychol. 1, 4–18. doi: 10.1080/17509840701836867
Keywords: action anticipation, contextual information, expertise, perceptual-cognitive skills, visual-search behavior
Citation: Gredin NV, Thomas JL, Broadbent DP, Fawver B and Williams AM (2023) Skill-based differences in the impact of opponent exposure during anticipation: the role of context-environment dependency. Front. Cognit. 2:1100911. doi: 10.3389/fcogn.2023.1100911
Received: 17 November 2022; Accepted: 20 July 2023;
Published: 08 August 2023.
Edited by:
Simon Rosalie, SR Performance, AustraliaReviewed by:
Suvobrata Mitra, Nottingham Trent University, United KingdomJames W. Roberts, Liverpool John Moores University, United Kingdom
Copyright © 2023 Gredin, Thomas, Broadbent, Fawver and Williams. This is an open-access article distributed under the terms of the Creative Commons Attribution License (CC BY). The use, distribution or reproduction in other forums is permitted, provided the original author(s) and the copyright owner(s) are credited and that the original publication in this journal is cited, in accordance with accepted academic practice. No use, distribution or reproduction is permitted which does not comply with these terms.
*Correspondence: N. Viktor Gredin, dmlrdG9yLmdyZWRpbiYjeDAwMDQwO2hoLnNl