- 1Water Systems and Global Change Group, Wageningen University, Wageningen, Netherlands
- 2Climate Adaptation Services, Bussum, Netherlands
Given that climate variability and change present unprecedented challenges to the rail sector, efforts to produce relevant climate data/information for climate risk management and adaptive decision making in the rail sector are gaining traction. However, inadequate understanding of climate change impact and information needs raises several concerns for the sector. This paper addressed the question: What climate risk information services are needed to support the adaptation needs of the rail sector? Data from interviews, literature reviews, and workshops were used. The results show that changes in precipitation, temperature, sea-level rise, and thunderstorms are the top drivers of climate risk in the sector. Additionally, the need for tailor-made climate information to manage these changes is in high demand. Although insufficient, rail organizations use special protocols to manage climate risk. Understudied countries have operational and design standards formulated in metrics and codes related to specific critical weather conditions as part of their Natural Hazard Management process. However, desirable adjustments in the standards are currently based on past events rather than future climate conditions. Future climate change information is relevant for medium- to longer-term decisions, strategy, and policymaking. For operational and design standards, weather and climate information provided by national weather service agencies are used but they also refer to the European standards and databases. National level data/information is preferred for developing thresholds for standards yet pan-European level information is also relevant in filling in missing data gaps. Therefore, rail organizations operate on flexibility and a “use of best available data” policy. Understanding how climate information is used to support decision-making in the rail sector is by no means an easy task given the variety of decisions to be taken at different spatial and temporal scales. However, stakeholder engagement proved to be an important step to better inform tailor-made information that is user relevant.
Introduction
Governments and organizations are concerned that extreme weather events caused by climate change will increase, and cause significant damage to infrastructure which undermines the development of many economic activities (Auld and Maclver, 2006). Globally, extreme weather events are recorded almost every year, causing frequent disturbances to railway organizations, affecting the safety, reliability, availability, and functionality of operations (Quinn et al., 2017). In addition to people's travel behavior, timing, travel modes, and travel routes are influenced by weather conditions (Sumalee et al., 2011). Meanwhile, the IPCC AR6 has stated that temperatures in Europe continue to rise, and the frequency and intensity of hot extremes have increased in recent decades. The report also indicates that they are projected to increase even further. In addition, extreme precipitation and pluvial flooding are expected to increase in all regions except the Mediterranean as global warming exceeds 1.5°C. The relative sea level will rise in all of Europe except the Baltic Sea and is projected to continue beyond the twenty first century irrespective of the level of global warming. This will increase the frequency and intensity of extreme sea level events leading to more coastal flooding. Severe windstorms at global warming of 2°C are also expected to increase (IPCC, 2021).
Given that the current and future climate changes endanger society and many sectors, the need for adaptation has gained increasing attention at the national and continental levels in Europe. This is particularly true for the transport sector where infrastructure and operations are more sensitive to extreme events such as storm surges, floods, and wind drafts compared to incremental changes in precipitation or temperature (Christodoulou and Demirel, 2018). Transport operations are however, more sensitive to climate change than infrastructure (Christodoulou and Demirel, 2018). Reports from past decades show that different weather conditions, exacerbated by climate change have caused severe damage to railway networks in different parts of Europe, affecting the functionality of the rail infrastructure and operational activities (Love et al., 2010; Nemry and Demirel, 2012; Schweikert et al., 2014). For instance, track warping due to uneven thermal expansion in the summer or build-up of snow and ice in the winter, lead to decreased speeds and causes derailment. Extreme cold causes brittle tracks and track separation while directly affecting the performance of rail operators (Xia et al., 2013). The severity of the climate effect is however, dependent on the type of design, age, and usage of the infrastructure (Baker et al., 2010).
Most weather-related failures in the rail sector are caused by high temperatures, rainfall, icing, storm, and lightning (Sabir et al., 2007). In Netherlands, 4–10% of all rail infrastructure failures have been attributed to adverse weather conditions (Duinmeijer and Bouwknegt, 2004; Xia et al., 2013). In 2018, 1,230 rail disruptions were caused by extreme weather in Netherlands (Scholten, 2020). Similar observations occurred in the UK where extremely high temperatures were associated with increased failures of rail buckles. In 2003 alone, 137 rail buckles cost £2.5 million in delays and repairs meanwhile buckling events are expected to be four to five times more frequent in the 2050s (Dobney et al., 2009; Jenkins et al., 2014). Also, 20% of all unplanned delays are due to adverse weather conditions (Thornes and Davis, 2002). More recently, it is estimated that ~1.6 million delay minutes on the railway each year are caused by weather conditions (Dawson et al., 2016). In Austria, about 95% of all infrastructure damages are triggered by floods and rain (Bachner, 2017). These weather-induced impacts result in different kinds of costs including infrastructure, operation, and user costs (Doll et al., 2014).
Railways play an instrumental role in the European economy as they facilitate the production and distribution of goods and economic services and form the basis for the provision of basic social services (European Commission, 2010). The use of the railway transport system in Europe is expected to increase in the future because it can operate on low energy, making it environmentally friendly and necessary for the transition toward more sustainable and energy-efficient transport solutions (Rotaris et al., 2022). However, the combination of high future demand for rail and the need for long term planning and design raises many concerns about how climate change adaptation can be accounted for in the planning, design, and management of railways (Blackwood et al., 2022; Ferranti et al., 2022). Some studies have examined the implications of climate change on transport infrastructure networks including railways and concluded that climate change will alter the impact of weather-induced risk on the design rules, procedures for the operation, and maintenance of infrastructure (Armstrong et al., 2016; Adams and Heidarzadeh, 2021; Palin et al., 2021). A few case studies in Austria (Doll et al., 2014), the UK (Armstrong et al., 2016), and Netherlands (Scholten, 2020) have focused on building the resilience of the rail sector against the current climate hazards and future uncertainties. Additionally, another important research area is how to handle future climate risks and uncertainties with climate information services when planning adaptation for infrastructure (e.g., Wilson and Burtell, 2002; Auld and Maclver, 2006).
Climate information services are generally defined as the provision of science-based, user-specific high-quality data on climate variables such as temperature, rainfall, wind, soil moisture, and sea level. It also includes information on risk and vulnerability analyses, assessments, long-term projections, and scenarios to equip decision makers in climate-sensitive sectors to manage risks and explore opportunities created by climate variability and change. This helps society to become more resilient in coping with the increasing impacts of climate change (Vaughan and Dessai, 2014; Vaughan et al., 2016). Climate information services, therefore, involve the timely production, translation, and delivery of useful climate data and information. Climate knowledge is necessary for planning, societal decision-making, and climate-smart policy (Machingura et al., 2018). However, some challenges preclude the progress of climate information services. These challenges include lack of understanding of specific climate information, lack of capacity to use specific climate information, and use of information not locally relevant or fit-for-purpose. Information might also not be available on time or there might be reluctance to incorporate climate information into management practices. Poor understanding of scientific uncertainties and unrealistic expectations from end users cannot be addressed with the current state of knowledge (Dinku et al., 2014; Lackstrom et al., 2014). Other challenges are related to the multiple meaning of the services, the governing right which raises the tension between climate service as a public good or a business opportunity, funding structures, and mechanisms. Co-production as a pre-condition in climate services projects and initiatives have also been discussed (Bruno et al., 2019). Therefore, it is imperative to improve the provision of climate information services.
The application of climate information services for adaptative design, planning, and decision making is relevant in managing climatic risk and uncertainties [World Meteorological Organization (WMO), 2002]. This is particularly true for the rail sector given the numerous adaptation needs of the sector that requires climate information. However, only few studies focus on adaptation initiatives for European railway administrators (Lindgren et al., 2009; EEA, 2014). Copernicus Climate Change Service (C3S) is an example of such an entity that seeks to develop a sectoral climate information system to support infrastructure, transportation, and associated standards (https://climate.copernicus.eu/).
This paper, therefore, aims to address the question: what climate information services are needed to support the adaptation needs of the rail sector? In this regard, the paper focused on first, understanding how climate information is currently used within rail organizations and potential strategies to support access and use of climate information. Second, it identified the most important climate change impacts on the rail organizations and how climate risk information is handled by the railway organizations. Third, we elaborated on how climate information is represented in the design and operational standards of rail organizations. Finally, we explored the need for standardization of trans-national pan-EU rail trajectories and the logic of common standards regarding climatic extremes. The study also draws data mainly from Netherlands and UK rail organizations with some considerable inputs from Spain and Austria rail. Although the empirical data produced offers case-specific views and considerations, the findings of this study could serve as a template for researchers and policymakers in other European countries to develop proactive climate information services that improve the adaptive capacity and resilience of the rail systems.
Conceptual framework
Even with the ongoing extreme efforts toward greenhouse gas mitigation, scholars have concluded that certain climatic changes will be inevitable and as a result adaptation remains critical on the agenda of many scientists and policy makers especially large infrastructure managers (IPCC, 2021). Despite continuous advances in climate change adaptation for various sectors, the rail sector has not benefited enough from these adaptation efforts. This is largely because of significant knowledge gaps on the effects of climate change on the railway and the mismatch between adaptation needs and the information available (Bowyer et al., 2020). Rooted in the field of climate change adaptation, this research adopts a descriptive approach to explore the adaptation needs of the railway sector toward the development of effective climate information services that makes the railway system resilient. The study is based on the premise that if the right climate information is given in time, and in the appropriate format to the right stakeholders, good adaptation strategies can be implemented to avert the consequences of climate change.
More than ever, railways are exposed to harsh weather conditions due to climate change and thus the need for adaptation is growing. Different railway companies implement various strategies aimed at reducing the impacts of flooding, storms, intense rainfall, thunderstorms, and hot temperatures to survive the extreme weather conditions and to recover quickly. These strategies could be changes in operations and infrastructure design such as making changes to protective structures, erosion and drainage, material specifications, land use planning, early warning, and maintenance planning (Koetse and Rietveld, 2012; Ortega et al., 2020). For example, increased extremes in precipitation may demand an increase in drainage capacity, and wider temperature ranges will affect the rail tracks and thus demand proper maintenance. An adaptive railway organization, therefore, adjusts intelligently to the changing climate and delivers service sustainably with value for money (Quinn et al., 2017). Achieving this requires that adaptation needs be met with actionable climate information.
Climate change adaptation refers to the changes that are made to a system in response to actual or expected climatic effects to reduce harm and explore opportunities (McCarthy et al., 2001) Also, the term “need” as used in the context of this study refers to the “gap” between “what is” (current conditions) and “what should or could be” (desired conditions). The gap between “what is” and “what could or should be” must be measured to operationalize need. Therefore, we operationalized adaptation needs assessment as a systematic process for identifying, analyzing, and prioritizing current and future climate adaptation needs for the rail sector (Moore et al., 2018). The adaptation needs expounded in this study are not meant to be exhaustive because of the limited number of case studies, but rather, to represent the most commonly cited needs in the rail sector.
From existing literature, we also operationalize climate information services as the generation, translation, and transfer of timely and useful climate information for adaptative design, operational decision-making, and planning that makes the railway system resilient. Regarding the design of the rail system as used in this study refers to infrastructure and track engineering systems including earthworks, bridges, tunnels, steelwork, timber, and track systems which form the base upon which the railway runs. For example, to give a train a good ride, the track alignment must be set within a millimeter of the design. Operational decisions also include efficient passenger flow, ease of access, comfort of passengers, and healthy working conditions for personnel. Borrowing from the work of Quinn et al. (2017), resilience is defined as the ability of a rail organization to provide services effectively and sustainably as the climate changes. Further, the authors mentioned the need for a resilient rail organization to possess the element of (a) robustness: the ability to resist disruption, (b) redundancy: the ability to use backup facilities to provide service during disruption, and (c) recovery: the ability to rapidly return to service after a disruption.
This study, therefore, focuses on the interface of climate change impacts, emerging adaptation needs, provision of climate information, and data for the design and operations of the rail sector, as an interactive system that improves the resilience of the rail sector. The framework in Figure 1 starts by questioning how climate change impacts influence critical adaptation needs and affects the design and operations of the rail sector. This then demands effective climate information services that meet the needs and make the rail systems resilient. Analyzing such interaction in the different European case countries is expected to inform the development of a common pan-EU standard to meet the desired needs and make the rail organizations more resilient. According to Gharehgozli et al. (2019), even though standards will facilitate interoperability, quality, and safety of intermodal transportation for better performance, they have received little attention in literature. The authors argue that although many technical standards such as safety control systems for the electrical power network have been developed to improve the interoperability of the European rail network, these are not yet used throughout Europe. This is especially true for climate sensitive standards in the rail sector. Thus, results from this study will contribute to this effort.
The approach adopted in this study considers how the rail organization currently understands and deals with the impacts of climate change. The analysis identifies and describes various needs and data required to improve adaptation. This goes from incremental reactive adjustments that enhance resilience to current impacts, to significant transformations anticipating future changes (Matyas and Pelling, 2015).
Methods
This study uses data from document analysis, expert interviews, and stakeholder workshops (Figure 2) to address the main question: what climate information services are needed to support the adaptation needs of the rail sector? The study investigated; (a) how climate information is currently used within the rail organizations, (b) climate change impacts on the rail organizations, (c) how climate information is represented in the design and operational standards of rail organizations, and (d) the need for standardization of trans-national pan-EU rail trajectories and the logic of common standards regarding climatic extremes.
Literature review
The study adopted a traditional literature review approach to analyze and summarize relevant information required to answer the research question. According to Coughlan et al. (2007), a traditional literature review can help in refining, focusing, and shaping research questions as well as help in developing theoretical and conceptual frameworks. Thus, information from the literature was used to inform the background of the study, development of the theoretical framework, and preparation of the relevant questions to be asked during the interviews and workshop.
Aside from scientific articles, we used gray literature comprised of documents and reports produced by rail organizations including ProRail and Network rail (see references for the list of literature and documents consulted). For example, based on various documents from ProRail and Network Rail on the consequences of climate change, a list of the most important climate change threats was developed. These threats were further discussed during the interviews and workshop. However, the disadvantage of this type of literature review is that it has no standard study protocol and detailed search strategy. Also, the searches are conducted using keywords with no specific selection criteria and are usually subjective and non-exhaustive. Usually, well-known articles are selected for this type of review which is normally prone to bias (Fletcher and Fletcher, 1997; Demeyin, 2016).
Interviews
Prior to the workshop, representatives of ProRail in Netherlands and Network Rail in the United Kingdom were interviewed. A total of 15 interviews were conducted (9 from ProRail, 6 from network rail) with people from different departments in the rail organizations to identify the most important impacts. These individuals have expertise ranging from:
• Station inspection and system safety.
• Contract and asset management.
• Signaling, switches specialist and system and civil engineering.
• Energy supply and electrical.
• Rail research and projects management.
• Infrastructure and maintenance work.
Interviewees were nominated by the main contact person of the rail organization and through a snowballing approach, where initial interviewers from each organization help recruit subsequent interviewees with specific relevant expertise. The selection of interviewers was based on individuals with skills and expertise relevant to the subject of discussion. We did not focus on large numbers but rather focused on the details of the discussions. It aimed to consult people with expertise on different aspects of asset management related to different climate hazards. We determined the sufficiency of the interview when it reached saturation; when interview responses yield no new or additional information or interview subject. The interview was structured to have a better understanding of the following issues:
a. How climate information is used within rail organizations.
b. Identify the most important climate change impacts on the rail organizations.
c. How climate information is represented in operational and design standards.
Based on the initial document analysis from ProRail and Network Rail, a list of the most important climate change threats was developed and discussed during interviews with 15 experts from different departments in the rail organizations. Subsequently, these impacts were fashioned into “impact schemes” indicating the relationships between climate hazards and impacts on specific parts of the rail system. Each interview was about 1 h, and the outcomes of the interviews served as a basis for the workshop.
Workshop
The workshop was organized at Amersfoort, Netherlands on 21st November 2019. Participants at the workshop included representatives from rail organizations, weather and climate service professionals, and standardization experts from the UK, Netherlands, Austria, and Spain. A total of 15 participants were purposively selected for the workshop: 7 from Netherlands (ProRail and ClimatAS and wageningen University); 4 from the UK (Network Rail and University of reading and John Dora consulting); 2 from Austria (Ubimet); and 2 from Spain (Tecnalia).
The workshop was divided into two sessions. The first session aimed at conceptualizing climate information in rail standards to understand how climate information is and could be represented (current situation and desired future) in rail standards across Europe. The discussions among participants were structured to focus on the design standards in one group and operational standards in the other group. A set of guiding questions were used to facilitate the group discussions (see Appendix A). The outcomes of each group discussion were presented on brainstorm posters.
The second session explored the climate change information demand from the rail organizations and supply from the climate data store using C3S. Based on participants' perspectives, climate change impacts were prioritized and projected using impact scheme posters. Every participant prioritized five impacts on the impact schemes based on the following:
1. Impact on the rail system.
2. Potential for developing indicators from the available climate datastore because data available may not be sufficient to develop relevant indicators to meet all information needs. For example, are there enough quality data to determine the number of days/years exceeding xx mm rainfall, as input for flood-related standards (matching demand with supply)?
3. Potential for integrating this information into rail standards.
Furthermore, participants discussed the needs and possibilities for relevant climate change information in the climate data store. Appendix B shows the guiding questions of the second session.
Results
Climate information use in rail organizations
Results of the interviews show that climate change impacts are a serious threat to railway infrastructure and operations. Thus, climate change information is relevant in managing these impacts. Rail companies are performing analyses to understand and address these impacts. For example, ProRail in Netherlands is currently performing climate vulnerability and risk analysis of the railway system and has developed guidance for integrating adaptation into new Rail projects. Network Rail in the UK has a special Weather Resilience and Climate Change program that guides weather and climate risk assessment for the design, construction, and maintenance of the rail system.
Potential climate change impacts
Results of the initial document analysis and interviews were aggregated into climate change impact schemes of precipitation (high and low precipitation), temperature (high and low temperature), thunderstorms, and sea-level rise as shown in Table 1. The design and operations of the understudied railway organizations are under serious threat of climate change impacts (Table 2). Changes in precipitation, temperature, sea-level rise and thunderstorms pose serious hazards to the railway systems.
Climate information on standards
Results show that rail organizations in the participating countries have their national standards but often make references to European standards. Operational standards of rail organizations use tailored made current weather information that is provided by weather service providers. In the design standards, however, different types of climate/weather information are used. Temperature for instance, is relevant for developing standards for European electrical equipment. There are national annexes to the Structural Eurocodes for snow and wind loads. Precipitation and flooding related standards are determined at the national and international levels. There are continuous efforts at the national level to update these standards. Table 3 shows ProRail and Network Rail examples.
Operational standards
Currently, individual countries have their operational standards that are related to specific critical weather conditions as part of their Natural Hazard Management process. These standards focus on day-to-day operation of the railway and facilitate efficient passenger flows, ease of access, comfort of passengers, and healthy working conditions for personnel. Based on weather forecasts, the operations may be adjusted for the coming days while climate change information may be relevant to review the operational standards for climate resilient asset management. For instance, the indicators of heat stress for rail workers and passengers include temperature and humidity. This serves as input for operational standards related to working conditions or passenger comfort and health.
In Netherlands, rail weather service provides weather alarms according to the “Four Season Matrix.” This Matrix provides critical thresholds for weather conditions that inform risk assessment and operational adjustment (Weijers, 2013). There are also weather alarms for temperature, wind, excessive rainfall, lightning, snow, frost, and freeze-thaw events. In addition, three weather codes with underpinning thresholds are used based on operational errors that have occurred in the past for these specific weather conditions. Code 1 describes temperature ranges that do not demand alterations in the operation. Code 2 describes a critical threshold that demands extra measures. Code 3 describes even more extreme weather events. The Austrian Östereichische Bundesbahnen (ÖBB) also known as Federal railway has a similar system. However, currently information on changing climatic conditions is not represented in operational standards. There are no efforts to screen the current operational standards for desirable adjustments anticipating future climate conditions. Yet, operational standards are changed incrementally through the weather changes that have occurred in the past decades.
Looking at the expected conditions of the desired future, results show that future climate change information is relevant for informed decision making in rail organizations, especially for medium to longer-term decisions. Knowing expected changes in medium- and long-term climatic conditions is valuable to the rail organization at strategy and policy levels. If specific temperatures are frequently exceeded in 20 years, then rail organizations would have to revise their operational practices, change design and product specifications to minimize operational disruptions, or rethink their operational performance indicators.
The thresholds for weather conditions that relate to operational standards can be determined at the national, regional, or local levels. Such thresholds may be dependent on current climate conditions, robustness of the rail system, and level of tolerable and manageable operational failure. Therefore, it does not make sense to determine these thresholds at the pan-European level. Also, national level information is preferred if there is good quality climate information as this is more detailed and locally relevant. Moreover, climate change information from regional sources such as CS3 climate datastore has a resolution of 12 × 12 km and does not include local characteristics that are relevant for local scale impact assessments. Another reason for using more local climate change information is that national meteorological offices often develop specific downscaled climate projections. However, it is noted that the quality, availability, and relevance of climate change information at the national levels vary. Therefore, if the needed information is not available at the national level, the climate datastore could fill the gaps by providing services in two main ways:
1. Provide climate change data that national meteorological offices and weather services can use to model local impacts under future climates. This information could be used for climate stress tests, relevant to the operational and design standards of the rail system.
2. Provide a climate change information “dashboard” where rail organizations can find statistics for frequency, duration, and intensity of critical weather conditions under current and future climate conditions across Europe. The end-users would specify the climate variable and the thresholds they want to explore to make this dashboard sensitive to the various national contexts and needs. The dashboard then shows how often this weather event occurs in the current climate and how often it can be expected in future climates.
Design standards
Currently, ProRail and Network Rail are in the process of moving from using historical data to using climate projections for their design and operational policies. Network Rail also uses historical weather data and a guideline outlining projected changes to the baseline data based on UK Climate Projections. Similarly, ProRail uses historical data for railway design in addition to climate change projections. ProRail also has a guide for incorporating climate change projections into new projects though it is not yet officially part of their existing design standards nor the EN/ISO standards.
In the future, rail organizations would prefer flexibility and would adopt a “use of best available data” policy. European standards (EN) do not prescribe safety levels but rather provide information on different levels that can be used by national rail organizations to set their standards. EN standards should be flexible. For instance, it should not stipulate the use of the T100 hourly rainstorm but provide a range of rainstorms and their occurrence in Europe for the national organizations to use at their convenience.
As for the “best available data” policy, the desired situation would be one central NR/ProRail standard that can be used internally and when subcontracting work to engineering firms. This central standard should not contain data itself but rather refer to EN standards and United Kingdom climate projection (UKCP)/Royal Netherlands Meteorological Institute (KNMI) scenarios and data. It should abstractly describe the data to use. The EN standards should include information on relevant parameters (weather and climate variables/indicators) for specific climate risks that should be used and how they influence railway infrastructure. For instance, the number of days above a critical temperature threshold is relevant for track buckling.
Based on maximum hourly precipitation, a range of safety levels was significant to consider. The actual information for the location of the infrastructure can then be considered as the best available data source. For instance, UKCP does not provide data on maximum temperatures or extreme precipitation, at least these are not readily available in the Network Rail climate guidance note. Despite a reluctance to use anything that is not country-specific, providing such information could encourage railway organizations to use European scale information sources.
Climate change information needs for operational and design standards
Results of the participants' discussion on information needs and potential for rail operation and design standards are presented in Table 4.
Discussion
Generally, intermodal transport plays a vital role in the European Union (EU) policy on the future transport network. Road transport has remained the most dominant mode of movement in the last three decades because it is fast, flexible, and relatively cheaper. However, the use of road transport is reducing due to traffic jams, higher fuel prices, road taxes, and the increasing awareness of environmental issues. A shift to rail is considered the most effective way of reducing the pressure on European roads, saving money, and saving the environment (Gharehgozli et al., 2019). Unfortunately, rail transport faces several challenges including climate change impacts that obstruct the delivery of reliable, safe, affordable, and attractive rail services in the pan-European rail corridors. Hence the call for a Railway Standardization strategy in Europe and the sector vision for 2050 [European Railway and Infrastructure Companies (CER), 2013; International Union of Railways (UIC), 2016]. The role of climate services in meeting adaptation needs that will make the sector resilient to climate change impact is absent in these unified railway standardization documents. This paper, highlights the climate information required to support the adaptation needs of the railway sector.
We found that the participating railway organizations use climate information for different types of risk analysis that are intrinsically related to context and dependent on location. Some studies have observed that the relevance of weather and climate information is dictated by the nature of the risks being managed, the region of interest, the specific economic sector, the governance structures, and other context-specific realities (Adger et al., 2009; Goddard et al., 2010). This realization should drive the need for context specific climate services to assist decision-making in the rail sector. However, efforts to make climate information services actionable have rather concentrated on improving underlying prediction or observation systems with little attention to improving the acceptability and usability of climate information for decision-making (Lemos et al., 2012; Kennel et al., 2016). Consistently, the extent to which climate information is used to support decision-making in the railway sector is not clear. Understanding this is by no means an easy task given the different backgrounds of end-users in the rail sector and the variety of decisions to be taken at different spatial and temporal scales. However, stakeholder engagement implemented in this study proved to be an important step to better inform and tailor climate information to parameters and formats that are user relevant.
Access to useful and usable climate information is an essential step toward building a climate resilient rail sector where climate risks are anticipated and mitigated, and at the same time potential opportunities are exploited (Jacob et al., 2015; Goddard, 2016; Street, 2016). The different climate risk analysis and adaptation actions performed by rail organizations can be attributed to recent progress in the generation and provision of weather and climate information that has created opportunities to improve decision-making (Hewitt et al., 2012; Adams et al., 2015; Wilkinson et al., 2015). However, weather and climate information will become more relevant if they are tailored to match needs and are provided in formats that easily facilitate its integration into decision-making processes (Ranger et al., 2010; Daron, 2015).
Our results also show that the design and operations of railway organizations are under serious threat of climate change impacts. Changes in precipitation, temperature, sea-level rise, and thunderstorms pose serious hazards to the railway systems. Rail infrastructure is built to last a lifetime with many still expected to be functional by the end of the twentieth century (Auld and Maclver, 2006). However, some existing rail networks are located in areas that make them vulnerable to climate hazards such as floods. The degree of impact of the identified hazards however, varies among countries and largely depends on the design, age, and usage (Oslakovic et al., 2013). According to Auld and Maclver (2006), many of the rail infrastructures in Europe are aging at an unexpected and unsustainable replacement and maintenance rate. Consequently, these structures are increasingly vulnerable to climate extremes. Therefore, to address these impacts, historical and projected climate information is needed to perform risk assessments to guide infrastructure decision making and by extension develop the design and operational standards for the rail sector.
The use of climate information in the analysis of risk and development of potential adaption for the rail sector has improved in recent years. However, there are still significant gaps. Further improvements to transform this information into the design and operational standards are urgently required not only at the national but at the Pan-EU level. Technical guides produced by national rail organizations can be used as examples of good practice in developing climate sensitive Pan-EU rail standards. While the Pan-EU standards may serve as a relevant reference for developing rail infrastructure and related activities in the region, we suggest that it remains optional to allow individual countries the possibility to define their own nationally determined parameters to suit local geographical and climatic conditions. Currently, climate change resilience standards are yet to be introduced at the European level for the railway sector. The existing Eurocodes related to construction do not completely address all climate resilience issues such as flooding caused by intense and prolonged rainfall. Therefore, new guidance is recommended to address critical environmental impacts such as water action and flooding which pose a significant risk to infrastructure (Dora, 2018). Many standards have been developed already to improve the homogeneity of the European intermodal rail market. However, implementation of these standards has been problematic due to cost, hence taking several years for standards to be implemented throughout Europe (Gharehgozli et al., 2019). The main issues to overcome while implementing climate sensitive standards are to improve better communication and exchange of information between stakeholders. For example the language, timeliness, and accuracy of information communicated must be improved. The unit of measurement in which climate information is given must be standardized and well understood by end-users. Additionally, member states must have the required infrastructure to interpret and use climate information with ease.
In the face of a changing climate, the benefits of climate related standards for the design, operation, maintenance, and emergency responses of the rail sector are many. First, standards will increase awareness and understanding of climate-related risks and opportunities for railway organizations for better risk management, informed decision-making, and strategic planning. Secondly, they will serve as tools for designers, builders, operators, and users to ensure infrastructure safety, operability, and longevity of railway systems. Thirdly, standards can contribute to reducing costs when adopted throughout a project lifecycle due to retrofitting while building more resilient infrastructure.
However, many challenges also exist. These challenges include determining local thresholds in standards based on local climate conditions, the vulnerability of specific railway infrastructure (e.g., state of maintenance), and the local level of accepting failures. Moreover, the resilience of railway infrastructure is not only location-specific, but hazards may affect the entire network causing disturbances in operation. Another critical challenge is how to deal with trans-national railway design standards that intersect different climatic zones. The rail sector requires different climate data and information some of which are very ambitious. The questions that require attention are (a) which of the required data or information is feasible to generate at what level of uncertainty? and (b) what investment is required?
Quality data that is available in forms that are useable for local decision making is required to develop effective and useful climate sensitive standards. Copernicus Data Store could serve as a key data source, yet data currently available are coarse. This makes rail companies deem the data store useless for risk analysis and formulation of standards. Hence relying on weather and climate data from national sources. Nonetheless, Copernicus Data Store could meet the climate information needs of rail organizations by providing comprehensive data that is downscaled and bias-corrected to meet local specifications and needs.
To effectively develop climate information that meet adaptation needs of the rail sector, accurate appreciation of the problem through robust climate impact assessment needs to be conducted. In this regard, we recommend the use of digital technologies as they possess inherent abilities that ensure faster and more accurate evaluation of the conditions of assets relevant to decision-making and adaptation planning. According to Argyroudis et al. (2022), the deployment of emerging digital technologies supported by multi-stakeholder engagement accelerates the containment and recovery of infrastructure from multiple hazards in a sustainable manner making systems more resilient. The authors argue that although the traditional approach to infrastructure management whereby expert judgment is based on manual measurements and visual inspection are mostly used, it is limited in terms of responding in time to climate change impacts. Therefore, emerging digital technologies, such as the Internet of Things (IoT), Artificial Intelligence, and Building Information Modeling (BIM) (Sacks et al., 2020) can be leveraged for proactive decision making before, during, and after hazard occurrences. This will ensure a resilient rail system. Currently, the main digital technologies and solutions which have accelerated transformation in the railway sector include IoT, Cloud Computing, Big Data Analytics, and Automation and Robotics (Pieriegud, 2018). Furthermore, the development of vulnerability and restoration models is needed to quantify the risk and resilience of railway infrastructure exposed to climatic stressors. For instance, in Britain, scour has caused the failure of railway bridges crossing rivers due to flood events and as a result, Lamb et al. (2019) developed a probabilistic model to quantify scour risk in terms of passenger journey disruptions and associated economic costs. Also, given that climate change is expected to have an impact on the frequency and/or magnitude of flood events and thus on the restoration of bridges, Mitoulis et al. (2021) developed recovery models for traffic reinstatement and capacity restoration of flood critical bridges. These include restoration task prioritization, scheduling, inter-task dependencies, idle times, durations, and cost ratios for different damage levels, as well as the evolution of traffic capacity after floods.
We recognize that the method used in this study to identify the climate information required to support the adaptation needs of the rail sector may not be exhaustive. A limitation of this qualitative study is that it relies on perceptions collected from interviews and workshops which could be diverse depending on the knowledge level of individuals involved. This can cause differences in emphasis, though where possible it was supported with literature sources. Using a traditional rather than systematic literature review resulted in a broad overview of the research even so, the interviews and workshop offer more detail. A second limitation is that the respondents of the study were from a limited number of countries in Europe which may not be representative. However, it underscores the importance of co-production and collaborative efforts in identifying and understanding these needs. The different participating rail companies hold certain unique knowledge and understanding about climate impact and adaptation which could be useful to other companies.
Conclusion
This study is among a few that go a step further into the subject of climate adaptation and climate information services standardization in the European railway system. It combined a literature review and an empirical part based on interviews and a workshop. The study highlighted the most important climate change impacts on rail organizations, what climate information is needed, and how climate information is currently used for design and operational standards within rail organizations. In addition, we explored the need for a common pan-EU rail standard regarding climatic extremes. We found that weather and climate are the dominating factors that can shape not only the design but also the operational standards of railways when properly handled. Rail organizations from different countries have different approaches to dealing with extreme weather and climate impacts. For example Network Rail has a full program dedicated to weather resilience and climate change, with tools to perform climate risk assessments. ProRail has also developed various tools to map the rail systems' vulnerabilities, such as the national climate stress test for rail. The rail organization in Austria is specifically advanced in its natural hazard management, being able to recover quickly after extreme weather conditions. The organizations however, differ in their experiences about using the information on climate change to inform their operational and design standards. This is related to the availability and quality of climate change information at the national level. Nationally sourced information is preferred by railway organizations because such information is more specific and better for decision making at the local level. Nevertheless, across Europe, this kind of detailed weather and climate information is not always readily available, especially for rail relevant parameters. We suggest that the Copernicus Data Store can fill this data gap especially for “less mature” railway organizations or where data sources are less comprehensive. An example is frequency-intensity statistics for a set of weather conditions in the future climate. Also, we recommend the use of digital technologies, and probabilistic and recovery models in assessing climate change impact on railway infrastructure due to their ability to perform a faster and more accurate evaluation of the conditions of assets adaptive decision-making. To develop effective tailored climate information services for the railway sector, it is essential to engage relevant stakeholders such as researchers and railway decision-makers. The adaptation information needs identified in this study underscore the importance of collaborative efforts. Finally, there is high potential for knowledge exchange between rail organizations. Rail organizations that just started exploring climate risks can learn from organizations that are more experienced in addressing these risks.
Data availability statement
The original contributions presented in the study are included in the article/supplementary material, further inquiries can be directed to the corresponding author.
Author contributions
EA, MS, and EB conducted the research and wrote the first draft of the article. HG and FL assisted with the research question, framing, and writing of the article. All authors contributed to the article and approved the submitted version.
Funding
This study was conducted under the UNCHAIN and SIS infra projects (The COPERNICUS Climate Change Sectoral Information System to support infrastructure, transport and associated standards). The UNCHAIN project is part of AXIS, an ERA-NET initiated by JPI Climate, and funded by ANR France, FFG Austria, BMBF Germany, FORMAS Sweden, AEI Spain, NWO (NL), RCN Norway with co-funding by H2020 (grant number 2015-3631).
Acknowledgments
The authors wish to thank all participating stakeholders in the project especially those from including ProRail, NetworkRail, Ubimet, Tecnalia, and John Dora consulting.
Conflict of interest
The authors declare that the research was conducted in the absence of any commercial or financial relationships that could be construed as a potential conflict of interest.
Publisher's note
All claims expressed in this article are solely those of the authors and do not necessarily represent those of their affiliated organizations, or those of the publisher, the editors and the reviewers. Any product that may be evaluated in this article, or claim that may be made by its manufacturer, is not guaranteed or endorsed by the publisher.
References
Adams, K., and Heidarzadeh, M. (2021). A multi-hazard risk model with cascading failure pathways for the Dawlish (UK) railway using historical and contemporary data. Int. J. Disaster Risk Reduct. 56, 102082. doi: 10.1016/j.ijdrr.2021.102082
Adams, P., Eitland, E., Hewitson, B., Vaughan, C., Wilby, R., and Zebiak, S. (2015). Toward an Ethical Framework for Climate Services. A White Paper of the Climate Services Partnership Working Group on Climate Services Ethics. Geneva: Climate Services Partnership.
Adger, W. N., Dessai, S., Goulden, M., Hulme, M., Lorenzoni, I., Nelson, D. R., et al. (2009). Are there social limits to adaptation to climate change? Clim. Change 93, 335–354. doi: 10.1007/s10584-008-9520-z
Argyroudis, S. A., Mitoulis, S. A., Chatzi, E., Baker, J. W., Brilakis, I., Gkoumas, K., et al. (2022). Digital technologies can enhance climate resilience of critical infrastructure. Clim. Risk Manag. 35, 100387. doi: 10.1016/j.crm.2021.100387
Armstrong, J., Preston, J., and Hood, I. (2016). “Adapting railways to provide resilience and sustainability,” in Proceedings of the Institution of Civil Engineers-Engineering Sustainability, Vol. 170 (London: Thomas Telford Ltd), 225–234. doi: 10.1680/jensu.15.00017
Auld, H., and Maclver, D. (2006). “Changing weather patterns, uncertainty and infrastructure risks: emerging adaptation requirements,” in Proceedings of the 2006 IEEE EIC Climate Change Conference (Piscataway, NJ: IEEE), 1–10. doi: 10.1109/EICCCC.2006.277249
Bachner, G. (2017). Assessing the economy-wide effects of climate change adaptation options of land transport systems in Austria. Reg. Environ. Change 17, 929–940. doi: 10.1007/s10113-016-1089-x
Baker, C. J., Chapman, L., Quinn, A., and Dobney, K. (2010). Climate change and the railway industry: a review. J. Mech. Eng. Sci. 224, 519–528. doi: 10.1243/09544062JMES1558
Blackwood, L., Renaud, F. G., and Gillespie, S. (2022). Nature-based solutions as climate change adaptation measures for rail infrastructure. Nat. Based Solut. 2, 100013. doi: 10.1016/j.nbsj.2022.100013
Bowyer, P., Hänsel, S., Smalley, E., Velegrakis, A., Dagan, M., and Wyrowski, L. (2020). Climate Change Impacts and Adaptation for Transport Networks and Nodes (No. ECE/TRANS/283). New York, NY: UN Publications.
Bruno, S., M., and Buontempo, C. (2019). Challenges to the sustainability of climate services in Europe. Wiley Interdiscip. Rev. Clim. Change 10, e587. doi: 10.1002/wcc.587
Christodoulou, A., and Demirel, H. (2018). Impacts of Climate Change on Transport. A Focus on Airports, Seaports and Inland Waterways. Maastricht: European Union. Available online at: https://core.ac.uk/download/pdf/146996656.pdf (accessed April 14, 2022).
Coughlan, M., Cronin, P., and Ryan, F. (2007). Step-by-step guide to critiquing research. Part 1: quantitative research. Br. J. Nurs. 16, 658–663. doi: 10.12968/bjon.2007.16.11.23681
Daron, J. D. (2015). Challenges in using a robust decision making approach to guide climate change adaptation in South Africa. Clim. Change 132, 459–473. doi: 10.1007/s10584-014-1242-9
Dawson, R. J., Johns, D., Gosling, S., Darch, G., Watson, G., Powrie, W., et al. (2016). UK Climate Change Risk Assessment Evidence Report. London: Williams Lee Group Publications. Available online at: https://www.theccc.org.uk/wp-content/uploads/2016/07/UK-CCRA-2017-Chapter-4-Infrastructure.pdf (accessed April 18, 2022).
Demeyin, W. (2016). Traditional Reviews Vs. Systematic Reviews. Available online at: https://s4be.cochrane.org/blog/2016/02/03/traditional-reviews-vssystematic-reviews/ (accessed September 30, 2022).
Dinku, T., Block, P., Sharoff, J., Hailemariam, K., Osgood, D., del Corral, J., et al. (2014). Bridging critical gaps in climate services and applications in Africa. Earth Perspect. 1, 1–13. doi: 10.1186/2194-6434-1-15
Dobney, K., Baker, C. J., Quinn, A. D., andChapman, L. (2009). Quantifying the effects of high summer temperatures due to climate change on buckling and rail related delays in south?east United Kingdom. Meteorol. Appl. 16, 245–251. doi: 10.1002/met.114
Doll, C., Trinks, C., Sedlacek, N., Pelikan, V., Comes, T., and Schultmann, F. (2014). Adapting rail and road networks to weather extremes: case studies for southern Germany and Austria. Natl. Hazards 72, 63–85. doi: 10.1007/s11069-013-0969-3
Dora, J. (2018). Climate Change: Standards, and Their Role in Improving the Climate Resilience of Infrastructure Investments. London: European Bank for Reconstruction and Development (EBRD). Available online at: https://www.ebrd.com/documents/climate-finance/climate-change-standards-and-their-role-in-improving-the-climate-resilience-of-infrastructure-investments.pdf?blobnocache=true (accessed May 17, 2021).
Duinmeijer, A. G. P., and Bouwknegt, R. (2004). Betrouwbaarheid Railinfrastructuur 2003 (Reliability Rail Infrastructure 2003). Utrecht: Prorail.
EEA (2014). Adaptation of Transport to Climate Change in Europe: Challenges and Options Across Transport Modes and Stakeholders. Copenhagen: EEA. Available online at: https://www.eea.europa.eu/publications/adaptation-of-transport-to-climate (accessed November 13, 2021).
European Commission (2010). Risk Assessment and Mapping Guidelines for Disaster Management. Brussels: European Commission, SEC 1626. Available online at: http://ec.europa.eu/echo/civil_protection/civil/pdfdocs/prevention/COMM_PDF_SEC_2010_1626_F_staff_working_document_en.pdf (accessed November 5, 2021).
European Railway and Infrastructure Companies (CER) (2013). Challenge 2050; The Rail Sector Vision. Brussels: CER [European Railway and Infrastructure Companies]. Available online at: https://uic.org/europe/IMG/pdf/europe_challenge2050.pdf (accessed October 3, 2022).
Ferranti, E. J. S., Fontana Oberling, D., and David Quinn, A. (2022). Transport resilience to weather and climate: an interdisciplinary view from Rio de Janeiro. Proc. Inst. Civil Eng. Urban Des. Plan. 175, 1–19. doi: 10.1680/jurdp.21.00006a
Fletcher, R. H., and Fletcher, S. W. (1997). Evidence-based approach to the medical literature. J. Gen. Internal Med. 12(Suppl 2), S5–S14. doi: 10.1046/j.1525-1497.12.s2.1.x
Gharehgozli, A., de Vries, H., and Decrauw, S. (2019). The role of standardisation in European intermodal transportation. Maritime Bus. Rev. 4, 151–168. doi: 10.1108/MABR-09-2018-0038
Goddard, L., Aitchellouche, Y., Baethgen, W., Dettinger, M., Graham, R., Hayman, P., et al. (2010). Providing seasonal-to-interannual climate information for risk management and decision making. Proc. Environ. Sci. 1, 81–101. doi: 10.1016/j.proenv.2010.09.007
Hewitt, C., Mason, S., and Walland, D. (2012). The global framework for climate services. Nat. Clim. Change 2, 831–832. doi: 10.1038/nclimate1745
International Union of Railways (UIC) (2016). Railway Standardisation Strategy Europe. Paris: UIC [International Union of Railways]. Available online at: https://uic.org/europe/IMG/pdf/rail_standardisation_strategy_europe_light.pdf (accessed October 3, 2022).
IPCC. (2021). “Climate change 2021: the physical science basis,” in Contribution of Working Group I to the Sixth Assessment Report of the Intergovernmental Panel on Climate Change, eds V. Masson-Delmotte, P. Zhai, A. Pirani, S. L. Connors, C. Péan, S. Berger, N. Caud, Y. Chen, L. Goldfarb, M. I. Gomis, M. Huang, K. Leitzell, E. Lonnoy, J. B. R. Matthews, T. K. Maycock, T. Waterfield, O. Yelekçi, R. Yu, and B. Zhou (Cambridge: Cambridge University Press).
Jacob, D., Runge, T., Street, R., Parry, M., and Scott, J. (2015). A European Research and Innovation Roadmap for Climate Services. European Commission, Directorate-General for Research and Innovation. Available online at: https://data.europa.eu/doi/10.2777/702151
Jenkins, K., Gilbey, M., Hall, J., Glenis, V., and Kilsby, C. (2014). Implications of climate change for thermal discomfort on underground railways. Transport. Res. Part D: Transport. Environ. 30, 1–9. doi: 10.1002/met.1910
Kennel, C. F., Briggs, S., and Victor, D. G. (2016). Making climate science more relevant. Science 354, 421–422. doi: 10.1126/science.aag3248
Koetse, M. J., and Rietveld, P. (2012). Adaptation to climate change in the transport sector. Transp. Rev. 32, 267–286. doi: 10.1080/01441647.2012.657716
Lackstrom, K., Kettle, N. P., Haywood, B., and Dow, K. (2014). Climate-sensitive decisions and time frames: A cross-sectoral analysis of information pathways in the Carolinas. Weather Clim. Soc. 6, 238–252. doi: 10.1175/WCAS-D-13-00030.1
Lamb, R., Garside, P., Pant, R., and Hall, J. W. (2019). A probabilistic model of the economic risk to Britain's railway network from bridge scour during floods. Risk Anal. 39, 2457–2478. doi: 10.1111/risa.13370
Lemos, M. C., Kirchhoff, C. J., and Ramprasad, V. (2012). Narrowing the climate information usability gap. Nat. Clim. Change 2, 789–794. doi: 10.1038/nclimate1614
Lindgren, J., Jonsson, D. K., and Carlsson-Kanyama, A. (2009). Climate adaptation of railways: lessons from Sweden. Eur. J. Transp. Infrastruct. Res. 9, 3295. doi: 10.18757/ejtir.2009.9.2.3295
Love, P. E., Edwards, D. J., Watson, H., and Davis, P. (2010). Rework in civil infrastructure projects: determination of cost predictors. J. Const. Eng. Manag. 136, 275–282. doi: 10.1061/(ASCE)CO.1943-7862.0000136
Machingura, F., Nyamwanza, A, Hulme, D., and Stuart, E. (2018). Climate information services, integrated knowledge systems and the 2030 Agenda for Sustainable Development. Sustain. Earth. 1, 1–7. doi: 10.1186/s42055-018-0003-4
Matyas, D., and Pelling, M. (2015). Positioning resilience for 2015: the role of resistance, incremental adjustment and transformation in disaster risk management policy. Disasters 39, s1–s18. doi: 10.1111/disa.12107
McCarthy, J. J., Canziani, O. F., Leary, N. A., Dokken, D. J., and White, K. S. (Eds.) (2001). Climate Change 2001: Impacts, Adaptation, and Vulnerability: Contribution of Working Group II to the Third Assessment Report of the Intergovernmental Panel on Climate Change, Vol. 2. Cambridge: Cambridge University Press.
Mitoulis, S. A., Argyroudis, S. A., Loli, M., and Imam, B. (2021). Restoration models for quantifying flood resilience of bridges. Eng. Struct. 238, 112180. doi: 10.1016/j.engstruct.2021.112180
Moore, D. E., Chappell, K., Sherman, L., and Vinayaga-Pavan, M. (2018). A conceptual framework for planning and assessing learning in continuing education activities designed for clinicians in one profession and/or clinical teams. Med. Teach. 40, 904–913. doi: 10.1080/0142159X.2018.1483578
Nemry, F., and Demirel, H. (2012). “Impacts of climate change on transport: A focus on road and rail transport infrastructures,” in European Commission, Joint Research Centre (JRC), Institute for Prospective Technological Studies (Luxembourg: European Union Publication), 93.
Ortega, E., Martín, B., and Aparicio, Á. (2020). Identification of critical sections of the Spanish transport system due to climate scenarios. J. Transp. Geogr. 84, 102691. doi: 10.1016/j.jtrangeo.2020.102691
Oslakovic, I. S., ter Maat, H. W., Hartmann, A., and Dewulf, G. (2013). “Risk assessment of climate change impacts on railway infrastructure,” in Proceedings - EPOC 2013 Conference. p. 1–17. Available online at: https://theicnet.org/wp-content/uploads/2015/07/2013-09-EPOC-Railroads-2013.pdf
Palin, E. J., Stipanovic Oslakovic, I., Gavin, K., and Quinn, A. (2021). Implications of climate change for railway infrastructure. Wiley Interdiscipl. Rev. Clim. Change 12, e728. doi: 10.1002/wcc.728
Pieriegud, J. (2018). Digital Transformation of Railways. Warszawa: Siemens Sp. z o.o. Available online at: https://rail-research.europa.eu/wp-content/uploads/2018/04/DIGITAL_TRANSFORMATION_RAILWAYS_2018_web.pdf (accessed October 26, 2018).
Quinn, A. D., Jack, A., Hodgkinson, S., Ferranti, E. J. S., Beckford, J., and Dora, J. (2017). Rail Adapt: Adapting the Railway for the Future. A Report for the International Union of Railways (UIC). Paris: International Union of Railways. Available online at: https://uic.org/IMG/pdf/railadapt_final_report.pdf (accessed March–April 4, 2022).
Ranger, N., Millner, A., Dietz, S., Fankhauser, S., Lopez, A., and Ruta, G. (2010). Adaptation in the UK: a decision-making process. Environ. Agency Policy Brief. 9, 1–62. Available online at: https://www.lse.ac.uk/granthaminstitute/publication/adaptation-in-the-uk-a-decision-making-process/ (accessed April 20, 2022).
Rotaris, L., Tonelli, S., and Capoani, L. (2022). Combined transport: cheaper and greener. A successful Italian case study. Res. Transp. Bus. Manag. 43, 100792. doi: 10.1016/j.rtbm.2022.100792
Sabir, M., Koetse, M. J. J., and Rietveld, P. (2007). “The impact of weather conditions on mode choice: empirical evidence for Netherlands,” in Proceedings of the BIVEC-GIBET Transport Research Day. p. 512–527.
Sacks, R., Girolami, M., and Brilakis, I. (2020). Building information modelling, artificial intelligence and construction tech. Develop. Built Environ. 4, 100011. doi: 10.1016/j.dibe.2020.100011
Scholten, J. (2020). Climate resilience of railway stations in Netherlands: a risk assessment for passenger stations (Master thesis)., Delft University of Technology. Available online at: http://resolver.tudelft.nl/uuid:ed2deeb7-e2b8-486c-b617-11d984c1af18 (accessed December, 2020).
Schweikert, A., Chinowsky, P., Kwiatkowski, K., and Espinet, X. (2014). The infrastructure planning support system: analyzing the impact of climate change on road infrastructure and development. Transp. Policy 35, 146–153. doi: 10.1016/j.tranpol.2014.05.019
Street, R. B. (2016). Towards a leading role on climate services in Europe: a research and innovation roadmap. Clim. Serv. 1, 2–5. doi: 10.1016/j.cliser.2015.12.001
Sumalee, A., Uchida, K., and Lam, W. H. (2011). Stochastic multi-modal transport network under demand uncertainties and adverse weather condition. Transp. Res. C Emerg. Technol. 19, 338–350. doi: 10.1016/j.trc.2010.05.018
Thornes, J. E., and Davis, B. (2002). “Managing the effects of weather on the rail network,” in Proceedings of the 5th Rail Engineering Conference(London).
Vaughan, C., Buja, L., Kruczkiewicz, A., and Goddard, L. (2016). Identifying research priorities to advance climate services. Clim. Serv. 4, 65–74. doi: 10.1016/j.cliser.2016.11.004
Vaughan, C., and Dessai, S. (2014). Climate services for society: origins, institutional arrangements, and design elements for an evaluation framework. Wiley Interdiscipl. Rev. Clim. Change 5, 587–603. doi: 10.1002/wcc.290
Weijers, E. (2013). 4 Seasons Matrix. File: 4 Seasons Weather Bureau vs 09-2013. Amsterdam: ProRail. Available online at: https://adoc.pub/4-seizoenen-matrix-auteur-etienne-weijersversie-datum-ingan.html (accessed March 18, 2022).
Wilkinson, E., Budimir, M., Ahmed, A. K., and Ouma, G. (2015). Climate Information and Services in BRACED Countries. BRACED Resilience Intel. London: IDI Publications. Available online at: https://cdn.odi.org/media/documents/10128.pdf (accessed March 1, 2022).
Wilson, M. I., and Burtell, M. H. (2002). Prioritizing Future Construction Research and Adapting to Climate Change: Infrastructure (Transport and Utilities). TRL Report PR/IS/13/02, prepared for the CRISP Commission, UK.
World Meteorological Organization (WMO) (2002). WMO questionnaire on the role and operation of national meteorological services: some findings. WMO Bull. 51, 342. Available online at: https://library.wmo.int/doc_num.php?explnum_id=6403
Xia, Y., Van Ommeren, J. N., Rietveld, P., and Verhagen, W. (2013). Railway infrastructure disturbances and train operator performance: the role of weather. Transp. Res. D Transp. Environ. 18, 97–102. doi: 10.1016/j.trd.2012.09.008
Appendix
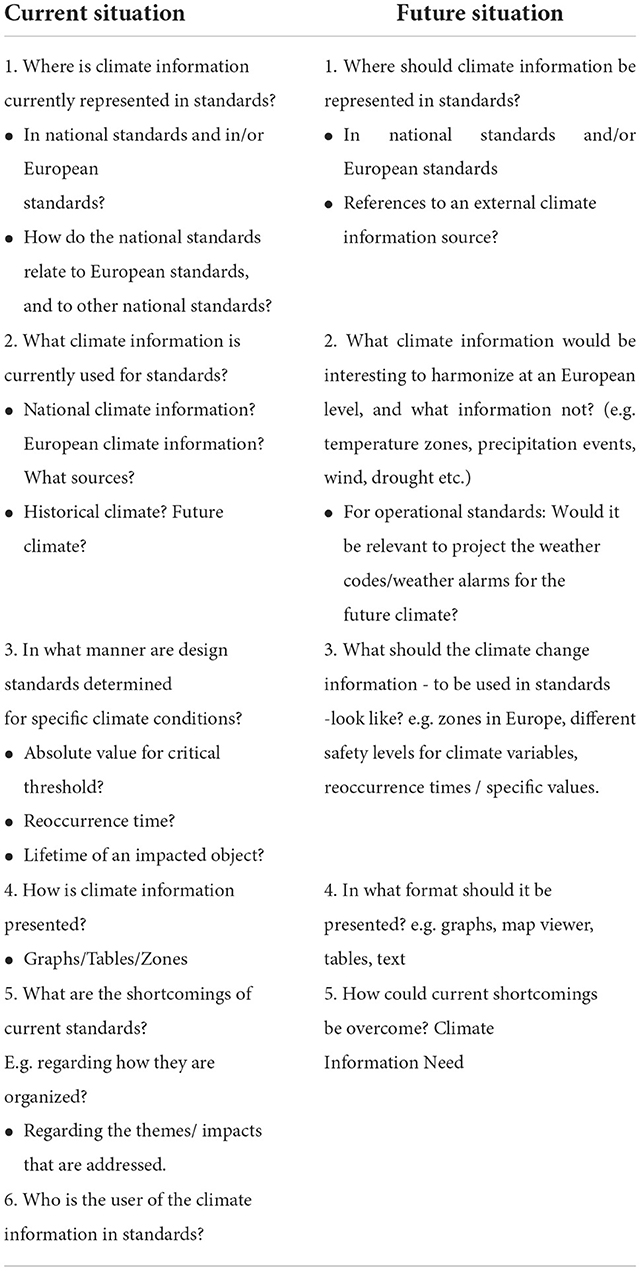
APPENDIX A. Questions for evaluating current and future situations of representing climate information in design and operational rail standards.
Keywords: climate change, climate services, adaptation, railway, Europe
Citation: Attoh EMNAN, Goosen H, van Selm M, Boon E and Ludwig F (2022) Climate services for the railway sector: A synthesis of adaptation information needs in Europe. Front. Clim. 4:968298. doi: 10.3389/fclim.2022.968298
Received: 13 June 2022; Accepted: 14 November 2022;
Published: 30 November 2022.
Edited by:
Scott Bremer, University of Bergen, NorwayReviewed by:
Sotirios Argyroudis, Brunel University London, United KingdomMeaghan E. Daly, University of New England, United States
Copyright © 2022 Attoh, Goosen, van Selm, Boon and Ludwig. This is an open-access article distributed under the terms of the Creative Commons Attribution License (CC BY). The use, distribution or reproduction in other forums is permitted, provided the original author(s) and the copyright owner(s) are credited and that the original publication in this journal is cited, in accordance with accepted academic practice. No use, distribution or reproduction is permitted which does not comply with these terms.
*Correspondence: Emmanuel M. N. A. N. Attoh, ZW1tYW51ZWwuYXR0b2hAd3VyLm5s; ZW55YWR6aUB5YWhvby5jb20=