- Department of Biology, University of Prince Edward Island, Charlottetown, PE, Canada
The number of reports highlighting the services provided by seagrass beds continues to be matched by those reporting on local seagrass declines across the world coastlines. Among the many factors driving the fate of seagrass beds, this Mini Review focuses on the interactions between seagrasses and algae, more specifically, macroalgae. Seagrasses are known to respond to sudden increases in co-occurring macroalgae, and the ongoing warming of ocean waters suggests that these interactions are most likely to grow in frequency and possibly in intensity in the decades ahead. What remains unclear is the nature (positive, neutral, negative) and the local outcome of those interactions. We examined the published evidence on explicit seagrass-algal interactions and found that in most cases these interactions have been negative, with seagrass species most often found at a competitive disadvantage with regards to macroalgae. Rising ocean temperatures are likely to add to this imbalance as at least some studies already suggest that the negative effects of macroalgae and warming are either additive or synergistic. The further examination of these effects will help predict likely future scenarios and aid in the prioritization of conservations efforts.
1 Introduction
Seagrass beds are among the most efficient carbon sinks (Duarte et al., 2010; Gattuso et al., 2018), are highly productive (Koopmans et al., 2020) and, as foundation species, influence the structure, and function of coastal communities and ecosystems. Seagrass beds also provide a variety of services including stabilization of the seabed, nutrient transfer, buffering of the negative effects of eutrophication, water oxygenation through photosynthetic activity, and habitat for the settlement and nursery of multiple invertebrates and fish (Costanza et al., 1997; Spalding et al., 2007; Heck et al., 2008; Chung et al., 2011; Smale et al., 2013; Thomson et al., 2015; Teagle et al., 2017; Surugiu et al., 2021). Unfortunately, most of these critical ecosystem services are threatened by the worldwide decline in seagrass meadows resulting from the warming of the oceans, among a series of other climate events (e.g., Orth et al., 2006; Waycott et al., 2009; Valdez et al., 2020). Ocean warming is known to cause species' ranges and distribution patterns to shift (Breeman, 1988; Parmesan and Yohe, 2003), with plant and seaweed local communities becoming reorganized due to the addition and loss of species (Wootton et al., 2008; Harley, 2011). When not in excess, macroalgae coexist with seagrass meadows and provide support to community and ecosystem secondary productivity. For instance, drift algae in the Northern Gulf of Mexico have been found to positively influence seagrass-related nekton recruitment and biomass (Correia and Smee, 2022; Correia et al., 2022b). However, the recent IPCC Special Report on the Ocean and Cryosphere in Changing Climate (Bindoff et al., 2019) reported a widespread increase in coastal stress levels, noting that since 1980, shifts in seaweeds and harmful algal blooms, that often interact negatively with seagrass meadows, have also increased in range and frequency. Rather than subside and normalize, this temporal pattern is more likely to intensify in the future.
Competitive success among primary producers is heavily dependent on stress sources and availability of resources (Harley et al., 2012), including among others light availability and attenuation (see Minguito-Frutos et al., 2023). The behavior and physiology of seagrasses is often dictated by their ability to photosynthesize and grow in the presence of algal shading (Brun et al., 2003), their efficiency during nutrient uptake (Alexandre et al., 2021), their ability to avoid harmful effects mediated by changes in the sediment (e.g., pH; Mvungi et al., 2012), and their response to indirect interactions with epiphytes (e.g., Irlandi et al., 2004). It follows that drastic changes in local algae and algal beds are likely to alter the fitness of seagrasses, in the same way that has been repeatedly demonstrated for algal-coral interactions (e.g., Carpenter et al., 2008; Anton et al., 2020). In a warming ocean, the outcome of seagrass-algal interactions is equally important, yet the mechanisms mediating their dynamics have not gathered the same attention. This article aimed to explore mechanisms and local outcomes from the examination of the literature available on seagrass-macroalgal interactions. Assuming such interactions continue to increase in frequency and possibly in severity, as most forecasts suggest, their examination may help predict likely future scenarios and aid on the prioritization of conservations efforts (Nordlund et al., 2018; He and Silliman, 2019).
This Mini review examined 46 peer-reviewed articles, deemed representative from geographic regions where seagrass and macroalgal beds coexist and their interactions have been documented. Some coastal areas are known to support extensive seagrass meadows and macroalgae (e.g., sites in Kenya and other coastal areas in Africa; e.g., Coppejans et al., 1992), but as this overview suggests, they are still affected by a geographic gap in the literature (see Figure 1). The articles summarized in Table 1, with their key features and outcomes, were found using relevant keywords such as “seagrass,” “macroalgae,” “seaweed,” “seagrass interactions',” and “algal-seagrass interactions,” among others, from databases, networks, and search engines available to us (e.g., OneSearch, Google Scholar, ResearchGate), and through examination of cross-references within multiple articles. Although we loosely use the term alga or algae in Table 1, we are in all cases referring to any type of marine macroscopic algae (macroalgae) or seaweed, excluding any forms of microalgae that may be associated with, for instance, blooms. The same applies to the paragraphs below detailing and discussing the results of the brief overview.
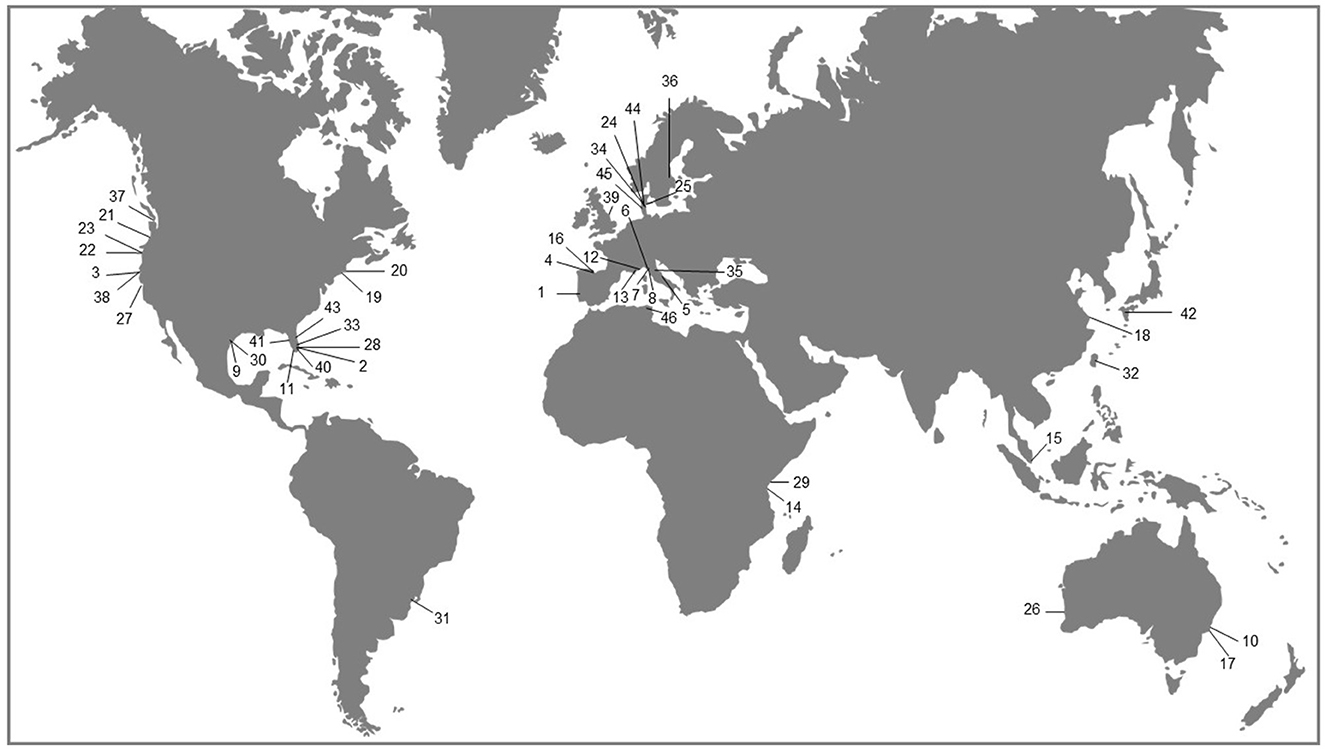
Figure 1. Map outlining the geographic distribution of the 46 studies used in the Mini review and summarized in Table 1. The numbers correspond to those cited at the bottom of Table 1.
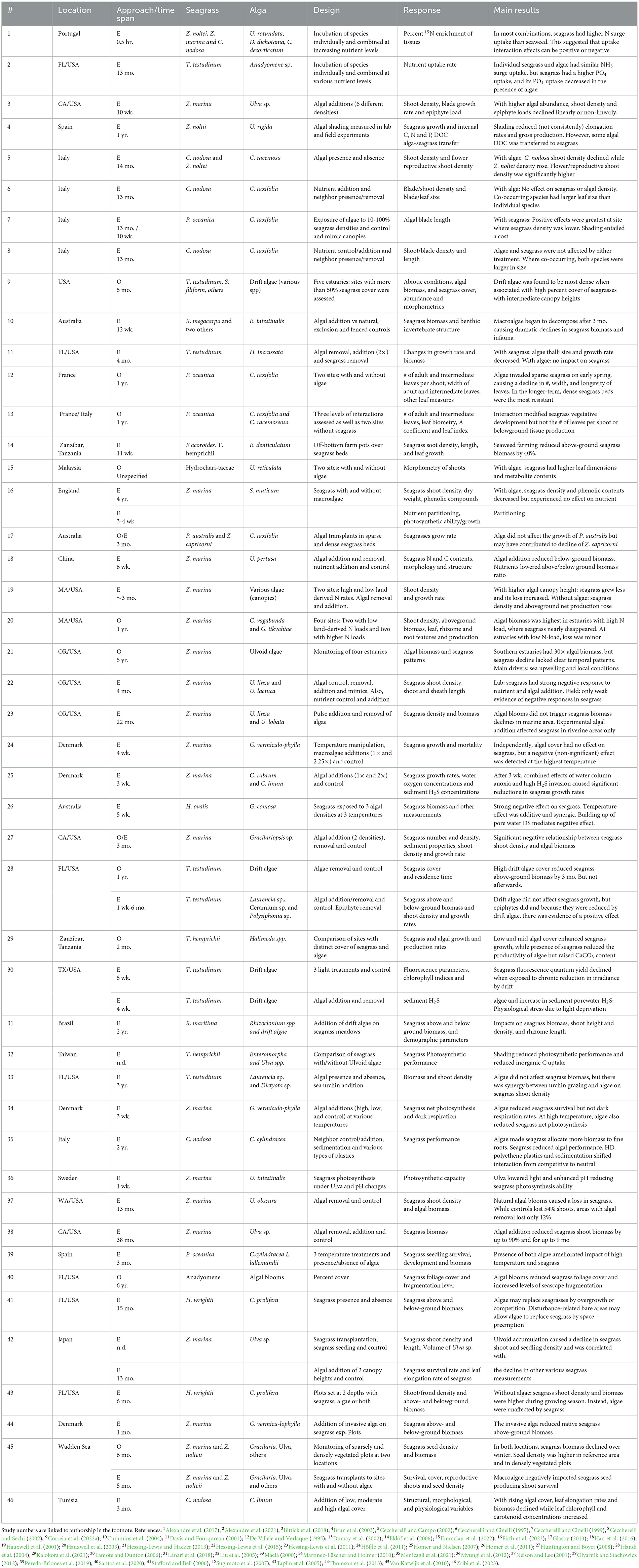
Table 1. A compilation of studies assessing the interactions between seagrasses and macroalgae, identifying studies with numbers (#), and providing details on location, approach [observational (O) or experimental (E)], time span, species, main design features, main responses measured, and main results reported.
2 The overwhelming frequency of negative interactions
Nearly 80% of the studies examining interactions between seagrasses and macroalgae have found evidence of negative interactions, with a clear imbalance against seagrasses: most of these studies found that macroalgae had direct or indirect negative effects on seagrasses (e.g., Ceccherelli and Cinelli, 1997; Ceccherelli and Campo, 2002; Alexandre et al., 2017, 2021; Bittick et al., 2018; among several others). Nonetheless, a few studies have also found negative impacts of seagrasses on macroalgae (Davis and Fourqurean, 2001; Alexandre et al., 2017; Menicagli et al., 2021). Harmful effects on seagrasses were often associated with losses on a competition for light (De Villele and Verlaque, 1995; Dumay et al., 2002; Irlandi et al., 2004; Hessing-Lewis et al., 2011; Zribi et al., 2023) or through light reduction via shading (Hauxwell et al., 2001, 2003; Liu et al., 2005; Eklöf et al., 2006; Lamote and Dunton, 2006; Huntington and Boyer, 2008; Van Katwijk et al., 2010; Homer et al., 2011; Mvungi et al., 2012; Hessing-Lewis et al., 2015; Han et al., 2016; Correia et al., 2022a). Shading by macroalgae has also been associated with the creation of unfavorable biogeochemical conditions (Hauxwell et al., 2001; Van Katwijk et al., 2010; Mvungi et al., 2012; Hessing-Lewis et al., 2015). As a result, seagrass under chronically reduced light conditions have experienced a decline in biomass (Eklöf et al., 2006; Huntington and Boyer, 2008; Hessing-Lewis et al., 2011, 2015; Han et al., 2016), shoot density (Hauxwell et al., 2003; Huntington and Boyer, 2008; Hessing-Lewis et al., 2011, 2015), canopy height (Correia et al., 2022a), leaf elongation rates (Zribi et al., 2023), metabolic efficiency (Irlandi et al., 2004), photosynthetic efficiency (De Villele and Verlaque, 1995; Liu et al., 2005; Lamote and Dunton, 2006; Mvungi et al., 2012; Zribi et al., 2023), and growth rates (Hauxwell et al., 2001; Huntington and Boyer, 2008; Homer et al., 2011). In addition, due to algal growth, some seagrasses have seen their vegetative development modified (De Villele and Verlaque, 1995; Dumay et al., 2002; Van Katwijk et al., 2010; Hessing-Lewis et al., 2011) or have been harmed with a decline in shoot density (Ceccherelli and Cinelli, 1997; Taplin et al., 2005), shoot biomass (Taplin et al., 2005), metabolic efficiency and, ultimately, survival rates (De Villele and Verlaque, 1995; Hauxwell et al., 2001; Martínez-Lüscher and Holmer, 2010; Homer et al., 2011).
Seagrass-macroalgal negative interactions have also resulted from the competition for nutrients (Ceccherelli and Cinelli, 1997; Davis and Fourqurean, 2001; Homer et al., 2011; Alexandre et al., 2017, 2021). While a few studies found that seagrass nutrient uptake rates were negatively affected by the presence of algae (Alexandre et al., 2017, 2021), at least one study has found that seagrass could also harm the nutrient uptake of macroalgae (Alexandre et al., 2017). Other studies have found that has a result of nutrient competition, seagrasses have been affected by a reduction in growth rates (Homer et al., 2011), shoot density (Ceccherelli and Cinelli, 1997), and short-shoot size (Davis and Fourqurean, 2001). The alternative outcome (i.e., a reduction in macroalgal growth and thalli size) has been documented in one study only (Davis and Fourqurean, 2001). Partially related phenomena, such as algal decomposition, have been found to account for several indirect impacts on seagrasses (Homer and Nielsen, 2007; Han et al., 2016). Algal decomposition increased nitrogen and reduced carbon content of seagrasses, rapidly impacting their C:N ratio (Han et al., 2016). The same factor has been shown to increase sulfide pools in the sediment, invading seagrass roots and indirectly causing a decline in their growth rates (Homer and Nielsen, 2007).
A frequent focus of studies documenting negative interactions is the role of sudden macroalgal blooms on seagrasses (Nelson and Lee, 2001; Brun et al., 2003; Cummins et al., 2004; Stafford and Bell, 2006; Sugimoto et al., 2007; Hessing-Lewis et al., 2011, 2015; Olyarnik and Stachowicz, 2012; Bittick et al., 2018; Santos et al., 2020). These studies have found that as a result of a bloom, seagrasses have experienced declines in shoot density (Nelson and Lee, 2001; Sugimoto et al., 2007; Hessing-Lewis et al., 2011, 2015; Olyarnik and Stachowicz, 2012; Bittick et al., 2018), shoot height (Sugimoto et al., 2007; Hessing-Lewis et al., 2011), shoot production (Olyarnik and Stachowicz, 2012), seedling density (Sugimoto et al., 2007), foliage cover (Santos et al., 2020), elongation rates (Brun et al., 2003), biomass (Hessing-Lewis et al., 2011, 2015; Olyarnik and Stachowicz, 2012), growth rates (Sugimoto et al., 2007), and survival rates (Olyarnik and Stachowicz, 2012). Additionally, more persistent macroalgal blooms have increased seagrass fragmentation (Santos et al., 2020) and in some instances have allowed macroalgae to replace seagrass (Stafford and Bell, 2006). Decomposition following algal blooms has also been linked to a decrease in seagrass biomass (Cummins et al., 2004). A few additional studies have reported negative seagrass-algal interactions but have not conclusively identified the causing or mediating mechanisms (Ceccherelli and Campo, 2002; Taplin et al., 2005; Martínez-Lüscher and Holmer, 2010; Thomson et al., 2013; Kalokora et al., 2021; Menicagli et al., 2021; Firth et al., 2023).
3 Evidence of seaweed-seagrass positive and neutral interactions
Although negative interactions dominate the relationships between seagrasses and macroalgae, there are instances in which positive interactions have been also documented (Ceccherelli and Cinelli, 1999; Ceccherelli and Campo, 2002; Irlandi et al., 2004; Hessing-Lewis et al., 2011; Alexandre et al., 2017; Pereda-Briones et al., 2019; Kalokora et al., 2021; Correia et al., 2022a; Emmclan et al., 2022). While most of these studies have shown that seagrasses could benefit from the presence of macroalgae (Ceccherelli and Campo, 2002; Irlandi et al., 2004; Hessing-Lewis et al., 2011; Pereda-Briones et al., 2019; Kalokora et al., 2021; Emmclan et al., 2022), a couple of studies found the opposite, i.e., a benefit primarily toward the macroalgae (Ceccherelli and Cinelli, 1999; Alexandre et al., 2017; Correia et al., 2022a). The presence of macroalgae has been causally associated with increased seagrass shoot density (Ceccherelli and Campo, 2002), enhanced growth (Kalokora et al., 2021) positive changes in seagrass morphology (Ceccherelli and Campo, 2002; Emmclan et al., 2022), and added protection from low-tide associated stressors, excessive epiphyte cover, hydrodynamic disturbance, and ocean warming (Irlandi et al., 2004; Hessing-Lewis et al., 2011; Pereda-Briones et al., 2019). Meanwhile, the presence of seagrasses has been shown to enhance nutrient uptake in macroalgae (Alexandre et al., 2017), increase macroalgal density (Correia et al., 2022a), and provide protection against currents, tides, and hydrodynamic disturbance (Ceccherelli and Cinelli, 1999). Most of these positive interactions have been reported from off-shore, more marine-influenced zones (Ceccherelli and Cinelli, 1999; Hessing-Lewis et al., 2011), and have taken place between species of the families Zosteraceae (Ceccherelli and Campo, 2002; Hessing-Lewis et al., 2011; Alexandre et al., 2017) and Ulvaceae (Hessing-Lewis et al., 2011; Alexandre et al., 2017; Emmclan et al., 2022).
A few additional studies have also documented neutral seagrass-macroalgal interactions (Maciá, 2000; Ceccherelli and Sechi, 2002; Irlandi et al., 2004; Hessing-Lewis et al., 2011; Höffle et al., 2011; Glasby, 2013; Hessing-Lewis and Hacker, 2013; Alexandre et al., 2017; Menicagli et al., 2021; Firth et al., 2023). The results of these studies showed no significant effects of macroalgal presence on seagrass nutrient uptake rates (Alexandre et al., 2017), nutrient partitioning (Firth et al., 2023), shoot density (Ceccherelli and Sechi, 2002), growth (Irlandi et al., 2004; Höffle et al., 2011; Glasby, 2013), and biomass (Maciá, 2000; Hessing-Lewis et al., 2011). Likewise, seagrasses have been demonstrated to have only a minor influence (whether positive or negative) on macroalgae nutrient uptake rates (Alexandre et al., 2017) and blade density (Ceccherelli and Sechi, 2002). An interesting observational study found that the lack of seagrass responses to high fluctuations of macroalgal production at a given site was due to the mediation of tides and local currents (Hessing-Lewis and Hacker, 2013). In addition, an experimental study found that the interaction between seagrass and macroalgae could be shifted from competitive to neutral in the presence of high-density polyethylene plastics and sedimentation (Menicagli et al., 2021). These neutral interactions were most often found between species of the genus Zostera (Hessing-Lewis et al., 2011; Höffle et al., 2011; Hessing-Lewis and Hacker, 2013; Alexandre et al., 2017) and the families Ulvaceae (Hessing-Lewis et al., 2011; Hessing-Lewis and Hacker, 2013; Alexandre et al., 2017) and Caulerpaceae (Ceccherelli and Sechi, 2002; Glasby, 2013; Menicagli et al., 2021).
4 Drift algae
Although macroalgae are often anchored to the seafloor, evidence of interactions between seagrasses and macroalgae was also examined for a smaller (representative) complement of drift algae. We found no clear indication that drift algae were more often responsible for negative interactions (as in the study by Lanari et al., 2018) than anchored algae. Similarly, cases of positive interactions were found to occur with both anchored (Ceccherelli and Cinelli, 1999; Ceccherelli and Campo, 2002; Alexandre et al., 2017) and drifting macroalgae (Irlandi et al., 2004; Hessing-Lewis et al., 2011; Alexandre et al., 2017; Correia et al., 2022a; Emmclan et al., 2022). Interestingly, neutral interactions were more frequently found with drifting macroalgae (Maciá, 2000; Irlandi et al., 2004; Hessing-Lewis et al., 2011; Höffle et al., 2011; Hessing-Lewis and Hacker, 2013) than anchored forms (Ceccherelli and Sechi, 2002; Glasby, 2013). The latter results may be due to the shorter residence of drift algae on or around seagrasses, or possibly due to the seagrass' clonal nature, that potentially minimized the effects by these “transient” macroalgae (Irlandi et al., 2004). When found, positive interactions were often context-dependent and species-dependent. For example, Caulerpa taxifolia was found to have negative effects on Posidonia oceanica (De Villele and Verlaque, 1995) and Cymodocea nodosa (Ceccherelli and Campo, 2002) while having a positive impact on traits like the shoot density of Zostera nolteii (Ceccherelli and Campo, 2002). More research addressing context (habitat) and species dependency is clearly necessary, as local complements of species change as a result of climate events.
5 Some implications in the face of climate change
The evidence examined shows that interactions between seagrasses and macroalgae are predominantly negative and that seagrass species are most often at a competitive disadvantage. Extreme weather events resulting from climate change may increase the frequency and severity of floods and surface runoff (e.g., Chegwidden et al., 2020), which could change even further the balance of interactions between these primary producers. Increasing rates of eutrophication (Nazari-Sharabian et al., 2018) may account for some of these changes, as many of the articles reviewed, explicitly or not, point toward interactions between algae and seagrass that have been mediated by changing nutrient levels (Hauxwell et al., 2001, 2003; Ceccherelli and Campo, 2002; Ceccherelli and Sechi, 2002; Hessing-Lewis et al., 2015; Han et al., 2016; Alexandre et al., 2017, 2021). Macroalgae have been shown to be at a competitive advantage for nutrient acquisition, with only one study finding a neutral interaction when facing a nutrient load increase (Ceccherelli and Sechi, 2002). Rising ocean temperatures likely add to this imbalance: evidence of interactions taking place at different temperatures (e.g., Martínez-Lüscher and Holmer, 2010; Höffle et al., 2011; Homer et al., 2011) suggests that the negative effects of seaweeds and rising temperatures on seagrasses are either additive or synergistic.
Macroalgae seem generally more resistant to rising temperatures and, in some cases, can also take advantage of increased nutrient availability (Höffle et al., 2011; Duarte et al., 2018). Meanwhile, seagrass species become quickly stressed (and harmed), as they are generally more vulnerable to rising temperatures and the rapid growth of macroalgae (Martínez-Lüscher and Holmer, 2010; Höffle et al., 2011; Homer et al., 2011). This is concerning given that most climate change forecasts (see Bindoff et al., 2019) predict an increase in water temperatures and therefore more frequent increases in macroalgal biomass (Cressey, 2017; Gobler et al., 2017). Beyond changes associated with macroalgae and warming, various other co-occurring anthropogenic factors have already been found to be directly or indirectly responsible for seagrass decline or loss (Orth and Moore, 1983; Walker and McComb, 1992; Short and Wyllie-Echeverria, 1996; Orth et al., 2006; Waycott et al., 2009), making negative cumulative effects plausible, and the outcome of seagrass-algal interactions even more complex (see review by Pirotta et al., 2022). Even though the negative prospects for seagrass are unmistakable, Bennett et al. (2022) has highlighted the considerable resilience of these primary producers and the research by Kendrick et al. (1999, 2002) suggests that seagrass losses are not irreversible. In addition, and unlike other (more controversial) environmental issues, there seems to be a unified view in the public discourse addressing the role played by current conservation efforts (Nordlund et al., 2018; Unsworth et al., 2019): in the face of a changing ocean, these efforts are critical for protecting existing seagrass meadows and, where possible, for reversing some alarming seagrass losses.
Author contributions
MR: Data curation, Formal analysis, Investigation, Methodology, Writing – original draft, Writing – review & editing. PQ: Conceptualization, Formal analysis, Funding acquisition, Investigation, Methodology, Supervision, Writing – original draft, Writing – review & editing.
Funding
The author(s) declare that no financial support was received for the research, authorship, and/or publication of this article.
Acknowledgments
We thank Paula Tummon Flynn, Danielle Bryanton (UPEI) and three reviewers for their comments and feedback on earlier versions of this manuscript. A USRA fellowship (MR) and a Discovery grant (PAQ) both from the Natural Sciences and Engineering Research Council of Canada (NSERC) provided support during the preparation of this Mini Review.
Conflict of interest
The authors declare that the research was conducted in the absence of any commercial or financial relationships that could be construed as a potential conflict of interest.
The author(s) declared that they were an editorial board member of Frontiers, at the time of submission. This had no impact on the peer review process and the final decision.
Publisher's note
All claims expressed in this article are solely those of the authors and do not necessarily represent those of their affiliated organizations, or those of the publisher, the editors and the reviewers. Any product that may be evaluated in this article, or claim that may be made by its manufacturer, is not guaranteed or endorsed by the publisher.
Supplementary material
The Supplementary Material for this article can be found online at: https://www.frontiersin.org/articles/10.3389/fclim.2023.1283305/full#supplementary-material
References
Alexandre, A., Baeta, A., Engelen, A. H., and Santos, R. (2017). Interactions between seagrasses and seaweeds during surge nitrogen acquisition determine interspecific competition. Scient. Rep. 7, 13651. doi: 10.1038/s41598-017-13962-4
Alexandre, A., Collado-Vides, L., and Santos, R. (2021). The takeover of Thalassia testudinum by Anadyomene sp. at Biscayne Bay, USA, cannot be simply explained by competition for nitrogen and phosphorous. Marine Pollut. Bull. 164, 112326. doi: 10.1016/j.marpolbul.2021.112326
Anton, A., Randle, J. L., Garcia, F. C., Rossbach, S., Ellis, J. I., Weinzierl, M., et al. (2020). Differential thermal tolerance between algae and corals may trigger the proliferation of algae in coral reefs. Global Change Biol. 26, 4316–4327. doi: 10.1111/gcb.15141
Bennett, S., Alcoverro, T., Kletou, D., Antoniou, C., Boada, J., Buñuel, X., et al. (2022). Resilience of seagrass populations to thermal stress does not reflect regional differences in ocean climate. New Phytol. 233, 1657–1666. doi: 10.1111/nph.17885
Bindoff, N. L., Cheung, W. W. L., Kairo, J. G., Aristegui, J., Guinder, V. A., Hallberg, R., et al. (2019). “Changing ocean, marine ecosystems, and dependent communities.” in IPCC Special Report on the Ocean and Cryosphere in a Changing Climate, eds. H.-O. Portner, D. C. Roberts, V. Masson-Delmotte, P. Zhai, M. Tignor (Cambridge, UK and New York, NY, USA: Cambridge University Press) 447–587.
Bittick, S. J., Sutula, M., and Fong, P. (2018). A tale of two blooms: Negative and predictable effects of two common bloom-forming macroalgae on seagrass and epiphytes. Mar. Environ. Res. 140, 1–9. doi: 10.1016/j.marenvres.2018.05.018
Breeman, A. M. (1988). Relative importance of temperature and other factors in determining geographic boundaries of seaweeds: experimental and phenological evidence. Helgol. Wiss. Meeresunters. 42, 199–241. doi: 10.1007/BF02366043
Brun, F. G., Vergara, J. J., Navarro, G., Hernández, I., and Pérez-Lloréns, J. L. (2003). Effects of shading by Ulva rigida canopies on growth and carbon balance of the seagrass Zostera noltii. Mar. Ecol. Progress Series. 265, 85–96. doi: 10.3354/meps265085
Carpenter, K. E., Abrar, M., Aeby, G., Aronson, R. B., Banks, S., Bruckner, A., et al. (2008). One-third of reef-building corals face elevated extinction risk from climate change and local impacts. Science 321, 560–563. doi: 10.1126/science.1159196
Ceccherelli, G., and Campo, D. (2002). Different effects of Caulerpa acemose on two co-occuring seagrasses in the Mediterranean. Botan. Mar. 45, 71–76. doi: 10.1515/BOT.2002.009
Ceccherelli, G., and Cinelli, F. (1997). Short-term effects of nutrient enrichment of the sediment and interactions between the seagrass Cymodocea nodosa and the introduced greed alga Caulerpa taxifolia in a Mediterranean bay. J. Exper. Mar. Biol. Ecol. 217, 165–177. doi: 10.1016/S0022-0981(97)00050-6
Ceccherelli, G., and Cinelli, F. (1999). Effects of Posidonia oceanica canopy on Caulerpa taxifolia size in a north-western Mediterranean bay. J. Exper. Mar. Biol. Ecol. 240, 19–36. doi: 10.1016/S0022-0981(99)00044-1
Ceccherelli, G., and Sechi, N. (2002). Nutrient availability in the sediment and the reciprocal effects between the native seagrass Cymodocea nodosa and the introduced rhizophytic alga Caulerpa taxifolia. Hydrobiologia. 474, 57–66. doi: 10.1023/A:1016514621586
Chegwidden, O., Rupp, D. E., and Nijssen, B. (2020). Climate change alters flood magnitudes and mechanisms in climatically-diverse headwaters across the northwestern United States. Environ. Res. Lett. 15, 094048. doi: 10.1088/1748-9326/ab986f
Chung, I. K., Beardall, J., Mehta, S., Sahoo, D., and Stojkovic, S. (2011). Using marine macroalgae for carbon sequestration: a critical appraisal. J. Appl. Phycol. 23, 877–886. doi: 10.1007/s10811-010-9604-9
Coppejans, E., Beeckman, H., and De Wit, M. (1992). “The seagrass and associated macroalgal vegetation of Gazi Bay (Kenya),” in The Ecology of Mangrove and Related Ecosystems: Proceedings of the International Symposium held at Mombasa, Kenya (Springer Netherlands), 24–30. doi: 10.1007/978-94-017-3288-8_7
Correia, K. M., Alford, S. B., Belgrad, B. A., Darnell, K. M., Darnell, M. Z., Furman, B. T., et al. (2022a). Drift macroalgal distribution in northern Gulf of Mexico seagrass meadows. PeerJ. 10, e13855. doi: 10.7717/peerj.13855
Correia, K. M., Alford, S. B., Belgrad, B. A., Darnell, K. M., Darnell, M. Z., Furman, B. T., et al. (2022b). Drift macroalgae positively influence seagrass-associated nekton communities of the northern Gulf of Mexico. Front. Environ. Sci. 10, 939296. doi: 10.3389/fenvs.2022.939296
Correia, K. M., and Smee, D. L. (2022). Habitat suitability of drift macroalgae in two shallow coastal estuaries of the northern Gulf of Mexico. J. Exper. Mar. Biol. Ecol. 557, 151808. doi: 10.1016/j.jembe.2022.151808
Costanza, R., Arge, R., Groot, R., De Farber, S., Grasso, M., Hannon, B., et al. (1997). The value of the world's ecosystem services and natural capital. Nature. 387, 253–260. doi: 10.1038/387253a0
Cressey, D. (2017). Climate change is making algal blooms worse. Nature. 2, 84. doi: 10.1038/nature.2017.21884
Cummins, S. P., Roberts, D. E., and Zimmerman, K. D. (2004). Effects of the green macroalga Enteromorpha intestinalis on macrobenthic and seagrass assemblages in a shallow coastal estuary. Mar. Ecol. Progr. Series. 266, 77–87. doi: 10.3354/meps266077
Davis, B. C., and Fourqurean, J. W. (2001). Competition between the tropical alga, Halimeda acemosee, and the seagrass, Thalassia testudinum. Aquatic Botany. 71, 217–232. doi: 10.1016/S0304-3770(01)00179-6
De Villele, X., and Verlaque, M. (1995). Changes and degradation in a Posidonia oceanica bed invaded by the introduced tropical alga Caulerpa taxifolia in the North Western Mediterranean. Botanica Marina. 38, 79–87. doi: 10.1515/botm.1995.38.1-6.79
Duarte, B., Martins, I., Rosa, R., Matos, A. R., Roleda, M. Y., Reusch, T. B., et al. (2018). Climate change impacts on seagrass meadows and macroalgal forests: an integrative perspective on acclimation and adaptation potential. Front. Mar. Sci. 5, 190. doi: 10.3389/fmars.2018.00190
Duarte, C. M., Marba, N., Gacia, E., Fourqurean, J. W., Beggins, J., Barron, C., et al. (2010). Seagrass community metabolism: assessing the carbon sink capacity of seagrass meadows. Global Biogeochem. Cycles, 24, GB4032. doi: 10.1029/2010GB003793
Dumay, O., Fernandez, C., and Pergent, G. (2002). Primary production and vegetative cycle in Posidonia oceanica when in competition with the green algae Caulerpa taxifolia and Caulerpa acemose. J. Mar. Biol. Assoc. UK. 82, 379–387. doi: 10.1017/S0025315402005611
Eklöf, J. S., Henriksson, R., and Kautsky, N. (2006). Effects of tropical open-water seaweed farming on seagrass ecosystem structure and function. Mar. Ecol. Progr. Series. 325, 73–84. doi: 10.3354/meps325073
Emmclan, L. S. H., Zakaria, M. H., Ramaiya, S. D., Natrah, I., and Bujang, J. S. (2022). Morphological and biochemical responses of tropical seagrasses (Family: Hydrocharitaceae) under colonization of the macroalgae Ulva reticulata Forsskål. PeerJ. 10, e12821. doi: 10.7717/peerj.12821
Firth, L. B., Foggo, A., Watts, T., Knights, A. M., and deAmicis, S. (2023). Invasive macroalgae in native seagrass beds – vectors of spread and impacts. Ann. Botany. 72, mcad143. doi: 10.1093/aob/mcad143
Gattuso, J. P., Magnan, A. K., Bopp, L., Cheung, W. W., Duarte, C. M., Hinkel, J., et al. (2018). Ocean solutions to address climate change and its effects on marine ecosystems. Front. Marine Sci. 5, 337. doi: 10.3389/fmars.2018.00337
Glasby, T. M. (2013). Caulerpa taxifolia in seagrass meadows: killer or opportunistic weed? Biol. Invas. 15, 1017–1035. doi: 10.1007/s10530-012-0347-1
Gobler, C. J., Doherty, O. M., Hattenrath-Lehmann, T. K., Griffith, A. W., Kang, Y., and Litaker, R. W. (2017). Ocean warming since 1982 has expanded the niche of toxic algal blooms in the North Atlantic and North Pacific oceans. Proc. Natl. Acad. Sci. 114, 4975–4980. doi: 10.1073/pnas.1619575114
Han, Q., Soissons, L. M., Bouma, T. J., van Katwijk, M. M., and Liu, D. (2016). Combined nutrient and macroalgae loads lead to response in seagrass indicator properties. Mar. Pollut. Bull. 106, 174–182. doi: 10.1016/j.marpolbul.2016.03.004
Harley, C. D. G. (2011). Climate change, keystone predation, and biodiversity loss. Science. 334, 1124–1127. doi: 10.1126/science.1210199
Harley, C. D. G., Anderson, K. M., Demes, K. W., Jorve, J. P., Kordas, R. L., and Coyle, T. A. (2012). Effects of climate change on global seaweed communities. J. Phycol. 48, 1064–1078. doi: 10.1111/j.1529-8817.2012.01224.x
Hauxwell, J., Cebrián, J., Furlong, C., and Valiela, I. (2001). Macroalgal canopies contribute to eelgrass (Zostera marina) decline in temperate estuarine ecosystems. Ecology. 82, 1007–1022. doi: 10.1890/0012-9658(2001)082[1007:MCCTEZ]2.0.CO;2
Hauxwell, J., Cebrián, J., and Valiela, I. (2003). Eelgrass Zostera marina loss in temperate estuaries: relationship to land-derived nitrogen loads and effects of light limitation imposed by algae. Mar. Ecol. Progr. Ser. 247, 59–73. doi: 10.3354/meps247059
He, Q., and Silliman, B. R. (2019). Climate change, human impacts, and coastal ecosystems in the Anthropocene. Current Biol. 29, R1021–R1035. doi: 10.1016/j.cub.2019.08.042
Heck, K. L., Carruthers, T. J., Duarte, C. M., Hughes, A. R., Kendrick, G., Orth, R. J., et al. (2008). Trophic transfers from seagrass meadows subsidize diverse marine and terrestrial consumers. Ecosystems 11, 1198–1210. doi: 10.1007/s10021-008-9155-y
Hessing-Lewis, M. L., and Hacker, S. D. (2013). Upwelling-influence, macroalgal blooms, and seagrass production; temporal trends from latitudinal and local scales in northeast Pacific estuaries. Limnol. Oceanogr. 58, 1103–1112. doi: 10.4319/lo.2013.58.3.1103
Hessing-Lewis, M. L., Hacker, S. D., Menge, B. A., McConville, S., and Henderson, J. (2015). Are large macroalgal blooms necessarily bad? Nutrient impacts on seagrass in upwelling-influenced estuaries. Ecol. Applic. 25, 1330–1347. doi: 10.1890/14-0548.1
Hessing-Lewis, M. L., Hacker, S. D., Menge, B. A., and Rumrill, S. S. (2011). Context-dependent eelgrass-macroalgae interactions along an estuarine gradient in the Pacific Northwest, USA. Estuar. Coasts. 34, 1169–1181. doi: 10.1007/s12237-011-9412-8
Höffle, H., Thomsen, M. S., and Holmer, M. (2011). High mortality of Zostera marina under high temperature regimes but minor effects of the invasive macroalga Gracilaria vermiculophylla. Estuar. Coastal Shelf Sci. 92, 35–46. doi: 10.1016/j.ecss.2010.12.017
Homer, M., and Nielsen, R. M. (2007). Effects of filamentous algal mats on sulfide invasion in eelgrass (Zostera marina). J. Exper. Mar. Biol. Ecol. 353, 245–252. doi: 10.1016/j.jembe.2007.09.010
Homer, M., Wirachwong, P., and Thomsen, M. S. (2011). Negative effects of stress-resistant drift algae and high temperature on a small ephemeral seagrass species. Mar. Biol. 158, 297–309. doi: 10.1007/s00227-010-1559-5
Huntington, B. E., and Boyer, K. E. (2008). Effects of red macroalgal (Gracilariopsis sp.) abundance on eelgrass Zostera marina in Tomales Bay, California, USA. Mar. Ecol. Progr. Ser. 367, 133–142. doi: 10.3354/meps07506
Irlandi, E. A., Orlando, B. A., and Biber, P. D. (2004). Drift algae-epiphyte-seagrass interactions in a subtropical Thalassia testudinum meadow. Mar. Ecol. Progr. Ser. 279, 81–91. doi: 10.3354/meps279081
Kalokora, O. J., Gullström, M., Buriyo, A. S., Mtolera, M. S. P., and Björk, M. (2021). Seagrass meadows mixed with calcareous algae have higher plant productivity and sedimentary blue carbon storage. Ecol. Evol. 12, e8579. doi: 10.1002/ece3.8579
Kendrick, G. A., Aylward, M. J., Hegge, B. J., Cambridge, M. L., Hillman, K., Wyllie, A., et al. (2002). Changes in seagrass coverage in Cockburn Sound, Western Australia between 1967 and (1999). Aquat. Bot. 73, 75–87. doi: 10.1016/S0304-3770(02)00005-0
Kendrick, G. A., Eckersley, J., and Walker, D. I. (1999). Landscape scale changes in seagrass distribution over time: a case study from Success Bank, Western Australia. Aquat. Botany. 65, 293–309. doi: 10.1016/S0304-3770(99)00047-9
Koopmans, D., Holtappels, M., Chennu, A., Weber, M., and De Beer, D. (2020). High net primary production of Mediterranean seagrass (Posidonia oceanica) meadows determined with aquatic eddy covariance. Front. Mar. Sci. 7, 118. doi: 10.3389/fmars.2020.00118
Lamote, M., and Dunton, K. H. (2006). Effects of drift macroalgae and light attenuation on chlorophyll fluorescence and sediment sulfides in the seagrass Thalassia testudinum. J. Exper. Mar. Biol. Ecol. 334, 174–186. doi: 10.1016/j.jembe.2006.01.024
Lanari, M., Copertino, M. S., Colling, L. A., and Bom, F. C. (2018). The impact of short-term depositions of macroalgal blooms on widgeon-grass meadows in a river-dominated estuary. Harmful Algae. 78, 36–46. doi: 10.1016/j.hal.2018.07.006
Liu, S., Wang, W., Dy, D. T., and Fu, C. (2005). The effect of ulvoid macroalgae on the inorganic carbon utilization by an intertidal seagrass Thalassia hemprichii. Bot. Bull. Acad. Sin. 46, 197–203.
Maciá, S. (2000). The effects of sea urchin grazing and drift algal blooms on a subtropical seagrass bed community. J. Exper. Mar. Biol. Ecol. 246, 53–67. doi: 10.1016/S0022-0981(99)00172-0
Martínez-Lüscher, J., and Holmer, M. (2010). Potential effects of the invasive species Gracilaria vermiculophylla on Zostera marina metabolism and survival. Mar. Environ. Res. 69, 345–349. doi: 10.1016/j.marenvres.2009.12.009
Menicagli, V., Balestri, E., Vallerini, F., Battisti, D. D., and Lardicci, C. (2021). Plastics and sedimentation foster the spread of a non-native macroalga in seagrass meadows. Sci. Total Environ. 757, 143812. doi: 10.1016/j.scitotenv.2020.143812
Minguito-Frutos, M., Adams, M. P., Alcoverro, T., Vilas, M. P., Alonso, D., Mayol, E., et al. (2023). Quantifying the role of photoacclimation and self-facilitation for seagrass resilience to light deprivation. Front. Plant Sci. 14, 1186538. doi: 10.3389/fpls.2023.1186538
Mvungi, E. F., Lyimo, T. J., and Björk, M. (2012). When Zostera marina is intermixed with Ulva, its photosynthesis is reduced by increased pH and lower light, but not by changes in light quality. Aquat. Botany. 102, 44–49. doi: 10.1016/j.aquabot.2012.04.007
Nazari-Sharabian, M., Ahmad, S., and Karakouzian, M. (2018). Climate change and eutrophication: a short review. Eng. Technol. Appl. Sci. Res. 8, 3668–3672. doi: 10.48084/etasr.2392
Nelson, T. A., and Lee, A. (2001). A manipulative experiment demonstrates that blooms of the macroalga Ulvaria obscura can reduce eelgrass shoot density. Aquat. Botany. 71, 149–154. doi: 10.1016/S0304-3770(01)00183-8
Nordlund, L. M., Jackson, E. L., Nakaoka, M., Samper-Villarreal, J., Beca-Carretero, P., and Creed, J. C. (2018). Seagrass ecosystem services–What's next? Mar. Pollut. Bull. 134, 145–151. doi: 10.1016/j.marpolbul.2017.09.014
Olyarnik, S. V., and Stachowicz, J. J. (2012). Multi-year study of the effects of Ulva sp. Blooms on eelgrass Zostera marina. Mar. Ecol. Progr. Ser. 468, 107–117. doi: 10.3354/meps09973
Orth, R. J., Carruthers, T. J. B., Dennison, W. C., Duarte, C. M., Fourqurean, J. W., Heck, K. L., et al. (2006). A global crisis for seagrass ecosystems. Bioscience. 56, 987–996. doi: 10.1641/0006-3568(2006)56[987:AGCFSE]2.0.CO;2
Orth, R. J., and Moore, K. A. (1983). Chesapeake bay: an unprecedented decline in submerged aquatic vegetation. Science. 222, 57–53. doi: 10.1126/science.222.4619.51
Parmesan, C., and Yohe, G. (2003). A globally coherent fingerprint of climate change impacts across natural systems. Nature. 421, 37–42. doi: 10.1038/nature01286
Pereda-Briones, L., Terrados, J., and Tomas, F. (2019). Negative effects of warming on seagrass seedlings are not exacerbated by invasive algae. Mar. Pollut. Bull. 141, 36–45. doi: 10.1016/j.marpolbul.2019.01.049
Pirotta, E., Thomas, L., Costa, D. P., Hall, A. J., Harris, C. M., Harwood, J., et al. (2022). Understanding the combined effects of multiple stressors: a new perspective on a longstanding challenge. Sci. Total Environ. 821, 153322. doi: 10.1016/j.scitotenv.2022.153322
Santos, R. O., Varona, G., Avila, C. L., Lirman, D., and Collado-Vides, L. (2020). Implications of macroalgae blooms to the spatial structure of seagrass seascapes: The case of the Anadyomene spp. (Chlorophyta) bloom in Biscayne Bay, Florida. Mar. Pollut. Bull. 150, 110742. doi: 10.1016/j.marpolbul.2019.110742
Short, F. T., and Wyllie-Echeverria, S. (1996). Natural and human-induced disturbance of seagrasses. Environ. Conserv. 23, 17–27. doi: 10.1017/S0376892900038212
Smale, D. A., Burrows, M. T., Moore, P., O'Connor, N., and Hawkins, S. J. (2013). Threats and knowledge gaps for ecosystem services provided by kelp forests: a northeast Atlantic perspective. Ecol. Evol. 3, 4016–4038. doi: 10.1002/ece3.774
Spalding, M. D., Fox, H. E., Allen, G. R., Davidson, N., Ferdana, Z. A., Finlayson, M., et al. (2007). Marine ecoregions of the world: a bioregionalization of coastal and shelf areas. Bioscience. 57, 707. doi: 10.1641/B570707
Stafford, N. B., and Bell, S. S. (2006). Space competition between seagrass and Caulerpa prolifera (Forsskaal) Lamouroux following simulated disturbances in Lassing Park, FL. J. Exper. Biol. Ecol. 333, 49–57. doi: 10.1016/j.jembe.2005.11.025
Sugimoto, K., Hiraoka, K., Ohta, S., Niimura, Y., Terawaki, T., and Okada, M. (2007). Effects of ulvoid (Ulva spp.) accumulation on the structure and function of eelgrass (Zostera marina L.) bed. Mar. Pollut. Bull. 54, 1582–1585. doi: 10.1016/j.marpolbul.2007.06.008
Surugiu, V., Teacă, A., Svedu, I., and Quijón, P.A. (2021). A hotspot in the romanian black sea: eelgrass beds drive local biodiversity in surrounding bare sediments. Front. Mar. Sci. 8, 745137. doi: 10.3389/fmars.2021.745137
Taplin, K. A., Irlandi, E. A., and Raves, R. (2005). Interference between the macroalga Caulerpa prolifera and the seagrass Halodule wrightii. Aquat. Botany. 83, 175–186. doi: 10.1016/j.aquabot.2005.06.003
Teagle, H., Hawkins, S. J., Moore, P. J., and Smale, D. A. (2017). The role of kelp species as biogenic habitat formers in coastal marine ecosystems. J. Exper. Mar. Biol. Ecol. 492, 81–98. doi: 10.1016/j.jembe.2017.01.017
Thomson, J. A., Burkholder, D. A., Heithaus, M. R., Fourqurean, J. W., Fraser, M. W., Statton, J., et al. (2015). Extreme temperatures, foundation species, and abrupt ecosystem change: an example from an iconic seagrass ecosystem. Global Change Biol. 21, 1463–1474. doi: 10.1111/gcb.12694
Thomson, M. S., Stæhr, P. A., Nejrup, L., and Schiel, D. R. (2013). Effects of the invasive macroalgae Gracilaria vermiculophylla on two co-occuring foundation species and associated invertebrates. Aquat. Invas. 8, 133–145. doi: 10.3391/ai.2013.8.2.02
Unsworth, R. K., McKenzie, L. J., Collier, C. J., Cullen-Unsworth, L. C., Duarte, C. M., Eklöf, J. S., et al. (2019). Global challenges for seagrass conservation. Ambio. 48, 801–815. doi: 10.1007/s13280-018-1115-y
Valdez, S. R., Zhang, Y. S., van der Heide, T., Vanderklift, M. A., Tarquinio, F., Orth, R. J., et al. (2020). Positive ecological interactions and the success of seagrass restoration. Front. Mar. Sci. 7, 91. doi: 10.3389/fmars.2020.00091
Van Katwijk, M. M., Bos, A. R., Kennis, P., and de Vries, R. (2010). Vulnerability to eutrophication of a semi-annual life history: a lesson learnt from an extinct eelgrass (Zostera marina) population. Biol. Conserv. 143, 248–254. doi: 10.1016/j.biocon.2009.08.014
Walker, D. I., and McComb, A. J. (1992). Seagrass degradation in Australian coastal waters. Mar. Pollut. Bull. 25, 191–195. doi: 10.1016/0025-326X(92)90224-T
Waycott, M., Duarte, M., Carruthers, T. J. B., Orth, R. J., Dennison, W. C., Olyarnik, S., et al. (2009). Accelerating loss of seagrasses across the globe threatens coastal ecosystems. Proc. Nat. Acad. Sci. USA. 106, 12377–12381. doi: 10.1073/pnas.0905620106
Wootton, J. T., Pfister, C. A., and Forester, J. D. (2008). Dynamic patterns and ecological impacts of declining ocean pH in a high-resolution multi-year dataset. Proc. Nat. Acad. Sci. USA. 105, 18848–18853. doi: 10.1073/pnas.0810079105
Zribi, I., Ellouzi, H., Mnasri, I., Abdelkader, N., Ben Hmida, A., Dorai, S., et al. (2023). Effects of shading imposed by the algae Chaeotomorpha linum loads on structure, morphology and physiology of the seagrass Cymodocea nodosa. Mar. Environ. Res. 188, 106001. doi: 10.1016/j.marenvres.2023.106001
Keywords: seagrass beds, macroalgae, interactions, resources, global warming
Citation: Richard M and Quijón PA (2023) Seagrass-macroalgal interactions in a changing ocean. Front. Clim. 5:1283305. doi: 10.3389/fclim.2023.1283305
Received: 25 August 2023; Accepted: 13 November 2023;
Published: 06 December 2023.
Edited by:
Lazaro Marín-Guirao, Spanish Institute of Oceanography, SpainReviewed by:
Christian Todd Hayes, Waynesburg University, United StatesMariano Lastra, University of Vigo, Spain
Lucia Fanini, University of Salento, Italy
Copyright © 2023 Richard and Quijón. This is an open-access article distributed under the terms of the Creative Commons Attribution License (CC BY). The use, distribution or reproduction in other forums is permitted, provided the original author(s) and the copyright owner(s) are credited and that the original publication in this journal is cited, in accordance with accepted academic practice. No use, distribution or reproduction is permitted which does not comply with these terms.
*Correspondence: Pedro A. Quijón, cHF1aWpvbiYjeDAwMDQwO3VwZWkuY2E=