- Department of Thematic Studies–Environmental Change, Centre for Climate Science and Policy Research, Linköping University, Linköping, Sweden
An increase in carbon capture and storage (CCS) projects, including bioenergy with CCS (BECCS), has led to an urgent demand for storage sites, and Norway stands out for its ongoing and planned geological storage sites in a European context. Even though there are no commercial carbon dioxide enhanced oil recovery (CO2-EOR) projects in Norway and the North Sea, there is scientific literature linking CO2-EOR and CCS in this geographical region. CO2-EOR utilizes CO2 to extract additional oil, counteracting the climate change mitigation purpose of geological storage. This review article explores how CCS is represented in the scientific literature on CO2-EOR in the North Sea and Norway, with a focus on system synergies and contradictions in relation to climate change mitigation. The main themes in the scientific literature on CO2-EOR in the North Sea are climate change, economics, and geological feasibility. Monitoring, safety, and leakage in addition to transportation of CO2 are less salient. The results show that there are contrasting framings in the literature. One framing is that CO2-EOR is a gateway to large-scale storage which maintains, or even expands, the extraction of fossil fuels and contributes to a sustainable transition in the long run through knowledge building and shared infrastructure. In contrast, another framing is that CO2-EOR combined with CCS have goal conflicts and are therefore not compatible, illustrating complexities with geological storage. Finally, this study reflects on how techno-economic research on CO2 storage in the North Sea and Norway is furthered through critical social science perspectives.
1. Introduction
In the context of rising climate change, there are different pathways to limit the temperature increase to 1.5°C as set out in the Paris Agreement, including various methods to store carbon dioxide (CO2) to reduce emissions in the atmosphere (IPCC, 2023). The Intergovernmental Panel on Climate Change's (IPCC) sixth assessment report includes carbon dioxide enhanced oil recovery (CO2-EOR) as a method that may store CO2 permanently and describes CO2-EOR as a possible bridge to a transition including carbon capture and storage (CCS) (IPCC, 2022). CO2-EOR is an industrial process to extract fossil fuels by injecting CO2 into oil fields, and some of this injected CO2 is stored in geological formations after the industrial process which is why it is characterized as a CO2 storage method (Núñez-López and Moskal, 2019). In fact, the Global CCS Institute (2022) states that 22 of 29 commercial CCS projects currently in operation apply CO2-EOR, which can also be considered carbon capture utilization and storage (CCUS) initiatives, where CO2 is first used in an industrial process before some of this CO2 is subsequently stored (Muffett and Feit, 2019). Meanwhile, other applications of EOR inject different gases and fluids and are therefore not methods for storing CO2. Besides the method of CO2-EOR for storing CO2, some projects store CO2 in dedicated geological storage facilities for the sole benefit of climate change mitigation, such as in Norway, but there are challenges in financing new transport and storage sites (Geske et al., 2015a) and acquiring knowledge on geological characteristics (Mathisen and Skagestad, 2017). Akerboom et al. (2021) acknowledge that new storage sites can take a long time to materialize due to social, technical, legal, and economic challenges. Even though there are several places for potential CO2 storage in Europe (Lux et al., 2023), there is a bottleneck in storage capacity due to a shortage of operating storage facilities and a lack of open-access knowledge on regional geological storage capacities (Fajardy et al., 2019). This lack of storage facilities, knowledge gaps, and challenges with CO2 storage have implications for carbon dioxide removal (CDR) approaches including direct air carbon capture and storage (DACCS) and bioenergy with carbon capture and storage (BECCS), which can be illustrated by the case of Norway and the North Sea.
In Europe, the North Sea is home to most of the projects developing CO2 storage, but Norway is the only country currently storing CO2 at its storage site called Sleipner (Global CCS Institute, 2022). Norway has the highest potential for CO2 storage volume in a European context (Lux et al., 2023). Fossil fuel companies that are involved in CCS projects in Norway are conducting research and development on EOR due to maturing oil fields in the North Sea region (Roefs et al., 2019), although CO2-EOR is not implemented on a commercial scale in Europe (e.g., Kemp and Kasim, 2013; Mathisen and Skagestad, 2017; Bergmo et al., 2018). Norway is the only country in the world with operational commercial-scale CCS projects driven by CO2 tax, and a Norwegian offshore CO2 tax introduced in 1991 contributed to economically viable offshore CO2 storage at Sleipner (North Sea) and Snøhvit (Barents Sea) which began storing CO2 for the natural gas processing industry in 1996 and 2008, respectively. EOR is not applied at either of these storage sites. In 2024, the Norwegian Government will initiate the first open-source cross-border CO2 storage project called Northern Lights which is part of the Longship CCS project, with the purpose of storing CO2 from Europe (Northern Lights, 2023). Some of the CO2 will be of biogenic origin such as from a waste-to-energy facility, so this can in part be characterized as a BECCS initiative (Schenuit et al., 2021). Since Norway has come the furthest of any country in Europe when it comes to CO2 storage in addition to having knowledge on prospective future locations for both CO2 storage and EOR (Norwegian Petroleum Directorate, 2011), this study focuses on Norway and its context in the North Sea region. Theoretically, Norway has the largest potential for both oil recovery from CO2-EOR and CO2 storage compared with neighbors in the North Sea region, followed by potentials in the UK section of the North Sea (Tzimas et al., 2005). Existing CO2-EOR projects in North America, China, Saudi Arabia, and the United Arab Emirates are onshore (Elmabrouk et al., 2017), while the only large-scale offshore CO2-EOR project in operation is in Brazil (Eide et al., 2019). Therefore, the North Sea region and Norway with its offshore CO2 storage sites and possible offshore applications of CO2-EOR stand out from most existing CO2-EOR projects onshore.
According to the European Commission, the Northern Lights project will connect CO2 capture initiatives in the United Kingdom (UK), Ireland, Belgium, the Netherlands, France, and Sweden (EC, 2023). Within the Nordic context, Sweden is increasingly investing in research and development on BECCS, and the Swedish Government and industry actors foresee the possibility of storing CO2 in Norway as a starting point (SOU, 2020; Rodriguez et al., 2021; Lefvert et al., 2022). However, out of the 1.5 million tons per annum (Mtpa) of CO2 capacity at Northern Lights starting in 2025, 0.8 Mtpa CO2 is earmarked for two Norwegian facilities: Norcem cement at Brevik and Hafslund Oslo Celsio waste-to-energy (Northern Lights, 2023). In addition, Yara Sluiskil, a Dutch fertilizer facility will export 0.8 Mtpa CO2 from the Netherlands to Norway (Yara, 2022). Even though there is additional demand, CO2 from these Norwegian and Dutch companies means that the Northern Lights project will initially be at full storage capacity, until Northern Lights increases storage capacity to 5 Mtpa in the longer term (Northern Lights, 2023).
The relationship between CCS and CO2-EOR is not new, and the shared history of CO2-EOR and CCS in the 1970s in Texas illustrates overlaps between these technologies which can be integrated in systems (Loria and Bright, 2021). This is problematic since CO2-EOR and CCS have fundamentally different purposes: CO2-EOR is driven by the economic benefit of extracting additional oil and gas from nearly depleted fields, while CCS projects have the goal of mitigating climate change by permanent disposal of CO2. As both technological systems evolve, it is important to distinguish them due to goal conflicts, but also to understand the relationship between CO2-EOR and CCS since CO2-EOR could be a catalyst for the development of CCS. Furthermore, CO2-EOR and CCS could have systemic overlaps such as shared infrastructures, common actors, and synergies in industrial applications. Social science can provide insights by studying the framing of CO2-EOR in the literature. Framing refers to a situated position on a topic which is relevant for policymaking (Waller et al., 2020). Framing illustrates perceptions from a certain viewpoint including its salient aspects which define problems and possible solutions (Megura and Gunderson, 2022). How an issue such as CCS and BECCS is framed can impact its deployment landscape (Gough and Mander, 2019). Studying the framing of the relationship between CO2-EOR and CCS in the literature contributes to understanding themes and complexities in climate change mitigation.
Taking into consideration the demands for CO2 storage and ongoing research and development linking CCS with CO2-EOR, this review article aims to explore how CCS is represented in the scientific literature on CO2-EOR in the North Sea and Norway, with a focus on system synergies and contradictions in relation to climate change mitigation. The research questions are: What are the main themes discussed in the scientific literature on EOR concerning the intersection of CO2-EOR and CCS? How is the relationship between CO2-EOR and CCS framed with the imperatives of climate change mitigation? The review article is divided into two parts: a descriptive thematic mapping of the literature followed by an analysis of the framings on the relationship between CO2-EOR and CCS which also incorporates social science literature on CDR.
This study on CO2-EOR has implications for CO2 storage, which is part of the value chain in CCS, CCUS, BECCS, and DACCS initiatives. Through a focus on the geographical region of the North Sea, this study contributes to social science literature on CDR (e.g., Carton et al., 2020; Stuart et al., 2020; Asayama, 2021; McLaren et al., 2021; Hansson et al., 2022) and adds a contrasting and broadened perspective to the existing CO2-EOR literature which, as will be illustrated in the review, is mostly treated in isolation from other branches of literature. This study also contributes focus on the North Sea empirical case to critical social sciences including literature on sustainability transitions which studies social, economic, and environmental aspects of systemic changes (Markard et al., 2012; Grubler et al., 2016) in addition to literature on carbon lock-in which refers to risks with prolonging an energy system based on fossil fuels in society (Unruh, 2000; Unruh and Carrillo-Hermosilla, 2006). According to Smith et al. (2023), there is a research gap on CDR studies in specific geographies, Markusson et al. (2020) suggest that more studies in specific geographies are needed to study the impacts of CDR, and Carton et al. (2023) state that empirically grounded studies on CDR could contribute to emissions reductions. By studying CO2-EOR in the North Sea and Norway, this study highlights how CDR social science studies could add to techno-economic literature with implications for storing CO2 as part of systems like BECCS, CCS, CCUS, and DACCS.
Section 2 describes the materials and methods for this study. Section 3 discusses themes in CO2-EOR research and modeling studies in the North Sea based on a semi-systematic literature review with a focus on the last 17 years. Section 4 presents two framings on the relationship between CO2-EOR and CCS, with support from perspectives in social science literature. Section 5 reflects on the literature review and discusses how critical social sciences could contribute to a deeper understanding of the socio-political context on CO2 storage in the North Sea region.
2. Materials and methods
This study is divided into two parts: (1) a literature review on CO2-EOR and CCS in the North Sea and (2) a critical analysis based on the results of the literature review in the light of social science literature to illustrate the framings on CO2-EOR and CCS.
2.1. Literature review
The first part of this study (Section 3) is a qualitative semi-systematic literature review. According to Snyder (2019), a semi-systematic literature review is a narrative approach to studying a topic that has been researched from different disciplines using various methods, and it can be used to map themes to synthesize the knowledge on a certain topic. The material for this study was gathered in January 2023 from both Scopus and Web of Science which are recognized databases in engineering, environmental and social sciences. Scopus is a database that searches article titles, abstracts, and keywords of peer-reviewed publications, and the Web of Science search including title, abstract, and author keywords was used for consistency. Several search word combinations were tried, and the search combination of: “CO2” and “EOR” and (“North Sea” or “Norway”) was used to identify articles for this literature review. The search with Norway was meant to include the fields in all seas in Norway such as the Norwegian Sea and Barents Sea, while the search for the North Sea is inclusive of fields in the UK and Denmark. Norway is in focus since it is the only country in Europe with ongoing geological storage of CO2, even though there are planned projects in the North Sea in Denmark, the UK, and the Netherlands (Global CCS Institute, 2022). There is extensive knowledge and mapping of prospective storage sites in Norway (Norwegian Petroleum Directorate, 2011). CO2-EOR is not conducted or developed on a large scale anywhere in Europe (Thorne et al., 2020; Global CCS Institute, 2022).
The timeframe 2006 to 2022 was used to include research in the leadup to the United Nations Framework Convention on Climate Change (UNFCCC) conference in Copenhagen in 2009 up to the research published through the end of 2022. In Scopus, this search combination led to 104 items, and in the Web of Science, a search in “all databases” led to 48 items. Next, the result list was refined by conducting an additional search “within result search” for: “CCS” or “CCUS” in both databases. Scientific literature was considered relevant for this study if it included a focus on the North Sea or Norwegian context, leading to a total of 35 articles from peer-reviewed journals and conference papers in this study. This literature review has implications for other European countries that are interested in storage in the North Sea, including the UK, the Netherlands, Sweden, Denmark, Ireland, France, and Belgium (Global CCS Institute, 2022). The articles include a combination of open access articles and articles behind paywalls accessed via the Linköping University library. Figure 1 lists these articles by publication year.
Next, patterns were identified in the articles based on an inductive thematic analysis (Saldaña, 2013). These themes (Section 3) were selected based on their inclusion in the articles from different perspectives on the relationship between CO2-EOR and CCS. These themes were identified by inductively mapping the content in the articles and then clustering the content into thematic areas. These themes are somewhat overlapping, but the purpose of the themes is to understand the common threads that link CO2-EOR and CCS in the literature. To minimize repetition in the results narrative, specific examples are only described under the most prominent theme. In addition, the quotations and references in the results section show examples from scientific literature in this review to highlight key points within each theme, but this study does not provide a conclusive overview of all articles. This means that some of the inferences from the scientific literature may in fact be included in a broader set of articles than indicated in the citations.
2.2. Framings
The second part of this study (Section 4) is largely based on the literature review and themes which served as the premise for identifying and discussing framings on CO2-EOR and CCS. According to Entman (1993), frames show what matters most and serve different functions such as a way of defining problems, identifying causes, making moral judgments, and offering solutions. Frames reflect underlying perceptions and epistemologies (Hulme, 2009), and it is possible to have different frames within a particular text as exemplified by Waller et al. (2020). The purpose of analyzing and discussing framings on the relationship between CO2-EOR and CCS is to understand the most salient aspects underpinning different perspectives on these technologies.
The use of frames in previous research on technological innovations, CDR, and CCS show that framings are a way to study salient aspects in perceptions on technologies related to climate change mitigation. In technological innovations research, framings of innovations can be portrayed in positive or negative ways (Magnusson et al., 2021). Frames are useful when studying CDR technologies to understand how they can be perceived as part of a climate change mitigation strategy (Gough and Mander, 2019). Due to uncertainties with innovations such as CCS, there are also different interpretations and perceptions that can be illustrated through frames (Hansson and Bryngelsson, 2009). Gunderson et al. (2020) apply frames in their research with fossil fuel companies to understand framings on CCS including what is absent from these framings. Framings can impact future solutions for climate change, and therefore studying framings contributes to future energy transitions.
In this study, the identified themes in the literature review are the starting point for identifying frames which illustrate perspectives on the relationship between CO2-EOR and CCS with the imperative of climate change. The themes include contrasting perspectives on the compatibility between CO2-EOR and CCS which are further discussed in the framings. While the themes are descriptive in nature, the framings illustrate polarized perspectives in how CO2-EOR and CCS are linked. Through a qualitative discussion, this article presents two contrasting framings to illustrate synergies and goal conflicts between CO2-EOR and CCS in this North Sea literature and within the broader context of critical social sciences literature on CO2 storage. Additional social sciences literature is discussed to highlight the paradox of the two emerging framings in this study, particularly literature on carbon lock-in and sustainability transitions. Social science literature contributes to understanding technical studies through analytical reflections on system boundaries and possible paradoxes impacting climate change mitigation.
3. Themes at the crossroads of CO2-EOR and CCS
This section describes each theme based on the literature review, with quotations and references that show examples from scientific literature. Many of the articles in this study are techno-economic studies, with development-oriented ambitions, written or funded by the fossil fuel industry. Based on a review of the scientific literature selected, various patterns emerged and were clustered into five broad themes which are: (1) climate change; (2) economics; (3) monitoring, safety, and leakage; (4) geology; and (5) transportation. This section highlights the perspectives of the authors in the 35 articles in this literature review, in addition to a few clarifying references that complement some of the information in the articles.
Table 1 shows the list of articles included in this literature review with a column for each theme showing the level of inclusion based on four gradients from not including it at all in a study (white color) to including the theme in depth (dark green). This shows which themes are in focus in each article, illustrating research priorities in studies on CO2-EOR in the North Sea. The dark green gradient indicates that a theme is included in depth, while medium green indicates that a theme is discussed. When a theme is merely mentioned, it is shown in light green. Taking the article by Gruson et al. (2015) as an example, here is an overview of how the themes were identified in an individual article: climate is only mentioned in the framing of the abstract (climate = light green), transportation pipelines are mentioned in one sentence (transportation = light green), injection wells and site-specific geologic conditions are discussed on several occasions (geology = medium green), and economic issues and monitoring are included in depth (economics = dark green; monitoring = dark green). Figure 2 also illustrates the prevalence of each of these themes in the review article. As depicted in the pie chart, economics was the most common theme, followed by climate change and then geology. Transportation and monitoring, safety, and leakage were the least recurring themes. This pie chart was designed by creating a numbering system for the themes based on the level of inclusion of each theme in each article as shown in Table 1: none (0), low (1), medium (2), and high (3) to create a sum for each of the themes in the reviewed articles.
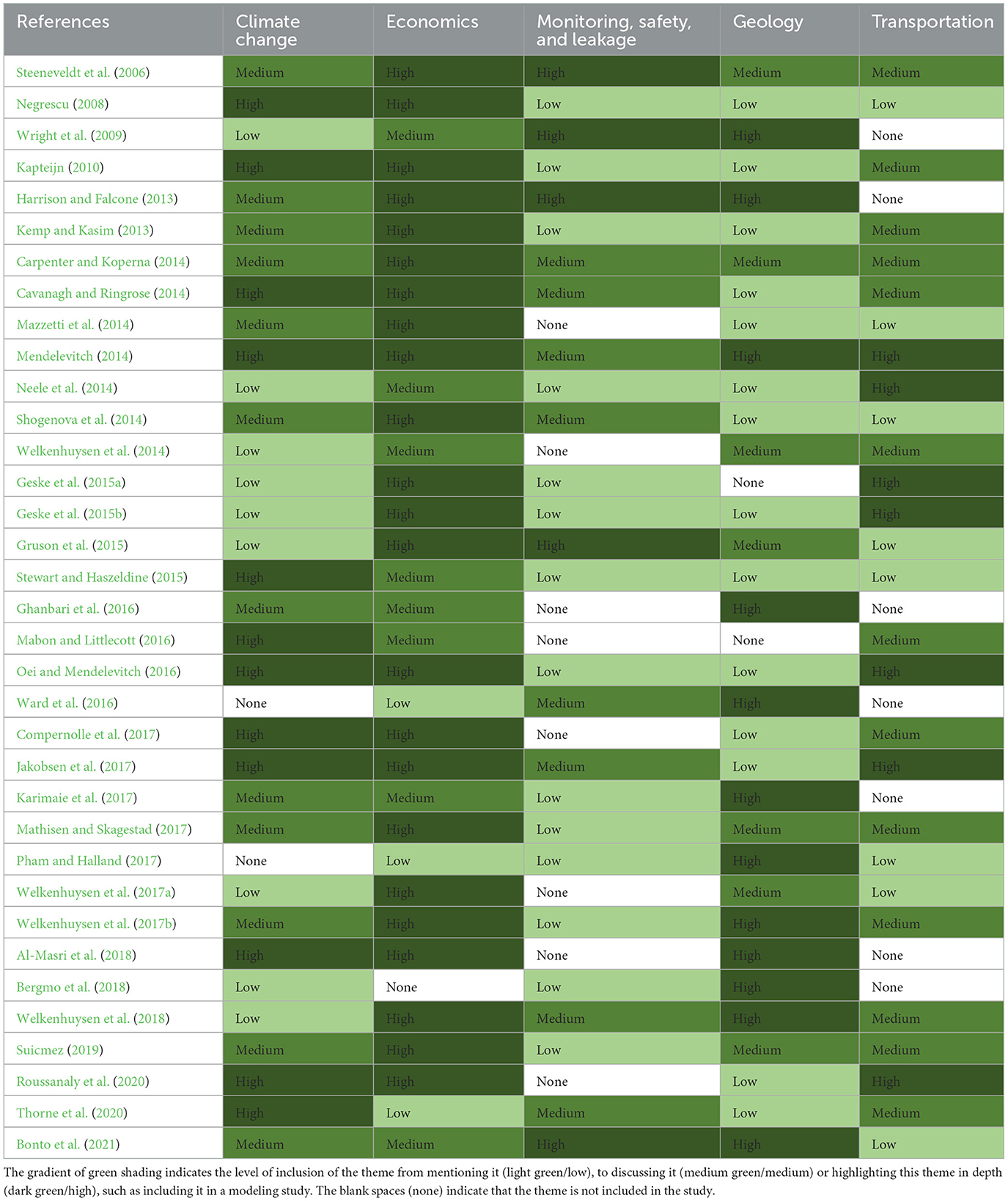
Table 1. The level of inclusion of the different themes in each of the 35 articles included in this study, listed by year of publication from 2006 to 2022.
3.1. Climate change
The first theme is climate change, which is discussed in about two-thirds of the articles. Although many of the articles in this literature review mention climate change or international climate agreements to reduce greenhouse gas emissions (GHG), only about half of the articles identify climate change as a key driver to store anthropogenic CO2 emissions, which are from human activities such as the use of fossil fuels. Several articles include climate change framing in the abstract or introduction with no other mention of climate change (Geske et al., 2015b; Gruson et al., 2015; Welkenhuysen et al., 2018). Other articles frame the issue of climate change at the beginning and end of an article, without discussing climate change in the content of the article (Karimaie et al., 2017; Welkenhuysen et al., 2017b; Suicmez, 2019; Bonto et al., 2021). Meanwhile, some articles do not mention climate explicitly but discuss environmental aspects, CCS and/or a reduction of industrial CO2 emissions (Negrescu, 2008; Mazzetti et al., 2014; Neele et al., 2014). Still, other articles do not include climate change or related concepts at all (Ward et al., 2016; Pham and Halland, 2017).
Several authors state that CO2-EOR could contribute to the commercial development of CCS infrastructure and the start of the carbon storage industry in the North Sea and Norway. This could include the sharing of infrastructure (Mazzetti et al., 2014), development of a market for CO2 (Pham and Halland, 2017), and initiation of large-scale storage (Neele et al., 2014). According to some authors, a benefit of CO2-EOR is that the fossil fuel industry's infrastructure and geological knowledge could set the groundwork for offshore CO2 storage via CCS in the North Sea. For example, Welkenhuysen et al. (2017a) state that CO2-EOR infrastructure could be used for CO2 storage after oil production. According to Mazzetti et al. (2014), there could be an economic motivation to store CO2 from natural gas streams in EOR projects in the North Sea, and they state that “… the combination of CO2-EOR with permanent CO2 storage in oil reservoirs may be a critical, near-term solution for creating economically viable CCS projects, facilitating early CCS infrastructure—and kick-starting deployment of CCS.” (p. 7289). This view shows that CO2-EOR could contribute to the near-term application of CCS with the necessary infrastructure for CO2 storage. Carpenter and Koperna (2014) discuss that there could even be a symbiotic relationship, “… not only does CCS need CO2-EOR to help promote economic viability for CCS, but CO2-EOR needs CCS to ensure adequate CO2 supplies to facilitate growth in oil production from CO2-EOR projects.” (p. 6718). This forwards a view that CO2-EOR and CCS could develop together and lead to integrated infrastructure for CO2 storage in the North Sea region.
Another perspective in the scientific literature is that CO2-EOR and CCS are incompatible with one another in creating a low-carbon future. According to some authors, this is because there is a goal conflict between these two applications, since CO2-EOR supports the fossil fuel industry which makes it even more challenging to mitigate climate change. For example, Mendelevitch (2014) states, “There is high regulatory uncertainty on the acceptance of CO2 abatement credentials generated from CO2-EOR.” (p. 151). This view questions if CO2-EOR is abated emissions since oil is produced. In addition, Cavanagh and Ringrose (2014) state that a CO2-EOR project would need to have significant CO2 storage to have a net zero GHG impact. Harrison and Falcone (2013) are also critical of the possible climate change benefit of CO2-EOR since this process produces oil while only displacing some oil produced from other sources, leading to a net increase in oil consumption. According to this view, oil production leads to goal conflicts when it comes to reducing GHG emissions since continued oil production both perpetuates the fossil fuel industry and makes it harder to reduce GHG emissions in the long term. Compernolle et al. (2017) also take the perspective that CO2-EOR would increase net oil extraction, negatively impacting efforts toward decarbonization. At the same time, another view is that there could be an opportunity to implement CO2-EOR in the near term as part of a sustainability transition. This is illustrated by focus groups with stakeholders in the UK conducted by Mabon and Littlecott (2016) that show some support to temporarily deploy CO2-EOR in the North Sea which could financially contribute to infrastructure for CCS, but this would only be favorable if it were part of a transition toward decarbonization and low-carbon technologies.
3.2. Economics
Some of the main patterns clustered within the theme of economics discussed in the articles in this review include oil price, CO2 price, the role of government, and legal and policy issues. CO2-EOR is an industrial application to extract fossil fuels, and Mathisen and Skagestad (2017) state that CO2-EOR is financially viable only if it leads to an increase in oil production. Oil price is named in several articles as a factor of the economic viability of CO2-EOR in the North Sea even though there are no commercial CO2-EOR projects in Europe (Geske et al., 2015b). Harrison and Falcone (2013) argue that 100 USD per barrel of oil would be needed to offset financial risks of CO2-EOR. Welkenhuysen et al. (2017a) state: “CO2-EOR is at this moment not applied in the North Sea oil fields, and especially with the current low oil prices, the profitability of such projects is questioned.” (p. 7068). This shows that oil price is a key aspect in the economic profitability of EOR, and a low oil price could be a barrier for the initiation of commercial scale projects according to this view. Welkenhuysen et al. (2014) also argue that oil price is the most sensitive parameter when assessing the profitability of CO2-EOR. Therefore, a low enough oil price contributes to an absence of the industrial application of offshore CO2-EOR in the North Sea.
CO2 tax is an example of an instrument that could stimulate CCS in either onshore or offshore projects according to several authors. Mendelevitch (2014) state that unless the CO2 price is high enough, CCS will not takeoff in the coming decade. Meanwhile, in the USA, tax incentives and tax breaks have supported the development of onshore CO2-EOR projects. In their financial models for CO2-EOR in the North Sea in the UK, Kemp and Kasim (2013) discuss how current tax rates on oil fields in the UK are too high to make EOR a viable business case, since these taxes include corporation tax (30%), Supplementary Charge (32%), and Petroleum Revenue Tax (50%) on fields older than March 1993. The UK's Carbon Price Floor to encourage low carbon investments could lead to more carbon capture projects if there is a high enough CO2 price (Kemp and Kasim, 2013). Therefore, they conclude that more research is needed on CO2 prices and tax incentives to determine the economic viability of CO2-EOR in the UK (Kemp and Kasim, 2013). Similarly, Compernolle et al. (2017) state that CO2 price is not enough to lead to investment in offshore CO2-EOR and CO2 storage in the North Sea, and that combined policy measures are needed.
Several authors highlight uncertainty around legal and policy issues as a key factor in making CO2 storage economically viable. According to Steeneveldt et al. (2006), “Apart from technical advance, legislation and regulatory changes will be the stronger drivers for closing the knowing-doing gap for CO2 capture and storage.” (p. 759). Geske et al. (2015b) state that CO2 is characterized as waste, and it is therefore governed under the London Convention which regulates marine dumping. The London Protocol to the London Convention was brought up by some authors as a barrier to CO2 transport and storage across national borders (Steeneveldt et al., 2006; Harrison and Falcone, 2013). According to the IMO (2019), a resolution in 2019 enables the provisional application of the 2009 amendment to Article 6 of the London Protocol enabling the export of CO2 across country borders for sub-seabed storage. Since the 2009 amendment is not in force, this resolution alleviates the legal barrier addressed in the scientific literature, which could lead to opportunities for CO2 export, if there is agreement between the countries involved.
In addition, there are other relevant global and European frameworks that impact the economic prospect of implementing CO2 storage including the EU CCS Directive and the EU Emissions Trading System (ETS). The EU CCS Directive provides a framework for safe geological storage of CO2 (EU, 2009b), and CCS is explicitly named as an activity in Annex I of the EU ETS (EU, 2009a), although the EU ETS does not currently include emissions from biomass, therefore there is no incentive for BECCS (EC, 2020; Rickels et al., 2021). The EU ETS is a cap-and-trade system to reduce GHG emissions in the European Economic Area which includes Norway (EC, 2021). Some authors argue that the low price of the carbon credits in the EU ETS, the European Union Allowance (EUA), has been a barrier for CCS (Cavanagh and Ringrose, 2014). Oei and Mendelevitch (2016) also share this view that the CO2 price in the EU ETS is not significant enough to motivate investment in CCS. Although the price has risen from a high of 6 EUR in 2016 to 97 EUR at the end of January 2023 (Trading Economics, 2023), the price remains insufficient, so other economic instruments could be necessary to incentivize a reduction of CO2 emissions according to this perspective.
3.3. Monitoring, safety, and leakage
This theme comprises the cluster of monitoring, safety, and leakage which are related issues addressed in the articles in this review. Although more than three-quarters of the articles mention monitoring, safety, or leakage, this theme is underdeveloped compared with the other themes. Some articles list monitoring, reporting, and verification (MRV) as a step in the CO2 storage process, such as framing it as a key part of the CCS value chain (Jakobsen et al., 2017). This is because MRV creates economic value in CCS when facilities emitting CO2 must comply with the EU MRV Regulation (EU, 2015) and EU CCS Directive (EU, 2009b) which are relevant in the North Sea region. CCUS such as CO2-EOR has economic value in the utilization of CO2 in an industrial process, while CCS without utilization relies on the CCS Directive and the price of EUAs under the EU ETS for monetary value. According to Mendelevitch (2014), “Permanent storage can only be credited if a monitoring scheme is in place that includes baseline monitoring and demonstrates and measures effective storage.” (p. 135). Therefore, many authors view monitoring as a key aspect of CO2 storage, especially if it were to contribute to climate change mitigation.
The scientific literature shows that there could be overlaps between monitoring at both CO2-EOR and dedicated CO2 storage sites. At the Sleipner CO2 storage facility in Norway, monitoring has been conducted since its inception in 1996, but long-term monitoring issues over a 100-year timeframe are unknown, such as impacts of CO2 on geological formations over time (Wright et al., 2009). This is because monitoring of offshore storage has only taken place over a few decades but monitoring over a longer timeframe could reduce uncertainties. According to Kapteijn (2010), surveillance infrastructure for EOR management could also be applied to survey dedicated CO2 storage. Cavanagh and Ringrose (2014) agree that there are benefits of having CO2 storage in gas fields which are already monitored. Furthermore, Steeneveldt et al. (2006) suggest that monitoring for CCS can learn from CO2-EOR, “… monitoring techniques and safe practice encompassing proper operator training, maintenance procedures, pressure monitoring, reliable gas detection systems, emergency shut-down procedures and other safety systems relevant for EOR can be adapted for CO2 storage” (p. 757-758). This perspective shows that monitoring CO2 storage for climate change mitigation purposes could benefit from knowledge from CO2-EOR monitoring programs (Steeneveldt et al., 2006). According to a study in the Danish North Sea, another aspect is the possibility of applying machine learning in monitoring approaches which could lower the costs of monitoring storage sites (Bonto et al., 2021).
The safety of CO2-EOR and CO2 storage is another issue addressed in the scientific literature. Some authors mention the importance of safe, long-term storage (Steeneveldt et al., 2006; Mendelevitch, 2014; Pham and Halland, 2017). According to Negrescu (2008) and Harrison and Falcone (2013), safety of storage sites is a reason monitoring is necessary. There are other concerns about safety when considering the long distance transport of CO2 from mainland Europe to a storage site in the North Sea, including the use of temporary storage for CO2 that is moved from one transport vessel to another (Geske et al., 2015a). Intermediate storage with additional safety measures could also increase the cost of transporting and storing CO2 (Geske et al., 2015a). In addition, other monitoring variables described in the scientific literature include the type of monitoring, its frequency, and duration over time. According to Shogenova et al. (2014) and Gruson et al. (2015), state level regulations impact the length of monitoring periods for CCS projects which can vary between EU Member States. This shows that in the EU, there are different standards and procedures in each country, even though there are IPCC standards for monitoring stored CO2 emissions, e.g., 2006 IPCC Guidelines for National Greenhouse Gas Inventories (Eggleston, 2006).
Leakage from storage sites is an aspect of monitoring that is included in some articles (Steeneveldt et al., 2006; Harrison and Falcone, 2013; Mathisen and Skagestad, 2017; Thorne et al., 2020). Leakage of CO2 could have a negative impact on climate change mitigation measures. According to Thorne et al. (2020), the amount of leakage in CO2-EOR processes is uncertain, but in their model of the Norwegian Continental Shelf, they show that even with 10% leakage in CO2-EOR due to transport and injection, there are less CO2-equivalent emissions per kilogram of oil resulting from CO2-EOR than from conventional oil extraction. Here, the reference case of what CO2-EOR is compared with is critical in identifying the hypothetical cost or benefit in terms of CO2 emissions. Another concern is CO2 leakage from power outages at CO2-EOR sites (Mathisen and Skagestad, 2017; Thorne et al., 2020).
3.4. Geology
Geological characteristics determine the feasibility of CO2-EOR and CO2 storage, but there are many uncertainties and knowledge gaps identified by the scientific literature. This includes a lack of detailed knowledge about geological formations which can be a barrier for CO2 storage (Mathisen and Skagestad, 2017; Al-Masri et al., 2018). According to Harrison and Falcone (2013), there is potential for CO2 storage in the North Sea, but it would require assessments which are already available for oil and gas fields. Therefore, taking advantage of existing sites and knowledge held by oil and gas companies could be more economical than assessing additional sites. Even though there could be potential to store CO2 in other geological formations, depleted oil and gas fields are currently the cheaper alternative since the cost to evaluate these new storage sites make it too expensive (Harrison and Falcone, 2013). Based on a literature review on the Danish North Sea by Bonto et al. (2021), there could also be potential to store CO2 in other types of rock such as offshore chalk formations in the North Sea, although more research would be needed to explore this possibility. This differs from the experience of storage in sandstone formations at Sleipner and Snøhvit, and planned at the Aurora storage site as part of the Northern Lights project.
Interest in CO2-EOR is rising in the North Sea partly due to declining oil fields. Studies show different potentials for storing CO2. Geske et al. (2015b) state that there could be potential for 1.4–4.0 Gigatonne (Gt) of CO2 storage per year when applying CO2-EOR over 40 years in the British and Norwegian oil fields in the North Sea. Mendelevitch (2014) published a review article showing that there are inconsistencies when compiling data on CO2-EOR potential in different countries in the North Sea, so even though they state that there is substantial oil recovery potential in the North Sea, equating to 1.2 Gt CO2 storage per year, these amounts are based on different calculation methods in the UK, Norway, and Denmark. These different calculation methods make it difficult to compare the possible amount of oil recovery and the possible CO2 storage capacity across geographies. This shows that there are uncertainties and inconsistent ways of measuring storage potential in the North Sea.
Another aspect discussed in several articles is onshore vs. offshore storage. Commercial scale CO2-EOR projects to date are onshore, and the models for offshore storage show that it could be more expensive partly due to higher compression costs offshore (Ghanbari et al., 2016). Geske et al. (2015a) suggest that offshore storage could lead to a higher rate of public acceptance. Conducting commercial scale CO2-EOR would be new in the North Sea, but it could be driven by climate change and economic variables according to the literature. To this end, Al-Masri et al. (2018) state that CO2-EOR using CO2 from anthropogenic sources could provide a significant environmental benefit and therefore motivate an investment in new storage sites, since this would decrease the net emissions in the atmosphere.
3.5. Transportation
Infrastructure for CO2 transport is discussed in depth in about one-fifth of the articles. According to Kemp and Kasim (2013), there could be economic benefits of a networked pipeline in the Central North Sea in the UK, using a combination of new and existing pipelines. Two associated transport studies show that there could be possibilities to optimize the vessel and pipeline transportation part of CCS infrastructure, but authors suggest that more studies are needed (Geske et al., 2015a,b). Oei and Mendelevitch (2016) state that the most likely storage option in a European context is a regional network of collaborations to store CO2 in the North Sea. This vision would necessitate regional cooperation to address the geographical difference between where CO2 is emitted and where it could potentially be stored. In their study on the EU CCS Directive (EU, 2009b), Shogenova et al. (2014) state that only three European countries have developed shared principles for managing transboundary cooperation on transporting, injecting, and storing CO2 in the North Sea—Germany, the Netherlands, and the UK—but they suggest that there could be more transboundary cooperation in the future. Despite these principles, investment costs, political support, and public acceptance remain challenges for CCS in Europe.
CO2 can be transported via pipelines and/or vessels, and most studies discuss having vessels which makes sense with offshore storage projects in the North Sea when industries applying carbon capture are near coastlines. In studies on transportation options for CO2 storage, several authors conclude that marine transport by vessel is more cost effective (Kapteijn, 2010; Geske et al., 2015a; Jakobsen et al., 2017). Furthermore, Neele et al. (2014) state that vessels could benefit both CO2-EOR and CCS at early projects instead of constructing pipeline networks. This is because vessels are more flexible, offering a timelier option with a lower investment cost than pipelines (Neele et al., 2014; Welkenhuysen et al., 2014; Geske et al., 2015b). Meanwhile, other authors focus on transportation pipelines in the North Sea region (Oei and Mendelevitch, 2016; Compernolle et al., 2017). Oei and Mendelevitch (2016) discuss the need for extensive pipelines for implementing CCS across Europe which would be very expensive, stating that sites with proximity to offshore storage in the North Sea have the highest possibility of being realized. Mazzetti et al. (2014) reiterate the concern that expensive transportation pipelines for CO2 are a barrier for CO2 storage. Therefore, the articles show that vessels and proximity to CO2 storage sites in the North Sea contribute favorable conditions for implementing CCS in a European context.
CO2-EOR could potentially finance infrastructure used by CCS projects such as transportation networks in the North Sea. It could thereby contribute to shared infrastructure for CCS and CO2-EOR according to some authors (Cavanagh and Ringrose, 2014; Mendelevitch, 2014; Mabon and Littlecott, 2016) and cover the costs of a European CO2 transportation and storage network (Geske et al., 2015b). At the same time, infrastructure development requires public acceptance and long-term planning such as having a “North Sea transition plan” (Mabon and Littlecott, 2016, p. 135). This means that developing CCS in the North Sea region is a long-term commitment including several actors and cross-border collaborations. Furthermore, according to Kapteijn (2010), combining CCS and CO2-EOR could lead to an integrated system which contributes to financing CCS infrastructure for industries to reduce CO2 emissions. An integrated system with shared infrastructure could enable CO2 storage. Nevertheless, there are contradictions in the literature regarding the optimal type of transportation network, and there are additional complexities to consider with offshore storage within Europe than with transport to onshore storage sites in North America (e.g., Kapteijn, 2010; Compernolle et al., 2017).
4. Framings of CO2-EOR and CCS
This section discusses framings of CO2-EOR and CCS, with the themes resulting from the literature review described in Section 3 as a starting point, in addition to incorporating social sciences literature to further illustrate the relationship between CO2-EOR and CCS with the imperatives of climate change mitigation. This literature review shows that there are different perspectives on CO2-EOR in the North Sea, including conflicting views on its relationship with CCS. Based on the themes in this study, there are two main framings of climate change in the North Sea in the CO2-EOR literature. The first framing is that CO2-EOR is a gateway to other types of large-scale storage with the sole purpose of storing CO2 for climate change mitigation purposes. In contrast, the second framing is that CO2-EOR and CCS are incompatible with one another due to goal conflicts. These framings show that there are complexities for future geological storage, yet a critical social science perspective is absent in the articles in this review, and such a perspective could contribute context regarding the realizability of future socio-technical transitions. Markusson et al. (2011) states that framings of CCS demonstration projects matter because framings impact project designs, even if framings can change over time. Cox et al. (2018) describe how framing of a problem and technology impacts governance and policy decisions. Therefore, framings on technologies in research shapes both future technology design and its implementation in society. Expanding on the literature review selection for this article, this section incorporates both literature from this review and additional related scientific literature to further contextualize the framings.
4.1. CO2-EOR as a gateway to storage for climate change mitigation
According to the first framing, which is the dominant framing in this literature review, CO2-EOR creates an opportunity for large-scale CO2 storage in the North Sea. This framing is also supported by the IPCC (2022) report which states that while EOR potential is a small fraction of the need for CCS, EOR is a gateway to CCS pilot and demonstration projects. Fossil fuel companies have geological knowledge due to decades of exploitation of oil and gas fields which could help identify potential CO2 storage sites for CCS (Mathisen and Skagestad, 2017; Al-Masri et al., 2018). Godec et al. (2013) show the economic advantages for fossil fuel companies and tax-collecting states in addition to CO2 storage benefits when merging CO2-EOR with CCS. Even though there are no concrete plans for commercial-level CO2-EOR projects in the North Sea, most of the literature in this review suggests that there could be benefits of linking CO2-EOR with CCS in at least an early stage toward decarbonization (e.g., Carpenter and Koperna, 2014; Cavanagh and Ringrose, 2014; Mazzetti et al., 2014). This is similar to the findings by Núñez-López and Moskal (2019) whose literature review highlights the potential of CO2-EOR to contribute to decarbonization in the short term when coupling it with CCS. This is because CO2-EOR is a commercially established process, and it could be combined with capture of anthropogenic CO2 emissions such as from large point sources at industrial facilities (Núñez-López and Moskal, 2019). Adding to the perspectives of many of the authors in this literature review, Raffa (2021) suggests that there are opportunities to combine old and new EOR technologies with CO2 storage as one of many solutions to reduce emissions to mitigate climate change.
Most articles in this literature review suggest that there is an opportunity for CO2-EOR and CCS projects to collaborate. Cavanagh and Ringrose (2014) state that CO2-EOR contributes to the development of CO2 transport and storage infrastructure in the European context, which could contribute to reducing costs for future CCS projects. There is a trend in Norway where different actors are responsible for parts of the CCS value chain, such as for capture vs. transport and storage (Chailleux, 2019). This could create opportunities for shared infrastructure for transport and storage for both CO2-EOR and CCS. Furthermore, until the global demand for fossil fuels starts decreasing, there could be an opportunity for CO2-EOR to contribute to the energy system (Raffa, 2021). Several authors argue that capturing and storing CO2 emissions from anthropogenic activities, opposed to using CO2 occurring in natural underground sources which would not otherwise end up in the atmosphere, is relevant to maximize the climate benefit of CO2-EOR (Mendelevitch, 2014; Karimaie et al., 2017; Al-Masri et al., 2018).
A tension is that CO2-EOR is only conducted when it is economically profitable for the extraction of fossil fuels, so it is a shift to consider maximizing CO2 storage instead. At the same time, Edwards and Celia (2018) state that it is most economical to use captured CO2 in EOR rather than in dedicated geological storage sites, unless there are economic instruments for climate change mitigation activities. Based on economic arguments, utilizing CO2 that is captured from anthropogenic CO2 sources for EOR is expensive, so most current CO2-EOR projects utilize CO2 from natural geological sources (Hill et al., 2013; Cooney et al., 2015). Based on their scenario study in the North Sea, Roefs et al. (2019) show that there could be economic and environmental benefits of combining CO2-EOR and CCS, but it depends on CO2 price and oil price, which is an argument that is consistent with several authors in the reviewed literature. According to some authors, CCS could benefit from the experiences of CO2-EOR in monitoring, and the two could work together in monitoring efforts (Steeneveldt et al., 2006), but there are different views on the compatibility of monitoring schemes for offshore storage in the North Sea region (Mendelevitch, 2014).
4.2. Incompatibilities between CO2-EOR and CCS
A second framing is that coupling CO2-EOR and CCS is not compatible with climate change mitigation measures since it perpetuates a cycle of GHG emissions, regardless of if CO2 comes from natural underground geological sources or from anthropogenic sources such as industries. Some authors are critical of the climate change mitigation benefit of storing CO2 via EOR due to goal conflicts since oil is produced (Harrison and Falcone, 2013; Mendelevitch, 2014; Compernolle et al., 2017). Stewart and Haszeldine (2015) suggest that CO2 utilized by EOR could otherwise be permanently stored in geological formations in the North Sea without supporting the fossil fuel industry. According to this perspective, using dedicated geological storage for CO2 is better than applying CO2-EOR in the North Sea region. Meanwhile, when using CO2 from natural underground geological sources vs. from anthropogenic sources, only the latter leads to less CO2 in the atmosphere. Even though there are tradeoffs depending on the alternatives being compared, CCS and CO2-EOR are incompatible with one another according to this framing.
System boundaries are relevant when estimating emission levels and considering the impact of CO2 storage on climate change mitigation. When expanding their system boundaries to include refining, transport, and combustion of oil during the project's lifetime, their model shows that CO2-EOR ranges from carbon negative to net positive emissions in the North Sea (Stewart and Haszeldine, 2015). According to Oei and Mendelevitch (2016), even though CO2-EOR could drive CCS projects, subsidizing the fossil fuel industry to advance CCS technology is problematic for emissions reductions. Based on this framing, using captured CO2 in CO2-EOR projects contradicts the climate change mitigation purpose of CCS projects. Therefore, this framing shows that there is some skepticism whether CO2-EOR could contribute to decarbonization. Another concern is uncertainties, and according to Thorne et al. (2020), addressing uncertainties in CO2-EOR processes such as minimizing the amount of leaking CO2 is relevant from a climate change mitigation perspective.
Although this framing is not as strong in the literature review as the first framing, it is more prevalent in social science literature addressing CCS in the context of climate change mitigation more generally without a specific focus on the North Sea region or Norway. Social science literature on CDR outside of the scope of this review emphasize this framing. Carton et al. (2020) show that investing in technologies such as CCS supports the continuation of fossil fuel industries and thereby counters a sustainability transition. McLaren et al. (2021) state that utilizing CO2 such as through EOR could be more economically viable than dedicated geological storage, but EOR is problematic since it contributes to the fossil fuel industry. Furthermore, Asayama (2021) state that carbon capture in CCS projects is often associated with retrofitting power plants or industries using fossil fuels and thereby prolongs carbon lock-in and the fossil fuel regime. In addition, Buck (2018) points out that the main market for captured CO2 is EOR which perpetuates dependence on fossil fuels. At the same time, 80% of global energy supply is from fossil fuels, a level that has been consistent during the past few decades, despite an increase in the renewable energy supply (Ritchie and Roser, 2020). Since there is still an upwards trend in primary energy consumption (apart from 2020 during the COVID pandemic), there is a strong reliance on fossil fuels, even while there is a shift toward more renewable energy. Despite these energy trends, Markard et al. (2021) state that technologies like EOR and CCS could lead to further sustainability challenges by perpetuating unsustainable systems reliant on fossil fuels.
5. Discussion
This section reflects on the scientific literature in this review and discusses how critical social sciences could contribute to a deeper understanding of the socio-political context of CO2 storage in the North Sea region and its impact on climate change mitigation. In their study in Sweden, Nurdiawati and Urban (2022) show that refining companies are the strongest proponents of CCS followed by industries and researchers, while NGOs and politicians are more skeptical. The dominant perspective in this literature review is from the fossil fuel industry, which have a stake in the implementation of CO2-EOR since it is an industrial application that extends fossil fuel extraction.
About one third of the articles in the review merely mention climate change or emissions reductions without any discussion (e.g., Neele et al., 2014; Gruson et al., 2015) or do not mention climate change at all (Ward et al., 2016; Pham and Halland, 2017). These articles instead focus on the economic advantage of implementing CO2-EOR for oil extraction purposes as a motivation for CO2-EOR in geological and engineering feasibility studies that investigate the possibilities for CO2-EOR in the North Sea region. Given the possibilities to position CO2-EOR positively for the fossil fuel industry, it is somewhat surprising that some articles do not mention climate change, but it shows that there are strong economic motivations for CO2-EOR that legitimate such studies. The goal conflicts between CO2-EOR and CCS depend on how the technologies are implemented such as how much CO2 is stored and if negative emissions are also feasible in the CO2 accounting. In addition, even if there are goal conflicts in the short term, there could be synergies in the long term if CO2-EOR contributes to reduced emissions through a faster transition to CCS toward a lower-carbon society.
This literature review shows a lack of literature on social science perspectives when considering the key words used in this study focusing on CO2-EOR in Norway and the North Sea. Only one of the articles is from social sciences, by Mabon and Littlecott (2016) which discusses a “transition” in the North Sea with CO2-EOR as a bridge toward CCS and other climate change mitigation measures. Additional social sciences literature could add new perspectives on the context in which CO2-EOR and CCS technologies are embedded. This study's focus on CO2-EOR in the North Sea region and Norway highlights the relationship between CO2-EOR and CCS in addition to illustrating a social sciences research gap in this area, which has implications for storing CO2 from CDR projects since CO2-EOR can be seen as part of the transition to CCS and dedicated geological storage.
When it comes to studies on BECCS and CDR research more broadly, this social science research gap is also evident. A new report, The State of Carbon Dioxide Removal by Smith et al. (2023) illustrates a research gap in social science studies including empirical studies in a specific geography. Markusson et al. (2020) emphasize the importance of such geographical studies to understand impacts of CDR in different places in addition to aspects of politics and justice. Similarly, in their review article, Carton et al. (2023) state that more empirically focused cases are relevant to prevent mitigation deterrence, which refers to substituting short-term mitigation measures with CDR, but empirical cases could contribute to reducing any delays in mitigating climate change. Besides a lack of empirical studies with a focus on a particular geography in CDR research which often addresses overarching questions, there also seems to be a lack of media coverage and scientific debate on the storage of CO2 (Hansson et al., 2022). An analysis by Hansson et al. (2022) shows that there is less critique in the public debate on geological storage after 2014, and the authors conclude that critical social sciences could contribute new insights on environmental challenges such as CO2 storage.
In addition to the lack of empirical case studies and media coverage related to CO2 storage, half of the literature in this review is concentrated in two journals: Energy Procedia (10 articles) and the International Journal of Greenhouse Gas Control (7 articles). This shows that the topic of CO2-EOR in the North Sea region and Norway is not widespread outside of certain disciplines that are most apt to publish in these two journals.
Even though the articles in this study focus on conventional CCS, it is surprising that most articles do not mention BECCS since they are usually discussed in tandem in the literature. Only one article in this review mentions conducting CCS on biogenic sources in a study on a waste-to-energy plant that would include both fossil and biogenic emissions (Roussanaly et al., 2020). This could partly be since the research community and IPCC scientists' focus on BECCS is new in recent years, as shown by the IPCC (2018) special report on 1.5°C. In addition, the large point sources of biogenic CO2 emissions do not occur in the North Sea countries but rather take place in Sweden and Finland in the Nordic context (Rodriguez et al., 2021) which is outside of the geographical region of this literature review. At the same time, Norway has some point sources of biogenic emissions from waste incineration just like Sweden, and there are ongoing projects to implement BECCS in both countries. Although the CO2-EOR literature in the North Sea and Norway do show that it could contribute as a gateway to CCS, the CO2-EOR literature do not mention acting as a bridge to BECCS. Another possible reason that literature on CO2-EOR focus on CCS but not BECCS is that the EU ETS considers emissions from fossil sources but not from biogenic sources (EC, 2020; Rickels et al., 2021). Even though the literature on CO2-EOR in the North Sea and Norway usually does not include BECCS, there could be overlaps in actors and infrastructures such as for transporting CO2, so there could be a benefit to including BECCS in the narrative of CO2-EOR research to understand the broader context and possible carbon lock-in.
The literature on CDR and net emissions is separate from the body of literature on EOR and CCS studied in this literature review, even though these two bodies of literature have complementary analyses and deal with infrastructures that may be integrated. The literature in this review and the CDR literature show that there are economic barriers to scaling up these technologies for climate change mitigation, in addition to different ways of accounting for emissions. For example, Carton et al. (2020) state that net emissions accounting is socially constructed and politically designed by the UNFCCC, and there are inconsistencies in how emissions are counted. Therefore, they argue that alternative ways of understanding emissions are needed. Stuart et al. (2020) state that when accounting for CO2 emissions, CO2-EOR does not consider the additional CO2 emissions in the lifecycle of the extracted fossil fuels. Brauers et al. (2021) suggest accounting for lock-in when taking decisions in the context of energy transitions to balance climate goals with energy security in the long term. Another issue is double counting the CO2 emissions reduced in both CCS and CO2-EOR systems (Muffett and Feit, 2019). Even though there are challenges regarding policies and emissions calculations when integrating carbon capture and EOR systems, Cooney et al. (2015) show that using CO2 from anthropogenic sources in EOR could contribute to emissions reductions in comparison with using CO2 from natural geological sources. Therefore, whether CO2-EOR reduces emissions depends on the source of CO2 utilized and system boundaries (Cooney et al., 2015).
Storing CO2 via EOR could lead to less urgency for the fossil fuel industry to decrease its operations, thereby strengthening the fossil fuel regime despite climate change concerns. Within the Nordic context, the Swedish Government, which is one of very few that explicitly addresses this concern, has stated that it is against contributing to EOR and perpetuating the fossil fuel industry (SOU, 2020). This shows that there are political preferences against EOR, even if CO2 is eventually stored. Endres et al. (2016) describe the framing shift from CCS to CCUS and its implications for climate change when CO2 is viewed as a commodity for industrial processes such as EOR. This framing legitimates the future existence of fossil fuel companies, even though there are inconsistencies in how the terms CCUS and CCS are used which causes ambiguity (Olfe-Kräutlein et al., 2022). Palm and Nikoleris (2020) show that there are contrasting expectations on CO2-EOR depending on if fossil fuels are framed as the problem or if GHG emissions are considered the main concern. There seems to be a preference for the latter framing in CCS projects. In fact, most countries with CCS projects are also major fossil fuel producers, including Canada, USA, and Norway (Reiner, 2016), and at least 85% of CCS projects involve fossil fuel companies as an affiliate or funder (Chalmin, 2021). Rauter (2022) points to the paradox that Norway is both an exporter of fossil fuels and a progressive climate change leader at the same time with a national electricity mix which relies on hydropower. This means that Norway economically benefits from the fossil fuel industry and may also gain financially when CO2 is returned for storage.
Social acceptance is discussed in the social sciences literature on CCS. Stakeholders often have concerns about the feasibility of large-scale technologies such as CCS (Bellamy et al., 2022). Yet, public or social acceptance is only discussed in two of the articles in this review (Geske et al., 2015a; Mabon and Littlecott, 2016). Hansson et al. (2022) hypothesize that there could be less focus on the question of storage when it is offshore, such as in the North Sea context, rather than onshore where there have historically been more public concerns, but the climate aspect of storage is still relevant. Furthermore, this lack of public concern or engagement in the question of offshore storage could lead to less public pressure on actors to handle storage in a way that is safe and prioritizes the climate change benefit of long-term CO2 storage (Hansson et al., 2022). Although there may be less public concern about offshore storage, there could be concerns that are specific to the North Sea regional context. For example, Merk et al. (2022) studies the social acceptability of CCS in Europe and finds that there could be acceptance challenges regarding the storage of imported CO2 in the Norwegian North Sea. This shows that perspectives from stakeholders are relevant to understand the feasibility of storing CO2 in a European context, especially in the case of international collaborations and transport across country borders.
6. Conclusions
Growing interest in CO2 storage in Europe, including in the Nordics warrants suitable and economically feasible storage facilities that could contribute to decreasing GHG in the atmosphere. This study focuses on how CCS is represented in the scientific literature on CO2-EOR in the North Sea and Norway. This is a specific case to study possible overlaps between these two technologies since CO2-EOR does not take place on a large scale anywhere in Europe, and there are few storage sites for CCS in Europe, with Norway taking the lead with two storage sites in operation and new sites developing through the Northern Lights project. There are five emerging themes in the literature which are: climate change; economics; monitoring, safety, and leakage; geology; and transportation. Two polarized framings on the relationship between CO2-EOR and CCS are illustrated in the results. The first framing focuses on synergies between CO2-EOR and CCS whereby CO2-EOR is framed as a gateway to storing CO2. This is due to economics and financial arguments such as opportunities to share and scale up infrastructure to transport and store CO2, knowledge sharing about geology and monitoring practices, and possibilities to store CO2 in geological formations. The second framing, however not as prevalent in the review, is that there are incompatibilities between CO2-EOR and CCS including goal conflicts between the two technologies. This includes that EOR and CCS have different underlying main purposes, EOR perpetuates the fossil fuel infrastructure which counteracts societal and industrial transitions and possibly also climate change mitigation. Although the two framings identified in this study serve as a starting point to discuss the possible role of CO2-EOR and CCS in the context of sustainability transitions and carbon lock-in, this literature review also shows that there are several research gaps that could be addressed to create a deeper understanding of the socio-political context and how it relates to the relationship between these two technologies.
This literature review highlights several tensions and areas where critical social sciences research could contribute to understanding, or at least initiate more critical discussions, of potential future pathways for CO2 storage as a climate change mitigation measure. First, this literature review shows that knowledge on CO2-EOR in the North Sea is driven by fossil fuel industry research, with a techno-economic focus. Second, most of this body of research on CO2-EOR is not critical toward the phenomenon of EOR that perpetuates the production and consumption of fossil fuels or draw narrow system boundaries when analyzing the phenomenon. Third, most EOR research in the North Sea focuses on reducing emissions while considering fossil fuels as part of a sustainability transition, without taking the starting point that fossil fuels are the underlying problem causing climate change. Fourth, the body of research on EOR in the North Sea is separate from the literature on CDR which includes research on CCS and BECCS from critical social science perspectives, and Hansson et al. (2022) also highlight that the BECCS literature with few exceptions omits analysis of geological storage. This means that there are more opportunities for critical social science research on geological storage, including analytical integration of different research disciplines. Furthermore, an understanding of the impact of CCS and BECCS on climate and energy presupposes that research on EOR and CDR communicate with each other, such as by publishing in the same journals and by initiating critical social sciences research at the crossroads between CO2-EOR and CCS.
This article shows that the current system where EOR is cheaper for storing and handling CO2 than having dedicated storage is highly complex and that changing tax rates is not enough to change how the system works to benefit climate change mitigation. Even though EOR is driven by an economic incentive for the fossil fuel industry in a society that perpetuates the fossil fuel regime, the framings in this review highlight different viewpoints on how compatible EOR is in a future sustainability transition in Norway, which also has implications for other countries that are interested in storage options in the North Sea region, including the UK, the Netherlands, Sweden, Denmark, Ireland, France, and Belgium (Global CCS Institute, 2022). Social science research on geological storage could also benefit CCS, BECCS, DACCS, and CCUS initiatives in Europe, including opportunities for scaling up storage. For example, there is proposed geological storage in the North Sea off the coast of the UK totaling up to 26 Mtpa through Humber Zero and Zero Carbon Humber (Global CCS Institute, 2022). In Denmark, Project Greensand plans to store CO2 in the North Sea through a state-supported initiative with the capacity of 8 Mtpa by 2030 (Project Greensand, 2023). In the Netherlands, there are a few ongoing CCS projects including Aramis, @Antwerp, and Porthos with at least one of these projects planning to ship CO2 for storage in Norway via Northern Lights while domestic storage opportunities in the Dutch North Sea are explored (CCUS Hub, 2023).
The absence of social science literature on geological storage in the North Sea point to opportunities for new research perspectives on CO2-EOR and CCS, e.g., to challenge or empirically validate key concepts like carbon lock-in by testing or operationalizing them in concrete geographical contexts. This could contribute to navigating future pathways for the geological storage in more sustainable directions. Demand for CO2 storage capacity demands more social science research to ensure that CO2 storage from CCS and BECCS initiatives is deployed in a way that is sustainable from long-term societal and environmental perspectives and contributes to net CDR to meet climate change mitigation goals. In addition, techno-economic studies can be furthered by including qualitative social science perspectives to understand embedded socio-political aspects, expand system boundaries, and contribute to social acceptance. Therefore, there are opportunities for both techno-economic studies and critical social sciences to expand their research areas within CDR to impact future sustainability transitions.
When considering the urgency to mitigate climate change in society by reducing GHG emissions, CCS could reduce the fossil fuel industry's impact on the atmosphere, although there are different perspectives on how this benefit is perceived and under what conditions CCS supports a sustainable transition. CCS is often considered as an add-on technology for the capture part of the value chain when applied to an existing industrial facility or power plant, while the transport, injection, and storage aspects of the value chain are new, and there could be synergies for industrial integration with CO2-EOR, both in the North Sea region and in other geographies. Most of the literature in this review show that CO2-EOR and CCS are handled as two operational processes that can be integrated side-by-side with some regulatory and tax adjustments, without highlighting scientific literature that challenges these arguments. Therefore, an integrated systems perspective could contribute by critically challenging the feasibility of these systems, such as addressing a shortcoming that synergies between CO2-EOR and CCS are viewed from an idealized market perspective without prioritizing climate change mitigation. Future pathways that implement CO2 storage will contribute to CDR methods, and the type of CO2 storage method applied may lead to different levels of CO2 reduction and impacts on climate change mitigation.
Author contributions
The author confirms being the sole contributor of this work and has approved it for publication.
Funding
This work has been carried out within the scope of the Graduate School in Energy Systems (FoES) (46036-1) financed by the Swedish Energy Agency.
Acknowledgments
I would like to thank my supervisors Anders Hansson, Simon Haikola, and Stefan Grönkvist for supporting the development of this text. I am also grateful to the Linköping University Negative Emission Technologies (LUNETS) seminar group for feedback on an earlier draft.
Conflict of interest
The author declares that the research was conducted in the absence of any commercial or financial relationships that could be construed as a potential conflict of interest.
Publisher's note
All claims expressed in this article are solely those of the authors and do not necessarily represent those of their affiliated organizations, or those of the publisher, the editors and the reviewers. Any product that may be evaluated in this article, or claim that may be made by its manufacturer, is not guaranteed or endorsed by the publisher.
References
Akerboom, S., Waldmann, S., Mukherjee, A., Agaton, C., Sanders, M., and Kramer, G. J. (2021). Different this time? The prospects of CCS in the Netherlands in the 2020s. Front. Energy Res. 9, 644796. doi: 10.3389/fenrg.2021.644796
Al-Masri, W., Papaspyrou, C., Shapiro, A., and Suicmez, V. S. (2018). “Study of the feasibility of the carbon dioxide injection in a North Sea Petroleum Reservoir,” in: Society of Petroleum Engineers - SPE Europec Featured at 80th EAGE Conference and Exhibition 2018 (Copenhagen), 1271–1286.
Asayama, S. (2021). The Oxymoron of Carbon Dioxide Removal: Escaping Carbon Lock-In and yet Perpetuating the Fossil Status Quo? Front. Clim. 3, 673515. doi: 10.3389/fclim.2021.673515
Bellamy, R., Chilvers, J., Pallett, H., and Hargreaves, T. (2022). Appraising sociotechnical visions of sustainable energy futures: a distributed deliberative mapping approach. Energy Res. Soc. Sci. 85, 102414. doi: 10.1016/j.erss.2021.102414
Bergmo, P. E. S., Grimstad, A.-A., and Kurtev, K. (2018). Mapping of paleo residual oil zones on the NCS and the potential for production by CO2-EOR. Int. J. Greenhouse Gas Cont. 75, 254–261. doi: 10.1016/j.ijggc.2018.06.005
Bonto, M., Welch, M. J., Lüthje, M., Andersen, S. I., Veshareh, M. J., Amour, F., et al. (2021). Challenges and enablers for large-scale CO2 storage in chalk formations. Earth-Sci. Rev. 222, 103826. doi: 10.1016/j.earscirev.2021.103826
Brauers, H., Braunger, I., and Jewell, J. (2021). Liquefied natural gas expansion plans in Germany: The risk of gas lock-in under energy transitions. Energy Res. Soc. Sci. 76, 102059. doi: 10.1016/j.erss.2021.102059
Buck, H. J. (2018). The politics of negative emissions technologies and decarbonization in rural communities. Global Sustain. 1, 1–7. doi: 10.1017/sus.2018.2
Carpenter, S. M., and Koperna, G. (2014). Development of the first internationally accepted standard for geologic storage of carbon dioxide utilizing enhanced oil recovery (EOR) under the international standards organization (ISO) technical committee TC-265. Energy Proc. 63, 6717–6729. doi: 10.1016/j.egypro.2014.11.707
Carton, W., Asiyanbi, A., Beck, S., Buck, H. J., and Lund, J. F. (2020). Negative emissions and the long history of carbon removal. WIREs Climate Change 11, 1–25. doi: 10.1002/wcc.671
Carton, W., Hougaard, I. M., Markusson, N., and Lund, J. F. (2023). Is carbon removal delaying emission reductions? WIREs Climate Change. e826, 1–18. doi: 10.1002/wcc.826
Cavanagh, A., and Ringrose, P. (2014). Improving oil recovery and enabling CCS: a comparison of offshore gas-recycling in Europe to CCUS in North America. Energy Proc. 63, 7677–7684. doi: 10.1016/j.egypro.2014.11.801
CCUS Hub (2023). Available online at: https://ccushub.ogci.com (accessed March 27, 2023).
Chailleux, S. (2019). Making the subsurface political: How enhanced oil recovery techniques reshaped the energy transition. Environ. Plann. C Polit. Space 38, 733–750. doi: 10.1177/2399654419884077
Chalmin, A. (2021). Fossil Fuel Industry and Investments in CCS and CCUS. Geoengineering Monitor. Available online at: https://www.geoengineeringmonitor.org/2021/11/fossil-fuel-industry-and-investments-in-ccs-ccus/ (accessed January 17, 2022).
Compernolle, T., Welkenhuysen, K., Huisman, K., Piessens, K., and Kort, P. (2017). Off-shore enhanced oil recovery in the North Sea: the impact of price uncertainty on the investment decisions. Energy Policy 101, 123–137. doi: 10.1016/j.enpol.2016.11.034
Cooney, G., Littlefield, J., Marriott, J., and Skone, T. J. (2015). Evaluating the climate benefits of CO2-enhanced oil recovery using life cycle analysis. Environ. Sci. Technol. 49, 7491–7500. doi: 10.1021/acs.est.5b00700
Cox, E. M., Pidgeon, N., Spence, E., and Thomas, G. (2018). Blurred lines: the ethics and policy of greenhouse gas removal at scale. Front. Environ. Sci. 6, 1–7. doi: 10.3389/fenvs.2018.00038
EC (2021). EU Emissions Trading System (EU ETS). European Commission. Available online at: https://ec.europa.eu/clima/eu-action/eu-emissions-trading-system-eu-ets_en (accessed March 9, 2022).
EC (2023). Northern Lights Project – A Commercial CO2 Cross-Border Transport Connection Project Between Several European Capture Initiatives (United Kingdom, Ireland, Belgium, the Netherlands, France, Sweden) and Transport the Captured CO2 by Ship to a Storage Site on the Norwegian Continental Shelf. Brussels: European Commission.
Edwards, R. W. J., and Celia, M. A. (2018). Infrastructure to enable deployment of carbon capture, utilization, and storage in the United States. Proc. Natl. Acad. Sci. U. S. A. 115, E8815–E8824. doi: 10.1073/pnas.1806504115
Eggleston, H. (2006). “Estimation of emissions from CO2 capture and storage: the 2006,” in IPCC Guidelines for National Greenhouse Gas Inventories. Presentation at the UNFCCC workshop on carbon dioxide capture and storage 2006 (Bonn).
Eide, L. I., Batum, M., Dixon, T., Elamin, Z., Graue, A., Hagen, S., et al. (2019). Enabling large-scale carbon capture, utilisation, and storage (CCUS) using offshore carbon dioxide (CO2) infrastructure developments—a review. Energies 12, 945. doi: 10.3390/en12101945
Elmabrouk, S. K., Bader, H. E., and Mahmud, W. M. (2017). “An overview of power plant CCS and CO2-EOR projects,” in International Conference on Industrial Engineering and Operations Management (Rabat).
Endres, D., Cozen, B., O'Byrne, M., Feldpausch-Parker, A. M., and Peterson, T. R. (2016). Putting the U in carbon capture and storage: rhetorical boundary negotiation within the CCS/CCUS scientific community. J. Appl. Commun. Res. 44, 362–380. doi: 10.1080/00909882.2016.1225160
Entman, R. M. (1993). Framing: towards clarification of a fractured paradigm. J. Commun. 43, 51–58. doi: 10.1111/j.1460-2466.1993.tb01304.x
EU (2009a). Directive 2009/29/EC of the European Parliament and of the Council of 23 April 2009 Amending Directive 2003/87/EC so as to Improve and Extend the Greenhouse Gas Emission Allowance Trading Scheme of the Community, Oj L 140. Brussels: The European Union.
EU (2009b). Directive 2009/31/EC on the Geological Storage of Carbon Dioxide and Amending Council Directive 85/337/EEC, European Parliament and Council Directives 2000/60/EC, 2001/80/EC, 2004/35/EC, 2006/12/EC, 2008/1/EC And Regulation (EC) No 1013/2006, Oj L 140. Brussels: The European Union.
EU (2015). Regulation (EU) 2015/757 of the European Parliament and of the Council of 29 April 2015 on the Monitoring, Reporting and Verification of Carbon Dioxide Emissions from Maritime Transport, and Amending Directive 2009/16/EC, Oj L 123. Brussels: The European Union.
Fajardy, M., Patrizio, P., Daggash, H. A., and Mac Dowell, N. (2019). Negative emissions: priorities for research and policy design. Front. Clim. 1, e00006. doi: 10.3389/fclim.2019.00006
Geske, J., Berghout, N., and Van Den Broek, M. (2015a). Cost-effective balance between CO2 vessel and pipeline transport. Part I – Impact of optimally sized vessels and fleets. Int. J. Greenhouse Gas Control 36, 175–188. doi: 10.1016/j.ijggc.2015.01.026
Geske, J., Berghout, N., and Van Den Broek, M. (2015b). Cost-effective balance between CO2 vessel and pipeline transport: Part II – Design of multimodal CO2 transport: the case of the West Mediterranean region. Int. J. Greenhouse Gas Control 33, 122–134. doi: 10.1016/j.ijggc.2014.12.005
Ghanbari, S., Mackay, E. J., and Pickup, G. E. (2016). Comparison of CO2-EOR performance between offshore and onshore environments. Offshore Technol. Conf. Asia. 2016, 762–776. doi: 10.4043/26590-MS
Global CCS Institute (2022). Global Status of CCS 2021: CCS Accelerating to Net Zero. Melbourne, VIC: Global CCS Institute.
Godec, M. L., Kuuskraa, V. A., and Dipietro, P. (2013). Opportunities for using anthropogenic CO2 for enhanced oil recovery and CO2 storage. Energy Fuels 27, 4183–4189. doi: 10.1021/ef302040u
Gough, C., and Mander, S. (2019). Beyond social acceptability: applying lessons from CCS social science to support deployment of BECCS. Curr. Sustain. Renew. Energy Rep. 6, 116–123. doi: 10.1007/s40518-019-00137-0
Grubler, A., Wilson, C., and Nemet, G. (2016). Apples, oranges, and consistent comparisons of the temporal dynamics of energy transitions. Energy Res. Soc. Sci. 22, 18–25. doi: 10.1016/j.erss.2016.08.015
Gruson, J. F., Serbutoviez, S., Delprat-Jannaud, F., Akhurst, M., Nielsen, C., Dalhoff, F., et al. (2015). Techno-economic assessment of four CO2 storage sites. Oil Gas Sci. Technol. Revue d'IFP Energies nouvelles 70, 753–766. doi: 10.2516/ogst/2014057
Gunderson, R., Stuart, D., and Petersen, B. (2020). The fossil fuel industry's framing of carbon capture and storage: faith in innovation, value instrumentalization, and status quo maintenance. J. Clean. Prod. 252, 1–9. doi: 10.1016/j.jclepro.2019.119767
Hansson, A., Anshelm, J., Fridahl, M., and Haikola, S. (2022). The underworld of tomorrow? How subsurface carbon dioxide storage leaked out of the public debate. Energy Res. Soc. Sci. 90, 102606. doi: 10.1016/j.erss.2022.102606
Hansson, A., and Bryngelsson, M. (2009). Expert opinions on carbon dioxide capture and storage—A framing of uncertainties and possibilities. Energy Policy 37, 2273–2282. doi: 10.1016/j.enpol.2009.02.018
Harrison, B., and Falcone, G. (2013). Deciding whether to fund either CCS or CCUS offshore projects: are we comparing apples and pears in the North Sea? Proc. SPE Ann. Techn. Conf. Exhibition 5, 3681–3690. doi: 10.2118/166388-MS
Hill, B., Hovorka, S., and Melzer, S. (2013). Geologic carbon storage through enhanced oil recovery. Energy Proc. 37, 6808–6830. doi: 10.1016/j.egypro.2013.06.614
IMO (2019). Resolution LP.5(14) on the Provisional Application of the 2009. Amendment to Article 6 of the London Protocol. London: International Maritime Organization.
IPCC (2018). “Global warming of 1.5°C,” in An IPCC Special Report on the Impacts of Global Warming of 1.5°C Above Pre-industrial Levels and Related Global Greenhouse Gas Emission Pathways, in the Context of Strengthening the Global Response to the Threat of Climate Change, Sustainable Development, and Efforts to Eradicate Poverty, eds V. Masson-Delmotte, P. Zhai, H.-O. Pörtner, D. Roberts, J. Skea, P.R. Shukla, A. Pirani, W. Moufouma-Okia, C. Péan, R. Pidcock, S. Connors, J.B.R. Matthews, Y. Chen, X. Z., M.I. Gomis, E. Lonnoy, T. Maycock, M. Tignor, and T. Waterfield (Cambridge: Cambridge University Press).
IPCC (2022). “Climate change 2022: mitigation of climate change,” in Contribution of Working Group III to the Sixth Assessment Report of the Intergovernmental Panel on Climate Change, eds P. R. Shukla, J. S. R. Slade, A. Al Khourdajie, R. Van Diemen, D. Mccollum, M. Pathak, S. Some, P. Vyas, R. Fradera, M. Belkacemi, A. Hasija, G. Lisboa, S. Luz, J. Malley (Cambridge; New York, NY: Cambridge University Press).
IPCC (2023). Synthesis Report of the IPCC Sixth Assessment Report (AR6): Summary for Policymakers. Cambridge; New York, NY: Cambridge University Press.
Jakobsen, J., Roussanaly, S., and Anantharaman, R. (2017). A techno-economic case study of CO2 capture, transport and storage chain from a cement plant in Norway. J. Clean. Prod. 144, 523–539. doi: 10.1016/j.jclepro.2016.12.120
Kapteijn, P. (2010). Energy and environment: the ultimate test of EandP “Intelligence”. SPE Intellig. Energy Conf. Exhib. 2, 1245–1248. doi: 10.2118/128999-MS
Karimaie, H., Nazarian, B., Aurdal, T., Nøkleby, P. H., and Hansen, O. (2017). Simulation study of CO2 EOR and storage potential in a North Sea reservoir. Energy Proc. 114, 7018–7032. doi: 10.1016/j.egypro.2017.03.1843
Kemp, A. G., and Kasim, S. (2013). The economics of CO2-EOR cluster developments in the UK Central North Sea. Energy Policy 62, 1344–1355. doi: 10.1016/j.enpol.2013.07.047
Lefvert, A., Rodriguez, E., Fridahl, M., Grönkvist, S., Haikola, S., and Hansson, A. (2022). What are the potential paths for carbon capture and storage in Sweden? A multi-level assessment of historical and current developments. Energy Res. Soc. Sci. 87, 102452. doi: 10.1016/j.erss.2021.102452
Loria, P., and Bright, M. B. H. (2021). Lessons captured from 50 years of CCS projects. Electr. J. 34, 106998. doi: 10.1016/j.tej.2021.106998
Lux, B., Schneck, N., Pfluger, B., Männer, W., and Sensfus, F. (2023). Potentials of direct air capture and storage in a greenhouse gas-neutral European energy system. Energy Strat. Rev. 45, 101012. doi: 10.1016/j.esr.2022.101012
Mabon, L., and Littlecott, C. (2016). Stakeholder and public perceptions of CO2 -EOR in the context of CCS – Results from UK focus groups and implications for policy. Int. J. Greenhouse Gas Control 49, 128–137. doi: 10.1016/j.ijggc.2016.02.031
Magnusson, D., Sperling, K., Veenman, S., and Oteman, M. (2021). News media framing of grassroots innovations in Denmark, the Netherlands and Sweden. Environ. Commun. 15, 641–662. doi: 10.1080/17524032.2021.1880460
Markard, J., Raven, R., and Truffer, B. (2012). Sustainability transitions: an emerging field of research and its prospects. Res. Policy 41, 955–967. doi: 10.1016/j.respol.2012.02.013
Markard, J., Van Lente, H., Wells, P., and Yap, X.-S. (2021). Neglected developments undermining sustainability transitions. Environ. Innov. Soc. Trans. 41, 39–41. doi: 10.1016/j.eist.2021.10.012
Markusson, N., Balta-Ozkan, N., Chilvers, J., Healey, P., Reiner, D., and McLaren, D. (2020). Social science sequestered. Front. Clim. 2, e00002. doi: 10.3389/fclim.2020.00002
Markusson, N., Ishii, A., and Stephens, J. C. (2011). The social and political complexities of learning in carbon capture and storage demonstration projects. Global Environ. Change 21, 293–302. doi: 10.1016/j.gloenvcha.2011.01.010
Mathisen, A., and Skagestad, R. (2017). Utilization of CO2 from emitters in Poland for CO2-EOR. Energy Proced. 114, 6721–6729. doi: 10.1016/j.egypro.2017.03.1802
Mazzetti, M. J., Skagestad, R., Mathisen, A., and Eldrup, N. H. (2014). CO2 from natural gas sweetening to kick-start EOR in the North Sea. Energy Proced. 63, 7280–7289. doi: 10.1016/j.egypro.2014.11.764
McLaren, D., Willis, R., Szerszynski, B., Tyfield, D., and Markusson, N. (2021). Attractions of delay: Using deliberative engagement to investigate the political and strategic impacts of greenhouse gas removal technologies. Environ. Plan. E Nat. Space. 1–22. doi: 10.1177/25148486211066238
Megura, M., and Gunderson, R. (2022). Better poison is the cure? Critically examining fossil fuel companies, climate change framing, and corporate sustainability reports. Energy Res. Soc. Sci. 85, 102388. doi: 10.1016/j.erss.2021.102388
Mendelevitch, R. (2014). The role of CO2-EOR for the development of a CCTS infrastructure in the North Sea Region. Int. J. Greenhouse Gas Control 20, 132–159. doi: 10.1016/j.ijggc.2013.11.007
Merk, C., Nord,ø, Å. D., Andersen, G., Lægreid, O. M., and Tvinnereim, E. (2022). Don't send us your waste gases: public attitudes toward international carbon dioxide transportation and storage in Europe. Energy Res. Soc. Sci. 87, 102450. doi: 10.1016/j.erss.2021.102450
Muffett, C., and Feit, S. (2019). Fuel to the Fire: How Geoengineering Threatens to Entrench Fossil Fuels and Accelerate the Climate Crisis. Geneva: Center for International Environmental Law.
Neele, F., Haugen, H. A., and Skagestad, R. (2014). Ship transport of CO2 – breaking the CO2-EOR deadlock. Energy Proc. 63, 2638–2644. doi: 10.1016/j.egypro.2014.11.286
Negrescu, M. (2008). Economic modeling of an oil and gas project involving carbon capture and storage: Snøhvit LNG field (Barents Sea, Norway). SPE Projects Facil. Construct. 3, 1–15. doi: 10.2118/107430-PA
Northern Lights (2023). Northern Lights. Available online at: https://norlights.com (accessed March 27, 2023).
Núñez-López, V., and Moskal, E. (2019). Potential of CO2-EOR for near-term decarbonization. Front. Clim. 1, e00005. doi: 10.3389/fclim.2019.00005
Nurdiawati, A., and Urban, F. (2022). Decarbonising the refinery sector: a socio-technical analysis of advanced biofuels, green hydrogen and carbon capture and storage developments in Sweden. Energy Res. Soc. Sci. 84, 102358. doi: 10.1016/j.erss.2021.102358
Oei, P.-Y., and Mendelevitch, R. (2016). European scenarios of CO2 infrastructure investment until 2050. Energy J. 37, 171–194. doi: 10.5547/01956574.37.SI3.poei.OJEA
Olfe-Kräutlein, B., Armstrong, K., Mutchek, M., Cremonese, L., and Sick, V. (2022). Why terminology matters for successful rollout of carbon dioxide utilization technologies. Front. Clim. 4, 830660. doi: 10.3389/fclim.2022.830660
Palm, E., and Nikoleris, A. (2020). Conflicting expectations on carbon dioxide utilisation. Technol. Anal. Strat. Manag. 33, 217–228. doi: 10.1080/09537325.2020.1810225
Pham, V., and Halland, E. (2017). Perspective of CO2 for storage and enhanced oil recovery (EOR) in Norwegian North Sea. Energy Proc. 114, 7042–7046. doi: 10.1016/j.egypro.2017.03.1845
Project Greensand (2023). Available online at: https://www.projectgreensand.com/en/hvad-er-project-greensand (accessed March 27, 2023).
Raffa, P. (2021). Where is research on fossil fuels going in times of climate change? A perspective on chemical enhanced oil recovery. MRS Commun. 11, 716–725. doi: 10.1557/s43579-021-00131-y
Rauter, A. R. K. K. (2022). Elite energy transitions: leaders and experts promoting renewable energy futures in Norway. Energy Res. Soc. Sci. 88, 102509. doi: 10.1016/j.erss.2022.102509
Reiner, D. (2016). Learning through a portfolio of carbon capture and storage demonstration projects. Nature Energy 1, 7. doi: 10.1038/nenergy.2015.11
Rickels, W., Proels, A., Geden, O., Burhenne, J., and Fridahl, M. (2021). Integrating carbon dioxide removal into European emissions trading. Front. Clim. 3, 690023. doi: 10.3389/fclim.2021.690023
Ritchie, H., and Roser, M. (2020). Energy. Published online at OurWorldInData.org. Available online at: https://ourworldindata.org/energy (accessed March 31, 2022).
Rodriguez, E., Lefvert, A., Fridahl, M., Grönkvist, S., Haikola, S., and Hansson, A. (2021). Tensions in the energy transition: Swedish and Finnish company perspectives on bioenergy with carbon capture and storage. J. Clean. Prod. 280, 124527. doi: 10.1016/j.jclepro.2020.124527
Roefs, P., Moretti, M., Welkenhuysen, K., Piessens, K., and Compernolle, T. (2019). CO2-enhanced oil recovery and CO2 capture and storage: an environmental economic trade-off analysis. J. Environ. Manage 239, 167–177. doi: 10.1016/j.jenvman.2019.03.007
Roussanaly, S., Ouassou, J. A., Anantharaman, R., and Haaf, M. (2020). Impact of uncertainties on the design and cost of CCS from a waste-to-energy plant. Front. Energy Res. 8, e00017. doi: 10.3389/fenrg.2020.00017
Schenuit, F., Colvin, R., Fridahl, M., Mcmullin, B., Reisinger, A., Sanchez, D. L., et al. (2021). Carbon dioxide removal policy in the making: assessing developments in 9 OECD cases. Front. Clim. 3, e638805. doi: 10.3389/fclim.2021.638805
Shogenova, A., Piessens, K., Holloway, S., Bentham, M., Martínez, R., Flornes, K. M., et al. (2014). Implementation of the EU CCS directive in Europe: results and development in 2013. Energy Proc. 63, 6662–6670. doi: 10.1016/j.egypro.2014.11.700
Smith, S. M., Geden, O., Nemet, G., Gidden, M., Lamb, W. F., Powis, C., et al. (2023). The State of Carbon Dioxide Removal - 1st Edition. The State of Carbon Dioxide Removal.
Snyder, H. (2019). Literature review as a research methodology: an overview and guidelines. J. Bus. Res. 104, 333–339. doi: 10.1016/j.jbusres.2019.07.039
SOU (2020). The Pathway to a Climate- Positive Future – Strategy and Action Plan for Achieving Negative Greenhouse Gas Emissions After 2045. [Vägen till en klimatpositiv framtid], eds Governmental Inquiry [Statens Offentliga Utredningar] (Stockholm: Government Offices [Regeringskansliet]).
Steeneveldt, R., Berger, B., and Torp, T. A. (2006). CO2 capture and storage. Chem. Eng. Res. Design 84, 739–763. doi: 10.1205/cherd05049
Stewart, R. J., and Haszeldine, R. S. (2015). Can producing oil store carbon? Greenhouse gas footprint of CO2EOR, offshore North Sea. Environ. Sci. Technol. 49, 5788–5795. doi: 10.1021/es504600q
Stuart, D., Gunderson, R., and Peterson, B. (2020). Carbon geoengineering and the metabolic rift: solution or social reproduction? Crit. Sociol. 46, 1233–1249. doi: 10.1177/0896920520905074
Suicmez, V. S. (2019). Feasibility study for carbon capture utilization and storage (CCUS) in the Danish North Sea. J. Nat. Gas Sci. Eng. 68, 102924. doi: 10.1016/j.jngse.2019.102924
Thorne, R. J., Sundseth, K., Bouman, E., Czarnowska, L., Mathisen, A., Skagestad, R., et al. (2020). Technical and environmental viability of a European CO2 EOR system. Int. J. Greenhouse Gas Control 92, 102857. doi: 10.1016/j.ijggc.2019.102857
Trading Economics (2023). EU Carbon Permits. Available online at: https://tradingeconomics.com/commodity/carbon (accessed February 2, 2023).
Tzimas, E., Georgakaki, A., Garcia Cortes, C., and Peteves, S. D. (2005). Enhanced Oil Recovery using Carbon Dioxide in the European Energy System. Petten: Institute for Energy.
Unruh, G. (2000). Understanding carbon lock-in. Energy Policy 28, 817–830. doi: 10.1016/S0301-4215(00)00070-7
Unruh, G. C., and Carrillo-Hermosilla, J. (2006). Globalizing carbon lock-in. Energy Policy 34, 1185–1197. doi: 10.1016/j.enpol.2004.10.013
Waller, L., Rayner, T., Chilvers, J., Gough, C. A., Lorenzoni, I., Jordan, A., et al. (2020). Contested framings of greenhouse gas removal and its feasibility: Social and political dimensions. WIREs Climate Change 11, 1–17. doi: 10.1002/wcc.649
Ward, N. I. P., Alves, T. M., and Blenkinsop, T. G. (2016). Reservoir leakage along concentric faults in the Southern North Sea: Implications for the deployment of CCS and EOR techniques. Tectonophysics 690, 97–116. doi: 10.1016/j.tecto.2016.07.027
Welkenhuysen, K., Compernolle, T., Piessens, K., Ramírez, A., Rupert, J., and Swennen, R. (2014). Geological uncertainty and investment risk in CO2-enhanced oil recovery. Energy Proced. 63, 7878–7883. doi: 10.1016/j.egypro.2014.11.823
Welkenhuysen, K., Meyvis, B., and Piessens, K. (2017a). A profitability study of CO2-EOR and subsequent CO2 storage in the North Sea under low oil market prices. Energy Proced. 114, 7060–7069. doi: 10.1016/j.egypro.2017.03.1848
Welkenhuysen, K., Meyvis, B., Swennen, R., and Piessens, K. (2018). Economic threshold of CO2-EOR and CO2 storage in the North Sea: a case study of the Claymore, Scott and Buzzard oil fields. Int. J. Greenhouse Gas Control 78, 271–285. doi: 10.1016/j.ijggc.2018.08.013
Welkenhuysen, K., Rupert, J., Compernolle, T., Ramirez, A., Swennen, R., and Piessens, K. (2017b). Considering economic and geological uncertainty in the simulation of realistic investment decisions for CO2-EOR projects in the North Sea. Appl. Energy 185, 745–761. doi: 10.1016/j.apenergy.2016.10.105
Wright, I., Ringrose, P., Mathieson, A., and Eiken, O. (2009). “An overview of active large-scale CO2 storage projects,” in: SPE International Conference on CO2 Capture, Storage, and Utilization 2009 (San Diego, CA). 345–355.
Yara (2022). Available online at: https://www.yara.com/news-and-media/news/archive/news-2022/major-milestone-for-decarbonising-europe/ (accessed Februrary 1, 2023).
Keywords: enhanced oil recovery (EOR), carbon capture and storage (CCS), Norway, North Sea, carbon dioxide storage, sustainability transition, bioenergy with carbon capture and storage (BECCS), carbon dioxide removal (CDR)
Citation: Rodriguez E (2023) Storing carbon dioxide for climate's sake: contradictions and parallels with enhanced oil recovery. Front. Clim. 5:1166011. doi: 10.3389/fclim.2023.1166011
Received: 14 February 2023; Accepted: 02 May 2023;
Published: 18 May 2023.
Edited by:
Miranda Boettcher, German Institute for International and Security Affairs (SWP), GermanyReviewed by:
Nils Matzner, Technical University of Munich, GermanyDanny Otto, Helmholtz Association of German Research Centres (HZ), Germany
Copyright © 2023 Rodriguez. This is an open-access article distributed under the terms of the Creative Commons Attribution License (CC BY). The use, distribution or reproduction in other forums is permitted, provided the original author(s) and the copyright owner(s) are credited and that the original publication in this journal is cited, in accordance with accepted academic practice. No use, distribution or reproduction is permitted which does not comply with these terms.
*Correspondence: Emily Rodriguez, ZW1pbHkucm9kcmlndWV6QGxpdS5zZQ==