- 1Department of Geoinformatics—Z_GIS, Paris Lodron University of Salzburg, Salzburg, Austria
- 2Christian Doppler Laboratory for Geospatial and EO-Based Humanitarian Technologies, Department of Geoinformatics—Z_GIS, Paris Lodron University of Salzburg, Salzburg, Austria
- 3Adaptive Reflective Teams, Fraunhofer Institute for Intelligent Analysis and Information Systems IAIS, Sankt Augustin, Germany
- 4Stockholm Environment Institute (SEI), Stockholm, Sweden
- 5Western Norway Research Institute, Sogndal, Norway
- 6Institute of Economic Structures Research (GWS) mbH, Osnabrück, Germany
- 7Centre Oceanográfic de Balears, Instituto Español de Oceanografía (CSIC-IEO), Palma, Spain
- 8LEESU, École des Ponts, Marne-la-Vallée, France
- 9AMUP, INSA de Strasbourg, Strasbourg, France
- 10Ramboll France SAS, Aix-en-Provence, France
- 11Water Systems and Global Change Group, Wageningen University, Wageningen, Netherlands
- 12Nordland Research Institute, Bodø, Norway
- 13Center for Climate Change and Transformation, Eurac Research, Bolzano, Italy
- 14Departamento de Física de la Tierra y Astrofísica, Facultad de Ciencias Físicas, Universidad Complutense de Madrid, Madrid, Spain
- 15RiskLab – Weather, Climate & Natural Hazards, GeoSphere Austria, Vienna, Austria
As the climate crisis continues to worsen, there is an increasing demand for scientific evidence from Climate Risk and Vulnerability Assessments (CRVA). We present 12 methodological advancements to the Impact Chain-based CRVA (IC-based CRVA) framework, which combines participatory and data-driven approaches to identify and measure climate risks in complex socio-ecological systems. The advancements improve the framework along five axes, including the existing workflow, stakeholder engagement, uncertainty management, socio-economic scenario modeling, and transboundary climate risk examination. Eleven case studies were conducted and evaluated to produce these advancements. Our paper addresses two key research questions: (a) How can the IC-based CRVA framework be methodologically advanced to produce more accurate and insightful results? and (b) How effectively can the framework be applied in research and policy domains that it was not initially designed for? We propose methodological advancements to capture dynamics between risk factors, to resolve contradictory worldviews, and to maintain consistency between Impact Chains across policy scales. We suggest using scenario-planning techniques and integrating uncertainties via Probability Density Functions and Reverse Geometric Aggregation. Our research examines the applicability of IC-based CRVAs to address transboundary climate risks and integrating macro-economic models to reflect possible future socio-economic exposure. Our findings demonstrate that the modular structure of IC-based CRVA allows for the integration of various methodological advancements, and further advancements are possible to better assess complex climate risks and improve adaptation decision-making.
1. Introduction
European policymakers face growing pressure to take action on climate change, such as following local climate actions plans recommended by the European Union (European Commission, 2021). However, effective climate change adaptation (hereafter adaptation) requires a scientific information-base of empirically grounded local, actionable knowledge concerning climate risks and adaptation options (Kirchhoff et al., 2013; Scherhaufer, 2014).
Assessing climate risks at the local level and providing actionable evidence remains a challenge, despite increasing sophistication of climate projections. The complexity and variability of factors that shape climate risks, which can lead to uncertain results complicate the assessment process (Viner et al., 2020; Aall and Groven, 2022). Furthermore, influence of factors such as policy decisions being driven by economics or views that seem to contradict with adaptation goals hinder the uptake of outcomes into action (Storbjörk, 2007; Klein and Juhola, 2014). As a result, the outcomes of such assessments are rarely utilized in decision-making processes (Larsen et al., 2012; Klein and Juhola, 2014).
The European Environmental Agency (EEA) has identified key lessons learned from conducting national and subnational CRVA and developing national adaptation plans (Füssel et al., 2018). The report highlights that while national CRVA provide general overviews and assist in setting thematic and regional priorities, targeted adaptation measures require subnational and local information. Additionally, the report, inter alia, underscores the need for common metrics to compare and identify priority areas, proactive stakeholder engagement, systematic assessment of uncertainties, exploration of non-climatic factors influencing exposure and vulnerability, and attention to cross-sectoral interactions and transboundary impacts (Füssel et al., 2018). Furthermore, CRVA frameworks should follow standardized procedures to produce comparable results across time and space while being adaptable to different contexts and policy scales (European Commission, 2019, 2020, 2021; ISO 14090, 2019; Bundesregierung, 2022).
This paper examines the Impact Chain-based CRVA (IC-based CRVA) framework as the departure point to address these challenges. Numerous guidelines and handbooks advise on CRVA (Daze et al., 2009; UNDP, 2010; Bharwani et al., 2013). However, the IC-based CRVA framework goes further by providing a standardized approach that covers various sectors and spatial levels, as well as time horizons. It offers step-by-step guidance for designing and implementing CRVA (Zebisch et al., 2021). As such, the framework holds promise for being advanced in a way to respond to the needs identified by the EEA. In here, we advance the framework by suggesting methodological advancements resulting from case studies conducted during the course of the “UNCHAIN - Unpacking Climate Change Impact Chains” project. By doing so, eventually the accuracy, insightfulness, and impact of CRVA outcomes may be improved. Furthermore, testing the method's potential for use in research and policy domains it was not originally designed for may ultimately upscale its potential and increase the uptake of assessment results into decision-making. Accordingly, our two research questions are:
(a) How can the IC-based CRVA framework be advanced methodologically to produce more accurate, insightful or impactful results?
(b) How effectively can the IC-based CRVA framework be applied in research and policy domains that it was not initially designed for?
The article is targeted toward practitioners and researchers who are considering implementing the IC-based CRVA framework, and second, to inform and enhance the upcoming 2023 update of the Vulnerability Sourcebook (VS) (Fritzsche et al., 2014).
2. The IC-based CRVA framework and the vulnerability sourcebook
The IC-based CRVA framework assesses climate-related risks through a combination of collaborative knowledge creation and quantitative data analysis to eventually identify specific adaptation measures. The framework is based on Impact Chains (Schneiderbauer et al., 2013) and explained in detail in the Vulnerability Sourcebook (Fritzsche et al., 2014) and the Risk Supplement to the Vulnerability Sourcebook (GIZ Eurac, 2017). These resources were developed for the German Agency for International Cooperation (GIZ) as an indicator-based approach to measure and compare vulnerability in different locations, originally for policymaking and national adaptation plan design in low-income countries (Zebisch et al., 2021).
The VS has been applied in national adaptation plans and scientific studies since 2014. As a result, is has been modified for specific contexts (Table 1) and incorporated into the ISO Standard for Adaptation to climate change (ISO 14090, 2019). Supplementary Table 1 provides a detailed overview of past applications. The term “IC-based CRVA” refers to the framework outlined in the VS, the Risk Supplement to the VS, and the other derivatives (GIZ, 2018; Rome et al., 2018).
The IC-based CRVA framework comprises eight modules, which are divided into four participatory and four operational ones (see Figure 1, left side). The participatory modules focus on knowledge co-production techniques and provide the backbone for the operational modules, which assess quantitative, indicator-based data and models1 (Zebisch et al., 2021).
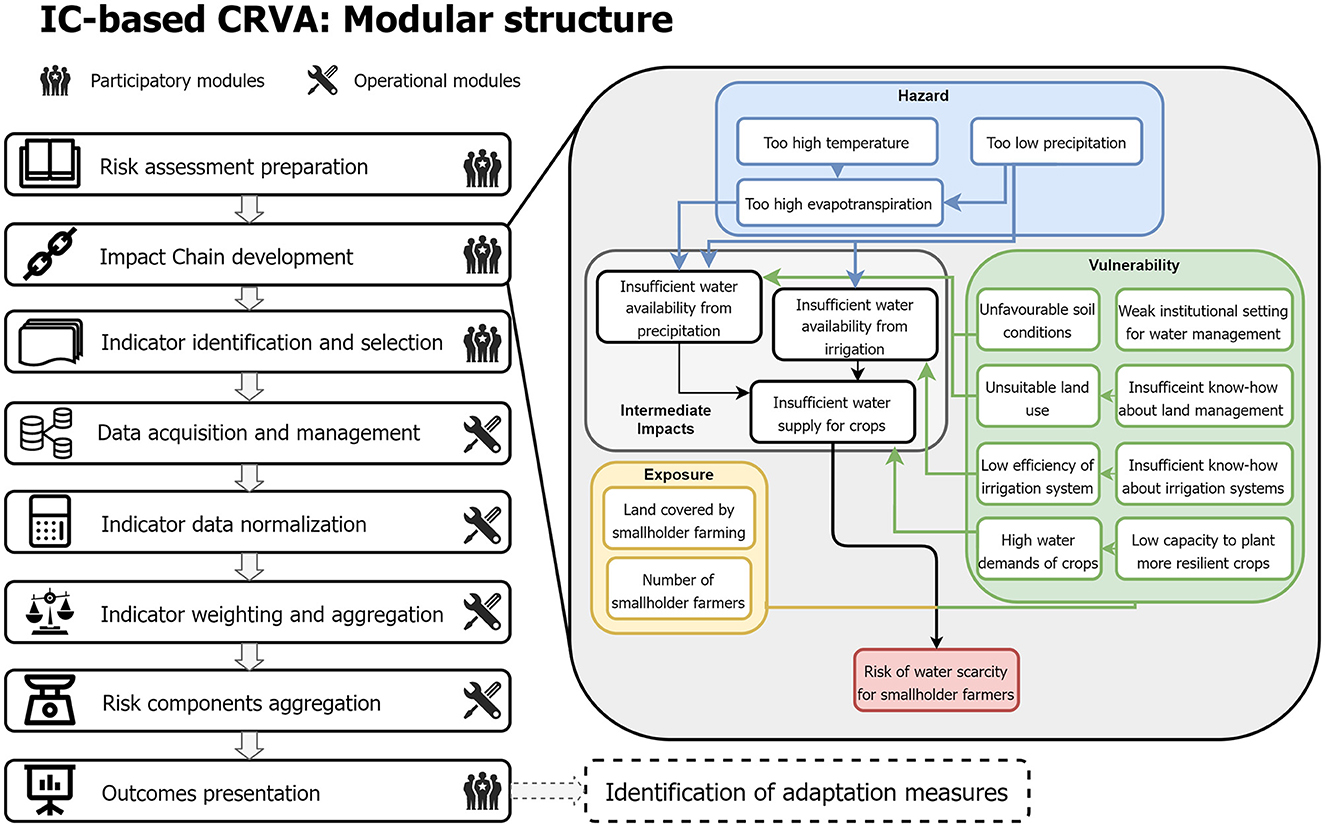
Figure 1. The IC-based CRVA modules of the Risk Supplement to the VS (left) and a simple Impact Chain (right). The Impact Chain development module is the distinctive characteristic on which all other modules build.
Collaborative efforts between researchers and the policy- and decision-making community are increasingly being used to integrate assessment outcomes into decision-making (Lövbrand and Stripple, 2011; Hoppe and Wesselink, 2014; Dannevig and Aall, 2015; Graham and Mitchell, 2016). These efforts are moving toward a more interactive approach, where researchers and practitioners work together to create actionable results, instead of just transferring knowledge from research to practice (Klein and Juhola, 2014; Runhaar et al., 2018; Palutikof et al., 2019; Norström et al., 2020). Such partnerships can enhance the perceived saliency, credibility, and legitimacy of outcomes, facilitate the inclusion of multiple knowledge systems, and foster mutual learning and problem ownership (Gusfield, 1989; Kabisch et al., 2014; Greiving et al., 2015; Kienberger et al., 2016; Hansson and Polk, 2018; Bremer et al., 2019; Cvitanovic et al., 2019; Kahlenborn et al., 2021).
The VS's participatory modules offer guidance on establishing communication between researchers and stakeholders, identifying information needs, developing Impact Chains collaboratively, selecting appropriate indicators, and presenting and validating outcomes through various means such as maps, risk matrices, tables, diagrams or narratives. The validation of the Impact Chains involves independent experts who were not involved in the co-production process. At the core of the participatory approach is the development of Impact Chains, which are conceptual diagrams illustrating the qualitative cause-effect structures that lead to climate change risks (see Figure 1, right side).
An Impact Chain organizes risk factors based on hazard, exposure, and vulnerability factors as defined in the IPCC AR5 (Field, 2014; Huq et al., 2014).2 Figure 1 (right side) shows how an Impact Chain focuses on specific hazard factors (such as high temperatures), and identifies exposure factors (such as smallholder farmers in a specific location), intermediate impacts on biophysical elements, and the final human-centered risk. Vulnerability factors, which can increase or decrease the risk, include non-climatic dimensions. Identifying the most influential vulnerability factors is critical for creating a meaningful, context-specific Impact Chain, and requires local knowledge and a deep understanding of driving forces involved (Fritzsche et al., 2014; Zebisch et al., 2021; Menk et al., 2022).
To back the Impact Chains with quantitative (spatial) data, the framework provides operational modules describing indicator-based assessments. Indicators are a useful tool for turning complex structures into something measurable and comparable across regions and over time (Vincent and Cull, 2014). They are effective in conveying messages and providing policy information, especially when used comparatively across a large number of regions. Once data is acquired to populate the indicators, they are normalized, weighted and aggregated into a composite risk indicator. Examples of a complete assessment workflow can be found in the annex of the VS (GIZ, 2014) i.e., applied to assess risk of water scarcity for smallholder farmers in Bolivia.
As part of the UNCHAIN project, we conducted eleven case studies that followed the IC-based CRVA framework with varying degrees of strictness. However, all of them incorporated “new” elements into the framework, which we refer to as “methodological advancements” in this paper. The method section details how we distilled the most noteworthy methodological advancements from the various case studies. In the results section, we explain each methodological advancement in the context in which it was applied. Then, we discuss the main findings, their implications and limitations and close with a conclusion.
3. Materials and methods
3.1. The UNCHAIN research pipeline and the case studies
To better incorporate the EEA-identified requirements into the IC-based CRVA framework, we advanced the framework across five “innovation areas.” Some of these areas relate to existing modules in the framework, such as (1) elaborating the existing modules or (2) improving stakeholder engagement. Other areas are not yet reflected in the framework, such as (3) managing uncertainty and (4) modeling socio-economic scenarios. Finally, we explored the application of the framework in a new context by (5) examining transboundary climate risks through Impact Chains. As a methodological advancement we understand developing or refining a method, technique, or approach to improve research or problem solving (based on Bergh et al., 2022).
Our research pipeline (Figure 2) began with a literature-based State-of-the-Art analysis to identify research questions related to challenges and opportunities with regards to the innovation areas, which were then addressed through a common case study protocol. This protocol provided guidelines for preparing, conducting and evaluating the case studies, facilitating consistency and an overarching case evaluation. Case study protocols are particularly useful in research projects involving multiple researchers and data collection across multiple locations and time periods (such as UNCHAIN) (Yin, 1994; Pervan and Maimbo, 2005).
Besides their focus on varying innovation areas, the case studies differed in several other respects, their topical and geographical foci, spatial and administrative scales and disciplinary representation of researchers. The project partners selected the topics, scope, and stakeholder groups for the case studies individually based on contextual relevance and predicted climate risks. The research pipeline accounted for the alignment of individual characteristics with the objectives of the project. Some cases were planned before the project phase began, and stakeholder contacts were established at that time. Other case studies were planned and conducted during the project phase (2019–2022).
3.2. Validating advancements
As part of the research pipeline, we developed an evaluation framework and validation criteria based on the works of Zeil and Lang (2009) and d'Oleire Oltmanns et al. (2015). These criteria were applied to identify the most noteworthy methodological advancements from the case studies. The validation process involved an evidence-based self-evaluation approach. Additionally, nine of the eleven case studies are being published in peer-reviewed publications and thereby provide an independent validation step.3 To determine whether a methodological advancement met the validation criteria, we assessed its relevance, applicability, comprehensibility, scientific validity, effectiveness, transferability and scalability. The criteria were not set with fixed thresholds due to the multifaceted nature of the cases.
We evaluated the criteria as follows:
• Relevance: Is the advancement relevant to the field, and does it address current research questions in the field of climate risk assessment? Does it provide useful and actionable information for decision-making?
• Applicability: Is the advancement generic and accessible to a wide range of users, regardless of their technical background?
• Comprehensibility: Is the advancement explained with sufficient detail for others to understand and replicate it?
• Scientific validity: Is the advancement built upon existing scientific knowledge and has it been peer-reviewed?
• Effectiveness: Can the advancement be implemented without primary data collection? Does it allow the integration of heterogeneous data? Was the implementation practical regarding timeframe and team size? Did it take into account stakeholder needs and perspectives and did it receive positive feedback?
• Transferability and scalability: Can the method be applied across a range of different contexts, locations, and scales?
3.3. Evaluating validation criteria
Following the case study phase, the leading researchers of each case study evaluated whether a methodological advancement met the relevant validation criteria based on their evidence. The main author (of this article) and each individual case study leading researcher then discussed the selection in an online interview. We chose to focus on one methodological aspect per case study to be able to explain its application in more detail in this article.4 The case studies themselves might have encompassed many more methodological aspects. In the results section, we will focus on how the advancements were applied and the lessons learned, rather than presenting how they passed individual validation criteria. However, for the sake of scientific validity, we provide all relevant material on the validation procedure in Supplementary Table 2.
3.4. Limitations
While we established validation criteria as guidelines to steer the selection process, not all methodological advancements needed to meet all criteria, due to the multifaceted nature of the case studies. To some extent, the decision was left to the main researchers. A further limitation is that we did not seek feedback from stakeholders specifically on the validation process to avoid overburdening them, as their insights and feedback were already required before in the research pipeline.
4. Results
The methodological advancements will be presented according to the “innovation areas” they contribute to, in the following order: (1) elaborating the existing modules of the IC-based CRVA framework, (2) improving stakeholder engagement, (3) managing uncertainty, (4) modeling socio-economic scenarios, and (5) examining transboundary climate risks. We organized each case study section into three parts: the addressed challenge or opportunity, the methodological advancement, and a conclusion. Figure 3 gives an overview of the case studies.
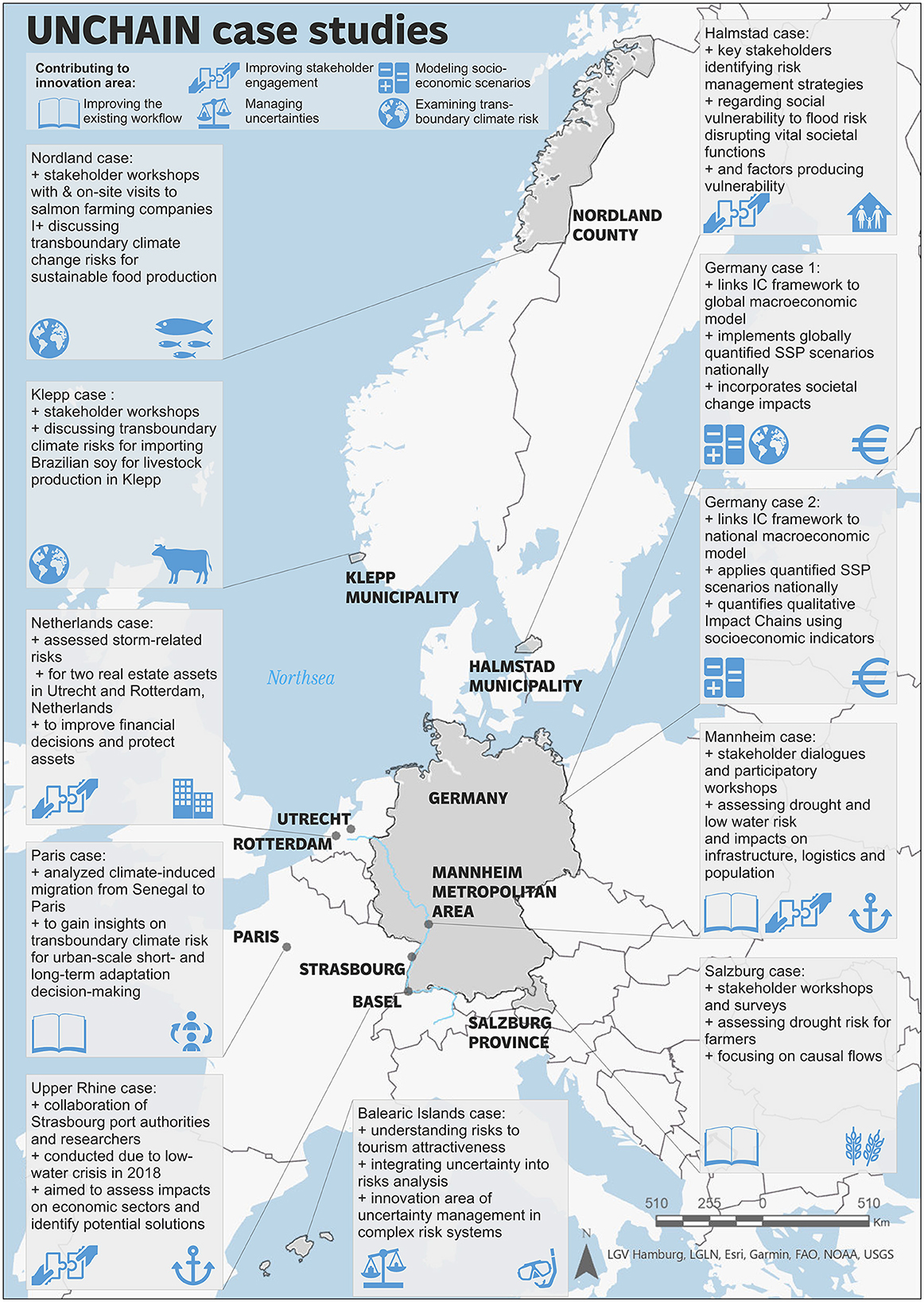
Figure 3. Case study locations and notable characteristics relevant to this article. The lower left icons indicate each case study's innovation area, while the lower right icon suggests the thematic focus.
4.1. Elaborating the existing modules of the IC-based CRVA framework
4.1.1. The Mannheim case A: using national impact chains for efficient and consistent CRVA at the regional level
Related publication: (Lückerath et al., 2023).
4.1.1.1. Challenge/opportunity
The Mannheim case built on the national climate Impact Chains (IC) included in the German Adaptation Strategy (Umweltbundesamt, 2016, 2019), to avoid developing local Impact Chains from scratch. The German National Impact Chains have been co-produced by the experts who developed the VS, by stakeholders from German Federal Agencies and Ministries, and by domain experts. These national ICs characterize the possible climate impacts on a national level, clustered into 15 fields of action. The Impact Chains visualize components of climate risk as a diagram. This graphical representation includes (1) direct physical impacts of climate related hazards on exposed system elements, (2) the sensitivities of the exposed system elements, (3) the nature of the damage, and (4) impacts of damage to system elements on other system elements. Indirect impacts may propagate and thus form ICs and are not restricted to one field of action or one sector.
4.1.1.2. Methodological advancement
For assessing the specific risk of two hazards in the Mannheim case, only a subset of the national Impact Chains was needed. That is, we extracted the relevant subset of components from the national Impact Chains regarding the selected hazards, the sectors represented by the participating stakeholders, the related national fields of action, and all related sensitivities and relational information. The original layout of this true subset of the national Impact Chain has been optimized for result documentation and reference, but it is not ideal as a basis for further elaboration. In the next step, the layout of this subset was transferred into a suitable online collaboration tool and collaboratively enriched with regional risk factors (exposures, sensitivities, capacities, stressors, impacts). The resulting Impact Chain was subsequently improved and validated in several post-processing cycles.
4.1.1.3. Conclusion
Based on the stakeholders' oral feedback and completed questionnaires, we believe that this method was a successful and efficient way to conduct IC-based CRVA, mitigating the often-criticized time demand of the approach. Furthermore, the multi-stakeholder regional CRVA fostered information exchange and awareness regarding climate risk and adaptation opportunities in this heterogeneous group.
The benefits of this approach included (a) a faster start and general time saving by starting with concrete examples, and (b) a resulting regional Impact Chain that is consistent with the national ICs (Umweltbundesamt, 2016) and does not “re-invent the wheel.” We believe that the latter point could be an advantage for planning subsequent adaptation measures and for acquiring their funding, because national funding is indicated per national field of (climate) action. Therefore, building local climate risk analysis on national Impact Chains and structuring elements of risk (exposure, sensitivity, capacity, impact) per national field of action facilitates the identification of funding opportunities. The regional IC, in turn, can serve as a starting point and context for local CRVA. The approach of nested scales allows to establish consistent links between local, regional, and even national adaptation measures. However, while national Impact Chains were available for Germany, globally this availability is still an exception. In the future, standard Impact Chains for various sectors and systems could be the basis for local adaptations.
4.1.2. The Salzburg case: moving beyond static impact chains to dynamic causal loop diagrams
4.1.2.1. Challenge/opportunity
The current Impact Chain notation style has limitations in capturing the dynamic nature of climate risk, which is influenced by complex interrelationships between system factors spanning socio-economic, political, and environmental realms (Menk et al., 2022). Factors contributing to risk are summarized into a final risk score that does not feed back into the system, failing to account for the feedback loops that shape risk over time.
4.1.2.2. Methodological advancement
To address this limitation, the Salzburg case study employed a Causal Loop Diagram (CLD), which depicts causal flows within a system and allows for a more nuanced understanding of the dynamic interrelationships between risk factors (Figure 4). Using CLDs to map a risk system acknowledges the balancing or reinforcing effects of these factors, providing a more comprehensive view of the risk landscape. The study used a combination of stakeholder engagement, spatial data analysis, and literature review to create a CLD for drought risk, highlighting the role of water availability as a major driver of risk, and identifying the wider range of factors that influence agricultural success or failure.
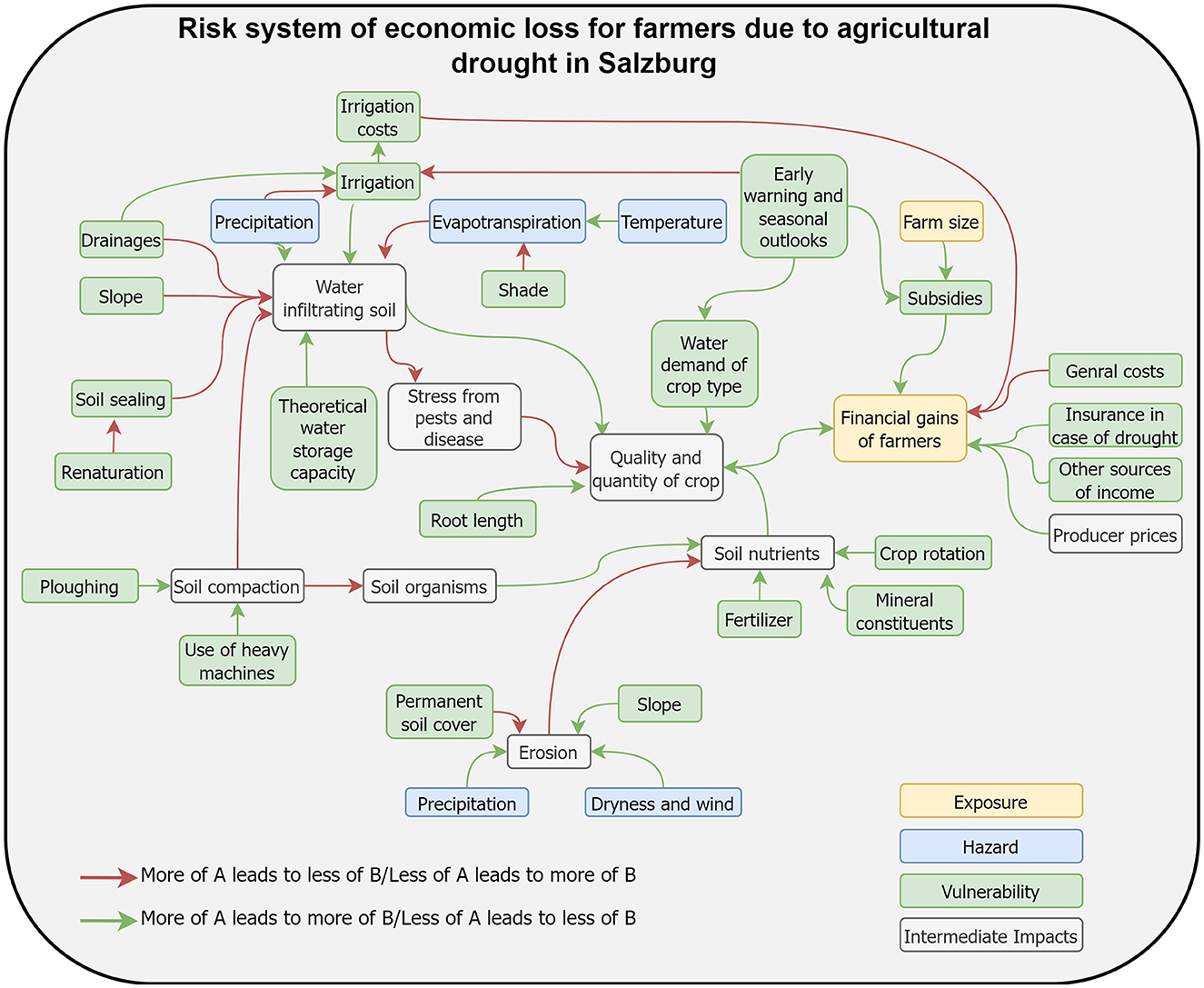
Figure 4. The impact chain in the style of a causal loop diagram. It has been developed based on a literature review, a stakeholder survey and workshop and has been approved in a second stakeholder workshop. The relationships are to be read as pairs of two.
4.1.2.3. Conclusion
The CLD-based approach was well-received by stakeholders, who appreciated the larger system understanding it provided and the identification of entry points for medium- and long-term planning. The study suggested that the use of CLDs can support the development of more effective and sustainable adaptation measures. However, the study also recognized that more extensive CLDs should be developed and presented as comprehensible sub-systems before being combined to create a bigger picture of the risk system.
4.1.3. The Paris case: moving beyond weighted arithmetic aggregation to reverse weighted geometric aggregation
4.1.3.1. Challenge/opportunity
Abstracting a complex Impact Chain into a simplified indicator requires careful attention to weighting and aggregation methods. To aggregate indicators into a composite indicator, the Vulnerability Sourcebook recommends weighted arithmetic aggregation. This method multiplies individual indicators by their weights, sums them, and then divides by the sum of their weights (Fritzsche et al., 2014). If individual indicators show extreme negative values, an alternative method, weighted geometric aggregation, may be used (as already suggested in the VS as a side-note). This method is popular in indicator construction and decision-making, as it is a prioritization tool in Analytic Hierarchy Process (Krejčí and Stoklasa, 2018) and other multi-criteria analyses. Weighted geometric aggregation limits substitutability between risk factory due to its bias toward low values. Notably, the Human Development Index has shifted from the arithmetic to the geometric method.
4.1.3.2. Methodological advancement
This methodological advancement to IC-based CRVA involved the use of reverse weighted geometric aggregation instead of the weighted arithmetic aggregation recommended by the VS. The reverse weighted geometric aggregation method assigns greater weight to particularly high-risk factors and avoids the risk of low-risk factors compensating for them in the final risk score, which can result in an underestimation of risk. In the Paris case study, risk scores resulting from arithmetic and reverse weighted geometric aggregations were compared. The results consistently showed that the scores produced by the reverse weighted geometric method were higher, particularly in cases where sub-indicators had relatively high dispersion among coefficients.
4.1.3.3. Conclusion
We argue that for most of the applications using the risk value obtained by IC-based CRVA it is more favorable to overestimate, than to underestimate, risk. Therefore, we suggest using reverse geometric aggregation, to shift the bias toward high values instead (Guillaumont, 2009). Given that the method accounts for the interdependencies of the system and produces higher scores, we believe that it is relevant to apply it. To ensure clarity and consistency, we recommend providing a more detailed explanation of the method in the next edition of the VS. Alternatively, the quadratic average method could be used to address this issue.
4.2. Improving stakeholder engagement
4.2.1. The Upper Rhine case: integrating TRIZ into IC-based CRVA for participatory identification of adaptation measures in contradictory situations
Related publication: (Coulibaly et al., 2022; Gobert and Rudolf, 2022).
4.2.1.1. Challenge/opportunity
Stakeholder knowledge and needs do not necessarily result in one Impact Chain that everyone can agree on, due to possibly diverging interests and opinions (Schneiderbauer et al., 2020).
4.2.1.2. Methodological advancement
The Upper Rhine case utilized TRIZ (Theory of Inventive Problem Solving) Inventive Design Method, which was originally developed in the engineering domain, and combined it with IC-based CRVA to address problems and find solutions in contradictory situations. The method involves breaking down a problem into its various components and is easily integrated with the IC-based CRVA framework. However, unlike Impact Chain development, this method is primarily focused on identifying solutions, particularly adaptation measures. The idea is to identify partial solutions for various parts of the problem. For instance, for a problem such as low waters inhibiting barges to transport regular volumes of freight, a partial solution, such as barges carrying less freight, could be identified. Partial solutions that are straightforwardly quantifiable are usually easy to resolve. However, this method also accounts for situations where different stakeholders hold differing “worldviews” about a problem and potential solutions. The method captures these worldviews in a special software, highlights contradictions, and then a consensus is sought in the next step.
4.2.1.3. Conclusion
Enriching IC-based CRVA with the TRIZ method improved participatory Impact Chain development and to identify and select indicators and adaptation measures. The approach involved a combination of workshops and individual interviews to account for the issue of individual stakeholders dominating discussions and influencing the general opinion. Working with the TRIZ software enabled a cross-breeding of qualitative and quantitative methods. This increased the perceived impartiality of the methods and results among stakeholders, which helps counteract the issue that simple qualitative methods are often considered insufficient or biased by stakeholders (Cheek et al., 2004).
However, the approach faced some challenges. Contradictory situations, especially on inherently subjective issues such as the level of social acceptability of a partial solution, were not always easy to solve. This difficulty resulted in a marginalization of qualitative problem dimensions, which are difficult to weight but are nonetheless essential to the identification of viable solutions. Additionally, the process was time-consuming and required deep involvement of the stakeholders, as well as a certain level of expertise to work with the TRIZ software. Nonetheless, the approach's combination of sociological and engineering expertise made it possible to convince stakeholders of the reliability and robustness of the results.
4.2.2. The Halmstad case: exploring social vulnerability through exploratory scenarios in the IC-based CRVA framework
Related publication: (Englund et al., 2023).
4.2.2.1. Challenge/opportunity
Climate change is expected to have significant impacts on social groups, with those with known vulnerabilities likely to be disproportionately affected and others becoming newly vulnerable. However, there is limited understanding of which groups will be affected and how, as well as the economic, social, and physical factors that drive their vulnerabilities. Social aspects of vulnerability are often difficult to measure and quantify (Hudson et al., 2019), and while the IC-based CRVA approach identifies knowledge, technology, institutions, and the economy as key drivers of vulnerability, it provides only a limited understanding of vulnerable groups, with generic representation of women and disadvantaged groups. To address this limitation, a method to assess social vulnerability was searched (see Birkmann, 2013 for an overview) that would engage stakeholders in a co-production process and align with the IC-based CRVA approach.
4.2.2.2. Methodological advancement
The Halmstad case explored flood hazards triggering cascades in the infrastructure system and their impacts on vulnerable groups in Halmstad Municipality, Sweden (Barquet et al., 2022). An exploratory scenario approach was adopted which is a form of storytelling that forecast how evets unfolds over time (Kok et al., 2007). A transdisciplinary process was designed in which key stakeholders from Halmstad Municipality were engaged using surveys, interviews, focus group discussions, and workshops (for further details see André et al., 2022; Englund et al., 2022). Exploratory scenarios helped to overcome issues related to data confidentiality as stakeholders could discuss a hypothetical scenario instead of sharing sensitive information on infrastructures and their services. It also allowed adding information on social aspects of risk, normally not considered when developing an IC-based CRVA. In an online workshop, scenarios with key stakeholders were co-developed to forecast cascading effects in flooding events and their impacts on people. The exploratory scenario and the Impact Chain were used as boundary objects to help stakeholders understand the social dimension of risk. Using digital tools Miro and MentiMeter, stakeholders identified possible infrastructure disruption and mapped social groups at risk in case of a disruption. Following the workshop, we conducted online interviews with the same stakeholders to refine scenarios and identify additional vulnerable groups.
4.2.2.3. Conclusion
Exploratory scenarios were found to be a valuable addition to the IC-based CRVA framework. While scenario planning is already widely used to support climate risk decision-making (Brown et al., 2016; Star et al., 2016; Flynn et al., 2018), in our case study, it helped overcome challenges associated with limited data availability and measuring intangible risks. It supported stakeholders in considering low-frequency but high-impact risks where historical data is scarce. The exploratory scenario also led to various co-benefits, such as increased awareness, simplified complexity, and possibly also improved decision-making support. In line with previous research, the boundary object improved the quality of discussion and data (Star and Griesemer, 1989). Our case study indicates that the IC-based CRVA framework is flexible and adaptable to suit diverse stakeholder needs and objectives.
4.2.3. The Mannheim case B: implementing value chain CRVA for mobilizing industrial stakeholders
Related publication: (Lückerath et al., 2023).
4.2.3.1. Challenge/opportunity
Although the available guidelines to IC-based CRVA emphasize the importance of including relevant local stakeholders in the assessment process, specific guidance for addressing specific groups of local stakeholders is rare. For mobilizing industrial stakeholders, it is essential to understand how they manage operational risk. Typically, this is an integral part of their business continuity management or enterprise risk management. We concluded that results of any CRVA conducted for a participating corporate stakeholders need to be integrable with their risk management approach. One way to achieve this is to pinpoint climate risk factors to business units, activities, and processes, since these are the places in which operational risk management procedures are anchored in. An established model for describing these business elements is the Value Chain (Porter, 1985). Porter divided a company's activities into primary and supporting activities. Value Chain diagrams describe these activities in graphical form (Figure 5). The further division of activities into units, areas, and interlocking processes that take place in these areas and across areas offers opportunities for analysis and optimization, including risk assessment. Since Business Continuity Management (BCM) is typically process- or unit-oriented, the mapping of climate risk factors (sensitivities, capacities) and intermediate impacts (knock-on effects from direct physical impacts) to Value Chain C elements is suited to inform BCM managers on climate specific risk.
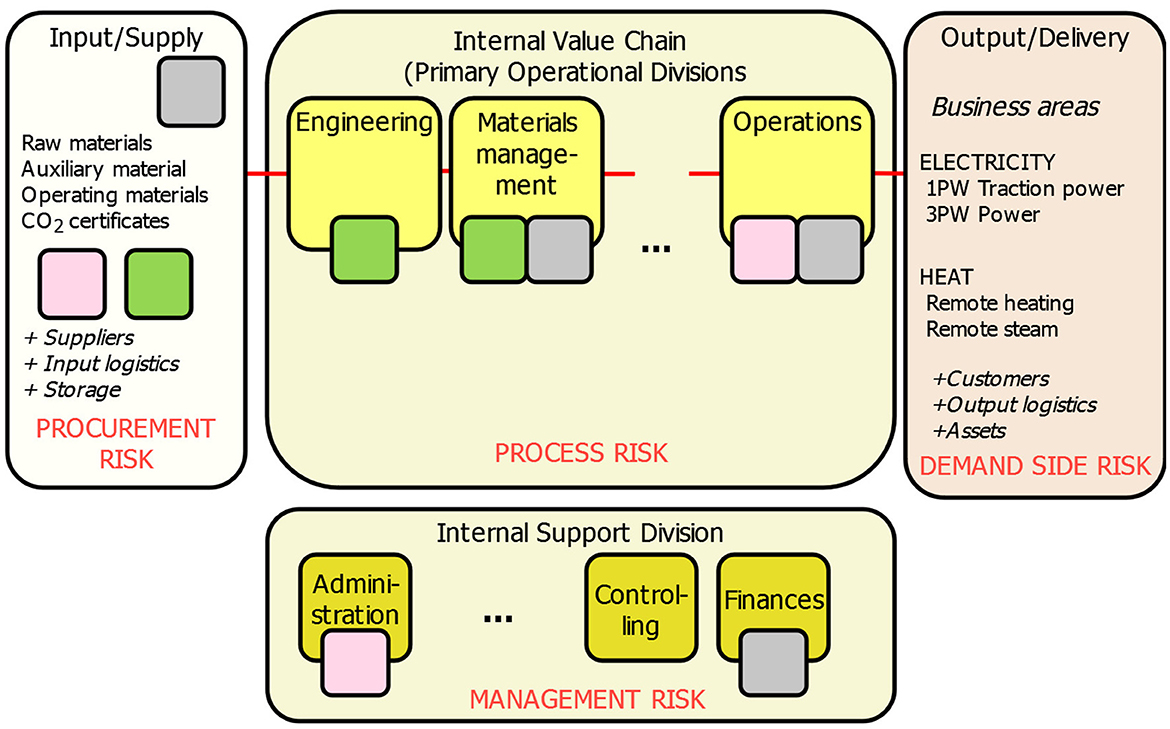
Figure 5. An energy producer's Value Chain, incorporating risk factors and intermediate impacts from an Impact Chain. Each unit, activity, or process is assigned a corresponding group of risk factors. Additionally, each element may have assigned capacities (green squares), sensitivities (pink squares), and intermediate impacts (gray squares). The complete enriched Value Chain is confidential. Adapted from Melo-Aguilar et al. (2022).
4.2.3.2. Methodological advancement
For implementing the Value Chain CRVA for a single business as the last phase of the Mannheim case study, we built upon a previously developed Impact Chain. We started by validating the relevant climate risk factors for the corporate stakeholder, the Mannheim Large Powerplant, ruling out factors that were only relevant for other participating stakeholders. We continued with eliciting the company's Value Chain, then pinpointing the remaining climate risk factors to the Value Chain elements and identifying suitable measurable indicators for each factor. Furthermore, we conducted a quantitative risk assessment focused on the economic effect of low waters of the Rhine River on costs of transporting freight, i.e., coal, via inland waterway.
4.2.3.3. Conclusion
We investigated just one indicator due to resource limitations. However, we produced a tangible, actionable decision support for one element of climate risk, which the stakeholders appreciated. Users of this approach should be aware that businesses may keep certain information and data private. In our case, we worked with disclosed surcharges instead of undisclosed total costs. Bringing these results into the Value Chain of businesses reduces entry barriers for the implementation of adaptation measures, as one could incentivize organizations to invest in climate change adaptation by bringing the topic into regular business continuity practice.
4.2.4. The Netherlands case: an IC-based CRVA on the asset level for financial risk portfolios
Related publication: (Attoh et al., 2022).
4.2.4.1. Challenge/opportunity
Climate change risks have become increasingly important for (large) financial investments in the real estate sector. Yet, meaningfully integrating climate risk information into investment portfolios remains difficult. To address this, the Netherlands case explored potential climate risks affecting two real estate assets in Utrecht and Rotterdam using IC-based CRVA. The case study aimed to assess and find effective ways to secure assets against flood risks by combining knowledge co-production and scientific information to support financial decisions.
4.2.4.2. Methodological advancement
To engage industry stakeholders and gather data for a climate risk assessment, the case utilized semi-structured interviews and a workshop. Real estate and finance companies, as well as consultancy companies, were involved as stakeholders and end-users of the final risk assessment. The knowledge co-production process was central to the assessment, and the aim was to advance the IC-based CRVA's user interface. The case study relied on open-access data collection and interactive maps showing current and future flood risks in the Netherlands for hazard and exposure data. However, data at the asset scale were not readily available in open-access databases, so close working partnerships with stakeholders from real estate companies were needed to obtain this data.
4.2.4.3. Conclusion
Feedback analysis of the process showed that knowledge co-production was well appreciated and the dialogue and interaction helped refine the companies' needs. Some information requested by the companies were impossible to meet with available climate knowledge, but through continuous engagement enabled by co-production, these expectations were addressed after explanations were given. However, knowledge co-production presents key challenges such as the subjectivity of some data collected that could impact the quality of the results. The process was laborious with a series of back and forth with stakeholders including workshops, interviews, meetings and phone calls. As a result, applying the IC-based CRVA framework became complex and time consuming.
4.3. Managing uncertainties
4.3.1. The Balearic Islands case: addressing uncertainty in IC-based CRVA using probability density functions
Related publication: (Agulles et al., 2022; Melo-Aguilar et al., 2022).
4.3.1.1. Challenge/opportunity
Uncertainties in data and the selection of weighting and aggregation processes make it difficult to confidently turn results into adaptation action. Incorporating qualitative indicators and knowledge gaps can also be challenging and may jeopardize the validity of the assessment. Even with extensive stakeholder and expert involvement, our understanding of the factors which influence climate risk remains imperfect (Booysen, 2002; Gall, 2015; Gawith et al., 2016). Thus, there is a need to account for uncertainty in the development of Impact Chains, that remains unaddressed by the current methodological framework which relies heavily on data that may not be available. Some initiatives include uncertainties management strategies in CRVA, such as the probabilistic impact risk model software CLIMADA (Aznar-Siguan and Bresch, 2019) or CAPRA (Cardona et al., 2012). However, although they are valuable tools that provide a way to consider uncertainty measures in different risk components, they are restricted to specific software that requires some level of expertise, thus, limiting its usability.
4.3.1.2. Methodological advancement
The Balearic Islands case developed a general formalism for integrating uncertainties into IC-based CRVA. The formalism uses probability density functions (PDFs) instead of scalar quantities for weights and indicator values. PDFs are propagated through the entire Impact Chain using a Monte-Carlo approach, resulting in a final risk PDF (Figure 6; Melo-Aguilar et al., 2022). The PDFs can be defined freely, but a Gaussian function is a common choice, where the amplitude of the PDF is determined by the assigned uncertainty. In the Balearic Islands case, indicator-related uncertainties were estimated from data (e.g., spread of climate projections for future temperatures) or the standard deviation of time series, while weight-associated uncertainties were established through an Analytical Hierarchy Process survey with stakeholders (Melo-Aguilar et al., 2022). The formalism can be implemented by developing the suitable computer codes (e.g., in Python or MATLAB) or using the UNTIC tool (untic.pythonanywhere.com) which can be used without much technical expertise.
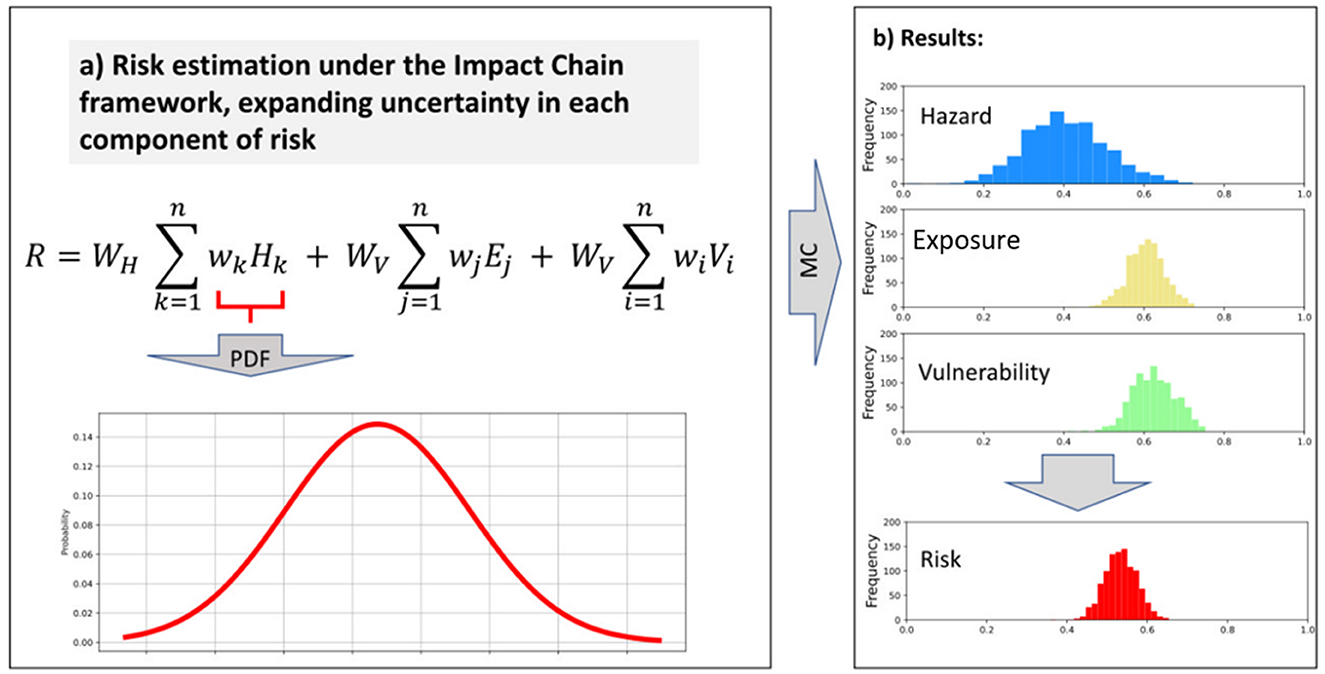
Figure 6. Schematic of the proposed methodology for integrating uncertainties into IC-based CRVA. (A) Risk aggregation formula. (B) Results of uncertainty expanding in each component of risk. Adapted from Melo-Aguilar et al. (2022).
4.3.1.3. Conclusion
Our proposed methodological advancement is robust and flexible, allowing to be integrated with different aggregation methods (for example the reverse geometric aggregation as applied in the Paris case) and a wide range of situations. It offers a new perspective on Impact Chains for integrating factors even when knowledge of their severity and role is limited. This allows to include all relevant factors and indicators without requiring data availability. This methodological advancement is valuable as current IC-based CRVA heavily relies on data, and when no data is available, indicators tend to be discarded (Kienberger et al., 2016), leading to biased results. Future work should focus on developing strategies to validate risk analysis, producing robust information that is as independent from subjective choices as possible. Calibrating the IC operationalization by computing the risk for past situations and comparing it to real-world impacts would increase the validity of the results.
4.4. Modeling socioeconomic scenarios
4.4.1. Challenge/opportunity
In IC-based CRVA, cause-effect relationships are often only analyzed qualitatively and not converted into numerical simulation models (Menk et al., 2022) due to the time and resource intensive process of numerical parameterization. Thus, to perform a more comprehensive quantitative assessment of climate risks, mitigation and adaptation strategies, it appears cost-effective to use existing simulation models. The IPCC relies on Biophysical climate models and Integrated Assessment Models (IAMs) to project future emission pathways and climate scenarios. However, traditional IAMs may not account for cross-sectoral interdependencies in detail. For detailed analyses of the socio-economic impacts of energy and climate policy scenarios, macro-economic simulation models are generally utilized (Pollitt and Mercure, 2018).5 Among the few assessments of transboundary macro-economic climate risks conducted to date, trade in agricultural commodities received relative frequent attention. However, these assessments often do not model future socio-economic developments, which will substantially affect exposure and vulnerability levels. As a result, there is a need for further advancements in this area to better account for the role of socio-economic factors in transboundary climate risks risk assessments.
In UNCHAIN, two case studies used macro-econometric models to assess the effects of climate change on different sectors and under different socio-economic scenarios. However, the cases differed in scope and modeling approach. One focuses on the impacts of transboundary climate risks in Germany [it is therefore also a contribution to the last innovation area “Examining transboundary climate risks” (TCR)], while the other focused on the monetary costs of climate impacts on specific sectors in Germany.
4.4.2. The Germany cases (transboundary and national): dynamic modeling of cross-sectoral (socio-) economic interlinkages
4.4.2.1. Methodological advancement (transboundary)
The transboundary Germany case uses a global multi-regional input-output model (MRIO), called GINFORS-E (GWS, 2022a) to assess the impacts of transboundary climate risks on agricultural commodity markets. The model combines detailed agricultural information with integrated MRIO-based modeling of global economic developments, mapping the multinational interplay of demand, trade, and production for 20 crop commodities and eight livestock products.
4.4.2.2. Conclusion (transboundary)
The case study demonstrates that using a global and regional economic modeling approach can provide a dynamic assessment of the indirect economic impacts of climate change, including trans-regional impacts, and is thus valuable for evaluating TCRs in agriculture commodities. However, there is still room for further development of MRIO models to fully utilize the available information and involve stakeholders at all stages of the participatory Impact Chain development process. In the UNCHAIN project, this potential was not fully realized, highlighting the need for continued research in this area. The main advantage of this approach is its ability to project self-contained and consistent multi-national socio-economic developments over time, allowing for the assessment of the impacts of climate risks on trade and production in different regions.
4.4.2.3. Methodological advancement (national)
In contrast, the national Germany case assesses the future monetary costs of domestic climate impacts in Germany under different socio-economic scenarios (a trend scenario, a sustainable scenario, and a dynamic scenario), by applying the national macro-econometric model PANA RHEI (GWS, 2022b). The model simulates the impacts of climate change on transportation, health care, and electricity sectors and the effects of possible adaptation measures. The results are presented using socio-economic indicators such as the gross domestic product, employment, or production, making them comparable so that they function as decision supporting information.
4.4.2.4. Conclusion (national)
The main methodological challenges arose from quantifying effects of adaptation measures. Additionally, since the direct macroeconomic effects of climate impacts depend on many factors, it remains challenging to quantify the resulting monetary costs, especially for medium to long-term projections. As a result, more research is needed to strengthen the evidence base for individual effects of future adaptation measures on increasing resilience and avoiding direct economic. So far, no quantified national reference values have been established for (socio-) economic scenario analyses at the sector level. The more detailed representations of economic developments in macroeconomic models compared to most IAMs can be used to derive corresponding projections. Hence, it would be promising to obtain further detailed macroeconomic assessment results through complementary modeling studies of climate impacts, adaptation costs, and benefits for focal sectors in a joint project.
4.4.2.5. Overall conclusion
From a methodological perspective, both applications highlight similar challenges. Furthermore, as the estimated macroeconomic costs of climate impacts and adaptation measures vary significantly across individual socioeconomic scenarios, the case studies illustrate the need for dynamic risk assessments. Compared to earlier approaches that projected future adaptation actions and implied adaptation costs by applying traditional IAMs (see, for example, Agrawala et al., 2011), this approach differs methodologically in that it does not rely on any optimization assumptions. This implies that the modeling approach is not rooted in a highly abstract theoretical framework (like the “Ramsey optimal growth framework” applied by both models compared by Agrawala et al., 2011) as it does not intend to deduce any normative conclusions about “optimal” mitigation and adaptation measures. Instead, a positive analytical approach is followed by this approach as well as the by the MRIO Model GINFORS-E featured for transboundary climate risk assessments: Both models project the future evolution of inter-sectoral economic impact relationships from historical empirical observations under alternative socio-economic scenarios. Given these pathways projections, researchers as well as involved stakeholders can then examine in detail how different direct climate impacts and adaptation measures in individual sectors and/or world regions affect other sectors, and/or world regions economically.
4.5. Examining transboundary climate risks
4.5.1. Addressing transboundary climate risks in Norway
4.5.1.1. Challenge/opportunity
Impacts of climate change are not confined or experienced only at a national and local level. According to the Intergovernmental Panel on Climate Changes' (IPCC) Sixth Assessment Report (AR6) Working Group III it is a problem that affects all parts of economy and society and requires actions across sectors and scales (13-16f; IPCC, 2022b). As in most European countries, Norwegian municipalities have largely been given responsibility for climate adaptation and focus so far has been mostly on adaptation to local climatic changes. However, a recent trend is shifting transboundary risks into focus, asking how Norway may be impacted by climate impacts elsewhere on the planet through trade, finance, people (tourism, health, and migration) and through biophysical processes. Therefore, it is important to have information about the patterns and magnitudes of climate risks in and between different regions and sectors, and methodological frameworks for the assessment of climate-related interactions between economic sectors and world regions have been promoted recently.6 Currently, most assessments of transboundary climate risks are conducted at the global or regional (cross-country), and to sometimes at the national level. However, there is a lack of information about how these risks affect the sub-national level of governance (Harris et al., 2022).
In UNCHAIN, two case studies addressed transboundary risks for Norway through stakeholder integration in participatory workshops.
4.5.2. The Klepp case: challenges and opportunities at the sub-national level
4.5.2.1. Methodological advancement
The IC-based CRVA approach was employed to identify transboundary climate risks that are pertinent to food production in Norway, through consultation with key stakeholders in Klepp Municipality. By breaking down the climate risks into nodes and links, this method seemed to be well-suited for analyzing TCRs.
4.5.2.2. Conclusion
The IC-based CRVA framework was useful for communicating the concept of transboundary climate risks to local stakeholders, showing the differences, similarities, and possible interactions between traditional “local” risks and new “transboundary” risks. However, producing numerical indicators and indexes was too complicated and time-consuming for the case's scope, making it challenging to produce actionable knowledge for addressing transboundary risks at the local governance level. Focus from practitioners on TCRs is correlated to specific governance measures (i.e., requirements), a focus often lacking as policy mostly has focused on carbon footprint and “local” climate risks and the strengthening of resilience and adaptive capacity to such.
Practitioners tended to focus on local climate risks and building resilience and adaptive capacity to those risks, rather than on transboundary risks. However, it is important to link work and analyses on local and transboundary risks to avoid potential conflicts and unintended consequences. Local climate risks may be reduced at the expense of increasing transboundary risks, which was presented to local stakeholders. They responded that other means of adapting to local climate risks need to be investigated, which is also discussed in a recent report from the Environmental Protection Agency of Norway on climate change and food security (Bardalen et al., 2022).
4.5.3. The Nordland case: transboundary climate risks and the need for improved risk assessment and governance - insights from a salmon farming industry workshop
4.5.3.1. Methodological advancement
A workshop was arranged to identify risks associated with salmon farming, and to develop Impact Chains that would span the inputs, production and markets involved. The participants were farmers who belonged to an industry cluster and who were responsible for addressing environmental issues in their respective companies. None of the companies had a specific climate change risk assessment plan developed yet. The identified risks ranged from environmental degradation, such as the potential collapse of soy production in Brazil, to geopolitical concerns that may or may not be triggered by climate change, and from increases in freight prices to a collapse in local salmon markets.
4.5.3.2. Conclusion
During the workshop the participants discussed and acknowledged the complexity and multiplicity of issues related to risk identification, and they recognized the need for clear risk ownership as the basis for decision-making and for tailoring measures to strengthen adaptability. The participants also recognized that timing is crucial when investing in improved adaptive capacity, as premature investments could create new risks. Therefore, there is a clear need for assessing the identified risk(s) and establishing risk ownership and regulatory guidance. These discussions highlighted the potential for improving risk assessment practices within individual producer organizations.
The application of an IC-based CRVA was found to be useful in discussing these complex issues. This approach facilitated a high level of co-production and allowed for a focus on the interconnections between different risks, across scales, geographical boundaries, and sectors. The overall experience suggests that a mixed-methods approach with knowledge co-production, literature analysis, media analysis, and assessment of industry strategies on environmental issues, is the most effective. The workshop revealed a certain sensitivity and awareness of the interconnectedness of climate change challenges across borders, which is often overlooked in more nationally-focused climate policy discussions. However, when it comes to identifying actionable steps, the industry stakeholders tended to focus on areas that are already prioritized, such as specific policy measures, obligations, and challenges. This underscores the importance of governance risk ownership in shaping decision-making, as confirmed by these co-production and workshop settings.
5. Discussion
We have addressed the needs and research gaps identified by the EEA regarding CRVA in five key innovation areas with methods to improve the existing IC-based CRVA framework, proactive stakeholder involvement, systematic assessments of uncertainty, and exploration of non-climatic factors that influence exposure and vulnerability. Additionally, we emphasized the importance of paying attention to cross-sectoral interactions and transboundary impacts.
5.1. Innovation area “elaborating the IC-based CRVA framework”
This innovation area is unique in the sense that it encompasses multiple methodological advancements that did not fit into any other category and that it closely aligns with the original IC-based CRVA framework. While these methods are not entirely groundbreaking, they serve as valuable additions to the original framework. Combining the framework with methodological additions is something which is already suggested in the Vulnerability Sourcebook.
We identified three key messages from this innovation area:
• Incorporating feedback loops via Causal Loop Diagrams (Salzburg case) better accounts for the dynamic and interwoven nature of climate risk. CLDs enable identification of feedback loops in a wider risk driver system, allowing for a shift in focus to important factors and cascading effects, and identifying mid- to long-term adaptation measures. However, CLDs require a better understanding of the system, or else uncertainties may be exacerbated.
• Using national Impact Chains to develop local Impact Chains (Mannheim case) can ensure consistency between different scales and save time in developing Impact Chains. Optimizing Impact Chain development leaves more resources to discuss and identify entry points for adaptation measures.
• Different aggregation methods can modify the sensitivity of aggregated indicators to extreme values (Paris case).
5.2. Innovation area “improving stakeholder engagement”
Improving stakeholder engagement is a promising approach for the IC-based CRVA framework, but it can be time and resource intensive. This approach offers co-benefits beyond the co-production of knowledge, including increased awareness and perceived saliency of results, as demonstrated in other studies (André et al., forthcoming; Bremer et al., 2019; Cvitanovic et al., 2019).
The MCI-TRIZ Inventive Design Method provides a structured approach for problem definition and consensus-oriented identification of adaptation measures (Upper Rhine case). By modeling differences and commonalities of viewpoints of participating stakeholders, this method helps to “objectify” problem- and solution definition.
In the Halmstad case, restructuring the risk-oriented Impact Chains can help identify social vulnerabilities and trends in climate change impacts. This enables awareness raising for future social inequalities and identifying consensus-based adaptation measures. The method helps understand which social groups already are or will be bearing the brunt of climate change impacts and which groups might be able to shoulder a larger share of adaptation measures.
IC-based CRVA is readily applicable in the Mannheim and Netherland cases. IC-based CRVA can be integrated with business Value Chains, and by assessing future climate risks for real estate assets, the IC-based CRVA workflow can inform financial investment portfolios.
5.3. Innovation area “managing uncertainty”
Our findings suggest that incorporating a probabilistic framework, such as probability density functions (PDFs), can account for missing or uncertain information in IC-based CRVAs (Balearic Islands case). Moreover, macro-econometric models can address uncertainties in future socio-economic impacts. While the proposed PDF formalism is compatible with various indicator aggregation approaches, we note that results from different aggregation methods (e.g., arithmetic, geometric, or reversed geometric) can differ significantly, as demonstrated in the Paris case. Therefore, it is crucial to test the sensitivity of the chosen methods, and the UNTIC web-tool offers a straightforward way to conduct sensitivity analysis. However, while Agulles et al. (2022) and Melo-Aguilar et al. (2022) have proposed retroanalyses to partially validate results, validation in IC-based CRVA remains underexplored. Developing more advanced validation strategies, even in the absence of comprehensive knowledge and data, could increase the general credibility of IC-based CRVAs and should be further explored.
5.4. Innovation area “modeling socio-economic scenarios”
An important enhancement for IC-based CRVA is to estimate the future monetary costs of climate impacts under different socioeconomic scenarios using macro-econometric models such as PANTA RHEI (national Germany case) and GINFORS-E (transboundary Germany case). These models provide a more detailed analysis of socioeconomic impacts and developments, which can result in better quantitative assessments of uncertainty for decision-making. Such simulation models are already widely used in ex-ante assessments of policy measures and strategies.
It is worth noting that traditional IC-quantification approaches can involve uncertain and somewhat arbitrary indicator selection, weighting, and aggregation procedures, even with extensive stakeholder and expert involvement. Therefore, relying on established modeling approaches for ex-ante assessments of future exposure levels and relevant economic drivers is a logical choice. To further refine these models, detailed inputs for focal sectors can be derived from complementary biophysical modeling studies on climate impacts and adaptation measures, and co-production approaches can be integrated into the proposed probabilistic framework to enhance the accuracy of the model's results.
5.5. Innovation area “examining transboundary climate risks”
We found that while it is conceptually easy to integrate transboundary climate risk into IC-based CRVAs, assessing this type of risk in practice poses significant challenges (as observed in both Norway cases). Although incorporating transboundary risk in IC-based CRVAs can raise awareness of risk origins and impacts, we encountered difficulties in assessing such risks due to unresolved issues of risk ownership and limited access to remote data. Addressing these challenges requires further research and international collaboration.
In terms of using IC-based CRVAs to assess transboundary risks, we found that while the approach can stimulate awareness and prompt discussions about risk ownership, the operational modules can be complex due to the intricate interplay of risk factors. However, the transboundary Germany case showed that existing dynamic macro-econometric MRIO models can be applied to assess transboundary risks related to trade in agricultural products. These models offer a promising starting point for integrating insights into the multi-national economic feedback effects that are critical to understanding transboundary risks.
5.6. Implications
Although the methodological advancements presented in this study were applied in separate cases, we believe that they are generalizable and applicable in other contexts. To account for this, we developed validation criteria to ensure that our findings contribute to the development of standardized CRVA frameworks that produce comparable results across different contexts and policy scales, while remaining adaptable to changing circumstances. We have shown that our contributions are relevant for policymakers, practitioners, and other stakeholders involved in developing adaptation plans. By improving policy decisions and supporting sustainable development, our study can help to assess risks for companies, as required by the European Union taxonomy (European Union, 2021). Overall, our work provides practical guidance and insights for a wide range of stakeholders involved in climate risk assessment and management. With standardized and validated CRVA frameworks, we can facilitate better decision-making and help build resilience to climate change impacts.
5.7. Limitations
One limitation of our study is that the cases were not initially designed to align perfectly with each other, as the research team consisted of individuals from diverse backgrounds who needed time to develop a shared language and collaborate effectively. Coordinating a large project with many researchers from different domains takes significant time and effort, which should be budgeted for accordingly. In retrospect, we suggest planning future case studies with greater harmonization and a clearer plan for validating the best methodological advancements in a scientific and objective manner. Although we took measures to address this issue by establishing a research pipeline and developing validation criteria at the beginning of the project phase, we ultimately had to rely on some subjective judgment.
Furthermore, although the methodological advancements worked well in their respective case studies, there may be additional advancements that were not explored in UNCHAIN. Finally, because the innovations were tested in separate case studies, a joint application of the advancements should be tested and validated in future research.
6. Conclusion
Overall, the methodological advancements discussed here have made significant contributions to the field of CRVA. All eleven cases revealed at least one key advancement based on validation criteria and practical experiences gained during the studies. Our state-of-the-art analysis has also helped us identify and partially address various challenges and opportunities, which will enable the provision of tangible methodological advice in the forthcoming new edition of the Vulnerability Sourcebook and for practitioners in the field.
Although not all modules were implemented in all case studies due to resource constraints or lack of data, the modular structure of the framework allowed for barrier-free testing and integration of methodological advancements from different scientific disciplines. In the future, IC-based CRVA could be seen as a toolbox of methods and techniques that can be chosen according to the intended purpose, rather than a rigid workflow to be applied from start to finish. While the core method remains stable, there may be variations in specific recommendations for certain stakeholder groups, as well as variations in how it can be integrated with other risk management methods or specialized decision support tools.
It is worth noting that while the discussed methodological innovations have been effective in the cases they were applied in, there may be additional advancements that have yet to be explored. For example, the methods were tested in separate case studies, so a joint application of the advancements would need to be tested and validated. Furthermore, while IC-based CRVAs can be applied in different contexts, they must be adapted to take into account the different levels of competence and relevant solutions needed for each specific case.
Data availability statement
The original contributions presented in the study are included in the article/Supplementary material, further inquiries can be directed to the corresponding author.
Author contributions
Conceptualization: LP, ER, CA, and ÅG. Data curation: LP. Investigation: LP, JG, FR, DL, KM, ER, CA, ÅG, KA, ME, BD, MB, KR, AC, EA, MM, SR, GJ, SK, MA, and CM-A. Methodology and supervision: SK, ÅG, ER, and CA. Funding acquisition: CA, GJ, SK, and MM. Project coordination: CA, AC, and SK. Visualization: LP, ER, and ÅG. Writing–original draft: LP, JG, FR, DL, KM, ER, CA, ÅG, KA, ME, BD, MB, KR, AC, EA, MM, GJ, MA, and CM-A. Writing–review and editing: LP, JG, FR, DL, KM, ER, CA, ÅG, KA, ME, BD, MB, KR, AC, EA, MM, SR, GJ, MA, SK, and MZ. All authors contributed to the article and approved the submitted version.
Funding
UNCHAIN: Unpacking Climate Impact Chains was funded through the EU funding mechanisms Joint Programming Initiative (JPI) and Assessment of Cross (X)-sectoral climate impacts and pathways for Sustainable transformation (AXIS), with support from the EU (Grant Number: 776608). All partners are granted financial support through their national funding agency: FFG (AT/IT, reference 872000), FORMAS (SE), DLR/BMBF (DE, grant number FKZ 01LS1908B), AEI (ES), NOW (NL), ANR (FR, grant number ANR-19-AXIS-0002-01), and RCN (NO) with co-funding from H2020. The lead author was additionally supported by the Austrian Federal Ministry for Digital and Economic Affairs, the National Foundation for Research, Technology and Development, the Christian Doppler Research Association, and Médecins Sans Frontières (MSF) Austria. Moreover, the authors are also acknowledging the support by “HydroHazards - Managing vulnerabilities to multiple water hazards in Sweden”, funded by the Swedish Civil Contingency Agency (MSB 2019-0651) and FORMAS.
Conflict of interest
The authors declare that the research was conducted in the absence of any commercial or financial relationships that could be construed as a potential conflict of interest.
Publisher's note
All claims expressed in this article are solely those of the authors and do not necessarily represent those of their affiliated organizations, or those of the publisher, the editors and the reviewers. Any product that may be evaluated in this article, or claim that may be made by its manufacturer, is not guaranteed or endorsed by the publisher.
Supplementary material
The Supplementary Material for this article can be found online at: https://www.frontiersin.org/articles/10.3389/fclim.2023.1095631/full#supplementary-material
Footnotes
1. ^Trends point toward inclusion of stakeholder and expert knowledge in all assessment phases, rendering all modules essentially participatory (Zebisch et al., 2021). For readability and clarity reasons we, however, continue to distinguish between participatory and operational modules.
2. ^The original Vulnerability Sourcebook presented a different conceptualization. The goal of the Risk supplement was to align with the updated concepts found in the IPCC AR5 report (Field, 2014; Huq et al., 2014), which represents a shift from the concepts in the previous IPCC AR4 report (Parry et al., 2007). All case studies presented in the supplement adhere to the IPCC AR5 conceptual framework.
3. ^Nine of the eleven cases are being published in the special issue “New Approaches to Local Climate Change Risk Analysis” in Frontiers in Climate.
4. ^Except for the Mannheim case, which is featured twice.
5. ^Macroeconomic simulation models may be basically categorized into Computable General Equilibrium models and Macro-econometric models. For further methodological annotations in this regard and an introduction to policy-relevant differences between both modelling approaches we refer to (Scrieciu et al., 2013).
6. ^See, for example, the IPCC AR6 Working Group III's recognition of current global Multi-Region Input-Output models (Owen, 2017; Wiedmann and Lenzen, 2018) as a major area of advance since AR5 in terms of the availability of valid data and consistent methods for global footprint calculation approaches.
References
Aall, C., and Groven, K. (2022). The unpredictable truth: a proposed road map for a reflect-then-act approach to climate uncertainties and lessons learned from norwegian municipalities. Weather Clim. Soc. 14, 337–347. doi: 10.1175/WCAS-D-21-0078.1
Agrawala, S., Bosello, F., Carraro, C., De Cian, E., and Lanzi, E. (2011). Adapting to climate change: costs, benefits, and modelling approaches. Int. Rev. Environ. Resour. Econ. 5, 245–284. doi: 10.1561/101.00000043
Agulles, M., Melo-Aguilar, C., and Jorda, G. (2022). Risk of loss of tourism attractiveness in the western mediterranean under climate change. Front. Clim. 4:1019892. doi: 10.3389/fclim.2022.1019892
André, K., Englund, M., and Gerger Swartling, A. (2022). Testing the Impact Chain Model: Exploring Social Vulnerability to Multiple Hazards and Cascading Effects. doi: 10.51414/sei2022.041
André, K., Gerger Swartling, A., Englund, M., Menk, L., Mawuli Nii, Armah Nyadzi Attoh, E., and Milde, K. (forthcoming). Improving stakeholder engagement in climate change risk assessments: insights from six co-production initiatives in Europe. Front. Clim.
Attoh, E. M. N. A. N., de Bruin, K., Goosen, H., van Veldhoven, F., and Ludwig, F. (2022). “Making Physical Climate Risk Assessments Relevant to the Financial Sector–Lessons Learned from Real Estate Cases in the Netherlands.” Climate Risk Management 37:100447. doi: 10.1016/j.crm.2022.100447
Aznar-Siguan, G., and Bresch, D. N. (2019). CLIMADA v1: a global weather and climate risk < ? Xmltex\break?> assessment platform. Geosci. Model Dev. 12, 3085–3097. doi: 10.5194/gmd-12-3085-2019
Bardalen, A., Pettersen, I., Dumbu, S. V., Rosnes, O., Mittenzwei, K., and Skulstad, A. (2022). Climate Change Challenges the Norwegian Food System. Knowledge Base for Assessing Climate Risk in Value Chains with the Food System as a Case. NIBIO report 110/2022. Ås: Norsk institut for bioøkonomi (NIBIO). Available online at: https://www.miljodirektoratet.no/publikasjoner/2022/august/klimaendring-utfordrer-det-norske-matsystemet-kunnskapsgrunnlag-for-vurdering-av-klimarisiko-i-verdikjeder-med-matsystemet-som-case/
Barquet, K., Vieira Passos, M., André, K., Segnestam, L., Englund, M., Inga, K., et al. (2022). Assessing Cascading Effects from Multiple Hazards: An Example from Sweden. doi: 10.51414/sei2022.042
Bergh, D. D., Boyd, B. K., Byron, K., Gove, S., and Ketchen, D. J. (2022). What Constitutes a Methodological Contribution? Journal of Management. Vol. 48. Los Angeles, CA: SAGE Publications Sage CA. doi: 10.1177/01492063221088235
Bharwani, S., Klein, R. J. T., and Davis, M. (2013). PROVIA Guidance on Assessing Vulnerability, Impacts and Adaptation to Climate Change.
Birkmann, J. (2013). Measuring Vulnerability to Natural Hazards: Towards Disaster Resilient Societies Second Edition. United Nations University Press. Available online at: https://unu.edu/publications/books/measuring-vulnerability-to-natural-hazards-towards-disaster-resilient-societies-second-edition.html#overview.
Booysen, F. (2002). An overview and evaluation of composite indices of development. Soc. Indic. Res. 59, 115–151. doi: 10.1023/A:1016275505152
Bremer, S., Wardekker, A., Dessai, S., Sobolowski, S., Slaattelid, R., and van der Sluijs, J. (2019). Toward a multi-faceted conception of co-production of climate services. Clim. Serv. 13, 42–50. doi: 10.1016/j.cliser.2019.01.003
Brown, I., Martin-Ortega, J., Waylen, K., and Blackstock, K. (2016). Participatory scenario planning for developing innovation in community adaptation responses: three contrasting examples from Latin America. Reg. Environ. Change 16, 1685–1700. doi: 10.1007/s10113-015-0898-7
Bundesregierung (2022). Deutsche Strategie Zur Stärkung Der Resilienz Gegenüber Katastrophen – Umsetzung Des Sendai Rahmenwerks Für Katastrophenvorsorge (2015–2030) – Der Beitrag Deutschlands 2022–2030. Berlin, Germany: Bundesministerium des Inneren und für Heimat.
Cardona, O. D., Ordaz, M., Reinoso, E., Yamín, L. E., and Barbat, A. H. (2012). “CAPRA–comprehensive approach to probabilistic risk assessment: international initiative for risk management effectiveness,” in Proceedings of the 15th World Conference on Earthquake Engineering. Vol. 1.
Cheek, J., Onslow, M., and Cream, A. (2004). Beyond the divide: comparing and contrasting aspects of qualitative and quantitative research approaches. Adv. Speech Lang. Pathol. 6, 147–152. doi: 10.1080/14417040412331282995
Coulibaly, A., Rudolf, F., Ory, M., Cavallucci, D., Bastian, L., and Gobert, J. (2022). “Open Inventive Design Method (OIDM-Triz) Approach for the Modeling of Complex Systems and the Resolution of Multidisciplinary Contradictions,” in Application to the Exploration of Innovative Solutions to Deal with the Climate Change Impacts.” Springer Computer Science Proceedings. doi: 10.1007/978-3-031-17288-5_11
Cvitanovic, C., Howden, M., Colvin, R. M., Norström, A., Meadow, A. M., and Addison, P. F. E. (2019). Maximising the benefits of participatory climate adaptation research by understanding and managing the associated challenges and risks. Environ. Sci. Policy 94, 20–31. doi: 10.1016/j.envsci.2018.12.028
Dannevig, H., and Aall, C. (2015). The regional level as boundary organization? an analysis of climate change adaptation governance in Norway. Environ. Sci. Policy 54, 168–175. doi: 10.1016/j.envsci.2015.07.001
Daze, A., Ambrose, K., and Ehrhart, C. (2009). “Climate Vulnerability and Capacity Analysis Handbook.” Care International.
d'Oleire Oltmanns, S., Riedler, B., Pernkopf, L., Weinke, E., and Lang, S. (2015). Validation Strategy for User-Specific Map Products for the European Copernicus Security Service. Journal for Geographic Information Science 1. Available online at: https://pdfs.semanticscholar.org/bf28/ad0a0aea50f288c2312d40e2b4f14ceacc4e.pdf
Englund, M., André, K., Barquet, K., and Segnestam, L. (2022). “Weather, Wealth and Well-Being: Cascading Effects of Water-Related Hazards and Social Vulnerability in Halmstad, Sweden.” SEI Discussion Brief. Stockholm Environment Institute. doi: 10.51414/sei2022.038
Englund, M., Passos, M. V., André, K., Swartling, Å. G., Swgnestam, L., and Barquet, K. (2023). Constructing a social vulnerability index for flooding: insights from a municipality in Sweden. Front. Clim. 5, 39. doi: 10.3389/fclim.2023.1038883
European Commission (2019). “European Commission COM(2019) 640 Final. Brussels, Belgium: The European Green Deal.
European Commission (2020). Directive of the European Parliament and of the Council - On the Resilience of Critical Entities. Brussels, Belgium. Available online at: https://eur-lex.europa.eu/legal-content/EN/TXT/?uri=CELEX%3A52020PC0829
European Commission (2021). Forging a Climate-Resilient Europe - the New EU Strategy on Adaptation to Climate Change. Brussels. Available online at: https://eur-lex.europa.eu/legal-content/EN/TXT/PDF/?uri=CELEX:52021DC0082andfrom=EN
European Union (2021). Commission Delegated Regulation (EU) 2021/2178. Available online at: https://eur-lex.europa.eu/legal-content/EN/TXT/PDF/?uri=CELEX:32021R2178
Field, C. B. (2014). Climate Change 2014–Impacts, Adaptation and Vulnerability: Regional Aspects: Cambridge University Press.
Flynn, M., Ford, J. D., Pearce, T., Harper, S. L., and I. H. A. C. C. Research Team (2018). Participatory scenario planning and climate change impacts, adaptation and vulnerability research in the arctic. Environ. Sci. Policy 79, 45–53. doi: 10.1016/j.envsci.2017.10.012
Fritzsche, K., Schneiderbauer, S., Bubeck, P., Kienberger, S., Buth, M., Zebisch, M., et al. (2014). The Vulnerability Sourcebook: Concept and Guidelines for Standardised Vulnerability Assessments.
Füssel, H. M., Capela Lourenço, T., Downing, C., Hildén, M., Leitner, M., Marx, A., et al. (2018). National Climate Change Vulnerability and Risk Assessments in Europe, 2018.
Gall, M. (2015). the suitability of disaster loss databases to measure loss and damage from climate change. Int. J. Glob. Warm. 8, 170–190. doi: 10.1504/IJGW.2015.071966
Gawith, D., Daigneault, A., and Brown, P. (2016). Does community resilience mitigate loss and damage from climaterelated disasters? evidence based on survey data. J. Environ. Plann. Manag. 59, 2102–2123. doi: 10.1080/09640568.2015.1126241
GIZ (2014). Vulnerability Sourcebook Annex. Available online at: https://www.adaptationcommunity.net/publications/vulnerability-sourcebook-annex/
GIZ and Eurac (2017). Risk Supplement to the Vulnerability Sourcebook. Guidance on How to Apply the Vulnerability Sourcebook's Approach with the New IPCC AR5 Concept of Climate Risk. Giz Bonn, Germany.
GIZ, Eurac, and United Nations University. (2018). Climate Risk Assessment for Ecosystem-Based Adaptation–A Guidebook for Planners and Practitioners. Deutsche Gesellschaft für Internationale Zusammenarbeit (GIZ) GmbH. Available online at: https://bia.unibz.it/esploro/outputs/report/Climate-Risk-Assessment-for-Ecosystem-based-Adaptation/991006391295601241
Gobert, J., and Rudolf, F. (2022). Rhine low water crisis: from individual adaptation possibilities to strategical pathways 2. Front. Clim. 4, 241. doi: 10.3389/fclim.2022.1045466
Graham, A., and Mitchell, C. L. (2016). The role of boundary organizations in climate change adaptation from the perspective of municipal practitioners. Clim. Change 139, 381–395. doi: 10.1007/s10584-016-1799-6
Greiving, S., Zebisch, M., Schneiderbauer, S., Fleischhauer, M., Lindner, C., Lückenkötter, J., et al. (2015). A consensus based vulnerability assessment to climate change in germany. Int. J. Clim. Change Strat. Manage. 7, 306–326. doi: 10.1108/IJCCSM-11-2013-0124
Guillaumont, P. (2009). “Caught in a Trap.” Identifying the Least Developed Countries. Clermont-Ferrand: FERDI.
Gusfield, J. R. (1989). Constructing the ownership of social problems: fun and profit in the welfare state. Soc. Probl. 36, 431–441. doi: 10.2307/3096810
GWS (2022a). The Institute Of Economic Structures Research. “GINFORS-E.” Available online at: https://www.gws-os.com/en/energy-and-climate/models/detail/ginfors-e
GWS (2022b). “PANTA RHEI.” Available online at: https://www.gws-os.com/en/energy-and-climate/models/detail/panta-rhei
Hansson, S., and Polk, M. (2018). Assessing the impact of transdisciplinary research: the usefulness of relevance, credibility, and legitimacy for understanding the link between process and impact. Res. Eval. 27, 132–144. doi: 10.1093/reseval/rvy004
Harris, K., Lager, F., Jansen, M. K., and Benzie, M. (2022). Rising to a new challenge: A protocol for case-study research on transboundary climate risk. Weather Clim. Soc. 14, 755–768. doi: 10.1175/WCAS-D-21-0022.1
Hoppe, R., and Wesselink, A. (2014). Comparing the role of boundary organizations in the governance of climate change in three EU member states. Environ. Sci. Policy 44, 73–85. doi: 10.1016/j.envsci.2014.07.002
Hudson, P., Botzen, W., Poussin, J., and Aerts, J. C. H. J. (2019). Impacts of flooding and flood preparedness on subjective well-being: A monetisation of the tangible and intangible impacts. J. Happiness Stud. 20, 665–682. doi: 10.1007/s10902-017-9916-4
Huq, I. R. S., Anokhin, Y. A., Carmin, J., Goudou, D., Lansigan, F. P., Osman-Elasha, B., et al. (2014). Adaptation needs and options. Structure 14, 833–868. doi: 10.1017/CBO9781107415379.019
IPCC (2022a). Annex II: Glossary. Climate Change 2022: Impacts, Adaptation and Vulnerability. Available online at: https://www.ipcc.ch/report/ar6/wg2/downloads/report/IPCC_AR6_WGII_Annex-II.pdf
IPCC (2022b). Climate Change 2022: Mitigation of Climate Change. Contribution of Working Group III to the Sixth Assessment Report of the Intergovernmental Panel on Climate Change. Cambride, UK and New York, NY, USA: Cambride University Press.
ISO 14090 (2019). ISO 14090:2019 Adaptation to Climate Change - Principles, Requirements and Guidelines. ISO, issued June 2019.
Kabisch, S., Chakrabarti, R., Wolf, T., Kiewitt, W., Gorman, T., Chaturvedi, A., et al. (2014). Climate change impact chains in the water sector: observations from projects on the East India coast. J. Water Clim. Chang. 5, 216–232. doi: 10.2166/wcc.2013.118
Kahlenborn, W., Porst, L., Voss, M., Fritsch, U., Renner, K., Zebisch, M., et al. (2021). “Climate Impact and Risk Assessment 2021 for Germany. Summary.” Umweltbundesamt (UBA).
Kienberger, S., Borderon, M., Bollin, C., and Jell, B. (2016). Climate change vulnerability assessment in mauritania: reflections on data quality, spatial scales, aggregation and visualizations. J. Geogr. Inf. Sci. 4, 167–175. doi: 10.1553/giscience2016_01_s167
Kirchhoff, C. J., Lemos, M. C., and Dessai, S. (2013). Actionable knowledge for environmental decision making: broadening the usability of climate science. Ann. Rev. Environ. Resourc. 38, 393–414. doi: 10.1146/annurev-environ-022112-112828
Klein, R. J. T., and Juhola, S. (2014). A framework for nordic actor-oriented climate adaptation research. Environ. Sci. Policy 40, 101–115. doi: 10.1016/j.envsci.2014.01.011
Kok, K., Biggs, R., and Zurek, M. (2007). Methods for developing multiscale participatory scenarios: insights from Southern Africa and Europe. Ecol. Soc. 12, 1–16. doi: 10.5751/ES-01971-120108
Krejčí, J., and Stoklasa, J. (2018). Aggregation in the analytic hierarchy process: why weighted geometric mean should be used instead of weighted arithmetic mean. Expert Syst. Appl. 114, 97–106. doi: 10.1016/j.eswa.2018.06.060
Larsen, R. K., Gerger Swartling, A., Powell, N., May, B., Plummer, R., Simonsson, L., et al. (2012). A framework for facilitating dialogue between policy planners and local climate change adaptation professionals: cases from Sweden, Canada and Indonesia. Environ. Sci. Policy 23, 12–23. doi: 10.1016/j.envsci.2012.06.014
Lövbrand, E., and Stripple, J. (2011). Making climate change governable: accounting for carbon as sinks, credits and personal budgets. Crit. Policy Stud. 5, 187–200. doi: 10.1080/19460171.2011.576531
Lückerath, D., Bogen, M., Rome, E., Sojeva, B., Ullrich, O., Worst, R., et al. (2018). “The RESIN climate change adaptation project and its simple modeling approach for risk-oriented vulnerability assessment,” in Proceedings of ASIM-Workshop STS/GMMS, 28:21–26. Hochschule Heilbronn, Germany. doi: 10.11128/sne.28.pn.10412
Lückerath, D., Rome, E., and Milde, K. (2023). Using impact chains for assessing local climate risk–a case study on impacts of extended periods of fluvial low waters and drought on a metropolitan region. Front. Clim. 5:1037117. doi: 10.3389/fclim.2023.1037117
Melo-Aguilar, C., Agulles, M., and Jordà, G. (2022). Introducing uncertainties in composite indicators. the case of the impact chain risk assessment framework. Front. Clim. 4:1019888. doi: 10.3389/fclim.2022.1019888
Menk, L., Terzi, S., Zebisch, M., Rome, E., Lückerath, D., Milde, K., et al. (2022). Climate change impact chains: a review of applications, challenges, and opportunities for climate risk and vulnerability assessments. Weather Clim. Soc. 14, 619–636. doi: 10.1175/WCAS-D-21-0014.1
Norström, A. V., Cvitanovic, C., Löf, M. F., West, S., Wyborn, C., Balvanera, P., et al. (2020). Principles for knowledge co-production in sustainability research. Nat. Sustainabil. 3, 182–190. doi: 10.1038/s41893-019-0448-2
Owen, A. (2017). Techniques for Evaluating the Differences in Multiregional Input-Output Databases. Cham: Springer International Publishing. doi: 10.1007/978-3-319-51556-4
Palutikof, J. P., Street, R. B., and Gardiner, E. P. (2019). Decision support platforms for climate change adaptation: an overview and introduction. Clim. Change 153, 459–476. doi: 10.1007/s10584-019-02445-2
Parry, M., Parry, M. L., Canziani, O., Palutikof, J., Van der Linden, P., and Hanson, C. (2007). Climate Change 2007-Impacts, Adaptation and Vulnerability: Working Group II Contribution to the Fourth Assessment Report of the IPCC. Vol. 4. Cambridge University Press.
Pervan, G., and Maimbo, M. (2005). “Designing a case study protocol for application in is research,” in Proceedings of the Ninth Pacific Asia Conference on Information Systems, 1281–92. PACIS. Available online at: http://hdl.handle.net/20.500.11937/45440
Pollitt, H., and Mercure, J. –F. (2018). The role of money and the financial sector in energy-economy models used for assessing climate and energy policy. Clim. Policy 18, 184–197. doi: 10.1080/14693062.2016.1277685
Rome, E., Ullrich, O., Lückerath, D., Worst, R., Xie, J., and Bogen, M. (2018). “IVAVIA: Impact and Vulnerability Analysis of Vital Infrastructures and Built-up Areas.” Springer. p. 84–97. doi: 10.1007/978-3-030-05849-4_7
Runhaar, H., Wilk, B., Persson, A., Uittenbroek, C., and Wamsler, C. (2018). Mainstreaming Climate adaptation: taking stock about ‘what works' from empirical research worldwide. Reg. Environ. Change 18, 1201–1210. doi: 10.1007/s10113-017-1259-5
Scherhaufer, P. (2014). Bridging the gap between the theory and practices of stakeholder participation in integrated vulnerability assessments of climate change. Syst. Pract. Action Res. 27, 449–463. doi: 10.1007/s11213-013-9294-8
Schneiderbauer, S., Baunach, D., Pedoth, L., Renner, K., Fritzsche, K., Bollin, C., et al. (2020). Spatial-explicit climate change vulnerability assessments based on impact chains. findings from a case study in burundi. Sustainabil. 12, 6354. doi: 10.3390/su12166354
Schneiderbauer, S., Pedoth, L., Zhang, D., and Zebisch, M. (2013). Assessing adaptive capacity within regional climate change vulnerability studies—an alpine example. Nat. Hazard. 67, 1059–1073. doi: 10.1007/s11069-011-9919-0
Scrieciu, S., Rezai, A., and Mechler, R. (2013). On the economic foundations of green growth discourses: the case of climate change mitigation and macroeconomic dynamics in economic modeling. Wiley Interdiscip. Rev. Energy Environ. 2, 251–268. doi: 10.1002/wene.57
Star, J., Rowland, E. L., Black, M. E., Enquist, C. A. F., Garfin, G., Hoffman, C. H., et al. (2016). Supporting adaptation decisions through scenario planning: enabling the effective use of multiple methods. Clim. Risk Manag. 13, 88–94. doi: 10.1016/j.crm.2016.08.001
Star, S. L., and Griesemer, J. R. (1989). Institutional ecology,translations' and boundary objects: amateurs and professionals in berkeley's museum of vertebrate zoology, 1907-39. Soc. Stud. Sci. 19, 387–420. doi: 10.1177/030631289019003001
Storbjörk, S. (2007). Governing climate adaptation in the local arena: challenges of risk management and planning in Sweden. Local Environ. 12, 457–469. doi: 10.1080/13549830701656960
Umweltbundesamt (2016). “Klimawirkungsketten Umweltbundesamt.” Dessau-Roßlau: Umweltbundesamt. Available online at: https://www.umweltbundesamt.de/sites/default/files/medien/380/dokumente/klimawirkungsketten_umweltbundesamt_2016.pdf
Umweltbundesamt (2019). “Folgen Des Globalen Klimawandels Für Deutschland. Erster Teilbericht: Die Wirkungsketten in Der Übersicht.” CLIMATE CHANGE 20/2019. Dessau-Roßlau: Umwelbundesamt. Available online at: https://www.umweltbundesamt.de/sites/default/files/medien/1410/publikationen/2019-10-02_cc_20-2019_impactchain_ap1_fuer_climate_change_reihe_final_2019_plain.pdf
UNDP (2010). “Mapping Climate Change Vulnerability and Impact Scenarios. A Guidebook for Sub-National Planners.” Available online at: https://www.adaptationcommunity.net/download/va/vulnerability-guides-manuals-reports/Mapping_Vulnerability_publication-November_2010.pdf
Vincent, K., and Cull, T. (2014). Using indicators to assess climate change vulnerabilities: are there lessons to learn for emerging loss and damage debates? Geogr. Compass 8, 1–12. doi: 10.1111/gec3.12105
Viner, D., Ekstrom, M., Hulbert, M., Warner, N. K., Wreford, A., and Zommers, Z. (2020). Understanding the dynamic nature of risk in climate change assessments—a new starting point for discussion. Atmos. Sci. Lett. 21:e958. doi: 10.1002/asl.958
Wiedmann, T., and Lenzen, M. (2018). Environmental and social footprints of international trade. Nat. Geosci.11, 314–321. doi: 10.1038/s41561-018-0113-9
Yin, R. K. (1994). Discovering the future of the case study. method in evaluation research. Eval. Pract. 15, 283–290. doi: 10.1016/0886-1633(94)90023-X
Zebisch, M., Schneiderbauer, S., Fritzsche, K., Bubeck, P., Kienberger, S., Kahlenborn, W., et al. (2021). The vulnerability sourcebook and climate impact chains–a standardised framework for a climate vulnerability and risk assessment. Int. J. Clim. Chang. Strat. Manag. 13, 35–59. doi: 10.1108/IJCCSM-07-2019-0042
Keywords: Climate Impact Chain, climate risk and vulnerability assessment, climate change, knowledge co-production, uncertainty management, transboundary climate risk, socioeconomic scenarios
Citation: Petutschnig L, Rome E, Lückerath D, Milde K, Gerger Swartling Å, Aall C, Meyer M, Jordá G, Gobert J, Englund M, André K, Bour M, Attoh EMNAN, Dale B, Renner K, Cauchy A, Reuschel S, Rudolf F, Agulles M, Melo-Aguilar C, Zebisch M and Kienberger S (2023) Research advancements for impact chain based climate risk and vulnerability assessments. Front. Clim. 5:1095631. doi: 10.3389/fclim.2023.1095631
Received: 11 November 2022; Accepted: 02 May 2023;
Published: 31 May 2023.
Edited by:
Jana Sillmann, Independent Researcher, Hamburg, GermanyReviewed by:
Laurens Bouwer, Climate Service Center Germany (GERICS), GermanyTiago Capela Lourenço, University of Lisbon, Portugal
Copyright © 2023 Petutschnig, Rome, Lückerath, Milde, Gerger Swartling, Aall, Meyer, Jordá, Gobert, Englund, André, Bour, Attoh, Dale, Renner, Cauchy, Reuschel, Rudolf, Agulles, Melo-Aguilar, Zebisch and Kienberger. This is an open-access article distributed under the terms of the Creative Commons Attribution License (CC BY). The use, distribution or reproduction in other forums is permitted, provided the original author(s) and the copyright owner(s) are credited and that the original publication in this journal is cited, in accordance with accepted academic practice. No use, distribution or reproduction is permitted which does not comply with these terms.
*Correspondence: Linda Petutschnig, TGluZGEuUGV0dXRzY2huaWdAcGx1cy5hYy5hdA==