- 1Department of Computer Sciences, College of Computer Engineering and Sciences, Prince Sattam Bin Abdulaziz University, Al-Kharj, Saudi Arabia
- 2Higher School of Communication of Tunis (SUP'COM), LR11TIC04, Communication Networks and Security Research Lab. & LR11TIC02, Green and Smart Communication Systems Research Lab, University of Carthage, Tunis, Tunisia
There are a number of effects that are associated with climate change, some of which include an increase in the frequency of severe weather events, an increase in temperatures, and changes in the patterns of rainfall. The Sustainable Development Goal 13 (SDG13) established by the United Nations emphasizes the importance of reducing green house gas emissions, improving climate resilience, and mobilizing resources to effectively tackle climate change. The use of optical wireless communication (OWC) networks is a realistic approach that has the potential to improve climate resilience and sustainable development. However, in order to successfully integrate OWC with climate change mitigation activities, it is necessary to overcome a number of obstacles. In addition to economic factors, the limitations encompass a range of obstacles and intricate legal frameworks. The purpose of this article is to study the ways in which OWC can contribute to the mitigation and adaptation to climate change effects within SGD 13, with a special emphasis on the challenges and opportunities related to its implementation.
1 Introduction
In the twenty-first century, climate change presents a significant challenge that impacts economies, ecosystems, and communities all around the world. The harmful effects of climate change, which include higher temperatures around the globe, altered patterns of rainfall, and an increase in the frequency and severity of extreme weather events, highlight the critical necessity of taking action. The United Nations developed Sustainable Development Goal 13 (SDG 13) to address this issue, emphasizing reducing greenhouse gas emissions, enhancing climate resilience, and ensuring sufficient resources for climate change efforts.
In light of the growing climate crisis, there is an increasing recognition of the critical role that technology can play in advancing sustainable solutions. Optical Wireless Communication (OWC), with its ability to transmit data at high speeds and over long distances, presents a compelling avenue for addressing climate change challenges across various sectors, as demonstrated in these studies (Aragane, 2023; Asha et al., 2023; Li et al., 2023; Ghassemlooy et al., 2015; El-Mottaleb et al., 2022). Aragane (2023) introduce the Innovative Optical and Wireless Network (IOWN) framework, emphasizing the integration of optical and wireless communication technologies to create energy-efficient, high-performance networks. This integration seeks to minimize energy consumption in data transmission by utilizing the high bandwidth and low power characteristics of optical networks, combined with the flexibility and accessibility offered by wireless networks. The IOWN program boosts network efficiency. Sustainable communication infrastructures are essential for the Internet of Things, smart cities, and future data-intensive applications. Asha et al. (2023) study optical network energy-efficient communication. The study underlines the importance of decreasing networking energy use for sustainable growth and climate change. Li et al. (2023) underline the relevance of optical communication in quantum network carbon footprint detection, which advances sustainable architectures. El-Mottaleb et al. (2022) present a new technique for optical network QoS control. The proposed fixed right shift coding technique improves optical network resource allocation and energy usage, promoting sustainable smart cities. The algorithm effectively addresses a variety of QoS requirements, thereby contributing to the increasing demand for energy-efficient and high-performing communication infrastructure.
However, it can be challenging to integrate OWC into climate change adaptation and mitigation strategies since there are numerous economic, regulatory, and technological constraints that impede the adoption and utilization of this technology. In order to effectively address climate-related environmental challenges, it is crucial to conduct a thorough investigation and develop a comprehensive understanding of the potential applications and future opportunities that OWC provides. The objective of this investigation is to conduct a comprehensive examination of the contributions that OWC has made in the struggle against climate change. This examination will encompass its applications, challenges, and potential future prospects. The objective of this initiative is to investigate the potential contributions of OWC to the advancement of sustainable development and the improvement of climate resilience. This initiative aims to enhance the public's understanding of the connection between technological advancements in communication and environmental sustainability. Consequently, this will illuminate legislative decisions, facilitate the attainment of technological advancements, and foster collaborative initiatives that will ensure a sustainable future.
We structure the remainder of the paper as follows: Section 2 will analyze several risks related to climate change risks to communication systems and critical infrastructure. In Section 3, we explore the fundamental concepts linked to optical wireless networks. Section 4 discusses the applications of optical wireless networks in climate action. Section 5 presents several real-world examples demonstrating the utilization of optical wireless networks in combating climate change. Section 6 examines the challenges related to the adoption of optical wireless networks in the context of climate action. Section 7 examines the prospective future directions and opportunities for optical wireless networks.
2 Climate change risks to communication systems and critical infrastructure
Climate change presents a considerable risk to ecosystems and infrastructure, especially concerning data transmission networks. Heatwaves, floods, storms, increase in average temperatures are cases of extreme weather phenomena that can negatively affect these networks. Figure 1 illustrates some effects of climate change. The IPCC WGII Summary for Policymakers (Intergovernmental Panel on Climate Change, 2022) indicates that environmental changes increase the vulnerabilities of infrastructure, potentially leading to the failure of particular equipment and interruptions in service during critical times. Severe weather events can significantly impact the infrastructure of communication technologies, such as towers, cables, and satellite ground stations, as noted in Liu et al. (2020).
Underground communication cables face susceptibility to flooding, resulting in potential insulation failure, signal degradation, and prolonged service interruptions. Severe winds and hurricanes pose a significant risk to communication towers and satellite ground stations, which could lead to disruptions in emergency services and essential communications (Qarahasanlou et al., 2024). Coastal infrastructure and communication networks, which depend on subterranean cables and towers, face significant risks from rising sea levels. These systems are particularly susceptible to erosion and flooding (Mohanty, 2021; Mrabet and Sliti, 2024; Desai and Rajput, 2024; Kumari, 2023). The integration of OWC into communication infrastructure has the potential to reduce reliance on susceptible physical systems while improving network resilience to disruptions caused by climate-related factors. Furthermore, OWC systems allow the rapid deployment of temporary networks in areas impacted by disasters, thereby supporting emergency communication without the need for infrastructure development. Therefore, OWC significantly improves emergency warnings by providing reliable and rapid connectivity, which in turn enhances response times and protects communities from the effects of climate change. During natural disasters like hurricanes and tsunamis, the provision of timely information is essential for preserving lives and reducing damage.
3 Optical wireless networks: fundamentals
Optical Wireless Communication technologies (Ghassemlooy et al., 2015; Chowdhury et al., 2018; Uysal and Nouri, 2014; Xu and Sadler, 2008; Kahn and Barry, 1994), including Visible Light Communication (VLC), Light Fidelity (LiFi), Optical Camera Communication (OCC), and FSO, offer significant potential for improving communication systems and supporting climate change mitigation initiatives (Chowdhury et al., 2018, 2019). Figure 2 illustrates the fundamental design of each technology. The transmitter, receiver, and communication medium fulfill specific functions within the infrastructure. Wei et al. (2019) demonstrated that VLC utilizes light-emitting diodes (LEDs) to achieve data transmission speeds exceeding 40 Gb/s through the application of advanced modulation algorithms. LiFi represents a significant advancement, targeting data rates surpassing 100 Gb/s and offering a viable alternative to conventional Wi-Fi in high-density settings (Tsonev et al., 2015). FSO facilitates secure and high-bandwidth data transmission over extended distances, eliminating the requirement for physical cabling. This provides flexibility, especially in urban areas where ground-based infrastructure might be limited (Malik and Singh, 2015). OCC employs photogrammetry to enhance vehicle localization and advance sustainable urban transportation solutions (Hossan et al., 2018).
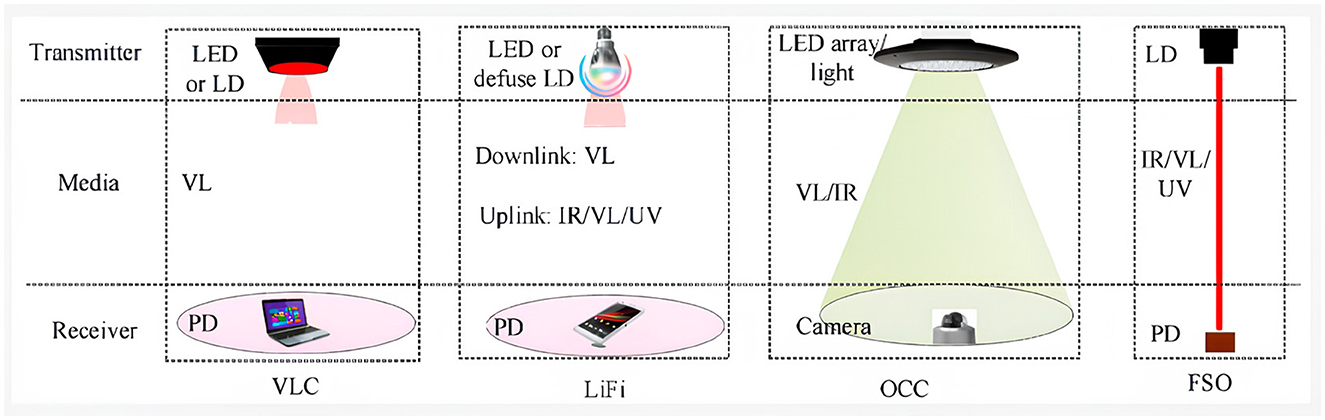
Figure 2. Various OWC technologies (Chowdhury et al., 2019).
VLC utilizes LEDs or laser diodes (LDs) for signal transmission, while photodetectors (PDs) are employed for reception. In other terms, VLC depends solely on visible light as the means of communication. VLC enables rapid wireless communication and lighting by utilizing LEDs or diffuse LDs as transmitters and PDs as receivers. VLC functions as the medium for the forward path, while LiFi utilizes infrared for the return path. The constraints of uplink connectivity in VLC and LiFi are mainly influenced by the capabilities of receiving devices, like smartphones. The link lights of optical wireless networks can interfere with the uplink, causing light dispersion that may reduce the efficiency of the return path.
OCC uses LED arrays for transmission and relies on cameras or image sensors for reception. Additionally, it features complementary metal-oxide semiconductor cameras that can capture both images and videos. The visible light spectrum, infrared spectrum, and ultraviolet spectrum serve as effective mediums for communication. In the realm of transmission, FSO generally uses laser diodes for sending signals, whereas photodiodes are employed for receiving them. The main operational principle of FSO utilizes infrared (IR) wavelengths; however, it is also capable of operating with visible light and ultraviolet wavelengths. This is what sets it apart from LiFi systems. The OCC utilizes camera or image sensor receivers in a manner that is unique compared to other methods. Conversely, FSO technology utilizes the accuracy and strength of laser light to convey information through the atmosphere, facilitating efficient and swift data transmission over extended distances. As a result, FSO systems are particularly advantageous for applications where traditional wired connections are impractical or unavailable. Table 1 presents a comparative examination of performance metrics across a variety of OWC technologies. A unique set of optical wireless network characteristics distinguishes each technology.
4 Applications of optical wireless networks in climate action
OWC provides innovative solutions to help address and decrease the effects of climate change in various sectors. OWC is critical in the fight against climate change because of its ability to transmit data at high speeds, monitor in real time, and create efficient networks using light-based communication technologies. This section examines the role of OWC in climate action initiatives across various sectors, highlighting its essential functions in both mitigation and adaptation strategies related to climate change. In this context, mitigation focuses on decreasing greenhouse gas emissions and improving energy efficiency through the use of renewable energy sources and the optimization of energy consumption. OWC technologies enable the transition by facilitating high-speed data transmission in smart grids and energy management systems, which results in reduced energy consumption and a greater reliance on sustainable energy solutions (Asha et al., 2023; Raza et al., 2024). The combination of OWC with renewable energy systems enhances the efficiency of energy delivery and consumption, a critical factor for achieving international climate targets (Li et al., 2023). On the other hand, adaptation strategies focus on the importance of real-time environmental monitoring and the establishment of regulatory frameworks designed to improve resilience against climate-related challenges. OWC significantly enhances the capacity of infrastructure and communities to respond rapidly to extreme weather events, thus promoting resource sustainability (El-Mottaleb et al., 2022). OWC enhance data transmission capabilities, enabling rapid responses to environmental changes and supporting the implementation of efficient resource management strategies (Aragane, 2023).
4.1 Environmental monitoring and sensing
The use of optical sensor networks makes it possible to deploy optical sensors in a wide variety of environmental situations. Figure 3 provides examples of OWC application in the context of environmental monitoring.
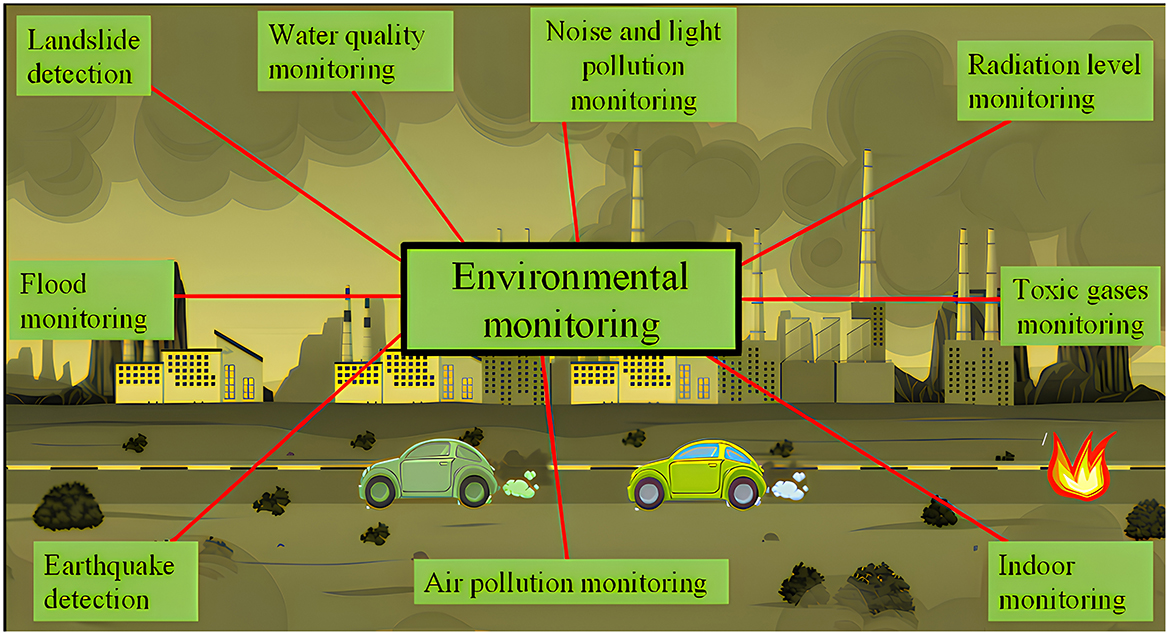
Figure 3. Application of OWC in environmental monitoring (Butt et al., 2022).
Sensors that are integrated with OWC technology are used to monitor the levels of water, the flow rates of rivers, and the patterns of rainfall in areas that are prone to floods (Shilengwe et al., 2023; Tarpanelli and Benveniste, 2019) respectively. Taking advantage of the real-time data collected by these sensors and utilizing it to develop prediction models that accurately reflect flooding events is something that governments should do in order to better its readiness and response plans. In addition, optical water control systems make use of optical sensors that are able to detect potentially harmful impurities such as carbon monoxide and sulfur dioxide. As said in the citation (Bílek et al., 2021), the fundamental purpose of this technology is to ensure the protection of the health of the general population through the activation of alarms and the development of measures to solve issues. As a result of the fact that they are able to detect concentrations as low as parts per billion (ppb), these sensors are absolutely suitable for use in metropolitan settings, which are places where it is of the utmost importance to monitor the quality of the air.
The exploitation of plasmonic nanoparticles and nanowall honeycomb structures is now conceivable for the development of ultrasensitive optical sensors for carbon dioxide gas, as demonstrated by Elrashidi et al. (2022) and Kumar and Reddy (2022). The enhancements will significantly aid in localized monitoring, facilitating the detection of pollution sources and the implementation of targeted remedies. In the context of land movement and seismic waves, the OWC has established seismic monitoring stations. In accordance with the findings presented in Huang et al. (2023) and Johni et al. (2022), this indeed contributes significantly to disaster preparedness and guarantees prompt earthquake alerts. This capability is extremely significant for reducing the intensity of the effects that earthquakes have on people and the things that they own. Notifying people in a timely manner can make a significant difference, perhaps saving lives and significantly reducing the amount of time it takes to respond to an emergency situation.
However, OWC-based environmental monitoring systems need reliable power, well-positioned sensors, and strong connectivity. In remote or underdeveloped areas, solar panels and other renewable energy sources are more reliable. Modern data compression methods improve data transit efficiency, which speeds up emergency reaction times. Data management and analysis solutions are essential for managing vast amounts of environmental data collected by OWC-equipped sensors. Table 2 illustrates the main applications of optical wireless communications in environmental monitoring.
4.2 Smart grids and energy efficiency
OWC networks significantly enhance the advancement of smart grid technologies, which are crucial for the modernization of energy infrastructure and the optimization of energy consumption in urban and rural settings as demonstrated by Zhang et al. (2023), Zhou et al. (2020), Rodríguez et al. (2023), Asha et al. (2023), and Fresno-Hernndez et al. (2023).
Integrating personalized smart meters, sensors, and actuators is really important for making demand response strategies and load balancing work effectively. This could lead to a 15% reduction in energy waste (U.S. Department of Energy, 2016) and a 10%–20% decrease in utility costs (American Council for an Energy-Efficient Economy, 2019). Demand-side management programs, including peak shaving and time-of-use pricing, encourage energy conservation and support the integration of renewable energy sources, thus improving grid sustainability. Studies demonstrate that smart grids employing optical wireless communication can enhance the integration of renewable energy by roughly 30% (National Renewable Energy Laboratory, 2018). This enhancement diminishes dependence on fossil fuels and supports the transition to a low-carbon energy future (Khan et al., 2023).
The study by Zhang et al. (2023) highlights the significance of adaptive resource provisioning in optical networks for power grids, enabling dynamic resource allocation, improving performance, and reducing operational costs. OWC enhances data transmission rates and meets the increased bandwidth requirements of 5G-enabled smart grids, facilitating real-time monitoring and management of energy resources (Zhou et al., 2020). The incorporation of wireless communication technologies in smart grid distribution networks improves reliability and efficiency, thus enhancing adaptability to variations in energy supply and demand (Rodríguez et al., 2023). Prioritizing energy-efficient communication strategies is crucial for sustainable operations. Optimized communication protocols in optical networks eliminate unnecessary path reservation criteria, thereby ensuring reliable packet transmission between nodes and enhancing the overall energy efficiency of the network (Asha et al., 2023). The advancements highlight the capacity of optical wireless networks to enhance grid stability, resilience, and sustainability.
Furthermore, OWC networks facilitate the integration of distributed energy resources such as solar panels and wind turbines, thereby advancing a more decentralized energy system. This approach not only cuts down on transmission losses but also helps lower greenhouse gas emissions. Supporting local energy generation is really important for adapting to climate change. These systems help us become more energy independent and better equipped to handle supply chain disruptions that can occur due to climate events. Meinardi et al. (2024) point out how combining certification grade quantum dot luminescent solar concentrators with OWC technology could boost energy capture and improve communication in connected sustainable architecture. Their research shows that adding OWC to solar concentrators can boost energy efficiency by as much as 25%, all while enabling real-time data transmission for improved energy management. This new approach not only helps cut down the carbon footprint of energy systems but also boosts the overall effectiveness of efforts to tackle climate change. Table 3 illustrates the main applications of optical wireless communications in smart grids and energy efficiency.
4.3 Precision agriculture and sustainable food production
OWC networks are crucial in the field of precision agriculture because they have the ability to improve resource usage, boost agricultural productivity, and lessen the impact on the environment. As depicted in Figure 4, the precision agricultural systems that are developed by OWC make use of optical sensors in the fields to measure the levels of soil moisture and nutrients, as well as to assess the general health of the crops that are being produced.
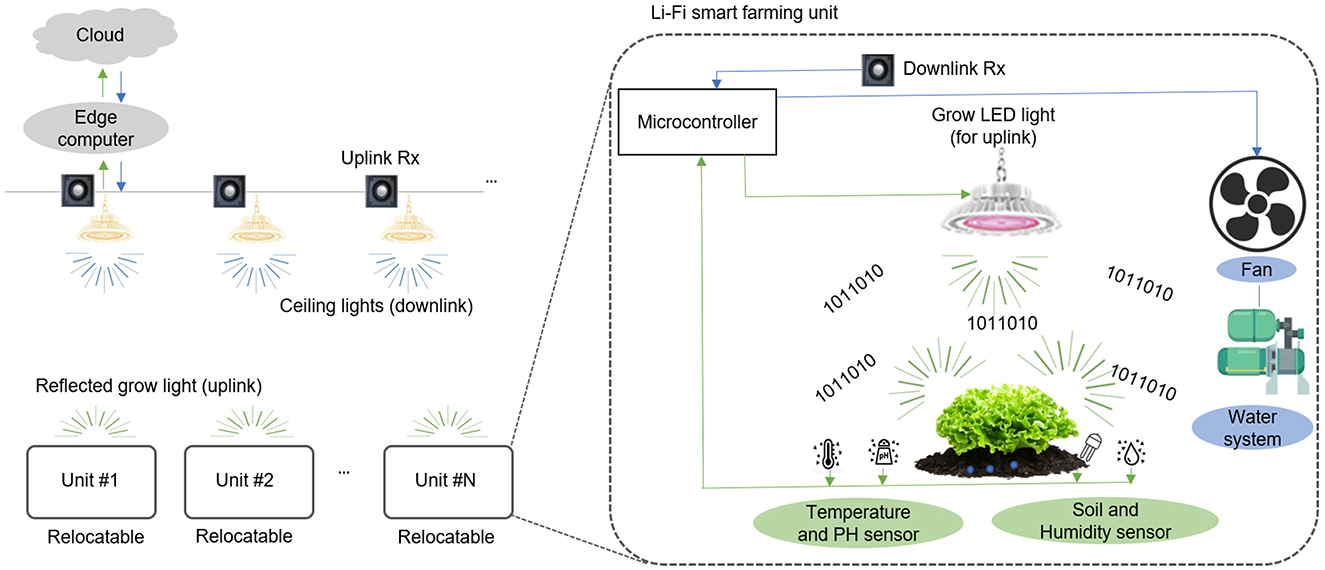
Figure 4. Applications of OWC in smart agriculture (Javed et al., 2021).
Agriculture is integrating OWC networks with sensor networks. Sensors and optical transceivers regularly monitor temperature, humidity, and soil moisture. Optical sensors perform throughout spectrums, including visible and near-infrared wavelengths (Yue et al., 2023). They enable chlorophyll detection and plant health assessments. Sensors have high electromagnetic interference resistance and appropriate data bandwidth. They established a wireless connection to a central node or gateway using optical signals. The central node is responsible for gathering information from a wide variety of sensors and sending it to a cloud-based platform for comprehensive analysis. By utilizing mobile applications or internet interfaces, farmers are given the ability to access environmental data in real time. This results in the application of procedures that are driven by data, which leads to improvements in irrigation scheduling, water conservation, and increased agricultural yields. OWC technology has been found to improve irrigation techniques by delivering water directly to the roots of plants. This is accomplished by using real-time monitoring of soil moisture. Putting these strategies into action not only lowers the operational costs that farmers have to bear, but it also improves water conservation, which leads to significant enhancements in water-use efficiency, which could potentially reach as high as 30% (Sairam, 2023). These devices are designed to reduce the likelihood of over-watering, which ultimately leads to an increase in precision and, as a consequence, a reduction in the amount of water pollution that occurs. A further indication of the significance of this matter is the possibility of fertilizer penetration into groundwater.
OWC-facilitated nutrient monitoring systems provide farmers with the ability to apply fertilizers with higher precision, which ultimately leads to enhanced nutrient uptake and less runoff into waterways (Lincy et al., 2023). The results of studies suggest that the use of these methods could result in a substantial reduction of 20%–25% in the amount of fertilizer applied, while simultaneously increasing crop yields to a higher extent. According to controlled research, farmers that make adjustments to their fertilizer treatments through the use of real-time data see a 15% boost in yields, which ultimately leads to an improvement in profitability. The study by Kirkeby et al. (2021) uses optical sensors and machine learning techniques to automatically identify flying insects. The study shows that machine learning models can classify insects trapped on yellow sticky traps using RGB images and a maximum recall of 93.97%. It highlights significant issues, since caged insects deteriorated after seven days, reducing categorization accuracy and model reliability. Model training and validation are crucial to ecological monitoring, and the authors suggest that pooling procedures may improve insect category identification rates.
Through the implementation of smart farming solutions that are made possible by OWC, it is possible to achieve the goals of increasing the effectiveness of agricultural supply chains and strengthening the resistance of food production to the effects of climate change at the same time. These kind of accomplishments are attainable through the application of a wide variety of cutting-edge agricultural practices. The utilization of data from environmental monitoring systems, in conjunction with crop growth models and weather forecasts, enables farmers to efficiently address the problems that are posed by climate extremes, such as heatwaves, droughts, and flooding. Table 4 illustrates the main applications of optical wireless communications in smart grids and energy efficiency.
4.4 Intelligent transportation systems and urban mobility
In Intelligent Transportation Systems (ITS) (Figure 5), OWC can improve vehicle-to-everything (V2X) data transfer speed and reliability. This technology allows autonomous vehicle navigation, real-time traffic management, and safety alerts. By integrating OWC, ITS can optimize traffic flow and reduce congestion, contributing to more sustainable urban mobility solutions.
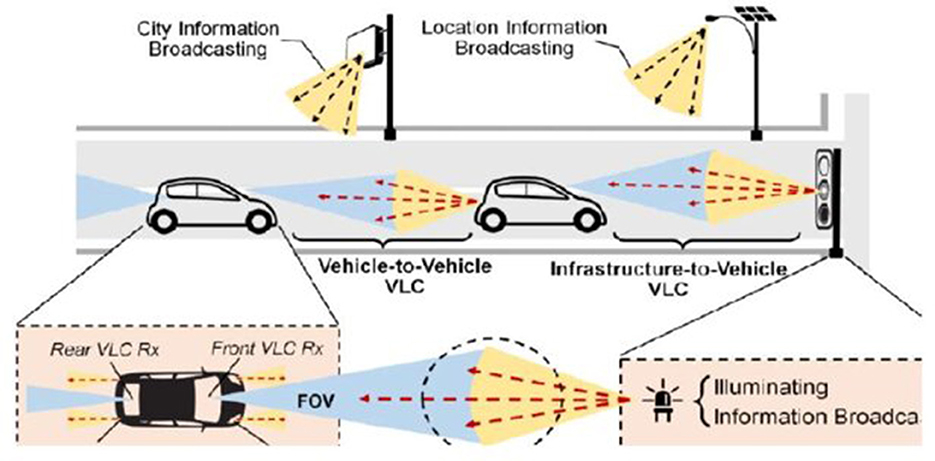
Figure 5. Application of OWC in intelligent transportation systems (Ahmed et al., 2020).
The research conducted by El-Garhy et al. (2023) examines the integration of OWC with Intelligent Transportation Systems, emphasizing the transformative potential of this technology in urban environments. This article provides a detailed analysis of the benefits and drawbacks of employing WOC in transportation networks, comparing it with other wireless technologies that are presently utilized. The research findings indicate that OWC can improve data transmission speed, decrease latency, and mitigate radio frequency congestion in urban settings. This capability is essential considering the increasing population in urban regions. ITS utilizes VLC for data transmission via light-emitting diodes. The capability for data transfer rates exceeding gigabits per second is facilitated by the rapid modulation of the LED light's intensity. This capability enables integration with diverse lighting systems and streetlights, leading to a decrease in infrastructure costs. Research has shown that vehicle-to-light communication technology facilitates the instantaneous exchange of information among vehicles, traffic signals, and infrastructure, thereby greatly improving communication within urban environments (Garai et al., 2016). This function enables drivers to remain cognizant of potential hazards, thereby enhancing traffic flow, minimizing congestion, and elevating the overall safety of the roadway for all users. The research outlined in Krichen et al. (2016) details the development of a reliable optical wireless communication system designed for monitoring the condition of railway networks. This system aims to streamline the real-time transfer of monitoring data by employing optical wireless technology. This would lead to enhanced maintenance strategies and improved safety protocols for railways. The study's findings indicate that optical networks can provide reliable and high-bandwidth communication in challenging environments, such as tunnels and rural areas, where conventional radio communication may fail. To reduce radio frequency congestion and enhance the efficiency of communication networks, FSO technology is essential in densely populated urban areas. The combination of ITS and OWC technologies, including the hybrid RF-VLC V2X communication systems examined by Singh et al. (2023), has led to significant progress in traffic management and monitoring. The use of solar-powered LED lighting alongside VLC technology positively influences pedestrian movement in urban settings. This integration facilitates high-speed communication networks, thereby playing a significant role in the reduction of carbon emissions (Huang et al., 2024).
Collecting and analyzing data from various sources, such as infrastructure and autos, is essential for intelligent algorithms to predict traffic patterns and facilitate proactive management techniques (Alharbi et al., 2023). The integration of blockchain technology and sensor networks into intelligent transportation systems enhances safety and boosts the efficiency of optical wireless communication. This combination allows intelligent transportation systems to utilize wireless sensor networks for monitoring vehicle status, identifying potential issues, and enabling timely repairs (Danys et al., 2021). Blockchain technology further improves interactions between infrastructure and vehicles while enhancing data transmission security.
The advancement of OWC technology and its integration into intelligent transportation systems is expected to significantly alter urban mobility dynamics. By combining sensor networks, machine learning algorithms, and secure data transfer protocols with VLC and FSO, there are substantial opportunities to enhance urban mobility management, reduce emissions, and build resilience against climate-related challenges. Table 5 illustrates the main applications of optical wireless communications in intelligent transportation systems.
4.5 Disaster management and resilience
Disaster management has become increasingly important in light of the impacts of climate change, with OWC technologies such as FSO and VLC presenting substantial opportunities to improve disaster resilience (Figure 6). These technologies enhance communication systems in disaster-prone regions, facilitating the rapid transmission of real-time data essential for response emergencies. Recent studies indicate that FSO systems can attain data rates similar to those of fiber optics, thereby providing dependable communication under challenging conditions. The research conducted by Jeyaseelan et al. (2019) highlights the resilience and adaptability of FSO communication in post-disaster contexts, especially under adverse weather conditions such as fog and rain. The study use adaptive modulation techniques for reliable data transmission, offering a rapid deployment solution for disaster response operations. Additionally, Mrabet and Sliti (2024) study FSO communications in arid environments, demonstrating that OWC networks can operate effectively even in extreme temperatures and during sandstorms which enhances their efficacy in managing disasters.
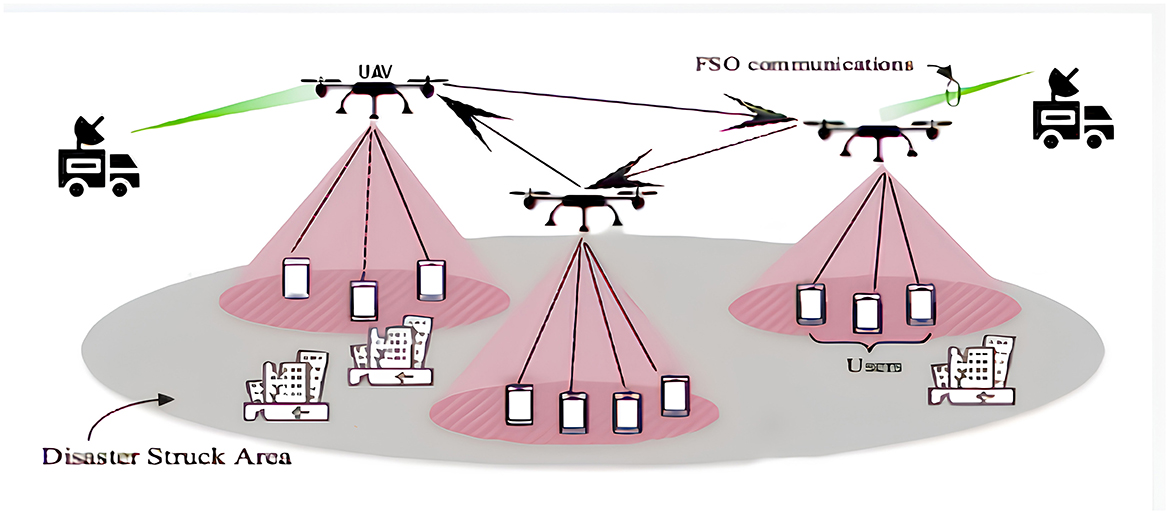
Figure 6. Application of OWC in disaster management systems (Rak et al., 2021).
In emergency situations, the implementation of VLC systems that employ LEDs for rapid data transmission can significantly improve situational awareness (Varghese et al., 2022). LEDs are capable of modulating light intensity at high frequencies, thereby enabling data transmission rates to exceed 1 Gbps.The integration of VLC with existing infrastructure, such as streetlights, results in substantial cost savings and enhanced reliability in communication networks. Research indicates that FSO communication technology can enhance real-time data transmission in environments where conventional communication infrastructures are compromised due to natural disasters. This facilitates a more timely and effective response from emergency teams. This capability is essential for preventing delays and improving overall coordination during evacuations, potentially saving lives in critical situations.
OWC technology allows drones to autonomously establish communication links, thus removing the need for ground-based infrastructure. This innovation results in a significant forty percent reduction in information transmission delays, as highlighted in Varghese et al. (2022). These enhancements are critical for protecting communities and optimizing the coordination of relief efforts. The system improves the disaster response capabilities of emergency management teams by facilitating the swift transmission of reports. However, obstacles persist in the integration of OWC technology into current disaster management systems. There is substantial potential for urban areas to address the challenges associated with climate change through the integration of OWC networks into disaster management systems. By reducing emissions and enhancing energy efficiency, the combination of FSO and VLC enhances urban resilience to climate-related disasters and promotes sustainability. Table 6 illustrates the primary applications of optical wireless communications in disaster management systems.
4.6 Satellite earth observation and climate research
Optical wireless networks play a crucial role in climate research and Earth observation by employing advanced satellite communication systems. The systems are engineered to gather, relay, and evaluate data from remote sensing platforms located in orbit around the Earth, as depicted in Figure 7. Earth observation satellites transmit substantial amounts of climate data, which encompasses sea surface temperatures, alterations in land cover, glacier melt rates, and atmospheric composition, to terrestrial receiving stations via high-capacity optical communication networks. For example, the Sentinel-2 satellites, managed by the European Space Agency, generate a daily data output of 10 gigabytes. The satellites deliver high-resolution imagery and achieve sensor data transmission rates that exceed 1 Gbps utilizing optical communication techniques. This significantly improves data transport speed compared to conventional RF systems, which operate within the range of 10–100 Mbps (European Space Agency, 2021; ApogeoSpatial, 2023).
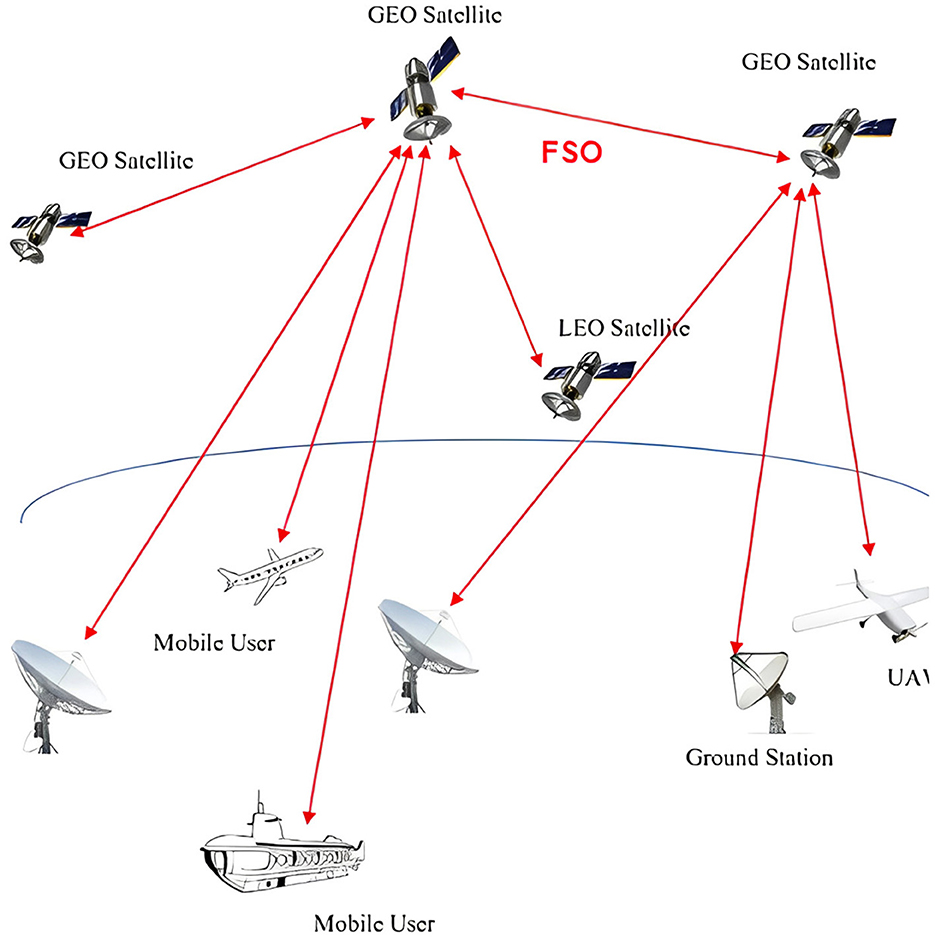
Figure 7. Utilization of OWC in satellite-based earth observation (Viswanath et al., 2016).
Satellites that utilize optical communication technology have the ability to monitor carbon dioxide levels, which reached an average of 417 ppm in 2023. This reflects a notable rise from 280 ppm before the onset of the industrial revolution (Dath and Faye, 2020). The collection of real-time data is crucial for understanding the processes underlying climate change and improving climate modeling initiatives. In addition, optical communication systems improve the functionality of catastrophe monitoring satellites. This technology enables swift reactions to natural disasters like hurricanes, floods, and wildfires by providing critical information in minutes rather than hours (Arya et al., 2023). Recent studies suggest that optical communications could significantly transform satellite systems, especially in deep space communications, while also revealing global climatic patterns. This study emphasizes the growing significance of optical communication technology in Earth monitoring and interplanetary data transmission (Karmous et al., 2024). Table 7 illustrates the main applications of optical wireless communications in satellite earth observation.
4.7 Marine conservation and ocean monitoring
Marine conservation and ocean monitoring, as depicted in Figure 8, are essential in addressing the intricate challenges posed by climate change. OWC technologies, particularly underwater visible light communication (UVLC) and free-space optical (FSO) systems, are essential for facilitating real-time data transmission and analysis in marine environments. The implementation of these technologies enhances our ability to monitor ocean conditions, which is essential for effective conservation initiatives.
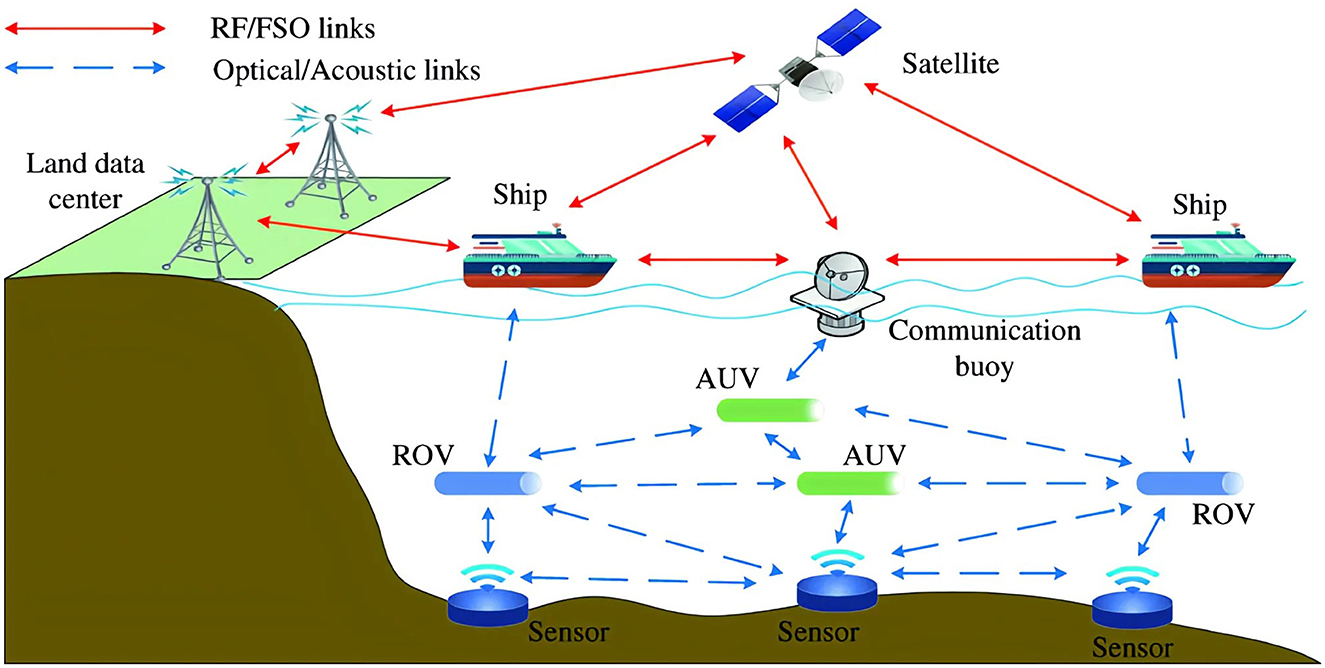
Figure 8. Application of OWC in ocean monitoring (Huang et al., 2019).
Recent advancements in optical wireless communication have shown notable improvements in data transmission rates and reliability. For example, Ali et al. (2022) developed a dual-hop mixed FSO-VLC underwater communication link that achieved a significant data rate of 1.2 Gbps over distances of up to 100 meters, even under turbulent water conditions (Arya et al., 2023). The system's performance is critical for applications such as real-time monitoring of underwater ecosystems. Rapid data transfer is essential for improving decision-making processes in conservation initiatives. Furthermore, Ali et al. (2023) conducted a performance evaluation of a vertical VLC link across various water mediums, demonstrating the technology's effectiveness in diverse aquatic environments. The results indicate that this method can maintain a low bit error rate (BER) of 10−6 over varying distances (Ali et al., 2023).
The integration of intelligent sensor systems with ocean wave energy conversion technologies enables continuous monitoring of underwater conditions. A sensor-based monitoring system was developed by Premkumardeepak and Krishnan (2017) capable of detecting pollutant concentrations as low as 0.01 mg/L in real time. The demonstrated sensitivity is crucial for timely interventions that can mitigate pollution and safeguard marine ecosystems (Premkumardeepak and Krishnan, 2017). Additionally, Biswal et al. (2023) investigated a novel underwater channel model for optical wireless communication in log-normal turbulent conditions, highlighting the importance of robust communication protocols to ensure reliable data transmission in fluctuating underwater environments (Biswal et al., 2023).
The implementation of Multiple Input Multiple Output (MIMO) systems enhances the reliability and throughput of underwater communication. A MIMO-UVLC system can achieve data rates greater than 5 Mbps while operating effectively at distances ranging from 30 to 50 meters (Sliti and Garai, 2023). This advancement enables comprehensive data collection, essential for formulating mitigation strategies and executing adaptive measures in marine conservation.
The implementation of OWC systems in marine environments is crucial for monitoring and optimizing renewable energy sources. The implementation of OWC for connecting offshore wind farms reduces carbon emissions by facilitating efficient energy distribution, thus supporting climate change mitigation efforts. Adaptation involves altering practices to reduce the impacts of climate change. In ocean monitoring, adaptation strategies may include the protection of essential habitats and the improvement of resilience in marine ecosystems. Table 8 outlines the key applications of optical wireless communications in marine conservation and ocean monitoring.
5 Real-world examples of optical wireless networks applications in climate action
OWC has a wide range of practical applications in climate action as shown in Table 9, including the promotion of environmental monitoring, the optimization of resource management, and the enhancement of renewable energy systems. Examining these examples reveals the profound impact that OWC can instigate, showcasing how technological advancements can catalyze tangible progress toward a more sustainable future.
5.1 Saudi Arabia: smart city initiative in Riyadh
The Smart Cities initiative was launched as part of Saudi Vision 2030 to address the challenges associated with rapid urbanization in Saudi Arabia, a nation with a population of 32.2 million. Seventeen localities have successfully implemented smart urban initiatives, resulting in Riyadh achieving coverage for 72% of its population. Riyadh's evolution into a smarter city serves as a case study of Saudi Arabia's transformation, as evidenced by its ranking of 30th out of 141 cities in the Smart City Index 2023 (World Competitiveness Center, 2023). Riyadh demonstrates strong performance across multiple areas, particularly in the E-Government Development Index, the Local Online Service Index, and the E-Participation Index, as indicated by evaluations from the United Nations. Riyadh is experiencing a notable transformation, propelled by financial resources and advanced technology that enable urban sustainability and development.
The communication infrastructure of the smart city initiative in Riyadh is significantly dependent on optical wireless networks (Gaul, 2021; Aldegheishem, 2023). Communications networks, positioned throughout the city, are designed to provide reliable connectivity in critical locations such as government buildings, public spaces, and major thoroughfares. The OWC networks in Riyadh provide critical high-speed wireless connectivity, enabling real-time applications such as environmental monitoring, traffic management, and video surveillance. This is accomplished by employing intricate modulation schemes and sophisticated signal processing methods. The network architecture incorporates fiber optic backhaul alongside traditional cable connections, thereby guaranteeing dependable data transmission and strong network performance, even in adverse weather conditions or other difficult scenarios. OWC networks play a vital role in the deployment of IoT sensors designed to monitor environmental conditions, facilitating the observation of pollutants, temperature, and air quality. The sensors utilize the OWC infrastructure to facilitate the wireless transmission of data to centralized monitoring centers. This enables swift analysis and informed decision-making to address environmental challenges effectively.
5.2 Saudi Arabia: smart grid infrastructure and renewable energy deployment
Saudi Arabia is making significant progress in its pursuit of sustainable energy alternatives. The country's energy framework is transforming due to continuous endeavors to modernize its energy infrastructure via projects such as Vision 2030 and the National Renewable Energy Program (NREP) (Khan et al., 2023). Saudi Arabia is investing in smart grid infrastructure. This investment enables real-time monitoring and management of energy usage, grid stability, and power quality, aligning well with the nation's aim to digitize its energy industry. This financial investment facilitates the development of a more robust and efficient energy infrastructure, hence enhancing the seamless integration of renewable energy sources.
The nation is evidently dedicated to embracing renewable energy sources, as seen by its ambitious objectives and strategic financial commitments. Saudi Arabia is advancing significantly toward a low-carbon economy by utilizing its natural resources, particularly emphasizing solar and wind energy. Coastal regions and arid zones have significant opportunities for the utilization of wind energy. The expansive desert terrains and abundant sunlight create ideal circumstances for extensive solar energy initiatives. Saudi Arabia is implementing measures to diminish its dependence on fossil resources. The nation is enhancing its energy security and diversifying its energy sources by exploring renewable options.
Furthermore, advancements in smart grid technologies, energy storage systems, and grid stability methods facilitate the integration of renewable energy sources into the power grid. These developments enable the smooth integration of intermittent renewable energy sources, such as wind and solar power, into our electrical infrastructure, while ensuring reliability and stability. Saudi Arabia is engaging in international initiatives to address climate change and advance sustainable development. This pertains to the use of renewable energy technology and a dedication to innovation that guarantees the fulfillment of our energy requirements.
5.3 Singapore: Smart Nation initiative
A sophisticated network of optical wireless communication systems meticulously established across the city-state underpins Singapore's Smart Nation initiative (Sipahi and Saayi, 2024). Singapore deliberately positions optical wireless communication networks on lampposts, skyscrapers, and other urban structures to create a robust mesh network infrastructure, guaranteeing comprehensive connectivity and coverage. Singapore's OWC networks employ millimeter-wave technology to deliver high-bandwidth wireless communication capabilities. This enables the transmission of gigabit-speed data, essential for bandwidth-demanding applications like real-time video streaming and autonomous car operation. Integrating edge computing capabilities into the OWC network's infrastructure improves the network's efficiency by facilitating local data processing. This methodology seeks to reduce time delays and diminish data requirements for cloud-based apps, ultimately improving the overall efficiency of Singapore's smart city infrastructure.
The OWC networks in Singapore are essential for environmental sensing and monitoring, as well as facilitating high-speed connectivity and data processing. The networks facilitate continuous observation of various environmental parameters, such as air quality, temperature, and meteorological conditions, utilizing IoT devices and sensors. The OWC architecture facilitates the efficient transfer of data from multiple sensors to centralized data centers for processing purposes. This data analysis delivers essential insights into environmental trends, evaluates the effects of urban activities on the environment, and supports informed decision-making aimed at mitigating environmental risks and enhancing sustainability.
5.4 Germany: urban mobility in Berlin
The integration of optical wireless communication networks plays a vital role in facilitating intelligent transportation systems and promoting sustainable urban mobility solutions in Berlin (Menge et al., 2014). The strategic implementation of OWC networks in urban environments improves traffic flow management and alleviates congestion. This is accomplished by facilitating seamless, real-time communication among vehicles, traffic signals, and transportation management centers. The incorporation of millimeter-wave technology into the OWC networks of Berlin facilitates rapid wireless communication.
The integration of edge computing into Berlin's OWC networks enhances the city's mobility ecosystem through localized data processing capabilities. This decentralized approach allows the network to analyze data and make decisions in real-time right at the interface. This means it can quickly respond to changing traffic conditions and unexpected events. Besides their important function in traffic management, OWC networks also act as essential infrastructure for shared mobility platforms and electric vehicle charging systems. OWC networks in Berlin play a significant role in reducing congestion, lowering emissions, and easing overall environmental impact by encouraging the adoption of electric vehicles and supporting shared mobility options. These initiatives align with the city's goals for sustainable urban development and its aim to improve the quality of life for its residents.
5.5 China: environmental monitoring in Beijing
Setting up optical wireless communication networks is crucial in Beijing, China, as it addresses the challenges related to environmental monitoring and pollution control (Gou and Zhao, 2020). In both suburban and urban areas, you will come across a thoughtfully designed network of optical wireless devices that forms the backbone of OWC networks. These nodes establish speedy wireless connections through advanced optical communication technology, allowing for quick and efficient data transmission.
In Beijing's OWC networks, IoT sensors are keeping an eye on various environmental parameters in real-time. These parameters include important air quality indicators like PM2.5, NO2, SO2, O3, and VOCs. We utilize advanced algorithms and models to thoughtfully examine the data we have collected. This allows us to pinpoint areas that experience high pollution levels, examine pollution trends, and even forecast air quality conditions. The information provided here is crucial for steering policy decisions and ensuring effective regulatory enforcement based on solid evidence. It provides important insights for environmental protection efforts aimed at improving the quality of life in the city and ensuring public health is prioritized.
Additionally, the OWC network in Beijing promotes community participation by launching innovative citizen science projects and hosting engaging public outreach campaigns. Involving residents in environmental monitoring initiatives allows the OWC networks to raise awareness about the environment while fostering a sense of shared responsibility. This teamwork not only boosts the community's grasp of environmental issues but also builds a sense of pride and empowerment among the residents. This, in turn, promotes positive changes in behavior and reinforces the city's dedication to sustainable development and environmental stewardship.
5.6 Japan: disaster recovery network
Japan's approach to disaster preparedness and resilience includes the creation of a Disaster Recovery Network (DRN) that leverages FSO technology (Rak et al., 2021). Setting up reliable communication systems is crucial, especially considering Japan's vulnerability to earthquakes, tsunamis, and typhoons. These systems need to be resilient enough to handle natural disasters effectively. FSO technology offers a practical solution by using optical signals to establish fast connections between nodes, instead of depending on physical cables. This technology brings a number of benefits in disaster situations, including improved security, easy deployment, resistance to physical damage, and quick data transfer capabilities.
Japan strategically places its FSO-based DRN in locations that are vulnerable to disasters, such as urban areas, coastal zones, and remote islands. It is an essential method for communicating in times of crisis. FSO transceivers make it easier for emergency response teams to coordinate and quickly share critical information over long distances. The resilience and quick connectivity truly enhance the efficiency and effectiveness of disaster response efforts. This enables swift rescue efforts, comprehensive damage evaluations, and the prompt delivery of supplies to those who require assistance.
Japan's development of FSO-based DRN really shows how proactive they are when it comes to disaster preparedness and response. It highlights just how crucial innovation and tech-driven solutions can be in these situations. The continuous investments in FSO technology are paving the way for better transmission range and reliability. This opens up some exciting opportunities to boost the resilience and strength of Japan's communication infrastructure, particularly in areas that are vulnerable to natural disasters. Japan shows its dedication to protecting people and communities during natural disasters by using FSO technology, which guarantees dependable and quick communication for emergency response teams when it matters most.
5.7 Brazil: precision agriculture
Precision agriculture employs optical wireless networks to enhance productivity, sustainability, and resource efficiency, thereby transforming the agricultural landscape in Brazil (Cherubin et al., 2022). Brazil plays a significant role in global agriculture; however, it faces the challenge of meeting increasing food demand while mitigating environmental impacts. OWC technology enhances agricultural practices by facilitating real-time monitoring of environmental factors such as temperature, crop health, and soil moisture, thereby supporting informed decision-making.
The OWC infrastructure, distributed across vast agricultural regions, facilitates effective communication among sensors, actuators, and control systems. This establishes an interconnected system that collects, analyzes, and utilizes data in real-time. This connectivity allows farmers to customize crop monitoring, precision fertilization, and variable-rate irrigation strategies to address the specific requirements of each field. Brazilian farmers can enhance sustainable agricultural practices and conserve resources through the implementation of OWC technology. This method enhances water efficiency, reduces input expenditures, and mitigates environmental effects. Real-time data insights facilitate proactive management of pests and diseases, enhancing crop resilience and decreasing dependence on chemical pesticides. In Brazil, the implementation of optical wireless networks in precision agriculture significantly enhances farm management efficiency. The focus is on optimizing yields and enhancing process efficiency. Advanced analytics and machine learning algorithms analyze extensive sensor data to yield actionable insights. These insights enable farmers to mitigate production risks, enhance their planting schedules, and more accurately forecast yield outcomes.
6 Challenges in implementing optical wireless networks for climate action
Implementing optical wireless communication networks for climate action initiatives comes with several challenges, even though they offer potential benefits. We can generally group these challenges into two main areas: technical and environmental considerations. Each of these presents its own set of obstacles that we need to tackle in order to enhance the effectiveness of OWC networks in supporting climate action initiatives.
6.1 Technical challenges
Optical wireless communication networks encounter a number of technical challenges that make it difficult for them to be widely adopted in climate action initiatives.
6.1.1 Bandwidth and data rate limitations
While optical wireless network technologies offer impressive high-speed data transmission, they often encounter bandwidth limitations, especially in bustling urban areas or regions with significant demand. To overcome bandwidth limits, we need to improve modulation methods, signal processing algorithms, and spectrum utilization strategies. This will help boost data throughput and support new applications in fields like resource management and climate monitoring.
6.1.2 Line-of-sight
Optical wireless networks communication usually depends on line-of-sight (LOS) propagation, which means that there needs to be clear visual contact between the transmitter and receiver. In cities with tall buildings, thick trees, or bad weather, line-of-sight issues can create challenges, making it difficult to set up optical wireless networks in some areas. By developing adaptive beamforming methods, relay systems, and hybrid communication architectures, we can tackle the challenges posed by line-of-sight issues and enhance the reliability, accessibility, and coverage of optical wireless networks.
6.1.3 Interference and noise
Optical wireless network communication can be affected by interference and noise from a range of sources, such as ambient light, electromagnetic radiation, and atmospheric conditions. Interference and noise can really mess with signal quality, causing issues like packet loss, increased latency, and lower data throughput. To cut down on interference and noise, it is important to use robust modulation schemes, effective error correction coding, and signal processing techniques that are tailored for optical channels. Additionally, spectrum management policies and regulatory frameworks are essential for reducing interference and ensuring that optical wireless network systems can coexist with other wireless technologies.
6.2 Regulatory challenges
Regulatory barriers, along with technical challenges, are hindering the deployment of optical wireless networks for climate action initiatives.
6.2.1 Spectrum regulations
The way spectrum allocation and licensing policies are set can really influence how optical wireless network systems are deployed and operated, especially when it comes to licensed frequency bands for RF communication. Even though optical frequencies usually do not have strict regulations, there can be challenges with spectrum sharing and managing interference in shared frequency bands. This situation often calls for coordination between various stakeholders and regulatory bodies. To meet the increasing demand for optical wireless network services and promote fair and efficient use of the spectrum, we should focus on flexible spectrum access methods, dynamic spectrum sharing frameworks, and efforts toward spectrum harmonization.
6.2.2 Security and privacy concerns
Optical wireless networks face various security threats, such as eavesdropping, jamming, and spoofing attacks. These issues can seriously compromise the confidentiality, integrity, and availability of data. To ensure the security of optical wireless network communications, it is essential to implement strong encryption methods, reliable authentication protocols, and effective intrusion detection systems. These measures help protect against malicious activities and unauthorized access. Additionally, there are privacy concerns that come into play with the collection and processing of sensitive environmental data. This brings up important ethical and legal questions about consent and accountability. Using privacy-enhancing technologies, data anonymization methods, and clear data governance frameworks can help tackle privacy issues and foster trust among stakeholders in climate action initiatives that utilize optical wireless networks.
6.3 Economic and social challenges
In addition to the technical and regulatory challenges, deploying optical wireless networks also encounters economic and social issues that impact how scalable, affordable, and accepted they are in society.
6.3.1 Cost of deployment
Deploying optical wireless networks comes with significant initial investment and operational costs. This includes expenses for equipment, installation, maintenance, and spectrum licensing fees. These costs can be quite challenging, particularly for communities with limited resources and for developing regions. To reduce the costs associated with deploying optical wireless networks, we need to focus on achieving economies of scale, developing standardized hardware and software platforms, and encouraging public-private partnerships. These steps can help drive investment and enhance market competition. Additionally, creative financing options like pay-as-you-go plans and crowdfunding efforts can help bring optical wireless network technologies to underserved areas and marginalized communities.
6.3.2 Digital divide
The uneven access to digital technologies and internet connectivity makes the gaps in climate resilience and adaptive capacity among communities around the world even wider. Deploying optical wireless networks needs to tackle the digital divide by making sure everyone has fair access to affordable broadband services, providing digital literacy training, and encouraging inclusive participation in climate action initiatives. To bridge the digital divide, we need focused interventions, strategies that engage the community, and teamwork among governments, civil society organizations, and private sector players. This collaboration is essential to empower marginalized groups and close the gap in digital access.
To tackle these challenges, we need to work together and form partnerships among various stakeholders. This collaboration is essential to navigate the technical, regulatory, economic, and social hurdles that come with deploying optical wireless networks for climate action initiatives. By tackling these challenges, optical wireless network technologies can truly realize their potential to empower communities, boost environmental sustainability, and promote climate resilience worldwide.
7 Future directions and opportunities
As optical wireless networks keep advancing, a number of future directions and opportunities come to light, opening up possibilities for innovative applications that support climate action and sustainability.
7.1 Advancements in quantum communication
Quantum communication has a lot of potential to enhance the security, reliability, and efficiency of optical wireless networks. Quantum technologies, like quantum key distribution (QKD) and communication based on quantum entanglement, provide exceptional security. They use the principles of quantum mechanics to encrypt and transmit data safely over optical channels. Future research and development in quantum communication will pave the way for incorporating quantum-enhanced security features into the infrastructure of optical wireless networks. This will help protect vital environmental data and communications from cyber threats and vulnerabilities.
7.2 Integration with emerging technologies
Optical wireless networks are set to increasingly connect with emerging technologies like blockchain, edge computing, and 5G networks, opening up exciting new opportunities for synergy and innovation. Blockchain technology provides a way to manage data that is both decentralized and transparent. This means it can enable secure and verifiable transactions in applications related to climate action, like carbon trading, renewable energy certificates, and environmental credits, especially in optical wireless networks. Edge computing platforms boost the scalability and responsiveness of optical wireless networks by handling data right at the network edge. This approach cuts down on latency and bandwidth needs for real-time applications. Additionally, the rollout of 5G networks will offer fast, low-latency connections that can enhance bandwidth-heavy applications in optical wireless networks. This includes exciting technologies like augmented reality (AR), virtual reality (VR), and autonomous systems aimed at environmental monitoring and disaster resilience.
7.3 Space-based optical communication
The emergence of space-based optical communication technologies is paving the way for exciting new possibilities in the deployment and connectivity of optical wireless networks. Satellite-based optical communication systems provide global coverage and fast data transmission, making it possible to connect everywhere and exchange information in real-time. This technology is essential for climate monitoring, disaster response, and keeping an eye on the environment. Advancements in space-based optical communication are set to enable the launch of clusters of low Earth orbit (LEO) satellites equipped with optical terminals. This will seamlessly connect remote and underserved areas, such as the polar regions, islands, and developing countries, without any issues. Space-based optical wireless networks are set to be crucial in closing the digital divide. They will empower communities by providing access to essential environmental information and resources, no matter where they are located or the limitations of existing ground infrastructure.
7.4 Green and sustainable optical wireless networks solutions
Future research and innovation will really hone in on developing green and sustainable solutions for optical wireless networks. Sustainable optical wireless network technologies will focus on energy efficiency, environmental conservation, and circular economy principles at every stage of the optical wireless network infrastructure's life cycle, including manufacturing, deployment, operation, and decommissioning. Green optical wireless network solutions will use renewable energy sources like solar and wind power to power optical transceivers, base stations, and network equipment. This approach helps to reduce carbon emissions and lessen environmental impact. We can also use sustainable materials and manufacturing processes to reduce resource consumption, waste generation, and electronic waste (e-waste) when producing and deploying optical wireless networks. Embracing green and sustainable practices in optical wireless network technologies can really help us move toward a carbon-neutral and environmentally friendly society. This aligns perfectly with global efforts to tackle climate change and reach sustainable development goals.
Author contributions
MM: Writing – original draft, Writing – review & editing. MS: Writing – original draft, Writing – review & editing.
Funding
The author(s) declare financial support was received for the research, authorship, and/or publication of this article. This project was sponsored by Prince Sattam Bin Abdulaziz University (PSAU) as part of funding for its SDG Roadmap Research Funding Programme project number PSAU-2023-SDG-2023/SDG/64.
Conflict of interest
The authors declare that the research was conducted in the absence of any commercial or financial relationships that could be construed as a potential conflict of interest.
Publisher's note
All claims expressed in this article are solely those of the authors and do not necessarily represent those of their affiliated organizations, or those of the publisher, the editors and the reviewers. Any product that may be evaluated in this article, or claim that may be made by its manufacturer, is not guaranteed or endorsed by the publisher.
References
Ahmed, M., Atta, M. A., and Bermak, A. (2020). Fast detection and tracking of a moving transmitter via visible light communication link. IEEE Photon. J. 12, 1–11. doi: 10.1109/JPHOT.2020.3012531
Aldegheishem, A. (2023). Assessing the progress of smart cities in saudi arabia. Smart Cities 6, 1958–1972. doi: 10.3390/smartcities6040091
Alharbi, F., Zakariah, M., Alshahrani, R., Albakri, A., Viriyasitavat, W., and Alghamdi, A. A. (2023). Intelligent transportation using wireless sensor networks blockchain and license plate recognition. Sensors 23:2670. doi: 10.3390/s23052670
Ali, M. F., Jayakody, D. N. K., Beko, M., and Correia, S. D. (2023). “Performance evaluation of vertical vlc link in mixed water mediums,” in 2023 6th Conference on Cloud and Internet of Things (CIoT), 195–199. doi: 10.1109/CIoT57267.2023.10084904
Ali, M. F., Jayakody, D. N. K., Garg, S., Kaddoum, G., and Hossain, M. S. (2022). Dual-hop mixed FSO-VLC underwater wireless communication link. IEEE Trans. Netw. Serv. Manag. 19, 3105–3120. doi: 10.1109/TNSM.2022.3181169
American Council for an Energy-Efficient Economy (2019). The role of demand-side management in energy savings. Technical report, ACEEE.
ApogeoSpatial. Moving Data at the Speed of Light. (2023). Available at: https://apogeospatial.com/moving-data-at-the-speed-of-light/ (accessed October 14, 2024).
Aragane, Y. (2023). “Innovative optical and wireless network (IOWN) for a sustainable world,” in 2023 Optical Fiber Communications Conference and Exhibition (OFC), 1–3. doi: 10.23919/OFC49934.2023.10117358
Arya, V., Kumari, M., Al-Khafaji, H. M. R., and Aljunid, S. A. (2023). Modeling of satellite-to-underwater integrated fso-pon system using noma-VLC. Symmetry 15:739. doi: 10.3390/sym15030739
Asha, P., Kalaavathi, B., Kumari, K. S., Malarvizhi, K., Kumar, A. K., and Ahila, S. S. (2023). Energy-efficient and sustainable communication in optical networks for eliminating path reservation criteria and providing guaranteed packet transmission between nodes. Neur. Comput. Applic. 2023, 1–8. doi: 10.1007/s00521-023-08866-6
Avar, E., and Mowla, M. N. (2022). Wireless communication protocols in smart agriculture: a review on applications, challenges and future trends. Ad Hoc Netw. 136:102982. doi: 10.1016/j.adhoc.2022.102982
Bagha, H., Yavari, A., and Georgakopoulos, D. (2021). “Iot-based plant health analysis using optical sensors in precision agriculture,” in 2021 Digital Image Computing: Techniques and Applications (DICTA), 01–08. doi: 10.1109/DICTA52665.2021.9647066
Bílek, J., Bílek, O., Maršolek, P., and Buček, P. (2021). Ambient air quality measurement with low-cost optical and electrochemical sensors: an evaluation of continuous year-long operation. Environments. 8:114. doi: 10.3390/environments8110114
Biswal, M. R., Delwar, T. S., Siddique, A., Behera, P., and Ryu, J.-Y. (2023). Analysis and investigation of a novel underwater channel model for owc under log-normal turbulent condition. Photonic Netw. Commun. 47, 1–8. doi: 10.1007/s11107-023-01006-z
Butt, M. A., Voronkov, G. S., Grakhova, E. P., Kutluyarov, R. V., Kazanskiy, N. L., and Khonina, S. N. (2022). Environmental monitoring: a comprehensive review on optical waveguide and fiber-based sensors. Biosensors 12:1038. doi: 10.3390/bios12111038
Cherubin, M. R., Damian, J. M., Tavares, T. R., Trevisan, R. G., Colao, A. F., Eitelwein, M. T., et al. (2022). Precision agriculture in brazil: the trajectory of 25 years of scientific research. Agriculture 12:1882. doi: 10.3390/agriculture12111882
Chowdhury, M. Z., Hossan, M. T., Islam, A., and Jang, Y. M. (2018). A comparative survey of optical wireless technologies: architectures and applications. IEEE Access 6, 9819–9840. doi: 10.1109/ACCESS.2018.2792419
Chowdhury, M. Z., Shahjalal, M., Hasan, M. K., and Jang, Y. M. (2019). The role of optical wireless communication technologies in 5g/6g and iot solutions: Prospects, directions, and challenges. Appl. Sci. 9:4367. doi: 10.3390/app9204367
Danys, L., Martínek, R., Jaros, R., Baros, J., Simoník, P., and Snás~el, V. (2021). Enhancements of sdr-based fpga system for v2x-vlc communications. Comput. Mater. Continua. 68:e17333. doi: 10.32604/cmc.2021.017333
Dath, C. A. B., and Faye, N. A. B. (2020). Resilience of long range free space optical link under a tropical weather effects. Sci. Afr. 7:e00243. doi: 10.1016/j.sciaf.2019.e00243
de Arruda Viana, L., Tomaz, D. C., Martins, R. N., Rosas, J. T. F., dos Santos, F. F. L., and Portes, M. F. (2019). Optical sensors for precision agriculture: an outlook. J. Exper. Agric. Int. 35, 1–9. doi: 10.9734/jeai/2019/v35i230203
Desai, N. S., and Rajput, S. J. (2024). Exploring fso link performance in varied atmospheric conditions to optimize 5G communication with a polarized quasi-diffuse transmitter. J. Opt. Commun. 2024:49. doi: 10.1515/joc-2024-0049
El-Garhy, S. M., Khalaf, A. A. M., Abaza, M. R., and Aly, M. H. (2023). Intelligent transportation system using wireless optical communication: a comparative study. Opt. Quant. Electr. 56, 1–15. doi: 10.1007/s11082-023-05811-8
El-Mottaleb, S. A. A., Métwalli, A., Chehri, A., Ahmed, H. Y., Zeghid, M., and Khan, A. N. (2022). New algorithm for a fixed right shift code to support different quality of services in smart and sustainable optical networks. Sustainability 14:10337. doi: 10.3390/su141610337
Elrashidi, A., Traversa, E., and ElZein, B. (2022). Highly sensitive ultra-thin optical co2 gas sensors using nanowall honeycomb structure and plasmonic nanoparticles. Front. Energy Res. 10:909950. doi: 10.3389/fenrg.2022.909950
European Space Agency (2021). SENTINEL-2 User Handbook [Online]. Available at: https://sentinels.copernicus.eu
Fauzi, N. I. M., Fen, Y. W., Omar, N. A. S., and Hashim, H. S. (2021). Recent advances on detection of insecticides using optical sensors. Sensors 21:3856. doi: 10.3390/s21113856
Fresno-Hernndez, A., Rodrguez-Guerra, M., Rodrguez-Garrido, R., and Vzquez, C. (2023). Downtaper on multimode fibers towards sustainable power over fiber systems. Photonics 10:513. doi: 10.3390/photonics10050513
Garai, M., Sliti, M., and Boudriga, N. (2016). “Access and resource reservation in vehicular visible light communication networks,” in 18th International Conference on Transparent Optical Networks, ICTON 2016, Trento, Italy, July 10–14, 2016 (IEEE), 1–6. doi: 10.1109/ICTON.2016.7550370
Gaul, V. (2021). Saudi arabia smart cities market. Available at: https://www.alliedmarketresearch.com/saudi-arabia-smart-cities-market-A10247 (accessed April 14, 2024).
Georlette, V., Moeyaert, V., Bette, S., and Point, N. (2020). “Outdoor optical wireless communication: potentials, standardization and challenges for smart cities,” in 2020 29th Wireless and Optical Communications Conference (WOCC), 1–6. doi: 10.1109/WOCC48579.2020.9114953
Ghassemlooy, Z., Arnon, S., Uysal, M., Xu, Z., and Cheng, J. (2015). Emerging optical wireless communications-advances and challenges. IEEE J. Selected Areas Commun. 33, 1738–1749. doi: 10.1109/JSAC.2015.2458511
Gou, R., and Zhao, J. (2020). Eco-environmental quality monitoring in Beijing, China, using an rsei-based approach combined with random forest algorithms. IEEE Access 8, 196657–196666. doi: 10.1109/ACCESS.2020.3034376
Hossan, M., Chowdhury, M. Z., Hasan, M., Shahjalal, M., Nguyen, T., Le, N. T., et al. (2018). A new vehicle localization scheme based on combined optical camera communication and photogrammetry. Mobile Inform. Syst. 2018, 1–10. doi: 10.1155/2018/8501898
Huang, J., Yu, T., Zhu, X., Yang, F., Lai, X., Alfarraj, O., et al. (2024). Energy efficiency maximization in uav-assisted intelligent autonomous transport system for 6g networks with energy harvesting. IEEE Trans. Intell. Transpor. Syst. 2024, 1–11. doi: 10.1109/TITS.2024.3445088
Huang, W., Zhang, W., Li, L., Zhang, H.-Y., and Li, F. (2023). Review on low-noise broadband fiber optic seismic sensor and its applications. J. Lightwave Technol. 41, 4153–4163. doi: 10.1109/JLT.2023.3253661
Huang, X., Yang, F., and Song, J. (2019). Hybrid ld and led-based underwater optical communication: state-of-the-art, opportunities, challenges, and trends. Chin. Opt. Lett. 17:100002. doi: 10.3788/COL201917.100002
Intergovernmental Panel on Climate Change (2022). Climate change 2022: Impacts, adaptation, and vulnerability. Summary for Policymakers. Genebra, Sua. doi: 10.1017/9781009325844
Jain, N., Awasthi, Y., and Jain, R. K. (2023). An iot-based soil analysis system using optical sensors and multivariate regression. Int. J. Exp. Res. Rev. 31, 23–32. doi: 10.52756/10.52756/ijerr.2023.v31spl.003
Javed, S., Issaoui, L., Cho, S.-D., and Chun, H. (2021). Utilization of led grow lights for optical wireless communication-based RF-free smart-farming system. Sensors 21:6833. doi: 10.3390/s21206833
Jeyaseelan, J., Kumar, D. S., and Caroline, B. E. (2019). Disaster management using free space optical communication system. Photonic Netw. Commun. 39, 1–14. doi: 10.1007/s11107-019-00865-9
Johni, R. A., Tariq, K. R., Ahmadhamdi, R. T., and Forsyth, D. I. (2022). Investigation into fiber optic seismic sensor incorporating fiber Bragg grating array. Passer J. Basic Appl. Sci. 4, 91–99. doi: 10.24271/psr.2022.318987.1111
Kahn, J. M., and Barry, J. R. (1994). Wireless infrared communications. Proc. IEEE 85, 265–298. doi: 10.1109/5.554222
Karmous, S., Adem, N., Atiquzzaman, M., and Samarakoon, S. (2024). How can optical communications shape the future of deep space communications? A survey. IEEE Commun. Surv. Tutor. 2024, 1–11. doi: 10.1109/COMST.2024.3403873
Khan, A., Quamar, M. M., Al-Qahtani, F. H., Asif, M., Alqahtani, M., and Khalid, M. (2023). Smart grid infrastructure and renewable energy deployment: a conceptual review of Saudi Arabia. Energy Strat. Rev. 50:101247. doi: 10.1016/j.esr.2023.101247
Kirkeby, C. T., Rydhmer, K., Cook, S. M., Strand, A., Torrance, M. T., Swain, J. L., et al. (2021). Advances in automatic identification of flying insects using optical sensors and machine learning. Sci. Rep. 11:1555. doi: 10.1038/s41598-021-81005-0
Krichen, D., Abdallah, W., and Boudriga, N. (2016). “An optical wireless network for railways condition monitoring,” in 2016 22nd Asia-Pacific Conference on Communications (APCC), 153–160. doi: 10.1109/APCC.2016.7581506
Kumar, A. P., and Reddy, C. A. (2022). “Comprehensive analysis on low power health monitoring system using fog computing in passive optical networks,” in 2022 Sixth International Conference on I-SMAC (IoT in Social, Mobile, Analytics and Cloud) (I-SMAC), 476–484. doi: 10.1109/I-SMAC55078.2022.9987329
Kumari, M. (2023). “Symmteric and integrated fso and rgb leds based vlc system under severe climate conditions,” in 2023 3rd International Conference on Mobile Networks and Wireless Communications (ICMNWC), 1–6. doi: 10.1109/ICMNWC60182.2023.10435736
Li, X., Wang, T., and Li, L. (2023). Impact optical communication model in sustainable building construction over the carbon footprint detection using quantum networks. Opt. Quant. Electr. 55, 1–17. doi: 10.1007/s11082-023-05191-z
Lincy, C. T., Lenin, F. A., and Jalbin, J. (2023). Deep residual network for soil nutrient assessment using optical sensors. J. Plant Nutr. Soil Sci. 187, 181–194. doi: 10.1002/jpln.202300310
Liu, Y., Wei, S., Zhao, D., and bo Gao, J. (2020). Progress in research on the influences of climatic changes on the industrial economy in China. J. Resour. Ecol. 11, 1–12. doi: 10.5814/j.issn.1674-764x.2020.01.001
Malik, A., and Singh, P. (2015). Free space optics: current applications and future challenges. Int. J. Opt. 2015, 1–10. doi: 10.1155/2015/945483
Meinardi, F., Bruni, F., Castellan, C., Meucci, M., Umair, A. M., Rosa, M. L., et al. (2024). Certification grade quantum dot luminescent solar concentrator glazing with optical wireless communication capability for connected sustainable architecture. Adv. Energy Mater. 14:2304006. doi: 10.1002/aenm.202304006
Menge, J., Horn, B., and Beck, B. (2014). Berlin's Urban Transportation Development Plan 2025 - Sustainable Mobility. Berlin: Berlin's Senate Department for Urban Development and the Environment.
Mohanty, A. K. (2021). “Impacts of climate change on human health and agriculture in recent years,” in 2021 IEEE Region 10 Symposium (TENSYMP), 1–4. doi: 10.1109/TENSYMP52854.2021.9550876
Mrabet, M., and Sliti, M. (2024). Performance analysis of FSO communications in desert environments. Opt. Quant. Electr. 68:659. doi: 10.1007/s11082-024-06315-9
National Renewable Energy Laboratory (2018). Smart grid and renewable energy integration. Technical report, NREL.
Oladimeji, D., Gupta, K., Kose, N. A., Gundogan, K., Ge, L., and Liang, F. (2023). Smart transportation: an overview of technologies and applications. Sensors 23:3880. doi: 10.3390/s23083880
Premkumardeepak, S., and Krishnan, M. B. M. (2017). “Intelligent sensor based monitoring system for underwater pollution,” in 2017 International Conference on IoT and Application (ICIOT), 1–4. doi: 10.1109/ICIOTA.2017.8073626
Putra, E. P., Theivindran, R., Hasnul, H., Lee, H. J., Ker, P. J., bin Jamaludin, M. Z., et al. (2023). Technology update on patent and development trend of power over fiber: a critical review and future prospects. J. Photon. Energy 13:011001. doi: 10.1117/1.JPE.13.011001
Qarahasanlou, A. N., Garmabaki, A. H. S., Kasraei, A., and Barabady, J. (2024). “Climate change impacts on mining value chain: a systematic literature review,” in International Congress and Workshop on Industrial AI and eMaintenance 2023, eds. U. Kumar, R. Karim, D. Galar, and R. Kour (Cham. Springer Nature Switzerland), 115–128. doi: 10.1007/978-3-031-39619-9_9
Rak, J., Girão-Silva, R., Gomes, T., Ellinas, G., Kantarci, B., and Tornatore, M. (2021). Disaster resilience of optical networks: state of the art, challenges, and opportunities. Optics Switch. Netw. 42:100619. doi: 10.1016/j.osn.2021.100619
Raza, A., Liaqat, M., Adnan, M., Iqbal, M. S., Li, J., and Ahmad, I. (2024). Saarc super smart grid: navigating the future - unleashing the power of an energy-efficient integration of renewable energy resources in the Saarc region. Comput. Electr. Eng. 118:109405. doi: 10.1016/j.compeleceng.2024.109405
Rodríguez, J. C., Grijalva, F., García, M., Barragán, D. E. C., Acurio, B. A. A., and Carvajal, H. (2023). “Wireless communication technologies for smart grid distribution networks,” in XXXI Conference on Electrical and Electronic Engineering. doi: 10.3390/engproc2023047007
Safari, A., and Kharrati, H. (2023). “Application of optical wireless communications in iot devices of smart grids within smart sustainable cities: with hybrid perspectives to metaverse &quantum iot,” in 2023 8th International Conference on Technology and Energy Management (ICTEM), 1–7. doi: 10.1109/ICTEM56862.2023.10083835
Sairam, M. (2023). Hand-held optical sensors for optimizing nitrogen application and improving nutrient use efficiency. Int. J. Bioresour. Sci. 10, 9–18. doi: 10.30954/2347-9655.01.2023.2
Shilengwe, C., Nyimbili, P. H., Msendo, R., Banda, F., Mukupa, W., and Erden, T. (2023). Synthetic aperture radar and optical sensor techniques using google earth engine for flood monitoring and damage assessment — a case study of Mumbwa District, Zambia. Zambia ICT J. 7, 7–15. doi: 10.33260/zictjournal.v7i1.122
Singh, H., Miglani, R., Mittal, N., Gupta, S., Tubbal, F. E. M., Raad, R., et al. (2023). Designing an optimized free space optical (FSO) link for terrestrial commercial applications under turbulent channel conditions. Opt. Quant. Electr. 55, 1–24. doi: 10.1007/s11082-023-04805-w
Sipahi, B., and Saayi, Z. (2024). The world's first ‘smart nation' vision: the case of singapore. Smart Cities Reg. Dev. J. 8, 41–58. doi: 10.25019/dvm98x09
Sliti, M., and Boudriga, N. (2021a). “Bridge structural health monitoring using an fbg-based architecture,” in 2021 IEEE International Midwest Symposium on Circuits and Systems (MWSCAS), 621–625. doi: 10.1109/MWSCAS47672.2021.9531805
Sliti, M., and Boudriga, N. (2021b). “Building structural health monitoring: an fbg-based estimation of external vibrations,” in 2021 18th International Multi-Conference on Systems, Signals &Devices (SSD), 1026–1031. doi: 10.1109/SSD52085.2021.9429378
Sliti, M., and Garai, M. (2023). “Performance analysis of mimo-underwater optical wireless communication,” in 2023 28th Asia Pacific Conference on Communications (APCC), 301–306. doi: 10.1109/APCC60132.2023.10460667
Tarpanelli, A., and Benveniste, J. (2019). “On the potential of altimetry and optical sensors for monitoring and forecasting river discharge and extreme flood events,” in Extreme Hydroclimatic Events and Multivariate Hazards in a Changing Environment (Elsevier), 267–287. doi: 10.1016/B978-0-12-814899-0.00011-0
Tsonev, D., Videv, S., and Haas, H. (2015). Towards a 100 gb/s visible light wireless access network. Opt. Expr. 23, 1627–1637. doi: 10.1364/OE.23.001627
U.S. Department of Energy (2016). Smart Grid System Report. Dataset. Available at: https://www.energy.gov (accessed October 14, 2024).
Uysal, M., and Nouri, H. (2014). “Optical wireless communications–an emerging technology,” in 2014 16th International Conference on Transparent Optical Networks (ICTON), 1–7. doi: 10.1109/ICTON.2014.6876267
Varghese, B., Kannan, P. S., Jayanth, R. S., Thomas, J. H., and Kumar, K. M. B. S. (2022). Drone deployment algorithms for effective communication establishment in disaster affected areas. Computer 11:139. doi: 10.3390/computers11090139
Viswanath, A., Jain, V. K., and Kar, S. (2016). “Performance evaluation of satellite-to-earth fso link in presence of turbulence and weather conditions for different im schemes,” in 2016 Twenty Second National Conference on Communication (NCC), 1–5. doi: 10.1109/NCC.2016.7561092
Wei, L. Y., Chow, C. W., Chen, G. H., Liu, Y., Yeh, C. H., and Hsu, C. W. (2019). Tricolor visible-light laser diodes based visible light communication operated at 40.665 gbit/s and 2 m free-space transmission. Opt. Expr. 27, 25072–25077. doi: 10.1364/OE.27.025072
World Competitiveness Center (2023). Imd smart city index 2023. Available at: https://www.imd.org/wp-content/uploads/2023/06/SmartCityIndex-2023-V8.pdf (accessed April 14, 2024).
Xu, Z., and Sadler, B. M. (2008). Ultraviolet communications: potential and state-of-the-art. IEEE Commun. Mag. 46, 67–73. doi: 10.1109/MCOM.2008.4511651
Yahia, O. B., Erdogan, E., Kurt, G. K., Altunbas, I., and Yanikomeroglu, H. (2021). A weather-dependent hybrid rf/fso satellite communication for improved power efficiency. IEEE Wirel. Commun. Lett. 11, 573–577. doi: 10.1109/LWC.2021.3136444
Yue, J., Zhou, C., Feng, H., Yang, Y., and Zhang, N. (2023). Novel applications of optical sensors and machine learning in agricultural monitoring. Agriculture 13:1970. doi: 10.3390/books978-3-0365-9799-7
Zhang, H., Fu, Z., Wang, Q., Zhang, X., Wang, H., and Gu, R. (2023). “Adaptive resource provisioning in optical network for power grid,” in 2023 8th IEEE International Conference on Network Intelligence and Digital Content (IC-NIDC), 331–335. doi: 10.1109/IC-NIDC59918.2023.10390889
Keywords: climate change, optical wireless communication networks, applications, challenges, opportunities, Sustainable Development Goal 13
Citation: Mrabet M and Sliti M (2024) Climate change mitigation and adaptation through optical-wireless communication networks: applications, challenges, and opportunities. Front. Clim. 6:1480190. doi: 10.3389/fclim.2024.1480190
Received: 14 August 2024; Accepted: 01 November 2024;
Published: 29 November 2024.
Edited by:
Gengxin Chen, Chinese Academy of Sciences (CAS), ChinaReviewed by:
Rongwang Zhang, Chinese Academy of Sciences (CAS), ChinaCarlos Luis Mendez Vallejo, Venezuelan Institute for Scientific Research (IVIC), Venezuela
Copyright © 2024 Mrabet and Sliti. This is an open-access article distributed under the terms of the Creative Commons Attribution License (CC BY). The use, distribution or reproduction in other forums is permitted, provided the original author(s) and the copyright owner(s) are credited and that the original publication in this journal is cited, in accordance with accepted academic practice. No use, distribution or reproduction is permitted which does not comply with these terms.
*Correspondence: Manel Mrabet, TS5iZW5yYXNoZWQmI3gwMDA0MDtwc2F1LmVkdS5zYQ==