- 1Department of Political Science, University of Houston, Houston, TX, United States
- 2Department of Chemical and Biomolecular Engineering, University of Houston, Houston, TX, United States
- 3Department of Chemistry, University of Houston, Houston, TX, United States
Deforestation fires are hindering climate change mitigation efforts and compounding global environmental challenges. Indonesia and Brazil have experienced high rates of deforestation fires in recent years, with many of the fires burning in peatland-rich ecosystems. We quantify the greenhouse gas (GHG) emissions associated with the 2019 and 2020 deforestation fires in both countries and determine the share of emissions originating from peatlands. Specifically, we use publicly available data for deforested area estimates and quantify the above-ground biomass (AGB), dry matter, and peat soil emissions associated with these fires. We find that the cumulative emissions impact from deforestation fires in Indonesia and Brazil was 3.7 (±0.4) and 1.9 (±0.2) Gt CO2eq in 2019 and 2020, respectively. Nearly half of this GHG impact can be attributed to emissions from peatlands. However, real-time monitoring tools can underestimate these emissions as fires in peatlands smolder underground and can go undetected by satellites. We compare our results with publicly available land-use and fire emissions data and find that the magnitude of underestimation is of the order of 200–300% for severe fire years and highest in the peatland-rich Brazilian Pantanal. We identify the gaps in current policies that are exacerbating the GHG and climate impacts of deforestation fires in Indonesia and Brazil and emphasize the need for regular pre- and post-fire ground measurement, transparent data sharing, and robust policy enforcement for effective forest and peatland protection.
Introduction
Global forest fires in 2019 and 2020, like those in Indonesia, Brazil, Central Africa, Siberia, Australia, and California, accounted for 10–15% of all global greenhouse gas emissions (Guo et al., 2019). Among these, the deforestation fires in Indonesia and Brazil were set to clear forest land to support anthropogenic activities like cattle farming, soybean farming, and palm oil farming (Macedo et al., 2012; Global Forest Watch, 2019; Marengo et al., 2021). Many of these commodity production-driven deforestation fires burned in critical peatland ecosystems. However, preliminary emissions data reported by monitoring tools and databases cannot account for the bulk of the emissions from peatlands as the fires smolder underground, are challenging to detect, isolate, and extinguish, and result in thick smoke plumes which limit the efficacy of satellite measurements. Hence, extensive ground measurements are essential to account for ecosystem-level impacts from peatland fires. While the emissions data are continually updated as post-fire ground measurements become available, the process of providing reliable estimates can suffer from a time lag of 5 years or longer (Ribeiro-Kumara et al., 2020). Consequently, reactive policies that are based on preliminary data fail to account for and to mitigate the true GHG impact of deforestation fires.
Peatlands cover only 3% of the world's landmass, but exist across 180 countries and are the largest terrestrial sink for carbon (Food and Agriculture Organization, 2020). Current estimates suggest that the carbon stored by peatlands is at least twice as much as all other vegetation types together, when above- and below-ground carbon are accounted for (UNEP, 2019). When drained and burned, peatlands emit the sequestered carbon and turn from net carbon sinks to sources. Typically, “slash and burn” methods have been used to induce deforestation fires to convert lands, including peatlands, to agricultural or commercial lands. Large swaths of peatlands in Europe, Southeast Asia, and South America have undergone significant degradation to support human development activities in this manner. Resultantly, 15% of the world's known peatlands have been irreversibly damaged or are currently experiencing extreme degradation, making them one of the most vulnerable and disproportionately impacted ecosystems (UNEP, 2019).
Unlike fast-spreading fires in other ecosystems, peatland fires are characterized by the slow-burning of surface vegetation followed by the gradual and sustained smoldering of peat soils over several days or weeks resulting in thick smoke plumes. Some megafires in peatlands have been known to burn for months extending laterally and in the sub-surface through layers of flammable carbon-rich soil (National Interagency Fire Center, 2019). The climate impacts of peatland fires are primarily attributed to CO2 emissions followed by carbon monoxide (CO) and methane (CH4) emissions. Peatland fires also contribute to pollution through smoke and haze. The emitted particulate matter severely affects biodiversity and human health and has detrimental socioeconomic impacts. The fires also cause local subsidence, soil leaching, and disruption to natural water regulation patterns resulting in increased flooding and contamination of freshwater sources.
Historically, global soil patterns have been analyzed at coarse physiographic scales ranging from 1:150,000 at the sub-national level to 1:1,000,000 at the national level (Food and Agriculture Organization, 2020). At these resolutions, the remote sensing methods can help identify large peat reserves, but the resultant maps do not provide substantial information about their depth or accurate estimation of their expanse. Peat depths can be mapped more comprehensively through in-situ probing or ground-penetrating radar; however, the time and labor-intensive nature of ground measurements and challenges of interpolation and representative mapping preclude widespread use. Many mapping inventories have not been updated to reflect how recent land-use changes have impacted peat reserves over time or to distinguish peatlands from wetlands (Food and Agriculture Organization, 2020). The high cost of representative and regular ground-based measurement (Vernimmen et al., 2020; O'Leary, 2021) and the lack of policy support at national and sub-national levels for peatland protection against deforestation results in poor initial estimates of their carbon sequestration capacity in their natural state and the associated emissions during fires or draining that occur to support human activities.
We utilize the 2019 and 2020 fires in Indonesia and Brazil as case studies to evaluate how under-accounting for the expanse of peatlands, the scale of deforestation fires in peat-rich ecosystems, and the carbon intensity of the emissions can lead to an initial under-estimation of their GHG impact. We estimate the GHG impact of these fires by using history-matched emissions factors (Akagi et al., 2011; Drösler et al., 2013; Banin et al., 2014; Giglio et al., 2017; van der Werf et al., 2017) with ecosystem- and country-level attributes (Yamakura et al., 1986; Levine, 1999; Neto et al., 2009; da Silva et al., 2013; Ratnaningsih and Prasytaningsih, 2017; Cassol et al., 2018; Ferraz et al., 2018; Moore et al., 2018; Serrati, 2018; Instituto Nacional de Pesquisas Espaciais, 2019; Global Fire Emissions Database, 2020; Greenpeace, 2020; Filho et al., 2021). Our results indicate that nearly half of the emissions from deforestation fires in Indonesia and Brazil can be attributed to peatlands, which can go undetected by satellites. For severe fire years in Indonesia and Brazil, the mismatch between the emissions estimated by our model and those reported by databases is as much as 2- to 3-fold. Therefore, real-time satellite measurements are found to severely under-account for the true GHG impact of deforestation fires. Even though the magnitude of the mismatch is reduced as new data becomes available, the effects of the underestimation persist over time. Lastly, we evaluate the current policy landscape in Indonesia and Brazil to understand the feedback between policies, deforestation fires, the under-mapping of peatlands, and their carbon impact. Deforestation fires and peatland degradation in both countries are caused by measurement challenges associated with representative, ground-based peatland mapping, inefficiencies in current policies and lax regulatory enforcement that fail to disincentivize commodity production-driven deforestation or administer appropriate punitive measures.
Materials and Methods
Our analysis is centered on deforestation fires in all Indonesian provinces, and the Legal Amazon (includes the states of Acre, Amapa, Amazonas, Pará, Rondônia, Roraima, Tocantins, Moranhão, and Mato Grosso) and the Pantanal (includes the states of Mato Grosso do Sul and Mato Grosso) in Brazil, as presented in Figure 1 (Hahn et al., 2014; Müller-Hansen et al., 2017). Indonesia's Ministry of Environment and Forest reported that the fires burned a forest area of ~6,400 sq. miles in 2019 and ~1,100 sq. miles in 2020 (Ministry of Environment and Forestry of the Republic of Indonesia, 2019; Greenpeace, 2020). Brazil's National Institute for Space Research (INPE) reported a burned forest area of ~3,900 sq. miles in 2019 and ~4,300 sq. miles in 2020 in the Amazon (Instituto Nacional de Pesquisas Espaciais, 2019). About 80% of the Pantanal lies within Brazil and the rest of the area in Bolivia and Paraguay (Serrati, 2018). Of the Brazilian Pantanal, about 65% of the area is in the state of Mato Grosso do Sul and 35% in Mato Grosso. Since INPE only monitors fires in the Amazon and Cerrado biomes, we used Global Forest Watch's estimates of ~200 sq. miles in 2019 and ~500 sq. miles in 2020 in Mato Grosso do Sul as our basis for the area impacted by deforestation fires in the Brazilian Pantanal. Based on these estimates of the burned area, we calculate the total GHG impact of deforestation fires by accounting for emissions from above-ground biomass, and peat soils and dry matter in peat-rich ecosystems. We present a conservative estimate of the GHG impact of the deforestation fires in the Pantanal since we assume that the same share of peat fires occurred in the Pantanal and the Legal Amazon, even though the Pantanal has greater peat depth and expanse (Marengo et al., 2021), and therefore, likely witnessed a larger share of peat fires.
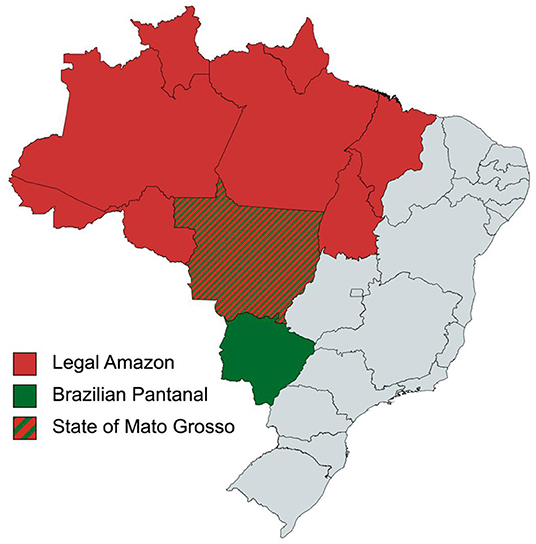
Figure 1. The map of Brazil showing the extents of the Legal Amazon and the Pantanal. The state of Mato Grosso includes 35% of the Brazilian Pantanal and the state of Mato Grosso do Sul includes the remaining 65%. The northern parts of the state of Mato Grosso include areas of Legal Amazon.
First, we determine the emissions from above-ground biomass (AGB) in the burned area using AGB = 0.0509 X ρD2H, where ρ is wood density in g/cm3, D is the tree's diameter in cm at breast height, and H is the average tree height in meter (Chave et al., 2005, 2014; Feldpausch et al., 2012; Banin et al., 2014). We assumed a carbon fraction of 0.48 for AGB, and a combustion efficiency of 0.9 in Indonesia and 0.89 in Brazil based on previous works (Andreae, 1991; Kaufman et al., 1992; Ward and Hao, 1992; Babbitt et al., 1996; Ferek et al., 1998; Levine, 1998, 1999; Innes et al., 2000; Neto et al., 2009; Ferraz et al., 2018). Ground-based analyses have found average tree heights of 29 m in Indonesia and 26 m in Brazil, average diameter at breast height 0.29 m in Indonesia and 0.25 m in Brazil, and similar wood densities of 600 kg/m3 per tree and fuel loads of ~152,000 trees per sq. mile (Yamakura et al., 1986; Malhi et al., 2004; Hunter et al., 2013; Banin et al., 2014; Cassol et al., 2018; Ferraz et al., 2018).
Next, to quantify the peat soil and dry matter emissions, we rely on previous studies in tropical peatlands which have reported average values of peat depth ranging from 0.3 m in both countries (Page et al., 2002; Kiely et al., 2019), bulk density of ~0.3 g/cm3 in Indonesia (Ratnaningsih and Prasytaningsih, 2017), 0.5 g/cm3 in the Legal Amazon (da Silva et al., 2013), 0.88 g/cm3 in the Brazilian Pantanal (Filho et al., 2021), and emissions factors for CO2, CH4, and CO (Akagi et al., 2011; Drösler et al., 2013; Banin et al., 2014; Giglio et al., 2017; van der Werf et al., 2017). We note that the Brazilian Pantanal has a significantly greater depth of peat, but in the absence of ecosystem-specific data, we considered the average burn depth to be 0.3 m, the same as in the Legal Amazon. These were used to quantify peat soil emissions, dry matter emissions from surface biomass, and the cumulative CO2 impact (Supplementary Tables 3–5) (Drösler et al., 2013; van der Werf et al., 2017). We assume that organic carbon forms 56% of the mass of tropical peat soils in Indonesia and 50% in Brazil and applied widely used emission factors from Indonesia and Brazil which are averaged over 20 years and adjusted for the time-effects of fires and land-use change (Akagi et al., 2011; Giglio et al., 2017; van der Werf et al., 2017; Moore et al., 2018; Kiely et al., 2019; Global Fire Emissions Database, 2020). In the absence of data on ecosystem-level attributes, we apply IPCC recommended emissions factors for tropical peatlands for Indonesia and Brazil and assume that organic carbon forms 54% of the mass of dry matter, which presents a fuel load of ~440 t/ha in both countries (Drösler et al., 2013). We do not account for the emissions that occur when flooded peatlands are drained, the water table is lowered, and the peat surface is first exposed to aerobic conditions. Other bases for calculations are listed in Supplementary Table 1. We accounted for the uncertainties in measurement, wherever applicable, by including the range of values used in the cited literature. These are the basis of the range of emissions included in our results.
Results
Our results indicate that Indonesia and Brazil collectively emitted nearly 2 Gt CO2eq (1.98 ± 0.11) in 2019 and 1 Gt CO2eq (0.97 ± 0.03) in 2020 from the burning of above-ground biomass due to deforestation fires (Table 1). Most notably, we found that including the emissions from deforestation in peatland ecosystems in both countries doubles the combined GHG impact to 3.65 ± 0.41 GtCO2eq in 2019 and ~1.89 ± 0.22 GtCO2eq in 2020 (Figure 2). The smaller burned area in Indonesia resulted in a 5-fold reduction in emissions from 2.44 ± 0.25 Gt CO2eq in 2019 to 0.44 ± 0.04 Gt CO2eq in 2020. While emissions from the Legal Amazon are comparable for 2019 and 2020, the peat-rich Pantanal is experiencing rapid degradation, and emissions from the region, per our results, have doubled between 2019 and 2020.
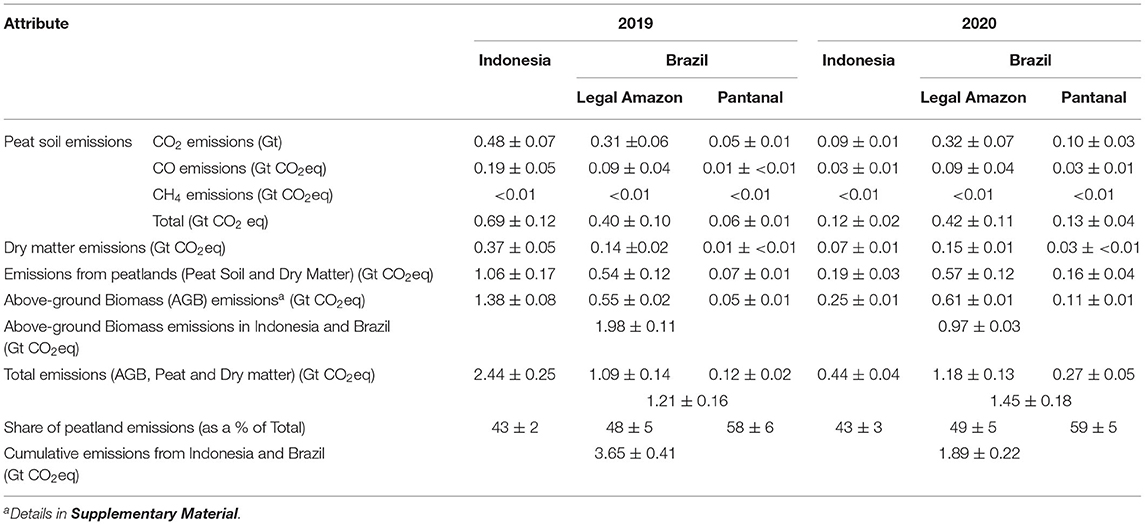
Table 1. GHG impact of deforestation fires in Indonesia and the Legal Amazon in Brazil, 2019 and 2020, calculated in this work.
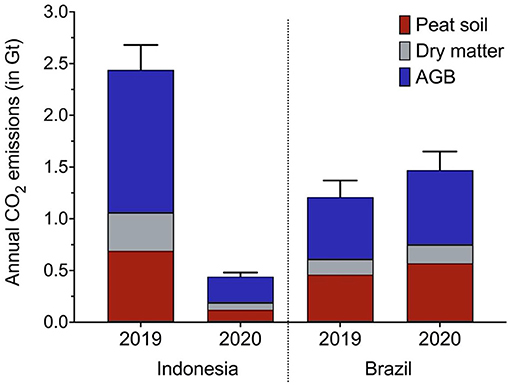
Figure 2. Assessment of the annual GHG impact of deforestation fires in Indonesia, the Legal Amazon in Brazil, and the Pantanal in Brazil during 2019 and 2020. The error bars represent the cumulative uncertainty associated with the calculated GHG impact, consisting of peat soil emissions, dry matter emissions, and aboveground biomass (AGB) emissions. For peat soil emissions, the error bars are calculated based on the uncertainties reported for the average burn depth from previous fires, the associated emission factors for CO2, CO, and CH4, and the mass of burned peat. For dry matter emissions, the error bars are calculated based on the uncertainties reported for the dry matter per sq. mile. For the AGB emissions, the error bars are calculated based on the uncertainties reported in the number of trees per sq. mile, the aboveground biomass per sq. mile, and the associated carbon content. The uncertainties are reported in Table 1 and Supplementary Material.
Additionally, since we find that peat emissions account for 40–60% of the GHG impact from deforestation fires across different biomes in Indonesia and Brazil, land-use or fire analyses that are based only on real-time satellite measurements present a partial and skewed outlook of the emissions impact of deforestation fires in peatland ecosystems. This can result in underreporting peatland emissions in the short-term and under-accounting for peatland degradation, in the short- and long-term. To understand the magnitude of underestimation, we present a comparison of our results with peer-reviewed estimates reported by Global Forest Watch (Hansen et al., 2013) in Table 2. While the estimates presented by the databases are continually updated as post-fire ground measurements become available, the process of providing better estimates typically takes 3–5 years. Therefore, we modeled the emissions from the 2015 fires in Indonesia and Brazil and compared our results with those reported by Global Forest Watch. Additional comparisons with the data reported by Copernicus Atmospheric Services (CAMS) and the Global Fire Emissions Database (GFED) in 2015, 2019, and 2020 and peer-reviewed analyses for 2015 are included in the Supplementary Material, along with the sources, scope, and methodologies relevant to each of these databases.
For Indonesia, we find a difference of nearly 1.8 ± 0.25 Gt CO2eq (~260%) between the results from our model (2.44 ± 0.25 Gt CO2eq) and those reported by Global Forest Watch (0.68 Gt CO2eq) for 2019, and a lower variation of 1.4 ± 0.25 Gt CO2eq (~130%) between our results (2.53 ± 0.25 Gt CO2eq) and the updated emissions reported by the database (1.1 Gt CO2eq) for 2015. We observe the smallest difference of <0.1 ± 0.04 Gt CO2eq (~19%) between our results (0.44 ± 0.04 Gt CO2eq) and those reported by Global Forest Watch (0.37 Gt CO2eq) for 2020, when favorable weather conditions, a pandemic-induced weakening of the economic incentives for deforestation, and strong policy enforcement helped arrest deforestation fires in Indonesia. The results from our model for the Legal Amazon concur with the data reported by Global Forest Watch (Figure 3 and Table 2). In contrast, we find substantial differences between the results from our model and the data reported by Global Forest Watch for the Brazilian Pantanal in 2019. The underestimation highlights that satellite-based measurements and post estimation are potentially under-accounting for the GHG impact of deforestation fires in peatlands by 2- to 3-fold immediately after the fires occur. The magnitude of the mismatch is reduced to half, i.e., 1- to 1.5-fold, as new data from ground measurements becomes available, but the effects of the underestimation remain substantial. Additionally, the Brazilian Pantanal is witnessing a sustained increase in deforestation fires and their GHG impact is poorly quantified.
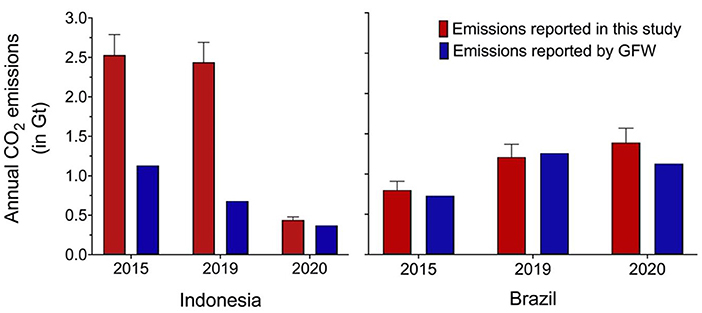
Figure 3. Comparison of the results of this study and the annual emissions reported by Global Forest Watch for 2015, 2019, and 2020 in Indonesia. The results of this study consist of peat soil emissions, dry matter emissions, and aboveground biomass (AGB) emissions and the uncertainties associated with each of these components yields the cumulative error bar presented for each year in the figure. The uncertainties are reported in Table 2 and Supplementary Material.
To evaluate how the emissions reported by databases change over time, we compared previous and publicly available estimates reported during or immediately after the fires occurred with current estimates from the Global Fire Emissions Database (GFED) (Global Fire Emissions Database, 2015, 2020, 2021). These trends are presented in Table 3.
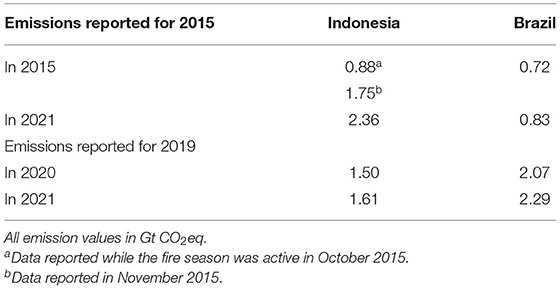
Table 3. Data reported by Global Fire Emissions Database (GFED) for Indonesia and Brazil (Legal Amazon) for 2015 and 2019.
The emissions reported by GFED during the fire season in 2015 in Indonesia have since changed by nearly 170%, while those for the Legal Amazon have changed by 15%. Similarly, 2020 estimates for Indonesia have changed by 7% and by 10% for the Legal Amazon. Overall, the reported emissions have consistently increased over time, with the most substantial increase observed for Indonesia's 2015 emissions. The trends in Table 3 are consistent with our hypothesis that real-time satellite data reported during or immediately after the fire season can underestimate the GHG impact of deforestation fires.
Policy Implications and Conclusions
Our findings indicate that the emissions from deforestation fires in Indonesia and Brazil accounted for 7 and 3% of total global GHG emissions in 2019 and 2020, respectively. Moreover, emissions from the fires that occurred in peatlands accounted for nearly half of this GHG contribution from both countries. Deforestations fires are not only resulting in substantial forest and carbon sink loss but are also a significant source of GHG emissions. Peatlands, that store at least twice as much carbon as all other vegetation types, can turn from carbon sinks to sources when perturbed by fires. Therefore, understanding the role of peatlands as carbon sinks and the GHG impact associated with deforestation fires in these critical ecosystems is an urgent emissions reduction priority that requires effective, timely, and transparent measurement, data collection, management, and sharing.
In the case of Indonesia and the Pantanal in Brazil, we observe that emissions from peatlands are underestimated during severe fire years, but periodic updates can reduce the magnitude of the mismatch. Specifically, satellite-based measurements and post estimation are potentially under-accounting for the GHG impact of deforestation fires in peatlands by 2- to 3-fold immediately after the fires occur, but the underestimation is reduced to half over a 5-year period. The mismatch was found to be the lowest for 2020, when favorable weather conditions, stringent policy enforcement, and the global recession weakened the drivers of deforestation in Indonesia. In contrast, the Brazilian Pantanal is experiencing progressive degradation through deforestation and the emissions from the region were found to have significantly increased between 2015 (0.07 ± 0.02 Gt CO2eq) and 2020 (0.27 ± 0.05 Gt CO2eq). Regular pre- and post-fire ground measurement when the forests are accessible during periods of low fire activity, and verification of ground-based data with real-time satellite data can help reduce the incongruity between reported emissions and the true GHG impact of deforestation fires.
It follows from our findings that the absence of policy efforts to map peatlands is a significant barrier toward peatland conservation and restoration in both countries; however, better mapping alone will be insufficient in mitigating the challenges associated with deforestation fires. The ASEAN Peatland Forests Project (APFP) and the UN's Global Peatlands Initiative were established over the last two decades for comprehensive mapping and to enable multi-stakeholder community-based programs to restore peatlands, reduce the rate of degradation, and the risk of fire and haze. In addition to being a partner country of both the initiatives, the Indonesian government issued a permanent moratorium on clearing peatlands and new concessions in primary forests in 2011. Penalty provisions under Indonesia's National Forestry Law and Environmental Protection and Management Law were also expanded to include imprisonment and fines ranging between 3 and 10 billion Indonesian Rupiah ($200,000–700,000 USD), while international support from the UN's Office of Drugs and Crime was directed at strengthening enforcement (United Nations Office of Drugs and Crime, 2011). These measures, along with Indonesia's Freedom of Information Act and the One-Map initiative to map peatlands at a resolution of 1:25,000 and establish a single national database for forests, peatlands, and land-use were expected to boost peatland conservation (Shahab, 2016).
Despite these efforts, large swaths of peatlands remain unmapped, data sharing through OneMap is restrained by the government, and indigenous areas with some of the largest peat reserves have been excluded by regulation. In 2019, the conversion of forests to palm and paper plantations drove many of the fires, and the government drew criticism over the shape-shifting nature of the maps included in the moratoria that were altered to support commodity production-driven agriculture. Contrastingly, in 2020, the economic downturn from the COVID-19 pandemic weakened demand for palm oil, and milder weather conditions and international pressure to control GHG emissions and deforestation led to peatland rewetting and strict enforcement of the moratoria on peatland draining.
In contrast, Brazil has witnessed dramatic and significant erosion of forest protection policies over the last 2 years which has facilitated an increase in deforestation for commodity production, especially in regions beyond the Legal Amazon such as the peatlands in the Pantanal. In 2019, INPE first initiated efforts to monitor deforestation across all biomes through REDD+. However, the effort is expected to cease in 2022 as Germany and Norway have frozen future funding, and the tripartite Amazon Fund Guidance Committee between Brazil, Norway, and Germany was dissolved in reaction to the Brazilian government's weakened policy stance on forest protection and weak enforcement of the moratoria on illegal soy and beef farming (Presidency of the Republic of Brazil, 2019a). Simultaneously, Brazil's anti-deforestation law enforcement agency, the Institute of Environment and Renewable Natural Resources (IBAMA), witnessed a 25% reduction in its budget appropriations and all logging fines have been suspended since 2019 (Presidency of the Republic of Brazil, 2019b). As for peatlands, the known deposits in Brazil are managed under the 1971 Ramsar Convention on Wetlands; but the 25 recognized Ramsar sites in Brazil have not been mapped to assess the peat thickness, and beyond the known sites, the extent and depth of peatlands remain largely undiscovered (The Ramsar Convention Secretariat, 2014). As a result, Brazil does not have peatland protection policies in place even though a mapping effort by the Center for International Forestry Research (CIFOR) and the United States Agency for International Development in 2017 revealed that South America may have the highest tropical peat deposits and Brazil potentially leads the region in peatland area and volume contribution (Gumbricht et al., 2017).
While both countries have established forest protection policies, and peatland protection policies in the case of Indonesia, along with attracting financial resources to better map peatlands through several international mechanisms, weak enforcement and reversal of policies continue to enable large-scale deforestation. Our findings emphasize the need for regular pre- and post-fire ground measurement verification with real-time satellite data across all biomes. Especially in peatlands, pre-fire ground assessments can help appropriately map their extent and depth, resulting in better post-fire estimates which can complement satellite measurements. If the presence, depth, and expanse of peatlands close to areas experiencing human development are unknown, then the potential of peatlands as carbon sinks and as sources of emissions during deforestation fires are underestimated and misrepresented in global carbon cycle fluxes. As a result, appropriate mitigation policies corresponding to the true impact of fires cannot be established and enforcement gaps and inefficiencies in current policies continue to incentivize the drivers for commodity production-driven deforestation. Monitoring across all biomes and transparent data sharing must be encouraged and appropriately funded by the government and international mechanisms, robust policy enforcement must be coordinated across the national and local levels, and severe punitive actions which outweigh the economic benefits of commodity production-driven deforestation must be established for effective forest and peatland protection and climate change mitigation.
Data Availability Statement
The original contributions presented in the study are included in the article/Supplementary Material, further inquiries can be directed to the corresponding author/s.
Author Contributions
RK developed and directed the project. RK and AD have contributed equally to the design, research, analysis, and writing. All authors have made a direct and substantial intellectual contribution to the work and have approved it for publication.
Conflict of Interest
The authors declare that the research was conducted in the absence of any commercial or financial relationships that could be construed as a potential conflict of interest.
Publisher's Note
All claims expressed in this article are solely those of the authors and do not necessarily represent those of their affiliated organizations, or those of the publisher, the editors and the reviewers. Any product that may be evaluated in this article, or claim that may be made by its manufacturer, is not guaranteed or endorsed by the publisher.
Supplementary Material
The Supplementary Material for this article can be found online at: https://www.frontiersin.org/articles/10.3389/fclim.2022.799632/full#supplementary-material
References
Akagi, S. K., Yokelson, R. J., Wiedinmyer, C., Alvarado, M. J., Reid, J. S., Karl, T., et al. (2011). Emission factors for open and domestic biomass burning for use in atmospheric models. Atmos. Chem. Phys. 4039, 4039–4072. doi: 10.5194/acp-11-4039-2011
Andreae, O. M. (1991). Biomass Burning-Its History, Use, and Distribution and Its Impact on Environmental Quality and Global Climate. Cambridge: MIT Press.
Babbitt, R. E., Ward, D. E., Susott, R. A., Artaxo, P., and Kauffman, J. B. (1996). “A comparison of concurrent airborne and ground based emissions generated from biomass burning in the Amazon Basin,” in SCAR-B Proceedings (São Paulo).
Banin, L., Lewis, S. L., Lopez-Gonzalez, G., Baker, T. R., Quesada, C. A., Chao, K., et al. (2014). Tropical forest wood production: a cross-continental comparison. J. Ecol. 102, 1025–1037. doi: 10.1111/1365-2745.12263
Cassol, H. L. G., Shimabukuro, Y. E., de Brito Carreiras, J. M., and Moraes, E. C. (2018). Improved tree height estimation of secondary forests in the Brazilian Amazon. Acta Amazon. 48, 179–190. doi: 10.1590/1809-4392201700844
Chave, J., Andalo, C., Brown, S., Cairns, M. A., Chambers, J. Q., Eamus, D., et al. (2005). Tree allometry and improved estimation of carbon stocks and balance in tropical forests. Oecologia 145, 87–99. doi: 10.1007/s00442-005-0100-x
Chave, J., Réjou-Méchain, M., Búrquez, A., Chidumayo, E., Colgan, M. S., Delitti, W. B. C., et al. (2014). Improved allometric models to estimate the aboveground biomass of tropical trees. Glob. Change Biol. 20, 3177–3190. doi: 10.1111/gcb.12629
da Silva, M. L., Silva, A. C., Silva, B. P. C., Barral, U. M., Soares, P. G., and Vidal-Torrado, P. (2013). Surface mapping, organic matter and water stocks in peatlands of the Serra do Espinhaço Meridional-Brazil. Revista Brasileira de Ciência do Solo. 37, 1149–1157. doi: 10.1590/S0100-06832013000500004
Drösler, M., Verchot, L. V., and Pan, G. (2013). Drained Inland Organic Soils. Hayama: Institute for Global Environmental Strategies (IGES).
Feldpausch, T. R., Lloyd, J., Lewis, S. L., Brienen, J. W. R., Gloor, M., Monteagudo Mendoza, A., et al. (2012). Tree height integrated into pantropical forest biomass estimates. Biogeosciences 9, 3381–3403. doi: 10.5194/bg-9-3381-2012
Ferek, R. J., Reid, J. S., Hobbs, P. V., Blake, D. R., and Liousse, C. (1998). Emission factors of hydrocarbons, halocarbons, trace gases and particles from biomass burning in Brazil. J. Geophys. Res. Atmos. 103, 32107–32118. doi: 10.1029/98JD00692
Ferraz, A., Saatchi, S., Xu, L., Hagen, S., Chave, J., Yu, Y., et al. (2018). Carbon storage potential in degraded forests of Kalimantan, Indonesia. Environ. Res. Lett. 13:095001. doi: 10.1088/1748-9326/aad782
Filho, G. C. K., Bufacchi, P., Costa, F., Cortez, E. V., Andrade, J. C., Ribeiro, K., et al. (2021). Smoldering characteristics of high bulk density peat. Proc. Combust. Inst. 38, 5053–5062.
Food and Agriculture Organization (2020). Peatland Mapping and Monitoring- Recommendations and Technical Overview. Rome: United Nations.
Giglio, J. L., Randerson, J. T., and van der Werf, G. R. (2017). Analysis of daily, monthly, and annual burned area using the fourth-generation global fire emissions database (GFED4). J. Geophys. Res. Biogeosci. 118, 317–328. doi: 10.1002/jgrg.20042
Global Fire Emissions Database (2015). Fire Season: Indonesia. Available online at: http://globalfiredata.org/pages/category/indonesia/
Global Fire Emissions Database (2020). Analysis Tool. Available online at: http://www.globalfiredata.org/analysis.html
Global Fire Emissions Database (2021). Amazon Dashboard. Available online at: http://globalfiredata.org/pages/amazon-dashboard/#faq_methods
Global Forest Watch (2019). Indonesia Oil Palm Concessions. Available online at: https://data.globalforestwatch.org/datasets/indonesia-oil-palm-concessions/geoservice?geometry=95.111%2C-5.971%2C126.752%2C1.709
Gumbricht, T., Roman-Cuesta, R. M., Verchot, L., Herold, M., Wittmann, F., Householder, E., et al. (2017). An expert system model for mapping tropical wetlands and peatlands reveals South America as the largest contributor. Glob. Change Biol. 23, 3581–3599. doi: 10.1111/gcb.13689
Guo, M., Li, J., Wen, L., and Huang, S. (2019). Estimation of CO2 emissions from wildfires using OCO-2 data. Atmosphere 10, 581. doi: 10.3390/atmos10100581
Hahn, M. B., Gangnon, R. E., Barcellos, C., Asner, G. P., and Patz, J. A. (2014). Influence of deforestation, logging, and fire on malaria in the Brazilian Amazon. PLoS ONE 9, e85725. doi: 10.1371/journal.pone.0085725
Hansen, M. C., Potapov, P., Moore, R., Hancher, M., Turubanova, S. A., Tyukavina, A., et al. (2013). High-resolution global maps of 21st-century forest cover change. Science 342, 6160. doi: 10.1126/science.1244693
Hunter, M. O., Keller, M., Victoria, D., and Morton, D. C. (2013). Tree height and tropical forest biomass estimation. Biogeosciences 10, 8385–8399. doi: 10.5194/bg-10-8385-2013
Innes, J. L., Beniston, M., and Verstraete, M. M. (2000). Biomass Burning and Its Inter-Relationships with the Climate System. Dordrecht: Springer. doi: 10.1007/0-306-47959-1
Instituto Nacional de Pesquisas Espaciais (2019). Terra Brasilis| PRODES (Deforestation). Available online at: http://terrabrasilis.dpi.inpe.br/app/dashboard/deforestation/biomes/legal_amazon/rates
Kaufman, Y. J., Setzer, A., Ward, D., Tanre, D., Holben, B. N., Menzel, P., et al. (1992). Biomass burning airborne and spaceborne experiment in the Amazonas (BASE-A). J. Geophys. Res. Atmos. 97, 14581–14599. doi: 10.1029/92JD00275
Kiely, L., Spracklen, D. V., Wiedinmyer, C., Conibear, L., Reddington, C. L., Archer-Nicholls, S., et al. (2019). New estimate of particulate emissions from Indonesian peat fires in 2015. Atmos. Chem. Phys. 19, 11105. doi: 10.5194/acp-19-11105-2019
Levine, J. S. (1998). Gaseous and particulate emissions released to the atmosphere from vegetation fires. Health Guidelines Veget Fire Events 6, 284–308.
Levine, J. S. (1999). The 1997 fires in Kalimantan and Sumatra, Indonesia: gaseous and particulate emissions. Geophys. Res. Lett. 26, 815–818. doi: 10.1029/1999GL900067
Macedo, M. N., DeFries, R. S., Morton, D. C., Stickler, C. M., Galford, G. L., and Shimabukuro, Y. E. (2012). Decoupling of deforestation and soy production in the southern Amazon during the late 2000s. Proc. Natl. Acad. Sci. U.S.A. 109:1341–1346. doi: 10.1073/pnas.1111374109
Malhi, Y., Baker, T. R., Phillips, O. L., Almeida, S., Alvarez, E., Arroyo, L., et al. (2004). The above-ground coarse wood productivity of 104 Neotropical forest plots. Glob. Change Biol. 10, 563–591. doi: 10.1111/j.1529-8817.2003.00778.x
Marengo, J. A., Cunha, A. P., Cuartas, L. A., Deusdará Leal, K. R., Broedel, E., Seluchi, M. E., et al. (2021). Extreme drought in the Brazilian Pantanal in 2019-2020: characterization, causes, and impacts. Front. Water 3:639204. doi: 10.3389/frwa.2021.639204
Ministry of Environment Forestry of the Republic of Indonesia (2019). SiPongi Karhutla Monitoring System. Available online at: http://sipongi.menlhk.go.id/home/main
Moore, T. R., Large, D., Talbot, J., Wang, M., and Riley, J. L. (2018). The stoichiometry of carbon, hydrogen, and oxygen in peat. J. Geophys. Res. Biogeosci. 123:3101–3110. doi: 10.1029/2018JG004574
Müller-Hansen, F., Cardoso, M. F., Dalla-Nora, E. L., Donges, J. F., Heitzig, J., Kurths, J., et al. (2017). A matrix clustering method to explore patterns of land-cover transitions in satellite-derived maps of the Brazilian Amazon. Nonlinear Process. Geophys. 24, 113–123. doi: 10.5194/npg-24-113-2017
Neto, T. G. S., Carvalho, J. A., Veras, C. A. G., Alvarado, E. C., Gielow, R., Lincoln, E. N., et al. (2009). Biomass consumption and CO2, CO and main hydrocarbon gas emissions in an Amazonian forest clearing fire. Atmos. Environ. 43, 438–446. doi: 10.1016/j.atmosenv.2008.07.063
O'Leary, D. (2021). Mapping peat using radiometrics. Nat. Rev. Earth Environ. 2, 523–523. doi: 10.1038/s43017-021-00200-9
Page, S. E., Siegert, F., Rieley, J. O., Boehm, V. H., Jaya, A., and Limin, S. (2002). The amount of carbon released from peat and forest fires in Indonesia during 1997. Nature 420, 61–65. doi: 10.1038/nature01131
Presidency of the Republic of Brazil. (2019a). DECREE No. 9,759. Planalto.gov.br. Available online at: http://www.planalto.gov.br/ccivil_03/_ato2019-2022/2019/decreto/D9759.htm
Presidency of the Republic of Brazil. (2019b). DECREE No. 9,760. Planalto.gov.br. Available online at: http://www.planalto.gov.br/ccivil_03/_ato2019-2022/2019/Decreto/D9760.htm
Ratnaningsih, A. T., and Prasytaningsih, S. R. (2017). The characteristics of peats and CO2 emission due to fire in industrial plant forests. Earth Environ. Sci. 97, 012029. doi: 10.1088/1755-1315/97/1/012029
Ribeiro-Kumara, C., Köster, E., Aaltonen, H., and Köster, K. (2020). How do forest fires affect soil greenhouse gas emissions in upland boreal forests? A review. Environ. Res. 184, 109328. doi: 10.1016/j.envres.2020.109328
Serrati, V. B. (2018). Exploring Ecosystem Services Provided by the Pantanal Wetland, South America. A Preliminary Review of Methods to Improve the Knowledge on the Benefits Provided by the Wetlands. Wageningen University and Research.
The Ramsar Convention Secretariat (2014). Ramsar Convention: Brazil. Available online at: https://www.ramsar.org/wetland/brazil
UNEP (2019). Peatlands Store Twice as Much Carbon as all the World's Forests. Available online at: https://www.unep.org/news-and-stories/story/peatlands-store-twice-much-carbon-all-worlds-forests
United Nations Office of Drugs Crime (2011). Forest Crimes. Available online at: https://www.unodc.org/southeastasiaandpacific/en/indonesia/forest-crime.html
van der Werf, G. R., Randerson, J. T., Giglio, L., van Leeuwen, T. T., Chen, Y., Rogers, B. M., et al. (2017). Global fire emissions estimates during 1997-2016. Earth Syst. Sci. Data 9, 697–720. doi: 10.5194/essd-9-697-2017
Vernimmen, R., Hooijer, A., Akmalia, R., Fitranatanegara, N., Mulyadi, D., Yuherdha, A., et al. (2020). Mapping deep peat carbon stock from a LiDAR based DTM and field measurements, with application to eastern Sumatra. Carbon Balance Manage. 15:4. doi: 10.1186/s13021-020-00139-2
Ward, D. E., and Hao, W. M. (1992). “Air toxic emissions from burning of biomass globally-preliminary estimates,” Paper Presented at 85th Annual Meeting (Vancouver, BC: Air and Waste Management Association).
Keywords: peatlands, deforestation fires, peat emissions, climate change, greenhouse gas emissions
Citation: Datta A and Krishnamoorti R (2022) Understanding the Greenhouse Gas Impact of Deforestation Fires in Indonesia and Brazil in 2019 and 2020. Front. Clim. 4:799632. doi: 10.3389/fclim.2022.799632
Received: 21 October 2021; Accepted: 11 January 2022;
Published: 08 February 2022.
Edited by:
Daniel L. Swain, University of California, Los Angeles, United StatesReviewed by:
Leticia Gomes, University of Brazilia, BrazilRichard Birdsey, Woods Hole Research Center, United States
Copyright © 2022 Datta and Krishnamoorti. This is an open-access article distributed under the terms of the Creative Commons Attribution License (CC BY). The use, distribution or reproduction in other forums is permitted, provided the original author(s) and the copyright owner(s) are credited and that the original publication in this journal is cited, in accordance with accepted academic practice. No use, distribution or reproduction is permitted which does not comply with these terms.
*Correspondence: Ramanan Krishnamoorti, ramanan@uh.edu