- 1Environmental Change Institute, School of Geography, University of Oxford, Oxford, United Kingdom
- 2Smith School of Enterprise and the Environment, School of Geography, University of Oxford, Oxford, United Kingdom
- 3Atmospheric, Oceanic and Planetary Physics, Department of Physics, University of Oxford, Oxford, United Kingdom
The deployment of carbon dioxide removal (CDR) needs to be scaled up to achieve net zero emission pledges. In this paper we survey the policy mechanisms currently in place globally to incentivise CDR, together with an estimate of what different mechanisms are paying per tonne of CDR, and how those costs are currently distributed. Incentive structures are grouped into three structures, market-based, public procurement, and fiscal mechanisms. We find the majority of mechanisms currently in operation are underresourced and pay too little to enable a portfolio of CDR that could support achievement of net zero. The majority of mechanisms are concentrated in market-based and fiscal structures, specifically carbon markets and subsidies. While not primarily motivated by CDR, mechanisms tend to support established afforestation and soil carbon sequestration methods. Mechanisms for geological CDR remain largely underdeveloped relative to the requirements of modelled net zero scenarios. Commercialisation pathways for CDR require suitable policies and markets throughout the projects development cycle. Discussion and investment in CDR has tended to focus on technology development. Our findings suggest that an equal or greater emphasis on policy innovation may be required if future requirements for CDR are to be met. This study can further support research and policy on the identification of incentive gaps and realistic potential for CDR globally.
1. Introduction
Most scenarios for meeting the Paris Agreement objective of limiting warming to well-below 2°C and pursue efforts to 1.5°C include carbon dioxide removal (CDR), with a primary focus on compensating for temporary carbon budget overshoot and offsetting expensive-to-abate emissions (IPCC, 2022). Scaling up CDR to the level required in these scenarios will require policies, incentives, obligations, and commercialisation mechanisms (hereafter “mechanisms”). However, the degree to which current and proposed mechanisms will contribute to achieving net zero targets is unclear.
As CO2 remains in the atmosphere for millennia after being emitted, a durable state of net zero emissions will only be achieved if CO2 removed from the atmosphere is stored on an equivalent timescale (Fankhauser et al., 2021). Currently, among other factors, the prices, durability, and development of both biological and geological CDR approaches differ greatly (IPCC, 2022). The likely outcome with biological CDR is shorter-term carbon storage, which makes it best suited to addressing land-use emissions (Alcalde et al., 2018). Although geological CDR has the potential to permanently remove emissions, these techniques can have higher costs, need to demonstrate efficacy and be proven at scale (Fuss et al., 2018).
Different CDR approaches require different policy support based on factors including their business model, maturity and cost. For instance, R&D pilot project subsidies may be more effective at the early stages of development, followed by long term policy that is results-based and included in broader policy instruments (Honegger et al., 2021). Beyond early-stage research and development funding, government action to address CDR's “incentive gap” remains limited (Fridahl et al., 2020). Few results-based policy instruments for CDR are currently in use, especially for geological CDR, which continues to be heavily dependent on funding for pilot projects (Schenuit et al., 2021). Interventions will be required alongside direct financial incentives to address additional barriers, such as developing skills and CO2 transport and storage (Zhou et al., 2022). Portfolio-based risk management approaches may also be required to support the diversity of risk by each CDR type (Nemet et al., 2018).
To our knowledge, this is the first paper to survey policy mechanisms around the world to catalogue their present cost and scale. A combination of web-based keyword searches, reviews of policy documents and interviews with stakeholders were used to identify mechanisms. Key criteria for the inclusion of a mechanism in this analysis includes the presence of some form of government oversight and support beyond a first-of-a-kind project. These conditions preclude some voluntary carbon markets, which also have inherent uncertainty and issues with environmental integrity, and non-scalable mechanisms including funds for trials, research and innovation. We note recent innovations, including Frontier's advanced market commitment model, are emerging to overcome some of these voluntary carbon market limitations (Frontier Climate, 2022).
We find that globally, there are limited examples of mechanisms which explicitly incentivise the deployment of CDR. Operational market-based mechanisms pay between €1/tCO2 and €88/tCO2, public procurement type mechanisms pay between €10/tCO2 and €100/tCO2 and direct fiscal incentives pay between €0.5/tCO2 and €180/tCO2. While not primarily motivated by CO2 removal, mechanisms tend to support established afforestation and soil carbon sequestration methods. Although geological storage is covered in some schemes, the incentives they provide are inadequate and, in some cases, not available to geological CDR. Outside of California, there are few current examples of a coordinated portfolio of mechanisms which attempt to achieve a balance between geological and biological CDR options. Existing mechanism explicitly focused on CDR are geographically concentrated in the UK, USA (mostly California) and New Zealand.
The remainder of this paper is organised as follows: the next section introduces a survey of CDR commercialisation pathways, including an explanation of how they work as well as an analysis of their prices and scale. The analysis concludes in Section 3.
2. Mechanisms
Mechanisms which support CDR can operate on a scale between fully market-based or dependant on fiscal incentives. We identify three broad incentive structures:
Market-based mechanisms include carbon markets and prices and tradable obligation schemes. They focus on the principle of payment by polluters or suppliers of fossil fuels. In these markets “removal units” (RUs),1 representing one tonne of CO2 removed, can be bought and sold.
Public procurement mechanisms can provide a minimum level of return, price or demand to suppliers within an existing market-based mechanism. These incentives tend to focus on results-based payments of CO2 removed or reduced rather than grants focused on costs incurred.
Subsidy and tax credit mechanisms are the purest form of fiscal incentive in which the state contributes a significant amount to the cost of developing and operating the CDR rather than the direct polluter.
Once mechanisms were identified through web-based searches, reviewing of policy documents and interviews with stakeholders they were grouped into the typology in Figure 1. The Appendix contains a list of the key search terms used, and the analysis has been updated as of September 2022.
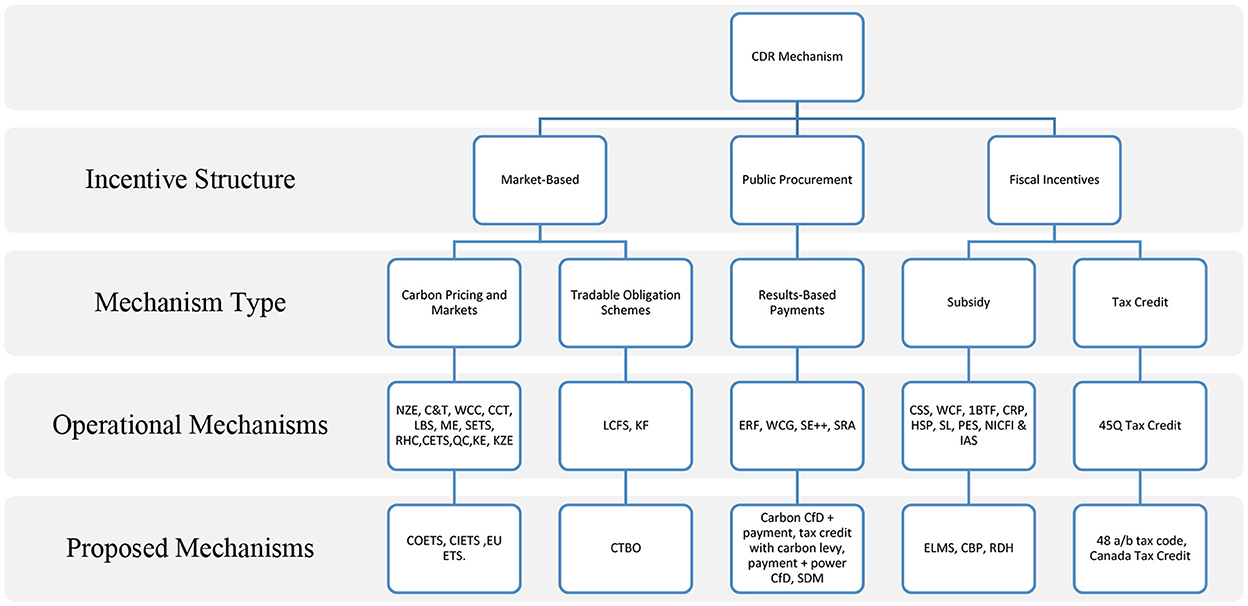
Figure 1. Categorisation of mechanisms. Carbon pricing and markets: WCC, Woodland Carbon Code; C&T, California Cap and Trade; NZE, New Zealand ETS; LBC, Label Bas Carbone; RHC, Registro de Huella de Carbono; CCT, Colombia's Carbon Tax; CETS, China ETS; QC, Québec Cap-and-Trade System; KE, Korean ETS; KZE, Kazakhstan ETS; SETS, Saitama's ETS; COETS, Colombia ETS. Tradeable obligation scheme: LCFS, Low-Carbon Fuel Standard; KF, Klik foundation; CTBO, Carbon Take Back Obligation. Results-based payments: WCG, Woodland Carbon Guarantee; ERF, Emissions Reductions Fund; CfD, Contract for Difference; SRA, Swedish Reverse Auction. Subsidy: CSS, Countryside Stewardship Scheme; WCF, Woodland Carbon Fund; 1BTF, One Billion Trees Fund; CRP, Conservation Reserve Program; HSP, CDFA Healthy Soils Program; SL, Sowing Life; IAS, Ireland's Afforestation Scheme; PES, Costa Rica's; NICFI, Norway's; ELMS, Environment Land Management Scheme; RDH, Regional Direct Air Capture Hubs. Tax credit: 45Q, 48a/b tax code, Canada Tax Credit.
Figure 1 generalises all of the mechanisms reviewed into five mechanism type categories based on how costs are distributed between polluters (market-based) and governments (fiscal incentives). Mechanism types include: Carbon Markets and Prices, Tradable Obligation Schemes, Results-based Payments, Subsidy and Tax Credit. Mechanism types may also need to ensure that cash flows from projects are predictable, consistent and have certainty to be more attractive to investors.
2.1. Carbon pricing and markets
Carbon pricing and market schemes targeting emissions reductions in certain sectors of the economy can allow for the use of CDR through offsets. While we focus here on markets for CDR, it should be noted that the majority of carbon offsets traded in markets currently come from reduced or avoided emissions rather than removals (Shankleman and Rathi, 2021). The UKs Woodland Carbon Code (WCC), Spain's Registro de Huella de Carbono (RHC), and France's Label Bas-Carbone (LBC) operate as domestic carbon standards which participate in voluntary carbon markets. Requirements of issued certificates to be surrendered to buffer accounts range from 20% for the WCC, 10% for the RHC and 10–25% for the LBC depending on the projects reversal risk (Cevallos et al., 2019; WCC, 2019a,b). Each standard is administered directly or indirectly by a government ministry and forestry projects are expected to last 30 years in the RHC and LBC or up to 100 years in the WCC. The WCC and RHC use public registries to sell RUs whereas in the LBC RUs are negotiated and traded directly between the project owner and the buyer. LBC largely focuses on afforestation and reforestation projects but is expected to expand to other forms of sequestration including agroforestry soil carbon in agriculture and mangroves (Cevallos et al., 2019). WCC units cannot be used in the UK Emissions Trading Scheme (ETS) or outside the UK (including shipping and aviation) and reporting is required every 10 years after the first 5 years. Follow up reports are required every 5 years with Spain's RHC and third party verification of ex-post GHG emissions if a buyer retires RUs.
Both Colombia and Chile have a carbon tax of approximately US$5/tCO2e. In Columbia companies can avoid paying the tax by purchasing carbon offsets from projects located in Colombia. Accepted offsets include afforestation, improved forest management and REDD+ projects as well as agricultural and grassland management projects. The Colombian Government is expected to trial an ETS (COETS) which will allow for removals to offset emissions as a complementary policy to the carbon tax between 2023 and 2024 (Cevallos et al., 2019). The Chilean government is currently working on developing offset regulation which could allow regulated entities to offset part or all of their emissions subject to the tax. Offsets, their threshold limits, and their transactions are expected to be operational in 2023 (ICAP, 2021b).
Some ETSs already have experience with the use of RUs, albeit primarily in the context of offsets from afforestation and reforestation projects. Generally, offset projects must be located within the same nation as the ETS or in regions where ETSs are linked. Examples include in California (C&T), Québec (QC), China (CETS) and the Korean ETS (KE)—all of which established quantitative limits and qualitative criteria for offset use. Compliance entities may use RUs to meet up to 4% of their emissions compliance in the C&T, 5% in the CETS and 8% in the QC (EDF, 2020; CARB, 2021; ICAP, 2021a). Each project that is issued offset credits contributes between 17 and 19% in the C&T and 3% in the QC of their total credits into a buffer account (ARB, 2021; ICAP, 2021a). Mexico's proposed national ETS (ME), currently in a pilot phase, is expected to allow proposed participants to meet up to 10% of their compliance obligations with offsets generated domestically. Kazakhstan's Emissions Trading Scheme (KZE) allows for the use of domestic offsets without quantitative limits but projects are expected to apply Clean Development Mechanism principles (EDF, 2015; ICAP, 2021c). In Japan, the Saitama's ETS is linked to Tokyo's Cap-and-Trade Program and allows for forest absorption credits to be used as offsets (ICAP, 2022a). New Zealand's ETS (NZE) awards allowances for removals from forestry, without a limit on the total number of units from those activities that can go into the ETS. Owners of forest land created after 1989 in New Zealand can voluntarily join its ETS and receive RUs as their forest grows. Owners of non-exempt pre-1990 forest land are required to participate in the system and are subject to its obligations if they deforest (ICAP, 2022b).
Operators who perform CCS in Europe can retain allowances when emissions have been verified as captured and transported for permanent storage under the European ETS (EU ETS) (Council Directive 2018/410/EC). While there is no current provision for CDR in the EU ETS, several potential methods for inclusion have been proposed. Rickels et al. (2021) propose that a regulatory authority could procure removal units into the market as a method to incentivise geological CDR too, a prerequisite to the approach would be the creation of an unused allowance pool that could be designated as RUs (Rickels et al., 2021). The European Commission has proposed that a Carbon Removal Certification Scheme could be integrated into the EU ETS in 2030 (European Commission, 2021a). Hickey and Allen (2021) propose a “European Removals Fund”, funded by allowances from the ETS and other sources, which could use a reverse auction to procure removals required to offset hard-to-abate sectors in Europe. Applying a temporary multiplier value on allowances to match the cost of RUs from geological CDR is also considered in their analysis i.e., operators of removals receive a multiple of allowances which match their costs (Hickey and Allen, 2021). ETS allowances could also be limited to a specific global-warming goal, but allowances for further emissions in the form of carbon debt which needs to be removed in future could be issued. Emitters pay for temporary storage in the atmosphere through interest payments on their carbon debt (Bednar et al., 2021). Bednar et al. (2021) conclude that this would lead both to earlier reductions in carbon emissions and to earlier application of removal technologies.
Cabral et al. (2019) find repurposed coal to Bioenergy with Carbon Capture and Storage (BECCS) plants could reduce the levelized cost of BECCS plants to between $70/MWh and $100/MWh, achieved through auctioning RUs at between $90/tCO2 and $135/tCO2, with a sequestration potential of 9GtCO2 (Cabral et al., 2019). Subsequent analysis has found that incentivising CDR through RUs could mean that lower levels of carbon taxation are needed to meet the Paris Agreement, which in turn lowers electricity costs (Daggash and Mac Dowell, 2019). Value pool analysis of business models for BECCS, DACs and Distributed Biomass supports these findings, concluding that access to carbon credit mechanisms, has by far the greatest near-term potential to drive negative emissions technologies (Platt et al., 2018).
2.2. Tradable obligation schemes
Governments can place obligations or standards on companies to reduce their emissions directly or trade with other companies to offset their emissions, though this is less common. For example, the US Renewable Portfolio Standards require that a specified percentage of the electricity utilities sell comes from renewable resources. Under the Swiss CO2 Act, importers of motor fuels are required to offset up to 90% of their emissions. In support of the obligation, the Foundation for Climate Protection and Carbon Offset (KliK) was founded as a sector-wide carbon offset grouping for motor fuel suppliers. The KliK Foundation funds projects that generate carbon offset credits based on a Swiss carbon standard, which could include forestry, through a levy placed on consumers (st1, 2021). California's Low-Carbon Fuel Standard (LCFS) sets annual carbon intensity benchmarks on the full lifecycle emissions of transport fuels sold, supplied or offered for sale in California. Projects that directly capture CO2 from the air can generate LCFS credits and be sold to buyers who exceed their carbon intensity benchmark (Townsend and Havercroft, 2019). Operators must agree to monitor the project for 100 years after injection, demonstrate a low probability (< 10%) of leakage with 8–16.4% of credits allocated to a buffer account. Funds must also be ring fenced for maintenance, project closure, and potential accidents (CARB, 2018). Projects are not restricted to California and may be located anywhere.
In a Carbon Take Back Obligation (CTBO), currently at a conceptual stage, producers or importers of carbon compounds must sequester/store an increasing fraction of the CO2 produced from their products by acquiring RUs in-house or externally (100% by 2050) (Allen et al., 2009; Kuijper et al., 2021). Jenkins et al. (2021) find a CTBO could be comparable in cost, including economic costs, to similar ambition scenarios dominated by demand-side measures simulated by a global carbon price. In the near term, demand-side policy is required to incentivise emissions reductions before the CTBO stored fraction increases significantly enough to disincentive fossil fuel use (Jenkins et al., 2021).
2.3. Results-based payments
Governments can also use mechanisms to procure a fixed level of demand or price per tonne of CDR in an existing mechanism. Australia's Emissions Reduction Fund (ERF) provides incentives to businesses to cut the amount of greenhouse gases they create and to undertake activities that store carbon. RUs can be sold to the government through a carbon abatement contract or in the unregulated secondary market as an offset (Inoplex, 2018). RUs are secured through a competitive bidding process based on lowest cost per tCO2 (Clean Energy Regulator, 2022). The UK's Woodland Carbon Guarantee (WCG) allows landowners, who can also participate in the WCC, to sell captured carbon from their forests to the government in the form of verified RUs for a guaranteed price every 5 or 10 years up to 2055/56 (Forestry Commission, 2020, 2021). At years 5 and 15 the UK Accreditation Service checks if the predicted or actual carbon sequestration is materially correct (WCC, 2019c).
SDE++ in the Netherlands covers the cost of CCS operation above the EU ETS price and exempts industrial facilities from the Dutch carbon tax for CO2 captured and stored. Projects are ranked based on their subsidy intensity and only projects below the cost threshold for CCS could participate in the auction. Calculating the subsidy intensity is done by taking the base rate from the application and subtracting the long-term price divided by the emission factor (Andreas, 2021). Between 2026 and 2040, the Swedish government will use a reverse auction (SRA) to distribute SEK 400 million per year to BECCS operators who submit the lowest cost bids (Regeringen, 2021). Zetterberg et al. (2021) proposes a similar auction approach focused on guarantees, followed by a larger quota obligation scheme or integration of BECCS into the EU ETS to scale up BECCS in Sweden. They also considered a voluntary compensation scheme or international transfers of BECCS outcomes, but conclude these two approaches were too uncertain for the short term (Zetterberg et al., 2021). Honegger and Reiner (2018) also consider international transfers of outcomes through the Sustainable Development Mechanisms (SDM), which allow for voluntary transfers of mitigation units in exchange for payment of a price per tonne of CO2 avoided emissions or removal by the receiving country. The received units might then be counted towards the buyer country's mitigation target or its climate finance pledges (Honegger and Reiner, 2018).
A CfD is a contract that pays the differences in the market price and an agreed price or cost for CDR. Conceptual policy analysis suggests funding for BECCS in the UK from CfD mechanisms on electricity pricing (CfDe), with a Negative Emissions Payment (NEP) and a standalone carbon CfD (CfDc) on the ETS price, with NEPs set at £92/tCO2 and CfDc set at £107/tCO2 (Element Energy and Vivid Economics, 2021). The state could recoup NEP costs by selling a NEP on the voluntary market, or the plant operator could sell a NEP at a discount (£40/tCO2) on the voluntary market, with the state covering the difference. Europe's Innovation Fund, funded through allowances it sells in the EU ETS, could hypothetically provide support to projects through competitive tendering mechanisms such as CfDcs, but may be limited to second, or third of a kind projects (European Commission, 2021b). CfDcs may raise problems for an ETS forward market, effectively reducing the need for market participants to hedge their risks leading to reduced overall liquidity and thus less efficient price-formation (Europex, 2021).
2.4. Subsidies
Subsidies can involve the direct payment by governments to CDR project operators for the costs they incur. The UK's Countryside Stewardship Scheme (CSS) and Woodland Carbon Fund (WCF), New Zealand's One Billion Trees Fund (1BTF) and Ireland's Afforestation Scheme (IAS) offer direct grants paid per hectare (DEFRA, 2021b; MPI, 2021; Rural Payments Agency, 2021; Department of Agriculture Food and the Marine, 2022). IAS also covers the cost of afforestation. Mexico's Sowing Life (SL), intended to help meet climate goals with synergistic aims to support the country's efforts to reduce poverty and inequality, provides direct grants paid per farmer rather than per hectare. The US Conservation Reserve Program (CRP) operates on both a cost-share assistance basis (up to 50%) and financial incentives (additional 20% of soil rental rate) (Newton, 2020). Each mechanism can fund forestry, CSS and CRP consider soil restoration too, but only the WCF and 1BTF focus on carbon sequestration. Participants in the 1BTF can register their forests for carbon credits in the NZ ETS and therefore must adhere to its liability mechanism (Menzies, 2020). The proposed Environmental Land Management Scheme (ELMS) in the UK could fund some forms of soil carbon sequestration, biochar, and enhanced weathering (DEFRA, 2021a). The Climate-Smart Practice Incentive aims to increase sequestration through trees, permanent grasses and wetland restoration under the CRP although a funding mechanism is not yet established (USDA, 2021). California's Healthy Soils Program (HSP) is funded from the State's cap and trade proceeds. The HSP distributes funds through grants for: cover cropping, no-till, reduced-till, mulching, compost application, and conservation plantings (CDFA, 2022). The Regional Direct Air Capture Hubs (RDH) program in the USA will support four large-scale, regional direct air capture hubs that each comprise a network of CDR projects worth $3.5 billion (USDOE, 2022). Costa Rica's Payments for Environmental Services Program (PES) provides landowners with direct payments for the environmental services that their lands produce when adopting sustainable land-use and forest-management techniques. Funding comes from a variety of sources including fuel tax and water charge, Certificates of Conservation of Biodiversity, carbon credits, and strategic alliances with the public and private sector (UN Climate Change, 2020). Through its international climate and forest initiative (NICFI), the Norwegian government seeks to promote efforts to slow, halt, and ultimately reduce greenhouse gas emissions caused by deforestation and forest degradation in developing countries (REDD+) (Norad, 2020).
2.5. Tax credits
Tax credits reduce the amount of income tax companies operating CDR owe to governments. Section 45Q of the US tax code offers a tax credit for geologic CO2 storage in general, and so both BECCS and Direct Air Carbon Capture and Storage (DACCS) are eligible (Bright, 2021). The credit is based on the amount of CO2 captured and is provided to the company that captures it. CO2 must either be placed in permanent geological storage or utilised in products (e.g., plastics and cement), as well as enhanced oil recovery (EOR). CDR projects are likely to require higher financial incentives than CCS projects involving high-purity CO2 capture or EOR. The taxpayer has to repay the tax credit (credit recapture) to the Treasury if the carbon dioxide ceases to be captured, disposed of, or used in a qualifying manner (CRS, 2021). The Inflation Reduction Act of 2022 increased the 45Q tax credit to between $85/tCO2 and $180/tCO2 for geological CDR (Clean Air Task Force, 2022). For DAC facilities, the CO2 capture threshold decreased from 100,000 tonnes captured per year to 1,000 tonnes per year. Sanchez et al. (2018) estimate that a $60/tCO2 sequestration credit (e.g., the 45Q) may result in the sequestration of 30 MtCO2, while a $90/tCO2 carbon abatement credit (e.g., LCFS in Section 2.1) might incentivise the reduction of 38 MtCO2 (Sanchez et al., 2018). Edwards and Celia (2018) estimate that the use of direct government financing in addition to the 45Q tax credit could lead to capturing an additional 19–30 MtCO2 profitably each year (Edwards and Celia, 2018).
Vivid Economics (2019) propose a similar tax credit for the UK, potentially funded by a carbon levy. They also suggest the tax credit could apply to the initial capital investment (similar to the US 48 a/b tax code) and be tradeable between companies (Vivid Economics, 2019). Canada recently proposed a CCUS tax credit offered on the cost of purchasing or installing eligible equipment used in a CCUS project. The credit works on an initial sliding scale of 60% for equipment on a direct air capture project, 50% for other eligible capture equipment and 37.5% for transport, storage and use equipment (Johnson et al., 2022). Liability for released CO2 is expected to be managed through a recovery of tax credit mechanism (EY, 2022).
2.6. Costs and scale by mechanism type
This section illustrates the price and potential scale for each mechanism described in Sections 2.1–2.5 where possible. For some market-based mechanisms the level of CDR is not reported and the theoretical potential is used i.e., the maximum amount of CDR that could receive a payment in that market.
Figure 2 shows the range of average prices per tonne of CO2 available in each mechanism by CDR type, as well as the potential scale for each mechanism. Supplementary Table A1 presents the prices and scale for each of the mechanisms assessed. Mechanisms without either a price or scale reported which could not be plotted are available in the Appendix.
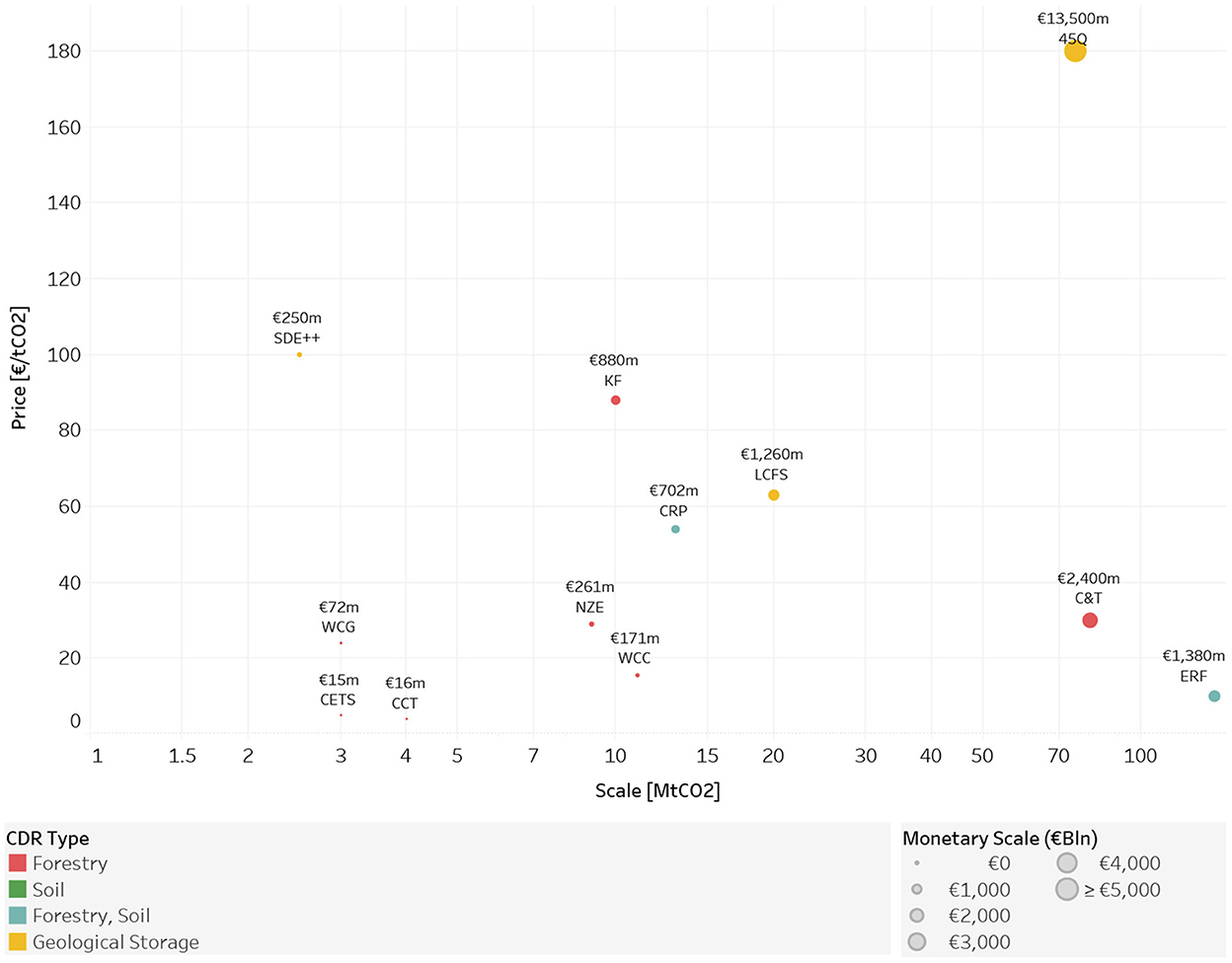
Figure 2. Current potential scale and price of mechanisms by CDR type (converted from base currency to € in September 2022). Carbon pricing and markets: WCC, Woodland Carbon Code; C&T, California Cap and Trade; NZE, New Zealand ETS; LBC, Label Bas Carbone; RHC, Registro de Huella de Carbono; CCT, Colombia's Carbon Tax; CETS, China ETS; QC, Québec Cap-and-Trade System; KE, Korean ETS; KZE, Kazakhstan ETS; SETS, Saitama's ETS; COETS, Colombia ETS. Tradeable obligation scheme: LCFS, Low-Carbon Fuel Standard; KF, Klik foundation; CTBO, Carbon Take Back Obligation. Results-based payments: WCG, Woodland Carbon Guarantee; ERF, Emissions Reductions Fund; CfD, Contract for Difference; SRA, Swedish Reverse Auction. Subsidy: CSS, Countryside Stewardship Scheme; WCF, Woodland Carbon Fund; 1BTF, One Billion Trees Fund; CRP, Conservation Reserve Program; HSP, CDFA Healthy Soils Program; SL, Sowing Life; IAS, Ireland's Afforestation Scheme; PES, Costa Rica's; NICFI, Norway's; ELMS, Environment Land Management Scheme; RDH, Regional Direct Air Capture Hubs. Tax credit: 45Q, 48a/b tax code, Canada Tax Credit.
Current prices for forestry can come close to or exceed cost estimates of €40/tCO2 in KF, WCG, and CRP, however upper estimates by the IPCC can rise to €240/tCO2 (Fuss et al., 2018; IPCC, 2022). The remaining mechanisms, which comprise the majority of biological CDR's total scale, are estimated to pay below €20/tCO2. IPCC cost estimates of DACCS and BECCS which can rise to €300/tCO2 and €400/tCO2 in cost, respectively are above current prices in all mechanisms. Policy documents for BECCS in the UK indicate a price of between €107/tCO2 and €151/tCO2 (Element Energy and Vivid Economics, 2021). Spot prices for DACCS today can rise to between €204/tCO2 and €2,132/tCO2 for voluntary purchases (cdr.fyi, 2022). However, voluntary markets have had an average price of €4/tCO2 in 2021, which is also below the costs of these technologies (Ecosystem Marketplace, 2022). Contracts with better terms are possible outside of the voluntary market. In these instances, the purchaser of credits is typically a large corporation or consortia (e.g., Microsoft, Airbus and Frontier) placing a low volume prepurchase of CDR based on a maximum spend (Joppa et al., 2021; 1PointFive, 2022; Frontier Climate, 2022). Reforms to the 45Q result in prices for DAC and BECCS of €180/tCO2 and €85/tCO2, respectively. SDE++ may incentivise geological storage but could be too low to support removal. The LCFS maintained a price of ~€200/tCO2 in 2019 and 2020 until recently declining to €68/tCO2 in September 2022. The price decline arose from a sharp rise in new credits from renewable gas projects due to a combination of interlocking incentives (Blackburn, 2022). It is possible to combine mechanisms to achieve higher prices to cover CDR costs. For example, the LCFS and 45Q, NZE and 1BTF, the WCF and CSS with the WCC. Generally, subsidies can be combined with other mechanisms.
The theoretical potential scale of operational mechanisms which support geological storage of CO2 in this survey is estimated at 0.95 GtCO2 in total, the total scale of operational mechanisms supporting biological storage is estimated at 0.273 GtCO2. These numbers represent the potential scale of mechanisms for which estimates could be established, not how much CO2 is currently being removed and stored.
IPCC scenarios which keep temperatures at 1.5C with low overshoot indicate annual volumes in 2050 for geological storage total 2.77 GtCO2 yr−1, with 2.75 (0.52–9.45) GtCO2 yr−1 coming from BECCS and 0.02 (0–1.74) GtCO2 yr−1 from DACCS. Net CO2 removal on managed land (including forestry) is estimated at 2.98 (0.23–6.38) GtCO2 yr−1 (IPCC, 2022). IEA estimates are lower for geological storage of 0.027 GtCO2 yr−1 by 2030 and 1.93 GtCO2 yr−1 by 2050, through 0.2 GtCO2 yr−1 of BECCS by 2030 and 1.3 GtCO2 yr−1 by 2050 and DACCS at 0.07 GtCO2 yr−1 and 0.63 GtCO2 yr−1, respectively (IEA, 2021). A significant proportion of biological CDR is not covered by mechanisms today, it is therefore difficult to compare it with future biological CDR requirements in scenarios. However, geological CDR is considerably underdeveloped relative to future requirements.
Figure 3 indicates that the majority of currently operational mechanisms are concentrated in carbon markets and subsidies which mostly support biological CDR. Average prices in carbon pricing and market mechanisms tend to be lower than other mechanisms. One reason for this is that prices are set independently of the cost of CDR and can reflect the cost of allowances targeting mitigation. Higher prices for CDR are generally offered in the remaining mechanism types as they can be tied towards the cost of CDR, excluding the LCFS and ERF.
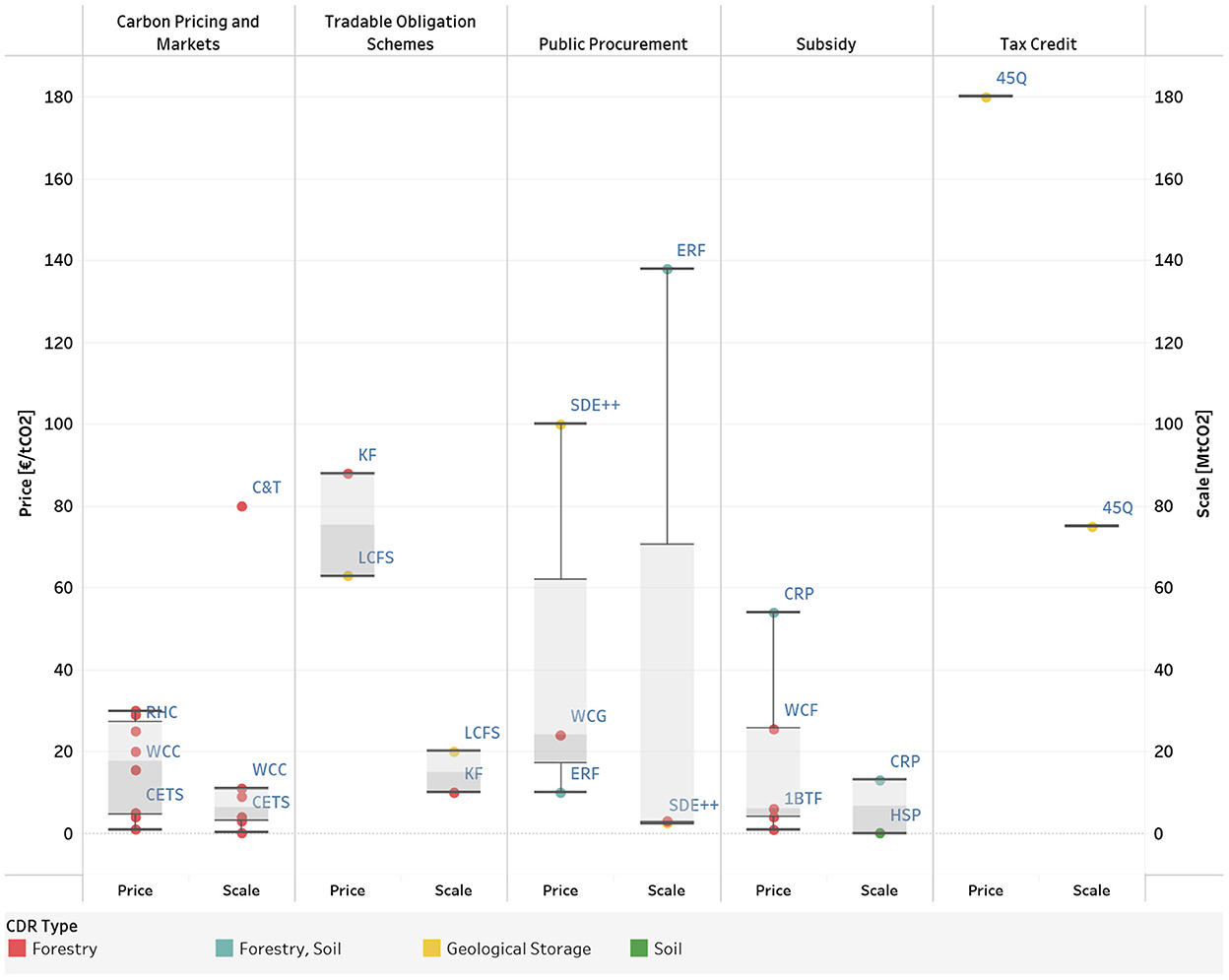
Figure 3. Current potential scale and price by mechanism type (converted from base currency to € in September 2022).
With the exception of the WCC, NZE, ERF and KF, the estimates of scale for carbon pricing and markets and tradeable obligation mechanisms represent their potential scale. Potential scale represents the maximum amount of RUs that could theoretically be operating in the mechanism, reporting on the values of CDR in these mechanisms is limited. 45Q's scale includes several projects that will have received varying prices, but 45Q in Figure 3 indicates the greatest price that could be obtained through that mechanism. For all other mechanisms and mechanism types the values for scale are either reported or estimated levels of direct CDR activity. The approached used to generate estimates is outlined in the Appendix. One implication of overlapping mechanisms is that the mechanism scales in Figure 3 are not necessarily additive.
3. Conclusion
Mechanisms that support CDR are important to scale up and deliver the removal capacity required in most net zero modelling scenarios. This review surveys current and proposed commercialisation mechanisms for CDR globally. We estimate each mechanism's payment per tonne of CO2 removed today and scale of removal, then categorise mechanisms based on how they distribute costs between the public and private sectors. The results presented in this paper are subject to the uncertainty inherent to the data and assumptions used to estimate prices and scale. It is possible that not all mechanisms that focus on afforestation, agricultural CDR and conservation have been captured. Using web-based searches for mechanisms is the main source of this limitation, with mechanism details being published in languages outside of the search terms used.
With minor exceptions the cost of both biological and geological CDR generally exceeds the funding that is available under existing support mechanisms, particularly for market-based mechanisms. We find the majority of mechanisms currently in operation are under-resourced and pay too little to ensure sufficient development of CDR capacity compatible with net zero scenarios. Mechanisms tend to promote established practises like afforestation and soil carbon sequestration and are not primarily motivated by CO2 removal. Existing mechanisms explicitly focused on carbon removal are geographically concentrated in the UK, USA (mostly California) and New Zealand. There are multiple barriers, of which lack of demand for CDR is one. The policy regime must address them all and that may require a suite of interventions (Zhou et al., 2022).
As fiscal space potentially tightens, policymakers that are considering the results of this research in the current economic climate may think about making greater use of markets and tradable obligation schemes to allocate a greater portion of the cost on the polluter. However, these mechanisms can increase the risk of investment creating further barriers that the certainty of contracts (e.g., CfD, feed-in tariffs) would overcome. Policymakers could also mandate firms to remove an increasing fraction of their CO2 over time, if the cost of geological CDR per tonne of CO2 remains too expensive.
There is a broad spectrum of CDR techniques, and a variety of support mechanisms may be needed to reflect their differences. Many CDR techniques are in the early stages of development and may require more immediate types of support before progressing to the longer-term mechanisms analysed in this review. For example, there could be a progression from subsidies to results-based mechanisms. Larger mechanisms may emerge once CDR techniques reach a suitable level of maturity and scale, but in order to obtain investment, CDR projects are likely to need clarity on those longer-term larger mechanisms today.
CDR is not a substitute for emissions reductions. However, like other forms of carbon offsets, incentives for CDR can have negative impacts on the level of emissions reductions and increase the uncertainty over whether an emissions target has been reached (Haya et al., 2020). Direct inclusion of CDR through RUs in existing mechanisms can also still be limited in scaling CDR deployment. For example, low and volatile carbon prices, liability risks for CDR, and questions of public acceptability if emissions don't appear to be declining could all be factors limiting CDR inclusion in carbon markets (Hickey et al., 2022). Legislation on the neutral reporting of biomass in carbon markets may also limit the potential of BECCS (Rickels et al., 2021). Mechanisms for CDR will need effective protocols and oversight to mitigate these uncertainties and unintended consequences. Focusing on near term climate action with clear plans to achieve it will be fundamental. The plan should prioritise emissions reductions and define a clear role for CDR in a net zero target. Policies should be required to prove that they will be able to deliver a required level of geological or biological CDR in suitable timelines. Emissions should be offset with like-for-like CDR, with CO2 from fossil fuel sources being offset with geological storage. Robust lifecycle accounting and monitoring for CDR will also support the certainty of achieving climate targets.
Author contributions
CH conceptualised the research with contributions from MA, SF, and SS. CH drafted the manuscript and gathered the data. All authors revised and improved the manuscript. All authors contributed to the article and approved the submitted version.
Funding
This project has received funding from the European Union's Horizon 2020 Research and Innovation Program under grant agreement No. 869192 (NEGEM). We also acknowledge the support of Oxford Net Zero through the University of Oxford's Strategic Research Fund and CO2RE funded by UKRI (grant reference NE/V013106/1).
Acknowledgments
We are grateful for comments from Mirte Boot, David Reiner, Keith Whiriskey, and Nijia Zhou.
Conflict of interest
The authors declare that the research was conducted in the absence of any commercial or financial relationships that could be construed as a potential conflict of interest.
Publisher's note
All claims expressed in this article are solely those of the authors and do not necessarily represent those of their affiliated organizations, or those of the publisher, the editors and the reviewers. Any product that may be evaluated in this article, or claim that may be made by its manufacturer, is not guaranteed or endorsed by the publisher.
Supplementary material
The Supplementary Material for this article can be found online at: https://www.frontiersin.org/articles/10.3389/fclim.2022.1101525/full#supplementary-material
Footnotes
1. ^Known as Air Resource Board offset credits in the C&T, China Certified Emission Reductions in the CETS, CO2 Removal Credits in Rickels et al. (2021), negative emission credits in Cabral et al. (2019), Australian carbon credit units or carbon abatement contracts in the ERF, Woodland Carbon Units in the WCG, New Zealand Units in (NZE), negative emissions payment (NEPs) in Element Economics and Vivid Economics.
References
1PointFive (2022). 1PointFive Announces Agreement With Airbus for the Purchase of 400,000 Tonnes of Carbon Removal Credits. Available online at: https://www.oxy.com/news/news-releases/1pointfive-announces-agreement-with-airbus-for-the-purchase-of-400000-tonnes-of-carbon-removal-credits/
Alcalde, J., Flude, S., Wilkinson, M., Johnson, G., Edlmann, K., Bond, C. E., et al. (2018). Estimating geological CO2 storage security to deliver on climate mitigation. Nat. Commun. 9, 1–13. doi: 10.1038/s41467-018-04423-1
Allen, M. R., Frame, D. J., and Mason, C. F. (2009). The case for mandatory sequestration. Nat. Geosci. 2, 813–814. doi: 10.1038/ngeo709
Andreas, J. (2021). The Industrial CCS Support Framework in the Netherlands. Available online at: https://network.bellona.org/content/uploads/sites/3/2021/07/The-Industrial-CCS-Support-Framework-in-the-Netherlands-1.pdf
ARB (2021). California's Compliance Offset Program. Available online at: https://ww2.arb.ca.gov/sites/default/files/2021-10/nc-forest_offset_faq_20211027.pdf
Bednar, J., Obersteiner, M., Baklanov, A., Thomson, M., Wagner, F., Geden, O., et al. (2021). Operationalizing the net-negative carbon economy. Nature 596, 1–7. doi: 10.1038/s41586-021-03723-9
Blackburn, E. (2022). Falling LCFS Credit Price Narrows RNG Prospects. Argus Media. Available online at: https://www.argusmedia.com/en/news/2333804-falling-lcfs-credit-price-narrows-rng-prospects
Bright, M. (2021). 45Q: The “Most Progressive CCS-Specific Incentive Globally” Is Now Open for Business - Global CCS Institute. Available online at: https://www.globalccsinstitute.com/news-media/insights/45q-the-most-progressive-ccs-specific-incentive-globally-is-now-open-for-business/
Cabral, R. P., Bui, M., and Mac Dowell, N. (2019). A synergistic approach for the simultaneous decarbonisation of power and industry via bioenergy with carbon capture and storage (BECCS). Int. J. Greenh. Gas Control 87, 221–237 doi: 10.1016/j.ijggc.2019.05.020
CARB (2018). Carbon Capture and Sequestration Protocol Under the Low Carbon Fuel Standard. Available online at: https://ww2.arb.ca.gov/sites/default/files/2020-03/CCS_Protocol_Under_LCFS_8-13-18_ada.pdf
CARB (2021). Compliance Offset Program. California Air Resources Board. Available online at: https://ww2.arb.ca.gov/our-work/programs/compliance-offset-program/about
CDFA (2022). CDFA Healthy Soils Program. Available online at: https://www.cdfa.ca.gov/oefi/healthysoils/docs/HSP_flyer_2022.pdf
cdr.fyi (2022). cdr.fyi Methodology. Available online at: https://www.cdr.fyi/
Cevallos, G., Grimault, J., and Bellassen, V. (2019). Domestic carbon standards in Europe overview and perspectives.
Clean Air Task Force (2022). Carbon Capture Provisions in the Inflation Reduction Act of 2022 45Q Enhancements in the Inflation Reduction Act. Available online at: https://cdn.catf.us/wp-content/uploads/2022/08/19102026/carbon-capture-provisions-ira.pdf
Clean Energy Regulator (2022). ERF Auction April 2022. Available online at: https://www.cleanenergyregulator.gov.au/ERF/auctions-results/april-2022
CRS (2021). The Tax Credit for Carbon Sequestration (Section 45Q). Available online at: https://crsreports.congress.gov
Daggash, H. A., and Mac Dowell, N. (2019). Higher carbon prices on emissions alone will not deliver the Paris agreement. Joule 3, 2120–2133. doi: 10.1016/j.joule.2019.08.008
DEFRA (2021a). Environmental Land Management Schemes: Overview. GOV.UK. Available online at: https://www.gov.uk/government/publications/environmental-land-management-schemes-overview
DEFRA (2021b). Woodland Carbon Fund. Available online at: https://www.gov.uk/guidance/woodland-carbon-fund
Department of Agriculture Food and the Marine (2022). Forestry Grants and Schemes. Available online at: https://www.gov.ie/en/publication/e384e-forestry-grants-and-schemes/
Ecosystem Marketplace (2022). Ecosystem Marketplace's State of the Voluntary Carbon Markets 2022 Q3. Available online at: https://www.ecosystemmarketplace.com/publications/state-of-the-voluntary-carbon-markets-2022/
EDF (2015). Kazakhstan: An Emissions Trading Case Study. Available online at: https://www.edf.org/sites/default/files/kazakhstan-case-study-may2015.pdf
EDF (2020). Analytical Report on the Status of the China GHG Voluntary Emission Reduction Program. Available online at: https://www.edf.org/sites/default/files/documents/Analytical-Report_on_the_Status_of_the_China_GHG_Voluntary_Emission_Reduction_Program-ENG.pdf
Edwards, R. W. J., and Celia, M. A. (2018). Infrastructure to enable deployment of carbon capture, utilization, and storage in the United States. Proc. Natl. Acad. Sci. U. S. A. 115, E8815–E8824. doi: 10.1073/pnas.1806504115
Element Energy and Vivid Economics (2021). Investable Commercial Frameworks for Power BECCS Prepared by Element Energy and Vivid Economics. Available online at: https://assets.publishing.service.gov.uk/government/uploads/system/uploads/attachment_data/file/1026637/investable-commercial-framework-power-beccs.pdf
European Commission (2021a). Communication From the Commission to the European Parliament and the Council on Sustainable Carbon Cycles. Available online at: https://ec.europa.eu/transparency/documents-register/detail?ref=COM(2021)800&lang=en
European Commission (2021b). Revised EU ETS With Annex. Available online at: https://ec.europa.eu/info/sites/default/files/revision-eu-ets_with-annex_en_0.pdf
Europex (2021). Carbon Contracts for Difference (CCfDs) and Their Potentially Distortive Effects on Emission Markets: Call for a Comprehensive Impact Assessment. Available online at: https://www.europex.org/wp-content/uploads/2021/05/20210531_Europex-position-paper-on-CCfDs.pdf
EY (2022). EY Tax Alert 2022 no 41 - Proposed Federal Investment Tax Credit for Carbon Capture, Utilization and Storage — Update. Available online at: https://www.eylaw.ca/en_ca/newsroom/2022/tax-alert-2022-no-41
Fankhauser, S., Smith, S. M., Allen, M., Axelsson, K., Hale, T., Hepburn, C., et al. (2021). The meaning of net zero and how to get it right. Nat. Clim. Change 12, 1–7. doi: 10.1038/s41558-021-01245-w
Forestry Commission (2020). Woodland Carbon Guarantee. GOV.UK. Available online at: https://www.gov.uk/guidance/woodland-carbon-guarantee
Forestry Commission (2021). Guide to the Fourth Auction. Available online at: https://wcag.naturebid.org.uk/
Fridahl, M., Bellamy, R., Hansson, A., and Haikola, S. (2020). Mapping multi-level policy incentives for bioenergy with carbon capture and storage in Sweden. Front. Clim. 2, 604787. doi: 10.3389/fclim.2020.604787
Frontier Climate (2022). How Frontier Works. Available online at: https://frontierclimate.com/
Fuss, S., Lamb, W. F., Callaghan, M. W., Hilaire, J., Creutzig, F., Amann, T., et al. (2018). Negative emissions—Part 2: costs, potentials and side effects. Environ. Res. Lett. 13, 063002. doi: 10.1088/1748-9326/aabf9f
Haya, B., Cullenward, D., Strong, A. L., Grubert, E., Heilmayr, R., Sivas, D. A., et al. (2020). Managing uncertainty in carbon offsets: insights from California's standardized approach. Clim Policy 20, 1112–1126. doi: 10.1080/14693062.2020.1781035
Hickey, C., and Allen, M. R. (2021). Quantitative Survey of Commercialisation Mechanisms. Available online at: https://www.negemproject.eu/wp-content/uploads/2021/12/D-2.1-Quantitative-survey-of-commercialisation.pdf
Hickey, C., Dodab, B., Fankhauser, S., Fischer, C., Fuss, S., Macinante, J., et al. (2022). Carbon dioxide removal in emissions trading schemes.
Honegger, M., Poralla, M., Michaelowa, A., and Ahonen, H. M. (2021). Who Is Paying for Carbon Dioxide Removal? Designing policy instruments for mobilizing negative emissions technologies. Front. Clim. 3, 672996. doi: 10.3389/fclim.2021.672996
Honegger, M., and Reiner, D. (2018). Climate Policy. The Political Economy of Negative Emissions Technologies: Consequences for International Policy Design. Available online at: https://www.tandfonline.com/action/journalInformation?journalCode=tcpo20
ICAP (2021a). Canada-Québec Cap-and-Trade System General Information. Available online at: https://icapcarbonaction.com/en/?option=com_etsmap&task=export&format=pdf&layout=list&systems%5B%5D=73
ICAP (2021b). Chile ETS. Available online at: https://icapcarbonaction.com/en/?option=com_etsmap&task=export&format=pdf&layout=list&systems[]=54
ICAP (2021c). Kazakhstan Emissions Trading Scheme General Information. Available online at: https://icapcarbonaction.com/en/?option=com_etsmap&task=export&format=pdf&layout=list&systems%5B%5D=46
ICAP (2022a). Japan - Saitama Target Setting Emissions Trading System. International Carbon Action Partnership. Available online at: https://icapcarbonaction.com/en/ets/japan-saitama-target-setting-emissions-trading-system
ICAP (2022b). New Zealand Emissions Trading Scheme General Information ETS Description. Available online at: https://icapcarbonaction.com/system/files/ets_pdfs/icap-etsmap-factsheet-48.pdf
IEA (2021). Net Zero by 2050 - A Roadmap for the Global Energy Sector. Available online at: https://iea.blob.core.windows.net/assets/deebef5d-0c34-4539-9d0c-10b13d840027/NetZeroby2050-ARoadmapfortheGlobalEnergySector_CORR.pdf
Inoplex (2018). What is the Emissions Reduction Fund? Environmentally Sustainable Solutions | Lowering Carbon Emissions. Available online at: https://www.inoplex.com.au/information/what-is-the-emissions-reduction-fund
IPCC (2022). Working Group III Contribution to the IPCC Sixth Assessment Report (AR6). Available online at: https://report.ipcc.ch/ar6wg3/pdf/IPCC_AR6_WGIII_FinalDraft_FullReport.pdf
Jenkins, S., Mitchell-Larson, E., Ives, M. C., Haszeldine, S., and Allen, M. (2021). Upstream decarbonization through a carbon takeback obligation: an affordable backstop climate policy. Joule. 5, 2777–2796. doi: 10.1016/j.joule.2021.10.012
Johnson, G., McInerney, T., Gilmour, B., Sigalet, B., and Ciechanowski, P. (2022). Canadian Budget Proposes New Investment Tax Credit for Carbon Capture, Utilization and Storage. Bennett Jones. Available online at: https://www.bennettjones.com/Blogs-Section/Canadian-Budget-Proposes-New-Investment-Tax-Credit-For-Carbon-Capture-Utilization-and-Storage
Joppa, L., Luers, A., Willmott, E., Friedmann, S. J., Hamburg, S. P., and Broze, R. (2021). Microsoft's million-tonne CO2-removal purchase — lessons for net zero. Nature 597, 629–632. doi: 10.1038/d41586-021-02606-3
Kuijper, M., Holleman, E., and Paul van Soest, J. (2021). Carbon Takeback Obligation | A Producers Responsibility Scheme on the Way to a Climate Neutral Energy System Carbon Takeback Obligation. Available online at: www.gemeynt.nl
Menzies, C. (2020). One Billion Trees Programme - What Does It Mean for You?. Available online at: https://www.awslegal.co.nz/one-billion-trees-programme-what-does-it-mean-for-you/
MPI (2021). Direct Grant Funding Categories for Tree Planting. MPI| NZ Government. Available online at: https://www.mpi.govt.nz/forestry/funding-tree-planting-research/one-billion-trees-programme/direct-landowner-grants-from-the-one-billion-trees-fund/direct-grants-funding-categories-for-tree-planting/
Nemet, G. F., Callaghan, M. W., Creutzig, F., Fuss, S., Hartmann, J., Hilaire, J., et al. (2018). Negative emissions—Part 3: innovation and upscaling. Environ. Res. Lett. 13, 063003. doi: 10.1088/1748-9326/aabff4
Newton, J. (2020). Reviewing 2020 CRP Enrollment. Available online at: https://www.fb.org/market-intel/reviewing-2020-crp-enrollment1
Norad (2020). Climate and Forest Initiative Support Scheme. Available online at: https://www.norad.no/en/front/funding/climate-and-forest-initiative-support-scheme/
Platt, D., Workman, M., and Hall, S. (2018). A novel approach to assessing the commercial opportunities for greenhouse gas removal technology value chains: developing the case for a negative emissions credit in the UK. J. Clean. Prod. 203, 1003–1018 doi: 10.1016/j.jclepro.2018.08.291
Regeringen (2021). Nya och utökade satsningar på industrins gröna omställning. Available online at: https://www.regeringen.se/pressmeddelanden/2021/09/nya-och-utokade-satsningar-pa-industrins-grona-omstallning/
Rickels, W., Proel,ß, A., Geden, O., Burhenne, J., and Fridahl, M. (2021). Integrating carbon dioxide removal into european emissions trading. Front. Clim. 62, 690023. doi: 10.3389/fclim.2021.690023
Rural Payments Agency (2021). Countryside Stewardship Grants. GOV.UK. Available online at: https://www.gov.uk/countryside-stewardship-grants
Sanchez, D. L., Johnson, N., McCoy, S. T., Turner, P. A., and Mach, K. J. (2018). Near-term deployment of carbon capture and sequestration from biorefineries in the United States. Proc. Natl. Acad. Sci. U. S. A. 115, 4875–4880. doi: 10.1073/pnas.1719695115
Schenuit, F., Colvin, R., Fridahl, M., McMullin, B., Reisinger, A., Sanchez, D. L., et al. (2021). Carbon dioxide removal policy in the making: assessing developments in 9 OECD cases. Front. Clim. 3, 7. doi: 10.3389/fclim.2021.638805
Shankleman, J., and Rathi, A. (2021). Carbon Offsets: New $100 Billion Market Faces Disputes Over Trading Rules – Bloomberg. Bloombergy. Available online at: https://www.bloomberg.com/news/features/2021-06-02/carbon-offsets-new-100-billion-market-faces-disputes-over-trading-rules
st1 (2021). Analysis of the Market Demand Mechanisms and the Demand Potential for Land-Based Carbon Credits. Available online at: https://content.st1.fi/sites/default/files/2021-02/LIFE-CarbonFarmingScheme-MarketAnalysis-040221.pdf
Townsend, A., and Havercroft, I. (2019). The LCFS and CCS Protocol: An Overview for Policymakers and Project Developers. Available online at: https://www.globalccsinstitute.com/wp-content/uploads/2019/05/LCFS-and-CCS-Protocol_digital_version-2.pdf
UN Climate Change (2020). Payments for Environmental Services Program. Costa Rica: UNFCCC. Available online at: https://unfccc.int/climate-action/momentum-for-change/financing-for-climate-friendly-investment/payments-for-environmental-services-program
USDA (2021). USDA Expands and Renews Conservation Reserve Program in Effort to Boost Enrollment and Address Climate Change. Available online at: https://www.fsa.usda.gov/news-room/news-releases/2021/usda-expands-and-renews-conservation-reserve-program-in-effort-to-boost-enrollment-and-address-climate-change
USDOE (2022). Biden Administration Launches $3.5 Billion Program to Capture Carbon Pollution from the Air. Department of Energy. Available online at: https://www.energy.gov/articles/biden-administration-launches-35-billion-program-capture-carbon-pollution-air-0
Vivid Economics (2019). Greenhouse Gas Removal (GGR) Policy Options-Final Report. Available online at: https://www.vivideconomics.com/wp-content/uploads/2019/09/Greenhouse_Report_Gas_Removal_policy_options.pdf
WCC (2019a). Management of Risks and Permanence. UK Woodland Carbon Code. Available online at: https://woodlandcarboncode.org.uk/standard-and-guidance/2-project-governance/2-3-management-of-risks-and-permanence
WCC (2019b). UK Woodland Carbon Code. Available online at: https://woodlandcarboncode.org.uk/
WCC (2019c). Verification - Ongoing Check of Project Sequestration. UK Woodland Carbon Code. Available online at: https://woodlandcarboncode.org.uk/landowners-apply/4-verification-ongoing-check-of-project-sequestration
Zetterberg, L., Johnsson, F., and Möllersten, K. (2021). Incentivizing BECCS—A Swedish case study. Front. Clim. 99, 685227. doi: 10.3389/fclim.2021.685227
Zhou, N., Boot, M., Hickey, C., Fankhauser, S., Sen, A., and Smith, S. (2022). Policy Brief Deployment Support for Geological Greenhouse Gas Removals (GGR) in the UK. Available online at: https://www.smithschool.ox.ac.uk/sites/default/files/2022-06/Policy-brief-Deployment-support-for-geological-GGR-in-UK.pdf
Keywords: carbon dioxide removal, net zero, climate policy, business models, commercialisation
Citation: Hickey C, Fankhauser S, Smith SM and Allen M (2023) A review of commercialisation mechanisms for carbon dioxide removal. Front. Clim. 4:1101525. doi: 10.3389/fclim.2022.1101525
Received: 17 November 2022; Accepted: 28 December 2022;
Published: 18 January 2023.
Edited by:
Soheil Shayegh, European Institute of Economics and the Environment, ItalyReviewed by:
Asbjørn Torvanger, Centre for International Climate and Environmental Research (CICERO), NorwayUdayan Singh, Northwestern University, United States
Copyright © 2023 Hickey, Fankhauser, Smith and Allen. This is an open-access article distributed under the terms of the Creative Commons Attribution License (CC BY). The use, distribution or reproduction in other forums is permitted, provided the original author(s) and the copyright owner(s) are credited and that the original publication in this journal is cited, in accordance with accepted academic practice. No use, distribution or reproduction is permitted which does not comply with these terms.
*Correspondence: Conor Hickey, conor.hickey@ouce.ox.ac.uk