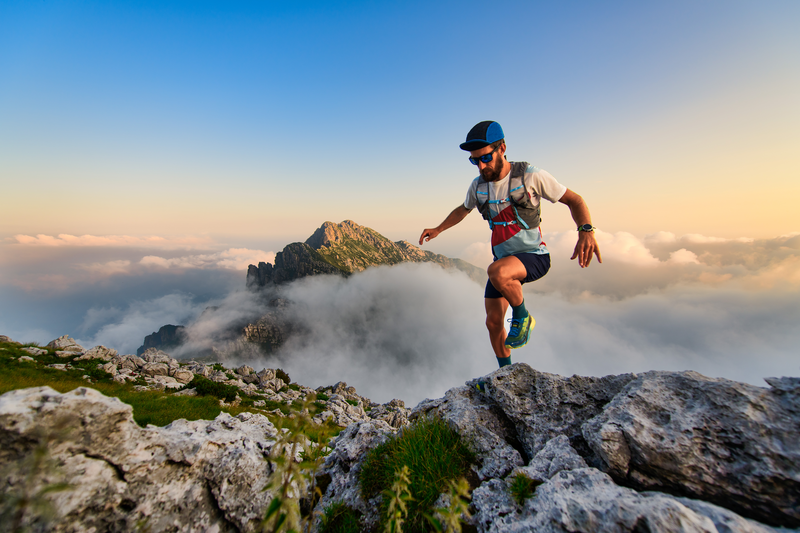
95% of researchers rate our articles as excellent or good
Learn more about the work of our research integrity team to safeguard the quality of each article we publish.
Find out more
REVIEW article
Front. Clim. , 28 October 2022
Sec. Predictions and Projections
Volume 4 - 2022 | https://doi.org/10.3389/fclim.2022.1014138
This article is part of the Research Topic Interactions among Climates of Ocean Basins View all 8 articles
Coherently coupled ocean-atmosphere variability of the tropical Indo-Pacific Oceans gives rise to the predictability of Asian summer climate. Recent advances in Indo-western Pacific Ocean capacitor (IPOC) theory and the relationship with El Niño-Southern Oscillation (ENSO) are reviewed. The IPOC features tropical Indian Ocean (TIO) warming and an anomalous anticyclonic circulation over the western North Pacific (WNPAAC), the latter driving water vapor transport to East Asia and causing extreme events, e.g., heavy rainfalls from central China to Japan during the boreal summer. IPOC events often occur in post-ENSO summers, but the significant TIO warming could sustain the WNPAAC without a strong El Niño, forced instead by a strong antecedent positive Indian Ocean Dipole (IOD). In latter cases, the Indian Ocean and WNP act as a self-sustaining system, independent of external forcings. El Niño or positive IOD induces the oceanic downwelling Rossby waves and thermocline warming in the southwest TIO, leading to SST warming and a “C-shaped” wind anomaly during winter and early spring. Furthermore, the southwest TIO downwelling Rossby waves reflect as oceanic Kelvin waves on the African coast. In the early summer, the resultant southeast TIO SST warming induces a second “C-shaped” wind anomaly. Both southwest and southeast TIO warming contribute to the WNPAAC. The WNPAAC modulates the water vapor pathways to East Asia in the late spring and summer, which mostly converge over the South China Sea and adjacent regions before flowing further to the north. More water vapor is transported from the western Pacific warm pool and less from the southern hemisphere and the Indian Ocean. The enhanced Asian Summer monsoon and moisture content lead to extreme rainfalls in central China and Japan during the boreal summer.
El Niño-Southern Oscillation (ENSO) is the largest interannual variability in the equatorial Pacific. It has been widely reported that ENSO affects the neighboring Indian Ocean (Klein et al., 1999; Alexander et al., 2002; Du et al., 2009; Yuan et al., 2013; Xie et al., 2016; Chen et al., 2019). The tropical Indian Ocean (TIO) variability was often viewed as a passive response to ENSO (Latif and Barnett, 1995). However, recent studies suggested that TIO could have its intrinsic variability and evolve independently of ENSO (e.g., Du et al., 2020; Zhang et al., 2020).
The Asian summer monsoon onset starts in May, with the strong cross-equatorial flow off Somali Peninsula. After that, the monsoonal winds eventually prevail in the north TIO and western North Pacific (WNP) (Wang and LinHo, 2002; Chen et al., 2021). The Asian monsoon brings a large amount of moisture to Asian landmass, especially for South Asia, East Asia, and Southeast Asia. The monsoonal rainfall is essential for agricultural activity in densely populated Asian landmass and affects people's livelihoods. It is of great societal importance and economic value to accurately predict the Asian summer monsoon (Ma et al., 2017).
Surface ocean thermal condition in the Indo-Pacific Oceans modulates large-scale atmospheric circulation and moisture transport associated with the Asian summer monsoon. The boreal summer SST warming in the north TIO, the anomalous anticyclonic circulation (AAC) over the WNP (WNPAAC), and SST cooling in the WNP during boreal spring and early summer are essential parts of an inter-basin climate mode called Indo-western Pacific Ocean capacitor (IPOC, Xie et al., 2016). In spring, the AAC and local SST coolings are coupled in the WNP trade winds regime, while in summer, the WNP trade winds regime collapses, and the AAC and north TIO warming are coupled in the summer monsoon regime as the AAC further extends westward toward the north TIO. IPOC is a self-sustained coupled mode. ENSO could set some favorable conditions, such as Indian Ocean SST warming or WNP SST cooling, to trigger this climate mode. Recent studies suggested that IPOC could be excited independently of ENSO (Kosaka et al., 2013; Takaya et al., 2020; Wang et al., 2020; Chen et al., 2021; Zhou et al., 2021). Kosaka et al. (2013) used a coupled climate model and designed an experiment to suppress the model's SST variability in the central and eastern Pacific. Their results showed that the IPOC could be excited by atmospheric/oceanic internal variability in the absence of Pacific ENSO forcing. Chen et al. (2021) showed that the southwest TIO SST warming alone could excite trans-basin coupling within the north TIO and WNP. As an important participant in the positive phase of IPOC, the AAC is the southern part of the positive Pacific-Japan/East Asian Pacific pattern (PJ/EAP; Nitta, 1987; Huang and Wu, 1989) that plays an essential role in East Asian summer climate. Thus, IPOC strongly affects East Asian summer rainfall and surface temperature (Hu et al., 2011, 2017; Chen et al., 2016, 2018, 2019; Ma et al., 2017; Cai et al., 2022).
The review of Xie et al. (2016) summarized recent progress in studying how the Indo-Pacific Oceans respond to remote ENSO forcing and affect climate over surrounding continents. Since then, new findings suggest that the intrinsic variability of the TIO could be essential in driving the Indo-Pacific climate even without ENSO. Complementary to the review by Xie et al. (2016), this paper updates recent studies on IPOC.
The paper is organized as follows. Section Summer conditions of the Indo-western Pacific describes the summer mean state of the Indo-western Pacific. Section ENSO-triggered IPOC shows the Indian Ocean Rossby wave dynamics and Indian Ocean basin-wide warming mode (IOB). Section IOD events without significant ENSO presents the Indian Ocean Dipole (IOD) events without significant ENSO. Section Influence on East Asia shows the influence of IPOC on East Asia. The final section is a summary and discussion.
The Indo-Pacific warm pool is characterized by sea surface temperature (SST) warmer than 28°C (Figure 1A). It is an important region to fuel the global hydrologic cycle: Intense atmospheric convection and tremendous latent heat release drive global atmospheric circulation (Yoneyama and Zhang, 2020). The Indo-Pacific warm pool changes seasonally in size, area, and location (Hu et al., 2015). In summer, most of the TIO is a part of the Indo-Pacific warm pool (Figure 1A). SST variability in the TIO could affect the climatic condition in situ and even in the western Pacific and East Asia (Chen et al., 2019; Basconcillo et al., 2021). The Walker Cell in the TIO features a strong westward pressure gradient that results in low-level westerlies with opposing high-level easterlies, ascending movement in the eastern TIO, and large-scale descending movement in the west near the East African coastline (Hirons and Turner, 2018).
Figure 1. The boreal early summer (May-Jun-July) climatology in the Indo-Pacific region during 1981-2020: (A) for SST (shading, °C) from HadISST (Rayner et al., 2003) and 10 meter wind (vector, m s−1) from ERA5 (Hersbach et al., 2020), (B) for rainfall (shading, mm day−1) from GPCP (Adler et al., 2003) and vertical integral of zonal and meridional water vapor flux (abbreviated as IVT, vector, kg m−1 s−1) from ERA5, and (C) for thermocline depth calculated as the depth of 20°C isotherm of sea water temperature (D20, shading, m) from GODAS reanalysis (Behringer et al., 1998).
There is a unique area in the southwest TIO where its thermocline is shallow, called the Seychelles dome (Figure 1C), facilitating in-situ surface warming/cooling via efficient thermocline feedback (Huang and Kinter, 2002; Xie et al., 2002; Izumo et al., 2008; Du et al., 2009; Chen et al., 2021). It is one of the regions in the TIO with large interannual variability and strong air-sea coupling. It is documented that the interannual variability within the southwest TIO could be attributed to oceanic Rossby waves originating from the eastern IO associated with ENSO (Izumo et al., 2008). Besides, the local effects, such as IOD, also play a role (Tozuka et al., 2010; Zhang et al., 2018).
The thermocline in the equatorial Indian ocean is flat and deep (Figure 1C). The influence of thermocline displacement on SST may be weak (Schott et al., 2009). However, TIO is strongly affected by the Asian monsoon (Figure 1A). The low-level wind direction changes seasonally over TIO. For example, the low-level winds change from northeasterly winds to southwesterly winds in the north TIO in May. As part of the Indo-Pacific warm pool, the mean SST in the southeast TIO is high during boreal early summer (Figure 1A), with small SST perturbation allowing to induce anomalous convection (Chen et al., 2018, 2019). In the monsoon seasons, the cross-equatorial winds near Sumatra and Java cause coastal upwelling and cool the SST in the southeast TIO (Figure 1A). It also shallows the thermocline in the eastern Indian Ocean and sets a favorable condition for the thermocline-SST feedback (Zheng et al., 2010).
After the Asian monsoon onset, the southwesterly monsoonal winds prevail in the north TIO around May, gradually establishing in the South China Sea and WNP (Chen et al., 2019). In June, the Pacific trade winds still reign the WNP region. The southwesterly winds substitute the trade winds in the late boreal summer. The monsoonal westerly winds and easterly trades meet over the WNP (Figure 1A). The prevailing westerly winds are thought to be a necessary condition of Indo-Pacific inter-basin coupling (Kosaka et al., 2013; Xie et al., 2016). Besides, Hu et al. (2019) investigated the effect of meanflow on the AAC via energetics analysis. Their results showed that the mean flow over the WNP plays a role in the AAC development in the post-El Niño summer. The conversion of kinetic energy from the confluent flow to perturbations contributes to the amplification of easterly wind anomalies in WNP, which in turn provide positive feedback onto the AAC through inducing surface Ekman divergence and suppressing in-situ convection (Hu et al., 2019). Based on the energetic perspective, a recent paper further points out the importance of mean flow to the intensification and north extension of WNPAAC in El Niño decaying mid-summer (Tang et al., 2022). The remarkable feature of the East Asian summer climate is a rainband extending from eastern China to Japan, called the Meiyu/Baiu (Sampe and Xie, 2010; Takaya et al., 2020). The moisture supporting Meiyu/Baiu rains comes from the Asian summer monsoon (Figure 1B). The following sections show that the SST variability in Indo-Pacific Oceans modulates large-scale monsoonal circulation and moisture transport.
ENSO is recognized as the most significant interannual variability of the climate system, which has a profound impact on the global climate through atmospheric and oceanic teleconnections throughout its lifetime (e.g., Alexander et al., 2002; Liu and Alexander, 2007; Yeh et al., 2018; Wang, 2019; Taschetto et al., 2020). ENSO strongly influences the interannual variability of the tropical Indian Ocean (TIO) (Figure 2; Klein et al., 1999; Guo et al., 2018).
Figure 2. Correlations with Nino3.4 SST in November-December-January (NDJ) for 1982/83-2020/21 from OISST (Reynolds et al., 2002) after removing linear trend and seasonal cycle. Thickened lines indicate confidence level >95% in students' t-test. TIO, tropical Indian Ocean; NTIO, north tropical Indian Ocean; SETIO, southeast tropical Indian Ocean; SWTIO, southwest Indian Ocean.
The ENSO-induced Walker Circulation anomalies can directly affect the SST of the TIO through cloud-radiation-SST and wind-evaporation-SST (WES) feedback. During El Niño events, the eastward shift of Walker Circulation leads to subsidence over the Indian Ocean, causing reduced cloudiness and anomalous anticyclone that enhance the downward shortwave radiation and reduce the upward latent heat flux, thus contributing to increasing SST in the TIO (Klein et al., 1999; Lau and Nath, 2003; Tokinaga and Tanimoto, 2004).
After the mature phase of El Niño, the TIO displays a basin-wide SST warming in the spring (Figure 2), referred to as the Indian Ocean basin mode (IOB). The IOB peaks in spring and persists into summer (Figure 2), extending the ENSO effect on East Asia through the IPOC effect, despite the dissipation of ENSO (Yang et al., 2007, 2010; Xie et al., 2009, 2016; Chowdary et al., 2011; Wang et al., 2018; Kosaka et al., 2021).
Over the southwest TIO (SWTIO), the climatological thermocline is shallow. Thus thermocline variability can strongly affect SST (Huang and Kinter, 2002; Xie et al., 2002; Vialard et al., 2009; Chen et al., 2021; Du et al., 2021). Previous studies found that ocean dynamics rather than surface heat fluxes dominate the SST variations on an interannual time scale (Chambers et al., 1999; Murtugudde and Busalacchi, 1999; Huang and Kinter, 2002; Xie et al., 2002; Vialard et al., 2009). In climatology, the SWTIO thermocline dome is maintained by the upwelling induced by negative wind stress curl between the weak equatorial westerlies and the southeasterly trades over the TIO (Hermes and Reason, 2008; Yokoi et al., 2008, 2012). The thermocline depth exhibits large variability, associated with the anomalous wind stress curl induced by ENSO (Masumoto and Meyers, 1998; Jury and Huang, 2004; Tozuka et al., 2010; Yang et al., 2019; Eabry et al., 2021; Wu et al., 2021). During the developing and mature phase of El Niño, the anomalous anticyclonic wind stress curls force downwelling oceanic Rossby waves in the southeast TIO, which propagate slowly westward and reach the SWTIO within one or two seasons (Figure 3). These planetary waves deepen the thermocline and warm the subsurface, causing SST warming through thermocline-SST feedback in the SWTIO (Figure 4; Xie et al., 2002). The slow-propagating oceanic Rossby waves sustain the SWTIO SST warming till the El Niño decaying summer (Huang and Kinter, 2002; Xie et al., 2002; Annamalai et al., 2005; Du et al., 2009; Guo et al., 2018; Chen et al., 2019; Figures 3, 4).
Figure 3. Monthly SSHa (shading, cm) and SSTa (contour, °C) averaged over 5°S−15°S regressed onto NDJ Niño 3.4 SST for 1993/94-2019/20. Stippling indicates confidence level >95% for SSHa based on students' t-test. Based on CMEMS SSH from 1993-2020 and OISST from 1982-2021, after removing the linear trend and seasonal cycle.
Figure 4. Regression of IAP potential temperature anomalies (shading, °C) averaged meridionally in 5S°-15S° in SON(0) (A), D(0)JF(1) (B), MAM(1) (C), and JJA(1) (D) upon the NDJ Niño3.4 SST from 1982-2021. Solid back lines show the climatological mean potential temperature for every 2°C with a thickened 20°C line. Gray dashed line indicates the mixed-layer depth. Stippling indicates confidence level >95% in students' t-test. Linear trends and seasonal cycles are removed.
The persistent SWTIO SST warming induces northerly wind anomalies across the equator. The anomalous northerly winds shift to northeasterly north and northwesterly south of the equator (Figure 5) due to the reverse of the Coriolis force. During winter and early spring, the “C-shaped” wind anomalies strengthen the mean northeasterly monsoon north and weaken the southeasterly wind south of the equator, enhancing the north-south SST gradient, which further induces the equatorially-antisymmetric convection and precipitation patterns (Figures 5B,C,F,G, 6B,C,F,G; Kawamura et al., 2001; Wu et al., 2008; Du et al., 2009, 2013b, 2021; Wu and Yeh, 2010; Chakravorty et al., 2013). Following the summer monsoon onset, the “C-shaped” wind anomalies counteract the climatological southwesterly monsoon in the north TIO, reduce surface evaporation and latent heat flux, and increase the SST, following wind-evaporation-SST (WES) feedback (Figures 5C,D,G,H, 6C,D,G,H; Xie and Philander, 1994; Xie, 1996; Du et al., 2009). The north TIO SST warming results in more moisture convergence and consequent rainfall over the windward side of the Western Ghats and south of Himalaya (Izumo et al., 2008; Chowdary et al., 2019).
Figure 5. Anomalies of monthly SST (shading, °C) and precipitation (contour, mm day−1) (A–D), and monthly SSH (shading, cm) and wind (vector, m s−1) (E–H) regressed onto NDJ Niño 3.4 SST. Red circle with arrows denotes WNP AAC in (F–H). Stippling indicates confidence level >95% for SSTa and SSHa based on students' t-test. Based on OISST from 1982-2021, GPCP precipitation from 1982-2021, CMEMS SSH from 1993-2020, and CCMP wind from 1988-2021. Linear trends and seasonal cycles are removed.
Figure 6. Anomalies of ERA5 monthly Qnet (sum of latent heat flux, sensible heat flux, shortwave radiation and longwave radiation, shading, W m−2) and shortwave plus longwave radiation (contour, W m−2) (A–D), and monthly latent heat flux (shading, W m−2) (E–H) regressed onto NDJ Niño 3.4 SST from 1982-2021. Positive means heat into the ocean. Stippling indicates a confidence level >95% for Qnet and latent heat flux based on students' t-test. Linear trends and seasonal cycles are removed.
Furthermore, the downwelling Rossby waves reach the western boundary, turn into coastal-trapped waves toward the equator, and transform into the equatorial downwelling Kelvin waves, propagating eastward (Jensen, 1993; Le Blanc and Boulanger, 2001; Chen et al., 2019; Zhang and Du, 2022). The downwelling Kelvin waves induce thermocline and SST warming in the east (Figures 5E–H).
The WNPAAC connects ENSO and the East Asian climate, usually forms before the El Niño mature phase and persists into the post-El Niño summer (Wang et al., 2000; Li and Wang, 2005; Zhou et al., 2014; Li et al., 2017). Several theories have been proposed to explain the formation and maintenance of the WNPAAC during different phases of ENSO (Wang et al., 2000, 2013; Wu et al., 2009, 2017a,b; Xie et al., 2009; Xiang et al., 2013; Stuecker et al., 2015). Wang et al. (2000) suggested that the local atmosphere-ocean interaction is the responsible mechanism before El Niño peak winter. They extended this theory to a self-sustained inter-basin coupled mode in the Indo-western Pacific to explain the maintenance of the WNPAAC during the decay phase of the El Niño event (Wang et al., 2013). Another theory named combination mode emphasizes non-linear interactions of atmospheric response to the slow evolution of equatorial central and eastern Pacific SST anomalies with the seasonal climatology in the rapid growth of WNPAAC (Stuecker et al., 2013, 2015). Another theory highlighted the importance of the moist enthalpy advection/Rossby wave modulation during the initial development of WNPAAC during the El Niño developing year fall season (Wu et al., 2017a,b). When the El Niño turns to the decay phase, the AAC is sustained by the TIO warming to summer (Figures 5E–H), based on a Kelvin-wave induced Ekman divergence mechanism (Wu et al., 2009; Xie et al., 2009). The TIO warming forces a Matsuno-Gill response in the troposphere (Matsuno, 1966; Gill, 1980), accompanied by an eastward-propagating atmospheric Kelvin wave extending into the western Pacific. Off the equators, the Kelvin wave induces low-level Ekman divergence through the wind shear between the anticyclonic wind and easterly wind anomalies over the WNP, suppressing deep convection over the WNP and contributing to the development of AAC (Terao and Kubota, 2005; Xie et al., 2009, 2016; Yuan et al., 2012; Li et al., 2017; He et al., 2019). The WNPAAC establishes internal positive feedback between the TIO and WNP. In spring, the anomalous northeasterly on the south flank of the AAC strengthens the prevailing northeast winds and cools the WNP SST via the positive WES feedback (Figures 5G, 6G). Namely, the SST cooling enhances the AAC with an atmospheric Rossby wave response by suppressing local convection (Wang et al., 2003, 2017; Li et al., 2017). When the Pacific northeast trade winds retreat eastward, and the north TIO monsoon westerlies expand eastward into the WNP in summer, the AAC is coupled with TIO SST (Figure 5H). The anomalous easterly on the south flank of the AAC extends to the Bay of Bengal, reinforcing the north TIO SST warming (Figures 5H, 6H; Kosaka et al., 2013; Xie et al., 2016). The coupled ocean-atmosphere processes described above are referred to as the IPOC effect, which can influence East Asian (e.g., Chang et al., 2000a,b; Wang et al., 2000; Wang and Li, 2004; Park et al., 2010; Kosaka, 2021) and Indian summer monsoons (Izumo et al., 2008; Huang et al., 2018; Chowdary et al., 2019; Du et al., 2021; Darshana et al., 2022) and their water vapor transport (Zhou et al., 2021; Cai et al., 2022), as well as typhoon activity (Du et al., 2011; Zhan et al., 2011; Wang and Wang, 2019), leading to a series of extreme weather events in Asia, such as heat waves, heavy rainfalls, and severe floods (e.g., Wu et al., 2009, 2010; Hu et al., 2011, 2017; Yuan et al., 2019).
IOD, featuring an SST contrast along the equatorial Indian Ocean, is another major climate mode that can affect weather and climate over the surrounding regions and even the globe (Saji et al., 1999; Webster et al., 1999). The IOD is tied with the Indian Ocean summer monsoon evolution, featuring a significant seasonal phase lock (Yang et al., 2015; Zhang et al., 2021a). A typical IOD event tends to develop in boreal summer, peak in fall, and rapidly decay during winter. In the positive IOD phase, the SST shows west warming and east cooling, which further changes the sea level pressure gradient and induces anomalous surface easterly winds along the equator (Behera et al., 2021). Wind anomalies force oceanic adjustments that shoal the thermocline and favor the upwelling in the east pole of IOD, enhancing local SST cooling. As such, atmospheric convection is suppressed, resulting in drought and bushfires over the Maritime Continent and Australia (Wang and Cai, 2020; Zhang et al., 2021c). On the other side, warm water piles up in the west, strengthening local convection, leading to severe rainfall and flood over East Africa (Saji and Yamagata, 2003; Vinayachandran et al., 2009). The IOD also affects the rainfall variability in South and East Asia (Guan and Yamagata, 2003; Anil et al., 2016; Heng et al., 2019; Xiao et al., 2020; Ratna et al., 2021).
These anomalies are associated with adjustments of the Walker Circulation due to the Bjerknes feedback (Annamalai et al., 2003). Any perturbation in this loop may drive the IOD onset. Consequently, ENSO is one of the drivers of the IOD (Alexander et al., 2002). During the development of an El Niño event, the Indo-Pacific Walker Circulation interacts as a pair of gear wheels, in which the SST warming in the central-eastern Pacific induces eastward movement of the ascending branch of the Walker Circulation, while anomalous descending branch forms over the Maritime Continent, leading to higher sea level pressure. Correspondingly, easterly wind anomalies appear in the tropical IO, which could trigger positive IOD. The 1997 positive IOD is one of the typical cases of the ENSO-IOD connection, which directly promotes the IOD concept (Saji et al., 1999).
Whether the IOD can develop independently from ENSO or not has been discussed since 20 years ago (Allan et al., 2001; Dommenget and Latif, 2002; Yamagata et al., 2003; Stuecker et al., 2017; Le et al., 2020). Lately, observations and coupled climate models experiments show that the IOD is an intrinsic mode in the Indian Ocean (Fischer et al., 2005; Behera et al., 2006; Luo et al., 2008; Du et al., 2013a; Guo et al., 2015; Crétat et al., 2018; Zhang et al., 2020).
Strong IOD usually co-occurs with strong ENSO, but there are exceptions. These IOD events are facilitated by the regional ocean-atmosphere interaction, independent of ENSO, e.g., in 1994, 2006, and 2019 (Figure 7), with the dipole mode index (DMI) over two times the standard deviation (STD) (Doi et al., 2020; Wang and Cai, 2020; Ratna et al., 2021; Shi and Wang, 2021; Zhang et al., 2021c). Only weak SST warming signatures in those three events have been identified in the central or eastern equatorial Pacific (Chiang and Vimont, 2004; Kao and Yu, 2009), implying the ENSO condition in the Pacific does not always determine IOD strength. Furthermore, some positive IOD events concur with the La Niña, e.g., in 2007 and 2008 (Luo et al., 2008; Cai et al., 2009).
Figure 7. (A) Time series of standardized SST indices of Nino3.4, DMI, IOB, SETIO and SWTIO from 1982-2021. (B–E) SST (shading, °C), precipitation (contour, mm/day), and wind anomalies (vectors, m/s) of IOD peak season (Aug-Nov) for strong positive events in 1997, 1994, 2006, and 2019, respectively. The DMI is defined by the difference of area-mean SST anomalies between the western tropical Indian Ocean (WTIO, 50°-70°E,10°N−10°S) and the southeastern tropical Indian Ocean (SETIO, 90°-110°E, Eq.-10°S). Data are based on OISST from 1982-2021, CCMP wind from 1988-2021, CMAP precipitation from 1979-2021. Linear trends and seasonal cycle are removed based on each period coverage.
Despite the difference in the SST pattern (Jiang et al., 2022), all three events (1994, 2006, and 2019) show a significant anomalous reduction of precipitation and anticyclonic wind over the southeast TIO (Figure 7). Such anomalous anticyclonic winds directly force the downwelling Rossby waves that propagate westward in the southern TIO along 10°S (Figure 8). Among those three events, the 2019 positive IOD is the strongest, of which the strength of wind stress curl and sea level anomaly is close to that of the 1997 event (Zhang and Du, 2021; Zhang et al., 2021b).
Figure 8. Longitude-time diagram for sea level anomaly (shading, meter) and anomalous winds (vector, m/s) and SST anomaly (contour, °C) along the equator [(A–D), Eq.IO] and 10°S [(E,F), STIO] in the Indian Ocean for four positive IOD events in 1997 (A,E), 1994 (B,F), 2006 (C,G), and 2019 (D,H). Data are based on OISST from 1982-2021, CCMP wind from 1988-2021, CMEMS SLA from 1993-2020. Linear trends and seasonal cycles are removed based on each period coverage.
Since the positive IOD-related wind anomalies could force the downwelling Rossby waves, the impacts of strong IOD could persist into the next year via the IPOC effect, for example, the 2019 positive IOD event (Takaya et al., 2020; Ratna et al., 2021). The 2019 wintertime impacts are due to the meander of a subtropical jet associated with the warming of the IOD western pole (Doi et al., 2020), while the following summer climate variation in East Asia is tied to IPOC triggered by IOD without significant ENSO influence (Zhou et al., 2021). Southwest TIO SST warming persisted to the 2020 summer, sustained by the deepening of thermocline associated with the Rossby wave, inducing north TIO and basin-wide warming in the 2020 spring (Figures 8, 9; Zhang and Du, 2021). Consequently, the 2020 IOB impacted the WNPAAC and caused excessive rainfall in East Asia (Chen et al., 2021; Zhou et al., 2021). Further investigation showed that the TIO regional warming could exert influence on the WNP. Chen et al. (2019) suggested that the southwest or the southeast tropical Indian Ocean SST warming could impact WNPAAC during early summer.
Figure 9. Time-latitude diagram for sea surface temperature anomalies (shading, °C) and surface wind anomalies (vector, m/s) meridionally averaged over 50-60°E in the Indian Ocean for four positive IOD events in 1997 (A), 1994 (B), 2006 (C), and 2019 (D). Data are based on OISST from 1982-2021, CCMP (Atlas et al., 2011; Mears et al., 2019) wind from 1988-2021. Linear trends and seasonal cycles are removed based on each period coverage.
The WNPAAC is an important low-level circulation system. Previous studies indicated that the WNPAAC could modulate East Asia summer climate (Chang et al., 2000a,b; Wang and Li, 2004; Li and Wang, 2005; Yang et al., 2007; Li et al., 2008; Wu et al., 2009, 2010; Xie et al., 2009; Du et al., 2011; Wang et al., 2013; Chen et al., 2016; He et al., 2016). The WNPAAC largely influences the East Asia summer monsoon (Chang et al., 2000a,b; Wang and Li, 2004; Li and Wang, 2005; Yang et al., 2007; Li et al., 2008; Wang et al., 2013) and have a significant impact on the rainfall variability in East Asia (Wu et al., 2009, 2010; Du et al., 2011; Chen et al., 2016; He et al., 2016). Typically, due to the anomalous southwesterly winds on the northwestern flank, the AAC supplies abundant water vapor to China and Japan during summer (Ding, 1992; Chang et al., 2000a; Zhang and Sumi, 2002; Wu et al., 2003, 2017c; Zhou and Yu, 2005; Ding and Wang, 2008; Liu et al., 2008; Xie et al., 2009; Sampe and Xie, 2010; Wang et al., 2013; Chen et al., 2017, 2019; Zhang et al., 2017).
The above theories mentioned in Section WNPAAC and IPOC emphasized the effect of external forcing of ENSO. To quantify the intensity of the AAC, Huang et al. (2010) define a WNPAAC index, the 850hPa zonal wind difference between a southern region (100°E-130°E, 5°N-15°N) and a northern region (110°E-140°E, 20°N-30°N) during June-July (JJ) months. A positive (negative) WNPAAC index indicates an anomalous anticyclone (cyclone). Generally, the WNPAAC index is highly correlated with Niño 3.4 index, the correlation coefficient reaching 0.55 with a lag of 6 months (Figure 10). In most El Niño events, a significant WNPAAC carries excess water vapor to East Asia.
Figure 10. Standardized Nino 3.4 index and WNPAAC index (move 6 months ahead), a 13-month running smoother is applied. Gary shadings represent the strong ENSO events. SST is from OISST. Wind is from ERA5. The anomalies are relative to 1982-2021.
In 1995, 2007, and 2020, an anomalous strong WNPAAC was sustained in early summer without strong antecedent El Niño. Instead, strong positive IOD seems to act as forcing (Figures 7, 10). In these cases, the Western Pacific Subtropical High (WPSH) center is stronger and more southward than its climatology. Enhanced and westward extending WNPAAC supplies water vapor to East Asia through its northwestern flank. The 2020 rainfall event was extreme, making a historical record since the 1960s (Figure 11; Ding et al., 2021). Due to the position of the anomalous anticyclone, the positive rainfall regions are slightly different among the three cases (1995, 2007, and 2020) (Figure 11).
Figure 11. May to July, 850hPa wind anomalies (vectors, m/s), vertically integrated moisture divergence anomalies (shading, kg m−2, negative values mean convergence) and the center of WPSH (the 5880 gpm, mean and anomalies, red contour lines) of 1998 (A), 1995 (B), 2007 (C) and 2020 (D). The data were from ERA5. The anomalies are relative to 1979-2021.
IPOC theory could explain the relationship between TIO warming and the WNPAAC. The TIO warming follows the El Niño event, peaks in the boreal spring, and persists through boreal summer (Xie et al., 2016), sustaining the WNPAAC after El Niño decay (e.g., Yang et al., 2007; Wu et al., 2009; Xie et al., 2009, 2016; Chen et al., 2018).
For years without significant El Niño, like 1995, 2007, and 2020, the IPOC effect still works. The intensified and southward extending WNPAAC is sustained in response to the TIO warming. As a result, rainfall increases over the TIO and East Asia and decreases in the WNP and South Asia (Figures 11, 12). The rainfall pattern in 1995, 2007, and 2020 is similar to that in summer following a strong El Niño, e.g., 1998.
Figure 12. June to July, SST anomalies (contours, °C), rainfall anomalies (shadings, mm/day) of 1998 (A), 1995 (B), 2007 (C) and 2020 (D). The anomalies are relative to 1982-2021. SST is from OISST, and Rainfall is from CMAP (Xie and Arkin, 1997).
Climatologically, monsoonal winds from the TIO and trade winds from the western Pacific warm pool meet over the South China Sea, forming East Asia's three main water vapor sources in summer. The northwestern flank of the WNPAAC changes the proportions of the three water vapor sources in the late spring and summer. To quantify the water vapor transport, we integrate the water vapor flux across boundaries following the method in Richter and Xie (2010). In the above four IPOC events, water vapor transport decreased by about 0.16Sv (1998), 0.09Sv (1995), 0.08Sv (2007), and 0.24Sv (2020) from the north TIO but increased by about 0.26Sv (1998), 0.13Sv (1995), 0.15Sv (2007) and 0.28Sv (2020) from the western Pacific (Figure 13). More water vapor is transported from the western Pacific warm pool, which has the highest moisture in the tropics. Note that in 1995, 2007, and 2020, the significant TIO warming sustained the anomalous WNPAAC without concurrent strong El Niño but with leading strong positive IOD. As a result, the WNPAAC is sustained by the SWTIO and SETIO warming, and rainfall is decreased in the WNP and increased over the TIO basin and East Asia. In conclusion, IPOC could be established without significant ENSO influence (Figure 14).
Figure 13. Water vapor transport (blue arrows, Sv) through boundaries (black lines) and the water vapor transport anomalies (red arrows, Sv) through boundaries (red lines) from the Indian Ocean and the Pacific of climatology (A), 1998 (B), 1995 (C), 2007 (D), and 2020 (E). The data were from ERA5.
Figure 14. Schematic representation of IPOC effect. Green arrow denotes the WNPAAC; red circles and vertical wavy lines denote SST warming and enhanced atmospheric convection, respectively; blue (yellow) shading as well as cloud symbols denote increased (decreased) rainfall there.
Those processes have been tested in model experiments. Chen et al. (2021) confirmed that southwest TIO SST warming could induce easterly winds response in the north TIO and WNP (Figures 15A,B) in a coupled model experiment. The easterly wind response favors the north TIO SST warming and the WNP SST cooling with the different background winds via WES feedback in boreal early summer (Figures 15A,B). Both the positive north TIO SST and negative WNP SST anomalies facilitate the development of IPOC. Note that the atmospheric model only experiment cannot reproduce the observed characteristics due to southwest TIO SST warming (Figures 15C,D). Such a result, in turn, indicates the importance of both southwest TIO warming and the resultant air-sea coupling processes contributing to the IPOC in the coupled model experiment. The southwest TIO SST warming could result from strong antecedent positive IOD (Takaya et al., 2020; Chen et al., 2021; Zhou et al., 2021).
Figure 15. Responses to southwest TIO SST warming in CESM1 using a partial coupling framework: (A) for precipitation (shading, mm day−1) and low-level (i.e., 925-hPa) wind (vector, m s−1) response, (B) for SST (°C) and 10 meter wind (vector, m s−1) response. (C,D) showing the responses to southwest TIO SST warming in CAM4 (Atmospheric component of CESM1). The shading in (D) denotes the southwest TIO SST anomaly forcing using in CESM1 and CAM4. The shading in (A,C), the stippling in (B), and the black vector in (A–D) denote the response is significant at an 80% confidence level according to a student's t test.
IPOC is an intrinsic ocean-atmosphere coupled mode that could occur with or without ENSO forcing (Kosaka et al., 2013, 2021; Wang et al., 2018), featuring the coupling of the TIO SST warming and the WNPAAC in summer. The TIO SST warming interacts with the WNPAAC through a warm atmospheric equatorial Kelvin wave wedge penetrating the western Pacific and a cold atmospheric Rossby wave caused by the anomalous diabatic cooling in the tropical WNP westwards to the north TIO (Xie et al., 2009, 2016). The IPOC mode influences the atmospheric circulation and water vapor transport into East Asia, resulting in extreme weather events (Yang et al., 2007, 2010), e.g., heat waves, heavy rainfalls, and severe floods in South, Southeast, and East Asia.
ENSO had been considered the main driver responsible for IPOC. ENSO impacts TIO through an atmospheric bridge that directly causes surface heat flux changes in the sub-basins and excites thermocline-SST feedback in the SWTIO (Lau and Nath, 1996; Klein et al., 1999; Huang and Kinter, 2002; Xie et al., 2002). However, in 1995, 2007, and 2020, the TIO SST warmed up without concurrent strong El Niño but rather with strong positive IOD (Zhang and Du, 2021), which maintained the WNPAAC (Chen et al., 2021). These cases suggest that IPOC is an intrinsic mode in the Indo-western Pacific Ocean. The TIO SST warming and WNPAAC act as a self-sustaining system, independent of external forcings, such as ENSO.
The TIO warming induces marked climate anomalies in Asia and the Indo-western Pacific. The south TIO is the key. The oceanic downwelling Rossby waves propagate westward from the southeast TIO, deepen the thermocline, and warm the subsurface in the SWTIO, leading to SST warming via thermocline-SST feedback from winter to summer, associated with El Niño or positive IOD. The SWTIO SST warming enhances local convection and induces “C-shaped” wind anomalies during winter and early spring, resulting in basin-wide SST warming via WES feedback (Chen et al., 2021). Furthermore, the SWTIO downwelling Rossby waves propagate westward to the west boundary and reflect as eastward-propagating oceanic Kelvin waves in the following spring. The equatorial Kelvin waves eventually reach the east boundary and contribute to the southeast TIO SST warming in the early summer. The southeast TIO warming enhances local convection and forces second “C-shaped” wind anomalies over the east TIO (Chen et al., 2019). Both southwest and southeast TIO warming contributes to the WNPAAC and affect its extension/location in the late spring and summer.
Changes in extension and location of WNPAAC influence the East Asian and Indian summer monsoons and their water vapor transport over the Indo-western Pacific. The northwestern flank of WNPAAC alters water vapor transport to East Asia in late spring and summer, which mostly converge over the South China Sea and adjacent regions before flowing further north. Most water vapor is transported over the western Pacific warm pool with the highest moisture in the tropics and less from the southern hemisphere and the Indian Ocean (Cai et al., 2022). The enhanced Asian Summer monsoon and water vapor transport contribute to extreme rainfalls in central China and Japan during the boreal summer, leading to extreme weather disaster events and influencing millions of people's lives.
All authors listed have made a substantial, direct, and intellectual contribution to the work and approved it for publication.
This study was supported by the National Natural Science Foundation of China (42090042, 42175043, and 42106021), the Chinese Academy of Sciences (XDB42010305, 133244KYSB20190031, 183311KYSB20200015, ISEE2021PY02, and ISEE2021ZD01), Youth Innovation Promotion Association CAS (2022347), and the Southern Marine Science and Engineering Guangdong Laboratory (Guangzhou) (2019BT02H594).
We acknowledge the NOAA for providing OISST (Reynolds et al., 2002), GODAS oceanic reanalysis (Behringer et al., 1998), CMAP (Xie and Arkin, 1997) and GPCP (Adler et al., 2003) precipitation data at https://psl.noaa.gov/data/gridded/index.html. We also acknowledge Met Office Hadley Centre for providing HadISST (Rayner et al., 2003) dataset at https://www.metoffice.gov.uk/hadobs/hadisst/. CCMP Version-2.0 vector wind analyses (Atlas et al., 2011; Mears et al., 2019) are produced by Remote Sensing Systems, available at www.remss.com. SLA data are provided by CMEMS, available at https://resources.marine.copernicus.eu/product-detail (DOI: https://doi.org/10.48670/moi-00148). The gridded ocean subsurface temperature data are obtained from the Institute of Atmospheric Physics, Chinese Academy of Sciences (http://www.ocean.iap.ac.cn/pages/dataService/dataService.html?navAnchor=dataService) (Cheng et al., 2017). The atmospheric data are from the fifth generation European Centre for Medium-Range Forecasts (ECMWF) reanalysis (ERA5, https://cds.climate.copernicus.eu) (Hersbach et al., 2020).
The authors declare that the research was conducted in the absence of any commercial or financial relationships that could be construed as a potential conflict of interest.
The handling editor CM declared a shared affiliation with the author S-PX at the time of review.
All claims expressed in this article are solely those of the authors and do not necessarily represent those of their affiliated organizations, or those of the publisher, the editors and the reviewers. Any product that may be evaluated in this article, or claim that may be made by its manufacturer, is not guaranteed or endorsed by the publisher.
Adler, R. F., Huffman, G. J., Chang, A., Ferraro, R., Xie, P. P., Janowiak, J., et al. (2003). The version-2 Global Precipitation Climatology Project (GPCP) monthly precipitation analysis (1979–present). J. Hydrometeor., 4, 1147–1167. doi: 10.1175/1525-7541(2003)004<1147:TVGPCP>2.0.CO;2
Alexander, M. A., Blad,é, I., Newman, M., Lanzante, J. R., Lau, N. C., and Scott, J. D. (2002). The atmospheric bridge: The influence of ENSO teleconnections on air–sea interaction over the global oceans. J. Clim. 15, 2205–2231. doi: 10.1175/1520-0442(2002)015<2205:TABTIO>2.0.CO;2
Allan, R., Chambers, D., Drosdowsky, W., Hendon, H., Latif, M., Nicholls, N., et al. (2001). Is there an Indian Ocean dipole and is it independent of the El Niño-Southern Oscillation. CLIVAR Exchanges 21, 18–22.
Anil, N., Ramesh Kumar, M. R., Sajeev, R., and Saji, P. K. (2016). Role of distinct flavours of IOD events on Indian summer monsoon. Nat. Hazards 82, 1317–1326. doi: 10.1007/s11069-016-2245-9
Annamalai, H., Liu, P., and Xie, S. P. (2005). Southwest Indian Ocean SST variability: Its local effect and remote influence on Asian monsoons. J. Clim. 18, 4150–4167. doi: 10.1175/JCLI3533.1
Annamalai, H., Murtugudde, R., Potemra, J., Xie, S. P., Liu, P., and Wang, B. (2003). Coupled dynamics over the Indian Ocean: Spring initiation of the zonal mode. Deep Sea Res. Part II: Topical Stud. Oceanogr. 50, 2305–2330. doi: 10.1016/S0967-0645(03)00058-4
Atlas, R., Hoffman, R. N., Ardizzone, J., Leidner, S. M., Jusem, J. C., Smith, D. K., et al. (2011). A cross-calibrated, multiplatform ocean surface wind velocity product for meteorological and oceanographic applications. Bull. Amer. Meteor. Soc. 92, 157–174. doi: 10.1175/2010BAMS2946.1
Basconcillo, J., Moon, I. J., Wang, B., and Mistry, M. (2021). Possible influence of the warm pool ITCZ on compound climate extremes during the boreal summer. Environ. Res. Lett. 16, 114039. doi: 10.1088/1748-9326/ac30f8
Behera, S. K., Doi, T., and Ratnam, J. V. (2021). “Air–sea interactions in tropical Indian Ocean: The Indian Ocean Dipole,” in Tropical and Extratropical Air-Sea Interactions (p. 115–139). Elsevier. doi: 10.1016/B978-0-12-818156-0.00001-0
Behera, S. K., Luo, J. J., Masson, S., Rao, S. A., Sakuma, H., and Yamagata, T. (2006). A CGCM study on the interaction between IOD and ENSO. J. Clim. 19, 1688–1705. doi: 10.1175/JCLI3797.1
Behringer, D. W., Ji, M., and Leetmaa, A. (1998). An improved coupled model for ENSO prediction and implications for ocean initialization. Part I: The ocean data assimilation system. Mon. Wea. Rev. 126, 1013–1021. doi: 10.1175/1520-0493(1998)126<1013:AICMFE>2.0.CO;2
Cai, W., Cowan, T., and Raupach, M. (2009). Positive Indian Ocean dipole events precondition southeast Australia bushfires. Geophys. Res. Lett. 36. doi: 10.1029/2009GL039902
Cai, Y., Chen, Z., and Du, Y. (2022). The role of Indian Ocean warming on extreme rainfall in central China during early summer 2020: without significant El Niño influence. Clim. Dyn. 59, 951–960. doi: 10.1007/s00382-022-06165-9
Chakravorty, S., Chowdary, J. S., and Gnanaseelan, C. (2013). Spring asymmetric mode in the tropical Indian Ocean: role of El Niño and IOD. Clim. Dyn. 40, 1467–1481. doi: 10.1007/s00382-012-1340-1
Chambers, D. P., Tapley, B. D., and Stewart, R. H. (1999). Anomalous warming in the Indian Ocean coincident with El Niño. J. Geophys. Res: Oceans. 104, 3035–3047. doi: 10.1029/1998JC900085
Chang, C. P., Zhang, Y., and Li, T. (2000a). Interannual and interdecadal variations of the East Asian summer monsoon and tropical Pacific SSTs. Part I: Roles of the subtropical ridge. J. Clim. 13, 4310–4325. doi: 10.1175/1520-0442(2000)013<4310:IAIVOT>2.0.CO;2
Chang, C. P., Zhang, Y., and Li, T. (2000b). Interannual and interdecadal variations of the East Asian summer monsoon and tropical Pacific SSTs. Part II: Meridional structure of the monsoon. J. Clim. 13, 4326–4340. doi: 10.1175/1520-0442(2000)013<4326:IAIVOT>2.0.CO;2
Chen, Z., Du, Y., Wen, Z., Wu, R., and Wang, C. (2018). Indo-Pacific climate during the decaying phase of the 2015/16 El Niño: Role of southeast tropical Indian Ocean warming. Clim. Dyn. 50, 4707–4719. doi: 10.1007/s00382-017-3899-z
Chen, Z., Du, Y., Wen, Z., Wu, R., and Xie, S. P. (2019). Evolution of south tropical Indian Ocean warming and the climatic impacts following strong El Niño events. J. Clim. 32, 7329–7347. doi: 10.1175/JCLI-D-18-0704.1
Chen, Z., Li, Z., Du, Y., Wen, Z., Wu, R., and Xie, S. P. (2021). Trans-Basin Influence of Southwest Tropical Indian Ocean Warming during Early Boreal Summer. J. Clim. 34, 9679–9691. doi: 10.1175/JCLI-D-20-0925.1
Chen, Z., Wen, Z., Wu, R., and Du, Y. (2017). Roles of tropical SST anomalies in modulating the western north Pacific anomalous cyclone during strong La Niña decaying years. Clim. Dyn. 49, 633–647. doi: 10.1007/s00382-016-3364-4
Chen, Z., Wen, Z., Wu, R., Lin, X., and Wang, J. (2016). Relative importance of tropical SST anomalies in maintaining the western North Pacific anomalous anticyclone during El Niño to La Niña transition years. Clim. Dyn. 46, 1027–1041. doi: 10.1007/s00382-015-2630-1
Cheng, L., Trenberth, K. E., Fasullo, J., Boyer, T., Abraham, J., and Zhu, J. (2017). Improved estimates of ocean heat content from 1960 to 2015. Sci. Adv. 3, e1601545. doi: 10.1126/sciadv.1601545
Chiang, J. C. H., and Vimont, D. J. (2004). Analogous Pacific and Atlantic Meridional Modes of Tropical Atmosphere–Ocean Variability. J. Clim. 17, 4143–4158. doi: 10.1175/JCLI4953.1
Chowdary, J. S., Patekar, D., Srinivas, G., Gnanaseelan, C., and Parekh, A. (2019). Impact of the Indo-Western Pacific Ocean capacitor mode on South Asian summer monsoon rainfall. Clim. Dyn. 53, 2327–2338 doi: 10.1007/s00382-019-04850-w
Chowdary, J. S., Xie, S. P., Luo, J. J., Hafner, J., Behera, S., Masumoto, Y., et al. (2011). Predictability of Northwest Pacific climate during summer and the role of the tropical Indian Ocean. Clim. Dyn. 36, 607–621. doi: 10.1007/s00382-009-0686-5
Crétat, J., Terray, P., Masson, S., and Sooraj, K. P. (2018). Intrinsic precursors and timescale of the tropical Indian Ocean Dipole: insights from partially decoupled numerical experiment. Clim. Dyn. 51, 1311–1332. doi: 10.1007/s00382-017-3956-7
Darshana, P., Chowdary, J. S., Parekh, A., and Gnanaseelan, C. (2022). Relationship between the Indo-western Pacific Ocean capacitor mode and Indian summer monsoon rainfall in CMIP6 models. Clim. Dyn. 59, 393–415. doi: 10.1007/s00382-021-06133-9
Ding, Y. (1992). Summer monsoon rainfalls in China. J. Meteorol. Soc. Japan. Ser. II 70, 373–396. doi: 10.2151/jmsj1965.70.1B_373
Ding, Y., Liu, Y., and Hu, Z. Z. (2021). The record-breaking mei-yu in 2020 and associated atmospheric circulation and tropical SST anomalies. Adv. Atmos. Sci. 38, 1980–1993. doi: 10.1007/s00376-021-0361-2
Ding, Y., and Wang, Z. Y. (2008). A study of rainy seasons in China. Meteorol. Atmos. Phys. 100, 121–138. doi: 10.1007/s00703-008-0299-2
Doi, T., Behera, S. K., and Yamagata, T. (2020). Wintertime impacts of the 2019 super IOD on East Asia. Geophys. Res. Lett. 47, e2020GL089456. doi: 10.1029/2020GL089456
Dommenget, D., and Latif, M. (2002). A cautionary note on the interpretation of EOFs. J. Clim. 15, 216–225. doi: 10.1175/1520-0442(2002)015<0216:ACNOTI>2.0.CO;2
Du, Y., Cai, W., and Wu, Y. (2013a). A new type of the Indian Ocean Dipole since the mid-1970s. J. Clim. 26, 959–972. doi: 10.1175/JCLI-D-12-00047.1
Du, Y., Chen, Z., Zhang, Y., Hu, K., Zheng, X., and Yu, W. (2021). “Influence of South Tropical Indian Ocean dynamics on the Indian summer monsoon,” in Indian Summer Monsoon Variability (pp. 183–196). Elsevier. doi: 10.1016/B978-0-12-822402-1.00013-2
Du, Y., Xie, S. P., Huang, G., and Hu, K. (2009). Role of air–sea interaction in the long persistence of El Niño–induced north Indian Ocean warming. J. Clim. 22, 2023–2038. doi: 10.1175/2008JCLI2590.1
Du, Y., Xie, S. P., Yang, Y. L., Zheng, X. T., Liu, L., and Huang, G. (2013b). Indian Ocean variability in the CMIP5 multimodel ensemble: The basin mode. J. Clim. 26, 7240–7266. doi: 10.1175/JCLI-D-12-00678.1
Du, Y., Yang, L., and Xie, S. P. (2011). Tropical Indian Ocean influence on northwest Pacific tropical cyclones in summer following strong El Niño. J. Clim. 24, 315–322. doi: 10.1175/2010JCLI3890.1
Du, Y., Zhang, Y., Zhang, L. Y., Tozuka, T., Ng, B., and Cai, W. (2020). Thermocline warming induced extreme Indian Ocean dipole in 2019. Geophys. Res. Lett. 47, e2020GL090079. doi: 10.1029/2020GL090079
Eabry, M. D., Taschetto, A. S., Maharaj, A. M., and Sen Gupta, A. (2021). What Determines the Lagged ENSO Response in the South-West Indian Ocean? Geophys. Res. Lett. 48, e2020GL091958. doi: 10.1029/2020GL091958
Fischer, A. S., Terray, P., Guilyardi, E., Gualdi, S., and Delecluse, P. (2005). Two independent triggers for the Indian Ocean dipole/zonal mode in a coupled GCM. J. Clim.18, 3428–3449. doi: 10.1175/JCLI3478.1
Gill, A. E. (1980). Some simple solutions for heat-induced tropical circulation. Q. J. R. Meteor. Soc. 106, 447–462. doi: 10.1002/qj.49710644905
Guan, Z., and Yamagata, T. (2003). The unusual summer of 1994 in East Asia: IOD teleconnections. Geophys. Res. Lett. 30, 1554. doi: 10.1029/2002GL016831
Guo, F., Liu, Q., Sun, S., and Yang, J. (2015). Three types of Indian Ocean dipoles. J. Clim. 28, 3073–3092. doi: 10.1175/JCLI-D-14-00507.1
Guo, F., Liu, Q., Yang, J., and Fan, L. (2018). Three types of Indian Ocean basin modes. Clim. Dyn. 51, 4357–4370. doi: 10.1007/s00382-017-3676-z
He, C., Zhou, T., and Li, T. (2019). Weakened anomalous western North Pacific anticyclone during an El Niño-decaying summer under a warmer climate: Dominant role of the weakened impact of the tropical Indian Ocean on the atmosphere. J. Clim. 32, 213–230. doi: 10.1175/JCLI-D-18-0033.1
He, Z., Wu, R., and Wang, W. (2016). Signals of the South China Sea summer rainfall variability in the Indian Ocean. Clim. Dyn. 46, 3181–3195. doi: 10.1007/s00382-015-2760-5
Heng, C., Yoon, S. K., Kim, J. S., and Xiong, L. (2019). Inter-seasonal precipitation variability over southern China associated with commingling effect of Indian Ocean Dipole and El Niño. Water. 11, 2023. doi: 10.3390/w11102023
Hermes, J. C., and Reason, C. J. C. (2008). Annual cycle of the South Indian Ocean (Seychelles-Chagos) thermocline ridge in a regional ocean model. J. Geophys. Res: Oceans. 113, C04035. doi: 10.1029/2007JC004363
Hersbach, H., Bell, B., Berrisford, P., Hirahara, S., Horányi, A., Muñoz-Sabater, J., et al. (2020). The ERA5 global reanalysis, Q. J. R. Meteorol. Soc. 146, 1999–2049. doi: 10.1002/qj.3803
Hirons, L., and Turner, A. (2018). The impact of Indian Ocean mean-state biases in climate models on the representation of the East African short rains. J. Clim. 31, 6611–6631. doi: 10.1175/JCLI-D-17-0804.1
Hu, D., Wu, L., Cai, W., Gupta, A. S., Ganachaud, A., Qiu, B., et al. (2015). Pacific western boundary currents and their roles in climate. Nature 522, 299–308. doi: 10.1038/nature14504
Hu, K., Huang, G., and Huang, R. (2011). The impact of tropical Indian Ocean variability on summer surface air temperature in China. J. Clim. 24, 5365–5377. doi: 10.1175/2011JCLI4152.1
Hu, K., Huang, G., Xie, S. P., and Long, S. M. (2019). Effect of the mean flow on the anomalous anticyclone over the Indo-Northwest Pacific in post-El Niño summers. Clim. Dyn. 53, 5725–5741. doi: 10.1007/s00382-019-04893-z
Hu, K., Xie, S. P., and Huang, G. (2017). Orographically anchored El Niño effect on summer rainfall in central China. J. Clim. 30, 10037–10045. doi: 10.1175/JCLI-D-17-0312.1
Huang, B., and Kinter, J. L. (2002). Interannual variability in the tropical Indian Ocean. J. Geophys. Res: Oceans. 107, 20–21. doi: 10.1029/2001JC001278
Huang, G., Hu, K., and Xie, S. P. (2010). Strengthening of tropical Indian Ocean teleconnection to the northwest Pacific since the mid-1970s: An atmospheric GCM study. J. Clim. 23, 5294–5304. doi: 10.1175/2010JCLI3577.1
Huang, R. H., and Wu, Y. F. (1989). The influence of ENSO on the summer climate change in China and its mechanism. Adv. Atmos. Sci., 6, 21–32, doi: 10.1007/BF02656915
Huang, Y., Wang, B., Li, X., and Wang, H. (2018). Changes in the influence of the western Pacific subtropical high on Asian summer monsoon rainfall in the late 1990s. Clim. Dyn. 51, 443–455. doi: 10.1007/s00382-017-3933-1
Izumo, T., Montégut, C. B., Luo, J. J., Behera, S. K., Masson, S., and Yamagata, T. (2008). The role of the western Arabian Sea upwelling in Indian monsoon rainfall variability. J. Clim. 21, 5603–5623. doi: 10.1175/2008JCLI2158.1
Jensen, T. G. (1993). Equatorial variability and resonance in a wind-driven Indian Ocean model. J. Geophys. Res: Oceans. 98, 22533–22552. doi: 10.1029/93JC02565
Jiang, J., Liu, Y., Mao, J., Li, J., Zhao, S., and Yu, Y. (2022). Three types of positive Indian Ocean dipoles and their relationships with the South Asian summer monsoon. J. Clim. 35, 405–424. doi: 10.1175/JCLI-D-21-0089.1
Jury, M. R., and Huang, B. (2004). The Rossby wave as a key mechanism of Indian Ocean climate variability. D. Deep. Sea. Res. Part I Oceanogr. Res. Pap. 51, 2123–2136. doi: 10.1016/j.dsr.2004.06.005
Kao, H.-Y., and Yu, J.-Y. (2009). Contrasting Eastern-Pacific and Central-Pacific Types of ENSO. J. Clim. 22:615–632. doi: 10.1175/2008JCLI2309.1
Kawamura, R., Matsuura, T., and Iizuka, S. (2001). Role of equatorially asymmetric sea surface temperature anomalies in the Indian Ocean in the Asian summer monsoon and El Niño-Southern Oscillation coupling. J. Geophys. Res: Atmospheres. 106, 4681–4693. doi: 10.1029/2000JD900610
Klein, S. A., Soden, B. J., and Lau, N. C. (1999). Remote sea surface temperature variations during ENSO: Evidence for a tropical atmospheric bridge. J. Clim. 12, 917–932. doi: 10.1175/1520-0442(1999)012<0917:RSSTVD>2.0.CO;2
Kosaka, Y. (2021). “Coupling of the Indian, western North Pacific, and East Asian summer monsoons” in Indian Summer Monsoon Variability: El-Nino Teleconnections and Beyond, ed. J. S. Chowdary, A. Parekh and C. Gnanaseelan (Amsterdam, FL: Elsevier Press), 263–286. doi: 10.1016/B978-0-12-822402-1.00002-8
Kosaka, Y., Takaya, Y., and Kamae, Y. (2021). “The Indo-western Pacific Ocean capacitor effect” in Tropical and Extratropical Air-Sea Interactions: Modes of Climate Variations, ed. S. K. Behera (Amsterdam, FL: Elsevier Press), 141–169. doi: 10.1016/B978-0-12-818156-0.00012-5
Kosaka, Y., Xie, S. P., Lau, N. C., and Vecchi, G. A. (2013). Origin of seasonal predictability for summer climate over the Northwestern Pacific. Proc. Nat. Acad. Sci. 110, 7574–7579. doi: 10.1073/pnas.1215582110
Latif, M., and Barnett, T. P. (1995) Interactions of the tropical oceans. J. Clim. 8, 952–964. doi: 10.1175/1520-0442(1995)008<0952:Iotto>2.0.Co;2
Lau, N. C., and Nath, M. J. (1996). The role of the “atmospheric bridge” in linking tropical Pacific ENSO events to extratropical SST anomalies. J. Clim. 9, 2036–2057. doi: 10.1175/1520-0442(1996)009<2036:TROTBI>2.0.CO;2
Lau, N. C., and Nath, M. J. (2003). Atmosphere-ocean variations in the Indo-Pacific sector during ENSO episodes. J. Clim. 16, 3–20. doi: 10.1175/1520-0442(2003)016<0003:AOVITI>2.0.CO;2
Le Blanc, J. L., and Boulanger, J. P. (2001). Propagation and reflection of long equatorial waves in the Indian Ocean from TOPEX/POSEIDON data during the 1993–1998 period. Clim. Dyn. 17, 547–557. doi: 10.1007/s003820000128
Le, T., Ha, K. J., Bae, D. H., and Kim, S. H. (2020). Causal effects of Indian Ocean Dipole on El Niño–Southern oscillation during 1950–2014 based on high-resolution models and reanalysis data. Environ. Res. Lett. 15, 1040b1046. doi: 10.1088/1748-9326/abb96d
Li, S., Lu, J., Huang, G., and Hu, K. (2008). Tropical Indian Ocean basin warming and East Asian summer monsoon: A multiple AGCM study. J. Clim. 21, 6080–6088. doi: 10.1175/2008JCLI2433.1
Li, T., and Wang, B. (2005). A review on the western North Pacific monsoon: Synoptic-to-interannual variabilities. Terr. Atmos. Oceanic Sci. 16, 285–314. doi: 10.3319/TAO.2005.16.2.285(A)
Li, T., Wang, B., Wu, B., Zhou, T., Chang, C. P., and Zhang, R. (2017). Theories on formation of an anomalous anticyclone in western North Pacific during El Niño: A review. J. Meteorol. Res. 31, 987–1006. doi: 10.1007/s13351-017-7147-6
Liu, J., Wang, B., and Yang, J. (2008). Forced and internal modes of variability of the East Asian summer monsoon. Clim. Past. 4, 225–233. doi: 10.5194/cp-4-225-2008
Liu, Z., and Alexander, M. (2007). Atmospheric bridge, oceanic tunnel, and global climatic teleconnections. Rev. Geophys. 45, RG2005. doi: 10.1029/2005RG000172
Luo, J. J., Behera, S., Masumoto, Y., Sakuma, H., and Yamagata, T. (2008). Successful prediction of the consecutive IOD in 2006 and 2007. Geophys. Res. Lett. 35, L14SL02. doi: 10.1029/2007GL032793
Ma, J., Xie, S. P., and Xu, H. (2017). Intermember variability of the summer northwest Pacific subtropical anticyclone in the ensemble forecast. J. Clim. 30, 3927–3941. doi: 10.1175/JCLI-D-16-0638.1
Masumoto, Y., and Meyers, G. (1998). Forced Rossby waves in the southern tropical Indian Ocean. J. Geophys. Res: Oceans. 103, 27589–27602. doi: 10.1029/98JC02546
Matsuno, T. (1966). Quasi-geostrophic motions in the equatorial area. J. Meteorol. Soc. Japan. Ser. II. 44, 25–43. doi: 10.2151/jmsj1965.44.1_25
Mears, C. A., Scott, J., Wentz, F. J., Ricciardulli, L., Leidner, S. M., Hoffman, R., et al. (2019). A near real time version of the cross calibrated multiplatform (CCMP) Ocean surface wind velocity data set. J. Geophys. Res: Oceans 124, 6997–7010. doi: 10.1029/2019JC015367
Murtugudde, R., and Busalacchi, A. J. (1999). Interannual variability of the dynamics and thermodynamics of the tropical Indian Ocean. J. Clim. 12, 2300–2326. doi: 10.1175/1520-0442(1999)012<2300:IVOTDA>2.0.CO;2
Nitta, T. (1987). Convective activities in the tropical western Pacific and their impact on the Northern Hemisphere summer circulation. J. Meteorol. Soc. Japan. Ser. II. 65, 373–390. doi: 10.2151/jmsj1965.65.3_373
Park, J. Y., Jhun, J. G., Yim, S. Y., and Kim, W. M. (2010). Decadal changes in two types of the western North Pacific subtropical high in boreal summer associated with Asian summer monsoon/El Niño–Southern Oscillation connections. J. Geophys. Res. 115, D21129. doi: 10.1029/2009JD013642
Ratna, S. B., Cherchi, A., Osborn, T. J., Joshi, M., and Uppara, U. (2021). The Extreme Positive Indian Ocean Dipole of 2019 and Associated Indian Summer Monsoon Rainfall Response. Geophys. Res. Lett. 48, e2020GL091497. doi: 10.1029/2020GL091497
Rayner, N. A. A., Parker, D. E., Horton, E. B., Folland, C. K., Alexander, L. V., Rowell, D. P., et al. (2003). Global analyses of sea surface temperature, sea ice, and night marine air temperature since the late nineteenth century. J. Geophys. Res. 108, 4407, doi: 10.1029/2002JD002670
Reynolds, R. W., Rayner, N. A., Smith, T. M., Stokes, D. C., and Wang, W. (2002). An improved in situ and satellite SST analysis for climate. J. Climate 15, 1609–1625. doi: 10.1175/1520-0442(2002)015<1609:AIISAS>2.0.CO;2
Richter, I., and Xie, S. -P. (2010). Moisture transport from the Atlantic to the Pacific basin and its response to North Atlantic cooling and global warming. Clim. Dyn. 35, 551–566. doi: 10.1007/s00382-009-0708-3
Saji, N. H., Goswami, B. N., Vinayachandran, P. N., and Yamagata, T. (1999). A dipole mode in the tropical Indian Ocean. Nature. 401, 360–363. doi: 10.1038/43854
Saji, N. H., and Yamagata, T. J. C. R. (2003). Possible impacts of Indian Ocean dipole mode events on global climate. Clim. Res. 25, 151–169. doi: 10.3354/cr025151
Sampe, T., and Xie, S. P. (2010). Large-scale dynamics of the meiyu-baiu rainband: Environmental forcing by the westerly jet. J. Clim. 23, 113–134. doi: 10.1175/2009JCLI3128.1
Schott, F. A., Xie, S. P., and McCreary, J. P. Jr (2009). Indian Ocean circulation and climate variability. Rev. Geophys. 47, RG1002. doi: 10.1029/2007RG000245
Shi, W., and Wang, M. (2021). A biological Indian Ocean Dipole event in 2019. Sci. Rep. 11, 1–8. doi: 10.1038/s41598-021-81410-5
Stuecker, M. F., Jin, F. F., Timmermann, A., and McGregor, S. (2015). Combination mode dynamics of the anomalous northwest Pacific anticyclone. J. Clim. 28, 1093–1111. doi: 10.1175/JCLI-D-14-00225.1
Stuecker, M. F., Timmermann, A., Jin, F. F., Chikamoto, Y., Zhang, W., Wittenberg, A. T., et al. (2017). Revisiting ENSO/Indian Ocean dipole phase relationships. Geophys. Res. Lett. 44, 2481–2492. doi: 10.1002/2016GL072308
Stuecker, M. F., Timmermann, A., Jin, F. F., McGregor, S., and Ren, H. L. (2013). A combination mode of the annual cycle and the El Niño/Southern Oscillation. Nat. Geosci. 6, 540–544. doi: 10.1038/ngeo1826
Takaya, Y., Ishikawa, I., Kobayashi, C., Endo, H., and Ose, T. (2020). Enhanced Meiyu-Baiu rainfall in early summer 2020: Aftermath of the 2019 super IOD event. Geophys. Res. Lett. 47, e2020GL090671. doi: 10.1029/2020GL090671
Tang, H., Hu, K., Huang, G., Wang, Y., and Tao, W. (2022). Intensification and Northward extension of Northwest Pacific anomalous anticyclone in El Niño decaying mid-summer: an energetic perspective. Clim. Dyn. 58, 591–606. doi: 10.1007/s00382-021-05923-5
Taschetto, A. S., Ummenhofer, C. C., Stuecker, M. F., Dommenget, D., Ashok, K., Rodrigues, R. R., et al. (2020). ENSO atmospheric teleconnections. El Niño Southern Oscill. Chang. Clim. 309–335. doi: 10.1002/9781119548164.ch14
Terao, T., and Kubota, T. (2005). East-west SST contrast over the tropical oceans and the post El Niño western North Pacific summer monsoon. Geophys. Res. Lett. 32, L15706. doi: 10.1029/2005GL023010
Tokinaga, H., and Tanimoto, Y. (2004). Seasonal transition of SST anomalies in the tropical Indian Ocean during El Niño and Indian Ocean dipole years. J. Meteorol. Soc. Jpn. Ser. II. 82, 1007–1018. doi: 10.2151/jmsj.2004.1007
Tozuka, T., Yokoi, T., and Yamagata, T. (2010). A modeling study of interannual variations of the Seychelles Dome. J. Geophys. Res: Oceans. 115, C04005. doi: 10.1029/2009JC005547
Vialard, J., Duvel, J. P., Mcphaden, M. J., Bouruet-Aubertot, P., Ward, B., Key, E., et al. (2009). Supplement to cirene: Air—sea interactions in the seychelles—chagos thermocline ridge region. Bull. Am. Meteorol. Soc. 90, ES1–ES4. doi: 10.1175/2008BAMS2499.2
Vinayachandran, P. N., Francis, P. A., and Rao, S. A. (2009). Indian Ocean dipole: processes and impacts. Current Trends Sci. 46, 569–589.
Wang, B., and LinHo (2002). Rainy season of the Asian–Pacific summer monsoon. J. Clim. 15, 386–398. doi: 10.1175/1520-0442(2002)015<0386:RSOTAP>2.0.CO;2
Wang, B., Li, J., and He, Q. (2017). Variable and robust East Asian monsoon rainfall response to El Niño over the past 60 years (1957–2016). Adv. Atmos. Sci. 34, 1235–1248. doi: 10.1007/s00376-017-7016-3
Wang, B., and Li, T. (2004). “East Asian monsoon-ENSO interactions,” in East Asian Monsoon. p. 177–212. doi: 10.1142/9789812701411_0005
Wang, B., Wu, R., and Fu, X. (2000). Pacific–East Asian teleconnection: how does ENSO affect East Asian climate? J. Clim. 13, 1517–1536. doi: 10.1175/1520-0442(2000)013<1517:PEATHD>2.0.CO;2
Wang, B., Wu, R., and Li, T. I. M. (2003). Atmosphere–warm ocean interaction and its impacts on Asian–Australian monsoon variation. J. Clim. 16, 1195–1211. doi: 10.1175/1520-0442(2003)16<1195:AOIAII>2.0.CO;2
Wang, B., Xiang, B., and Lee, J. Y. (2013). Subtropical high predictability establishes a promising way for monsoon and tropical storm predictions. Proc. Natl. Acad. Sci. 110, 2718–2722. doi: 10.1073/pnas.1214626110
Wang, C. (2019). Three-ocean interactions and climate variability: a review and perspective. Clim. Dyn. 53, 5119–5136. doi: 10.1007/s00382-019-04930-x
Wang, C., and Wang, B. (2019). Tropical cyclone predictability shaped by western Pacific subtropical high: integration of trans-basin sea surface temperature effects. Clim. Dyn. 53, 2697–2714. doi: 10.1007/s00382-019-04651-1
Wang, C. Y., Xie, S. P., and Kosaka, Y. (2018). Indo-western Pacific climate variability: ENSO forcing and internal dynamics in a tropical Pacific pacemaker simulation. J. Clim. 31, 10123–10139. doi: 10.1175/JCLI-D-18-0203.1
Wang, C. Y., Xie, S. P., and Kosaka, Y. (2020). ENSO-unrelated variability in Indo–Northwest Pacific climate: Regional coupled Ocean–Atmospheric feedback. J. Clim. 33, 4095–4108. doi: 10.1175/JCLI-D-19-0426.1
Wang, G., and Cai, W. (2020). Two-year consecutive concurrences of positive Indian Ocean Dipole and Central Pacific El Niño preconditioned the 2019/2020 Australian “black summer” bushfires. Geosci. Lett. 7, 1–9. doi: 10.1186/s40562-020-00168-2
Webster, P. J., Moore, A. M., Loschnigg, J. P., and Leben, R. R. (1999). Coupled ocean–atmosphere dynamics in the Indian Ocean during 1997–98. Nature. 401, 356–360. doi: 10.1038/43848
Wu, B., Li, T., and Zhou, T. (2010). Asymmetry of atmospheric circulation anomalies over the western North Pacific between El Niño and La Niña. J. Clim. 23, 4807–4822. doi: 10.1175/2010JCLI3222.1
Wu, B., Zhou, T., and Li, T. (2009). Seasonally evolving dominant interannual variability modes of East Asian climate. J. Clim. 22, 2992–3005. doi: 10.1175/2008JCLI2710.1
Wu, B., Zhou, T., and Li, T. (2017a). Atmospheric dynamic and thermodynamic processes driving the western North Pacific anomalous anticyclone during El Niño. Part I: Maintenance mechanisms. J. Clim. 30, 9621–9635. doi: 10.1175/JCLI-D-16-0489.1
Wu, B., Zhou, T., and Li, T. (2017b). Atmospheric dynamic and thermodynamic processes driving the western north pacific anomalous anticyclone during El Niño. Part II: Formation Processes, J. Clim. 30, 9637–9650. doi: 10.1175/JCLI-D-16-0495.1
Wu, P., Ding, Y. H., and Liu, Y. J. (2017c). A new study of El Niño impacts on summertime water vapor transport and rainfall in China. Acta. Meteor. Sinica 75, 371–383.
Wu, R., Hu, Z. Z., and Kirtman, B. P. (2003). Evolution of ENSO-related rainfall anomalies in East Asia. J. Clim. 16, 3742–3758. doi: 10.1175/1520-0442(2003)016<3742:EOERAI>2.0.CO;2
Wu, R., Kirtman, B. P., and Krishnamurthy, V. (2008). An asymmetric mode of tropical Indian Ocean rainfall variability in boreal spring. J. Gophys. Res: Atmospheres. 113, D05104. doi: 10.1029/2007JD009316
Wu, R., and Yeh, S. W. (2010). A further study of the tropical Indian Ocean asymmetric mode in boreal spring. J. Gophys. Res: Atmospheres. 115, D08101. doi: 10.1029/2009JD012999
Wu, X., Li, G., Jiang, W., Long, S. M., and Lu, B. (2021). Asymmetric relationship between ENSO and the tropical Indian Ocean summer SST anomalies. J. Clim. 34, 5955–5969. doi: 10.1175/JCLI-D-20-0546.1
Xiang, B., Wang, B., Yu, W., and Xu, S. (2013). How can anomalous western North Pacific subtropical high intensify in late summer? Geophys. Res. Lett. 40, 2349–2354. doi: 10.1002/grl.50431
Xiao, F., Wang, D., and Leung, M. Y. T. (2020). Early and Extreme Warming in the South China Sea During 2015/2016: Role of an Unusual Indian Ocean Dipole Event. Geophys. Res. Lett. 47, e2020GL089936. doi: 10.1029/2020GL089936
Xie, P., and Arkin, P. A. (1997). Global precipitation: A 17-year monthly analysis based on gauge observations, satellite estimates, and numerical model outputs. Bull. Amer. Meteor. Soc., 78, 2539–2558. doi: 10.1175/1520-0477(1997)078<2539:GPAYMA>2.0.CO;2
Xie, S. P. (1996). Westward propagation of latitudinal asymmetry in a coupled ocean–atmosphere model. J. Atmos. Sci. 53, 3236-3250. doi: 10.1175/1520-0469(1996)053<3236:WPOLAI>2.0.CO;2
Xie, S. P., Annamalai, H., Schott, F. A., and McCreary, J. P. (2002). Structure and mechanisms of South Indian Ocean climate variability. J. Clim. 15, 864–878. doi: 10.1175/1520-0442(2002)015<0864:SAMOSI>2.0.CO;2
Xie, S. P., Hu, K., Hafner, J., Tokinaga, H., Du, Y., Huang, G., et al. (2009). Indian Ocean capacitor effect on Indo–western Pacific climate during the summer following El Niño. J. Clim. 22, 730–747. doi: 10.1175/2008JCLI2544.1
Xie, S. P., Kosaka, Y., Du, Y., Hu, K., Chowdary, J. S., and Huang, G. (2016). Indo-western Pacific Ocean capacitor and coherent climate anomalies in post-ENSO summer: A review. Adv. Atmos. Sci. 33, 411–432. doi: 10.1007/s00376-015-5192-6
Xie, S. P., and Philander, S. G. H. (1994). A coupled ocean-atmosphere model of relevance to the ITCZ in the eastern Pacific. Tellus. A. 46, 340–350. doi: 10.3402/tellusa.v46i4.15484
Yamagata, T., Behera, S. K., Rao, S. A., Guan, Z., Ashok, K., and Saji, H. N. (2003). Comments on “Dipoles, Temperature Gradients, and Tropical Climate Anomalies.” Bull. Am. Meteorol. Soc. 84, 1418–1422. doi: 10.1175/BAMS-84-10-1418
Yang, G., Liu, L., Zhao, X., Li, Y., Duan, Y., Liu, B., et al. (2019). Impacts of different types of ENSO events on thermocline variability in the southern tropical Indian Ocean. Geophys. Res. Lett. 46, 6775–6785. doi: 10.1029/2019GL082818
Yang, J., Liu, Q., and Liu, Z. (2010). Linking observations of the Asian Monsoon to the Indian Ocean SST: possible roles of indian ocean basin mode and dipole mode. J. Clim. 23, 5889–5902. doi: 10.1175/2010JCLI2962.1
Yang, J., Liu, Q., Xie, S.-P., Liu, Z., and Wu, L. (2007). Impact of the Indian Ocean SST basin mode on the Asian summer monsoon. Geophys. Res. Lett. 34. doi: 10.1029/2006GL028571
Yang, Y., Xie, S.-P., Wu, L., Kosaka, Y., Lau, N.-C., and Vecchi, G. A. (2015). Seasonality and predictability of the Indian Ocean Dipole Mode: ENSO Forcing and internal variability. J. Clim. 28, 8021–8036. doi: 10.1175/JCLI-D-15-0078.1
Yeh, S.-W., Cai, W., Min, S.-K., McPhaden, M. J., Dommenget, D., Dewitte, B., et al. (2018). ENSO Atmospheric Teleconnections and Their Response to Greenhouse Gas Forcing. Rev. Geophys. 56, 185–206. doi: 10.1002/2017RG000568
Yokoi, T., Tozuka, T., and Yamagata, T. (2008). Seasonal variation of the seychelles dome. J. Clim. 21, 3740–3754. doi: 10.1175/2008JCLI1957.1
Yokoi, T., Tozuka, T., and Yamagata, T. (2012). Seasonal and interannual variations of the SST above the seychelles dome. J. Clim. 25, 800–814. doi: 10.1175/JCLI-D-10-05001.1
Yoneyama, K., and Zhang, C. (2020). Years of the maritime continent. Geophys. Res. Lett. 47, e2020GL087182. doi: 10.1029/2020GL087182
Yuan, C., Liu, J., Luo, J.-J., and Guan, Z. (2019). Influences of tropical indian and pacific oceans on the interannual variations of precipitation in the early and late rainy seasons in South China. J. Clim. 32, 3681–3694. doi: 10.1175/JCLI-D-18-0588.1
Yuan, D., Zhou, H., and Zhao, X. (2013). Interannual climate variability over the tropical pacific ocean induced by the indian ocean dipole through the indonesian throughflow. J. Clim. 26, 2845–2861. doi: 10.1175/JCLI-D-12-00117.1
Yuan, Y., Yang, S., and Zhang, Z. (2012). Different evolutions of the philippine sea anticyclone between the eastern and central pacific El Niño: possible effects of Indian Ocean SST. J. Clim. 25, 7867–7883. doi: 10.1175/JCLI-D-12-00004.1
Zhan, R., Wang, Y., and Wu, C. C. (2011). Impact of SSTA in the east Indian Ocean on the frequency of northwest Pacific tropical cyclones: A regional atmospheric model study. J. Clim. 24, 6227–6242. doi: 10.1175/JCLI-D-10-05014.1
Zhang, L., Du, Y., and Cai, W. (2018). A spurious positive Indian Ocean Dipole in 2017. Sci. Bull. 63, 1170–1172. doi: 10.1016/j.scib.2018.08.001
Zhang, L., Du, Y., Tozuka, T., and Kido, S. (2021a). Revisiting ENSO impacts on the Indian Ocean SST based on a combined linear regression method. Acta. Oceanol. Sin. 40, 47–57. doi: 10.1007/s13131-021-1733-2
Zhang, L., Han, W., and Hu, Z. (2021b). Interbasin and multiple-time-scale interactions in generating the 2019 Extreme Indian Ocean Dipole. J. Clim. 34, 4553–4566. doi: 10.1175/JCLI-D-20-0760.1
Zhang, L. Y., Du, Y., Cai, W., Chen, Z., Tozuka, T., and Yu, J. Y. (2020). Triggering the Indian Ocean dipole from the southern hemisphere. Geophys. Res. Lett. 47, e2020GL088648. doi: 10.1029/2020GL088648
Zhang, R., Min, Q., and Su, J. (2017). Impact of El Niño on atmospheric circulations over East Asia and rainfall in China: Role of the anomalous western North Pacific anticyclone. Sci. China Earth Sci. 60, 1124–1132. doi: 10.1007/s11430-016-9026-x
Zhang, R., and Sumi, A. (2002). Moisture circulation over East Asia during El Niño episode in northern winter, spring and autumn. J. Meteorol. Soc. Japan. Ser. II. 80, 213–227. doi: 10.2151/jmsj.80.213
Zhang, W., Mao, W., Jiang, F., Stuecker, M. F., Jin, F. F., and Qi, L. (2021c). Tropical Indo-Pacific Compounding Thermal Conditions Drive the 2019 Australian Extreme Drought. Geophys. Res. Lett. 48, e2020GL090323. doi: 10.1029/2020GL090323
Zhang, Y., and Du, Y. (2021). Extreme IOD induced tropical Indian Ocean warming in 2020. Geosci. Lett. 8, 1–9. doi: 10.1186/s40562-020-00170-8
Zhang, Y., and Du, Y. (2022). Oceanic Rossby waves induced two types of ocean-atmosphere response and opposite Indian Ocean Dipole phases. J. Clim. 1–52. doi: 10.1175/JCLI-D-21-0426.1
Zheng, X.-T., Xie, S.-P., Vecchi, G. A., Liu, Q., and Hafner, J. (2010). Indian Ocean dipole response to global warming: Analysis of ocean-atmospheric feedbacks in a coupled model. J. Climate 23, 1240–1253. doi: 10.1175/2009JCLI3326.1
Zhou, Y., and Yu, R. (2005). Atmospheric water vapor transport associated with typical anomalous summer rainfall patterns in China. J. Geophys. Res. 110, D08104. doi: 10.1029/2004JD005413
Zhou, T., Wu, B., and Dong, L. (2014). Advances in research of ENSO changes and the associated impacts on Asian-Pacific climate. Asia. Pac. J. Atmos. Sci. 50, 405–422. doi: 10.1007/s13143-014-0043-4
Keywords: IPOC, ENSO - Indian monsoon teleconnections, Indian Ocean warming, western North Pacific anticyclone, IOD, oceanic Rossby wave
Citation: Du Y, Chen Z, Xie S-P, Zhang L, Zhang Y and Cai Y (2022) Drivers and characteristics of the Indo-western Pacific Ocean capacitor. Front. Clim. 4:1014138. doi: 10.3389/fclim.2022.1014138
Received: 08 August 2022; Accepted: 03 October 2022;
Published: 28 October 2022.
Edited by:
Carlos R. Mechoso, University of California, Los Angeles, United StatesReviewed by:
Weichen Tao, Institute of Atmospheric Physics (CAS), ChinaCopyright © 2022 Du, Chen, Xie, Zhang, Zhang and Cai. This is an open-access article distributed under the terms of the Creative Commons Attribution License (CC BY). The use, distribution or reproduction in other forums is permitted, provided the original author(s) and the copyright owner(s) are credited and that the original publication in this journal is cited, in accordance with accepted academic practice. No use, distribution or reproduction is permitted which does not comply with these terms.
*Correspondence: Yan Du, ZHV5YW5Ac2NzaW8uYWMuY24=
Disclaimer: All claims expressed in this article are solely those of the authors and do not necessarily represent those of their affiliated organizations, or those of the publisher, the editors and the reviewers. Any product that may be evaluated in this article or claim that may be made by its manufacturer is not guaranteed or endorsed by the publisher.
Research integrity at Frontiers
Learn more about the work of our research integrity team to safeguard the quality of each article we publish.