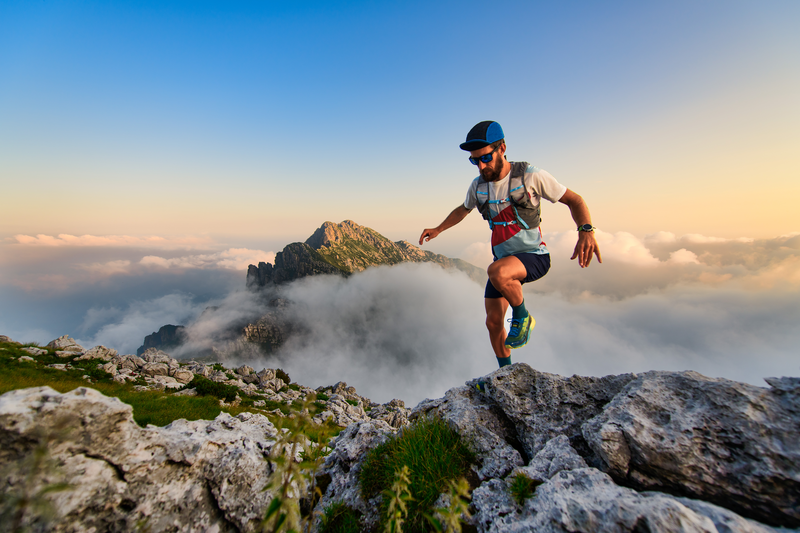
94% of researchers rate our articles as excellent or good
Learn more about the work of our research integrity team to safeguard the quality of each article we publish.
Find out more
REVIEW article
Front. Clim. , 05 November 2024
Sec. Climate Action
Volume 6 - 2024 | https://doi.org/10.3389/fclim.2024.1478923
This article is part of the Research Topic Climate change mitigation strategies for sustainable crop production in sub-Saharan Africa View all 6 articles
Land degradation is a major problem in Ethiopia, as it contributes to climate change by releasing greenhouse gases (GHG) and slowing carbon sequestration rates. The objective of this review was to assess the role of conservation agriculture (CA) in climate change adaptation and mitigation in Ethiopia. The critical review method processes for identifying and synthesizing peer-reviewed research and review articles, reports, proceedings, and book chapters were followed, with materials obtained from relevant search engines. The findings of the various reports revealed that minimum tillage assists in soil moisture conservation when compared to conventional tillage. Conservation tillage maintains crop residue, reduces soil temperature significantly, and increases nutrient buildup in the surface soil layer, all of which lead to higher crop growth and production thus help as adaptation to climate change. Furthermore, agriculture and other land uses significantly contribute to greenhouse gas emissions; nevertheless, conservation agricultural methods improve soil organic carbon (SOC), soil aggregation, and carbon in aggregate, as well as soil health that contribute to climate changing mitigation. Several studies found that soil health indicators such as soil aggregation, soil organic carbon storage, soil enzymes, and microbial biomass improved under conservation tillage practices, potentially improving the carbon-nitrogen cycle, soil stability, and overall crop productivity. In terms of climate adaptation and mitigation, CA is one of the non-substitutable choices for reducing greenhouse gas emissions. Crop diversity, increased nitrogen consumption efficiency, crop rotation, improved soil carbon sequestration methods; crop residue retention, minimum soil disturbance, manure incorporation, and integrated farming systems are all important factors in minimizing GHG emissions. Moreover, factors impeding CA adoption include a lack of appropriate equipment and machinery, weed control methods, the use of crop residue for fuel wood and animal feed, a lack of awareness about the benefits of CA on soil health and sustainability, and a lack of government technical and financial support for smallholder farmers. Adoption and scaling up of CA practices are critical for ensuring a sustainable development goal and resilient future. Thus, relevant stakeholders should consider the aforementioned considerations while promoting the technology on a large scale through integration with enhanced technology.
Land degradation occurs when soils lose their productive capacity over time due to interconnected processes such as decreased vegetation cover and biodiversity, erosion, and nutrient loss (Grinand et al., 2019). Globally, land degradation affects around 30% of land area, threatening billions of people who live in degraded areas (Nkonya et al., 2016). Although information on land degradation in sub-Saharan Africa (SSA) is sparse, data from various researches undertaken in SSA at broad regional and temporal dimensions revealed clear evidence of soil degradation (Tully et al., 2015). Further, land degradation is a major issue in Ethiopia, particularly in highland areas that occupy over half of the country. The Highland area has the majority of the people (90%), livestock (60%), and approximately 90% of the land suitable for cultivation. Soil erosion has been severe throughout the Highlands, mostly in cultivated areas, posing significant challenges to food security (Hurni et al., 2010). Furthermore, around 41.9 tons per hectare of organic carbon accumulates in the soil, with the bulk found in the highland areas of smallholder farms (Shumete, 2015). Gebreselassie et al. (2016) revealed that more than 85% of land area degraded to varying degrees, and roughly 23% of land degradation hot spot areas were assessed using satellite imagery over the last three decades.
Land degradation contributes to climate change by emitting greenhouse gases (GHG) and decreasing carbon sequestration rates. Climate change also exacerbates the severity and extent of existing land degradation processes, creating a conducive environment for novel degradation patterns. Land degradation have a substantial impact on the exchange of water, energy, GHGs, non-GHGs, and aerosols in the atmosphere (Jia et al., 2019; Olsson et al., 2019). As a result, the effects of land degradation and climate change are inextricably linked; hence, efforts to restore degraded land and avoid further exposure to the degradation process are critical in climate change mitigation efforts. Land degradation is the primary cause of Ethiopia’s deteriorating agricultural production, food insecurity, rural poverty, and other economic and social implications. The country’s minimum yearly cost of land degradation ranges between 2 and 3 percent of agricultural growth and growth domestic product (GDP). This is a huge loss for the agricultural sector, which accounts for about half of GDP, 90% of export revenue, and provides a living for more than 85% of the country’s people (Shumete, 2015).
Several studies have reported that the major factors that cause land degradation in Ethiopia are expansion of agriculture, repeated cultivation, removal of residuals and dungs, single cropping rather than intercropping, extraction of wood, expansion of infrastructure, rainfall variability, increasing rate of population growth, deforestation activities, soil and water erosion, cultivation on steep slopes, overgrazing, traditional way of cultivation, heavy wind, high intensity of rainfall, drought and landslides, absence of all-inclusive land use and lack of land use plans developed, inadequate extension services, political instability, rapid population growth, significant soil erosion, overgrazing, insufficient vegetative cover, uneven crop production on steep slopes, erosive rainfall patterns, a lack of fallowing, and insufficient conservation measures and additionally topography, soil factor, and agro-ecological factor of the area (Temesgen et al., 2014; Megerssa and Bekere, 2019; Muke, 2019; Tadesse and Hailu, 2024). Thus, the issue of land degradation has to be addressed by addressing its local causes. Identifying household behavior about land management, policy, and institutional elements that influence decisions is critical (Gebreselassie et al., 2016). The most essential choices for land degradation control include proper tillage, agricultural residue management, crop rotations, agroforestry, and the use of both organic and inorganic fertilizers, liming, and cover crops (Gedamu, 2020).
Agriculture contributed little to the expansion of the industrial or service sectors between 1978 and 1998. However, since 1989, its contribution to manufacturing sector growth has continuously expanded (Alemu et al., 2003). However, in recent years, Ethiopia’s agriculture sector has dominated the expansion of all other sectors as well as the national economy. Agriculture contributed significantly to the country’s GDP growth. This sector was responsible for more than half of the real GDP growth rate. The data suggested that there was a fundamental break in economic growth in 2004, followed by greater and more consistent growth (Dube et al., 2019). Ethiopian agriculture is distinguished by traditional farming practices, low production, and productivity (Yigezu Wendimu, 2021). These factors contribute to the decline in vegetation cover caused by increased farming on steep slopes, which degraded and depleted soils in the region, making soil degradation a widespread environmental issue. Inappropriate farming methods account for 28% of soil degradation (Mesene, 2017; Tadesse and Hailu, 2024). To address the aforementioned issues, the Ethiopian government is now launching a green legacy effort, assessing the potential of the country’s agroecologies and designing and implementing various measures to boost agricultural production and productivity.
Ethiopia has been experiencing both climate variability and change. Evidence indicates that the country’s mean annual temperature has risen by approximately 1.3°C since 1960, at an average pace of 0.28°C per decade, and that spatial and temporal rainfall variability has increased (Zegeye, 2018; Gemeda et al., 2021). Climate change has an impact on agricultural productivity and production by shortening maturity periods and decreasing crop yields, changing livestock feed availability, affecting animal health, growth and reproduction, lowering crop quality and quantity, changing distribution rates, and expanding desertification, among other things (Singh, 2019). The study examined scenarios of agricultural productivity change caused by climate change up to 2050. At the national level, the simulation results indicate that crop production will be significantly affected over the next four decades, with the severity increasing with time. By 2050, teff, maize, and sorghum production will be down by 25.4, 21.8, and 25.2 percent, respectively, compared to the baseline periods. Climate change will also result in a loss of 31.1% of agricultural GDP at factor cost by 2050. The country should begin mainstreaming adaption measures in order to maintain overall economic performance (Solomon et al., 2021). Thus, Ethiopian farmers use several coping measures, including afforestation, reforestation, crop rotation, mixed cropping, soil and water conservation, agroforestry practices, integrated soil fertility management, small-scale irrigation, the application of improved crop varieties, the use of improved livestock, mixed cropping, early and late planting, and the practice of income-generating activities (Megerssa and Bekere, 2019; Sinore and Wang, 2024).
Promoting climate-smart agricultural techniques, such as conservation agriculture, is critical in combating land degradation. Conservation agriculture is a farming technique that encourages minimal soil disturbance, the preservation of permanent soil cover, and plant species diversity. It encompasses practices such as zero tillage, crop rotation, and intercropping. Conservation agriculture improves crop yield by lowering temperatures, conserving soil moisture, boosting key soil nutrients in a sustainable manner, and raising net farm revenue (Zalalem, 2021; Gebirehiwot and Kibkab, 2022; Kolapo and Kolapo, 2023). Crop diversification and rotation, crop residue retention, and minimal soil disturbance are examples of conservation agricultural methods that can help reduce greenhouse gas emissions (Saha et al., 2022). Thus, it is regarded as a viable solution to climate change adaptation and mitigation in Ethiopia. Thus, the main objective of this review was to assess the potential of conservation agriculture for climate change adaptation and mitigation in Ethiopia.
We used a procedural method for our review, which included locating relevant literature and synthesizing peer-reviewed research and review articles, reports, conferences, and book chapters. The required materials were searched utilizing search engines such as Google, Google Scholar, Research Gate, and the most commonly used journal websites as primary data sources. More than 309 pdf files were downloaded, and relevant materials were checked and included in the review.
The search criteria includes material published in reputable publications recently (from 2001 to 2024), as well as studies undertaken on conservation agriculture in general, with a focus on Ethiopia. The search focused on keywords linked to the land degradation problem; land management methods, Climate smart agriculture (CSA) practices, and conservation agriculture’s potential for climate change mitigation and adaptation. The literature was searched using the following keywords: ‘Land degradation in Ethiopia’, ‘climate smart agriculture in Ethiopia’, ‘impact of conservation agriculture on soil organic carbon’, and ‘climate change adaptation in Ethiopia.
Following the literature search, the findings from various databases were reviewed using the criteria. The literature published prior to 2001 was deleted. Duplicated manuscripts were also excluded from the review. The inclusion and exclusion criteria were devised to meet the study’s aims, which were primarily concerned with the potential of conservation agriculture for climate change adaptation in Ethiopia. This study used study characteristics as well as report characteristics for inclusion and exclusion. Some examples of study characteristics employed in this study are inclusion and exclusion criteria relating to the study region, the outcome of the finding, and the context. Report characteristics include the year of publication and the type of report, which might be full-length research articles, meta-analyses, book chapters, or reports.
Some of the reported prevalent concerns include degradation of vegetation cover, loss of biodiversity, soil erosion, declining organic matter, reduced precipitation infiltration and water-holding capacity of the soil, loss of productivity, and impact on broader ecological processes (Zalalem, 2021). Conventional tillage has exacerbated the current global warming crisis, which is connected to greenhouse gas emissions. Furthermore, conventional tillage and agricultural residue burning could deteriorate the soil resource base and accelerate soil degradation, resulting in a reduction in crop production potential (Saha et al., 2022). Thus, to reduce the impact of climate change on smallholder and medium farmers, it is essential to identify and promote the influence of climate-smart agriculture on climate change adaptation and mitigation.
Conservation agriculture (CA) is a farming practice that promotes natural ecological processes in order to boost agricultural yields and sustainability by reducing soil disturbance, preserving permanent soil cover, and diversifying crop rotations (Milder et al., 2011). Minimum tillage assists to conserve soil moisture through enhancing infiltration and reducing runoff (Kassie et al., 2011; Petito et al., 2022). When compared to conventional tillage, conservation tillage retains crop residue, significantly lowers soil temperature, and enhances nutrient accumulation in the surface soil layer, resulting in increased crop growth and production. Thus, in Ethiopia, where soil moisture is a challenge, conservation agriculture can improve soil moisture status and enhance crop resilience to moisture stress (Abera et al., 2020). Conservation tillage reduces the soil disturbance and soil moisture evaporation. Crop residue application on the other hand helps to increase the soil moisture and soil nutrients that returned back from added crop residue (Lejissa et al., 2022).
Soil degradation due to soil erosion is a very serious problem of environmental damage. The process of soil degradation of the Ethiopia is very fast. The average annual rate of soil loss is estimated to be 12 tons/ha/yr., and it can extremely exceed this on steep slopes with soil loss rates greater than 300 tons/ha/year, where vegetation is removed (Gashaw, 2015; Djillo et al., 2024). Soil loss in cropland is very highly severe, which clearly shows that cropland should be prioritized to carry out land management practices such as minimum or zero tillage, intercropping, mulching, and planting crops or grasses along contour ridges, and use of manure/compost on to cropland land (Adugna et al., 2015). Conservation tillage decreases runoff and retains water in the soil after soil organisms loosen it by decomposing organic matter. It greatly reduces erosion, improves the soil structure and conserves organic matter in the soil (Hurni et al., 2016). According to Araya et al. (2011), plot under conservation tillage showed lower average soil losses and runoff as compared to others plot.
Conservation agriculture has been widely promoted as a method for smallholder farmers in Sub-Saharan Africa to boost yields while also making them more resilient to changing climate conditions (Michler et al., 2019). CA fosters climate resilience by improving agricultural systems’ ability to cope with climate-related shocks such as droughts, floods, and temperature extremes. By retaining soil cover and organic matter, CA helps crops withstand harsh weather conditions. To create resilient agricultural and food systems, good soil health, fertility, and water management are critical for determining food production quantity and quality. Soils are currently unable to produce to their full potential due to severe degradation, deforestation, crop residue trade-offs for livestock production, and rising temperatures. As a result, concerns about how to improve soil fertility and water retention capacity to increase production sustainably are advocated (Ogola, 2021).
The analysis of diverse meanings of important adaption terminology and ideas shows that definitions varied between institutions and stakeholder groups (Levina and Tirpak, 2006). Adaptation is an adjustment in natural or human systems in response to actual or anticipated climatic stimuli or their consequences that reduces harm or maximizes benefits. Several types of adaptation exist, including anticipatory and reactive adaptation, private and public adaptation, and autonomous and planned adaptation (IPCC, 2001).
Farmers use various adaptation strategies to adapt to adverse climate change based on their agro-ecologies, such as improved crop and livestock varieties, tree planting, soil conservation, livestock mobility, drought-tolerant species, crop diversification, planting date adjustment, small-scale irrigation, agronomic practice, livelihood diversification (value add and non-farm activities), and integrating livestock with crop production (Demem, 2023). The study’s findings show that the adoption of Climate Smart Agriculture (CSA) methods differs greatly between farmers and agro-ecology (Erekalo and Yadda, 2023).
Farmers use different adaption strategies such as better livestock management, fertilizer application, planting short-season crops, introducing new grass species, early sowing, and diversifying livelihoods (Feliciano et al., 2022). Climate smart agriculture based climate change adaptation strategies improve sustainable crop productivity by lowering temperatures, conserving soil moisture, and enhancing vital soil nutrients. Conservation agriculture boosts grain production by improving soil health and enhancing sustainable crop production by lowering temperatures, conserving soil moisture, and increasing key soil nutrients over time (Gebirehiwot and Kibkab, 2022).
According to the Food and Agricultural Organization (FAO), “Soil management is sustainable if the supporting, provisioning, regulating, and cultural services provided by soil are maintained or enhanced without significantly impairing either the soil functions that enable those services or biodiversity” (FAO, 2017). Agriculture and different land use contribute considerably to greenhouse gas emissions. Mbow et al. (2019) estimate that crop and animal activities within the farm gate provide 9–14% and land use changes contribute 5–14%, respectively. Tillage disturbance is a major element that lowers carbon stabilization in fine-textured soils and promotes soil organic carbon breakdown. However, conservation agriculture strategies can improve soil organic carbon (SOC) content and agro-ecosystem sustainability by retaining residue in coarse-textured soils and reducing SOC decomposition in fine-textured soils through reduced tillage (Chivenge et al., 2007). Furthermore, Jat et al. (2019) found that conservation agriculture captured more carbon in surface soil layers than conventional farming practices. That promotes the sequestration of carbon dioxide in the soil, thus contributing to climate change efforts. Conservation agricultural practices had a positive impact on SOC, soil aggregation, and C in aggregates in a soybean and maize cropping system (Fonteyne et al., 2021). A study conducted in southern Ethiopia found a significant difference (p < 0.05) in soil organic carbon (SOC) between conservation agriculture (1.8 + 0.07) and conventional tillage (1.6 + 0.05) (Lejissa et al., 2022). Liben et al. (2018) found a similar result: soil organic C for the 0–0.05 m soil profile was 16 g kg-1 with conservation agriculture versus 12 g kg-1 with conventional farming. Furthermore, Jayaraman et al. (2022) found that no till and reduced tillage had a substantial impact on soil organic carbon (12.9 to 19.4% increase) and soil aggregate (20.77 to 25.97% increase) when compared to conventional tillage. This demonstrates that conservation agriculture makes an important contribution to climate change mitigation and adaptation.
Conservation agriculture enhances soil health and the efficient use of natural resources, reducing the environmental consequences of agricultural activities, saving inputs, and lowering production costs and increase farmers annual income when compared to traditional practice (Cárceles Rodríguez et al., 2022; Gebeyehu, 2023). The study result revealed that soil health indicators such as soil aggregation, soil organic carbon storage, soil enzymes, and microbial biomass indicate soil health improvement under conservation tillage practices, which could enhance the carbon-nitrogen cycle, soil stability, and overall crop productivity. When the tillage practice integrates with crop rotation and residue management, it can offer long-term sustainability for soil management (Hou, 2023; Sangotayo et al., 2023). The conservation tillage method reduced the soil temperature, soil nutrients accumulation, crop growth, and yield. Furthermore, conservation tillage is the most effective way to improve soil health as compared to conventional tillage systems (Sadiq et al., 2021).
Different stakeholders have defined climate change mitigation in several ways: The Intergovernmental Panel on Climate Change (IPCC) defines ‘Implementing policies to reduce greenhouse gas emissions and enhance sinks’ as implementing policies (IPCC, 2007). While the United Nations Framework Convention on Climate Change (UNFCCC) defines “A human intervention to reduce the sources or enhance the sinks of greenhouse gases’ (UNFCCC, 1997). Conservation agriculture (CA) is a farming method that uses natural ecological processes to increase agricultural yields and sustainability by reducing soil disturbance, retaining permanent soil cover, and diversifying crop rotations (Milder et al., 2011; Kolapo and Kolapo, 2023). Thus, CA is among non-substitutable options to reduce greenhouse gas emission (Zalalem, 2021). Crop diversification, improved nitrogen use efficiency, crop rotation, improved soil carbon sequestration strategies; crop residue retention, reduced soil disturbance, and integrated farming systems are all essential contributors to lowering GHG emissions. Furthermore, conservation agriculture minimizes the adverse impacts associated with conventional agriculture, such as soil erosion, soil organic matter decline, water loss, and soil physical deterioration, while increasing biodiversity (Saha et al., 2022).
Carbon stocks in soils can be large, and they can fluctuate in response to soil properties and management activities such as crop type and rotation, tillage, drainage, residue management, and organic additions. Crop residue combustion emits considerable non-CO2 greenhouse gases (IPCC, 2007). In Ethiopia, the majority of farmers are smallholders who use mitigating strategies such as reduced tillage and manure incorporation into their soils. Soil organic matter storage can help retain carbon, improve water-holding capacity in dry conditions, and increase agricultural productivity (Feliciano et al., 2022). Conservation agriculture is based on three pillars: limited tillage, permanent ground cover, and crop diversity or rotation, all of which improve soil aggregation, water infiltration, and retention while also increasing soil organic carbon in the soil’s surface layers. This reduces excessive CO2 accumulation in the atmosphere (Jat et al., 2014; Stanojevic and Stanojevic, 2021).
Conservation agriculture has been practiced for a long time, but the promotion of the technique requires diverse strategies. Farmers and stakeholders should understand the benefits and long-term implications of CA, which is concerned with sustainability. This might be accomplished by actively engaging stakeholders at all phases and providing training on the impact of CA on soil health and sustainability (Lumpkin and Sayre, 2009; Jat et al., 2014).
To promote CA adoption, the government, and NGOs should provide subsidies for purchasing zero-till machinery and making credit available to participant farmers, protect participant rights, facilitate interlinks for farmers with input suppliers, research institutes, farmer self-groups, organize farmer visits and workshops, provide information on input supply, credit lines, and provide farmers with access to new technological advancements (Jat et al., 2014; Cárceles Rodríguez et al., 2022).
Weed control strategy remains a major barrier to successful CA adoption, demanding the development of an economic and effective weed control mechanism based on integrated weed management for site-specific applications (Jat et al., 2014; Hurni et al., 2016). Several factors and challenges impede the adoption of CA practices, including a lack of appropriate equipment and machinery, the use of crop residue for fuel wood and animal feed, a lack of awareness about the benefits of CA on soil health and sustainability, and a lack of technical and financial support from the government for smallholder and medium farmers (Cárceles Rodríguez et al., 2022).
CA practices help to mitigate and adapt to climate change, alleviate poverty, and conserve ecosystems, all of which require innovative methods. To do so, adequate time is required, as the CA’s complicated, knowledge-intensive nature and the requirement to establish local ability may take 3–5 years to build farmer trust (Milder et al., 2011). According to reports, the majority of CA activities were carried out at the plot level (Figure 1). Thus, they should be scaled up to the landscape level by integrating with the promotion of improved livestock feed and grazing systems, as well as improved fuel wood promotion, to reduce pressure or challenges associated with crop residue utilization for animal feed and fuel wood consumption. Strengthening research and promotion of the technology is essential through conducting experimentation, promoting the best practices, and capacity building for farmers and experts to improve adoption barriers and realize its full potential in enhancing agricultural productivity, resilience, and sustainability.
Figure 1. Conservation agriculture demonstration plot in the Nedi Gibe Kabala Watershed, Southwestern Ethiopia. Source: Own field photo shot during sowing.
Conservation agriculture (CA), with its three core concepts of minimum soil disturbance, permanent soil cover, and crop rotation, could be a feasible option for fulfilling the SDG targets. CA is aligned with the United Nations’ Sustainable Development Goals (SDGs) and contributes to their achievement, particularly SDGs 2: Zero Hunger, 6: Clean Water and Sanitation, 12: Responsible Consumption and Production, 13: Climate Action, and 15: Life on Land. SDG 2 aims to eradicate hunger by promoting sustainable agriculture and nutrition. CA techniques improve soil health and nutrient availability, boosting agricultural output while minimizing dependency on synthetic inputs such as pesticides and fertilizers. CA’s adaptability to climate change issues enhances food production. For SDG 6, CA’s limited tillage and soil cover components help reduce soil erosion, leading in cleaner water by avoiding sedimentation in water bodies. Improved water management and conservation help to ensure sustainable water use and sanitation. SDG 12 promotes appropriate consumption and production habits. CA encourages effective resource use, lowers waste, and mitigates the negative environmental impact of agricultural inputs, resulting in more sustainable production systems that rely less on external inputs. SDG 13 states that CA techniques improve soil and water management, boost soil carbon absorption, and reduce greenhouse gas emissions, all of which help agricultural systems become more resilient to climate change. Finally, SDG 15 focuses on life and land protection. CA promotes biodiversity through cover cropping and reduced chemical use, which offers habitat for creatures while also supporting ecosystem services and sustainable land use. Finally, CA contributes significantly to the achievement of numerous SDGs by promoting sustainable agriculture, saving resources, reducing climate change, and protecting biodiversity. Adoption and extension of CA practices are critical for ensuring a sustainable and resilient future (Chaudhari et al., 2021; Sachs et al., 2022; Farooq, 2023; Atapattu et al., 2024).
The reviewed analysis of several research findings demonstrated that minimum tillage helps to conserve soil moisture as compared to conventional tillage. Conservation tillage reduces soil temperature, retains crop residue, and improves nutrient buildup in the surface soil layer, all of which lead to improved growth and yield of crops. Furthermore, agriculture and other land uses significantly contribute to greenhouse gas emissions; yet, conservation agriculture methods have a favorable impact on SOC, soil aggregation, and carbon in aggregate, improving soil health. The study’s findings revealed that soil health indicators such as soil aggregation, soil organic carbon storage, soil enzymes, and microbial biomass improve with conservation tillage practices, which could enhance the carbon-nitrogen cycle, soil stability, and overall crop productivity. While considering climate adoption and mitigation alternatives, Conservation agriculture is one of the non-substitutable methods of reducing greenhouse gas emissions. Crop diversity, increased nitrogen consumption efficiency, crop rotation, improved soil carbon sequestration methods; crop residue retention, minimum soil disturbance, manure incorporation, and integrated farming systems are all important factors in minimizing GHG emissions. Moreover, factors limiting conservation agriculture adoption were identified as a lack of appropriate equipment and machinery, weed control methods, the use of crop residue for fuel wood and animal feed, a lack of awareness about the benefits of conservation agriculture on soil health and sustainability, and a lack of government technical and financial support for smallholder. Adoption and scaling up of CA practices are critical for ensuring a sustainable development goal and resilient future. Thus, interested stakeholders should consider the aforementioned considerations while integrating with enhanced technology to promote technology on a large scale. According to the review results, it was recommended to support the technology with awareness creation, integration with enhanced technology, and supporting the investigation’s results of the site-specific agroecology of the area.
LH: Conceptualization, Methodology, Resources, Writing – original draft, Writing – review & editing. WT: Writing – original draft, Writing – review & editing.
The author(s) declare that no financial support was received for the research, authorship, and/or publication of this article.
The authors declare that the research was conducted in the absence of any commercial or financial relationships that could be construed as a potential conflict of interest.
All claims expressed in this article are solely those of the authors and do not necessarily represent those of their affiliated organizations, or those of the publisher, the editors and the reviewers. Any product that may be evaluated in this article, or claim that may be made by its manufacturer, is not guaranteed or endorsed by the publisher.
The Supplementary material for this article can be found online at: https://www.frontiersin.org/articles/10.3389/fclim.2024.1478923/full#supplementary-material
Abera, D., Beshir, B., and Liben, F. M. (2020). The role of conservation agriculture for soil quality improvement: a review. Ethiopian J. Agricul. Sci. 30, 197–222.
Adugna, A., Abegaz, A., and Cerdà, A. J. S. E. D. (2015). Soil erosion assessment and control in northeast Wollega. Ethiopia. Solid Earth Discuss. 7, 3511–3540. doi: 10.5194/sed-7-3511-2015
Alemu, Z. G., Oosthuizen, K., and Van Schalkwyk, H. D. (2003). Contribution of agriculture in the Ethiopian economy: a time-varying parameter approach. Agrekon 42, 29–48. doi: 10.1080/03031853.2003.9523608
Araya, T., Cornelis, W. M., Nyssen, J., Govaerts, B., Bauer, H., Gebreegziabher, T., et al. (2011). Effects of conservation agriculture on runoff, soil loss and crop yield under rainfed conditions in Tigray, Northern Ethiopia. Soil Use Manag. 27, 404–414. doi: 10.1111/j.1475-2743.2011.00347.x
Atapattu, A. J., Ranasinghe, C. S., Nuwarapaksha, T. D., Udumann, S. S., and Dissanayaka, N. S. (2024). “Sustainable agriculture and sustainable development goals (SDGs)” in Emerging technologies and marketing strategies for sustainable agriculture (IGI Global), 1–27. doi: 10.4018/979-8-3693-4864-2.ch001
Cárceles Rodríguez, B., Durán-Zuazo, V. H., Soriano Rodríguez, M., García-Tejero, I. F., Gálvez Ruiz, B., and Cuadros Tavira, S. (2022). Conservation agriculture as a sustainable system for soil health: a review. Soil Systems 6:87. doi: 10.3390/soilsystems6040087
Chaudhari, S. K., Jat, M. L., Chakraborty, D., Biswas, A. K., Maity, P. P., and Mohapatra, T. (2021). Conservation agriculture and sustainable development goals: Potential opportunities and strategies in India. J. Agric. Sci., 21, 1–9.
Chivenge, P. P., Murwira, H. K., Giller, K. E., Mapfumo, P., and Six, J. (2007). Long-term impact of reduced tillage and residue management on soil carbon stabilization: implications for conservation agriculture on contrasting soils. Soil Tillage Res. 94, 328–337. doi: 10.1016/j.still.2006.08.006
Demem, M. S. (2023). Impact and adaptation of climate variability and change on small-holders and agriculture in Ethiopia: A review, vol. 9: Heliyon, e18972.
Djillo, S. C., Wolka, K., and Tofu, D. A. (2024). Assessing soil erosion and farmers’ decision of reducing erosion for sustainable soil and water conservation in Burji woreda, southern Ethiopia. Sci. Rep. 14:8638. doi: 10.1038/s41598-024-59076-6
Dube, A. K., Fawole, W. O., Govindasamy, R., and Özkan, B. (2019). Agricultural development led industrialization in Ethiopia: structural break analysis. Int. J. Agricul. Forest. Life Sci. 3, 193–201.
Erekalo, K. T., and Yadda, T. A. (2023). Climate-smart agriculture in Ethiopia: adoption of multiple crop production practices as a sustainable adaptation and mitigation strategies. World Dev. Sustain. 3:100099. doi: 10.1016/j.wds.2023.100099
FAO, (2017). “Voluntary guidelines for sustainable soil management. FAO, Available at: http://www2.unccd.int/convention/conference-parties-cop/unccdcop13-6-16-september-2017-ordos-china
Farooq, M. (2023). Conservation agriculture and sustainable development goals. Pak. J. Agric. Sci. 60, 291–298. doi: 10.21162/PAKJAS/23.170
Feliciano, D., Recha, J., Ambaw, G., MacSween, K., Solomon, D., and Wollenberg, E. (2022). Assessment of agricultural emissions, climate change mitigation and adaptation practices in Ethiopia. Clim. Pol. 22, 427–444. doi: 10.1080/14693062.2022.2028597
Fonteyne, S., Burgueño, J., Albarran Contreras, B. A., Andrio Enriquez, E., Castillo Villasenor, L., Enyanche Velazquez, F., et al. (2021). Effects of conservation agriculture on physicochemical soil health in 20 maize-based trials in different agro-ecological regions across Mexico. Land Degrad. Dev. 32, 2242–2256. doi: 10.1002/ldr.3894
Gashaw, T. (2015). Soil erosion in Ethiopia: extent, conservation efforts and issues of sustainability. Palgo J Agric 2, 38–48. doi: 10.4236/oalib.1101481
Gebeyehu, T. A. (2023). Adoption and impacts of conservation agriculture on smallholder farmers’ livelihoods in the case of Arba Minch Zuria district of southern Ethiopia. Cogent Soci. Sci. 9:2235782. doi: 10.1080/23311886.2023.2235782
Gebirehiwot, H. T., and Kibkab, E. (2022). Nature based climate change adaptation measures for sustainable crops production in Ethiopia: review. Arch. Crop Sci. 5, 141–150. doi: 10.36959/718/614
Gebreselassie, S., Kirui, O. K., and Mirzabaev, A. (2016). Economics of land degradation and improvement in Ethiopia. Econ. Land Degrad. Improv., 401–430. doi: 10.1007/978-3-319-19168-3_14
Gedamu, M. T. (2020). Soil degradation and its management options in Ethiopia: a review. Int. J. Res. Innov. Earth Sci 7, 59–76.
Gemeda, D. O., Korecha, D., and Garedew, W. (2021). Evidences of climate change presences in the wettest parts of Southwest Ethiopia. Heliyon 7:e08009. doi: 10.1016/j.heliyon.2021.e08009
Grinand, C., Bégué, A., Montfort, F., Leroux, L., and Randrianarivo, T. (2019). An analysis of land use changes and land degradation in Mozambique. Maputo: NITIDAE & CIRAD.
Hou, D. (2023). Sustainable soil management for food security. Soil Use Manag. 39, 1–7. doi: 10.1111/sum.12883
Hurni, H., Abate, S., Bantider, A., Debele, B., Ludi, E., Portner, B., et al. (2010). Land degradation and sustainable land management in the highlands of Ethiopia., global change and sustainable development. 187–207. doi: 10.13140/2.1.3976.5449
Hurni, H., Berhe, W. A., Chadhokar, P., Daniel, D., Gete, Z., Grunder, M., et al. (2016). Soil and water conservation in Ethiopia: Guidelines for development agents. 2nd Edn: Bern, Switzerland, Centre for Development and Environment, University of Bern, with Bern Open Publishing, 134.
IPCC (2001) in Climate change 2001: Impacts, adaptation and vulnerability, contribution of the working group II to the third assessment report of the intergovernmental panel on climate change. eds. J. J. McCarthy, O. F. Canziani, N. A. Leary, D. J. Dokken, and K. S. White (Cambridge, UK: Cambridge University Press), 1032.
IPCC (2007). “Climate change 2007: the scientific basis” in Contribution of working group I to the fourth assessment report of the intergovernmental panel on climate change. eds. S. Solomon, et al. (New York: Cambridge University Press).
Jat, H. S., Datta, A., Choudhary, M., Sharma, P. C., Yadav, A. K., Choudhary, V., et al. (2019). Climate smart agriculture practices improve soil organic carbon pools, biological properties and crop productivity in cereal-based systems of north-West India. Catena 181:104059. doi: 10.1016/j.catena.2019.05.005
Jat, R. A., Sahrawat, K. L., Kassam, A. H., and Friedrich, T. (2014). Conservation agriculture for sustainable and resilient agriculture: Global status, prospects and challenges. eds. R. A. Jat, K. L. Sahrawat, and A. H. Kassam. Conserv. Agricul. 1–25. doi: 10.1079/9781780642598.0001
Jayaraman, S., Sahu, M., Sinha, N. K., Mohanty, M., Chaudhary, R. S., Yadav, B., et al. (2022). Conservation agricultural practices impact on soil organic carbon, Soil Aggregation and Greenhouse Gas Emission in a Vertisol. Agriculture 2022:1004. doi: 10.3390/agriculture12071004
Jia, G., Shevliakova, E., Artaxo, P., De Noblet-Ducoudré, N., Houghton, R., House, J., et al. (2019). Land–climate interactions. In: Climate Change and Land: an IPCC special report on climate change, desertification, land degradation, sustainable land management, food security, and greenhouse gas fluxes in terrestrial ecosystems. eds. P. R. Shukla, J. Skea, E. Calvo Buendia, V. MassonDelmotte, H.-O. Pörtner, and D. C. Roberts, et al. doi: 10.1017/9781009157988.004
Kassie, M., Shiferaw, B., and Muricho, G. (2011). Agricultural technology, crop income, and poverty alleviation in Uganda. World Dev. 39, 1784–1795. doi: 10.1016/j.worlddev.2011.04.023
Kolapo, A., and Kolapo, A. J. (2023). Implementation of conservation agricultural practices as an effective response to mitigate climate change impact and boost crop productivity in Nigeria. J. Agricult. Food Res. 12:100557. doi: 10.1016/j.jafr.2023.100557
Lejissa, L. T., Wakjira, F. S., and Tanga, A. A. (2022). Effects of conservation agriculture and conventional tillage on the soil physicochemical properties and household income in southern Ethiopia. Int. J. Agronom. 2022:1224193. doi: 10.1155/2022/1224193
Levina, Ellina, and Tirpak, Dennis,(2006). Adaptation to climate change: key terms, OECD and IEA information papers for the Annex I Expert Group on the UNFCCC can be Available at: http://www.oecd.org/env/cc/.
Liben, F. M., Tadesse, B., Tola, Y. T., Wortmann, C. S., Kim, H. K., and Mupangwa, W. (2018). Conservation agriculture effects on crop productivity and soil properties in Ethiopia. Agron. J. 110, 758–767. doi: 10.2134/agronj2017.07.0384
Lumpkin, T. A., and Sayre, K. (2009). Enhancing resource productivity and efficiency through conservation agriculture. Plenary Session 3. (pp. 3–9), New Delhi, India.
Mbow, C., Rosenzweig, C., Barioni, L.G., Benton, T.G., Herrero, M., Krishnapillai, M., et al., (2019): Food Security. In: Climate Change and Land: an IPCC special report on climate change, desertification, land degradation, sustainable land management, food security, and greenhouse gas fluxes in terrestrial ecosystems. eds. P. R. Shukla, J. Skea, E. Calvo Buendia, V. MassonDelmotte, H.-O. Pörtner, and D. C. Roberts, et al. doi: 10.1017/9781009157988.007
Megerssa, G. R., and Bekere, Y. B. (2019). Causes, consequences and coping strategies of land degradation: evidence from Ethiopia. J. Degrade. Min. Land Manag. 7, 1953–1957. doi: 10.15243/jdmlm.2019.071.1953
Mesene, M. (2017). Extent and impact of land degradation and rehabilitation strategies: Ethiopian highlands. J. Environ. Earth Sci. 7, 22–32.
Michler, J. D., Baylis, K., Arends-Kuenning, M., and Mazvimavi, K. (2019). Conservation agriculture and climate resilience. J. Environ. Econ. Manag. 93, 148–169. doi: 10.1016/j.jeem.2018.11.008
Milder, J. C., Majanen, T., and Scherr, S. J. (2011). Performance and potential of conservation agriculture for climate change adaptation and mitigation in sub-Saharan Africa. An assessment of WWF and CARE projects in support of the WWF-CARE Alliance’s Rural Futures Initiative, Final Report February 2011. http://www.ecoport.org/
Muke, M. (2019). Reported driving factors of land-use/cover changes and its mounting consequences in Ethiopia: a review. Afr. J. Environ. Sci. Technol. 13, 273–280. doi: 10.5897/AJEST2019.2680
Nkonya, E., Mirzabaev, A., and von Braun, J. (2016). Economics of land degradation and improvement: an introduction and overview. Econ. Land Degrad. Improv., 1–14. doi: 10.1007/978-3-319-19168-3_1
Ogola, J. (2021). Climate resilient agriculture and food Systems in Ethiopia. Wageningen Centre for Development Innovation. Wageningen: Wageningen University & Research.
Olsson, L., Barbosa, H., Bhadwal, S., Cowie, A., Delusca, K., Flores-Renteria, D., et al., (2019). Land degradation. In: Climate change and land: An IPCC special report on climate change, desertification, land degradation, sustainable land management, food security, and greenhouse gas fluxes in terrestrial ecosystems. Lund University Centre for Sustainability Studies, 184 p.
Petito, M., Cantalamessa, S., Pagnani, G., Degiorgio, F., Parisse, B., and Pisante, M. (2022). Impact of conservation agriculture on soil erosion in the annual cropland of the Apulia region (southern Italy) based on the RUSLE-GIS-GEE framework. Agronomy 12:281. doi: 10.3390/agronomy12020281
Sachs, J., Kroll, C., Lafortune, G., Fuller, G., and Woelm, F. (2022). Sustainable development report 2022 : Cambridge University Press doi: 10.1017/9781009210058
Sadiq, M., Li, G., Rahim, N., and Tahir, M. M. (2021). (2021), sustainable conservation tillage technique for improving soil health by enhancing soil physicochemical quality indicators under wheat mono-cropping system conditions. Sustain. For. 13:8177. doi: 10.3390/su13158177
Saha, R., Barman, D., Behera, M., and Kar, G. (2022). Conservation agriculture and climate change: Impacts and adaptations. eds. R. Saha, D. Barman, M. Behera, and G. Kar. London: CRC Press. 496 pages.
Sangotayo, A. O., Chellappa, J., Sekaran, U., Bansal, S., Angmo, P., Jasa, P., et al. (2023). Long-term conservation and conventional tillage systems impact physical and biochemical soil health indicators in a corn–soybean rotation. Soil Sci. Soc. Am. J. 87, 1056–1071. doi: 10.1002/saj2.20563
Shumete, Y. (2015) Federal Democratic Republic of Ethiopia Ethiopia - Land Degradation Neutrality National Report, United Nations Convention to Combat Desertification. France. Retrieved from https://coilink.org/20.500.12592/4njvkg
Singh, S. N. (2019). Climate change and agriculture in Ethiopia: A case study of Mettu Woreda 3, 61–79. doi: 10.21272/sec.3(3).61-79.2019
Sinore, T., and Wang, F. (2024). Impact of climate change on agriculture and adaptation strategies in Ethiopia: A meta-analysis, vol. 10: Heliyon, e26103.
Solomon, R., Simane, B., and Zaitchik, B. F. (2021). The impact of climate change on agriculture production in Ethiopia: application of a dynamic computable general equilibrium model. Am. J. Clim. Chang. 10, 32–50. doi: 10.4236/ajcc.2021.101003
Stanojevic, A. B., and Stanojevic, A. B. (2021). Conservation agriculture and its principles. Annals Environ. Sci. Toxicol. 5, 018–022.
Tadesse, A., and Hailu, W. (2024). Causes and consequences of land degradation in Ethiopia: a review. Int. J. 10, 10–21. doi: 10.11648/j.ijsqa.20241001.12
Temesgen, G., Amare, B., and Silassie, H. G. (2014). Land degradation in Ethiopia: causes, impacts and rehabilitation techniques. J. Environ. Earth Sci. 4, 98–104.
Tully, K., Sullivan, C., Weil, R., and Sanchez, P. (2015). The state of soil degradation in sub-Saharan Africa: baselines, trajectories, and solutions. Sustain. For. 7, 6523–6552. doi: 10.3390/su7066523
UNFCCC. (1997). UN Climate Change Conference Kyoto Climate Change Conference - December 1997 Third Session of the Conference of the Parties (COP3). Available at: https://unfccc.int/event/kyoto-climate-change-conference-december-1997
Yigezu Wendimu, G. (2021). The challenges and prospects of Ethiopian agriculture. Cogent Food Agricul. 7:1923619. doi: 10.1080/23311932.2021.1923619
Zalalem, A. (2021). Mitigation of climate change through conservation agriculture. J. Natural Sci. 12. doi: 10.7176/JNSR/12-1-03
Keywords: crop residue, conservation tillage, conventional tillage, greenhouse gas, soil health
Citation: Hailu L and Teka W (2024) Potential of conservation agriculture practice in climate change adaptation and mitigation in Ethiopia: a review. Front. Clim. 6:1478923. doi: 10.3389/fclim.2024.1478923
Received: 11 August 2024; Accepted: 23 October 2024;
Published: 05 November 2024.
Edited by:
Benedicta Essel Ayamba, Council for Scientific and Industrial Research, GhanaReviewed by:
John Malcolm Gowdy, Rensselaer Polytechnic Institute, United StatesCopyright © 2024 Hailu and Teka. This is an open-access article distributed under the terms of the Creative Commons Attribution License (CC BY). The use, distribution or reproduction in other forums is permitted, provided the original author(s) and the copyright owner(s) are credited and that the original publication in this journal is cited, in accordance with accepted academic practice. No use, distribution or reproduction is permitted which does not comply with these terms.
*Correspondence: Leta Hailu, bGF0YWhhaWx1QGdtYWlsLmNvbQ==
Disclaimer: All claims expressed in this article are solely those of the authors and do not necessarily represent those of their affiliated organizations, or those of the publisher, the editors and the reviewers. Any product that may be evaluated in this article or claim that may be made by its manufacturer is not guaranteed or endorsed by the publisher.
Research integrity at Frontiers
Learn more about the work of our research integrity team to safeguard the quality of each article we publish.