- 1Economic Commission for Latin America and the Caribbean, Mexico City, Mexico
- 2Climate Service Center Germany (GERICS), Helmholtz-Zentrum Hereon, Hamburg, Germany
- 3Research Group Climate Change and Security, Institute of Geography, Universität Hamburg, Hamburg, Germany
- 4United Nations Educational, Scientific and Cultural Organization (UNESCO) Chair Con-E-Ect, Guatemala City, Guatemala
- 5Centro de Investigaciones Geofísicas (CIGEFI), Escuela de Física y Centro de Investigación en Matemática Pura y Aplicada (CIMPA), Ciudad de la Investigación, Universidad de Costa Rica, San José, Costa Rica
- 6Escuela de Geografía, Universidad de Costa Rica, San José, Costa Rica
- 7Independent, Washington, DC, United States
- 8Centro de Investigaciones Geofísicas (CIGEFI), Escuela de Física y Centro de Investigación en Ciencias del Mar y Limnología (CIMAR), Ciudad de la Investigación, Universidad de Costa Rica, San José, Costa Rica
- 9Centro de Estudios Ambientales y de Biodiversidad, Universidad del Valle de Guatemala, Guatemala City, Guatemala
Central America is highly impacted by current extreme events associated with climate variability and the adverse effects of climate change, showing high vulnerability compounded by its historical context and socioeconomic structure. In light of the important findings published by the WGII of the IPCC AR6 in 2022 on the adverse effects of climate change on the Central American region, there is still a clear need to improve data availability and to increase the number of studies on projections of changes in the climate, risks, impacts, vulnerability, and adaptation from the region to inform decision-makers and practitioners. The region has seen an increase in the number of adaptation projects implemented; however, there is limited information about their success or failure, and there are few case studies and reviews of lessons learned, highlighting an important gap in the implementation of effective adaptation measures. This article presents a current review of the literature on climatology, hydrology, impacts and vulnerability, mitigation and adaptation responses, action plans, and potential losses and damages in the region. It also proposes actionable recommendations based on the main gaps found and presents a case study of the Central American Dry Corridor, one of the climate change and underdevelopment hotspots of the region. We finish with a discussion highlighting the importance of considering system transitions perspectives and the need to plan and implement more transformational adaptation approaches to reduce further losses and damages and to further address adaptation gaps in Central America.
1 Introduction
Central America has historically been exposed to extreme weather events derived from the climate variability of both the Tropical North Atlantic (TNA) and Eastern Tropical Pacific (ETPac) oceans (Maldonado et al., 2018; Durán-Quesada et al., 2020). The countries in the region are consistently ranked highest in the world according to risk of being impacted by extreme weather events (Eckstein et al., 2021). This situation has been exacerbated in recent years due to climate change, and the region has been exposed to alternating events of more frequent intense rainfall, droughts, and changes in the onset and end of the rainy season (Hidalgo, 2021; Pascale et al., 2021). These changes in temperature and rainfall patterns due to climate change and natural climate variability have a profound impact on the already vulnerable population who live in high-risk areas prone to flooding or landslides.
Human and economic losses, which result in migration and displacement, changing water availability affecting annual crop systems of subsistence farmers, and increasing food insecurity as a result of crop losses changing water availability, are the most studied impacts of climate change in Central America, e.g., Figure 12.9 of Castellanos et al. (2022). However, despite its vulnerability, there is limited scientific information available about the region, as indicated by the Berrang-Ford et al. (2021), Nalau and Verrall (2021), and IPCC (2018). For example, according to Berrang-Ford et al. (2021), only 6% of adaptation-related publications focus on Central and South America. From there, we can infer that a very small amount of publications are directly related to Central America. Therefore, with this work, we aim to present the latest research about Central America, highlighting the adaptation gap.
The high vulnerability of the population derives from historical patterns of entrenched poverty and gender and ethnic inequality, poor governance, weak institutions, and high levels of malnutrition and food insecurity, particularly among rural and indigenous communities (CEPAL, 2015). The high dependency of the economy of most Central American countries on agriculture and the exploitation of natural resources makes the region highly sensitive to climate events, a situation aggravated by observed high rates of deforestation, particularly in Guatemala, Honduras, and Nicaragua (ECLAC/FAO, 2018).
Meteorological data for the region are sparse, but the trends show that aridity and agricultural and ecological droughts are increasing everywhere in the region (IPCC, 2021). Moreover, climate models show that the region will become drier with increasing temperatures (Hidalgo et al., 2013, 2021; Moreno et al., 2019), resulting in an increment of fire weather conditions, e.g., Table 12.6 of Ranasinghe et al. (2021). By 2100, water availability per capita is expected to decrease by 80%, even in low-emission scenarios of GHGs (ECLAC, 2010). These changes are affecting the already problematic food security situation, particularly for small farmers who rely on rain-fed agriculture (Hannah et al., 2017; Imbach et al., 2017; Harvey et al., 2018; Donatti et al., 2019). Even though adaptation strategies for agricultural production are increasing in the region, barriers limiting adaptive capacity persist and are derived from insufficient extension programs to support small farmers and institutional and financial constraints to provide support to these producers (Castellanos et al., 2022).
Central America is a global hotspot for climatic changes, biodiversity and a global priority for conservation (Giorgi, 2006; Feeley et al., 2013; Manes et al., 2021). Ecosystems in the region are projected to be negatively impacted by climate change, especially in the reduction of distribution areas for native species as the region becomes increasingly dryer (Hoegh-Guldberg et al., 2019; IPCC, 2022; Pörtner et al., 2023). Central American tropical rainforest could be replaced by savannah and grassland under high GHG emissions scenarios (Lyra et al., 2017). The dry and sub-humid vegetation of the so-called Central American Dry Corridor (CADC) (Quesada-Hernández, 2019) will expand to neighboring areas and replace the humid forest in the Selva Maya in northern Guatemala and southern Mexico, a tract of forest considered the second largest tropical forest in Latin America (Pons et al., 2018).
Despite the dramatic situation in Central America derived from the dire socioeconomic conditions exacerbated by the impacts of climate change (Hidalgo and Alfaro, 2012; Castellanos et al., 2022), the area shows a significant gap between its adaptation needs and the corresponding local actions (Hagen et al., 2022). The implementation of the proposed National Adaptation Plans by the governments of the region is still very limited, with strong barriers posed by weak institutions and limited funding (Castellanos et al., 2022). International support has focused mainly on mitigation activities, even though the region shows negligible emissions compared to the rest of the world. Therefore, Central America represents a perfect example of climate injustice: a region that has contributed very little to anthropogenic global warming but experiences a highly disproportionate level of impacts from climate change. Furthermore, Central American countries are indebted from facing and attending the emergencies derived from extreme weather events, limiting their future options for sustainable development, as the recent experience of the emergency response to hurricanes Eta and Iota in 2020 and other hurricanes that hit the region in the past decades demonstrated, with increasing costs in relation to their GDP (World Bank, 2021).
Thus, this policy and practice review article aims to serve as a call for action to increase attention on the current impacts of climate change and climate variability and also on the needs for adaptation initiatives in the region to close the growing gap between the adaptation needs of the local populations and the actual initiatives underway. There is a need to initiate a transformative adaptation process that focuses on reducing the sources of high vulnerability observed in the region rather than simply reacting to catastrophic events with temporary aid to impacted communities. This could be reached by aiming for a climate-resilient development as recommended by the IPCC (2022). Furthermore, we call for more investment and enhancement of research activities that can support more and better-informed decisions.
To support our call, we present a review of information, including an overview of the latest IPCC findings related to the region, highlighting recent relevant scientific publications from Central America. In the first section, we analyze the main macro- and mesoscale drivers of climate change in the region and the background to the current observed social vulnerability conditions and projected impacts of climate change. In a subsequent section, we assess the current responses to the climate change crisis by Central American countries, including relevant policies and plans to address and adapt to climate change and their access to climate finance. Finally, we present a discussion pointing to the urgent need for climate action for adaptation in the Central American region.
2 Climate change in climatology in Central America
2.1 Driving large/mesoscale forces
The Central American isthmus is a narrow band of land located between the Pacific Ocean and the Caribbean Sea that connects North and South America. Geographically, it consists of a complex topography dominated by a high-altitude volcanic mountain ridge along the Pacific coast from northwest to southeast and a series of valleys and plateaus. Located approximately between the latitudes of 5 and 20° North, this tropical region is influenced by atmospheric/oceanic large-scale and synoptic processes occurring in its surrounding seas/oceans and the interactions between them. Reviews of these climate precursors can be found in Hidalgo et al. (2015) and Maldonado et al. (2016). In Sáenz et al. (2023), an analysis of the atmospheric circulation types controlling rainfall in Central America is presented. Table 1 summarizes the characteristics of a non-exhaustive list of mesoscale to large-scale mechanisms acting in the region.
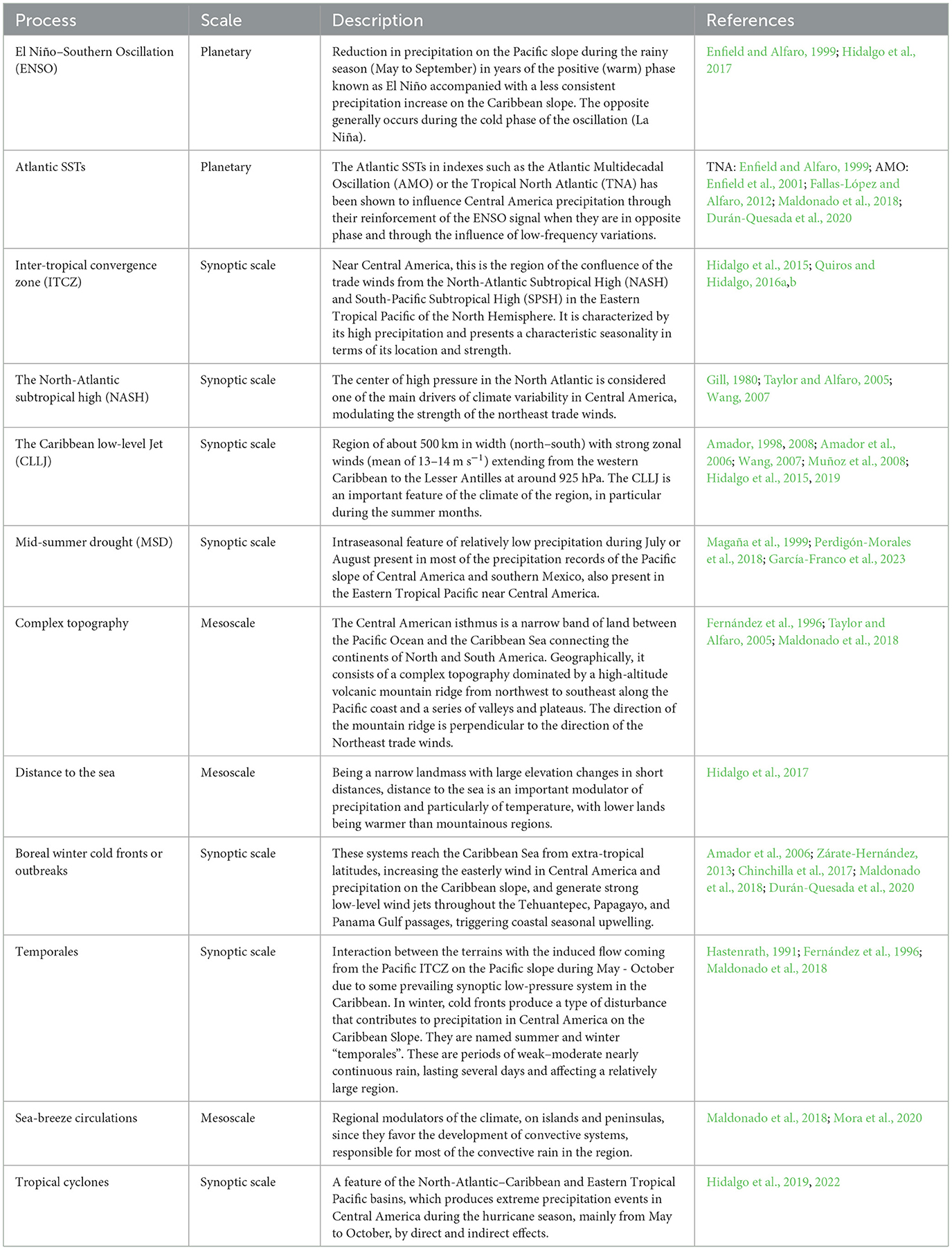
Table 1. Characteristics of some important drivers of climate variability in the Central American region.
2.2 Changes in precipitation and potential evapotranspiration resulting in increased aridity
Precipitation in Central America does not show consistent and widespread significant trends (IPCC, 2021), but temperature is significantly increasing in many locations of the isthmus (Hidalgo et al., 2017, 2019; Alfaro et al., 2020; Pascale et al., 2021). If the increase in water demand from the atmosphere (indexed by potential evapotranspiration or PET) associated with warming trends is not offset by compensating increases in the supply of water (indexed by precipitation or P), the aridity will increase. So far, from 1970 to 2010, the aridity trends, using an index of aridity (P/PET), have not yet become statistically significant in a widespread manner (Alfaro-Córdoba et al., 2020), but future temperature trends will bring significant increases in the future aridity levels in the region (smaller P/PET index) (Hidalgo et al., 2013, 2021; Moreno et al., 2019).
2.3 Models point to increased inter-seasonal variability
Most modeling efforts at the regional to local scale (Hidalgo et al., 2013, 2017, 2021; Imbach et al., 2017; Moreno et al., 2019; Almazroui et al., 2021) suggest little change in precipitation in the first half of the century and significant reductions (10–30%) at the end of the century in the northern isthmus countries. Southern countries would present increases or little change in precipitation for the future (Hidalgo et al., 2017). However, seasonal changes projected throughout the 21st century display exacerbations such as increase in the duration and intensity of the mid-summer drought (MSD) known as “canícula” or veranillo in Spanish (Steinhoff et al., 2015; Depsky and Pons, 2020). Temperature would increase monotonically by approximately +4°C compared to the historical (baseline) scenario at the end of the century in a high-emission scenario.
However, there are limitations to the models employed so far for the region. For example, Hidalgo and Alfaro (2015) revised the skill of the CMIP5 models in Central America. Their results show that models have good skills in reproducing temperature climatological patterns, while precipitation and the ENSO precipitation teleconnection patterns are reproduced with less skills. Other evaluations of the CMIP6 models can be found in Almazroui et al. (2021) and Zhang et al. (2022) are consistent with the observation for CMIP5.
2.4 Hydroclimatology of the region
The IPCC (2021) discusses the physical changes taking place across the world, and for Central America, the report states medium confidence in the increase of warm temperature extremes. Precipitation changes are mixed across the region. For heavy precipitation increases, there is low agreement on the type of change for the northern portion of Central America and low agreement due to low evidence on the type of change in the southern part of the region. Similarly, there is low agreement on the type of change in precipitation that would result in different types of drought. Individual flood events are projected to increase, although there is high uncertainty as to their magnitude. Most of the studies that assess climate change in Central America from a geophysical perspective are often mesoscale studies, which include substantial portions of either North America or South America, leaving little detail to specific changes in the region and their possible impacts (IPCC, 2021) [WG1 Physical Science Basis, Figure SPM.3: Synthesis of assessed observed and attributable regional changes (Almazroui et al., 2021)].
2.5 Data networking and dissemination in the region
Observational climate data across Central America vary largely by country (Sáenz et al., 2023). However, even in areas with better coverage, it is sparse and often contains large gaps in time series. Additionally, few of the weather and hydrological services in the region have adequate, user-friendly, and easy-access platforms to access this information. Most state-run meteorological and hydrological services are chronically underfunded and understaffed. As a result, meteorological observational data availability has decreased since around 2000, while access costs have increased (Quesada et al., 2019; Quesada-Hernández et al., 2020). The maintenance of meteorological networks is crucial for the isthmus since these observations are important for model and gridded dataset validation suitable for Central America, such as the Climate Hazards Group InfraRed Precipitation with Station data or CHIRPS (Funk et al., 2015; Centella-Artola et al., 2020). Hydrological data are even more difficult to access, and the spatial coverage of meteorological and hydrological stations on the Caribbean slope of Central America is worse than those located on the Pacific.
In response to these limitations, concerted efforts by Central American researchers in recent years have been undertaken to produce gridded data from observations and models (Hidalgo et al., 2013, 2017; Funk et al., 2015; Arciniega-Esparza and Birkel, 2020). However, more gauge-based, customized remote sensor products are needed, in addition to more dynamically downscaled data for the region, which shows scarce spatial and temporal data, limited models, and limited regionalization of GCM data. An evaluation of some of these datasets for Central America can be found in Centella-Artola et al. (2020) and Stewart et al. (2022) or at a few locations in Costa Rica (Quesada-Hernández, 2019; Ugalde, 2022).
3 Impacts of climate change in Central America
3.1 Socioeconomic determinants of vulnerability
As highlighted by the IPCC (2022), systemic vulnerabilities are one of the main drivers for the extreme vulnerability of between 3.3 and 3.6 billion people worldwide, and Central America is not exempt from this trend. Poverty levels range from 15% up to 60% (BCIE, 2020). Additionally, the COVID-19 pandemic has exacerbated poverty and inequity in the region (Programa Estado de la Nación, 2021). In particular, rural women and women living in marginal urban settlements are among the most vulnerable groups in the region, and the intersectional dimension of gender inequity needs to be addressed to adequately pursue disaster risk reduction and climate change adaptation (Segura et al., 2022).
Central America has historically suffered from poverty and inequity and the long-term consequences of colonialism, especially for Indigenous Populations (Wallman et al., 2018). The most vulnerable populations of the region, including Indigenous Peoples, are characterized, amongst others, by having a lack of access to basic services and infrastructure, accompanied by poor governance. For example, in the countries in the northern region, more than 50% of the population is still dependent on firewood and other biomass for heating and cooking, resulting in chronic respiratory problems and contributing to high deforestation rates, which, in turn, contribute to increasing ecological and social vulnerability to climate impacts. This is compounded by the high dependency on subsistence agriculture (CEPAL, 2021). Indigenous communities living in the poorest conditions (despite their wealth of culture and natural resources) in Central America are consistently more vulnerable to the impact of natural phenomena (CEPAL, 2015). El Buen Vivir (good living) is proposed as the model to follow; it is an indigenous contribution to society that seeks the complementarity of traditional knowledge with solutions to new problems based on the indigenous worldview, implying a greater respect for ethnic and cultural diversity and a peaceful and harmonious coexistence with nature in order to guarantee and not compromise the quality of life of future generations (CICA, 2015).
3.2 Impacts on agriculture and rural livelihoods
Agriculture is one of the most highly exposed economic sectors to the adverse effects of climate change. The impacts on agriculture are largely driven by changes in precipitation and other atmospheric variables leading to changes in water availability, mostly leading to reductions in crop production (Corwin, 2021; Liu et al., 2022; Rosa, 2022). Increasing levels of global warming are projected to result in higher reductions in yields of staple grains such as maize and wheat (Hoegh-Guldberg et al., 2019). Limiting global warming to 1.5°C, compared to 2°C, is projected to result in smaller net reductions in yields of maize, rice, wheat, and potentially other crops, particularly in Guatemala, Honduras, and Nicaragua, where more than two thirds of the population depends on agriculture (Imbach et al., 2017). These countries also lack the mechanisms for coping with the challenges of climate change, and there is evidence of negative impacts on the productivity of a variety of staple crops and commodities, including coffee, rice, maize, sorghum, and avocado (Jat et al., 2016; Hannah et al., 2017; Grüter et al., 2022). Projections of the effects of climate change on crops vary by crop type, elevation, and across countries (Hannah et al., 2017; Ray et al., 2019).
Climate change will have serious repercussions for agriculture, ecosystems, and farmer livelihoods in Central America (Castellanos et al., 2022). Increased aridity and seasonal variability will have impacts on natural and human systems related to reductions in water availability, also affecting environmental services (Moreno et al., 2019). Smallholder farmers are particularly vulnerable due to their reliance on agriculture and ecosystem services (Hannah et al., 2017; Imbach et al., 2017; Harvey et al., 2018; Donatti et al., 2019). There is an urgent need to develop national and local adaptation responses to reduce these impacts; however, evidence from historical climate change is still largely fragmentary.
Modeling efforts on impacts help to bridge this gap. Here, we have reviewed the past decade of research on agricultural and ecological climate change impact models for Central America. This evidence provides insights into the expected impacts of climate change, and policy actions that can help minimize these impacts have been suggested. Models point to future climate-driven changes and, often, project declines in suitability for staple crops. Several authors have applied climate vulnerability, exposure, and risk models to Central American countries (Nawrotzki et al., 2015; Garro-Quesada et al., 2023). Most of these vulnerability models focus on specific climate tolerances and thresholds for key crops but also point to intrinsic differences in exposure to hazards, household income, access to social welfare, and civil protection. Declines in suitability for coffee, a central crop in the regional economy, are noteworthy. Ecosystem models suggest that climate-driven changes are likely at low- and high-elevation montane forest transitions. Modeling of vulnerability suggests that smallholders in many parts of the region have one or more vulnerability factors that put them at risk (Hannah et al., 2017; Imbach et al., 2017; Harvey et al., 2018; Donatti et al., 2019). Subsistence agriculture and, therefore, food security are increasingly threatened by climate variability and change through the effects of storms, floods, and droughts (Imbach et al., 2017; Ewbank et al., 2019; Abeldaño Zuñiga et al., 2021; Ortiz-Bobea et al., 2021; Hagen et al., 2022). Climate change is also likely to impact husbandry by directly affecting not only livestock but also the crops and resources used in raising livestock (Meza-Herrera et al., 2022; de Jaramillo et al., 2023).
3.3 Impacts on energy
The region's energy sector is vulnerable to climate impacts (Ley, 2017). The region is highly dependent on hydropower, and therefore, changes in precipitation (both observed and projected) negatively impact the production of electricity (Lelieveld et al., 2019; Ocko and Hamburg, 2019). The current infrastructure for generation, transmission, and distribution is also vulnerable to extreme weather events, as can be seen in the destroyed and damaged infrastructure following specific extreme events, especially during hurricanes and tropical storms (Contreras and Cuba, 2008).
Energy is an enabler of other economic activities. Urban populations, particularly the elderly, are also vulnerable to heat waves, and power outages due to extreme weather events can also compound the impacts on vulnerable populations and key exposed sectors such communications. Therefore, the vulnerabilities in the energy sector have cascading and compounding effects on other sectors, such as communications, health, and food security, among others (Santamouris, 2020; Yalew et al., 2020).
3.4 Effects on health, sanitation, and hygiene (WASH)
Climate change effects on health across Central America are diverse and are related to different hydroclimatological drivers. Changes in precipitation resulting in increased variability of extremes including floods and droughts are likely to result in adverse effects on food production and freshwater quantity and quality (Moreno, 2006; Hagen et al., 2022; Pörtner H. O. et al., 2022).
Increases in temperature, increased pluvial and riverine floods, and decreased water quality are also likely to drive higher incidences of vector-borne diseases such as malaria, dengue, Zika, and Chikungunya (Moreno, 2006; Caminade et al., 2019; Agache et al., 2022) and the unprecedented spread of these diseases to places located at higher elevations (Romanello et al., 2021). Similarly, inadequate sanitation exacerbated by ecological changes could possibly expand cholera transmission, especially in coastal areas (Moreno, 2006).
Warmer water temperature is also likely to increase algae and phytoplankton blooms, thus increasing seafood contamination and reducing the usability of water for essential health and food production purposes (Moreno, 2006; Umaña, 2014). Already highly exploited coastal aquifers will be additionally subjected to saltwater intrusion associated with sea-level rise; this will also compromise the already limited access to potable water, with further impacts on emerging economic sectors such as tourism (Moreno, 2006). Recent and projected regional climate trends point to a heightened likelihood of wildfires, which can result in increased air pollution, thus causing widespread disruption of civil aviation, as the recurrent closure of major airports in Tegucigalpa and San Pedro Sula in Honduras has demonstrated. Worsening air pollution from wildfires and transport also cause a variety of respiratory effects and other ailments such as throat, lung, and eye irritations (Moreno, 2006; Lelieveld et al., 2019; Bakhtsiyarava et al., 2023). Warmer temperatures will also create occupational health risks in fields such as agriculture and industry, which will demand increased energy consumption for cooling in different industrial activities (Moreno, 2006; Kjellstrom and Crowe, 2011; Sheffield et al., 2013; Bakhtsiyarava et al., 2023). Health challenges, exacerbated by climate change, will overlap with other social challenges, largely affecting populations with limited access to resources and public health services (Yglesias-González et al., 2022). Populations located in the dry corridor in the northeastern portion of Central America (see Box 1) are highly vulnerable to climate-related health impacts due to its already compromised food security and chronic malnutrition (Gotlieb et al., 2019; Ray et al., 2019; Alfaro et al., 2020).
Box 1. In the spotlight: the Central American Dry Corridor (CADC).
The Central America Dry Corridor (CADC, Figure 1) spans Southern Mexico, Guatemala, Honduras, El Salvador, Nicaragua, and Costa Rica (sometimes its definition includes the Arco Seco region in Panama). According to Alfaro-Córdoba et al. (2020), it is defined by dry tropical forest ecosystems, with a marked and prolonged dry season of around six months from November to April. The limits of the CADC vary as they depend on climate variability, for example, from such phenomena as ENSO (El Niño–Neutral–La Niña) or the Tropical Atlantic variability (Hidalgo and Alfaro, 2012; Quesada-Hernández et al., 2020). Around 70% of the Central American population lives in the CADC territories, with access to only 30% of the region's water resources (Gotlieb and García Girón, 2020). Historically, the CADC has been structured around large-scale export-driven agriculture commodities models (Díaz Bolaños, 2019), which exerted further pressure on limited surface water supplies.
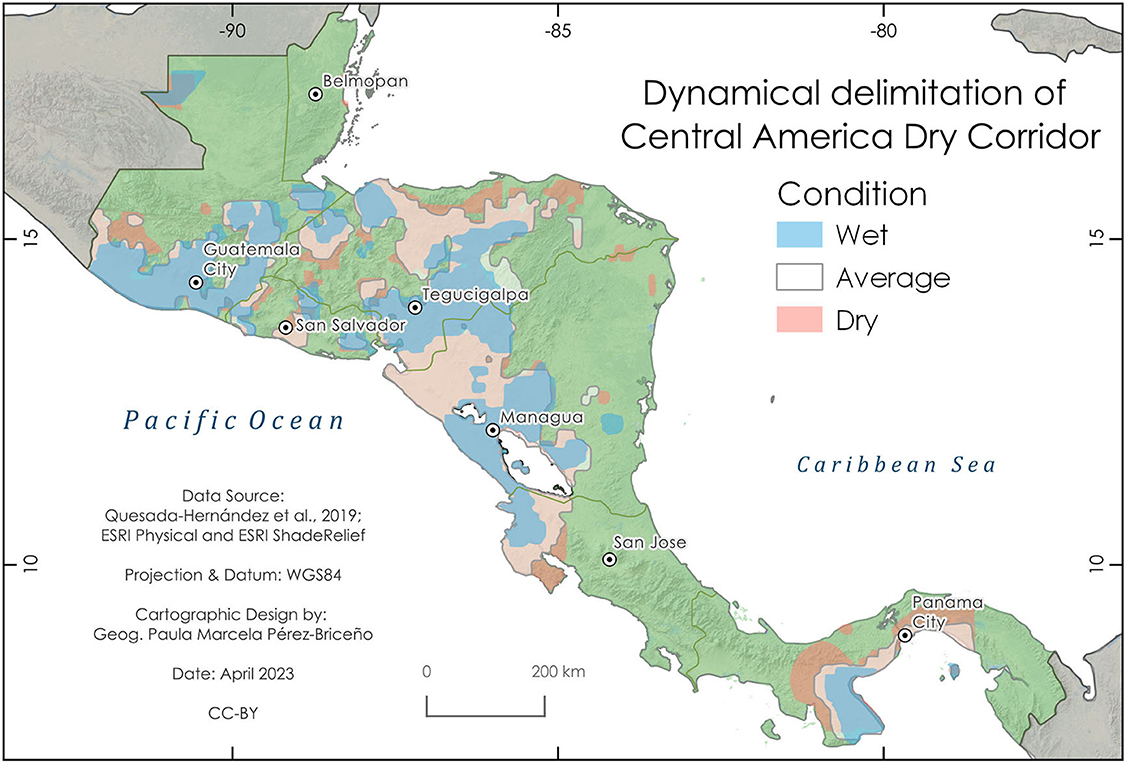
Figure 1. Delimitation of the Central American Dry Corridor (CADC) during years with wet, average (normal or neutral) and dry conditions. Modified from Quesada-Hernández (2019).
The CADC is characterized by higher relative aridity and low and high vulnerability to drought, with climate change exacerbating these challenges (Hidalgo et al., 2019; Depsky and Pons, 2020; Stewart et al., 2022). Here are some ways in which climate change impacts the CADC:
a. Drought: Climate change can lead to changes in precipitation patterns, resulting in more frequent and severe droughts (Hidalgo et al., 2019; Depsky and Pons, 2020; Stewart et al., 2022). Drought can cause crop failures, water scarcity, and food insecurity, impacting livelihoods and exacerbating poverty in indigenous and rural communities that depend on rain-fed agriculture (Imbach et al., 2017; Hoegh-Guldberg et al., 2019; Gotlieb and García Girón, 2020).
b. Water stress: Climate change reduces water availability in the CADC as higher temperatures and changing precipitation patterns can lead to decreased water replenishment in rivers, lakes, and groundwater aquifers. This can result in water stress, affecting agricultural production, livestock rearing, and access to clean drinking water (Hannah et al., 2017; Imbach et al., 2017).
c. Ecosystem degradation: Climate change can also worsen ecosystem degradation in the CADC. Higher temperatures, prolonged droughts, and changing precipitation patterns can lead to increased deforestation, land degradation, and desertification, which can further reduce the resilience of the natural ecosystems in the region (Hoegh-Guldberg et al., 2019; Gotlieb and García Girón, 2020).
d. Migration and displacement: Climate change and climate variability impacts in the CADC can contribute to increased migration and displacement (Vega-García, 2004). As livelihoods are disrupted by drought, water stress, and ecosystem degradation, communities may be forced to migrate in search of alternative livelihoods or water sources, leading to displacement and social disruption. There are socio-economic aspects that also influence the displacement of the population in the region, such as violence, insecurity, and increased poverty levels (Baez et al., 2017; WFP, 2017; Gotlieb and García Girón, 2020; Läderach et al., 2021; Miranda-Chacón et al., 2023).
e. Food insecurity: The combination of drought, water stress, ecosystem degradation, and displacement worsen existing increased food insecurity in the CADC. Crop failures, livestock losses, and reduced access to water and food can lead to malnutrition, particularly among vulnerable populations such as children, women, and indigenous communities (WFP, 2017; Gotlieb et al., 2019; Ray et al., 2019; Alfaro-Córdoba et al., 2020; Läderach et al., 2021; Miranda-Chacón et al., 2023).
f. Increased vulnerability: Climate change disproportionately affects vulnerable populations in the CADC, including small-scale farmers, indigenous communities, and marginalized groups (Gotlieb et al., 2019). These populations often have limited access to resources, infrastructure, and social services, which can exacerbate their vulnerability to climate change impacts (Miranda-Chacón et al., 2023).
Adaptation measures, such as sustainable agriculture practices, water conservation, ecosystem restoration, community resilience-building efforts, and early warning systems, are crucial for addressing the impacts of climate change in this vulnerable region (Ewbank et al., 2019; FAO, 2019; Moreno et al., 2020; Hagen et al., 2022). Three main options have been identified to increase resilience in the livelihoods of people living in the CADC (Alfaro et al., 2020): (1) provide food security by incorporating crop varieties that tolerate rising heat and changing precipitation patters; (2) transition away from export-oriented monoculture that is vulnerable to climate impacts and market volatility; and (3) employ agro-ecological systems that conserve and enrich soils and reduce water waste. In general, to increase resilience especially in the CADC, climate, security, and migration crises should be managed in an interconnected manner (Läderach et al., 2021).
3.5 Effects on ecosystems
Land-use change is the dominant driver of biodiversity loss worldwide. However, climate change has also been identified as a driver of biodiversity loss (Pörtner et al., 2023). An emerging body of scientific research has been documenting the adverse effect of climate change on biodiversity and human health (Parmesan and Singer, 2022) by proposing integrated approaches to measuring the biodiversity fingerprint of climate change (Parmesan and Yohe, 2003) and assessing global trends in biodiversity impacts through meta-data analysis (Barragan-Jason et al., 2022). Regional analysis of the impacts of climate change on biodiversity for the Central American region have been fewer (CATHALAC, 2008; Programa Estado de la Nación, 2013). Among these, Moreno et al. (2019) concluded that temperature and precipitation trends and the resulting increase in climatological aridity (lower aridity index) could reduce the provision of ecosystem services in Costa Rica's National Parks System in the future. Recent publications point to the vulnerability and exposure of coastal and estuarine ecosystems in Central America and the Caribbean to the impacts of coastal erosion and sea-level rise (Nagy et al., 2019; Pérez-Briceño and Lizano Rodríguez, 2021). Similar results were reported in a previous report on the vulnerability of Costa Rica's National System of Conservation Areas to the adverse effects of climate change, with additional impacts derived from increases in average sea levels and erosion in coastal protected areas (SINAC, 2013). The Mesoamerican region faces similar impacts attributable to climate change in those ecosystems most exposed to rapid change, as in the case of the Mesoamerican Barrier Reef System off the coast of the Yucatan Peninsula (CEPAL, 2015). This is also the case of the Páramo ecosystem in highland Costa Rica, which is particularly sensitive to higher temperatures associated with anthropogenic climate change (Kappelle and Horn, 2007; Veas-Ayala et al., 2018).
3.6 Effects on urban areas and human settlements
Inadequacies in local and national institutions and urban governance challenges in Central America have led to deficient regional planning systems, which, in turn, have generated heightened vulnerabilities in the urban areas of the region, particularly for informal settlements surrounding large cities (Hardoy and Pandiella, 2009; Demerutis and Vicuña, 2022). Most loss-and-damages reports, including fatalities, due to hydrometeorological hazards in Central America center on the most populated areas or municipalities (Pérez-Briseño et al., 2016). As of 2021, 59% percent of the population lived in urban areas with an annual growth of 3.8% (Ortiz et al., 2021). These settlements are not only overcrowded but also unsafely located on steep hills or floodplains, which increases their exposure to water-related hazards such as flooding and landslides (Hardoy and Pandiella, 2009; Bakhtsiyarava et al., 2023). The social characteristics of poverty, unemployment, the critical management of natural resources, and urban sprawl in capital cities exacerbate the negative impacts of climate-related hazards (Gencer, 2013; Pérez-Briseño et al., 2016). Rapid urbanization and loss of green areas also contribute to climate change impacts through the effects of urban heat islands (Santamouris, 2020) and increase vulnerabilities due to the loss of ecosystem services and increased exposure to hazards such as urban flooding (Vásquez et al., 2019).
Water quality is also a concern within poor communities in urban areas of Central America that lack the adequate infrastructure for drainage and waste management, especially in overcrowded settlements (Hidalgo et al., 2015; Peña-Guzmán et al., 2019; Bakhtsiyarava et al., 2023). Costa Rica has reported that sea-level rise and coastal erosion are and will also impact port infrastructure and coastal cities (Lizano and Lizano, 2010; Lizano, 2013; Lizano Araya and Lizano Rodríguez, 2020; Lizano-Araya and Lizano-Rodríguez, 2021; Lizano Araya and Lizano Rodríguez, 2022; Pérez-Briceño and Lizano Rodríguez, 2021), affecting key economic activities such as product exports and imports and tourism.
3.7 Migration
Historically, disasters such as hurricanes, floods, and droughts have created the conditions, e.g., loss or damage of livelihoods, that can trigger displacement and migration (Vega-García, 2004; Kaenzig and Piguet, 2014; Balsari et al., 2020; Kaczan and Orgill-Meyer, 2020). Internally displaced populations constitute the largest proportion of those displaced by disasters worldwide [Internal Displaced Monitoring Center (IMDC), 2023]. Following the back-to-back impacts of hurricanes Eta and Iota in 2020, at least 4.9 million people were affected, mainly in Nicaragua, Honduras, El Salvador, and Guatemala, and over 1 million people were internally displaced. For 2022, the Internal Displacement Monitoring Center (see Table 2 below) reported that Guatemala had 74,000 internally displaced people due to disasters, while Honduras reported 46.000 internal displaced people, and Nicaragua reported 4,500 internally displaced people due to disasters for the same year [Internal Displaced Monitoring Center (IMDC), 2023]. The Central American Dry Corridor, in the northeastern part of the region, is particularly battered by water scarcity and high poverty indexes, from which many migrants emerge, moving from rural to urban areas and to other countries, e.g., to the United States (Vega-García, 2004; Gotlieb et al., 2019). Estimations indicate that the number of migrants in the region might increase from 700,000 a year in 2025 to 1.5 million per year in 2050 (Balsari et al., 2020).
Although disasters derived from extreme rainfall and hurricanes are larger drivers of international migration, slow onset changes such as temperature increases and slow onset events such as droughts and sea-level rise are likely to trigger migration toward the largest cities in the region (Baez et al., 2017). An important distinction to be made between slow onset and sudden events is that the resulting migration is usually voluntary and involuntary, respectively (Cattaneo et al., 2019). Though climate impacts might trigger displacement and migration, in most cases, this is only one factor that exacerbates already dire socioeconomic conditions, such as limited land tenure, lack of access to markets, lack of infrastructure, and violence, which are the root causes of migration (Aide and Grau, 2004; IPCC, 2022). However, the process and relation between environmental change and migration are still poorly understood (Hoffmann et al., 2020).
4 Central America's response to the climate crisis
4.1 Current adaptation responses
Despite the region's negligible contribution to global warming and its exhibited high vulnerability to climate impacts, the Nationally Determined Contributions (NDCs) of the Central American countries have a greater focus on mitigation rather than on adaptation. The main sectors of focus of the Central American NDCs are land use, forestry and agriculture, waste, energy, transport, and industry (UNFCC, 2015). However, the recent updated (2020) NDCs have started incorporating greater adaptation commitments, such as in the case of Honduras, which has brought attention to the adaptation needs in different areas, such as low-lying coastal areas, agriculture, and infrastructure.
The trade-offs between mitigation responses and adaptation actions are seldom addressed, nor are the potential linkages between them. Scant attention to potential synergies and trade-offs between adaptation and mitigation approaches can be found under Goal 13 on Climate Action of the Sustainable Development Goals (SDGs). As noted, the level and resources required for adaptation are contingent upon global warming levels (i.e., mitigation actions), keeping in mind as well that certain adaptation responses may increase emissions (Singh C. et al., 2020), which, in turn, makes them maladaptive. Such undesirable outcomes can be avoided by adopting a system transitions approach, which contributes to analyzing these trade-offs (de Coninck et al., 2018; Hoegh-Guldberg et al., 2018; Donatti et al., 2019; Baumann et al., 2020; Jaramillo et al., 2020; IPCC, 2022). There are many current adaptation responses being implemented in the region, which are summarized in Table 3.
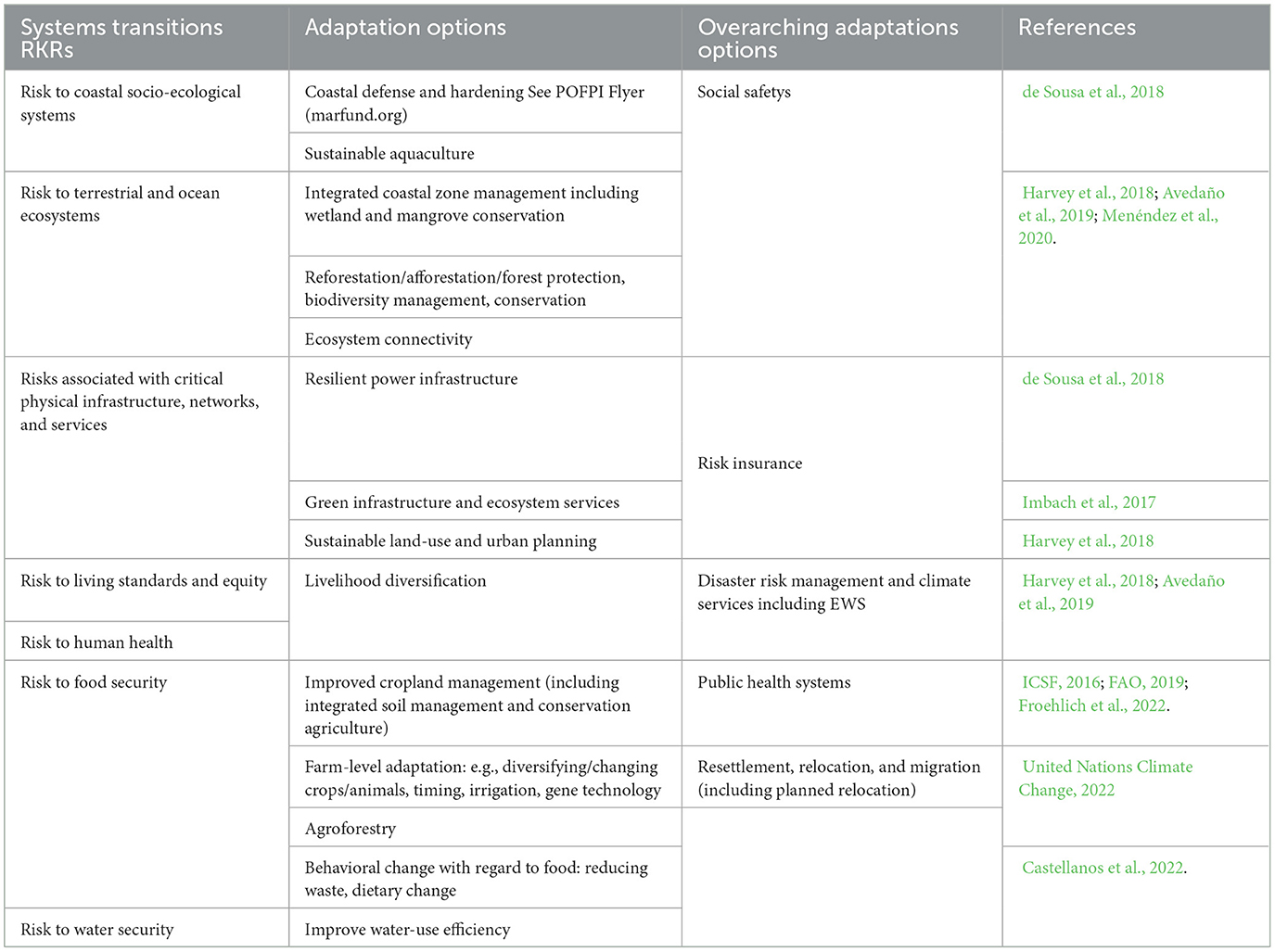
Table 3. Representative key risks and adaptation options in Central America linked to the representative key risks described above.
Adaptation responses for the agricultural sector are the most common in the countries of the region, especially due to the critical food security implications that observed and projected climate change has on food production (Imbach et al., 2017). Reforestation and agroforestry are identified among the preferred approaches implemented by Central American farmers (de Sousa et al., 2018). Adaptation responses for agriculture go beyond ecosystem-based adaptation and include soil and water management conservation, crop diversification, climate-smart agriculture, and shifting of plantations (Avedaño et al., 2019). Ecosystem-based adaptation (EbA) applied in terrestrial, freshwater, and marine ecosystems, as well as in agriculture, is also commonly and broadly implemented. It is one of the most common options used by smallholder farmers to adapt to climate impacts, for example, by planting shade trees in coffee plantations to provide shade (Harvey et al., 2018). Smallholder grain farmers in Guatemala and Honduras implement EbA practices based on local knowledge (e.g., live fences, home gardens, shade trees in coffee plantations, dispersed trees in corn fields, and other food insecurity risk reduction practices) (Castellanos et al., 2022).
Other actions include payment for environmental services (Miranda et al., 2003; UNFCC, 2015), water-use management (Schyns et al., 2019), implementation of marine biodiversity corridors such as the Eastern Tropical Pacific Seascape Project (CMAR, 2019; Acuña et al., 2020), protection of ecosystems (Menéndez et al., 2020), fisheries, and aquaculture (de Lacerda et al., 2019; Maestro et al., 2019; Caviedes et al., 2020; del Valle et al., 2020; Wright et al., 2021), and land-use management. It has been identified that community-based watershed management strategies, implemented in transboundary watersheds in Central America, have medium technological feasibility (Singh A. et al., 2020). There has also been a widespread adoption of rainwater harvesting techniques by smallholder farmers in the region (ACICAFOC, 2009). Although there is progress, and the implementation of the adaptation options has been scaled up over the years, significant gaps still remain (knowledge, finance, etc.).
Protected areas are an important instrument for adaptation policies and action in Central America (Castellanos et al., 2022). However, despite a fair proportion of the region's territories being officially declared protected areas, there are many challenges derived from the financial, technical, and human resources limitations to guaranteeing the enforcement of these regulations. Additionally, in many cases, protected areas overlap with indigenous territories in the region; therefore, any climate-related action needs to involve indigenous representatives to guarantee that their rights are protected. Protected areas in Central America are also often located in border regions, which, while contributing to transboundary biological connectivity, also contributes to the increase in poaching, human trafficking, contraband, and organized crime (Wrathall et al., 2020).
A number of actions have taken place throughout the region, although they have not been sufficient. Some examples include Panama's protection of a high number of marine areas with coral reefs and Costa Rica's protection of coral habitats within marine protected areas. The creation of reserves and private protection initiatives has been positive in other countries such as Honduras, Guatemala, and Belize (IPCC, 2022).
Adaptation in urban areas includes urban water management (Mahlknecht et al., 2020; Marcillo et al., 2020; Neumann and Hack, 2020) and resilience of infrastructure (housing, buildings, roads, etc.) (Allen et al., 2019; Sandoval et al., 2020). Resilient infrastructure goes beyond urban adaptation options as it also applies to other critical infrastructure, such as energy, roads, and telecommunications, among others. For example, increasing electricity access to non-served and underserved populations can help decrease vulnerability by allowing populations access to Early Warning Systems (EWS), radio communications with emergency services, and increased use of mobile telephone services. The reliability and resiliency of the energy sector are also required to avoid any blackouts or loss and damage of infrastructure (generation, transmission, and distribution) and the subsequent consequences to the populations served (Ley, 2017; IPCC, 2022).
Options such as climate services, including Early Warning Systems (EWS), have wider benefits as they can help the previous adaptation options to be more effective (for example, by providing information to smallholder farmers) (Ewbank et al., 2019) and provide additional services such as surveillance and observatories for health-related indicators (WMO, 2022).
4.2 Relevant institutional policies and plans by country
All Central American countries have developed policies related to climate adaptation and planning instruments in recent decades (UNEP, 2021; Segura et al., 2022; UNFCCC, 2023). However, Central America is behind in implementing adaptation responses compared with other regions (Moreno et al., 2020; Castellanos, 2022; Hagen et al., 2022; IPCC, 2022). Political and economic crisis and institutional fragility are mentioned as aspects that hinder adaptation progress in the region (Castellanos et al., 2022; New et al., 2022; Segura et al., 2022). Additionally, climate policies are not necessarily coordinated with other national policies, such as those related to development, land planning, and other sectoral policies. There is also a missing link within the science–society–policy process (Castellanos et al., 2022). There are three major drivers of climate adaptation policies in the region: international negotiations (e.g., UNFCCC), international cooperation, and extreme weather events and impacts (Segura et al., 2022). Water resource management, agriculture and food security, and biodiversity and forestry management are among the prioritized sectors where adaptation policy integration has been identified (European Commission, 2019; Segura et al., 2022; UNFCCC, 2023). As of March 2023, only Costa Rica and Guatemala have presented their National Adaptation Plans (NAPs) (UNFCCC, 2023). Other countries are in the process of preparing their NAPs (LEDS LAC, 2023). In terms of climate legislation, the UNFCCC (2023) reports that all countries have climate legislation in place. This would be an advance from the information previously presented (Segura et al., 2022), which, as of August 2018, reported that Costa Rica's climate law was under development. However, Nicaragua and Panama are lagging in their adaptation policy instruments compared to other countries (Segura et al., 2022).
Table 4 presents the status of the adaptation component in the second Central American NDCs and the National Adaptation Plans (NAPs). All Central American NDCs include an adaptation component (European Commission, 2019; UNFCCC, 2023). However, Guatemala and Nicaragua did not add any additional measures in their second NDC compared to their first. A weakness identified in Central American NDCs is that they have been developed without connection to critical development aspects such as poverty and livelihoods (Castellanos et al., 2022).
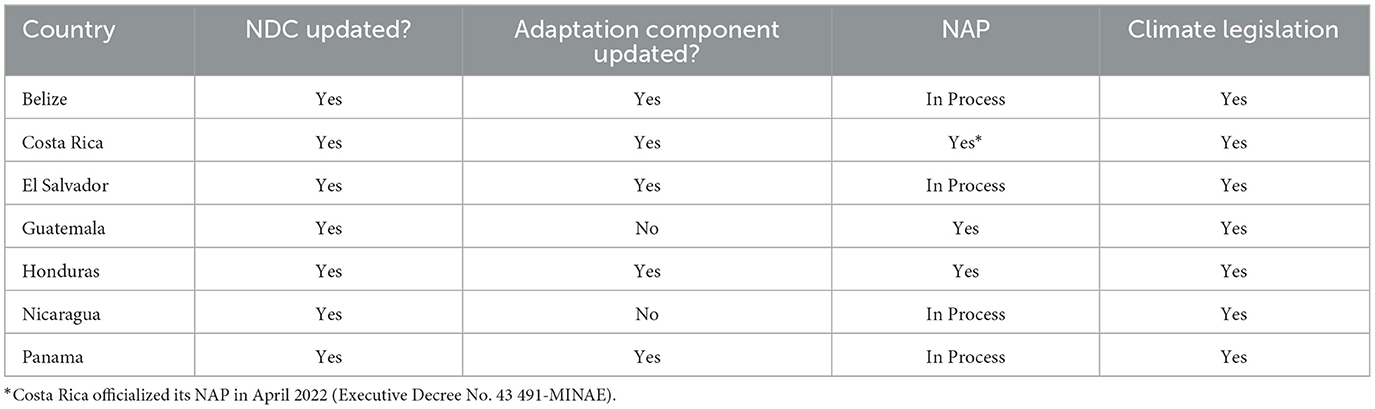
Table 4. Adaptation planning in Central America [adapted from LEDS LAC (2023) and UNFCCC (2023)].
In all Central American adaptation policies (except that of Belize), water resource management, agriculture and food security, and biodiversity and forestry management were identified as the most important sectors (Segura et al., 2022). Analysis related to the first round of the NDCs presented by Central American governments, as part of their commitments to the Paris Agreement, confirms these findings (e.g., European Commission, 2019) (see Table 5). All countries included the water sector, and adaptation options for infrastructure and health were also included, followed by forests, biodiversity, and coastal areas. It is interesting to note that energy, a critical sector for a region with a high potential for renewable energies (i.e., hydropower, solar, wind, or geothermal) was included only by Costa Rica. Tourism, an economic sector in which most countries invest, was also included only by Costa Rica. Additionally, land-use change and forestry, industry, transport, fisheries, and cities and human settlements were not included in the adaptation component of this second round of NDCs.
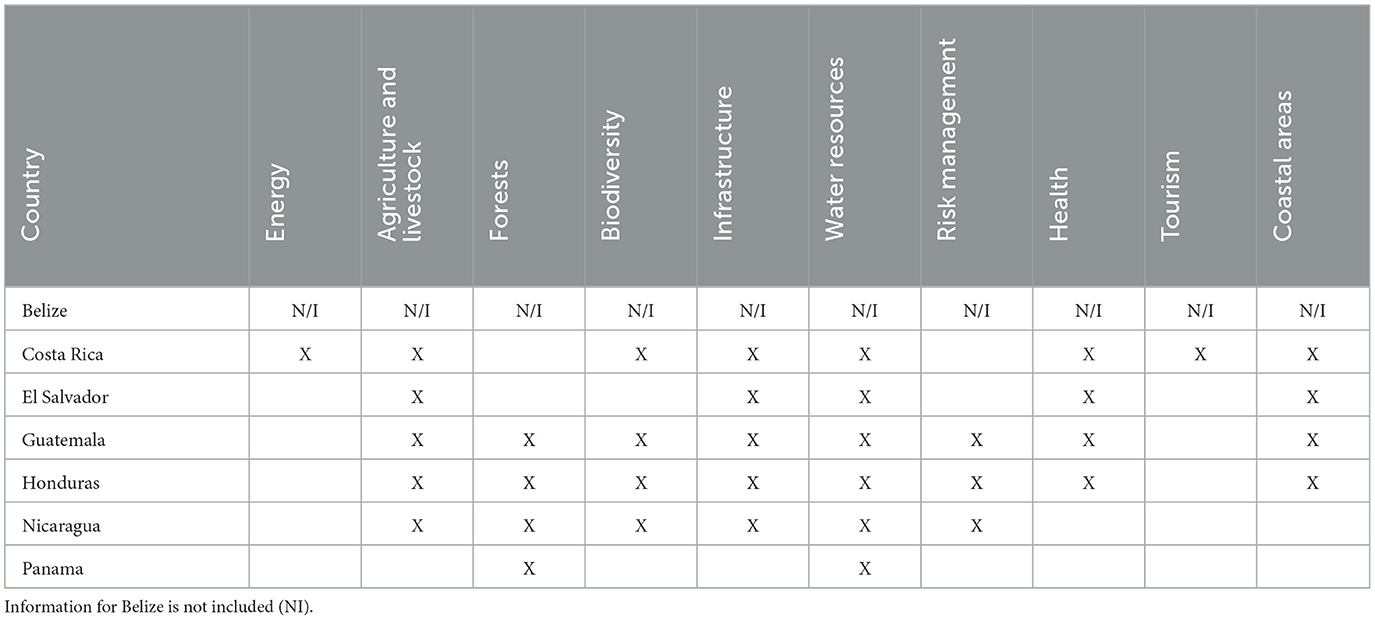
Table 5. Sectors included in Central American NDCs [own elaboration, based on European Commission (2019)].
Despite the policies in place and the increasing adaptation needs, there is limited information about the costs, needs, feasibility, effectiveness, and transformational potential of the adaptation options planned or implemented in the region (Guillén Bolaños et al., 2019, 2022; Castellanos et al., 2022).
4.3 Climate finance in Central America
Climate finance is critical to effective and accelerated climate action. However, the available climate finance is still insufficient. In addition, there is an imbalance between the resources allocated to adaptation and mitigation (UNEP/SEI, 2020). Furthermore, climate finance has been identified as one of the critical barriers to adaptation (IPCC, 2023).
The mismatch between finance allocated to mitigation and adaptation actions is also present in Central America. Contrary to the political discourses, where adaptation is highlighted as the region's priority, climate finance (national resources, and those derived from international cooperation) is mainly allocated to mitigation in most Central American countries (Guzmán et al., 2017; Castellanos et al., 2022). While climate finance has increased globally, the trends vary among countries in Central America. A high share of the climate finance allocated in the region is in the form of loans, representing additional financial burdens to a highly indebted region that has contributed little to climate change. Additionally, despite the region's high vulnerability, only a small share of the national expenditures is allocated to climate-related activities. For example, in 2015, Guatemala allocated 2.94%, Honduras 2.15%, and Nicaragua only 1.13%. At the same time, countries keep investing public resources into carbon-intensive activities (Guzmán et al., 2022).
Yet Central America presents a balanced climate finance scorecard compared to other Latin American sub-regions. Honduras, Nicaragua, and Guatemala are ranked in the medium category of the sustainable finance index (IFS), developed by the Latin American Group on Climate Finance (GFLAC, 2023) (Figure 2). The index is a revenue and expenditure monitoring tool that aims to track the resources allocated to climate-related and sustainable development activities and identify the allocation of resources to carbon-intensive activities; it is based on four indicators: sustainable revenues, carbon-intensive revenues, sustainable budget, and carbon-intensive budget. Therefore, according to the IFS, Central American countries have low revenues and expenditures associated with carbon-intensive activities. In comparison, more public funding is allocated to respond to climate-related issues compared to other countries. Costa Rica is the country that receives the highest share of climate finance in the region. On the other hand, El Salvador is one of the Latin American countries with lower carbon-intensive revenues. In terms of financial strategies to support the implementation of the activities included in their NDCs, NAPs, and other climate-related policy instruments, all countries, except Honduras and Panama, have climate finance strategies (UNFCCC, 2023).
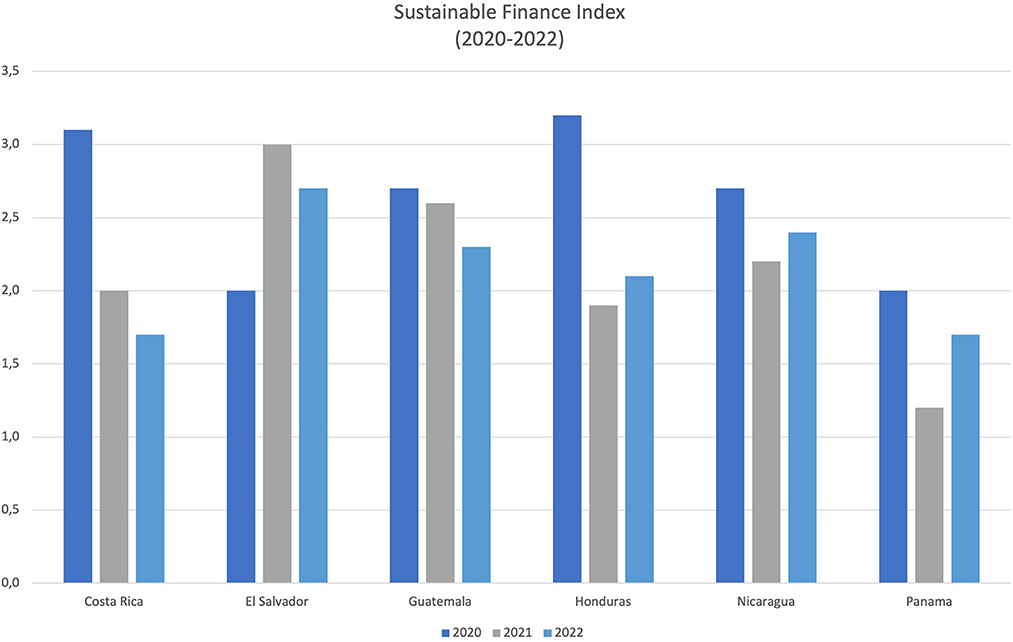
Figure 2. Sustainable finances index. Own elaboration with data from GFLAC (2023).
4.4 Observed and expected losses and damages
Estimations of losses and damages (period 1972–2010) are calculated to be up to US$ 106.4 billion, resulting from the impacts of hurricanes and storms. Damages from El Niño events totaled up to US$ 4 billion. The adaptation costs have been estimated to be up to 0.5% of the GDP (Moreno et al., 2020). In Guatemala, the economic impacts of climate-related disasters such as the hurricanes Eta and Iota, which impacted the region in 2020, were estimated to be up to 0,95% of the 2019 GDP (Bello and Peralta, 2021). Another effort estimates that losses from river flooding by 2,100 will be between 8.8 and 166.7% compared to the 2020 GDP values (according to current policies). The countries affected the most are Belize, followed by Guatemala and El Salvador. In the case of losses associated with tropical cyclones, the ranges are between 26.5 and 79.1% of GDP, with El Salvador, Guatemala, and Nicaragua being the most affected (ECLAC/FAO, 2018; Alatorre and Sepúlveda, 2022), with estimated losses, considering 22 major events, of up to US$23 billion at 2008 prices. From those countries, most losses were related to the agriculture, trade, and infrastructure sectors.
In Costa Rica, from 1988 to 2018, it was estimated that floods caused US$3,166,848,926 (2015-constant) of damages; conversely, droughts damages were estimated at US$183,860,907 (MIDEPLAN, 2019; Hidalgo et al., 2023). A single event in 2017, as part of the impact left by Tropical Storm Nate, accounted for losses and damages equivalent to 1% of GDP (Center for New Economy, 2015). It is important to note that extreme floods represented the largest source of damages, contributing to 69% of the accumulated cost of these types of impacts from extreme weather events. This is the result of the high natural climate variability associated with the influence of climate processes such as El Niño–Southern Oscillation (ENSO,) the Pacific Decadal Oscillation, the Atlantic Multidecadal Oscillation, and other phenomena in surrounding oceans (Maldonado et al., 2018; Durán-Quesada et al., 2020), as well as the effect of anthropogenic climate change (Hidalgo et al., 2019, 2021; Alfaro-Córdoba et al., 2020; Pascale et al., 2021) and the increasing vulnerability of the population (Quesada-Román, 2022).
We can infer that the Central American region will face heightened weather-related hazards, attributable to exposure due to its geographic position between two oceans and the increased likeliness of extreme weather events caused by climate change. Disaster risk is also compounded by the complex social and economic situation left by the COVID-19 pandemic, the impacts of which are still affecting the region's economies. Increased vulnerability and exposure to climate hazards have been well documented for the region (Keller et al., 2018; Garro-Quesada et al., 2023).
Although most countries in the Central American region have prioritized adaptation action in their climate change policies, the implementation of National Adaptation Policies and National Adaptation Plans has been limited and is still at an incipient stage. There are clear limits to how much adaptation measures will contribute to reducing risk scenarios associated with slow-onset events such as sea-level rise and recurrent drought (Harvey et al., 2018; Acuña et al., 2020). There are also many other drivers of climate-related risks that account for the heightened vulnerability and exposure in many urban areas of the region (UNDRR, 2021). Clearly, attribution science can help establish whether the loss and damage associated with the growing number of reported weather-related disasters in Central America can be explained by human-caused climate alterations. These changes manifest themselves as increases in the magnitude and frequency of extreme weather events, mostly explained by anthropogenic climate change. On the other hand, losses and damages are often compounded by human-related influences, such as the lack of adequate land-use planning and the deteriorating social and economic conditions due to low indices of sustainable human development in the region.
The need for more robust attribution science also requires solid and reliable hydrometeorological data sources and climate models with sufficient skill on the region. Furthermore, systematic registries and information in the region regarding extreme events, vulnerability and exposure, and climate-related loss and damage are often incomplete or unavailable. Teasing out these influences to establish clear causality and attribution of losses and damages is still a pending task.
5 Actionable recommendations
5.1 Collection, management, and dissemination of climate-related information
There is a need in the region for better access to hydroclimatic data for academic, professional, and operational use. Currently, access to data is generally limited by different types of obstacles or completely restricted in several of the countries of Central America, thus limiting the production of studies of the region's hydroclimate. Improvements are also needed in existing observation systems as the density and technological capacities of the networks of meteorological and hydrological stations have waned over the past few decades (Quesada-Montano et al., 2019; Sáenz et al., 2023). As mentioned before, the maintenance of the meteorological network is crucial for the isthmus since these ground observations are important for validating global models and gridded datasets suitable for Central America, such as CHIRPS (Funk et al., 2015; Centella-Artola et al., 2020). Additionally, observations are needed to calculate derived indices such as SPI or SPEI (Quesada et al., 2019). Measuring additional parameters (e.g., surface winds, net radiation, evapotranspiration, and others) and the use new methods and technologies for measuring parameters (e.g., meteorological radar, remote sensing, and others) are needed, as is the development of customized and validated high-resolution reanalysis and downscaled products. Giving priority attention to these improvements will enable climate-related scientific bodies to produce detailed assessments at the mesoscale and also to take analysis to basin and even catchment scales. Although CHIRPS rainfall data are freely available and can be used for rainfall variability studies in Central America, and drought indices such as SPEI are also freely available worldwide, these products are no substitutes for local data gathered from meteorological and hydrological stations.
There is also a need for the regionalization of Global Circulation Models (GCMs) projections. Although there have been several efforts that produced high-resolution downscaled estimates from GCMs by using a limited set of parameters (usually precipitation and temperature; see, for example, Hidalgo et al., 2017), there is a need for more dynamically downscaled estimates of the GCM projections for the region, especially of those GCMs that better reproduce the key climate aspects of the isthmus (Hidalgo and Alfaro, 2012; Hidalgo et al., 2015; Almazroui et al., 2021). An exception to this is the CORDEX simulations in the region, which are usually not as frequently updated as in other CORDEX regions (WCRP, 2015).
There is a need for more climate change detection and attribution studies of hydrometeorological extremes for the region. The study of Pascale et al. (2021) is perhaps the only regional study of detection and attribution in Central America. From this study, it is evident that several parameters are changing or will change in the future. For example, aridity (measured by the ratio of P/PET) is likely to increase due to future warming in the area, and the intensity of extreme precipitation events is beginning to show positive trends in some countries.
From a hydroclimatological perspective, a great deficit in the region is the study of the climatology and hydrology of its mountain areas. There is only scarce literature, e.g., Anchukaitis and Evans (2010), or grey literature on the topic, although it is often discussed in large-scale studies. Central America reaches elevations above 4,000 meters above sea level in the highlands of Guatemala, contains gradients of several thousand meters in 100–200 kms of horizontal distance, and its narrowness ranges from some 90 to 550 kms between the Pacific Ocean and Caribbean Sea. These geographical and topographical aspects generate complex climate dynamics that result in extensive precipitation and steep rivers that flush rapidly into the ocean. Mountains are considered water towers for humanity given their importance in receiving, storing, and channeling water and supplying to adjacent lower lands (Viviroli et al., 2007; Immerzeel et al., 2020). Moreover, they provide ecosystem services and opportunities for energy generation (Viviroli et al., 2007). A global study (Viviroli et al., 2007) developed a quantitative typology that estimated that the mountains of Central America are of high importance and essential in providing water to low-lying areas. Moreover, Immerzeel et al. (2020) determined that these mountain regions were highly vulnerable due to their exposure to hazards and their socioeconomic conditions. Therefore, special focus should be given to the hydroclimatological study of this mountainous region.
There is still limited availability of information and dwindling capacity of climatology modeling in the region. As a result, there is a lack of understanding of how climate change will impact key sectors such as energy, drinking water, and agriculture. For example, “the lack of up-to-date agroclimatic information is still a problem that hinders making the best decision regarding crop management, especially for the annual crops” (de Sousa et al., 2018, p. 17). Adequately addressing these gaps in information and knowledge requires concrete strategies to manage the climate outlooks at the seasonal and sub-seasonal scale for final climate users' information in socioeconomic sectors such as agriculture, energy, and health, i.e., from the national institution level to local users in CADC communities.
Even greater gaps can be found in the application of approaches based on social sciences to assess risk, vulnerability and exposure to climate hazards in order to develop socioeconomic sector assessments using the regionalization of hydroclimatic data with the inclusion of technification of sectoral assessment. Examples of this are:
- Use of hydroclimatic projections to evaluate how irrigation schedules would change and, therefore, affect crop development and, ultimately, food security.
- Use of hydroclimatic projections combined with environmental models to evaluate how environmental variables would change and thus affect specific species.
- Mapping of high vulnerability and exposure in marginal human settlements in Central America's fast-growing cities using emerging remote sensing technologies and geospatial data.
- Use of seasonal and sub-seasonal climate predictions for different sectors in the isthmus (García-Solera and Ramirez, 2012), as in the case of the successful experience developed in Guatemala, tailoring these tools for coffee farmers (Pons et al., 2021).
Finally, a unified regional measuring system is needed for the register of losses and damages attributable to the adverse effects of climate change by regional institutions in charge of the systematic observation of climate change under the Regional Center for Hydraulic Resources (CRRH in Spanish), along with the Coordination Centre for the Prevention of Natural Disasters in Central America (CEPREDENAC in Spanish) and civil defense organizations operating under the Central American Integration System (SICA in Spanish). Effectively assessing climate-related risks requires the sharing of data across the region and across different sectors through, for instance, a digital library of institutional and national plans. The transparency regarding the implementation of the plans and policies can support monitoring and evaluation actions, which, at the same time, provides governments with opportunities to identify and justify future sources of finance. Additionally, assessments on the implementation of adaptation actions can provide guidance on the need for more transformational approaches to face the challenges posed by higher levels of global warming to societies and ecosystems.
5.2 Focus on adaptation
Due to its high vulnerability but low contribution to global warming, Central America must allocate most of its resources to climate adaptation in all sectors. The actions should consider mitigation and sustainable development co-benefits to contribute to the region's climate-resilient development. Furthermore, at the current and further levels of global warming, the efficiency of adaptation options decreases, with some adaptation limits already being reached (Hoegh-Guldberg et al., 2019). Therefore, there is a need to refocus and accelerate adaptation efforts across the region. Adaptation in the region cannot focus exclusively on incremental adaptation. Incremental adaptation refers to the extension of current actions and behaviors that aim to reduce losses, as opposed to transformational adaptation, which refers to changes in the fundamental attributes of a social–ecological system (Pörtner H. et al., 2022).
As the IPCC (2018, 2022) has stated, we are already experiencing unprecedented climate conditions and measurable impacts. There is an urgent need to explore and implement transformational approaches that support adaptation efforts in the Central American region, especially for those that are most affected and vulnerable, including rural communities, women, and indigenous communities.
For this to occur, the region needs to convert current adaptation policies (NAPs, NDCs, etc.) into real action. There are opportunities for better articulating climate action plans with existing disaster risk reduction plans to avoid duplications and explore greater synergies. The adoption of the system transitions perspective proposed by the IPCC could facilitate its implementation in all levels for a more effective adaptation governance. Additionally, transboundary risks must be considered as climate actions could generate unintended impacts between neighboring countries, especially in shared international watersheds.
Adaptation planning in Central America needs to build on participative and multidisciplinary processes based on bottom-up approaches. At the local level, non-governmental organizations and community-based organizations implement and support adaptation processes but are not always included in government-driven processes. This situation might generate incomplete and one-sided policy instruments that contribute to greater maladaptation. Additionally, sub-national governments also need to be supported with financial and technical capacities.
The lack of literature on climate change in the region is not limited to projections and impacts. There is also a lack of case studies on adaptation responses that make it difficult to create a framework of the best practices for a range of options or lessons learned from the options already implemented (see Box 1). Therefore, monitoring and evaluation need to be carried out periodically and documented. Evaluations tend to happen during the funding period of donor agencies, thus not including what happens once the donor funding ends, or are limited to post-disaster recovery and reconstruction, thus not addressing long-term funding needs.
Besides finance, additional barriers to closing the adaptation gap exist, which include governance, institutions, greater availability and quality of information, capacity of development and education, and inclusion of traditional and indigenous knowledge in adaptation plans.
5.3 Priority access to climate finance
Due to the limited regional contribution to climate change but high level of vulnerability of the region, climate finance (public and private) allocated to the region needs to prioritize adaptation. This does not mean inaction on mitigation. On the contrary, in sectors such as agriculture, biodiversity, and forestry, the synergies between mitigation and adaptation approaches need to be reinforced (Castellanos et al., 2022).
Central American climate-related policies and planning instruments need to include estimation of the related costs. Additionally, countries should establish national climate finance strategies where the possible sources of finance and the sectors or systems where actions are needed are identified (Guzmán et al., 2017). These strategies can also help to identify the sectors where transitions to low-emission activities are needed and to guarantee the resources needed for monitoring and evaluation efforts, which usually stop once a project has finished.
International cooperation is an important source of climate finance in the region. However, climate action in Central America cannot only depend on international cooperation. Therefore, another important step is to reallocate resources, for example, from fossil fuel subsidies toward greater investments in renewable energies.
5.4 Declaration of internationally recognized hotspots
There are several sub-regions that have multisectoral importance with high vulnerabilities to climate change. Although precarious socioeconomic conditions are present throughout the region, global assessments, including IPCC reports (IPCC, 2022), rarely highlight hotspots in this region, in turn including hotspots under more severe conditions, e.g., Africa, or with higher economic losses. The lack of locally developed literature that highlights these conditions also contributes to the lack of visibility these hotspots may have in international venues. In the case of Costa Rica, some policy recommendations have been made recently observing the Lancet indices (Miranda-Chacón et al., 2021, 2023). Based on the literature reviewed in this article, the authors highlight the regions of the Central American Dry Corridor, the Central American Mountains, the Mesoamerican Barrier Reef, and the Eastern Tropical Pacific Seascape.
6 Discussion
In this policy and practice review article, we use the system transitions framework to enable transformative adaptation. The system transitions framework was first conceived in the IPCC's Special Report on Global Warming at 1.5°C (IPCC, 2018) to bring together the socio-technical transitions required for emissions reductions and socio-ecological resilience required to increase resilience. That is, system transitions consist of a framework to bring together mitigation, adaptation, and disaster risk reduction, recognizing that the levels of adaptation required and climate-related losses and damages are dependent upon global warming levels but also sustainable development and the synergies and trade-offs between mitigation and adaptation response options. Furthermore, a system transitions framework enables us to work across sectors to achieve climate-resilient development in Central America.
This article provides us with compelling evidence pointing to the urgent need for accelerated climate action for adaptation in Central America. Climate change is set to heighten weather-related hazards and compound existing risks due to structural socioeconomic causes of vulnerability and exposure. In order to face this existential threat, a system transition perspective is urgently required in Central America. Figure 2 proposes our modification in a series of steps that are required to adapt to the impending impact of climate change through a coupled climate–society–ecosystems transition approach. It proposes a three-prong approach to enable human system transitions while ensuring ecosystems can contribute to providing food, water, and energy security and addressing the climate crisis. Figure 2 also calls for the scaling up of adaptation finance in key exposed sectors to achieve the SDGs and a more resilient development model. This also means leaving no one behind and providing adaptation options for all. This also calls for new approaches to rural development based on sustainable livelihoods to increase food and water security. It calls for scaling up ecosystems-based approaches to adaptation through integrated landscape restoration, watershed, and integrated coastal zone management approaches to achieve water security in the face of climate impacts, including sea-level rise in coastal communities.
Figure 2 also calls for food, water, and energy security for all. To achieve this, a focus is suggested on ecosystems-based and community-based approaches as the cornerstone of this proposed system transition through the implementation the following key adaptation measures:
• Aquifer recharge and resilient water supply, including water harvesting and storage.
• Community agroforestry, simple irrigation systems for small farmers, regenerative agriculture, and landscape restoration.
• Conservation and regeneration of carbon sinks (including blue carbon) with adaptation co-benefits.
• Conservation of ecosystems, biodiversity and agrobiodiversity with mitigation co-benefits.
Food security in Central America is increasingly threatened by climate variability and change. According to the Famine Early Warning System1, heightened vulnerability and exposure compound risks as rural populations in Guatemala, Honduras, and Nicaragua are currently impacted by high food prices and shortages. Recurrent drought associated with climate variability, especially during dry–warm phases of the ENSO (i.e., El Niño), are also increasing water insecurity, mainly on the Pacific Slope, including the CADC. Continued reliance on fuelwood as the only energy source by large contingents of rural populations will also increase their vulnerability to loss of forest cover due to deforestation and recurrent forest fires. Road infrastructure and urban areas are regularly impacted by extreme weather events and associated flooding and landslides. Coastal areas and critical economic infrastructure in port cities will be impacted by rising sea levels, coastal erosion, and recurrent storm surges. Central America urgently needs to address its food, water, and energy security in the face of climate change through sound cross-cutting policies.
To plan for adaptation, we need to know what we are adapting to and to monitor whether adaptation measures are effective. Promising results from the science community in Central America point to the opportunities for improved climate and risk modeling. Initial adaptation policies can be guided by these existing modeling results. Improved modeling through tailored approaches is being developed that will allow policy action specifically targeted to stakeholders, especially vulnerable groups (Pons et al., 2021; Garro-Quesada et al., 2023).
We propose that more robust modeling of ecological responses to climate change, improved representation of the region in climate models, and simulation of climate influences on crop yields and diseases (especially coffee leaf rust) are key priorities for future research. There is also an emergent area of attribution science that includes the mapping and measuring of vulnerability and exposure and the propensity to suffer losses.
However, as we have pointed out in this work, there are limits to adaptation (Thomas et al., 2021; IPCC, 2022). The costs of inaction in adaptation are still unclear and need to be fathomed and more effectively communicated to bolster adaptive climate action. It is already clear that the costs of inaction are greater. Figure 3 identifies that in the face of inaction in both mitigation and adaptation, the likelihood of far greater losses and damages for the region increases. There is an urgent need to avert, minimize, and address losses and damages from flash floods and landslides, including slow-onset events such as sea-level rise, droughts and non-economic losses associated with the displacement of populations.
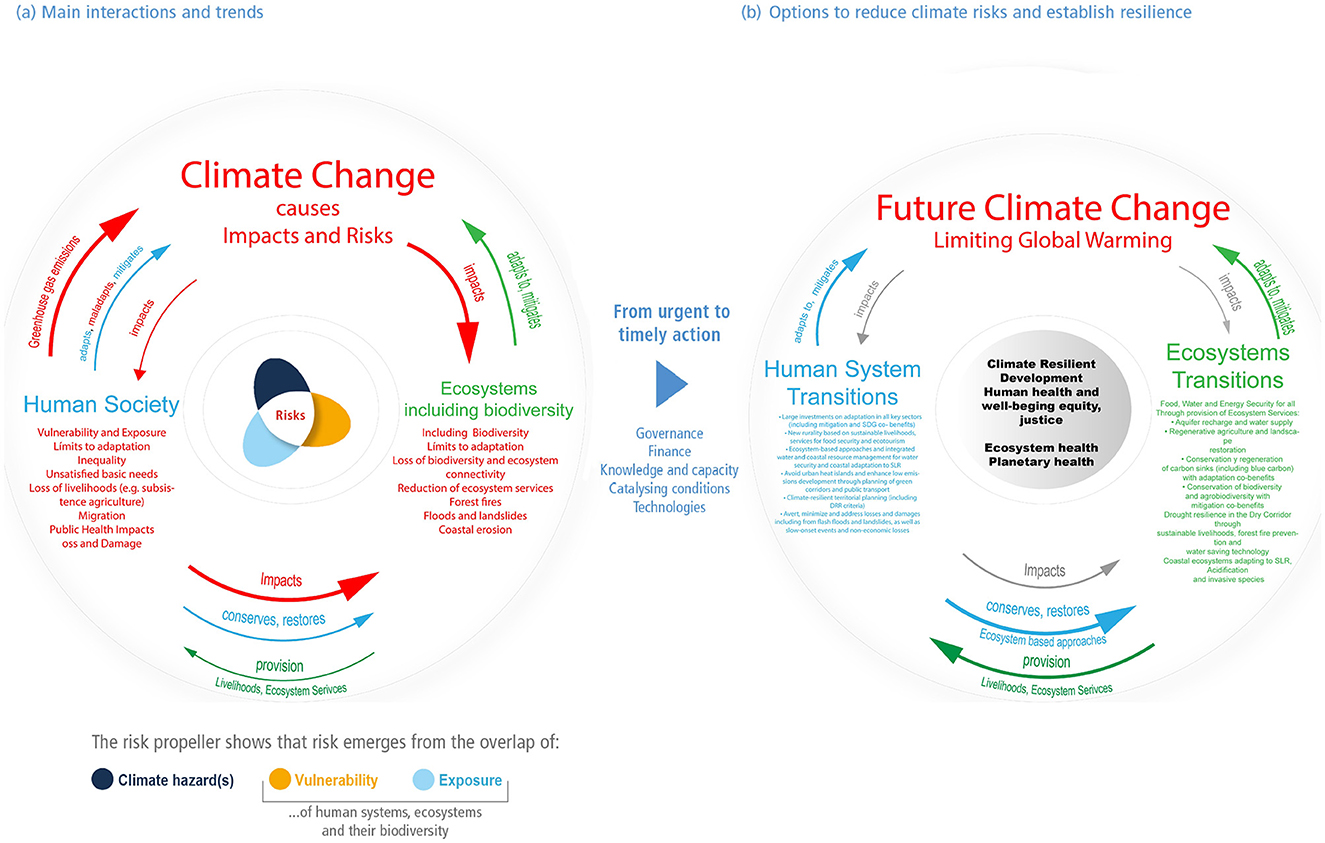
Figure 3. Interactions and transition among the coupled systems climate–society–ecosystems in Central America (modified from IPCC, 2022). From climate risk to resilient development: climate, ecosystems (including biodiversity), and human society as coupled systems. (A) Main interactions and trends. (B) Options to reduce climate risks and establish resilience.
The potential co-benefits between mitigation action and adaptation also need to be assessed through comprehensive monitoring and evaluation and policy reviews. Mitigation actions have adaptation co-benefits. For example, in the region, most mitigation efforts tend to focus on reforestation, which has multiple adaptation and sustainable development co-benefits. Protected areas function as a vehicle to integrate climate, environmental, and sustainable development objectives, and their creation and management can be carried out in an equitable and just manner. One barrier identified is that actions are implemented through vertically integrated structures that have little linkage between them, often impeding the potential of these co-benefits. A case in point is the need for more effective coordination between climate change adaptation actions and disaster risk reduction practices in Central America, which are currently working at cross-purpose in most countries. Other barriers include the segmented and limited access to climate financing sources, including the low coverage of climate insurance and re-insurance schemes in the region.
Many countries in Central America have developed a growing portfolio of projects in conservation and forest management aimed at reducing emissions from deforestation and land degradation (REDD). There are few examples of explicit approaches seeking synergies between forest landscape restoration and reforestation projects and adaptation to the impacts of climate change. Clearly, the presence of significant areas under conservation in Central America not only contributes to the long-term conservation of the region's extraordinary biodiversity but also provides growing opportunities for adaptation through sustainable livelihoods. Critical to the success of these opportunities will be our ability, as scientists from the region, to reach out and work effectively with Indigenous People, traditional knowledge holders, smallholder farmers, and rural women.
On the other hand, there are also growing risks associated with maladaptation as there is strong evidence of both soft and hard limits, particularly for adaptation responses taken by small-scale farmers (Thomas et al., 2021). Some adaptation actions can reduce the risks for some while heightening the risks for others, including downstream impacts of adaptation actions in watersheds (Montaña et al., 2016) and compounded impacts from soil degradation, crop loss, and the economic shocks associated with the post-pandemic transition in the region (Bárcena Ibarra et al., 2018). The system transitions framework presented in AR6 helps, amongst other things, to bring mitigation and adaptation together by highlighting synergies through co-benefits and identifying trade-offs, where early actions can be identified and implemented. This is especially important given the need to reduce emissions to avoid increasing the adaptation gap and reaching the limits to adaptation.
This will require a strong political commitment and a coordinated institutional response from the Central American governments, including the Central American Integration System (SICA in Spanish). Despite important efforts to address the impeding risks from climate change in the CADC, these are clearly insufficient in the light of the findings from this article. There is an urgent need for a concerted effort to bolster transformational adaptation policies in the Central American region in a strategic alliance with civil society organizations and academic institutions. Further inaction on adaptation will only result in greater hardships, losses, and damages and continued out-migration from impoverished and degraded rural landscapes and crowded and polluted urban centers in Central America. There is still time to address the transformational adaptation needs, to accelerate climate finance provision to the Central American region, and to harness the emerging opportunities linked to the launch of the Santiago Network under the Warsaw International Mechanism on Loss and Damage operating under the UNFCCC.
7 Conclusions
Global inaction in the face of growing climate impacts is rapidly closing the window of opportunity on adaptation to avoid catastrophic losses and damages in Central America. Given the high vulnerability to climate impacts and the emissions level of the region, there is a clear need for a greater focus on adaptation responses within existing public policy instruments.
The recent IPCC reports highlight the significant literature gaps for Central America. This is due to multiple factors, including the lack of accessible data and information at scales required for adaptation action, especially considering the existing microclimates in the region. This is also attributable to the lack of funding for scientific institutions, to the challenges faced by Central American researchers to publish in English-language peer-reviewed journals, and to the lack of public policies focused on science and technology geared to address the climate crisis, amongst others. This article has highlighted the limited but important contributions from Central America to the international climate science literature with recommendations on how to advance an adaptation agenda for the region.
Our call to action on adaptation also requires urgent attention to the fact that the monitoring network of climate through surface and aerial meteorological stations in the region is dwindling. The Central American region should at the very least seek to maintain the existing meteorological monitoring network in the short term and find support from different mechanisms to improve the spatial coverage of this network in the middle and long term. The Caribbean slope and stations located near mountain tops should receive special attention since more of the stations are in the Pacific slope at middle altitude. Climate observations in mountainous regions are also needed as the network of meteorological stations is very sparse in these locations. There are significant opportunities to strengthen collaboration in these areas between academic institutions and government agencies in charge of systematic earth observation and meteorological and hydrological monitoring.
The creation of public policies that address the increasing vulnerability of the region and the number of critical ecosystems at risk is much needed, in addition to mechanisms that will ensure their transparency and effectiveness. To enable this, the region needs reliable and up-to-date data, information, and studies to ensure decisions are made based on the best available science. There are also roles for other actors in the region. For example, donors and implementers of adaptation projects can help in documenting and systematizing their experiences, considering users' perceptions and expectations, and help to transition monitoring and evaluation actions to local institutions after the end of funding periods. Donors should also allocate more financial resources aligned to the region's priority of adaptation. Mitigation can be addressed as part of the co-benefits of adaptation actions and sustainable development goals.
The actionable recommendations presented here are a subset of what needs to happen to help the region become more proactive in gathering the required data, closing the adaptation gap, and moving toward the transformations we need to achieve climate-resilient development.
Author contributions
DL, TG, AC, HH, PG, RF, EA, and EC have provided substantial contributions to the conception and development of this work, drafted the manuscript, provided comments throughout the process, and agree to be accountable for all aspects of the work in ensuring that questions related to the accuracy or integrity of any part of the work are appropriately investigated and resolved. All authors contributed to the article and approved the submitted version.
Funding
HH and EA wish to acknowledge the support they received for this research through the following Vicerrectoría de Investigación, Universidad de Costa Rica, projects: B9454 (supported by Fondo de Grupos), A1715, B0-810, A5719, C2103, C3721, C3991, and A4906 (PESCTMA). TG acknowledges the support for this research by the Climate Service Center Germany (GERICS), Helmholtz-Zentrum Hereon, Germany.
Acknowledgments
Special acknowledgment to M.Sc. Paula Marcela Pérez Briceño for the drafting of the maps in Figure 1 and to Luis Vega Gonzalez for the redrafting of Figure 2. We also would like to thank Mr. Jorge Cabrera for the inputs he shared during the preparation of this article.
Conflict of interest
The authors declare that the research was conducted in the absence of any commercial or financial relationships that could be construed as a potential conflict of interest.
Publisher's note
All claims expressed in this article are solely those of the authors and do not necessarily represent those of their affiliated organizations, or those of the publisher, the editors and the reviewers. Any product that may be evaluated in this article, or claim that may be made by its manufacturer, is not guaranteed or endorsed by the publisher.
Footnotes
1. ^The Famine Early Warning System, accessed on June 25 2023, reveals high food insecurity indices in Guatemala, Honduras, and Nicaragua (see fews.net).
References
Abeldaño Zuñiga, R., Lima, G., and González Villoria, A. (2021). Impact of slow-onset events related to Climate Change on food security in Latin America and the Caribbean. Curr. Opin. Environ. Sustain. 50, 215–224. doi: 10.1016/j.cosust.2021.04.011
ACICAFOC (2009). Guía adaptada, manual forestal. p. 76. Available online at: https://www.acicafoc.org/publicaciones/#2009 (accessed April 17, 2023).
Acuña, F., Cortés, J., Garese, A., and González-Muñoz, R. (2020). The sea anemone Exaiptasia diaphana (Actiniaria: Aiptasiidae) associated to rhodoliths at Isla del Coco National Park, Costa Rica. Rev. Biol. Trop. 68, 7. doi: 10.15517/rbt.v68iS1.41191
Agache, I., Sampath, V., Aguilera, J., Akdis, C. A., Akdis, M., Barry, M., et al. (2022). Climate change and global health: A call to more research and more action. Allergy. 77, 1389–1407. doi: 10.1111/all.15229
Aide, T. M., and Grau, H. R. (2004). Ecology, globalization, migration, and Latin American ecosystems. Science. 305, 1915–1916. doi: 10.1126/science.1103179
Alatorre, J., and Sepúlveda, F. (2022). Impactos macroeconómicos del cambio climático en América Latina y el Caribe: revisión de la literatura, 2010-2021. Santiago, Chile. Available online at: https://repositorio.cepal.org/bitstream/handle/11362/48524/1/S2200758_es.pdf
Alfaro, E., Perez Briceño, P., Hidalgo, H., Gotlieb, Y., García, J., Rodríguez, J., et al. (2020). “Riesgos hidrometeorológicos en el Corredor Seco Centroamericano: Investigación, acción social y docencia dentro del Espacio de Estudios Avanzados de la Universidad de Costa Rica,” in Cambio Climático y Riesgos Hidrometeorológicos, eds. F. Polioptro, B. Martínez, C. Patiño, 3–29. Available online at: https://www.udlap.mx/catedraunesco/libro-cambio-climatico-capitulos.aspx#2 (accessed September 27, 2023).
Alfaro-Córdoba, M., Hidalgo, H., and Alfaro, E. (2020). Aridity trends in Central America: a spatial correlation analysis. Atmosphere 11, 13. doi: 10.3390/atmos11040427
Allen, T. R., Crawford, T., Montz, B., Whitehead, J., Lovelace, S., Hanks, A. D., et al. (2019). Linking water infrastructure, public health, and sea level rise: integrated assessment of flood resilience in coastal cities. Public Work Manag. Policy. 24, 380. doi: 10.1177/1087724X18798380
Almazroui, M., Islam, N., Saeed, F., Saeed, S., Ismail, M., Ehsan, M., et al. (2021). Projected changes in temperature and precipitation over the United States, Central America, and the Caribbean in CMIP6 GCMs. Earth Syst. Environ. 5, 1–24. doi: 10.1007/s41748-021-00199-5
Amador, J. (1998). A climatic feature of the tropical Americas: the trade wind easterly jet. Tópicos Meteorológicos y Oceanográficos 5, 91–102.
Amador, J., Alfaro, E., Lizano, O., and Magaña, V. (2006). Atmospheric forcing in the Eastern Tropical Pacific: a review. Progress Oceanogr. 69, 101–142. doi: 10.1016/j.pocean.2006.03.007
Amador, J. A. (2008). The Intra-Americas Seas Low-Level Jet (IALLJ): overview and future research. Annals N. Y Acad. Sci. 1146, 153–188. doi: 10.1196/annals.1446.012
Anchukaitis, K. J., and Evans, M. N. (2010). Tropical cloud forest climate variability and the demise of the Monteverde golden toad. Proc. Natl. Acad. Sci. U S A. 107, 5036–5040. doi: 10.1073/pnas.0908572107
Arciniega-Esparza, S., and Birkel, C. (2020). Hydrological simulations for Costa Rica from 1985 to 2019 using HYPE CR 1, 0. zenodo.
Avedaño, C., Hervas, A., and Rojas, O. (2019). Effects of land use on water quality in first-order streams across tropical rainforest, milpa agriculture, and oil palm farms in the lachua ecoregion, guatemala: preliminary results. Working Paper. Toronto.
Baez, J., Caruso, G., Mueller, V., and Niu, C. (2017). Heat exposure and youth migration in Central America and the Caribbean. Am. Econ. Rev. 107, 446–450. doi: 10.1257/aer.p20171053
Bakhtsiyarava, M., Schinasi, L. H., Sánchez, B. N., Dronova, I., Kephart, J. L., Ju, Y., et al. (2023). Modification of temperature-related human mortality by area-level socioeconomic and demographic characteristics in Latin American cities. Soc. Sci. Med. 317, 115526. doi: 10.1016/j.socscimed.2022.115526
Balsari, S., Dresser, C., and Leaning, J. (2020). Climate change, migration, and civil strife. Curr. Environ. Heal. Rep. 7, 404–414. doi: 10.1007/s40572-020-00291-4
Bárcena Ibarra, A., Samaniego, J., Peres, W., and Alatorre, J. (2018). The climate emergency in Latin America and the Caribbean: The path ahead - resignation or action? Santiago, Chile. Available online at: https://repositorio.cepal.org/handle/11362/45678
Barragan-Jason, G., de Mazancourt, C., Parmesan, C., Singer, M. C., and Loreau, M. (2022). Human–nature connectedness as a pathway to sustainability: A global meta-analysis. Conserv. Lett. 15, e12852. doi: 10.1111/conl.12852
Baumann, M. D., Zimmerer, K. S., and van Etten, J. (2020). Participatory seed projects and agroecological landscape knowledge in Central America. Int. J. Agric. Sustain. 18, 300–318. doi: 10.1080/14735903.2020.1775930
BCIE (2020). Estrategia Institucional BCIE, 2020-2024. Tegucigalpa: BCIE, 35. Available online at: https://www.bcie.org/acerca-del-bcie/estrategia-institucional (accessed July 14, 2023).
Bello, O., and Peralta, L. (2021). Evaluación de los efectos e impactos de las depresiones tropicales Eta y Iota en Guatemala. Comisión Económica para América Latina y el Caribe (CEPAL). Available online at: https://repositorio.cepal.org/bitstream/handle/11362/46681/S2100038_es.pdf?sequence=1&isAllowed=y (accessed April 15, 2023).
Berrang-Ford, L., Siders, A. R., Lesnikowski, A., Fischer, A. P., Callaghan, M. W., Haddaway, N. R., et al. (2021). A systematic global stocktake of evidence on human adaptation to climate change. Nat. Clim. Chang. 11, 989–1000. doi: 10.1038/s41558-021-01170-y
Caminade, C., McIntyre, M., and Jones, A. (2019). Impact of recent and future climate change on vector-borne diseases. Ann. NY Acad. Sci. 1436, 17. doi: 10.1111/nyas.13950
Castellanos, E., Lemos, M., Astigarraga, L., Chacón, N., Cuvi, N., Huggel, C., et al. (2022). “Chapter 12: Central and South America,” in Climate Change 2022: Impacts, Adaptation, and Vulnerability Contribution of Working Group II to the Sixth Assessment Report of the Intergovernmental Panel on Climate Change, eds. H.-O. Pörtner, D. C. Roberts, M. Tignor, E.S. Poloczanska, K. Mintenbeck, A. Alegría, et al. (Cambridge: Cambridge University Press), 1689–816. doi: 10.1017/9781009325844.014
Castellanos, E. J. (2022). Central America in dire need of inclusive climate resilient development with support from the international community. PLoS Clim. 1, 1–3. doi: 10.1371/journal.pclm.0000105
CATHALAC (2008). Poblados susceptibles a inundaciones en Costa Rica y Panamá. 32. Available online at: https://reliefweb.int/map/costa-rica/poblados-susceptibles-inundaciones-en-costa-rica-y-panamá-generado-po-cathalac-25
Cattaneo, C., Beine, M., Fröhlich, C. J., Kniveton, D., Martinez-Zarzoso, I., Mastrorillo, M., et al. (2019). Human migration in the era of climate change. Rev. Environ. Econ. Policy. 13, 8. doi: 10.1093/reep/rez008
Caviedes, V., Arenas-Granados, P., and Barragán-Muñoz, J. M. (2020). Regional public policy for integrated coastal zone management in Central America. Ocean Coast. Manag. 186, 105114. doi: 10.1016/j.ocecoaman.2020.105114
Centella-Artola, A., Bezanilla-Morlot, A., Taylor, M. A., Herrera, D. A., Martinez-Castro, D., Gouirand, I., et al. (2020). Evaluation of sixteen gridded precipitation datasets over the Caribbean region using gauge observations. Atmosphere (Basel). 11, 1334. doi: 10.3390/atmos11121334
Center for New Economy (2015). Annual report. Available online at: https://grupocne.org/2019/11/14/cne-2017-2018-annual-report/
CEPAL (2015). Climate Change in Central America, Potential Impacts and Public Policy Options. México City: ECLAC. 176. Available online at: https://repositorio.cepal.org/bitstream/handle/11362/39150/S1800827_~en.pdf?sequence=7andisAllowed=y
CEPAL (2021). Estadísticas del subsector eléctrico de los países del Sistema de la Integración Centroamericana (SICA), 2019 y avances a 2020. México City. 148. Available online at: https://repositorio.cepal.org/handle/11362/47019
Chinchilla, G., Gutiérrez, J., and Zárate, E. (2017). Análisis sinóptico de casos de eventos extremos de lluvia asociados a líneas de cortante de empujes fríos que han llegado a Costa Rica en el periodo invernal. Tópicos Meteorológicos y Oceanográficos 16, 5–18.
CICA (2015). Estrategia regional del CICA cambio climático y pueblos indígenas en centroamerica. San Salvador; 51. Available online at: https://www.consejocica.org/download/estrategia-regional-del-cica-cambio-climatico-y-pueblos-indigenas-en-centroamerica/
CMAR (2019). Plan de Acción Corredor Marino del Pacífico Este Tropical (CMAR) 2019-2024. 46. Available online at: https://www.cmarpacifico.org/biblioteca/publicaciones/plan-de-accion-corredor-marino-del-pacifico-este-tropical-cmar-2019-2024
Contreras, R., and Cuba, K. (2008). The potential impact of climate change on the energy sector in the Caribbean region. Department of Sustainable Development Organization of American States; 17. Available online at: https://www.oas.org/dsd/Documents/Effects_of_Climate_on_Energy_DSD_Energy_Division.pdf
Corwin, D. (2021). Climate change impacts on soil salinity in agricultural areas. Eur. J. Soil Sci. 72, 842–862. doi: 10.1111/ejss.13010
de Coninck, H., Revi, A., Babiker, M., Bertoldi, P., Buckeridge, M., Cartwright, A., et al. (2018). “Strengthening and implementing the global response,” in Global Warming of 1.5°C. An IPCC Special Report on the Impacts of Global Warming of 1.5°C Above Pre-Industrial Levels and Related Global Greenhouse Gas Emission Pathways, in the Context of Strengthening the Global Response to the Threat of Climate Change, Sustainable Development, and Efforts to Eradicate Poverty, eds V. Masson-Delmotte, P. Zhai, H.-O. Pörtner, D. Roberts, J. Skea, P. R. Shukla, et al. (Cambridge; New York, NY: Cambridge University Press), 313–444. doi: 10.1017/9781009157940.006
de Jaramillo, E., Trigo, E., and Campos, R. (2023). “The Role of Science, Technology and Innovation for Transforming Food Systems in Latin America and the Caribbean,” in Science and Innovations for Food Systems Transformation, eds. J. von Braun, K. Afsana, L. O. Fresco, M. H. A. Hassan (Cham: Springer International Publishing), 737–49. doi: 10.1007/978-3-031-15703-5_38
de Lacerda, L. D., Borges, R., and Ferreira, A. C. (2019). Neotropical mangroves: Conservation and sustainable use in a scenario of global climate change. Aquat. Conserv. 29, 1347–1364. doi: 10.1002/aqc.3119
de Sousa, K., Casanoves, F., Sellare, J., Ospina, A., Suchini, J. G., Aguilar, A., et al. (2018). How climate awareness influences farmers' adaptation decisions in Central America? J. Rural Stud. 64, 11–19. doi: 10.1016/j.jrurstud.2018.09.018
del Valle, A., Eriksson, M., Ishizawa, O. A., and Miranda, J. J. (2020). Mangroves protect coastal economic activity from hurricanes. Proc. Natl. Acad. Sci. 117, 265–270. doi: 10.1073/pnas.1911617116
Demerutis, J. Á., and Vicuña, M. (2022). “Urban and regional planning in Latin America and the Caribbean,” in The Routledge Handbook of Urban Studies in Latin America and the Caribbean: Cities, Urban Processes, and Policies. doi: 10.4324/9781003132622-18
Depsky, N., and Pons, D. (2020). Meteorological droughts are projected to worsen in Central America's dry corridor throughout the 21st century. Environ. Res. Lett. 16, 14001. doi: 10.1088/1748-9326/abc5e2
Díaz Bolaños, R. E. (2019). El Corredor Seco Centroamericano en perspectiva histórica. Anu. Estud. Centroam. 45, 26. doi: 10.15517/aeca.v45i0.40697
Donatti, C., Harvey, C., Martinez-Rodriguez, R., Vignola, R., and Rodriguez, C. (2019). Vulnerability of smallholder farmers to climate change in Central America and Mexico: current knowledge and research gaps. Clim. Dev. 11, 264–286. doi: 10.1080/17565529.2018.1442796
Durán-Quesada, A., Sorí, R., Ordoñez, P., and Gimeno, L. (2020). Climate perspectives in the intra–Americas seas. Atmosphere (Basel). 11, 32. doi: 10.3390/atmos11090959
Eckstein, D., Künzel, V., and Schäfer, L. (2021). The Global Climate Risk Index 2021 Germanwatch e.V. Berlin. Available online at: https://reliefweb.int/report/world/global-climate-risk-index-2021?gclid=Cj0KCQjw3a2iBhCFARIsAD4jQB2S6LwwGy67v4HPb3y9PX4f3_n4awubybss54NckUZmNG5aEfzGQGIaArH6EALw_wcB
ECLAC (2010). The Economics of Climate Change in Central America: Summary 2010. México City: ECLAC 146. Available online at: https://repositorio.cepal.org/bitstream/handle/11362/35229/lcmexl978i.pdf?sequence=1andisAllowed=y
ECLAC/FAO (2018). Atlas of migration in Northern Central America. Santiago, Chile. 45. Available online at: https://repositorio.cepal.org/bitstream/handle/11362/44288/1/S1801071_en.pdf
Enfield, D. B., and Alfaro, E. J. (1999). The dependence of Caribbean rainfall on the interaction of the tropical Atlantic and Pacific Oceans. J. Clim. 12, 2093–2103. doi: 10.1175/1520-0442(1999)012<2093:TDOCRO>2.0.CO;2
Enfield, D. B., Mestas-Nunez, A. M., and Trimble, P. J. (2001). The Atlantic multidecadal oscillation and its relationship to rainfall and river flows in the continental U.S. Geophys. Res. Lett. 28, 2077–2080. doi: 10.1029/2000GL012745
European Commission (2019). Progress on Climate Action in Latin America: Nationally Determined Contributions as of 2019 Brussels (p. 180). EUROCLIMA+ Programme, DG for International Development and Cooperation - EiropeAId, European Commission. Available online at: https://data.europa.eu/doi/10.2841/318319 (accessed April 15, 2023).
Ewbank, R., Perez, C., Cornish, H., Worku, M., and Woldetsadik, S. (2019). Building resilience to El Niño-related drought: experiences in early warning and early action from Nicaragua and Ethiopia. Disasters. 43, S345–S367. doi: 10.1111/disa.12340
Fallas-López, B., and Alfaro, E. (2012). Uso de herramientas estadísticas para la predicción estacional del campo de precipitación en América Central como apoyo a los Foros Climáticos Regionales. 1: análisis de tablas de contingencia. Revista Climatol. 12, 61–79.
FAO (2019). The State of Food and Agriculture: Moving Forward on Food Loss and Waste Reduction. The State of the World. Available online at: https://www.fao.org/3/ca6030en/ca6030en.pdf
Feeley, K. J., Hurtado, J., Saatchi, S., Silman, M. R., and Clark, D. B. (2013). Compositional shifts in Costa Rican forests due to climate-driven species migrations. Glob. Chang. Biol. 19, 3472–3480. doi: 10.1111/gcb.12300
Fernández, W., Chacón, R. E., and Melgarejo, J. W. (1996). On the rainfall distribution with altitude over Costa Rica. Rev. Geofísica 44, 57–72.
Froehlich, H. E., Koehn, J. Z., Holsman, K. K., and Halpern, B. S. (2022). Emerging trends in science and news of climate change threats to and adaptation of aquaculture. Aquaculture 549:737812.
Funk, C., Peterson, P., Landsfeld, M., Pedreros, D., Verdin, J., Shukla, S., et al. (2015). The climate hazards infrared precipitation with stations - A new environmental record for monitoring extremes. Sci. Data. 2, 21. doi: 10.1038/sdata.2015.66
García-Franco, J. L., Chadwick, R., Gray, L. J., Osprey, S., and Adams, D. K. (2023). Revisiting mechanisms of the mesoamerican midsummer drought. Clim. Dyn. 60, 549–569. doi: 10.1007/s00382-022-06338-6
García-Solera, I., and Ramirez, P. (2012). “Central America's seasonal climate outlook forum,” The Climate Services Partnership. 8.
Garro-Quesada, M., Del Vargas Leiva, M., Girot, P., and Quesada-Román, A. (2023). Climate risk analysis using a high-resolution spatial model in costa rica. Climate. 11, 1–17. doi: 10.3390/cli11060127
Gencer, E. A. (2013). An overview of urban vulnerability to natural disasters and climate change in Central America and The Caribbean region. SSRN Electron J. FEEM Working Paper. doi: 10.2139/ssrn.2334068
GFLAC (2023). Índice de Finanzas Sostenibles. Available online at: https://www.sustainablefinance4future.org/indice-de-finanzas-sostenibles (accessed April 16, 2023).
Gill, A. E. (1980). Some simple solutions for heat-induced tropical circulation. Q. J. R Meteorol. Soc. 106, 447–462.
Gotlieb, Y., and García Girón, J. (2020). The role of land use conversion in shaping the land cover of the Central American Dry Corridor. Land Use Policy. 94, 104351. doi: 10.1016/j.landusepol.2019.104351
Gotlieb, Y., Pérez-Briseño, P., Hidalgo, H., and Alfaro, E. (2019). The Central American Dry Corridor: a consensus statement and its background. “Yu'am” Rev. Mesoam. Biodivers. y Cambio. Climático. 3, 42–51. Available online at: https://www.revistayuam.com/the-central-american-dry-corridor-a-consensus-statement-and-its-background/ (accessed September 27, 2023).
Grüter, R., Trachsel, T., Laube, P., and Jaisli, I. (2022). Expected global suitability of coffee, cashew and avocado due to climate change. PLoS ONE. 17, 24. doi: 10.1371/journal.pone.0261976
Guillén Bolaños, T., Máñez Costa, M., and Nehren, U. (2019). “Development of a prioritization tool for climate change adaptation measures in the forestry Sector-A Nicaraguan case study,” in Economic Tools and Methods for the Analysis of Global Change Impacts on Agriculture and Food Security (Switzerland AG: Springer Nature), 165–77. doi: 10.1007/978-3-319-99462-8_10
Guillén Bolaños, T., Scheffran, J., and Costa, M. M. (2022). Climate adaptation and successful adaptation definitions: Latin American perspectives using the delphi method. Sustainability. 14, 5350. doi: 10.3390/su14095350
Guzmán, S., Barbosa, O., Montalvo, V., and Álvarez, F. (2022). Índice de Finanzas Sostenibles. Informe de resultados del Índice de Finanzas Sostenibles para América Latina y el Caribe 2022 (con datos a 2021). Una mirada al estado de las finanzas sostenibles en la región. México City: Grupo de Financiamiento Climático para Latinoamérica y el Caribe (GFLAC). Available online at: https://www.sustainablefinance4future.org/_files/ugd/32948d_ac13981ba67d448c98a571e1b3a8e3c2.pdf
Guzmán, S., Moncada, A., Canales, N., Castillo, M., and Guillén, T. (2017). “Toward climate finance reporting systems in Latin America,” in Toward Implementation The 2017 AdaptationWatch Report, eds. K. Adams, D. Fazon (Stockholm: Stockholm Environment Institute), 71.
Hagen, I., Huggel, C., Ramajo, L., Chacón, N., Ometto, J. P., Postigo, J. C., et al. (2022). Climate change-related risks and adaptation potential in Central and South America during the 21st century. Environ. Res. Lett. 17, 33002. doi: 10.1088/1748-9326/ac5271
Hannah, L., Donatti, C., Harvey, C., Alfaro, E., Rodriguez, D., Bouroncle, C., et al. (2017). Regional modeling of climate change impacts on smallholder agriculture and ecosystems in Central America. Clim. Change. 141, 29–45. doi: 10.1007/s10584-016-1867-y
Hardoy, J., and Pandiella, G. (2009). Urban poverty and vulnerability to climate change in Latin America. Environ. Urban. 21, 3019. doi: 10.1177/0956247809103019
Harvey, C., Saborio-Rodríguez, M., Martinez-Rodríguez, R., Viguera, B., Chain-Guadarrama, A., Vignola, R., et al. (2018). Climate change impacts and adaptation among smallholder farmers in Central America. Agric. Food Secur. 7, 57. doi: 10.1186/s40066-018-0209-x
Hidalgo, H. (2021). Climate variability and change in Central America: what does it mean for water managers? Front. Water. 2, 632739. doi: 10.3389/frwa.2020.632739
Hidalgo, H., and Alfaro, E. (2012). Some physical and socio-economic aspects of climate change in Central America. Progr. Phys. Geogr. 36, 380–399. doi: 10.1177/0309133312438906
Hidalgo, H., and Alfaro, E. (2015). Skill of CMIP5 climate models in reproducing 20th century basic climate features in Central America. Int. J. Climatol. 35, 3397–3421. doi: 10.1002/joc.4216
Hidalgo, H., Alfaro, E., Amador, J., and Bastidas, Á. (2019). Precursors of quasi-decadal dry-spells in the Central America Dry Corridor. Clim. Dyn. 53, 1307–1322. doi: 10.1007/s00382-019-04638-y
Hidalgo, H., Alfaro, E., and Pérez-Briceño, P. (2021). Projected climate changes of CMIP5 models in La Cruz, Guanacaste, Costa Rica. Rev. Biol. Trop. 69, 60–73. doi: 10.15517/rbt.v69iSuppl.2.48307
Hidalgo, H., Alfaro, E., and Quesada, A. (2022). Future Changes in Simulated Streamflow in Costa Rica from CMIP6 climate models. 16. Available online at: https://www.kerwa.ucr.ac.cr/handle/10669/86843 (accessed September 27, 2023).
Hidalgo, H., Alfaro, E., and Quesada-Montano, B. (2017). Observed (1970–1999) climate variability in Central America using a high-resolution meteorological dataset with implication to climate change studies. Clim. Change. 141, 13–28. doi: 10.1007/s10584-016-1786-y
Hidalgo, H., Amador, J., Alfaro, E., and Quesada, B. (2013). Hydrological climate change projections for Central America. J. Hydrol. 495, 94–112. doi: 10.1016/j.jhydrol.2013.05.004
Hidalgo, H., Durán-Quesada, A., Amador, J., and Alfaro, E. (2015). The Caribbean low-level jet, the inter-tropical convergence zone and precipitation patterns in the intra-americas sea: a proposed dynamical mechanism. Geogr. Ann. Ser. A, Phys. Geogr. 97, 41–59. doi: 10.1111/geoa.12085
Hidalgo, H. G., Alfaro, E. J., and Quesada-Román, A. (2023). Flood projections for selected Costa Rican main basins using CMIP6 climate models downscaled output in the HBV hydrological model for scenario SSP5-8.5. Hydrol. Res. Lett.
Hoegh-Guldberg, O., Jacob, D., Taylor, M., Bindi, M., Brown, S., Camilloni, I., et al. (2018). “Impacts of 1.5°C global warming on natural and human systems,” in Global Warming of 15°C an IPCC special report on the impacts of global warming of 15°C above pre-industrial levels and related global greenhouse gas emission pathways, in the context of strengthening the global response to the threat of climate change (Cambridge, UK, and New York, NY, USA: Cambridge University Press), 138. Available online at: http://report.ipcc.ch/sr15/pdf/sr15_chapter3.pdf (accessed October 29, 2018).
Hoegh-Guldberg, O., Jacob, D., Taylor, M., Guillén Bolaños, T., Bindi, M., Brown, S., et al. (2019). The human imperative of stabilizing global climate change at 1.5°C. Science 365, 6874. doi: 10.1126/science.aaw6974
Hoffmann, R., Dimitrova, A., Muttarak, R., Crespo Cuaresma, J., and Peisker, J. A. (2020). meta-analysis of country-level studies on environmental change and migration. Nat. Clim. Chang. 10, 904–912. doi: 10.1038/s41558-020-0898-6
ICSF (2016). Small-Scale Fishing in Central American Indigenous People: Governance, Tenure and Sustainable Management of Marine Resources. San José: ICSF. Available online at: https://www.fao.org/family-farming/detail/en/c/1025686/
Imbach, P., Beardsley, M., Bouroncle, C., Medellin, C., Läderach, P., Hidalgo, H., et al. (2017). Climate change, ecosystems and smallholder agriculture in Central America: an introduction to the special issue. Clim. Change. 141, 1–12. doi: 10.1007/s10584-017-1920-5
Immerzeel, W., Lutz, A., Andrade, M., Bahl, A., Biemans, H., Bolch, T., et al. (2020). Importance and vulnerability of the world's water towers. Nature. 577, 364–369. doi: 10.1038/s41586-019-1822-y
Internal Displaced Monitoring Center (IMDC) (2023). Global Report on Internal Displacement. Oslo: IDMC/Norwegian Council on Refugees.
IPCC (2018). “Global Warming of 1.5°C,” in An IPCC Special Report on the Impacts of Global Warming of 1.5°C Above Pre-Industrial Levels and Related Global Greenhouse Gas Emission Pathways, in the Context of Strengthening the Global Response to the Threat of Climate Change, Sustainable Development, and Efforts to Eradicate Poverty, eds V. Masson-Delmotte, P. Zhai, H.-O. Pörtner, D. Roberts, J. Skea, P. R. Shukla, A. Pirani, et al. (Cambridge; New York, NY: Cambridge University Press), 616. doi: 10.1017/9781009157940
IPCC (2021). “Summary for policymakers,” in Climate Change 2021: The Physical Science Basis. Contribution of Working Group I to the Sixth Assessment Report of the Intergovernmental Panel on Climate Change, eds V. Masson-Delmotte, P. Zhai, S. Piran, C. Connors, S. Péan, N. Berger, et al. (Cambridge; New York, NY: Cambridge University Press), 3–32. doi: 10.1017/9781009157896.001
IPCC (2022). “Climate change 2022: impacts, adaptation and vulnerability,” in Contribution of Working Group II to the Sixth Assessment Report of the Intergovernmental Panel on Climate Change, eds H.-O. Pörtner, D. C. Roberts, M. Tignor, E. S. Poloczanska, K. Mintenbeck, A. Alegría, et al. (Cambridge; New York, NY: Cambridge University Press), 3056. doi: 10.1017/9781009325844
IPCC (2023). “Summary for policy makers,” in Climate Change 2023: Synthesis Report.Contribution of Working Groups I, II and III to the Sixth Assessment Report of the Intergovernmental Panel on Climate Change, eds Core Writing Team, H. Lee, and J. Romero (Geneva: IPCC), 1–34. doi: 10.59327/IPCC/AR6-9789291691647.001
Jaramillo, S., Graterol, E., and Pulver, E. (2020). Sustainable transformation of rainfed to irrigated agriculture through water harvesting and smart crop management practices. Front. Sustain. Food Syst. 4, 437086. doi: 10.3389/fsufs.2020.437086
Jat, M., Dagar, J., Sapkota, T., Yadvinder-Singh, Govaerts, B., Ridaura, S., et al. (2016). “Chapter Three - Climate Change and Agriculture: Adaptation Strategies and Mitigation Opportunities for Food Security in South Asia and Latin America,” in Advances in Agronomy, ed. D. L. Sparks (London: Academic Press), 127–235. doi: 10.1016/bs.agron.2015.12.005
Kaczan, D. J., and Orgill-Meyer, J. (2020). The impact of climate change on migration: a synthesis of recent empirical insights. Clim. Change. 158, 281–300. doi: 10.1007/s10584-019-02560-0
Kaenzig, R., and Piguet, E. (2014). “Migration and climate change in Latin America and the Caribbean,” in People on the Move in a Changing Climate: The Regional Impact of Environmental Change on Migration, eds. E. Piguet, and F. Laczko (Dordrecht: Springer Netherlands), 155–76. doi: 10.1007/978-94-007-6985-4_7
Kappelle, M., and Horn, S. (2007). Páramos de Costa Rica. 95–7. Available online at: https://www.researchgate.net/publication/262129765_Paramos_de_Costa_Rica_by_Maarten_Kappelle_Sally_P_Horn
Keller, D., Lenton, A., Scott, V., Vaughan, N., Bauer, N., Ji, D., et al. (2018). The carbon dioxide removal model intercomparison project (CDRMIP): rationale and experimental protocol for CMIP6. Geosci. Model Dev. 11, 1133–1160. doi: 10.5194/gmd-11-1133-2018
Kjellstrom, T., and Crowe, J. (2011). Climate change, workplace heat exposure, and occupational health and productivity in Central America. Int. J. Occup. Environ. Health. 17, 270–281. doi: 10.1179/oeh.2011.17.3.270
Läderach, P., Kommerell, V., Schapendonk, F., Van Loon, J., Martinez-Baron, D., Castellanos, A, et al. (2021). “Climate Security in the Central American Dry Corridor,” in CGIAR. Available online at: https://hdl.handle.net/10568/116948 (accessed April 15, 2023).
LEDS LAC (2023). LEDSenLAC 2022: Hacia un desarrollo bajo en emisiones y resiliente al clima en Latinoamérica y el Caribe: Avances en las estrategias nacionales. (p. 46). LEDS LAC. https://www.ledslac.org/wpcontent/uploads/2023/04/Reporte-anual-LEDSenLAC-2022_c-2.pdf (accessed April 15, 2023)
Lelieveld, J., Klingmüller, K., Pozzer, A., Burnett, R., Haines, A., Ramanathan, V., et al. (2019). Effects of fossil fuel and total anthropogenic emission removal on public health and climate. Proc. Natl. Acad. Sci. 116, 7192–7197. doi: 10.1073/pnas.1819989116
Ley, D. (2017). “Sustainable Development, Climate Change, and Renewable Energy in Rural Central America,” in Evaluating Climate Change Action for Sustainable Development eds. J. I. Uitto, J. Puri, R. D. van den Berg (Cham: Springer International Publishing), 187–212. doi: 10.1007/978-3-319-43702-6_11
Liu, X., Liu, W., Tang, Q., Liu, B., Wada, Y., Yang, H., et al. (2022). Global agricultural water scarcity assessment incorporating blue and green water availability under future climate change. Earth's Futur. 10, 16. doi: 10.1029/2021EF002567
Lizano Araya, M., and Lizano Rodríguez, O. G. (2022). Escenarios de inundación ante el aumento del nivel del mar por Cambio Climático, para las Playas del Coco, Tamarindo y Sámara, Costa Rica. Entorno. Geográfico. 29, e21411903. doi: 10.25100/eg.v0i25.11903
Lizano Araya, M. A., and Lizano Rodríguez, O. G. (2020). Escenarios ante el aumento del nivel del mar por cambio climático para la localidad del Cocal, Puntarenas, Costa Rica. GeoFocus Int. Rev. Geogr. Inf. Sci. Technol. 26, 3–20. doi: 10.21138/GF.656
Lizano, M., and Lizano, O. (2010). Creación de escenarios de inundación en la Ciudad de Puntarenas ante el aumento del nivel del mar. InterSedes 11, 215–229. Available online at: https://revistas.ucr.ac.cr/index.php/intersedes/article/view/1010 (accessed September 27, 2023).
Lizano, O. (2013). Erosión en las playas de Costa Rica, incluyendo la Isla del Coco. InterSedes. 14, 24. doi: 10.15517/isucr.v14i27.10405
Lizano-Araya, M., and Lizano-Rodríguez, O. (2021). Creación de escenarios ante el aumento del nivel del mar, para las localidades de Moín y Cahuita, Limón, Costa Rica. Rev. Geográfica. Am. Cent. 1, 15031. doi: 10.15359/rgac.68-1.4
Lyra, A., Imbach, P., Rodriguez, D., Chou, S. C., Georgiou, S., Garofolo, L., et al. (2017). Projections of climate change impacts on Central America tropical rainforest. Clim. Change. 141, 93–105. doi: 10.1007/s10584-016-1790-2
Maestro, M., Pérez-Cayeiro, M. L., Chica-Ruiz, J. A., and Reyes, H. (2019). Marine protected areas in the 21st century: Current situation and trends. Ocean Coast Manag. 171, 28–36. doi: 10.1016/j.ocecoaman.2019.01.008
Magaña, V., Amador, J. A., and Medina, S. (1999). The mid-summer drought over México and Central America. J. Clim. 12, 1577–1588. doi: 10.1175/1520-0442(1999)012%3C1577:TMDOMA%3E2.0.CO;2
Mahlknecht, J., González-Bravo, R., and Loge, F. J. (2020). Water-energy-food security: A Nexus perspective of the current situation in Latin America and the Caribbean. Energy. 194, 116824. doi: 10.1016/j.energy.2019.116824
Maldonado, T., Alfaro, E., and Hidalgo, H. A. (2018). review of the main drivers and variability of Central America's Climate and seasonal forecast systems. Rev. Biol. Trop. 66, S153–S175. doi: 10.15517/rbt.v66i1.33294
Maldonado, T., Rutgersson, A., Alfaro, E., Amador, J., and Claremar, B. (2016). Interannual variability of the midsummer drought in Central America and the connection with sea surface temperatures. Adv. Geosci. 42, 35–50. doi: 10.5194/adgeo-42-35-2016
Manes, S., Costello, M. J., Beckett, H., Debnath, A., Devenish-Nelson, E., Grey, K.-A., et al. (2021). Endemism increases species' climate change risk in areas of global biodiversity importance. Biol. Conserv. 257, 109070. doi: 10.1016/j.biocon.2021.109070
Marcillo, C. E., Prado, G. G., Copeland, N., and Krometis, L. H. (2020). Drinking water quality and consumer perceptions at the point-of-use in san Rafael las Flores, Guatemala. Water Pract. Technol. 15, 25. doi: 10.2166/wpt.2020.025
Menéndez, P., Losada, I. J., Torres-Ortega, S., Narayan, S., and Beck, M. W. (2020). The global flood protection benefits of mangroves. Sci. Rep. 10, 4404. doi: 10.1038/s41598-020-61136-6
Meza-Herrera, C., Navarrete-Molina, C., Luna-García, L., Pérez-Marín, C., Altamirano-Cárdenas, J., Macías-Cruz, U., et al. (2022). Small ruminants and sustainability in Latin America and the Caribbean: Regionalization, main production systems, and a combined productive, socio-economic and ecological footprint quantification. Small Rumin. Res. 211, 106676. doi: 10.1016/j.smallrumres.2022.106676
MIDEPLAN (2019). COSTA RICA: Impacto de los Fenómenos Naturales para el período 1988-2018, por sectores, provincias, cantones y distritos. San José. Available online at: https://www.mideplan.go.cr/node/1825
Miranda, M., Porras, I., and Moreno, M. (2003). The social impacts of payments for environmental services in Costa Rica. A quantitative field survey and analysis of the Virilla watershed. IIED, 75. Available online at: https://iied.org/sites/default/files/pdfs/migrate/9245IIED.pdf (accessed September 27, 2023).
Miranda-Chacón, Z., Alfaro, E., Hidalgo, H., Arguedas, A., and Rivera-Chavarría, L. (2023). Policy brief for Costa Rica 2023 The Lancet Countdown on Health and Climate Change. 8. Available online at: https://www.dropbox.com/s/p7vbmc3p30mun4b/2023 Costa Rica Lancet Countdown policy~brief_EN.pdf?dl=0 (accessed September 27, 2023).
Miranda-Chacón, Z., Rivera-Chavarría, A. L., Rivera-Bermúdez, G., Troyo, A., Hidalgo, H. G., and Alfaro, E. J. (2021). Policy brief for Costa Rica (Informe de Políticas para Costa Rica) 2021. The Lancet Countdown on Health and Climate Change. Available online at: https://www.lancetcountdown.org/resources/ (accessed September 27, 2023).
Montaña, E., Diaz, H. P., and Hurlbert, M. (2016). Development, local livelihoods, and vulnerabilities to global environmental change in the South American Dry Andes. Reg. Environ. Chang. 16, 2215–2228. doi: 10.1007/s10113-015-0888-9
Mora, N., Amador, J. A., Rivera, E. R., and Maldonado, T. (2020). A Sea Breeze Study during Ticosonde-NAME 2004 in the Central Pacific of Costa Rica: observations and numerical modeling. Atmosphere 11:1333.
Moreno, A. (2006). Climate change and human health in Latin America: drivers, effects, and policies. Reg. Environ. Chang. 6, 157–164. doi: 10.1007/s10113-006-0015-z
Moreno, J. M., Laguna-Defior, C., Barros, V., Calvo Buendía, E., Marengo, J. A., Oswald Spring, U., et al. (2020). “Adaptación frente a los riesgos del cambio climático en los países iberoamericanos,” in Informe RIOCCADAPT, eds J. M. Moreno and C. Laguna-Defior (Madrid: McGraw-Hill).
Moreno, M., Hidalgo, H., and Alfaro, E. (2019). Cambio climático y su posible efecto sobre los servicios ecosistémicos en dos parques nacionales de Costa Rica. Revibec. 30, 16–38. Available online at: https://raco.cat/index.php/Revibec/article/view/360178 (accessed September 27, 2023).
Muñoz, E., Busalacchi, A. J., Nigam, S., and Ruiz-Barradas, A. (2008). Winter and summer structure of the Caribbean Low-level Jet. J. Clim. 21, 1260–1276. doi: 10.1175/2007JCLI1855.1
Nagy, G. J., Gutiérrez, O., Brugnoli, E., Verocai, J. E., Gómez-Erache, M., Villamizar, A., et al. (2019). Climate vulnerability, impacts and adaptation in Central and South America coastal areas. Reg. Stud. Mar. Sci. 29, 100683. doi: 10.1016/j.rsma.2019.100683
Nalau, J., and Verrall, B. (2021). Mapping the evolution and current trends in climate change adaptation science. Clim. Risk Manag. 32, 100290. doi: 10.1016/j.crm.2021.100290
Nawrotzki, R. J., Hunter, L. M., Runfola, D. M., and Riosmena, F. (2015). Climate change as a migration driver from rural and urban Mexico. Environ. Res. Lett. 10, 114023. doi: 10.1088/1748-9326/10/11/114023
Neumann, V. A., and Hack, J. A. (2020). Methodology of policy assessment at the municipal level: costa rica's readiness for the implementation of nature-based-solutions for urban stormwater management. Sustainability. 12, 230. doi: 10.3390/su12010230
New, M., Reckien, D., Viner, D., Adler, C., Cheong, S. M., Conde, C., et al. (2022). “Decision-making options for managing risk,” in Climate Change 2022: Impacts, Adaptation and Vulnerability. Contribution of Working Group II to the Sixth Assessment Report of the Intergovernmental Panel on Climate Change, eds H.-O. Pörtner, D. C. Roberts, M. Tignor, E. S. Poloczanska, K. Mintenbeck, A. Alegría, et al. (Cambridge; New York, NY: Cambridge University Press), 2539–654. doi: 10.1017/9781009325844.026
Ocko, I., and Hamburg, S. (2019). Climate impacts of hydropower: enormous differences among facilities and over time. Environ. Sci. Technol. 53, 14070–14082. doi: 10.1021/acs.est.9b05083
Ortiz, D. I., Piche-Ovares, M., Romero-Vega, L. M., Wagman, J., and Troyo, A. (2021). The impact of deforestation, urbanization, and changing land use patterns on the ecology of mosquito and tick-borne diseases in Central America. Insects. 13, 20. doi: 10.3390/insects13010020
Ortiz-Bobea, A., Ault, T. R., Carrillo, C. M., Chambers, R. G., and Lobell, D. B. (2021). Anthropogenic climate change has slowed global agricultural productivity growth. Nat. Clim. Chang. 11, 306–312. doi: 10.1038/s41558-021-01000-1
Parmesan, C., and Singer, M. C. (2022). Mosaics of climatic stress across species' ranges: tradeoffs cause adaptive evolution to limits of climatic tolerance. Philos. Trans. R Soc. London Ser. B, Biol. Sci. 377, 20210003. doi: 10.1098/rstb.2021.0003
Parmesan, C., and Yohe, G. (2003). A globally coherent fingerprint of climate change impacts across natural systems. Nature. 421, 37–42. doi: 10.1038/nature01286
Pascale, S., Kapnick, S., Delworth, T., Hidalgo, H., and Cooke, W. (2021). Natural variability vs forced signal in the 2015–2019 Central American drought. Clim. Change. 168, 21. doi: 10.1007/s10584-021-03228-4
Peña-Guzmán, C., Ulloa-Sánchez, S., Mora, K., Helena-Bustos, R., Lopez-Barrera, E., Alvarez, J., et al. (2019). Emerging pollutants in the urban water cycle in Latin America: A review of the current literature. J. Environ. Manage. 237, 408–423. doi: 10.1016/j.jenvman.2019.02.100
Perdigón-Morales, J., Romero-Centeno, R., Pérez, P. O., and Barrett, B. S. (2018). The midsummer drought in Mexico: perspectives on duration and intensity from the CHIRPS precipitation database. Int. J. Climatol 38, 2174–2186. doi: 10.1002/joc.5322
Pérez-Briceño, P. M., and Lizano Rodríguez, O. G. (2021). Coastal erosion and beach stability in Limón, Caribbean Sea, Costa Rica. Rev. Geográfica Chile. Terra Aust. 1, 96–110. doi: 10.23854/07199562.2021571esp.Lizano96
Pérez-Briseño, P. M., Alfaro, E., Hidalgo, H., and Jiménez, F. (2016). Distribución espacial de impactos de eventos hidrometeorológicos en América Central. Revista de Climatología 16, 63–75. Available online at: https://www.kerwa.ucr.ac.cr/handle/10669/74079 (accessed September 27, 2023).
Pons, D., Castellanos, E., Conde, D., Brincker, J., Incer, D., López, A., et al. (2018). Escenarios de aridez para Guatemala para los años 2030 2050 y 2070 utilizando modelos de cambio climático. Rev. Mesoam. Biodiv. y Cambio Climático. 4, 13. Available online at: https://www.revistayuam.com/escenarios-de-aridez-para-guatemala-para-los-anos-2030-2050-y-2070-utilizando-modelos-de-cambio-climatico/ (accessed September 27, 2023).
Pons, D., Muñoz, Á. G., Meléndez, L. M., Chocooj, M., Gómez, R., Chourio, X., et al. (2021). A coffee yield next-generation forecast system for rain-fed plantations: the case of the Samalá Watershed in Guatemala. Weather Forecast. 36, 2021–2038. doi: 10.1175/WAF-D-20-0133.1
Pörtner, H., Roberts, D. C., Poloczanska, E., Mintenbeck, K., Tignor, M., Alegría, A., et al. (2022). “Summary for Policymakers,” in Climate change 2022: Impacts, adaptation and vulnerability Contribution of Working Group II to the Sixth Assessment Report of the Intergovernmental Panel of Climate Change (IPCC), eds. H.-O. Pörtner, D. C. Roberts, M. Tignor, E. S. Poloczanska, K. Mintenbeck, A. Alegría, et al. (Cambridge, UK and New York, USA: Cambridge University Press). Available online at: https://www.ipcc.ch/report/ar6/wg2/#FullReport
Pörtner, H., Scholes, R. J., Arneth, A., Barnes, D. K. A., Burrows, M. T., Diamond, S. E., et al. (2023). Overcoming the coupled climate and biodiversity crises and their societal impacts. Science 380, eabl4881. doi: 10.1126/science.abl4881
Pörtner, H. O., Roberts, D. C., Adams, H., and Ibrahim, Z. Z. (2022). “Technical Summary,” in Climate change 2022: Impacts, adaptation and vulnerability Contribution of Working Group II to the Sixth Assessment Report of the Intergovernmental Panel of Climate Change (IPCC), eds. H.-O. Pörtner, D. C. Roberts, M. Tignor, E. S. Poloczanska, K. Mintenbeck, A. Alegría, et al. (Cambridge, UK and New York, USA: Cambridge University Press). Available online at: https://www.ipcc.ch/report/ar6/wg2/#FullReport
Programa Estado de la Nación (2013). Estado de la Nación en desarrollo humano sostenible. Pavas; 434. Available online at: https://repositorio.conare.ac.cr/handle/20.500.12337/895
Programa Estado de la Nación (2021). Sexto Estado de la Región, 71. p. Available online at: https://repositorio.conare.ac.cr/bitstream/handle/20.500.12337/8137/PEN_informe_estado_region_resumen_2021.pdf?sequence=7andisAllowed=y
Quesada, L., Calvo, O., Hidalgo, H., Pérez, P., and Alfaro, E. (2019). Dynamical delimitation of the Central American Dry Corridor (CADC) using drought indices and aridity values. Sage J. 43, 627–642. doi: 10.1177/0309133319860224
Quesada-Hernández, L. (2019). Respuesta de la hidrología superficial de la cuenca del río Tempisque a la variabilidad climática y cambio de cobertura de la tierra. Universidad de Costa Rica. Available online at: https://oacg.fcs.ucr.ac.cr/images/TFGs/Tesis_Luis_Quesada_Final.pdf
Quesada-Hernández, L., Hidalgo, H., and Alfaro, E. (2020). Asociación entre algunos índices de sequía e impactos socio-productivos en el Pacífico Norte de Costa Rica. Rev. Ciencias Ambient. 54, 17. doi: 10.15359/rca.54-1.2
Quesada-Montano, B., Wetterhall, F., Westerberg, I., Hidalgo, H., and Halldin, S. (2019). Characterising droughts in Central America with uncertain hydro-meteorological data. Theor. Appl. Climatol. 137, 14. doi: 10.1007/s00704-018-2730-z
Quesada-Román, A. (2022). Flood risk index development at the municipal level in Costa Rica: A methodological framework. Environmental Science and Policy. Environ. Sci. Policy. 133, 98–106. doi: 10.1016/j.envsci.2022.03.012
Quiros, E., and Hidalgo, H. G. (2016a). Interacción de la Zona de Convergencia Intertropical del Pacífico Este con Sistemas Tropicales. Tópicos Meteorológicos y Oceanográficos. 15, 33–47.
Quiros, E., and Hidalgo, H. G. (2016b). Variabilidad y conexiones climáticas de la zona de convergencia intertropical del Pacífico Este. Tópicos Meteorológicos y Oceanográficos 15, 21.
Ranasinghe, R., Ruane, A. C., Vautard, R., Arnell, N., Coppola, E., Cruz, F. A., et al. (2021). “Climate change information for regional impact and for risk assessment,” in Climate Change 2021: The Physical Science Basis Contribution of Working Group I to the Sixth Assessment Report of the Intergovernmental Panel on Climate Change, eds. V. Masson-Delmotte, P. Zhai, S. Piran, C. Connors, S. Péan, N. Berger, et al. (Cambridge, United Kingdom and New York, NY, USA: Cambridge University Press), 1767–1926.
Ray, D., West, P., Clark, M., Gerber, J., Prishchepov, A., Chatterjee, S., et al. (2019). Climate change has likely already affected global food production. PLoS ONE. 14, 18. doi: 10.1371/journal.pone.0217148
Romanello, M., McGushin, A., Di Napoli, C., Drummond, P., Hughes, N., Jamart, L., et al. (2021). The 2021 report of the Lancet Countdown on health and climate change: code red for a healthy future. Lancet. 398, 1619–1662. doi: 10.1016/S0140-6736(21)01787-6
Rosa, L. (2022). Adapting agriculture to climate change via sustainable irrigation: Biophysical potentials and feedbacks. Environ. Res. Lett. 17, 15. doi: 10.1088/1748-9326/ac7408
Sáenz, F., Hidalgo, H., Muñoz, Á., Alfaro, E., Amador, J., Vázquez-Aguirre, J., et al. (2023). Atmospheric circulation types controlling rainfall in the Central American Isthmus. Int. J. Climatol. 43, 22. doi: 10.1002/joc.7745
Sandoval, V., Sarmiento, P., Mazariegos, E., and Oviedo, D. (2020). Exploring network analysis for urban planning and disaster risk reduction in informal settlements: cases from Honduras, Jamaica, and Peru. Int. J. Disast. Resp. Emerg. Manage. 3, 16. doi: 10.4018/IJDREM.2020010103
Santamouris, M. (2020). Recent progress on urban overheating and heat island research. Integrated assessment of the energy, environmental, vulnerability and health impact. Synergies with the global climate change. Energy Build. 207, 109482. doi: 10.1016/j.enbuild.2019.109482
Schyns, J. F., Hoekstra, A. Y., Booij, M. J., Hogeboom, R. J., and Mekonnen, M. M. (2019). Limits to the world's green water resources for food, feed, fiber, timber, and bioenergy. Proc. Natl. Acad. Sci. 116, 4893–4898. doi: 10.1073/pnas.1817380116
Segura, L. D., van Zeijl-Rozema, A., and Martens, P. (2022). Climate change adaptation in Central America: A review of the national policy efforts. Lat Am Policy. Available online at: https://onlinelibrary.wiley.com/doi/10.1111/lamp.12277 doi: 10.1111/lamp.12277
Sheffield, P. E., Herrera, J. G. R., Lemke, B., Kjellstrom, T., and Romero, L. E. B. (2013). Current and future heat stress in Nicaraguan work places under a changing climate. Ind. Health. 51, 123–127. doi: 10.2486/indhealth.2012-0156
SINAC (2013). Análisis de vulnerabilidad al cambio climático de las áreas silvestres protegidas terrestres. San José; 76. Available online at: https://canjeporbosques.org/wp-content/uploads/2017/07/Informe-final-1.pdf
Singh, A., Sharma, J., Paichha, M., and Chakrabarti, R. (2020). Achyranthes aspera (Prickly chaff flower) leaves- and seeds-supplemented diets regulate growth, innate immunity, and oxidative stress in Aeromonas hydrophila-challenged Labeo rohita. J. Appl. Aquac. 32, 250–267. doi: 10.1080/10454438.2019.1615594
Singh, C., Ford, J., Ley, D., Bazaz, A., and Revi, A. (2020). Assessing the feasibility of adaptation options: methodological advancements and directions for climate adaptation research and practice. Clim. Change. 162, 255–277. doi: 10.1007/s10584-020-02762-x
Steinhoff, D., Monaghan, A., and Clark, M. (2015). Projected impact of twenty-first century ENSO changes on rainfall over Central America and northwest South America from CMIP5 AOGCMs. Clim. Dyn. 44, 1329–1349. doi: 10.1007/s00382-014-2196-3
Stewart, I., Maurer, E., Stahl, K., and Joseph, K. (2022). Recent evidence for warmer and drier growing seasons in climate sensitive regions of Central America from multiple global datasets. Int. J. Climatol. 42, 19. doi: 10.1002/joc.7310
Taylor, M., and Alfaro, E. (2005). “Climate of Central America and the Caribbean,” in Encyclopedia of World Climatology, eds E. John Oliver (Springer), 183-189. doi: 10.1007/1-4020-3266-8_37
Thomas, A., Theokritoff, E., Lesnikowski, A., Reckien, D., Jagannathan, K., Cremades, R., et al. (2021). Global evidence of constraints and limits to human adaptation. Reg. Environ. Chang. 21, 85. doi: 10.1007/s10113-021-01808-9
Ugalde, K. (2022). Estudio de inicio y término de la estación lluviosa en el pacífico norte de Costa Rica en el periodo 1950-2020. Universidad de Costa Rica; Available online at: https://www.kerwa.ucr.ac.cr/handle/10669/89114
Umaña, G. (2014). Ten years of limnological monitoring of a modified natural lake in the tropics: Cote Lake, Costa Rica. Rev. Biol. Trop. 62, 567–578. doi: 10.15517/rbt.v62i2.8446
UNDRR (2021). Annual report 2021. Available online at: https://www.undrr.org/publication/undrr-annual-report-2021
UNEP (2021). Adaptation Gap Report 2020. Nairobi: United Nations Environment Programme (UNEP). Available online at: https://www.unep.org/adaptation-gap-report-2020 (accessed March 16, 2022).
UNEP/SEI (2020). The discrepancy between countries' planned fossil fuel production and global production levels consistent with limiting warming to 1.5°C or 2°C. Production Gap Report 2020.
UNFCC (2015). Synthesis report on the aggregate effect of the intended nationally determined contributions. Bonn. Available online at: https://unfccc.int/resource/docs/2015/cop21/eng/07.pdf (accessed July 20, 2023).
UNFCCC (2023). Submitted NAPs. Available online at: https://napcentral.org/submitted-naps (accessed April 15, 2023).
United Nations Climate Change (2022). Payments for Environmental Services Program | Costa Rica. Payments for Environmental Services Program. Available online at: https://unfccc.int/climate-action/momentum-for-change/financing-for-climate-friendly-investment/payments-for-environmental-services-program?gclid=Cj0KCQjwlumhBhClARIsABO6p-wqCK8bvIfi4zORqchq2xwtE-oAzGVg39w-SFOUpqVcRGzXldMSA8caAiwuEALw_wcB
Vásquez, A., Giannotti, E., Galdámez, E., Velásquez, P., and Devoto, C. (2019). “Green infrastructure planning to tackle climate change in Latin American cities,” in Urban Climates in Latin America. doi: 10.1007/978-3-319-97013-4_13
Veas-Ayala, N., Quezada, A., Hidalgo, H., and Alfaro, E. (2018). Humedales del Parque Nacional Chirripó, Costa Rica: características, relaciones geomorfológicas y escenarios de cambio climático. Rev. Biol. Trop. 66, 13.16. doi: 10.15517/rbt.v66i4.31477
Vega-García, H. (2004). Migración ambiental inducida por variabilidad climática y su tratamiento en las políticas públicas regionales: el corredor centroamericano de la sequía. Universidad Nacional. Available online at: https://repositorio.una.ac.cr/handle/11056/21789
Viviroli, D., Dürr, H. H., Messerli, B., Meybeck, M., and Weingartner, R. (2007). Mountains of the world, water towers for humanity: Typology, mapping, and global significance. Water Resour. Res. 43, 13. doi: 10.1029/2006WR005653
Wallman, D., Wells, C., and Rivera-Collazo, I. C. (2018). The Environmental Legacies of Colonialism in the Northern Neotropics: Introduction to the Special Issue. Environ. Archaeol. 23, 1–3. doi: 10.1080/14614103.2017.1370857
Wang, C. (2007). Variability of the Caribbean low-level jet and its relations to climate. Clim. Dyn. 29, 411–422. doi: 10.1007/s00382-007-0243-z
WCRP (2015). Region 2: Central America. Available online at: https://cordex.org/domains/cordex-region-central-america-cordex/
WFP (2017). Food Security and Emigration. Why People Flee and the Impact on Family Members Left behind in El Salvador, Guatemala and Honduras. Available online at: https://docs.wfp.org/api/documents/WFP-0000022124/download/?_ga=2.110581765.1010989010.1540307778-1659157705.1540201825 (accessed July 14, 2023).
WMO (2022). State of the Climate in Latin America and the Caribbean. 44. Available online at: https://library.wmo.int/doc_num.php?explnum_id=11270
World Bank (2021). Nicaragua - Hurricanes Eta and Iota Emergency Response Project. Available online at: https://documents.worldbank.org/en/publication/documents-reports/documentdetail/911361611630074343/nicaragua-hurricanes-eta-and-iota-emergency-response-project (accessed July 14, 2023).
Wrathall, D., Devine, J. A., Aguilar-González, B., Benessaiah, K., Tellman, E., Sesnie, S. E., et al. (2020). The impacts of cocaine-trafficking on conservation governance in Central America. Glob. Environ. Chang. Policy Dimens. 63, 102098. doi: 10.1016/j.gloenvcha.2020.102098
Wright, G., Gjerde, K. M., Johnson, D. E., Finkelstein, A., Ferreira, M. A., Dunn, D. C., et al. (2021). Marine spatial planning in areas beyond national jurisdiction. Mar. Policy 132, 103384. doi: 10.1016/j.marpol.2018.12.003
Yalew, S. G., van Vliet, M. T. H., Gernaat, D. E. H. J., Ludwig, F., Miara, A., Park, C., et al. (2020). Impacts of climate change on energy systems in global and regional scenarios. Nat. Energy. 5, 794–802. doi: 10.1038/s41560-020-0664-z
Yglesias-González, M., Palmeiro-Silva, Y., Sergeeva, M., Cortés, S., Hurtado-Epstein, A., Buss, D. F., et al. (2022). Code Red for Health response in Latin America and the Caribbean: Enhancing peoples' health through climate action. Lancet Reg. Health Am. 11, 8. doi: 10.1016/j.lana.2022.100248
Zárate-Hernández, E. (2013). Climatología de masas invernales de aire frío que alcanzan Centroamérica y el Caribe y su relación con algunos índices árticos. Tópicos Meteorológicos y Oceanográficos 12, 35–55.
Keywords: Central America, climate change, climate adaptation, Central American Dry Corridor, climate policies
Citation: Ley D, Guillén Bolaños T, Castaneda A, Hidalgo HG, Girot Pignot PO, Fernández R, Alfaro EJ and Castellanos EJ (2023) Central America urgently needs to reduce the growing adaptation gap to climate change. Front. Clim. 5:1215062. doi: 10.3389/fclim.2023.1215062
Received: 01 May 2023; Accepted: 13 September 2023;
Published: 24 November 2023.
Edited by:
Sirkku Juhola, University of Helsinki, FinlandReviewed by:
Jose A. Marengo, Centro Nacional de Monitoramento e Alertas de Desastres Naturais (CEMADEN), BrazilByomkesh Talukder, York University, Canada
Copyright © 2023 Ley, Guillén Bolaños, Castaneda, Hidalgo, Girot Pignot, Fernández, Alfaro and Castellanos. This is an open-access article distributed under the terms of the Creative Commons Attribution License (CC BY). The use, distribution or reproduction in other forums is permitted, provided the original author(s) and the copyright owner(s) are credited and that the original publication in this journal is cited, in accordance with accepted academic practice. No use, distribution or reproduction is permitted which does not comply with these terms.
*Correspondence: Débora Ley, debbieannley@yahoo.com