- 1California Institute for Energy and Environment (CIEE), University of California, Berkeley, Berkeley, CA, United States
- 2Centre for Environmental Policy, Imperial College London, London, United Kingdom
Given the uncertainty around climate change and the need to design systems that anticipate future needs, risks, and costs or values related to resilience, the current rules-based regulatory and policy frameworks designed for the centralized system of large-scale energy generation and delivery may not be ‘fit for purpose' for smaller scale local installations centered on community microgrids. This research examines regulatory challenges and potential impediments to implementing a multi-customer community-based microgrid in California through discussion of lessons learned in current pilot projects supported in part by initiatives of the California Energy Commission's Electric Program Investment Charge (EPIC). The extent to which regulation has the flexibility to anticipate future needs and risks and support experimentation is evaluated in light of the state's complex and evolving energy system requirements. To illustrate challenges, two case studies of EPIC-supported projects are included. Multiple uncertainties, including future impacts of climate change, energy demands, and advances in technology, highlight the potential need to rethink best approaches to energy regulation. Principles drawn from Resilience Thinking and Anticipatory Regulation are discussed for their potential value in supporting development of new models for community-scale energy production, distribution, and use. Drawing on the experiences of the pilot projects, suggested principles to guide a new regulatory regime specific to microgrids are proposed.
1. Introduction
According to the Intergovernmental Panel on Climate Change (IPCC), the frequency and severity of extreme weather events will likely continue to increase as the earth's climate changes, with significant impacts on the water-energy-food nexus (IPCC, 2021). While this is a challenge globally, decarbonization and strategies of adaptation require action at multiple scales, including the local level (Quandt et al., 2023). In California, the effects of climate change are projected to include increasing average annual daily maximum temperatures, worsening water shortages, and coastal erosion and flooding (Bedsworth et al., 2018). Fire seasons are lengthening, and combustion sparked by aging and failing components of the centralized legacy electrical grid has led to loss of property and lives, adverse health effects, and energy insecurity (Radeloff et al., 2018; Kramer et al., 2019; Goss et al., 2020; O'Neill et al., 2021; Guirguis et al., 2023). This is an important justice issue, as areas with more frequent fires have lower household incomes and home values, and higher proportions of older residents, Native American populations, and undocumented immigrants; underrepresented populations have also been shown to face greater flood risk (Méndez et al., 2020; Masri et al., 2021; Sanders et al., 2023).
To mitigate wildfire risk, California's three largest electric utilities have initiated planned public safety power shutoffs (PSPSs) during heat or extreme weather events, leaving millions without power and leading to significant health, social, and economic impacts (Abatzoglou et al., 2020; Murillo, 2020; Wong-Parodi, 2020; RCRC, 2022). Power disruptions affect interconnected infrastructure, including access to food and water, health and social services, and communication and transportation networks, with particularly severe impacts on vulnerable populations. Planned and unplanned outages highlight the inadequacy of the legacy grid that depends on remote energy production and transmission at long distances to ensure uninterrupted energy access (Guliasi, 2021).
California is currently encouraging research on and development of projects that decentralize energy generation and transmission as one way to add reliability (Hess and Lee, 2020; Ajaz and Bernell, 2021a,b). Microgrid technology is a promising innovation for improving resilience, with potential for community self-sufficiency and control over energy access and management (Wu and Sansavini, 2020; Ajaz and Bernell, 2021a,b). California currently has 69 operational solar-plus-storage microgrids of varying sizes, designs, and purposes (US DOE, 2023). Most of these installations are for specific resilience needs, such as airports, hospitals, universities, agricultural sites, or remote locations. The potential application of the technology to more general community resilience, including in urban environments, is a newer development. Within a community, the resilience needs can be considerably more diverse with wide load variances as there may be health equipment needs of households, refrigeration needs of businesses and residents, battery charging, communications, emergency services, and more. An advantage is that a local energy solution can benefit from identifying and including community knowledge and resources that recognize the unique needs and assets of residents and their environment (Cox, 2023).
While microgrids are a new and developing technology applied to wider contexts, installations and modeling of potential applications show positive results (Anderson et al., 2017). The first community scale microgrid was installed in Bronzeville, Illinois, as a test project for resilience in a mixed income community and continues to operate successfully (Rickerson et al., 2022). In a suburb of Tampa, Florida, a neighborhood scale pilot microgrid serving 37 homes was able to maintain power when Hurricane Ian in 2022 caused other neighboring residences to lose power (Cassels, 2023). In California, after a catastrophic gas leak in Aliso Canyon in Los Angeles County, a nanogrid—a smaller microgrid that can be connected to other small grids—was installed to provide emergency energy services. The system was unexpectedly tested five weeks later when the main grid failed, and the new system provided seven critical hours of power (Lightner et al., 2021). Additionally, studies have modeled potential benefits. In a rural region of Southern California prone to PSPSs, analysis concluded that adding transmission lines or diesel generation capacity was cost prohibitive, and a now functional solar-generated microgrid was installed instead (Cohn, 2021). A study of risk mitigation in Puerto Rico, in the aftermath of the disastrous consequences of Hurricane Maria, concluded that a network of strategically placed microgrids could improve resilience and prevent catastrophic damage to critical infrastructure networks in a future event (Aros-Vera et al., 2021). In another example, a study of a hospital in Chino, California, concluded that implementing a microgrid could significantly improve resilience in case of an emergency (Hervás-Zaragoza et al., 2022).
With potential for generation and storage through solar-plus-storage battery systems1, community microgrids can also help the state meet its decarbonization goals. California is a global leader in decarbonization with incentives for innovation in new technologies and energy decentralization (Ajaz and Bernell, 2021b). The state is pursuing strategies and piloting projects that simultaneously reduce reliance on carbon fuels and lower emissions, but also improve resilience (CARB, 2022). Despite being a critical issue, resilience has only recently been addressed as an explicit goal in microgrid development in California and as of May 2023 definitions of and ways to value resilience are still being developed by the state (Schwartz, 2021).
Given the uncertainty around climate change and the need to design systems that anticipate future needs, risks, and costs or values related to resilience, the current rules-based regulatory and policy frameworks designed for the centralized system of large-scale energy generation and delivery may not be “fit for purpose” for smaller scale local installations centered on community microgrids.
This paper examines regulatory challenges and potential impediments to implementing a multi-customer community-based microgrid through discussion of lessons learned in current pilot projects. The extent to which regulation has the flexibility to anticipate future needs and risks and support experimentation is evaluated in light of California's complex and changing energy system requirements. Multiple uncertainties, including future impacts of climate change, energy demands, and advances in technology, highlight the need to rethink best approaches to energy regulation. To this end, principles drawn from Resilience Thinking and Anticipatory Regulation are discussed for their potential value in supporting development of new models for community-scale energy generation and distribution (Biggs et al., 2015; Armstrong and Rae, 2017; Armstrong et al., 2019).
2. Methodology
Support for pilot projects that test community-scale energy solutions is a key part of California's climate and decarbonization strategy. The Electric Program Investment Charge (EPIC), created under the umbrella of the California Energy Commission (CEC) in 2012, aims to incentivize the development and commercialization of clean energy. The CEC defines the goals of EPIC as to:
• “Expand the use of renewable energy.
• Build a safe and resilient electricity system.
• Advance electric technologies for buildings, businesses, and transportation.
• Enable a more decentralized electric grid.
• Improve the affordability, health, and comfort of California's communities.
• Support California's local economies and businesses” (CEC, n.d.).
This research examines the experiences of pilot projects that received EPIC grants in 2016 to develop innovative models for advanced energy communities (AECs) centered on clean electrical power. Pilot projects were awarded initial funding for a Phase I feasibility study through a competitive challenge grant procedure, with projects sited in geographically and socially diverse communities, including disadvantaged or under-resourced communities. Shared goals included energy resilience and decarbonization, as well as financial viability and sustainability beyond the grant period, but aimed to attract a variety of solutions. Of the 12 AECs submitting final Phase I reports, four subsequently received further support to construct their plans during Phase II (Box 1).
Box 1. EPIC phase I and phase II projects.
• Oakland EcoBlock*
• Lancaster Advanced Energy Community*
• Richmond Advanced Energy Community*
• Bassett-Avocado Advanced Energy Community (BAAEC)*
• Berkeley Energy Assurance Transformation (BEAT)
• Charge Bliss Advanced Renewable Energy Community (City of Carson)
• Biodico's Zero Net Energy Farm (Fresno)
• Santa Monica City Yards Advanced Energy District
• Peninsula Advanced Energy Community (AEC)
• Energize Fresno
• Huntington Beach Advanced Energy Community
• Groundwork San Diego: the Chollas EcoVillage
*received EPIC grant for Phase II build-out.
This research began with an extensive literature review to identify common lessons learned and challenges faced in building local scale energy solutions centered on microgrids. The experiences of the EPIC-funded pilot projects in the design stage were examined through two rounds of stakeholder consultation and interviews. An initial scoping study included reports submitted by all projects at the conclusion of Phase I as well as case studies and other relevant material. Semi-structured, open-ended interviews were conducted with design team members of 11 of the 12 Phase I projects and with team members of all four projects that continued to Phase II. CEC staff who evaluated and provided guidance to the EPIC projects were also interviewed. Interviews, conducted in 2021–2022 (after Phase I was completed and final reports submitted, and after those selected had begun the build-out phase), focused on challenges faced in developing project designs. Information was also collected and evaluated from quarterly meetings with CEC staff and microgrid ad hoc monthly working group meetings initiated to share information, including on regulatory changes with potential to facilitate microgrid development, that led to a (as yet unpublished) report (Reimagine Power, 2022).
The most frequently reported challenges related to the current regulatory framework, with a majority of the projects explaining that current regulations resulted in either a change in design or abandonment of plans. Based on the scoping study, a follow-up round of interviews was conducted to further analyze impacts of regulation on design and build-out. Case studies (following Yin (2009)) to illustrate regulatory impediments, were developed from two projects in Northern California: Berkeley Energy Assurance Transformation (BEAT), in Berkeley, and EcoBlock, in nearby Oakland (Section 4.3). Both projects proposed local energy solutions to improve energy resilience and equity centered on a microgrid. BEAT did not receive Phase II funding. EcoBlock received Phase II funding and is currently in the initial stages of construction. These projects were chosen as representative of regulatory challenges faced by other projects as well as their location in similar urban environments with diverse populations. Both are under the jurisdiction of the same utility company.
Some limitations in this research are acknowledged. Future interviewing could deepen the understanding of regulatory barriers as projects progress. Follow-up interviews with projects that did not continue to Phase II could identify what, if any, elements of the projects were retained. As project design is iterative, so too is data collection and evaluation. Additional interviews could extend to include members of the communities as well as businesses and potential contractors or other partners.
Principles from Resilience Thinking and Anticipatory Regulation are discussed for potential value in encouraging a flexible regulatory approach that supports experimentation with new energy models (Biggs et al., 2015; Armstrong and Rae, 2017; Shandiz et al., 2020; Aczel et al., 2022).
3. California's regulatory framework applied to local energy development
The following section describes California's current regulatory and policy framework for energy, including initiatives to support community microgrids.
3.1. Climate change and decarbonization context
California energy generation relies on an aging electricity grid that has been shown to be unreliable in extreme conditions, and that lacks sufficient capacity during periods of peak demand (Sultan et al., 2016; Guliasi, 2021). At the same time, the state is aggressively decarbonizing the energy sector with electrification a key policy component (Hess and Lee, 2020). Significant legislation to this end includes, among other initiatives, AB 32 (2006) that required greenhouse gas emissions (GHG) to be reduced to 1990 levels by 2020 (achieved in 2016) and SB 100 (2018) that mandates energy be composed of 60% renewable sources by 2030 and 100% clean energy by 2045 (California Legislature AB-32, 2006; California Legislature SB-100, 2018; Berkeley Law, 2022). In 2022, Governor Gavin Newsom approved a portfolio of additional laws, such as AB 1279 that codifies the statewide net zero goal and establishes an 85% emissions reduction target and SB 1020 that sets interim targets that require renewable energy and zero-carbon resources to supply 90% of all retail electricity sales by 2035 and 95% of all retail electricity sales by 2040 (California Legislature AB-1279, 2022; California Legislature SB-1020, 2022). As renewable energy sources, such as solar and wind, are implemented following these and other initiatives, there are demonstrated challenges in integrating them within the current electricity grid, leading to the potential for inequality as access for some communities may take longer to implement (Brockway et al., 2021; Jenn and Highleyman, 2022). The reduction of fossil fuel-based energy and expanded electrification increases stress on the centralized, aging grid, leading to potential energy insecurity during periods of peak demand (Abido et al., 2022; Jenn and Highleyman, 2022). California's rapid technical developments to reduce fossil fuel use through electrification and adoption of renewables have developed faster than policy or changes to regulatory mechanisms that can manage these developments.
3.2. Decentralization of energy generation and distribution
While California is decarbonizing through electrification and adoption of renewable energy sources, the legacy power grid that has operated in much the same way for more than 100 years needs to be modernized, as evidenced by wildfires sparked by aging transmission lines; there is additionally the challenge of integrating current and anticipated future expansion of distributed energy resources (DERs), such as electric vehicle charging capacity (Serna, 2019; CPUC, 2021a; Guliasi, 2021). California aims to include decentralization of the power sector as a resilience and decarbonization strategy through legislative actions that include SB 1339 and the Microgrid Incentive Program. This represents a major shift in how power is managed and distributed, as the grid currently relies on centralized power generation facilities and a network that transmits energy to users at sometimes vast distances (Hussain et al., 2019a; Ajaz and Bernell, 2021a; CPUC, 2021b; Smith et al., 2023).
The US Department of Energy (DOE) has called microgrids an important part of smart grid development “for improving power reliability and quality, increasing system energy efficiency, and providing the possibility of grid-independence to individual end-user sites” and defines a microgrid as “a group of interconnected loads and distributed energy resources within clearly defined electrical boundaries that acts as a single controllable entity with respect to the grid” (Ton and Smith, 2012).
A system of multiple interconnected local scale microgrids has the potential to “harden” the electric grid and improve resilience (Borghei and Ghassemi, 2020; Chen et al., 2020; Wang et al., 2020). The microgrid can support the main electrical grid by adding energy when needed and can strengthen resilience by islanding or detaching from the main grid when the grid fails or during periods of excess demand (Rickerson et al., 2022). As about 90% of current power outages occur at the distribution level, local scale delivery is a potentially important solution (Silverstein et al., 2018). Microgrids also permit integration of renewable sources such as solar and can increase delivery efficiency by reducing energy lost in the process of transmission at distances (Hussain et al., 2019a,b). Connected islandable microgrids can become resilient “building blocks” in a “bottom-up” or community-driven system of delivery, creating efficiency by spreading demand across multiple users and allowing the system to grow with need (Hirsch et al., 2018).
Figure 1 illustrates the technical components of a microgrid, which can integrate rooftop solar, vehicle charging, household appliances, and battery storage, and includes the ability to connect or disconnect from the main utility through a relay. The schematic includes behind the meter or home scale battery storage as well as front of the meter storage that connects directly to the grid.
3.2.1. Industry perspective
According to the International Energy Agency (IEA), proliferation of decentralized energy systems is creating unexpected challenges as most grids were developed for power systems that are now outdated but in which stable power demand and inelastic pricing could be assumed (Kim and Fischer, 2021). The nature of risks has changed as previously the concern was potential larger generator or network failures and there was little incentive to understand consumer energy use patterns. Now there is the new variable of increasing reliance on sources that are weather-dependent, such as solar or wind generation. Also, because the distribution system was designed for unidirectional power flow, when solar flows from a microgrid to the main grid, it can exceed the system's capacity (Kim and Fischer, 2021). There is uncertainty about impacts of expanding power needs, as well as the difficulty in estimating how much power will be added to the grid as more of microgrids come on-line (Rickerson et al., 2022; von Lazar, 2023).
In a survey of roughly 250 U.S. power industry stakeholders, Black and Veatch's 2022–2023 Electric Report identifies key challenges faced by the industry, including the difficult task of integrating renewables and distributed energy sources within the main grid and managing a more complicated energy network to ensure safety (von Lazar, 2023). As microgrids operate with smart technology, they may be vulnerable to cyberattack and managing this risk is fundamental to ensuring resilience (Gaggero et al., 2021). Other potential challenges include the need to establish a tariff for multi-customer microgrids that accurately and equitably reflects costs related to the main grid's infrastructure and maintenance and that avoids shifting expenses unfairly to non-microgrid customers (Borenstein et al., 2021; Nordman et al., 2023). There is also uncertainty over responsibility for ongoing maintenance of local scale microgrids and how connections to the main grid will be developed. Ensuring safe management and maintenance of local scale systems, as regulation changes to cover microgrids, needs to be addressed (Rickerson et al., 2022).
Despite the challenges and uncertainties, there is a recognized need to develop local scale solutions, such as microgrids, to replace fossil-fuel based emergency generation with clean solutions that can ensure energy resilience (Hwang et al., 2023). Replacing diesel back-up with a solar-plus-storage system in just one public building, for example, has been estimated to save $3M of public expenditures and reduce CO2 emissions by 20 tons over 20 years (Hwang et al., 2023). The state's large electric utilities are under increasing pressure to develop resilience strategies, including local scale solutions.
3.3. Landmark initiatives to support microgrid communities
In 2018, California passed SB 1339 (Stern), which directs the California Public Utilities Commission (CPUC) to evaluate the potential role of microgrids in strengthening the electrical grid and improving community energy resilience (California Legislature SB-1339, 2018; Ajaz and Bernell, 2021b). The bill aims to reduce some of the regulatory impediments to microgrid development that have been barriers to planned projects. To this end the CPUC was directed to create separate rates and tariffs for microgrids to produce a revenue stream and lower other barriers to deployment. The bill “would require the governing board of a local publicly owned electric utility to develop and make available a standardized process for the interconnection of a customer-supported microgrid, including separate electrical rates and tariffs, as necessary” (California Legislature SB-1339, 2018). A decision on implementation was set to take place in early April 2023, but (as of publication date) is still pending (CPUC, 2022; Wood, 2023). The implementation of SB 1339 has been slower than expected due to the complexity of developing tariff structures and interconnection applications as well as the lack of a process that would incorporate input from communities (Smeloff et al., 2020). The process of implementation that began in 2018 is now at the stage of considering potential tariffs for multi-property microgrids and is developing definitions for a valuation approach to resilience as resilience has not previously been assigned a value in policy or pricing structures (CPUC, 2023).
Another initiative is California's Microgrid Incentive Program, first authorized in January 2021 to provide funding for community, local, and tribal governments to develop microgrid projects. Goals include improving resilience in communities at heightened risk of power loss, increasing reliability for critical infrastructure facilities, reducing impacts of power disruptions within low-income households and vulnerable populations, and supporting clean energy rather than diesel-fueled emergency generators for resilience. While the program has been conceptually approved, the specific application and implementation details are still under development, with expected roll-out in 2023 (CPUC, 2021b).
3.4. Deep uncertainty and change: decentralization
Energy decentralization is a significant process of change as electrical generation and distribution shifts from centralized control “...to systems that can accommodate small-scale energy generation, enable ‘prosumer' behavior and demand management, and form islands in the event of power outages” (Hess and Lee, 2020). There is inherent uncertainty about the optimal process or path to the desired outcome of clean, reliable, safe, and equitable energy access and thus the need for an approach to regulation and policy that encourages experimentation (Brockway and Dunn, 2020; Helmrich and Chester, 2022). This suggests an approach to regulation that begins by identifying ideal outcomes or benefits of a community-based energy system, including “system resilience, sustainability, efficiency, affordability, and potentially also local democratic control over energy” (Hess and Lee, 2020) but that recognizes uncertainties, and supports exploration of multiple potential futures and models (Gilani et al., 2020; Wang et al., 2020; Workman et al., 2021).
While recent California policy initiatives aim to add resilience through technical innovations such as microgrids and other generation and storage options, there are regulatory impediments as the policy framework currently in place was developed to support a centralized electricity grid (Ajaz and Bernell, 2021a; Reimagine Power, 2022). The following section highlights examples of potential regulatory barriers to community microgrid development, based on lessons learned through pilot programs designed to explore and test new energy options (CEC, 2020). While a full evaluation of the experiences of these pilot programs is beyond the scope of this paper, these examples illustrate potential barriers of a regulatory framework designed for a centralized power system in supporting innovative energy solutions.
4. Piloting decentralized energy in California: a comparative case study focused on regulatory challenges
This section describes some regulatory barriers to implementation faced by California's microgrid communities, through the cases of two projects supported under the state's EPIC program.
4.1. Lessons from electric program investment charge projects
California's Electric Program Investment Charge (EPIC), under the California Energy Commission (CEC), incentivizes development and commercialization of energy solutions to decarbonize electricity—including projects centered around clean, community-based microgrids (CEC, 2020). In 2016, the EPIC Challenge: Accelerating the Deployment of Advanced Energy Communities engaged project teams of developers, local governments, utilities, businesses, researchers, community organizations, and other partners to design and test innovative plans to accelerate the deployment of these decentralized energy communities in California and beyond (CEC, n.d.; CEC, 2020).
The EPIC grant solicitation was divided into two phases: a planning period of approximately two years designed to produce a feasibility study to be followed by a Phase II implementation period of up to four years. The projects were located in diverse geographic settings that included all three of the state's major utility service regions, with emphasis on disadvantaged communities. Urban and rural settings (including a farm) ware represented. The funding solicitation emphasized diversity and sharing of knowledge and lessons learned through public workshops, webinars, and other venues (CEC, 2020). Lessons from these projects can help in identifying and understanding potential regulatory and other barriers to implementation of microgrid-centered communities and offer guidance in strengthening policy for decentralization of energy. As these are pilot projects, the goal of EPIC support was to identify potential challenges to future development and lessons learned for other iterations.
4.2. Regulatory challenges with implications for microgrids
The regulatory framework overseeing electrical generation and distribution in California is a complex system that was designed for the centralized legacy grid. The California Energy Commission (CEC) is the primary policy and planning agency with responsibility for setting energy policy under legislative direction. The California Public Utilities Commission (CPUC) sets rules for private utilities such as the three large investor-owned utilities (IOUs), that are for-profit, publicly traded entities operating as monopolies and that currently manage about 75% of the state's electricity: Pacific Gas and Electric (PG&E), Southern California Edison (SCE), and San Diego Gas and Electric (SDG&E)2. The California Independent System Operator (CAISO) is responsible for the network of long-distance transmission lines. The existing regulatory structure was developed to manage these large-scale utilities, but there are now alternative utility models providing energy in parts of the state, including 44 publicly owned utilities, and decentralization is introducing new complexities (Grosspietsch et al., 2019; Ajaz and Bernell, 2021a,b; California Government, n.d.).
The CPUC is responsible for creating specific rules within the regulatory framework and overseeing implementation. Under this current rules-based system, the three large IOUs are responsible for administering rules, with the CPUC overseeing the process and able to require the IOU to modify rules consistent with state policy and law. Each IOU maintains its own version of the rules, overseen by the CPUC. CPU Code Section 218(b), also colloquially called the “over-the fence rule”, was often cited by EPIC project team members as posing challenges for developers of community microgrid technology. Electric rules that may pose additional barriers and that were cited include Rule 2, Rule(s) 18/19, and Rule 21. Box 2 briefly summarizes CPU Code Section 218(b) and the three electric rules.
Box 2. CPUC rules with implications for microgrids.
Relevant CPUC Energy Rules
• CPU Code Section 218(b). “Over-the-Fence” Rule: limits the ability of project owners to distribute power to buildings on non-adjacent lines. Projects that serve multiple customers and cross rights of way, such as streets, must become energy corporations, and are considered public utilities. As a public utility, an entity is then subject to all CPUC regulations.
• Rule No. 2. California Electric Rule: allows IOUs to impose a “cost of ownership” charge on consumers to recover the expenses for new grid infrastructure that supports the customers” service.
• Rule No. 21. Interconnection: tariff that describes the interconnection, operating and metering requirements in order for generation facilities to connect to the utility's distribution system.
• Rule No. 18/19*. Separate metering: Prohibits separate premises from sharing the same meter, except in defined special circumstances such as maintaining critical infrastructure in the case of a grid power outage.
*Rule No. 18: PG&E and SCE; Rule No. 19: SDG&E (CPUC, 1951).
When microgrids serve multiple customers and cross public rights-of-way they are defined as a “public utility” under CPU Code Section 218(b). This means that a small community energy project can be over-burdened with costs related to the need for significant staff, and financial and legal resources equivalent to those required by a large utility (Reimagine Power, 2022). There is potential for an exemption under this Code if the electricity generated, stored, or distributed is limited to an owner's “own use,” subject to interpretation by the utility and CPUC (von Meier and Kammen, 2021).
Under Rule 2 the utility is allowed to recoup a variety of costs related to facility development and maintenance if microgrid infrastructure must be transferred to their ownership, with potentially project-halting costs. The “cost of ownership” or other expenses charged to consumers as allowed under the Rule is left to the regulators and the utility to apply on a case-by-case basis and may require excessive costs, need for construction of infrastructure, and requirements to develop capacity that would be more appropriate to a large utility.
Additionally, Rule 21 means that the utility can charge for costs related to interconnection, metering, and operations. As the three IOUs maintain their own version of the Rule (as with the other rules), interpretations and applications can vary. Moreover, there currently is no interconnection tariff, rate schedule, or incentive structure specific to microgrids that can provide fair value to users of the community system who may return excess power to the grid and contribute to resilience in case of an outage (Reimagine Power, 2022).
The Rule governing policy on separate metering (Rule 18 in PG&E and SCE and Rule 19 in SDG&E) currently prohibits an entity from selling or supplying electricity to another entity. This can act as a barrier to transferring electricity through a microgrid to multiple consumers. While the Rule allows for shared metering in certain “special” circumstances, such as connecting critical infrastructure, this provision is subject to interpretation by the IOU and CPUC.
California's SB 1339 mandates the CPUC to facilitate commercialization of microgrids, and to that end, must put in place new rates and tariffs (California Legislature SB-1339, 2018). There are currently proposals to develop tariffs and modify rules that now are barriers to microgrid implementation, but the process of modification of existing rules is complex as rules that were designed to apply to a large utility need to be “scaled down” (EcoBlock Interview, 2022). As discussed earlier, the complexity of developing tariffs and incorporating risk definitions has resulted in implementation delays. The EPIC pilot projects began development of their plans for decentralized energy generation in 2016, while SB 1339 was not signed into law until 2018, with implementation still in process.
The regulatory issues highlighted in this section are illustrated through the two cases that follow. The Berkeley Energy Assurance Transformation project aimed to develop a clean energy microgrid community (CEMC) to connect critical community services. EcoBlock's goal was to design a retrofit for an urban residential community centered on a shared microgrid. Application of the microgrid technology to an existing built environment within a city brings increased complexity compared with systems designed around a single customer or new construction. The cases illustrate regulatory challenges that may impede microgrid development in a climate of uncertainty, as California currently has no regulatory framework specific to microgrids.
4.3. BEAT and EcoBlock: two case studies focusing on community microgrid barriers
This section examines the experience of two EPIC projects, both situated in the PG&E service area.
4.3.1. Berkeley energy assurance transformation
The Berkeley Energy Assurance Transformation (BEAT) project was led by the City of Berkeley. The Phase I feasibility study evaluated “...how to design a clean energy microgrid community to serve key municipal buildings and to improve community resilience by maintaining essential city functions during a major power outage. The objective was to design a replicable, community microgrid3 for a dense urban area” (Van Dyke et al., 2019). Critical facilities to be connected included a 911 call center, emergency operations center, jail, police and fire headquarters, city hall, and city administrative buildings (BEAT Interview, 2021b). Components of the planned microgrid included: “automated controls; on-site renewable energy; and battery storage to minimize reliance on conventional backup diesel power” (Van Dyke et al., 2019).
BEAT proposed a system to generate solar electricity to operate microgrid-connected key city facilities, with the battery and smart controllers able to balance solar energy generation and building use demand by distributing and storing the solar energy in real time (City of Berkeley, 2018). The initial plan proposed connecting the microgrid to the main grid section, operated by PG&E, through a master meter with a single point of contact (City of Berkeley, 2018).
Figure 2 below shows the proposed microgrid plan with connection of city-owned buildings that are close in proximity but not directly adjacent (Prototype 1) and potential expansion to buildings not owned by the city (Prototype 2).
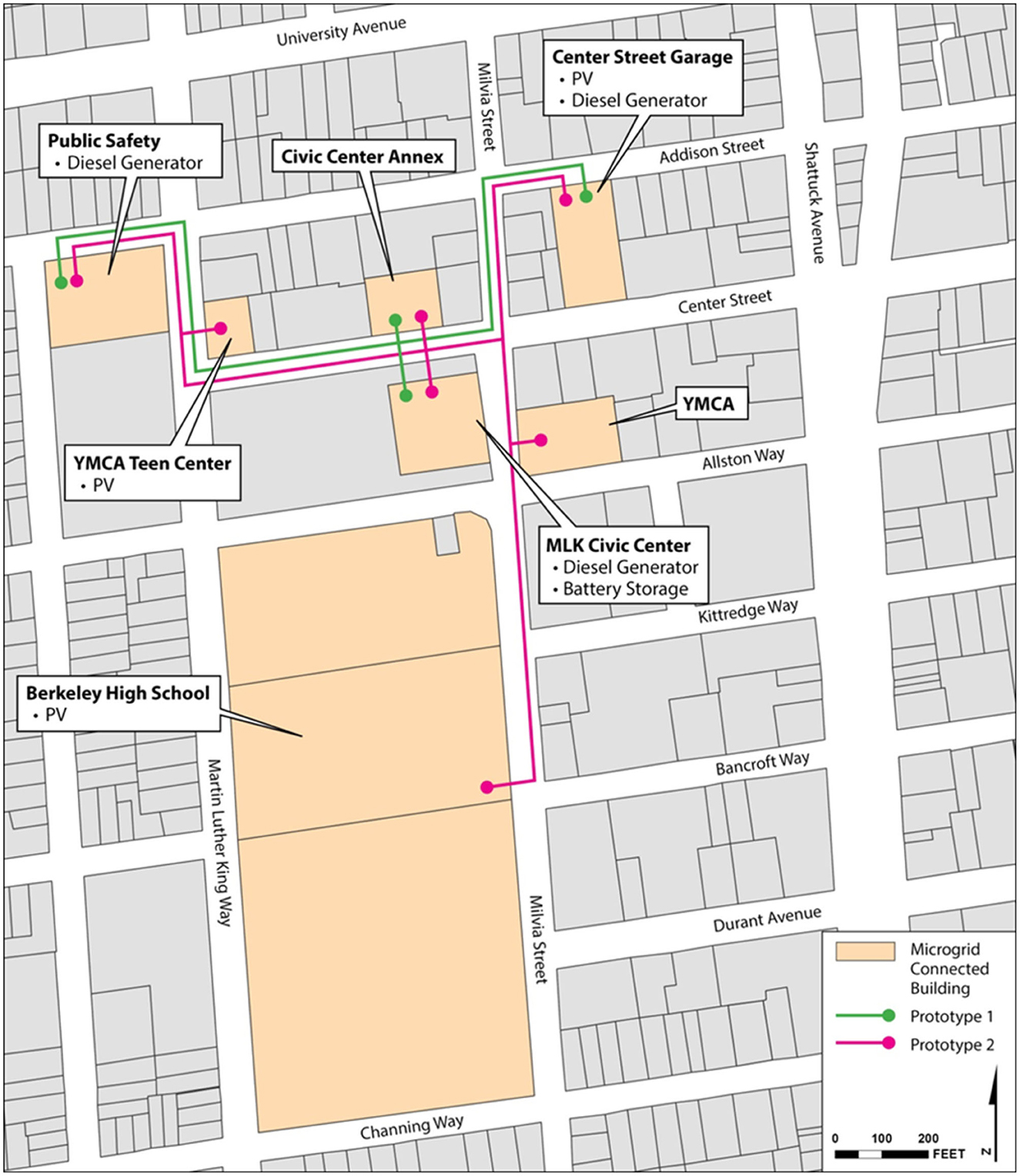
Figure 2. BEAT microgrid prototype (Van Dyke et al., 2019).
The BEAT project was not able to move forward with their plan to develop an islandable microgrid to connect city services due to regulatory roadblocks that resulted in insurmountable financial costs (Van Dyke et al., 2019; BEAT Interview, 2021a). The first impediment was CPU Code Section. 218(b) that, as discussed in 4.2, restricts projects' owners from distributing power on lines that are not on immediately adjacent properties. In the case of a microgrid connecting to multiple non-adjacent buildings, the Rule would require the local microgrid to become a utility and own and operate transmission lines. BEAT's proposed design called for city services included in the microgrid to be in “close proximity” but not directly adjacent. Thus, the plan was not feasible due to the unfavorable interpretation of CPU Code Section 218(b). As a potential workaround, with reduced efficiency, BEAT explored the possibility of switching to multiple nanogrids, meaning a smaller solar-plus-storage system rather than a single microgrid to connect all services (BEAT Interview, 2021a).
The second impediment was that BEAT was not granted an exemption per Rule No. 2 for special facilities, including critical services. Under Rule 2, in order to use existing utility distribution lines for an islandable microgrid, either all customers on that line must be part of the microgrid (such as at the end of a distribution line), or the utility must be willing and able to automatically shut-off any non-microgrid customers on the existing distribution lines in the case of a power outage. In addition, the utility would require legal contracts with all customers not served by the microgrids that would be shut-off in the case of a power outage. For the BEAT project, there were hundreds of customers on the lines between the proposed CEMC buildings who would not be microgrid participants. PG&E did not have automatic switches or a willingness to add that technology to their distribution lines, nor a shut-off agreement with customers. Therefore, the BEAT project would be required to construct new parallel distribution lines to connect buildings participating in the microgrid. New lines would come at a significant cost, including capital and installation costs, utility charges for operation and maintenance of the lines, and a transfer tax to deed assets to the utility. The construction of new distribution lines was estimated at about $1 million per mile. Additionally, PG&E would collect a one-time transfer tax per the Income Tax Component of Contributions (ITCC) Provision (to cover state and federal taxes) for deeding the new lines to PG&E, amounting to 24–34% of the total capital costs. PG&E would also charge for operation and maintenance of the new lines per interpretation of Rule No. 2 at a rate of 6.5% of the capital costs annually, indefinitely. This charge would equate to more than the total capital costs of the BEAT project after about 15 years (Van Dyke et al., 2019; BEAT Interview, 2021a).
Figure 3 summarizes the most significant reasons that microgrids may be cost-prohibitive under current regulation, according to the findings of the BEAT project team.
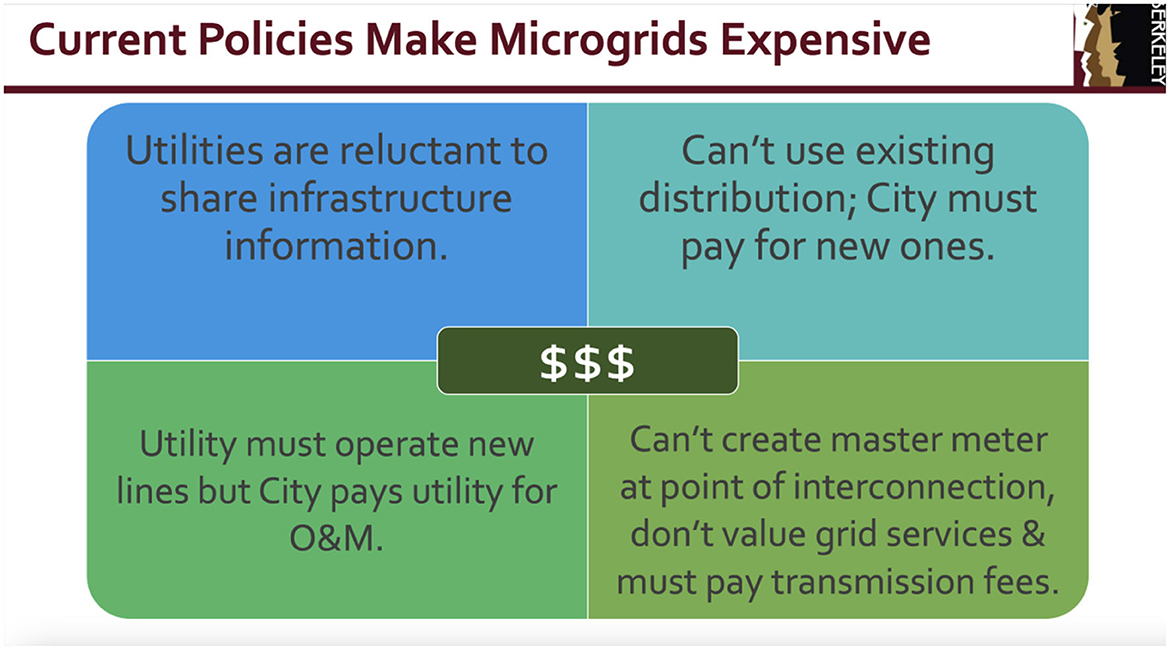
Figure 3. Why microgrid costs are high, creating a potential barrier. City of Berkeley, November 16, 2020. Available online at: https://epicpartnership.org/resources/Schwartz_PICG_PSPS_Workstream_Meeting_1.pdf.
The BEAT project concluded that it was not feasible to move forward with their plan to develop an islandable microgrid connecting vital city services due to regulatory requirements that would result in insurmountable financial costs (Van Dyke et al., 2019; BEAT Interview, 2021a).
4.3.2. Oakland EcoBlock
The Oakland EcoBlock project, led by University of California, Berkeley, aimed to develop a prototype for affordable urban decarbonization through retrofitting at the neighborhood block scale. A goal was to test the optimum size for a microgrid-based community development that scales up from a single housing unit. The main component of the plan was to replace natural gas as a fuel source with electricity and develop a solar-powered common microgrid to serve the block. The aims were to rapidly and equitably reduce carbon emissions; improve resilience by developing a solar microgrid to take advantage of fluctuations in consumer demand and provide resilience during and after power outages; and build community leading to eventual self-management and ownership of the system.
Phase I developed a design, including technical specifications, planning, permitting feasibility, and financing models, for a retrofit within a middle and low-income neighborhood in Oakland with a mix of single- and multiple-family buildings, homeowners and renters, and at least one small business (see Figure 4). The plan included “an integrated system of energy efficiency retrofits, a direct current solar/storage/electric vehicle microgrid, alternating/direct current houses, and water efficiency retrofits with rainwater capture” with projected results of close to zero net emissions in homes, reduction of carbon emissions for the block (65%), and significantly reduced water use (65–70%) (Barr et al., 2019).
The community energy plans incentivized through EPIC emphasized an iterative strategy to test approaches and models that could then be modified or scaled. The Oakland EcoBlock team emphasized that the focus is on lessons learned in the project's current iteration and possible future improvements and redesign, including scaling-up beyond the current block level for financial feasibility. As the eventual plan for EcoBlock is community management, and a community association has now been formed, given the technical, financial, legal, and regulatory complexity of the initial planning stages, it is not yet possible for the project to be truly community-designed although community control of decision-making is the ideal longer-term goal. The team explained that every step toward the goal of community-led development and control is a positive step that moves the project closer to the aim of democratic decision-making (EcoBlock Meeting, 2023).
The Oakland EcoBlock pilot project avoided the implications of CPU Code Section 218(b) in contrast to the experience of BEAT. As the project was located on a cul-de-sac terminated by a creek, the electrical connection of the homes and businesses joining the microgrid did not cross a public right-of way but were contiguous (von Meier and Kammen, 2021). This highlights the apparent arbitrariness of application of CPU Code Section 218(b), as the microgrid designs as originally conceived by the two projects were similar. As with the BEAT project, PG&E would collect a one-time transfer tax per the Income Tax Component of Contributions (ITCC) Provision for deeding EcoBlock's new lines to PG&E. However, as a nonprofit, EcoBlock could claim an exemption from the ITCC. Nonetheless, EcoBlock was charged a Cost of Ownership of 0.89% of the capital cost, paid either as a one-time cost or monthly over 15 years.
The second issue faced by EcoBlock related to the complexity of regulation and scale and whether rules that were designed to cover large industrial scale energy systems would apply reasonably to a block consisting of just 25 homes. EcoBlock's former project Principal Investigator Alexandra von Meier explained that “[a] crucial research question was whether the EcoBlock community could own and operate the microgrid infrastructure...[CPU Code 218(b)]. If you own the electrical wires, you become a regulated utility...the key problem is down to a question of scale—the regulations and processes that apply to a real utility were not envisioned as applying to an entity that small” (EcoBlock Interview, 2022).
Moreover, you cannot scale down the regulatory requirements. “If you scale down from utility scale to a fraction of a city—a neighborhood block, for example—the requirements no longer make sense, and you would not have the capacity to meet the requirements as intended for the utility scale” (EcoBlock Interview, 2022). Von Meier emphasized that until recently, the technology had not developed to enable a neighborhood microgrid to function as a utility and from the perspective of the investor-owned utility it was impractical for a small system to function that way. Large utilities can meet regulatory requirements because of economies of scale but these requirements “can be overly burdensome for a much smaller entity” (EcoBlock Interview, 2022). While the microgrid technology can scale down, the regulatory framework does not, meaning that a small community microgrid lacks the legal and financial resources and personnel to deal with regulatory requirements designed for a large utility.
4.3.2.1. Community model
The EcoBlock design aimed to create a model for a community-managed energy system that included resident participation and eventual democratic self-governance. After the initial feasibility study was completed and before build-out began, a new residential block was recruited in a competitive process with criteria that included a pre-established sense of community cohesion and interest in participating in a pilot energy project. Additionally, a community liaison with strong ties to and knowledge of the community became an integral component. More recently, a non-profit common interest development association (CID) has been formalized to allow participants to own the shared assets of the project. The goal of the pilot project is to create a community management model, as well as a technical model, that future projects can follow.
Developing a self-managed energy project is challenging as there is no precedent for this type of ownership structure that diverges significantly from the centralized control of electricity generation followed by the investor-owned utilities with little input from the community to full community control. This shift is particularly challenging in an under-resourced community due to the need for technical, legal, and other resources and to liaise with state regulatory agencies as well as the utility. EcoBlock includes residents with multiple first languages, as one example of complexity, with some interconnection documentation provided through the utility PG&E available only in English. While self-management for an under-resourced or socially and economically mixed community has the potential to improve energy justice through incorporating community perspectives and unique local knowledge, there is also risk due to regulatory complexity and uncertainty about such aspects as financial impacts, highlighting the need for support in this transition away from a centrally managed system. Community management means access to data due to the installation of smart systems, but also potential gaps in access and the need for developing ways of analyzing and utilizing data streams (Anderson et al., 2022; Blanke et al., 2022; Verba et al., 2022). The EcoBlock team emphasizes that community design and management of energy is a process, as the community builds capacity for the democratic, community-based model envisioned as the eventual outcome, illustrated in Figure 5.
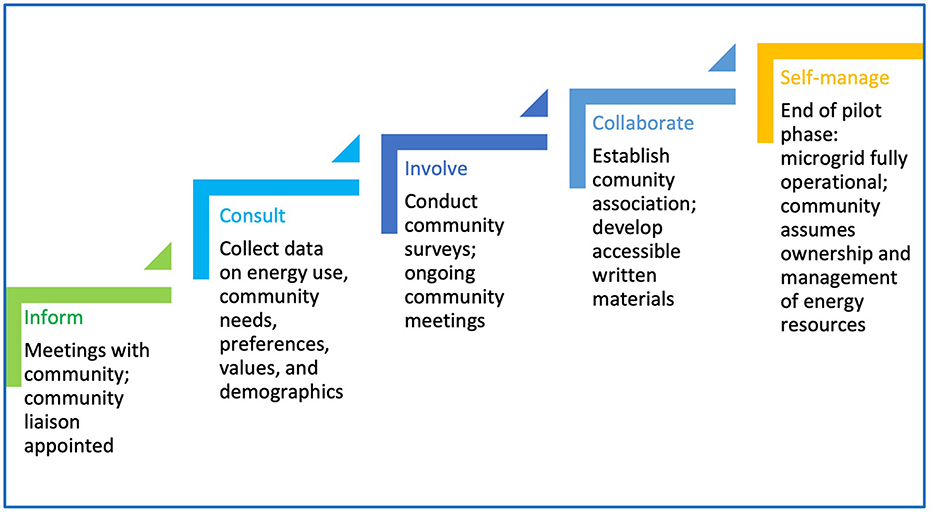
Figure 5. Model of a process leading to community decision-making, applied to EcoBlock. Adapted from USDN (n.d.).
Figure 5 describes sequential steps that aim to build capacity for community collaboration and governance, highlighting that local decision-making is a process that moves toward the aim of self-management. In the case of the EPIC AECs this is complicated by external factors such as utility company rules and policies, coordination with multiple stakeholders, financial constraints, and contractor and potential partner lack of capacity to meet the need for complex resources.
4.3.2.2. Accounting for uncertainty in design
In discussing the electrical design, the EcoBlock team described that “available technology and usage will change over time, which introduces uncertainty but can help make the design more relevant for future conditions if we consider the larger trends” (Barr et al., 2019). There may be more vehicles and ways to charge them, the main grid may change, and “[t]he utility grid will also change, in cost (tariffs), reliability, and services it provides” (Barr et al., 2019). There is also uncertainty around changes in the community itself, with potential for rising property values and related gentrification, as well as challenges related to being situated in an urban, disadvantaged, or mixed community. The iterative development of decentralized energy systems includes inherent uncertainty at every stage of the process, which needs to be accounted for in planning, with ability to explore and test multiple solutions.
4.4. Regulatory challenges
California's legacy grid, as is common with most large-scale monopolistic projects, has mainly been developed and amended through a top-down model, with policy and regulation determined by regulatory bodies that are then interpreted and executed through existing frameworks. This approach determines the design of technology and can restrict creative approaches to problem-solving and meaningful participation of residents who will benefit from and face impacts of the project. Industrial scale power plants are more likely to be sited in lower income or otherwise marginalized communities, elevating risk for pollution, and resulting health impacts (Lukanov and Krieger, 2019; Johnston and Cushing, 2020). While it is often assumed that greater community participation and control creates social benefits, there is a need for evaluation of impacts, particularly unintended impacts on vulnerable communities (Axon and Morrissey, 2020).
The regulatory impediment of CPU Code 218(b) and restrictive rules developed to manage a centralized system have resulted in excessive financial or other burdens for projects, as seen in the examples of BEAT and EcoBlock. Additionally, in looking for technical solutions within the existing rule-based frameworks, projects may be forced to enact less-efficient solutions, such as multiple smaller scale nanogrids rather than a single connected microgrid. This arguably means that creative problem-solving can be used to identify workarounds within the regulatory framework, rather than identifying optimum energy solutions. Microgrid communities face a regulation gap—as developing technologies to address these challenges are playing “catch up” with the existing regulatory framework.
4.4.1. Valuing resilience
As discussed in Section 3.3. above, under SB 1339, the CPUC was directed to develop a framework to facilitate the commercialization of microgrid technology (Guliasi, 2021; von Meier and Kammen, 2021). Resilience, however, was not part of the initial framework and not explicitly addressed in this process of rulemaking (19-09-009). As explained by Smeloff et al. (2020) “the implicit premise behind that proposal was that microgrids provide value only to the customers they directly serve, provide no value to the grid, to other ratepayers, or to California's policy goals, and make use of the services of the grid in such a way as to shift costs to other ratepayers if not strictly subjected to a slew of charges.” Commercialization—a goal of the EPIC projects—will be hindered if microgrids are not appropriately valued (Smeloff et al., 2020).
Equitable solutions that lead to resilience require flexibility in balancing community energy loads. This approach is not explicitly valued under the current regulatory framework and there is a need to develop a regulatory approach that incorporates incentives for stakeholders—including business partners, city governance agencies, NGOs, utilities, contractors, and community members—to co-create equitable solutions (Brockway et al., 2021).
5. Principles of resilience thinking and anticipatory regulation
The following section highlights the principles of Resilience Thinking and Anticipatory Regulation and their potential benefits for application to innovative energy models within an environment of uncertainty. The paper proposes using these principles to guide a non-prescriptive regulatory approach to anticipate and enable experimentation in diverse communities.
5.1. Resilience thinking
As there is mounting evidence of worsening impacts of a changing climate, strategies of mitigation increasingly focus on ways to build resilience or “survivability” for individuals and communities. The experience of California with worsening wildfires and their impacts highlights the need to emphasize resilience in planning for new systems. Resilience is defined by the UN Office for Disaster Reduction as “the ability of a system, community or society exposed to hazards to resist, absorb, accommodate to, and recover from, the effects of a hazard in a timely and efficient manner, including through the preservation and restoration of its essential basic structures and functions” (UNISDR, 2009).
Principles of Resilience Thinking (Box 3) provide guidance in developing an approach to thinking about the role of resilience in community energy planning (Biggs et al., 2015; Muñoz-Erickson et al., 2021).
Box 3. The key principles of resilience thinking.
1. Maintain redundancy and diversity: employ multiple knowledge systems, actors, cultural groups, and organizations as diverse approaches to change and uncertainty may lead to improved outcomes.
2. Manage connectivity: leverage existing connections and interactions to improve community-level resilience.
3. Manage slow variables and feedback: anticipate impacts of “slow changes,” such as those related to climate change on a longer time scale, to aid prediction of local level risk and guide responses.
4. Foster complex adaptive systems thinking: acknowledge the complexity—and uncertainty—of connections and interactions in energy systems.
5. Encourage learning: learn and experiment as part of an adaptive and collaborative approach with diverse types of knowledge equally valued.
6. Broaden participation: develop vehicles for inclusive participation to build trust, shared understanding, and uncover valuable perspectives beyond those acquired solely through scientific processes.
7. Promote polycentric governance systems: collaborate across multiple scales and among diverse governing bodies (Biggs et al., 2015).
Resilience is a high priority for energy systems as highlighted by recent disruptions such as winter storms and wildfires, but there is no agreed upon federal definition. This means that states in many cases are in the position of determining their own definitions. As part of implementing its microgrid strategy, California is currently developing definitions and an approach to valuation of resilience, which may be useful to other locations implementing their own resilience strategies (Smeloff et al., 2020; CPUC, 2022). While the need to value resilience is generally accepted, there is currently no standardized method of assessing value and methods of valuation are limited, complicating the process. Lack of valuation is a significant barrier to identifying financing and support from potential contractors and agencies, leading to under-investment in new projects as “[c]oncrete costs will always outweigh unquantified benefits” (Rickerson et al., 2022, p. 10). Assessing value is challenging as customers and utilities may under- or over-estimate duration of outages, as one example.
5.2. Anticipatory regulation
Uncertainty associated with a new or emerging technology can benefit from a regulatory framework that identifies and addresses potential risks but also supports and encourages innovation and experimentation (Sandys et al., 2017; Brockway and Dunn, 2020; Brockway et al., 2021). Anticipatory Regulation4 provides a set of tools and processes to help regulators and governments identify, build, and test solutions for emerging and evolving challenges. AR seeks to increase social legitimacy of new technologies by incorporating multiple stakeholders with equal voices in decision-making, including community residents, researchers, technical experts, business partners and contractors, city planners, state regulatory agents and legal experts, and others, to achieve equitable solutions to complex problems requiring new strategies (Armstrong and Rae, 2017; Aczel et al., 2022). The aim is to encourage innovation while following principles of “good governance” that include equity and justice in both outcomes and processes (Armstrong and Rae, 2017; O'Beirne et al., 2020; Aczel et al., 2022). Examining and evaluating interactions between industry and communities is a significant part of this approach (Firestone et al., 2018).
Anticipatory Regulation centers on six key principles (Armstrong and Rae, 2017; NESTA, 2022) (see Box 4). The aim is to move toward inclusive and collaborative decision-making to support and encourage solutions to complex problems in the face of uncertainties (Workman et al., 2020, 2021). Anticipatory Regulation recognizes the evolving role of the regulator, and the need to enable “safe spaces” for innovative solutions to develop in a controlled and experimental manner with a goal of development of regulations.
Box 4. The key principles of anticipatory regulation.
1. Inclusive and collaborative: include wide range of stakeholders with opportunities for discussion
2. Future-facing: identify factors important in the future, and potential impacts on outcomes
3. Proactive: ensure access to information and data; promote innovative ideas; space to test/evaluate
4. Iterative: design a flexible approach to test/review proposals, rather than aiming toward one solution
5. Outcomes-based (rather than rules-based): identify desired outcomes and measures of success and pathways
6. Experimental: encourage diversity of solutions to be developed and adapted to specific situation, following a decentralized planning model (NESTA, 2022).
The role of the consumer is changing as users of electricity increasingly are also assuming the role of producers of electricity. Similarly, the role of regulators is changing from that of rule makers and enforcers to supporters of innovation and experimentation as technology develops in rapid and sometimes unexpected ways. Regulators in this dynamic context can thus benefit from incorporating the principles of Anticipatory Regulation that ensures safety while supporting innovation, in an approach in which regulation is developed side-by-side with innovation or in the experimental context. For example, the idea of a regulatory sandbox—“a ‘safe space' in which businesses can test innovative products, services, business models, and delivery mechanisms without immediately incurring all the normal regulatory consequences of engaging in the activity in question” is potentially useful in the context of piloting energy communities (UK Financial Conduct Authority (FCA), 2015, p. 25). Community engagement, open sharing of data, and Resilience Thinking principles are proposed for inclusion in this approach. Experimental test beds such as the EPIC supported projects are examples of this iterative approach that starts with understanding community values and vulnerabilities and designs and tests multiple solutions.
6. Discussion
Examples of the failures of the centralized grid in California illustrate that “business as usual” cannot provide energy security. Moreover, there is a demonstrated need for an array of new and developing technologies that can improve community resilience. The state is pursuing innovative decentralized energy solutions—including clean microgrid communities—as one tool. However, as new technical solutions are needed so too are new or adapted regulatory solutions specific to microgrids required to enable decentralized energy communities. As California explores regulatory and technical solutions, other states and nations can learn from these experiences.
We are clearly witnessing widespread transformation across the energy sector and particularly fundamental shifts in how and where our energy is produced, transmitted, and used. The roles of energy producers and consumers are evolving, as increasingly energy is being produced and used locally, rather than transmitted at great distances as in the current model. Energy consumers are becoming active “prosumers”—both producing and consuming energy. At the same time, there is a changing role for regulation and the regulator. With the development of novel energy technologies such as microgrids, there is a demonstrated “lag” for the regulations to catch up to the technology, and a key opportunity to develop regulation specific to the technology at the same time as the technical development. There is an important role for enabling “regulatory sandboxes'5 to allow experimentation in the metaphorical sandbox without or with limited regulations. Developing clear “regulatory sandbox” environments could enable co-development of regulations together with the technology and ensure input and evolution of potential unintended consequences. At the same time, while the Anticipatory Regulation approach advocates for experimentation with developing regulations, the community-focused resilience framework ensures that the needs and values of communities are protected. A community asset map, in which needs and strengths are assessed (community organizations, emergency facilities, schools, housing, abilities and perspectives of residents, and more) can serve as a crucial first step in moving toward community resilience (Stein and Moser, 2014; Rapaport et al., 2015; Krawchenko et al., 2016).
6.1. Summary of regulatory barriers
The case studies of the two EPIC projects, BEAT and EcoBlock, highlight the value of testing new energy models, but also illustrate regulatory challenges due to the current emphasis on regulation based on rules designed for large utilities. Specific barriers to implementation, as discussed in this research include:
• Regulation for energy is a rules-based framework at present, meaning that specific rules, such as CPU Code 218(b) and Rules 2, 18/19 and 21, became barriers for implementation or resulted in workarounds or redesign of projects.
• Energy regulation was initially developed for large, industrial utilities and it is challenging to “scale down” regulations in a way that is “fit for purpose” for small block- or neighborhood-scale installations. There is no regulatory structure specific to microgrids.
• Lack of feasible financial models are a major impediment to build-out, including lack of microgrid-specific tariff structures to incentivize commercial developers and treat excess energy produced locally that is returned to the main grid “fairly.”6
In recognition of the current impediments to microgrid development and as part of the process of implementing the legislative directive of SB 1339, a series of policy recommendations were proposed in an unpublished study conducted by Reimagine Power (2022). The table below summarizes the policy recommendations and the relevant entity responsible for implementation:
The regulatory recommendations proposed by Reimagine Power (Figure 6) represent an important step toward enabling the design and development of community microgrids, such as those piloted by BEAT and EcoBlock, and if adopted quickly, could assist in removing some of the regulatory barriers to microgrid adoption. These recommendations are, however, adaptations of the current rule-based regulatory system and incorporating principles of Resilience Thinking and Anticipatory Regulation suggest that the regulatory framework for a new technology should move beyond a rule-based approach to emphasize outcomes. This research proposes a more comprehensive rethinking of how regulation is developed and defined, beginning with a regulatory framework specific to microgrids (as proposed in policy recommendation two, in Figure 6). This acknowledges that local-scale energy generation and transmission is a radical departure from industrial-scale developments that has provided energy historically to much of California. It is suggested that a new approach to regulation be devised in which the regulator is also the enabler and supporter of technical innovation.
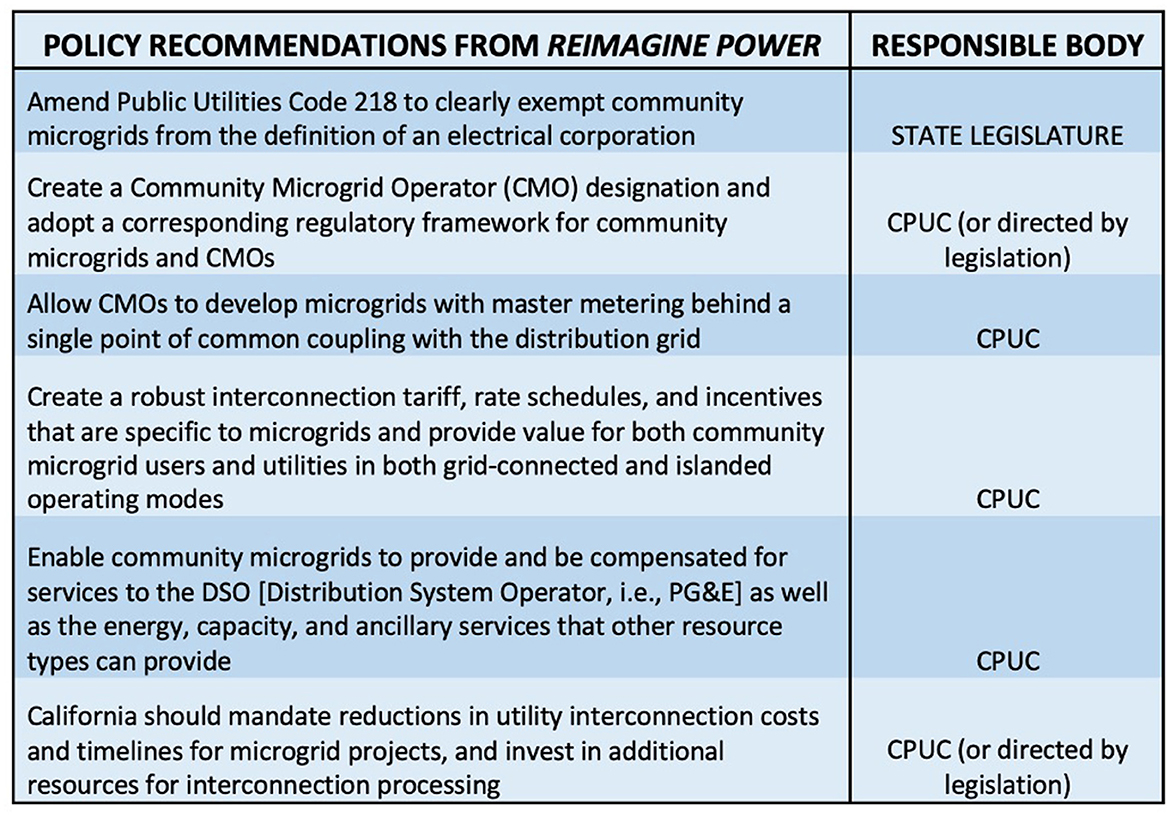
Figure 6. Policy recommendations for microgrid regulation (adapted from Reimagine Power, 2022).
Below are some suggested principles, based on Resilience Thinking and Anticipatory Regulation, to guide development of a proposed new regulatory framework specific to microgrids:
6.1.1. Resilience
• Establish an accepted definition of resilience and metrics to measure success in achieving resilience as a model that can be widely applied across communities, and potentially beyond California.
• Develop a method for valuing resilience that can be adopted and applied across communities and locations. Resilience is largely treated as an externality due to difficulty in estimating costs of outages, making it difficult to direct funding to projects that aim to improve resilience. Encourage investment and financing in community systems through valuation of resilience.
• Identify key community assets and potential vulnerabilities through a planning process, such as local asset mapping, as an important step in building resilience as well as community cohesiveness.
• Collect data on pilot projects that can be used to inform and modify resilience metrics.
6.1.2. Outcomes-based (rather than rules-based)
• Embed the intention to create an outcomes-based approach rather than rules-based approach in a new microgrid specific regulatory framework. Focusing on outcomes allows flexibility in design of new energy models.
6.1.3. Future-facing, experimental, and Iterative (flexibility)
• Co-create regulations with microgrid grid regulators and innovators, emphasizing flexibility and experimentation, and recognizing that energy and resilience needs, as well as technical solutions, will change.
• Incorporate flexibility and nimbleness in the framework to acknowledge that needs and technologies change.
6.1.4. Inclusive and collaborative
• Acknowledge that a microgrid has potential social implications as it moves control from a top-down to a community-based system. The regulatory framework encourages iterations and experimentation, while centering on the needs of the community.
• Enable communities to move toward empowerment, self-governance, and control over energy production and consumption, and recognize that this is an iterative process.
• Acknowledge that in the nimble and future-facing framework, communities and their priorities drive technical developments.
• Identify key community members to function as liaisons with regulatory agents to develop and ensure trust among all stakeholders in a project.
• Develop methods of communication, collaboration, and linkages among partners—regulators, utilities, multi-scale governance, communities, local organizations—with capacity to streamline processes and ensure meaningful partnerships with communities. This aims to reduce project delays and improve understanding of needs of communities by regulators and technical and governance needs by communities.
6.1.5. Proactive (information collection and access)
• Develop access to real time energy data for project development as well as wider climate and resilience goals.
• Acknowledge data and information threats and develop mechanisms to protect data and privacy while also ensuring timely access to critical information. Ensuring anonymization and privacy is particularly critical in developing trust in disadvantaged communities.
• Incorporate information on public opinion and perspectives broadly and for meaningful community input with state agencies that are creating rules and regulations that impact them.
• Develop best practices and benchmarks for interactions between industry and communities.
• Ensure clear communication with communities, designation of roles/responsibilities, and opportunities for providing feedback and meaningfully responding to and incorporating feedback.
Conclusion
In conclusion, community microgrids have the potential to revolutionize energy systems, bolster resilience, and enhance sustainability. Implementing a forward-thinking regulatory framework based on Resilience Thinking and Anticipatory Regulation principles can unlock the full potential of microgrids not only in California but also in other regions seeking to decentralize their energy systems.
Emphasizing the importance of innovative regulatory solutions, particularly in underserved and frontline communities, highlights the role of regulation in supporting the development of net-zero technologies such as microgrids while safeguarding communities and their valuable resources. Through fostering collaboration, adaptability, and inclusivity, such a framework can encourage development of transformative energy solutions that protect communities and lead to a more sustainable future.
Data availability statement
The original contributions presented in the study are included in the article/supplementary material, further inquiries can be directed to the corresponding author.
Author contributions
TP contributed to the conception and design of the work, analysis and interpretation of data, and drafting and revising the manuscript. MA is grateful for the contribution of the EcoBlock project team. All errors and omissions remain the author's own.
Funding
This study was funded by McQuown Postdoctoral Fellowship and California Energy Commission Contract Number: EPC-18-013.
Conflict of interest
The authors declare that the research was conducted in the absence of any commercial or financial relationships that could be construed as a potential conflict of interest.
Publisher's note
All claims expressed in this article are solely those of the authors and do not necessarily represent those of their affiliated organizations, or those of the publisher, the editors and the reviewers. Any product that may be evaluated in this article, or claim that may be made by its manufacturer, is not guaranteed or endorsed by the publisher.
Footnotes
1. ^https://www.energy.gov/eere/solar/articles/solar-plus-storage-101
2. ^Publicly Owned Utilities (POUs) and Investor-Owned Utilities (IOUs) have served California for more than a century. More recently, Community Choice Aggregators (CCAs) are being created to give local communities an even bigger voice in their energy future. Here are the basics about these different models (https://www.cmua.org/pou-explainer).
3. ^Referred to in the project as a clean energy microgrid community (CEMC).
4. ^While this approach was initially designed for the context of the technical innovations in the UK, the principles of AR have potential wider application including in development or revision of California's decentralized energy framework.
5. ^The United Kingdom (UK) Financial Conduct Authority (FCA) developed the term ‘regulatory sandbox' in 2014: “a ‘safe space' in which businesses can test innovative products, services, business models and delivery mechanisms without immediately incurring all the normal regulatory consequences of engaging in the activity in question.”
6. ^The CPUC, following requirement of SB 1339, is expected to release microgrid specific funding in 2023 for “front-line communities” at risk of power outages, wildfires, poor grid performance, and earthquakes (Wood, 2023).
References
Abatzoglou, J. T., Smith, C. M., Swain, D. L., Ptak, T., and Kolden, C. A. (2020). Population exposure to pre-emptive de-energization aimed at averting wildfires in Northern California. Environ. Res. Lett. 15, 094046. doi: 10.1088/1748-9326/aba135
Abido, M. Y., Mahmud, Z., Sánchez-Pérez, P. A., and Kurtz, S. R. (2022). Seasonal challenges for a California renewable-energy-driven grid. Iscience 25, 103577. doi: 10.1016/j.isci.2021.103577
Aczel, M., Heap, R., Workman, M., Hall, S., Armstrong, H., and Makuch, K. (2022). Anticipatory Regulation: Lessons from fracking and insights for Greenhouse Gas Removal innovation and governance. Energy Res. Soc. Sci. 90, 102683. doi: 10.1016/j.erss.2022.102683
Ajaz, W., and Bernell, D. (2021a). Microgrids and the transition toward decentralized energy systems in the United States: a multi-level perspective. Energy Policy 149, 112094. doi: 10.1016/j.enpol.2020.112094
Ajaz, W., and Bernell, D. (2021b). California's adoption of microgrids: A tale of symbiotic regimes and energy transitions. Renew. Sustain. Energy Rev. 138, 110568. doi: 10.1016/j.rser.2020.110568
Anderson, K., Farthing, A., Elgqvist, E., and Warren, A. (2022). Looking beyond bill savings to equity in renewable energy microgrid deployment. Renew. Energy Focus 41, 15–32. doi: 10.1016/j.ref.2022.02.001
Anderson, K. H., DiOrio, N. A., Cutler, D. S., and Butt, R. S. (2017). Increasing resiliency through renewable energy microgrids. Int. J. Energy Sector Manage. 2, 34. Available online at: https://www.osti.gov/biblio/1389210
Armstrong, H., Gorst, C., and Rae, J. (2019). Renewing regulation: “Anticipatory regulation” in an age of disruption. NESTA. Available online at: https://media.nesta.org.uk/documents/Renewing_regulation_v3.pdf (accessed July 20, 2023).
Armstrong, H., and Rae, J. (2017). A working model for anticipatory regulation: A working paper. NESTA. Available online at: https://www.nesta.org.uk/report/a-working-model-for-anticipatory-regulation-a-working-paper/ (accessed July 20, 2023).
Aros-Vera, F., Gillian, S., Rehmar, A., and Rehmar, L. (2021). Increasing the resilience of critical infrastructure networks through the strategic location of microgrids: A case study of Hurricane Maria in Puerto Rico. Int. J. Disaster Risk Reduct. 55, 102055. doi: 10.1016/j.ijdrr.2021.102055
Axon, S., and Morrissey, J. (2020). Just energy transitions? Social inequities, vulnerabilities and unintended consequences. Build. Cities 1, 393–411. doi: 10.5334/bc.14
Barr, Z., Bourassa, N., Bowie, J., Brown, R., DeCuir, N., Diamond, H. J., et al (2019). Accelerating the deployment of advanced energy communities: The Oakland EcoBlock. California Energy Commission. Publication Number: CEC-500-2019-043. Available online at: https://www.energy.ca.gov/sites/default/files/2021-06/CEC-500-2019-043.pdf (accessed July 20, 2023).
BEAT Interview (2021a). Interview with City of Berkeley, Berkeley Energy Assurance Transformation (BEAT), team members. May 25, 2021.
BEAT Interview (2021b). Interview with City of Berkeley, Berkeley Energy Assurance Transformation (BEAT), team members. October 27, 2021.
Bedsworth, L., Cayan, D., Franco, G., Fisher, L., and Ziaja, S. (2018). Statewide summary report. California's Fourth Climate Change Assessment. Publication number: SUM- CCCA4-2018-013. Available online at: https://www.energy.ca.gov/sites/default/files/2019-11/Statewide_Reports-SUM-CCCA4-2018-013_Statewide_Summary_Report_ADA.pdf (accessed July 20, 2023).
Berkeley Law (2022). California Climate Policy Dashboard. Available online at: https://www.law.berkeley.edu/research/clee/research/climate/climate-policy-dashboard/ (accessed November 29, 2022).
Biggs, R., Schlüter, M., and Schoon, M. L. (2015). Principles for Building Resilience: Sustaining Ecosystem Services in Social-Ecological Systems. Cambridge: Cambridge University Press. doi: 10.1017/CBO9781316014240
Blanke, J., Gonzalez, A. B., D'Oca, S., Niederkofler, M., and Nordlund, E. (2022). European small-town Renewable Energy Communities: Participatory design of supporting tools as a vehicle to engage and understand local communities and their energy related concerns. Open Res. Europe. 2, 129. doi: 10.12688/openreseurope.15114.1
Borenstein, S., Fowlie, M., and Sallee, J. (2021). Designing Electricity Rates for an Equitable Energy Transition. Energy Institute WP 314. Available online at: https://ww2.arb.ca.gov/sites/default/files/2021-11/UCB-sp22-electricity-ws-11-02-21.pdf (accessed July 20, 2023).
Borghei, M., and Ghassemi, M. (2020). A multi-objective optimization scheme for resilient, cost-effective planning of microgrids. IEEE Access. 8, 206325–206341. doi: 10.1109/ACCESS.2020.3038133
Brockway, A. M., Conde, J., and Callaway, D. (2021). Inequitable access to distributed energy resources due to grid infrastructure limits in California. Nat. Energy. 6, 892–903. doi: 10.1038/s41560-021-00887-6
Brockway, A. M., and Dunn, L. N. (2020). Weathering adaptation: Grid infrastructure planning in a changing climate. Clim. Risk Manage. 30, 100256. doi: 10.1016/j.crm.2020.100256
California Government (n.d.). The California Energy Commission California's Energy Governing Institutions. Available online at: https://www.energy.ca.gov/sites/default/files/2019-06/Fact_Sheet_California_Energy_Governing_Institutions.pdf (accessed May 10 2023)..
California Legislature AB-1279 (2022). California Climate Crisis Act. Available online at: https://leginfo.legislature.ca.gov/faces/billTextClient.xhtml?bill_id=202120220AB1279 (accessed July 20, 2023).
California Legislature AB-32 (2006). Air pollution: greenhouse gases: California Global Warming Solutions Act of 2006. Available online at: https://leginfo.legislature.ca.gov/faces/billTextClient.xhtml?bill_id=200520060AB32 (accessed July 20, 2023).
California Legislature SB-100 (2018). California Renewables Portfolio Standard Program: emissions of greenhouse gases. Available online at: https://leginfo.legislature.ca.gov/faces/billPdf.xhtml?bill_id=201720180SB100andversion=20170SB10087CHP (accessed July 20, 2023).
California Legislature SB-1020 (2022). Clean Energy, Jobs, and Affordability Act of 2022. Available online at: https://leginfo.legislature.ca.gov/faces/billTextClient.xhtml?bill_id=202120220SB1020 (accessed July 20, 2023).
California Legislature SB-1339 (2018). Electricity: microgrids: tariffs. Available online at: https://leginfo.legislature.ca.gov/faces/billTextClient.xhtml?bill_id=201720180SB1339 (accessed July 20, 2023).
CARB (2022). California releases final proposal for world-leading climate action plan that drastically reduces fossil fuel dependence, slashes pollution. Available online at: https://ww2.arb.ca.gov/news/california-releases-final-2022-climate-scoping-plan-proposal (accessed July 20, 2023).
Cassels, L. (2023). A solar grid in a Wimauma neighborhood withstood Hurricane Ian. Tampa Bay Times. Available online at: https://www.tampabay.com/news/business/2023/03/08/soar-grid-wimauma-neighborhood-withstood-hurricane-ian/ (accessed July 20, 2023).
CEC (2020). Electric Program Investment Charge 2020 Annual Report. CEC-500-2021-029. Available online at: https://www.energy.ca.gov/sites/default/files/2021-05/CEC-500-2021-029.pdf (accessed July 20, 2023).
CEC (n.d.) Electric Program Investment Charge Program – EPIC. Available online at: https://www.energy.ca.gov/programs-and-topics/programs/electric-program-investment-charge-epic-program (accessed May 10 2023).
Chen, B., Wang, J., Lu, X., Chen, C., and Zhao, S. (2020). Networked microgrids for grid resilience, robustness, and efficiency: A review. IEEE Trans. Smart Grid 12, 18–32. doi: 10.1109/TSG.2020.3010570
City of Berkeley (2018). Berkeley Energy Transformation (Beat) Project: Case study. Available online at: https://berkeleyca.gov/sites/default/files/2022-03/BEAT_Case_Study.pdf (accessed July 20, 2023).
Cohn, L. (2021). How rural electric cooperatives can power through California outages with microgrids. Microgrid Knowledge. Available online at: https://www.microgridknowledge.com/distributed-energy/article/11427860/how-rural-electric-cooperatives-can-power-through-california-outages-with-microgrids (accessed July 20, 2023).
Cox, E. (2023). “I hope they shouldn't happen”: Social vulnerability and resilience to urban energy disruptions in a digital society in Scotland. Energy Res. Soc. Sci. 95, 102901. doi: 10.1016/j.erss.2022.102901
CPUC (1951). Public Utilities Act, Section 218(b). Available online at: https://leginfo.legislature.ca.gov/faces/codes_displaySection.xhtml?sectionNum=218andlawCode=PUC (accessed November 30, 2022).
CPUC (2021a). CPUCtakes action to modernize electric grid for high distributed energy resources future. Available online at: https://www.cpuc.ca.gov/news-and-updates/all-news/cpuc-takes-action-to-modernize-electric-grid-for-high-distributed-energy-resources-future (accessed July 20, 2023).
CPUC (2021b). Resilience and microgrids. Available online at: https://www.cpuc.ca.gov/resiliencyandmicrogrids (accessed November 30, 2022).
CPUC (2022). Rulemaking 19-09-009. Order instituting rulemaking regarding microgrids pursuant to Senate Bill 1339 and Resiliency Strategies. Staff Proposal for Microgrid Incentive Program Plan Implementation. Available online at: https://www.cpuc.ca.gov/-/media/cpuc-website/divisions/energy-division/documents/resiliency-and-microgrids/ruling_mip_staff_proposal.pdf (accessed July 20, 2023).
CPUC (2023). Rulemaking 19-09-009. Order instituting rulemaking regarding microgrids pursuant to Senate Bill 1339 and Resiliency Strategies. Decision adopting implementation rules for the Microgrid Incentive Program. Available online at: https://www.cpuc.ca.gov/-/media/cpuc-website/divisions/energy-division/documents/resiliency-and-microgrids/505732868.pdf (accessed July 20, 2023).
Firestone, J., Hoen, B., Rand, J., Elliott, D., Hübner, G., and Pohl, J. (2018). Reconsidering barriers to wind power projects: community engagement, developer transparency and place. J. Environ. Policy Plann. 20, 370–386. doi: 10.1080/1523908X.2017.1418656
Gaggero, G. B., Girdinio, P., and Marchese, M. (2021). Advancements and research trends in microgrids cybersecurity. Appl. Sci. 11, 7363. doi: 10.3390/app11167363
Gilani, M. A., Kazemi, A., and Ghasemi, M. (2020). Distribution system resilience enhancement by microgrid formation considering distributed energy resources. Energy 191, 116442. doi: 10.1016/j.energy.2019.116442
Goss, M., Swain, D. L., Abatzoglou, J. T., Sarhadi, A., Kolden, C. A., Williams, A. P., et al. (2020). Climate change is increasing the likelihood of extreme autumn wildfire conditions across California. Environ. Res. Lett. 15, 094016. doi: 10.1088/1748-9326/ab83a7
Grosspietsch, D., Saenger, M., and Girod, B. (2019). Matching decentralized energy production and local consumption: A review of renewable energy systems with conversion and storage technologies. Energy Environ. 8, e336. doi: 10.1002/wene.336
Guirguis, K., Gershunov, A., Hatchett, B., Shulgina, T., DeFlorio, M. J., Subramanian, A. C., et al. (2023). Winter wet–dry weather patterns driving atmospheric rivers and Santa Ana winds provide evidence for increasing wildfire hazard in California. Clim. Dyn. 60, 1729–1749. doi: 10.1007/s00382-022-06361-7
Guliasi, L. (2021). Toward a political economy of public safety power shutoff: Politics, ideology, and the limits of regulatory choice in California. Energy Res. Soc. Sci. 71, 101842. doi: 10.1016/j.erss.2020.101842
Helmrich, A. M., and Chester, M. V. (2022). Reconciling complexity and deep uncertainty in infrastructure design for climate adaptation. Sustain. Resilient Infrastr. 7, 83–99. doi: 10.1080/23789689.2019.1708179
Hervás-Zaragoza, J., Colmenar-Santos, A., Rosales-Asensio, E., and Colmenar-Fernández, L. (2022). Microgrids as a mechanism for improving energy resilience during grid outages: A post COVID-19 case study for hospitals. Renew. Energy 199, 308–319. doi: 10.1016/j.renene.2022.08.132
Hess, D. J., and Lee, D. (2020). Energy decentralization in California and New York: Conflicts in the politics of shared solar and community choice. Renew. Sustain. Energy Rev. 121, 109716. doi: 10.1016/j.rser.2020.109716
Hirsch, A., Parag, Y., and Guerrero, J. (2018). Microgrids: A review of technologies, key drivers, and outstanding issues. Renew. Sustain. Energy Rev. 90, 402–411. doi: 10.1016/j.rser.2018.03.040
Hussain, A., Bui, V. H., and Kim, H. M. (2019a). Microgrids as a resilience resource and strategies used by microgrids for enhancing resilience. Appl. Energy 240, 56–72. doi: 10.1016/j.apenergy.2019.02.055
Hussain, A., Rousis, A. O., Konstantelos, I., Strbac, G., Jeon, J., and Kim, H. M. (2019b). Impact of uncertainties on resilient operation of microgrids: A data-driven approach. IEEE Access. 7, 14924–14937. doi: 10.1109/ACCESS.2019.2891786
Hwang, S., Tongsopit, S., and Kittner, N. (2023). Transitioning from diesel backup generators to PV-plus-storage microgrids in California public buildings. Sustain. Produc. Consumpt. 38, 252–265. doi: 10.1016/j.spc.2023.04.001
IPCC (2021). “Summary for Policymakers,” in Climate Change 2021: The Physical Science Basis. Contribution of Working Group I to the Sixth Assessment Report of the Intergovernmental Panel on Climate Change, eds. V., Masson-Delmotte, P. Zhai, A. Pirani, S.L. Connors, C. Péan, S. Berger, et al. (Cambridge, UK: Cambridge University Press) 3–32.
Jenn, A., and Highleyman, J. (2022). Distribution grid impacts of electric vehicles: A California case study. Iscience 25, 103686. doi: 10.1016/j.isci.2021.103686
Johnston, J., and Cushing, L. (2020). Chemical exposures, health, and environmental justice in communities living on the fenceline of industry. Curr. Environ. Health Rep. 7, 48–57. doi: 10.1007/s40572-020-00263-8
Kim, D., and Fischer, A. (2021). Distributed energy resources for net zero: An asset or a hassle to the electricity grid? International Energy Agency (IEA). Available online at: https://www.iea.org/commentaries/distributed-energy-resources-for-net-zero-an-asset-or-a-hassle-to-the-electricity-grid (accessed July 20, 2023).
Kramer, H. A., Mockrin, M. H., Alexandre, P. M., and Radeloff, V. C. (2019). High wildfire damage in interface communities in California. Int. J. Wildland Fire 28, 641–650. doi: 10.1071/WF18108
Krawchenko, T., Keefe, J., Manuel, P., and Rapaport, E. (2016). Coastal climate change, vulnerability and age friendly communities: Linking planning for climate change to the age friendly communities agenda. J. Rural Stud. 44, 55–62. doi: 10.1016/j.jrurstud.2015.12.013
Lightner, E., Leader, J., Berdahl, S., Cory, K., Morgenstein, J., and Schwabe, P. (2021). “Voices of Experience: Microgrids for Resiliency,” in National Renewable Energy Lab (NREL). NREL/BK-7A40-75909. Available online at: https://www.nrel.gov/docs/fy21osti/75909.pdf (accessed July 20, 2023).
Lukanov, B. R., and Krieger, E. M. (2019). Distributed solar and environmental justice: Exploring the demographic and socio-economic trends of residential PV adoption in California. Energy Policy 134, 110935. doi: 10.1016/j.enpol.2019.110935
Masri, S., Scaduto, E., Jin, Y., and Wu, J. (2021). Disproportionate impacts of wildfires among elderly and low-income communities in California from 2000–2020. Int. J. Environ. Res. Public Health 18, 3921. doi: 10.3390/ijerph18083921
Méndez, M., Flores-Haro, G., and Zucker, L. (2020). The (in) visible victims of disaster: Understanding the vulnerability of undocumented Latino/a and indigenous immigrants. Geoforum 116, 50–62. doi: 10.1016/j.geoforum.2020.07.007
Muñoz-Erickson, T. A., Selkirk, K., Hobbins, R., Miller, C., Feagan, M., Iwaniec, D. M., et al. (2021). Anticipatory Resilience Bringing Back the Future into Urban Planning and Knowledge Systems. In Resilient Urban Futures (Cham: Springer) 159–172. doi: 10.1007/978-3-030-63131-4_11
Murillo, R. I. (2020). A (Dangerous) new normal-public safety power shutoffs (PSPS): A look into California utility de-energization authority and the potential for its abuse. Santa Clara Law. Rev. 61, 653. Available online at: https://digitalcommons.law.scu.edu/lawreview/vol61/iss2/6
NESTA (2022). Anticipatory regulation. Available online at: https://www.nesta.org.uk/feature/innovation-methods/anticipatory-regulation/ (accessed November 30, 2022).
Nordman, B., Piette, M., and Khandekar, A. (2023). Electricity Price Communication in California and Beyond. UC Berkeley: California Institute for Energy and Environment (CIEE).
O'Beirne, P., Battersby, F., Mallett, A., Aczel, M., Makuch, K., Workman, M., et al. (2020). The UK net-zero target: Insights into procedural justice for greenhouse gas removal. Environ. Sci. Policy 112, 264–274. doi: 10.1016/j.envsci.2020.06.013
O'Neill, S. M., Diao, M., Raffuse, S., Al-Hamdan, M., Barik, M., Jia, Y., et al. (2021). A multi-analysis approach for estimating regional health impacts from the 2017 Northern California wildfires. J. Air Waste Manage. Assoc. 71, 791–814. doi: 10.1080/10962247.2021.1891994
Quandt, A., Grafton, D., Gorman, K., Dawson, P. M., Ibarra, C., Mayes, E., et al. (2023). Mitigation and adaptation to climate change in San Diego County, California. Mitigat. Adapt. Strat. Global Change 28, 7. doi: 10.1007/s11027-022-10041-6
Radeloff, V. C., Helmers, D. P., Kramer, H. A., Mockrin, M. H., Alexandre, P. M., Bar-Massada, A., et al. (2018). Rapid growth of the US wildland-urban interface raises wildfire risk. Proc. Nat. Acad. Sci. 115, 3314–3319. doi: 10.1073/pnas.1718850115
Rapaport, E., Manuel, P., Krawchenko, T., and Keefe, J. (2015). How can aging communities adapt to coastal climate change? Planning for both social and place vulnerability. Canadian Public Policy 41, 166–177. doi: 10.3138/cpp.2014-055
RCRC (2022). Rural County Representatives of California reports Legislators urge CPUC to adopt rules for recurring fast trip power outages. Available online at: https://goldrushcam.com/sierrasuntimes/index.php/news/local-news/41922-rural-county-representatives-of-california-reports-legislators-urge-cpuc-to-adopt-rules-for-recurring-fast-trip-power-outages-more-than-2-200-fast-trip-power-outages-in-pg-e-service-territory-this-year (accessed July 20, 2023).
Reimagine Power (2022). Community microgrid policy recommendations: California Advanced Energy Communities Knowledge Transfer [unpublished].
Rickerson, W., Zitelman, K., and Jones, K. (2022). Valuing resilience for microgrids: Challenges, innovative approaches, and state needs. Report for National Association of State Energy Officials (NASEO) and the National Association of Regulatory Utility Commissioners (NARUC) Microgrids State Working Group. Available online at: https://www.naseo.org/data/sites/1/documents/publications/NARUC_Resilience_for_Microgrids_INTERACTIVE_021122.pdf (accessed July 20, 2023).
Sanders, B. F., Schubert, J. E., Kahl, D. T., Mach, K. J., Brady, D., AghaKouchak, A., et al. (2023). Large and inequitable flood risks in Los Angeles, California. Nat. Sustain. 6, 47–57. doi: 10.1038/s41893-022-00977-7
Sandys, L., Hardy, J., and Green, R. (2017). Reshaping Regulation: Powering from the future. Available online at: https://spiral.imperial.ac.uk/bitstream/10044/1/83959/6/Reshaping-Regulation-Powering-from-the-future.pdf (accessed July 20, 2023).
Schwartz, B. (2021). Attempts to Commercialize Microgrids Fall Short in California. T&D World. Available online at: https://www.tdworld.com/microgrids/article/21153964/attempts-to-commercialize-microgrids-fall-short-in-california (accessed July 20, 2023).
Serna, J. (2019). Southern California Edison power lines sparked deadly Thomas fire, investigators find. Los Angeles Times. Available online at: https://www.latimes.com/local/lanow/la-me-ln-thomas-fire-edison-cause-20190313-story.html (accessed July 20, 2023).
Shandiz, S. C., Foliente, G., Rismanchi, B., Wachtel, A., and Jeffers, R. F. (2020). Resilience framework and metrics for energy master planning of communities. Energy 203, 117856. doi: 10.1016/j.energy.2020.117856
Silverstein, A., Gramlich, R., and Goggin, M. (2018). A Customer-Focused Framework for Electric System Resilience. New York: NRDC (Natural Resource Defense Council) and EDF (Environmental Defense Fund).
Smeloff, E., Kristov, L., and Hastings, W. (2020). Reply Comments of Vote Solar and The Climate Center (the “Joint Parties”) on the Track 2 Microgrid and Resiliency Strategies. Rulemaking 19-09-009. Available online at: https://theclimatecenter.org/wp-content/uploads/2020/08/200828-Final-VS-TCC-Reply-Comments-Track-2.pdf (accessed July 20, 2023).
Smith, I. D., Kirkegaard, J. K., and Szulecki, K. (2023). A functional approach to decentralization in the electricity sector: Learning from community choice aggregation in California. J. Environ. Plann. Manage. 66, 1305–1335. doi: 10.1080/09640568.2022.2027233
Stein, A., and Moser, C. (2014). Asset planning for climate change adaptation: lessons from Cartagena, Colombia. Environ. Urbaniz. 26, 166–183. doi: 10.1177/0956247813519046
Sultan, V., Alzahrani, A., Bitar, H., and Alharbi, N. (2016). Is California's aging infrastructure the principal contributor to the recent trend of power outage? J. Commun. Comput. 13, 225–233. doi: 10.17265/1548-7709/2016.05.003
Ton, D. T., and Smith, M. A. (2012). The U.S. department of energy's microgrid initiative. Electricity J. 25, 84–94. doi: 10.1016/j.tej.2012.09.013
UK Financial Conduct Authority (FCA) (2015). Regulatory sandbox. Available online at: https://www.fca.org.uk/publication/research/regulatory-sandbox.pdf (accessed July 20, 2023).
UNISDR (2009). 2009 UNISDRterminology on disaster risk reduction. Available online at: https://www.undrr.org/publication/2009-unisdr-terminology-disaster-risk-reduction (accessed July 20, 2023).
US DOE (2023). U.S. Department of Energy. Microgrid installations database. Available online at: https://doe.icfwebservices.com/microgrid (accessed July 20, 2023).
USDN (n.d.). From community engagement to ownership tools for the field with case studies of four municipal community-driven environmental racial equity committees. Facilitating Power Movement Strategy Center the National Association of Climate Resilience Planners. Available online at: https://www.usdn.org/uploads/cms/documents/community_engagement_to_ownership_-_tools_and_case_studies_final.pdf (accessed July 20, 2023).
Van Dyke, K., Schwartz, M., Teeter-Moore, K., Ventura, F., and Kumar, S. (2019). Berkeley Energy Assurance Transformation (BEAT) final project report: Advancing clean energy microgrid communities in an urban context. California Energy Commission. Publication Number: CEC-500-2019-014. Available online at: https://www.energy.ca.gov/sites/default/files/2021-05/CEC-500-2019-014.pdf (accessed July 20, 2023).
Verba, N., Nixon, J. D., Gaura, E., Dias, L. A., and Halford, A. (2022). A community energy management system for smart microgrids. Electr. Power Syst. Res. 209, 107959. doi: 10.1016/j.epsr.2022.107959
von Lazar, L. (2023). Rethinking the Modern Grid: Black and Veatch 2022-2023 Electric Report. Black and Veatch. Available online at: https://downloads.ctfassets.net/6aztiy11c9mv/1ga0mX4hJjcc5xoqg5LfAe/91be489d3f08901a1e7c411b87846da0/22_ElectricReport_Final__2_.pdf (accessed July 20, 2023).
von Meier, A., and Kammen, D. M. (2021). White Paper: The EcoBlock Project and the “own use” exemption under Public Utilities Codes Section 218 – A way forward for privately operated microgrids [white paper]. UC Berkeley: California Institute for Energy and Environment (CIEE). Available online at: https://escholarship.org/uc/item/1s88n8c6 (accessed December 20, 2022).
Wang, Y., Rousis, A. O., and Strbac, G. (2020). On microgrids and resilience: A comprehensive review on modeling and operational strategies. Renew. Sustain. Energy Rev. 134, 110313. doi: 10.1016/j.rser.2020.110313
Wong-Parodi, G. (2020). When climate change adaptation becomes a “looming threat” to society: Exploring views and responses to California wildfires and public safety power shutoffs. Energy Res. Soc. Sci. 70, 101757. doi: 10.1016/j.erss.2020.101757
Wood, E. (2023). Proposed decision moves California closer to making $200M available for community microgrids. Microgrid Knowledge. Available online at: https://www.microgridknowledge.com/community-microgrids/article/33000087/microgrid-incentive-program-for-communities-edges-closer-to-becoming-reality-in-california (accessed July 20, 2023).
Workman, M., Darch, G., Dooley, K., Lomax, G., Maltby, J., and Pollitt, H. (2021). Climate policy decision making in contexts of deep uncertainty-from optimisation to robustness. Environ. Sci. Policy. 120, 127–137. doi: 10.1016/j.envsci.2021.03.002
Workman, M., Dooley, K., Lomax, G., Maltby, J., and Darch, G. (2020). Decision making in contexts of deep uncertainty-An alternative approach for long-term climate policy. Environ. Sci. Policy 103, 77–84. doi: 10.1016/j.envsci.2019.10.002
Wu, R., and Sansavini, G. (2020). Integrating reliability and resilience to support the transition from passive distribution grids to islanding microgrids. Appl. Energy 272, 115254. doi: 10.1016/j.apenergy.2020.115254
Keywords: microgrid, natural disasters, resilience (environmental), community, energy, social science research
Citation: Aczel MR and Peffer TE (2023) Advancing California's microgrid communities through anticipatory energy resilience. Front. Clim. 5:1145231. doi: 10.3389/fclim.2023.1145231
Received: 15 January 2023; Accepted: 09 June 2023;
Published: 03 August 2023.
Edited by:
Adrian Gault, London School of Economics and Political Science, United KingdomReviewed by:
Helen Poulter, University of Edinburgh, United KingdomGeorge Day, Energy Systems Catapult, United Kingdom
Copyright © 2023 Aczel and Peffer. This is an open-access article distributed under the terms of the Creative Commons Attribution License (CC BY). The use, distribution or reproduction in other forums is permitted, provided the original author(s) and the copyright owner(s) are credited and that the original publication in this journal is cited, in accordance with accepted academic practice. No use, distribution or reproduction is permitted which does not comply with these terms.
*Correspondence: Miriam R. Aczel, aczel@berkeley.edu