- 1Climate Service Center Germany (GERICS), Helmholtz-Zentrum Hereon, Hamburg, Germany
- 2Department of Geography and Atmospheric Science, University of Kansas, Lawrence, KS, United States
- 3National Disaster Management Office, Majuro, Marshall Islands
- 4Pacific Center for Environment and Sustainable Development, The University of the South Pacific, Suva, Fiji
- 5Climate and Environmental Physics, University of Bern, Bern, Switzerland
- 6Oeschger Centre for Climate Change Research, University of Bern, Bern, Switzerland
- 7CSIRO Oceans and Atmosphere, Aspendale, VIC, Australia
- 8Institute of Geography, Universität Hamburg, Hamburg, Germany
- 9Institute of Coastal Systems Analysis and Modeling, Helmholtz-Zentrum Hereon, Geesthacht, Germany
- 10Marine Policy and Coastal Management Department, EPOMEX Institute, University of Campeche, Campeche, Mexico
Perspectives for risk management and adaptation have received ample attention in the recent IPCC Special Report on Changes in the Oceans and Cryosphere (SROCC). However, several knowledge gaps on the impacts of abrupt changes, cascading effects and compound extreme climatic events have been identified, and need further research. We focus on specific climate change risks identified in the SROCC report, namely: changes in tropical and extratropical cyclones; marine heatwaves; extreme ENSO events; and abrupt changes in the Atlantic Meridional Overturning Circulation. Several of the socioeconomic impacts from these events are not yet well-understood, and the literature is also sparse on specific recommendations for integrated risk management and adaptation options to reduce such risks. Also, past research has mostly focussed on concepts that have seen little application to real-world cases. We discuss relevant research needs and priorities for improved social-ecological impact assessment related to these major physical changes in the climate and oceans. For example, harmonised approaches are needed to better understand impacts from compound events, and cascading impacts across systems. Such information is essential to inform options for adaptation, governance and decision-making. Finally, we highlight research needs for developing transformative adaptation options and their governance.
Introduction
Over the recent years, significant advances have been made in understanding the impact of human-induced climate change in order to understand potential risks for the planet and people, and options for reducing such risks through mitigation and adaptation. The Special Report on Oceans and Cryosphere in a Changing Climate (SROCC) (IPCC, 2019) made a landmark assessment of the current state of scientific understanding in this regard, with a focus on the oceans and cryosphere.
An important conclusion from the report is that with improved observations and modelling of climatic changes in the ocean and the cryosphere, natural system understanding has improved. However, the precise impacts of such changes on social and ecological systems, and in particular impacts from large-scale and abrupt changes, and how these can be managed and how adaptation can be planned, has received much less attention in research. As we will show, several studies have been devoted to observations and modelling of changes in the ocean circulation for instance, but the number of studies that assessed the economic impacts of regional climatic implications associated with such changes is very limited, let alone the number of studies that have proposed or developed in-depth adaptation options. Chapter 6 in the SROCC report focussed on “Extremes, Abrupt Changes and Managing Risks” (Collins et al., 2019) and discussed several observed and projected changes in the oceans, as well as impacts and risks, and adaptation and risk management options, from the available literature. This paper partly updates, but foremost extends that assessment, by focussing on gaps in our knowledge and subsequent research needs.
The integration of adaptation and risk management perspectives is essential, as these provide important information for societies about what to expect in terms of negative (and potential positive) consequences of these changes. In addition, scientific and actionable information on the socioeconomic consequences of these risks helps to provide opportunities to modify impacts, reduce risks and increase societal resilience. Overall, this information is underrepresented in the current literature and research efforts on abrupt and extreme changes in the ocean and cryosphere with, instead, a heavy focus on the climatological and oceanographic processes.
In this paper, we focus on the research gaps in the assessment of physical and socio-economic impacts from, and adaptation to, several abrupt changes reported in the SROCC chapter, and provide recommendations on how these gaps can be reduced. We refer to the current state of the art related to the assessment of impacts and risk management and adaptation options for socioeconomic impacts of several of the extreme and abrupt climatic changes identified in the SROCC report. These changes are:
• Tropical and extratropical cyclones;
• Marine heatwaves;
• Extreme El Niño Southern Oscillation events;
• Abrupt changes in the Atlantic Meridional Overturning Circulation.
In addition, we discuss the overarching challenges related to the assessment on impacts and risks from compound events and cascading impacts; and transformational adaptation, and governance of transformations, in the context of changes in oceans and cryosphere. We draw on the literature that was included in the assessment for the SROCC report, but also refer to newer literature published since. We highlight the key knowledge gaps and research needs for these four extreme and abrupt changes in climate and oceans. The conclusions of this paper are summarised in Table 1, below.
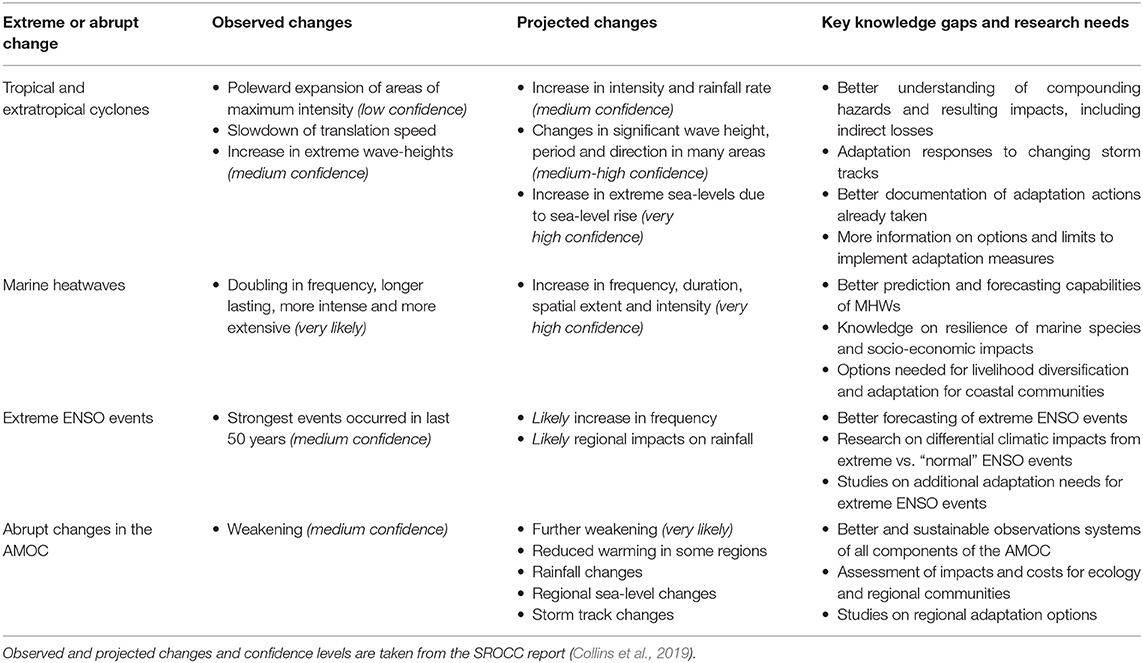
Table 1. Summary of extreme or abrupt changes in oceans and climate considered in this article, including observed and projected changes, and key knowledge gaps and research needs.
This paper is structured as follows: We first discuss the current state of knowledge on risks, impacts and adaptation for the four extreme and abrupt changes listed above. In those sections, we critically discuss relevant publications on risk management and adaptation followed by knowledge gaps and resulting research priorities. Next, we discuss compound events and cascading impacts; as well as resilient pathways and transformative governance in two separate sections. Finally, we provide some conclusions.
Changes in Tropical and Extratropical Cyclones
Tropical and extratropical cyclones are important extremes related to climatic variability and change. Cyclones have a large influence on sea-surface dynamics, and potential changes in these weather systems influence the frequency of high surge events and waves, as well as extreme rainfall events. These events can lead to large physical and socioeconomic impacts, through storm damages as well as flooding caused by storm surge, waves, and extreme rainfall.
Climatic Impacts
Several studies have shown that past changes in tropical cyclones include a poleward expansion of the area of maximum cyclone intensity in recent decades, and a slow-down of the tropical cyclone translation speeds, potentially leading to greater flood hazards. Regionally, there are areas that have experienced an increase in more intense tropical cyclones, no change, or decreases. Although there is still much uncertainty over past changes in tropical cyclone activity, there is some certainty that future cyclone intensities may increase. For future climate change, it was concluded that the future global tropical cyclone frequency will likely either decrease or remain unchanged, and that future global mean maximum tropical cyclone wind speed and precipitation rates will likely increase (Collins et al., 2019: Section 6.3.1.1). Since SROCC new studies have further examined cyclone trends over the observational period. Using an extended tropical cyclone intensity record from 1979 to 2017, (Kossin et al., 2020) and found a statistically significant 5% per decade increasing trend in the exceedance probability of category 3–5 cyclones. Wang and Toumi (2021) found that the proximity of the maximum intensity of tropical cyclones to land decreased by about 30 km per decade over the period 1982–2018, associated with a poleward and westward shift in cyclone occurrence, which was due to changes in the atmospheric steering flows rather than changes in tropical cyclone translation speed or genesis locations. Similarly, in the future, the poleward shift of westerlies projected by climate models leads to a reduction in the translation speed of TCs (Zhang et al., 2020). However, this reduction in translation speed of tropical cyclones is compensated by an increase in the relative frequency of tropical cyclones in higher latitudes (Yamaguchi et al., 2020).
For storm surges, it is expected that the effect of sea-level rise will dominate the projected increase in surge height, compared to the contribution of projected increases in maximum cyclone wind speed.
For extra-tropical cyclones, a small poleward shift is projected but the magnitude is uncertain. In some basins increases have been observed in significant wave heights and associated wave power, as well as increasing extreme sea levels that are associated with sea-level rise. Few studies have looked at changes in wave direction which are also important for coastal processes. A recent assessment of a multi-method ensemble of global wave climate projections for the end of the century under a high emissions scenario finds consistent changes in wave height, period and/or direction to about 50% of the world's coastline, and 40% to at least two variables (Morim et al., 2019). The ranges of change are 5–15% for significant wave height and mean wave period and 5–15° for direction (Morim et al., 2019). The combined changes in wave height and period have been shown to amount to coherent changes in the contribution of wave setup to extreme sea levels along global coastlines. Increases are projected for the western coasts of North and South America, the western coast of southern Africa, the southern coast of Australia, the western coast of India, most of the eastern coasts of the Indian Ocean, and the western coast of New Zealand. On the other hand, decreases are projected along the western coast of Europe and northwestern Africa, the US east coast and the northeastern coast of South America, most of the Mediterranean coasts, the tropical and subtropical western Pacific coasts, the east coast of New Zealand, most of the west coasts of the Indian Ocean, and the western coasts of the Tropical Atlantic Ocean (Melet et al., 2020).
Impacts on Ecology, Humans, and Economy
The SROCC reports the loss of habitats and marine life, human lives, and property from tropical cyclones and related coastal impacts. The magnitude of loss and associated economic and social costs depend on geographic variations of exposure, vulnerability, and adaptive capacity. Overall, it has been shown that property losses from cyclones (mostly wind-related damage, but also surge-related flood damage) have increased as a consequence of increasing property values in coastal zones (e.g., Weinkle et al., 2018). At the same time, mortality from surge related flooding has decreased over time around the world, because of improvements in forecasting, evacuation, and coastal protection (Bouwer and Jonkman, 2018).
As tropical cyclones are projected to change their track along with their frequency and intensity, impacts are increasingly difficult to discern in advance. In addition, it has been a challenge to distinguish between the direct and indirect impacts of cyclones. Indirect impacts are those outside the affected area, or relate to cumulative costs and impacts after the event. While several efforts such as the build-up of new models and economic databases have been made to improve the assessment of indirect impacts (e.g., Koks et al., 2019), there are still many uncertainties. The lack of common and agreed-upon methods and the varying magnitude of the cyclones create challenges to assess indirect impacts, and variable sizes of indirect impacts that are observed in different events.
Risk Management and Adaptation Options
Protection and prevention of risks related to cyclones have a long history, ranging from coastal protection, building codes and land-use zoning, to forecasting and early warning. Early warning systems coupled with forecasting and evacuation help to reduce death and adverse health impacts globally due to tropical cyclones and coastal flooding (Fakhruddin and Schick, 2019; Johnson et al., 2020). Efforts are also made to move beyond the individual basin forecasts by modelling such hazards at the global scale (Bloemendaal et al., 2019). With tropical cyclones that change tracks (Hall and Sobel, 2013; Lejano et al., 2016) it has been difficult to generate timely warnings or warnings easily understood and acted upon by the affected population.
Armoured coasts and natural barriers provide protection for people and property. They are, however, susceptible to the rising sea level and changing paths of the storm. Sometimes protective measures such as seawall construction can lead to adverse impacts on social-ecological systems leading to maladaptation (David et al., 2021). Using ecosystem-based adaptation such as natural vegetation barriers is promoted as an alternative but is difficult to implement (Nalau et al., 2018; Donatti et al., 2020) and its effectiveness is often more variable than traditional coastal protection. Relocation including managed retreat is the least favoured but a possible long-term adaptation option (Carey, 2020; Cutler et al., 2020; Mach and Siders, 2021). Efforts to relocate people after Superstorm Sandy and Typhoon Haiyan, for instance, were fraught with political and social obstacles that led to distributional and human rights issues associated with place attachment (Binder et al., 2019; Fitzpatrick and Compton, 2019; Ensor et al., 2021).
Gaps and Research Needs
Most previous studies have focussed on assessment of current and future hazards and risks, as well as potential adaptation options and their evaluation. To improve the knowledge base, more information about the success of actual adaptation implementation is needed. For instance, more detailed documentation of the local-level implementation of adaptation specific to coastal inundations can be collected, as there are still many gaps in our understanding of the best available options and approaches from technical, financial, legal, and governance perspectives. These include the current level of adaptation (Thomas et al., 2021) or lack thereof in many world regions, as well as potential options and limits to implement such measures. In addition, literature on anticipating social-ecological responses to unprecedented storms that change tracks, frequency, and intensity is scarce, and these issues need to be examined further. There is a strong need for interdisciplinary perspectives on the interaction of natural dynamics, societal demands for adaptation and risk reduction, and political decisions. They are crucial in providing insights on the potential impacts of physical changes such as wave action, erosion hazards and sediment accretion potential and the socio-political dimensions of vulnerabilities and adaptive capacities of people and places.
Marine Heatwaves
Marine heatwaves (MHWs) as defined in SROCC are “periods of extremely high ocean temperatures that persist for days to months, can extend up to thousands of kilometres and can penetrate multiple hundreds of metres into the deep ocean” (Collins et al., 2019, p. 607; Hobday et al., 2016). Although MHWs are triggered by natural variability, the SROCC report clearly indicated that their long-term decadal-scale increase in number and intensity is very likely caused by human-induced global warming (Frölicher and Gruber, 2018; Collins et al., 2019; Oliver, 2019); a conclusion reinforced by a 2020 study examining seven of the highest-impact marine heatwaves since 1981, concluding that the occurrence of all but one were at least partially due to human-driven ocean warming (Laufkötter et al., 2020). Under further global warming, MHWs are projected to become more frequent, longer, and more intense (Frölicher and Gruber, 2018).
Climatic Impacts
MHWs have been observed to impact the climate on lands, either close or away from their area of occurrence via teleconnections (e.g., Rodrigues et al., 2019). Impacts include extreme precipitation and flooding, for example in Tasmania in 2016 and the west coast of tropical South America in 2017 associated with a MHW in the Tasman Sea and Peru coastal area, respectively. However, a MHW in the northern Pacific Ocean in 2013–2015 was considered to have prevented winter storms from reaching the west coast of the US, leading to a drought in the entire region (Seager et al., 2015). Climate models suggest that such co-occurring drought and MHW events are projected to become more frequent under global warming (Shi et al., 2021). In the Mediterranean Seas, MHWs are often associated with increased precipitation and heatwaves in Central Europe (Collins et al., 2019). It should be noted that other climate processes may influence the impacts of MHWs; as a result, it is difficult to attribute these impacts only to MHWs (Collins et al., 2019).
Impacts on Ecology, Humans and Economy
Coral reefs and kelp forests are two iconic marine ecosystems that are often strongly affected by MHWs (Hughes et al., 2018; Smale et al., 2019). Bleaching, disease, and mortality in coral reefs regularly occur during or directly following MHWs. In recent years, several severe bleaching events in the tropical ocean were associated with extremely hot temperature events. For example, during an extreme temperature event in 2014–2017, 75% of the reefs located in the tropics bleached and the mortality reached 30% (Collins et al., 2019). The intensity of the bleaching is closely related to the temperature during the MHW and can vary geographically. The bleaching of coral reefs has strong implications for other species associated with this ecosystem, including fish and marine invertebrates that are essential for subsistence fishing and livelihood in many coastal communities in the tropics (Collins et al., 2019). In addition, coral reefs are an essential asset for the tourism industry in many tropical countries, and longer, more frequent, and severe bleaching events in combination with other stressors (Klein et al., in press) have significant consequences on the incomes of these countries (Doshi et al., 2012). In more temperate waters, kelp forests have diminished in response to MHWs and are replaced by small, turf forming algae (Wernberg et al., 2016). Similar to what is observed in coral reefs, these changes have significant impacts on the fish and invertebrate species associated with this ecosystem.
Many other marine organisms and ecosystems, both in coastal and open-ocean areas, including seabird populations (Piatt et al., 2020), can be strongly affected by MHWs. Generally, MHWs result in loss in biodiversity and genetic diversity, change in species distribution (Cheung and Frölicher, 2020), behaviour or performance leading to economic loss from changes in fishery catch (Cheung et al., 2021), decrease in adaptive capacity of fish and mortality or reduced production in aquaculture (see Smale et al., 2019; Holbrook et al., 2020). These impacts may either come from a change in species population as highlighted in the examples above, or may also result from blooms of harmful microorganisms. These microorganisms such as vibrio, Pseudo-nitzschia, and other harmful algae produce toxins that migrate through the food chain and generate food poisoning in humans (Collins et al., 2019).
For example, the lobster fishery in the Northwest Atlantic was severely impacted by a MHW in 2012, resulting in a collapse of lobster prices due to increased catch at a period when demand was low. Closure of fisheries due to harmful microorganisms blooms during or after a MHW were observed along the North American West Coast, the Baltic Sea, Alaskan Sea and Coastal Peru (Collins et al., 2019).
Since the publication of the SROCC, additional research suggests that globally the potential catch of fisheries will drop by 6 percent per year and 77 percent of exploited fishes and invertebrates will decrease in biomass during extremely hot years. This will occur on top of the projected decrease in fish stocks from long-term climate change (Cheung et al., 2021). However, at the regional scale, the impacts of MHWs on fisheries can be diverse. In the Northeast Pacific, the biomass of 22 exploited fish stocks is expected to decrease on average by almost 3% during MHWs with the greatest projected decrease at 20% (Cheung and Frölicher, 2020), which is consistent with the impacts observed during the 2013–2014 MHW, called the blob (Cavole et al., 2016). However, in the Great Barrier Reef, catches of coral trout (Plectropomus and Variola spp.) have increased by 18% for any given level of fishing effort during the 2016 MHW event (Brown et al., 2021).
Risk Management and Adaptation Options
The SROCC report indicated that risk management for MHWs relies on monitoring and forecasting (Collins et al., 2019), such as the NOAA Coral Reef Watch tool (Liu et al., 2014). Forecasting and predictions of MHWs on seasonal to interannual timescales is essential to support risk management and adaptation to the projected increase in frequency, intensity and duration of MHWs. The improvement of forecasting capacity relies on better understanding and modelling of the drivers of MHWs and associated changes in ocean biogeochemistry and ecosystems (Holbrook et al., 2020; Sen Gupta et al., 2020). However, for decision makers and stakeholders, the current models are not sophisticated enough and cannot provide information at fine-enough resolution to inform the kinds of policy decisions they need to prepare for a potential MHW (Viglioni, 2021). Dissemination of MHW forecasts, early warning and information to all interested stakeholders is essential to give them time to implement risk reduction measures. Moreover, the unpredictability of some extreme events needs to be considered when planning for adaptations to long-term climate change. In addition, appropriate decision-making processes, including exploration of options, and necessary governance frameworks are also needed to make adaptation actions effective, besides the provision of better risk information.
Active fisheries management, including adjusting catch quotas in years when fish stocks are suffering from extreme temperature events, may be vital, especially in regions with limited capacity to provide robust scientific predictions. Other tools to manage the risks and adapt to MHWs is the identification of refugia, where the water temperature increases less than in surrounding areas and that can provide some respite to marine organisms (Ainsworth T. D. et al., 2020). Development of marine protected areas, protection of marine ecosystems from additional stressors such as overfishing, eutrophication and pollution can also help marine organisms to survive MHWs. More active interventions, including habitat restoration or rehabilitation, assisted migration, species selection based on their resilience to MHWs, selection of resilient individuals from warm habitat and their relocation in degraded ecosystems, cross breeding with resilient species or genetic engineering are also part of a range of options, although they all have limitations and face challenges (Ainsworth T. D. et al., 2020; Holbrook et al., 2020).
Efforts are also on-going to decrease surface water temperature locally by shading areas or pumping deeper water (from 20 to 40 m deep) and mixing it with surface water. However, although theoretically interesting, some uncertainties remain (e.g., how to achieve the degree of mixing necessary to sufficiently decrease sea surface temperature), and risks associated with these methods exist, making their implementation difficult (Ainsworth T. D. et al., 2020).
Gaps and Research Needs
As mentioned in section Risk Management and Adaptation Options, the current models for MHW modelling and prediction or forecasting do not allow for precise early warning of local MHWs, although some tools exist such as NOAA's Coral Reef Watch and these can be improved. Also, current process understanding and modelling capacities are insufficient for projecting future locations of changes in MHWs, to allow adaptation planning. Research needs in this area include better understanding of the local and global drivers of MHWs and the generation of baseline information; that is: the natural background and frequency of these high water temperature events for specific locations. Although the response to specific ecosystems or species are studied, uncertainties remain regarding adaptive capacity of some species. For example, it seems that the adjustment in fatty acid composition in algae species allows them to be more resilient to MHWs (Britton et al., 2020). More research is needed to identify resilient species and adaptive capacity that can be used in different environments. In addition, research is needed on other options that are available for coastal communities to adapt, beyond introducing resilient species, including livelihood diversification and other options for local resilience with regard to MHW events.
Extreme El Niño Southern Oscillation Events
Although no formally accepted definition exists, extreme El Niño Southern Oscillation (ENSO) events have been proposed to be characterised by a pronounced eastward extension of the west Pacific warm pool and development of atmospheric convection, and a rainfall increase of >5 mm per day during December to February (above 90th percentile), in the usually cold and dry equatorial eastern Pacific (Niño 3 region, or 150–90°W, 5°S−5°N) (Collins et al., 2019). Although ENSO response to greenhouse forcing is variable and uncertain, models that better simulate the non-linear characteristics of ENSO project increased frequency of extreme El Niño and La Niña events in a changing climate (Cai et al., 2020). The SROCC highlighted the lack of literature on extreme ENSO events, although the strong 2015–2016 event generated additional literature (Collins et al., 2019). Since the SROCC, additional literature has been published to improve our understanding of extreme ENSO events but knowledge gaps still remain.
Climatic Impacts
Impacts from extreme El Niño, in particular from the 2015–2016 event, include increased variability of rainfall, severe weather events, and tropical cyclone activity, extreme temperatures in Southern Asia and impacts on the atmospheric CO2 cycle (Collins et al., 2019). Since this report, additional impacts of extreme El Niño events have been reported, including reduced spring coldness in northern America (Zhou et al., 2021); dry conditions in Western, Central and Northern Europe (King et al., 2020); flooding in Peru, Bolivia, Argentina, Angola, and Indonesia, heatwaves in California, and wet conditions in Seattle (USA) (Emerton et al., 2017).
These last two studies also indicate that the impact of extreme El Niño events either differ from what was expected (e.g., flooding in Indonesia while dry conditions were expected–Emerton et al., 2017) or the impacts do not correspond to an intensification of the impacts observed during strong El Niño events (e.g., dry conditions in Western Europe during extreme El Niño events, but rather wetter conditions during strong El Niño events over the same region—King et al., 2020), suggesting a non-linear relation between the intensity and the impacts of the El Niño events. However, it is difficult to conclude whether these differences are specific to the 2015–2016 El Niño event, or if they can be generalised to all extreme El Niño events.
Impacts on Ecology, Humans, and Economy
Ecological, agricultural and socioeconomic impacts from ENSO events have been widely studied and reported, and have been addressed in previous IPCC special reports, such as SREX (see e.g., Handmer et al., 2012). The study of extreme impacts beyond the natural variations, has received less attention. The SROCC report (Collins et al., 2019) mentioned impacts of extreme El Niño events on marine ecosystems, in particular more frequent intense coral bleaching and decrease in equatorial upwelling intensity. Terrestrial ecosystems are also impacted, in particular through the changes in rainfall patterns leading to intense fires, for example in Indonesia, and decrease in agricultural yields in other regions. Also intensified rainfall leads to river flooding, flash floods and mass movements. Such climatic events from ENSO can have severe consequences for agriculture, infrastructure and the built environment, and for human health and local and national economies (Collins et al., 2019).
A recent study indicates that the extreme 2015–2016 El Niño event affected sea turtle species nesting on Costa Rica beaches but with significant differences between species (Santidrián Tomillo et al., 2020). Another study in Guatemala however showed no variation in sea turtle nesting related to ENSO variability over a 16-year period (Ariano-Sánchez et al., 2020). Limited additional knowledge on ecological or socioeconomic impacts from extreme ENSO has been published since SROCC.
Risk Management and Adaptation Options
Obviously, many of the approaches, including forecasting early warning, and hedging against El Niño events and their impacts have benefits also for extreme El Niño events that we discuss here. Such approaches have been highlighted by many studies and assessments (IPCC, 2012). Also new studies have proposed the use of forecasts for preparations to reduce damages in households (Aguirre et al., 2019) and (financial) planning of responses (Coughlan de Perez et al., 2015). Below, we discuss a few lines of options for managing the risks of extreme ENSO events.
SROCC did not provide specific risk management and adaptation options for extreme ENSO events but highlighted the need to closely monitor the ENSO precursors to improve the forecasting of these events, in order to enable early warning and risk reduction measures (Collins et al., 2019). While much progress has been made in improving predictions and developing more sophisticated prediction models for ENSO (Tang et al., 2018), there is evidence that the predictability of recent severe ENSO events has in fact declined (Zhang et al., 2021), making the practical usefulness of the predictions for early warning and risk reduction responses more limited. Also, there are indications as mentioned above, that extreme ENSO events may have opposite climatic and consequential socioeconomic effects in some regions, making prediction and prevention of such impacts more challenging there.
Progress on our understanding of the impacts of extreme ENSO events helps to identify risk management and adaptation options; however, they are limited, and uncertainties remain. In particular, it was noted that each El Niño event generates a specific combination of impacts, making generalisations of what is observed or simulated for one event difficult. The recent information about the different impacts of strong and extreme El Niño events indicates that risk management strategies for extreme ENSO events cannot be limited to an intensification of the options in place for “normal” or strong events.
Gaps and Research Needs
Research needs for extreme El Niño events include the better forecasting of such events, in order to allow better prediction of regional climatic impacts and early warning for response measures, including for water resources planning, agriculture and crop production, and regional food supplies. Also, more research into the effects and impacts of past extreme El Niño events helps to better understand current and future risks, and allows communities to better prepare and plan adaptation measures. For example, research may show whether past impacts in extreme El Niño events are simply more intense changes in rainfall surplus or deficits, or whether such effects on temperature and rainfall are fundamentally different in localities exposed to these effects.
Abrupt Changes in the Atlantic Meridional Overturning Circulation
Observational and modelling studies have indicated that global warming could lead to weakening of the Atlantic Meridional Overturning Circulation (AMOC), which is a key circulation mechanism in the Earth's climate system (e.g., Caesar et al., 2018; Weijer et al., 2020; Boers, 2021). Changes in this system can have important implications for the global and local climate systems, with several detrimental effects. The SROCC report has summarised the state of understanding changes in the AMOC, and concluded that although collapse is deemed very unlikely over the twenty-first century, a substantial weakening may occur with many potential impacts. The SROCC report summarised the past research into this topic, and while several studies since then have confirmed earlier modelling and observation studies into a potentially weakening (e.g., Boers, 2021; Caesar et al., 2021), limited new insights have emerged, especially on the socio-economic impacts of a change in the circulation and possible management options, for which understanding is still limited.
Climatic Impacts
Several studies are available that have studied the impact of AMOC variations in the North Atlantic, and in the Gulf region. The most important climatic impacts of a reduction of the AMOC were included in Collins et al. (2019): section 6.7.2, and included shifts in storm track position and intensity over Europe, potential increase in the number of storms, possible changes in rainfall patterns on the British Isles, a higher probability of cold events in winter, and higher heat wave probabilities in summer. A decline in AMOC can also lead to a rapid warming in the Gulf region and a decrease in the tropical cyclone frequency, increased sea-levels on the European and US Atlantic coasts, potentially increasing coastal flood risks. Drought incidence could increase in Africa and South America, and more remote climatic impacts include a potential weakening of the monsoon in Asia.
Finally, a collapse of the AMOC may lead to other abrupt changes globally, although these are highly uncertain. Such impacts can include changes in ENSO characteristics, impacts on the Amazon rainforest, impact on melting of the West Antarctic Ice Sheet, warming of the Southern Ocean, and southern migration of the Intertropical Convergence Zone (Collins et al., 2019, section 6.7.2). The AMOC weakening can potentially also lead to a positive carbon cycle-climate feedback, as less of the anthropogenic carbon emissions may be taken up by the ocean and the terrestrial biosphere due to the weakening and associated changes in rain patterns, albeit with large uncertainties.
Impacts on Ecology, Humans, and Economy
The number of studies concerning the economical and societal impacts of a rapid shift of the AMOC is too small to make any comprehensive assessment on the overall potential societal impacts and costs. The SROCC reported that available sector studies indicate lower agricultural production in Mexico and Africa (Defrance et al., 2017; Azuz-Adeath et al., 2019); and impacts on marine ecosystems, in response to changes in upper-ocean nutrient content and dissolved oxygen concentration (Collins et al., 2019: section 6.7.2).
Only a few studies have evaluated wider-scale impacts of an AMOC slow-down on human systems. For instance, Kopits et al. (2014) reviewed existing studies. Economic studies have indicated that anticipated reduced warming in some regions in response to a reduction in the AMOC can lead to overall global benefits (Anthoff et al., 2016). Economic studies of fisheries show net losses for instance for the Barents Sea (Link and Tol, 2009). Other economic studies show that local losses can account for losses in the order of a few percent in GDP, albeit under a complete shutdown (Link and Tol, 2011).
New studies since the SROCC report have focussed on sectoral impacts, such as food production. For instance, the study by Ritchie et al. (2020) estimates the losses for the UK from cooling and reduced rainfall in response to a slowdown of AMOC to be larger than the impacts from climate change without such a slowdown, and costs for additional irrigation to compensate for such changes to be prohibitively large. Global estimates for such losses, or more integrated cross-sectoral analyses are however still lacking.
Risk Management and Adaptation Options
While much work has been done on assessing changes and variability of the AMOC system, and also on climatic and some of the socioeconomic impacts, less information is available on potential adaptation and risk management options. An obvious option for improved risk management is the improvement of monitoring and early warning of AMOC slow-down, including decadal prediction systems. Related to this could be systems to project or predict changes in rainfall and temperature, especially rainfall declines over Europe and Africa. However, there are problems of predictability because of large and poorly-understood natural variability within the system. Another challenge is to match the needs of users for prediction information, for instance on regional or local rainfall and temperature, to the temporal and spatial scales of available prediction systems.
At a strategic level, more risk assessment studies and integral frameworks for adaptation planning would help to improve risk management for AMOC changes, including assessment of sectoral adaptation options and cross-sectoral trade-offs. Especially with regard to the following expected impacts: drying and cooling conditions with impacts on agricultural production, ecology, and energy production. Also to be included are possible changes in extreme events, including regional sea-level rise, and changes in storminess, with possible impacts for coastal and wind damage risks.
Gaps and Research Needs
A stable and long-term observational system in the Atlantic is a prerequisite in order to be able to measure the state and changes of the circulation reliably and continuously. Despite the Global Ocean Observing System (GOOS) that has been installed, there is a lack of financial and institutional possibilities to further develop and sustainably operate long-term observations. Circulation measurements are only available selectively and cannot be easily integrated into models in order to determine more reliable indicators and statements about the variability of the Atlantic circulation and to make them available to decision-makers in a timely manner as a secure basis of information. A longer-term observation and analysis system can help to address open questions, including: How strong are the different components of the Atlantic circulation at present? Are fluctuations in the circulation interconnected? To what extent are such changes linked to human-induced climate change? What are the effects in the different marine and coastal regions across the Atlantic?
Comparatively little research has been done on the effects and costs for ecology and human communities, impacted by several regional climatic changes that are the result of changes in the overturning circulation. Such studies until now are limited to studies on ecological effects for fish species and other marine organisms and only few integrated or sectoral studies exist for wider production systems and economics effects (see section Impacts on Ecology, Humans, and Economy). Studies that have looked into the wider economic effect have above all emphasised the reduction in warming (section Impacts on Ecology, Humans, and Economy), leading to potential benefits compared to warming scenarios without such changes in overturning circulation, often without accounting for effects of other climatic changes. More integrated approaches are needed, to look into several sectoral effects related to many potential regional and local climate effects.
In addition, studies that target potential adaptation options, as well as integration of the climatic effects of overturning circulation changes in national climate change scenarios in potentially affected regions are missing; yet such changes could potentially be severe, affecting rainfall patterns, coastal water levels and storm tracks in many locations.
Compound Events and Cascading Impacts
There is a tradition of studying possible risk reduction and adaptation options, and strategies. These include hard and soft measures, such as structural protection as well as risk transfer and insurance systems, and these have been covered in recent assessment reports (IPCC, 2012, 2019). More recently, compound and cascading events have received particular attention, as risk multipliers that have to be considered when designing response measures and strategies. Compound events are defined as multiple weather and/or climate related events (hazards) that combine to challenge the capacity of natural and/or human systems (impact) (Leonard et al., 2013; Zscheischler et al., 2018, 2020). Although the impact of the single event might not necessarily be extreme, the impact of such combined multiple climate drivers and/or hazards is extreme, and contributes to societal and environmental risks. The single events either occur at the same time; or in sequence. Examples include; consecutive tropical cyclones, or a combination of wind-driven surge and rainfall. Combined, these cause increased flooding in the same geographic location; or at multiple locations within a given country or around the world and drive impacts such as reductions yields in different locations due to different causes: drought and flooding. The combination of the multiple hazards can also lead to cascades of impact that unfold and potentially impact across economic and societal realms.
For example, in 2018, heavy rains that fell one month after wildfires on steep slopes in southern California caused fatal mudflows that otherwise possibly would not have been as devastating (Lancaster et al., 2021). A wet winter with extensive plant growth followed by a hot and dry summer can increase the fire risk due to the build-up of fine fuels and shrubs. Extreme precipitation in low-lying coastal areas might overwhelm the existing drainage system or add to the rises in ground water level. Even the traditionally safe and massive dikes along rivers and coasts can become weak points of protection under drought conditions, when dike materials may potentially degrade and subsequently breach under extreme conditions. Also across social and economic systems, impacts can lead to a cycle of vulnerability (Reichstein et al., 2021). Such cascades across different natural and social systems have received increasing academic attention only in the past few years. However, the social aspects, in particular an integrative perspective of risk management and adaptation measures, are still not well-understood (Scolobig et al., 2017; Zscheischler et al., 2020; De Ruiter et al., 2021a).
Definition and Research Approaches
Compound events are extremes with additive or even multiplicative effects (Kopp et al., 2017). Compound events can result either from shared forcing factors (e.g., ENSO), large-scale circulation patterns, or greater regional sensitivity to global change. And compound events may well result from mutually reinforcing cycles between individual events, such as the relationship between drought and heat, linked through soil moisture and evaporation, in water-limited areas (IPCC, 2012; Kopp et al., 2017).
In the SROCC report, compound events and cascading impacts were spelled out as examples of deep uncertainty—due not only to data deficiency, often preventing the assessment of probabilities and consequences of the risks, but also, due to the missing incorporation of climate processes in the climate models that can contribute to feedbacks, compound extreme events, and abrupt and/or irreversible changes (Kopp et al., 2017). Climate drivers that contribute to compound events could cross tipping-points in the future (Collins et al., 2019).
There is a need to better understand compound events, improve the projections of potential high-impact events, and consider an integrated view of detection and attribution across climate scientists, engineers, social scientists, and decision-makers, who need to work closely together to understand these complex events and their impacts on socio-ecological systems.
Findings From the Recent Literature
Zscheischler et al. (2020) distinguish four types of compound events: (i) preconditioned, where a weather-driven or climate-driven precondition aggravates the impacts of a hazard; (ii) multivariate, where multiple drivers and/or hazards lead to an impact; (iii) temporally compounding, where a succession of hazards leads to an impact; and (iv) spatially compounding, where hazards in multiple connected locations cause an aggregated impact. And they emphasise the need for a new perspective of analysing compound events rather from an impact perspective than a hazard perspective. Not “what is physically plausible” but “what impacts are conceivable” “thinkable”? Rather than scrutinising if the co-occurrence is more likely than expected by pure chance, scrutinising the possible occurrence and impacts by the combination of hazards and their cascading effects is important (AghaKouchak et al., 2020). New advances have also been made in characterising, modelling and understanding compound events in the open ocean. Gruber et al. (2021) stressed that compound physical and biogeochemical ocean extreme events, such as co-occurring marine heatwaves, high acidity, low oxygen, and low nutrient events may be a particular stress for marine life and Le Grix et al. (2021) show that such high temperature and low nutrient events are particularly frequent in the equatorial Pacific.
There is little work addressing the practical challenges of managing compound events and cascading impacts. Khanam et al. (2021) show how the impact of compound flood events needs a combined consideration of the current climatic conditions, expected single impact events and as well the co-occurrence of compound drivers resulting in more extreme impacts on critical infrastructure. Such infrastructure is currently designed to function under outdated climatic circumstances, and is therefore operating outside of its tolerance levels. De Ruiter et al. (2020) address shortcomings in current modelling approaches for consecutive events, and provide a framework for improving this.
Gaps and Research Needs
Several gaps exist in the field of compound and cascading events and impacts. We list the following important research needs to fill these gaps, related to compound events, outcomes, and risk management strategies.
Multiple drivers of compound events have to be investigated, some combinations of events may not have historical analogues, which requires more research into the probability and hazards of these events (Zscheischler et al., 2018; Gruber et al., 2021). Understanding risks from hazards and risks that have not (yet) materialised in the real world are also important to consider, given their importance for the actual risk, including possible cascade and compound impacts, as well as consequent adaptation needs (Lin et al., 2020). There is also a lack of the understanding of the socioeconomic impacts of compound risks and cascading effects, compared to single risks. A key issue obviously is to identify those compound events that have a drastic increase of risks beyond the addition of two outcomes, some of which may show that there are threshold effects.
For cascading impact events, there is literature on theory, especially for infrastructure and networks in which impacts propagate, but there are very few studies on actual impacts from such events, let alone for adaptive responses. Cascading effects across societies emanating from extreme events are rarely considered in most countries' strategies for risk reduction and climate change adaptation, but need to be integrated in future strategies to address such compound events.
Human activities, especially urbanisation and critical infrastructure development, will interact with compound events, leading to increased risks. Studying these risks requires interdisciplinary collaboration to increase the understanding of compound events and their impacts on vulnerable communities. A potentially increasing role could be played by big data and artificial intelligence (AI) to assess such risks: Reichstein et al. (2021) argue that AI techniques and big data could help improve models of environment and agent interactions and build more trust in these complex models.
What has become obvious during the last decades of disaster prevention and risk management is that disasters are culturally sensitive and place-specific so more is needed than big-data efforts on an international or national level. Multi-stakeholder involvement and transdisciplinary co-evolution of adaptation measures is crucial for dealing with current and future extreme events. The preparation for an extreme event should include the strengthening of the actors involved in disaster risk reduction and adaptation. In transdisciplinary collaboration between science and actors, information needs must be identified and processed in an action-oriented manner, and precautionary measures and action routines for disaster situations must be developed. Simulation exercises, real world laboratories can help to establish emergency routines and raise awareness for potential event cascades. Through the playful simulation of a disaster, cross-sectoral cooperation and communication needs can be recognised, strategic points can be determined and courses of action can be identified that are helpful in dealing with the impacts and the uncertainties that arise (Schaper et al., 2019; Weisse et al., 2019). Such simulations can also include serious games, to explore possible outcomes and adaptation approaches (De Ruiter et al., 2021b).
The use and application of nature-based solutions (NBS) for integrating climate change adaptation and disaster risk reduction has recently received special attention in scientific and political discourses as well as in practical measures and project work. The recently published EU report on “Nature-based solutions in Europe” discusses the practical integration of adaptation and disaster risk reduction on the basis of NBS (European Environment Agency, 2021). The term “compound event,” in the sense used here, does not appear once. In addition to numerous practical examples of NBS implementation, only one example appears among the extensive European case studies, where coupling of water, fire and climate resilience with biomass production in forestry to adapt watersheds to climate change (European Environment Agency, 2021, p. 134) is considered. Here, too, it becomes clear that the concept of compound events and cascading impacts is not yet sufficiently embedded. More research, more awareness and acceptance are needed. Furthermore, the implementation of nature-based solutions to climate change adaptation and disaster risk reduction needs to consider the occurrence of coupled and or sequential events. Besides the development of technical standards, capacity building and cross-sectoral collaborative governance is urgently needed (see also the next section).
Transformative Adaptation and Transformative Governance
Definition and Research Approaches
Transformative adaptation includes adaptation actions that may change the fundamental attributes of a system in response to climate and its effects and find different solutions (European Environment Agency, 2021: glossary). Transformative governance is an approach to environmental governance that has the capacity to respond to, manage, and trigger regime shifts in coupled social-ecological systems (SESs) at multiple scales (Chaffin et al., 2016). Agency is key in social determinants of adaptive and transformative responses to climate change. Adaptive and transformative actions are related to social-ecological network structures between people and ecological resources as are aspects of social organisation (Barnes et al., 2017, p. 827). The discussion about a need for societal and governance transformation in the face of global environmental changes, and climate change has a long tradition (e.g., O'Brien, 2012), but has recently become even more important in academic literature as well as politics (Barnes et al., 2017; Blythe et al., 2018; Hay et al., 2018; Bennett, 2019; Bennett et al., 2019).
With increasing impacts from climate change, and more concrete projections of future risks, increasingly more attention has been paid to transformational approaches, both in outcome and process of adaptation actions, to address these challenges. In addition, given the current lack of adaptation actions, there is an increasing tendency to place more emphasis on the need for more drastic adaptation actions, beyond the incremental adaptation approaches from the past. Transformative adaptation is contrasted with incremental adaptation, as it changes the SESs more fundamentally, but also requires more investment (or human inputs); (see Figure 1).
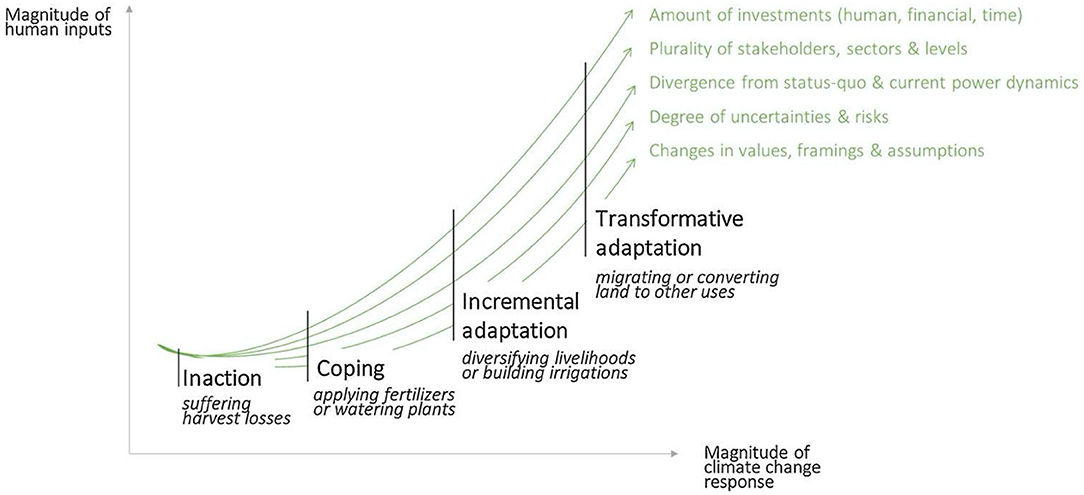
Figure 1. Types of strategies for reducing the impact of climate change on social-ecological systems, with examples from agriculture (taken from: Fedele et al., 2019) (Creative Commons CC-BY-NC-ND).
People and ecosystems are in the process of responding to extreme weather events. Fedele et al. (2019) mentioned several examples, where adaptation modifies the social system to accommodate changes modifying the core characteristics of the socio-ecological system, for instance by relocating houses from low lying coasts. “Transformative adaptation reduces the root causes of vulnerability to climate change, such as social, cultural, economic, environmental, and power relations by transforming them into more just, sustainable, or resilient states” (Fedele et al., 2019). At the same time, transformative governance is rarely considered in adaptation projects to reduce the impacts of climate change (Chaffin et al., 2016). It requires higher investments in human, financial and time resources (Adger et al., 2005; Kuntz and Gomes, 2012).
Transformative adaptation is emerging as an opportunity to address complex climate change effects (Chaffin et al., 2016). Fedele et al. (2019) found references to transformative adaptation for example, in the SDGs preamble (“transformative steps […] to a sustainable and resilient path”) (UN, 2018), in the Green Climate Fund mandate (“paradigm shift toward […] climate-resilient development pathways”) (UNFCCC, 2012), and in the Paris Agreement Article 7 (“greater adaptation needs can involve greater adaptation costs”) (UN, 2015).
More research is needed regarding the development of transformative governance in origins of theory of change in complex adaptive systems, distinguishing features between transformative and other forms of environmental governance, and potential guidance for encouraging transformative governance where appropriate (Chaffin et al., 2016).
An example of this transformation has been observed in the Great Barrier Reef management, which is influenced by internal dynamics and external disturbances that can generate a sudden change and a regime shift in its structure causing a system reorganisation and resulting in a new system configuration (Olsson et al., 2006, 2008). Another example on a Papua New Guinean island community provides evidence for the complex social-ecological interactions and how they relate to human behaviour (Barnes et al., 2017). Employing the influence of networks, facilitating individual and social learning, and carefully considering power dynamics add considerable value to existing approaches and encourage transformative actions. Agency is key and focussing on financial assets only is not sufficient for adapting to current and future changes. The authors identified six domains of societal adaptive capacity and suggest “… that harnessing the influence of networks, facilitating individual and social learning, and carefully considering power dynamics could add considerable value to existing approaches aimed at reducing climate vulnerabilities” (Barnes et al., 2017, p. 827).
Findings in SROCC and Recent Literature
Accepting diverse ways of knowing, understanding, and experiencing the multiple risks to ecosystems, populations, and infrastructure in low-lying coastal areas (McMichael et al., 2021), arguing for the importance of integrating scientific measurement and modelling with local knowledge and bringing together local/indigenous and scientific knowledge provides significant ways of knowing and sensing the world that can build the resilience of social-ecological systems.
Transformative governance has gained momentum in recent years. In contrast to adaptive governance, which has the goal of building resilience and enabling adaptive management (Brunner and Lynch, 2017), it describes the capacity to shape non-linear change in complex systems of people and nature and strives to actively shift a SES to an alternative and inherently more desirable regime by altering the structures and processes that define the system (Ratter and Leyshon, 2021). Transformation change involves a broad set of governance components (e.g., institutions, actors, networks, and organisations) and structures (e.g., legitimacy, power, and human behaviour), but requires additional capacity (e.g., leadership, innovation) including increased risk tolerance, significant systemic investment, and restructured economies and power relations (see Clarke et al., 2018).
The number of concrete case studies that implement transformation processes is increasing. A recent systematic global stocktake reveals evidence on human adaptation action to climate change in a broad range of examples (Berrang-Ford et al., 2021). The >48,000 articles systematically screened and 1,682 articles analysed documented that although the literature on adaptation to climate change is rapidly expanding, little is known about the actual extent of implementation. The academic literature (see also e.g., Mallette et al., 2021) shows a broad range of examples across most global regions and sectors but demonstrates that documented adaptations is largely fragmented, local and incremental, with only limited evidence of transformational adaptation and negligible evidence of risk reduction outcomes (Berrang-Ford et al., 2021, p. 993). Transformational change includes responses beyond usual incremental changes to activities and to address government, business and society at large (Shi and Moser, 2021). Understanding local community (Coates, 2015) and acknowledging social dynamics of community resilience (Fazey et al., 2021) is just as important as engaging publics about environmental and technology risks (Pidgeon, 2020) or the integration of scientific and local knowledge (Ainsworth G. B. et al., 2020; McMichael et al., 2021). Local governments are identified as drivers for societal transformation (Amundsen et al., 2018) for just transformations (Bennett et al., 2019). In addition, participation of stakeholders and civil society is essential (Forester, 1999), and has already received ample attention. For example, the PAR (participatory action-research) approach from Brazil demonstrated the high potential of early and active engagement of the community approaches in stakeholders and other management forums to rise from a low degree of social capital, mobilisation, and trust among themselves toward a better informed and engaged community, committed to the sustainability of Araçá Bay's ecosystem services and uses (Grilli et al., 2021). A sense of ownership, equalising power relationships, and enhancing social participation can boost and maintain the engagement needed for long-term participatory processes.
Gaps and Research Needs
Ocean governance still needs to address basic principles of ocean governance, the conservation and sustainable use of marine biological diversity, including benefit sharing of marine genetic resources, environmental impact assessment for new activities and the establishment of marine protected areas. As well as to study issues relating to the conservation and sustainable use of marine biological diversity beyond areas of national jurisdiction (GEF, 2016; Freestone, 2018). Van Assche et al. (2020) state the issue of rethinking the idea of coastal governance and the possibilities of a coastal governance adapted to challenges of climate change and intensified use of both land and sea.
Global ocean governance efforts face challenges and opportunities related to the nature of oceans and actors involved in leading to new and renewed global governance efforts directed toward ocean issues in areas of food production, biodiversity conservation, industrialisation, global environmental change, and pollution. Campbell et al. (2016) review these topics generally and in relation to nine new and emerging issues: small-scale fisheries, aquaculture, biodiversity conservation on the high seas, large marine protected areas, tuna fisheries, deep-sea mining, ocean acidification, blue carbon, and plastics pollution.
Haas et al. (2021) addressed the existing gaps and the question of how to move toward more sustainable ocean governance aligning with the sustainable development goals and the UN Ocean Decade, of three major risks to oceans that arise from governance-related issues: (1) the impacts of the overexploitation of marine resources; (2) inequitable distribution of access to and benefits from marine ecosystem services, and (3) inadequate or inappropriate adaptation to changing ocean conditions.
Meeting growing impacts of climate change requires significant and rapid societal change toward building community resilience. To further advance know-how about resilience building, much greater focus will be needed on the “how” of resilience. This, in turn, will require new framings of, and approaches for, community resilience and new framings of research, knowledge and knowing (Berrang-Ford et al., 2021; Bruno et al., 2021; Fazey et al., 2021; Mallette et al., 2021; Porto de Albuquerque et al., 2021).
For transformative governance, a high amount of theory has been developed, but only little on the ground ocean studies of actual practicability are available. More practical experience of implementing these concepts would be needed, in order to study the effectiveness, and be able to make recommendations on further improvements of transformative adaptation approaches and effective ways of governing the implementation.
Nalau and Verrall (2021) reviewed publications from 1978 to mid-2020, and identified the underpinning foundations of climate change adaptation literature, and explored how these have changed over time. They found that priority research topics and themes have been dynamic over time, while some core concepts (vulnerability, resilience, adaptive capacity) and sectors (water, agriculture) have remained relatively stable. The key challenge going forward is how to consolidate this vast research endeavour into a more coherent adaptation theory that in turn can better guide science of adaptation and support adaptation policy and practise (science for adaptation).
Conclusions
In conclusion, we have highlighted that although substantial improvements have been made in understanding and identifying key climate hazards, the assessment of risks (that is: socioeconomic impacts) and strategies to formulate risk management and adaptation responses, has fallen behind. This is also reflected by other authors such as Sutton (2019), who argues that more attention needs to be paid to risk assessment, including probability of climatic events but also the consequences if such events take place, and that improved studies require multiple disciplines to work together.
In addition, we have highlighted some key areas for research that need attention. The abrupt changes and extreme events projected by several studies point to the fact that compounding or consecutive events will require additional efforts by researchers to study risk and possible responses. The level of adaptive capacity for locally occurring “new” risks for some of the climatic hazards identified in the SROCC report that may lead to local “surprises,” such as MHWs, extreme ENSO events, or shifts in cyclone tracks, is largely unexplored.
In addition, cascading impacts through increasingly complex socioeconomic systems also require approaches to better understand and mitigate such risks. Finally, we have argued that transformative adaptation and transformative governance are emerging fields, but concrete applications and implementation of such approaches are largely lacking. Most of these fields will require the increasing collaboration between scientists from multiple fields, including climate and risk modelling, ecosystems, and social and economic sciences.
Author Contributions
All authors contributed to the conception and drafting of the manuscript.
Conflict of Interest
The authors declare that the research was conducted in the absence of any commercial or financial relationships that could be construed as a potential conflict of interest.
The handling Editor declared a past co-authorship with one of the author TF.
Publisher's Note
All claims expressed in this article are solely those of the authors and do not necessarily represent those of their affiliated organizations, or those of the publisher, the editors and the reviewers. Any product that may be evaluated in this article, or claim that may be made by its manufacturer, is not guaranteed or endorsed by the publisher.
References
Adger, W. N., Arnell, N. W., and Tompkins, E. L. (2005). Successful adaptation to climate change across scales. Glob. Environ. Change 15, 77–86. doi: 10.1016/j.gloenvcha.2004.12.005
AghaKouchak, A., Chiang, F., Huning, L. S., Love, C. A., Mallakpour, I., Mazdiyasni, O., et al. (2020). Climate extremes and compound hazards in a warming world. Annu. Rev. Earth Planet. Sci. 48, 519–548. doi: 10.1146/annurev-earth-071719-055228
Aguirre, J., De La Torre Ugarte, D., Bazo, J., Quequezana, P., and Collado, M. (2019). Evaluation of Early Action Mechanisms in Peru Regarding Preparedness for El Niño. Int J Disaster Risk Sci. 10, 493–510. doi: 10.1007/s13753-019-00245-x
Ainsworth, G. B., Redpath, S. M., Wilson, M., Wernham, C., and Young, J. C. (2020). Integrating scientific and local knowledge to address conservation conflicts: towards a practical framework based on lessons learned from a Scottish case study. Environ. Sci. Policy 107, 46–55. doi: 10.1016/j.envsci.2020.02.017
Ainsworth, T. D., Hurd, C. L., Gates, R. D., and Boyd, P. W. (2020). How do we overcome abrupt degradation of marine ecosystems and meet the challenge of heat waves and climate extremes? Glob. Change Biol. 26, 343–354. doi: 10.1111/gcb.14901
Amundsen, H., Hovelsrud, G. K., Aall, C., Karlsson, M., and Westskog, H. (2018). Local governments as drivers for societal transformation: towards the 1.5° C ambition. Curr. Opin. Environ. Sust. 31, 23–29. doi: 10.1016/j.cosust.2017.12.004
Anthoff, D., Estrada, F., and Tol, R. S. J. (2016). Shutting down the thermohaline circulation. Am. Econ. Rev. 106, 602–606. doi: 10.1257/aer.p20161102
Ariano-Sánchez, D., Muccio, C., Rosell, F., and Reinhard, S. (2020). Are trends in olive ridley sea turtle (Lepidochelys olivacea) nesting abundance affected by el niño southern oscillation (ENSO) variability? Sixteen years of monitoring on the Pacific coast of northern Central America. Glob. Ecol. Conserv. 24:e01339. doi: 10.1016/j.gecco.2020.e01339
Azuz-Adeath, I., Gonzalez-Campos, C., and Cuevas-Corona, A. (2019). Predicting the temporal structure of the atlantic multidecadal oscillation (AMO) for agriculture management in mexico's coastal zone. J. Coastal Res. 35, 210–226, doi: 10.2112/jcoastres-d-18-00030.1
Barnes, M. L., Bodin, Ö., Guerrero, A. M., McAllister, R. R., Alexander, S. M., and Robins, G. (2017). The social structural foundations of adaptation and transformation in social–ecological systems. Ecol. Soc. 22:16. doi: 10.5751/ES-09769-220416
Bennett, N. J. (2019). In political seas: engaging with political ecology in the ocean and coastal environment. Coastal Manag. 47, 67–87. doi: 10.1080/08920753.2019.1540905
Bennett, N. J., Blythe, J., Cisneros-Montemayor, A. M., Singh, G. G., and Sumaila, U. R. (2019). Just transformations to sustainability. Sustainability 11:3881. doi: 10.3390/su11143881
Berrang-Ford, L., Siders, A. R., Lesnikowski, A., Fischer, A. P., Callaghan, M. W., Haddaway, N. R., et al. (2021). A systematic global stocktake of evidence on human adaptation to climate change. Nat. Clim. Change 11, 989–1000. doi: 10.1038/s41558-021-01170-y
Binder, S. B., Barile, J. P., Baker, C. K., and Kulp, B. (2019). Home buyouts and household recovery: neighborhood differences three years after hurricane sandy. Environ. Hazards 18, 127–145. doi: 10.1080/17477891.2018.1511404
Bloemendaal, N., Muis, S., Haarsma, R. J., Verlaan, M., Apecechea, M. I., De Moel, H., et al. (2019). Global modeling of tropical cyclone storm surges using high-resolution forecasts. Clim. Dynam. 52, 5031–5044. doi: 10.1007/s00382-018-4430-x
Blythe, J., Silver, J., Evans, L., Armitage, D., Bennett, N. J., Moore, M. -L., et al. (2018). The dark side of transformation: latent risks in contemporary sustainability discourse. Antipode 50, 1206–1223. doi: 10.1111/anti.12405
Boers, N. (2021). Observation-based early-warning signals for a collapse of the Atlantic Meridional Overturning Circulation. Nat. Clim. Chang. 11, 680–688. doi: 10.1038/s41558-021-01097-4
Bouwer, L. M., and Jonkman, S. N. (2018). Global mortality from storm surges is decreasing. Environ. Res. Lett. 13:014008. doi: 10.1088/1748-9326/aa98a3
Britton, D., Schmid, M., Noisette, F., Havenhand, J. N., Paine, E. R., McGraw, C. M., et al. (2020). Adjustments in fatty acid composition is a mechanism that can explain resilience to marine heatwaves and future ocean conditions in the habitat-forming seaweed Phyllospora comosa (Labillardière) C.Agardh. Glob. Chang. Biol. 26, 3512–3524. doi: 10.1111/gcb.15052
Brown, C. J., Mellin, C., Edgar, G. J., Campbell, M. D., and Stuart-Smith, R. D. (2021). Direct and indirect effects of heatwaves on a coral reef fishery. Glob. Chang. Biol. 17, 1214–1225. doi: 10.1111/gcb.15472
Brunner, R. D., and Lynch, A. H. (2017). “Adaptive governance,” in Oxford Research Encyclopaedia, Climate Science, eds H. Von Storch, I. Auer, A. Bamzai, and H. Brooks. doi: 10.1093/acrefore/9780190228620.013.601
Bruno, M. F., Motta Zanin, G., Barbanente, A., and Damiani, L. (2021). Understanding the cognitive components of coastal risk assessment. J. Mar. Sci. Eng. 9:780. doi: 10.3390/jmse9070780
Caesar, L., McCarthy, G. D., Thornalley, D. J. R., Cahill, N., and Rahmstorf, S. (2021). Current Atlantic meridional overturning circulation weakest in last millennium. Nat. Geosci. 14, 118–120. doi: 10.1038/s41561-021-00699-z
Caesar, L., Rahmstorf, S., Robinson, A., Feulner, G., and Saba, V. (2018). Observed fingerprint of a weakening Atlantic Ocean overturning circulation. Nature 556, 191–196. doi: 10.1038/s41586-018-0006-5
Cai, W., Santoso, A., Wang, G., Wu, L., Collins, M., Lengaigne, M., et al. (2020). “Chapter 13. ENSO response to greenhouse forcing,” in AGU Geophysiocal Monograph: El Niño Southern Oscillation in a Changing Climate, eds M. J. McPhaden, A. Santoso, and W. Cai (Washington, DC: AGU & Wiley). doi: 10.1002/9781119548164.ch13
Campbell, L. M., Gray, N. J., Fairbanks, L., Silver, J. J., Gruby, R. L., Dubik, B. A., et al. (2016). Global oceans governance: new and emerging issues. Annu. Rev. Environ. Resour. 41, 517–543. doi: 10.1146/annurev-environ-102014-021121
Carey, J. (2020). Core Concept: Managed retreat increasingly seen as necessary in response to climate change's fury. Proc. Nat. Acad. Sci. U.S.A. 117, 13182–13185. doi: 10.1073/pnas.2008198117
Cavole, L. M., Demko, A. M., Diner, R. E., Giddings, A., Koester, I.C., et al. (2016). Biological impacts of the 2013–2015 warm-water anom-aly in the Northeast Pacific: winners, losers, the future. Oceanography 29, 273–285. doi: 10.5670/oceanog.2016.32
Chaffin, B. C., Garmestani, A. S., Gunderson, L. H., Benson, M. H., Angeler, D. G., Arnold, C. A., et al. (2016). Transformative Environmental Governance. Annu. Rev. Environ. Resour. 41, 399–423. doi: 10.1146/annurev-environ-110615-085817
Cheung, W. L., and Frölicher, T. (2020). Marine heatwaves exacerbate climate change impacts for fisheries in the northeast Pacific. Sci. Rep. 10:6678. doi: 10.1038/s41598-020-63650-z
Cheung, W. W. L., Frölicher, T. L., Lam, V. W. Y., Oyinlola, M. A., Reygondeau, G., Sumalia, U. R., et al. (2021). Marine high temperature extremes amplify the impacts of climate change on fish and fisheries. Sci. Adv. 7:eabh0895. doi: 10.1126/sciadv.abh0895
Clarke, D., Murphy, C., and Lorenzoni, I. (2018). Place attachment, disruption and transformative adaptation. J. Environ. Psychol. 55, 81–89. doi: 10.1016/j.jenvp.2017.12.006
Coates, T. (2015). Understanding local community construction through flooding: the ‘conscious community' and the possibilities for locally based communal action. Geo: Geogr. Environ. 2, 55–68. doi: 10.1002/geo2.6
Collins, M., Sutherland, M., Bouwer, L., Cheong, S.-M., Frölicher, T., Jacot Des Combes, H., et al. (2019). “Extremes, abrupt changes and managing risk,” in IPCC Special Report on the Ocean and Cryosphere in a Changing Climate, eds H. O. Pörtner, D. C. Roberts, V. Masson-Delmotte, P. Zhai, M. Tignor, E. Poloczanska, K. Mintenbeck, A. Alegría, M. Nicolai, A. Okem, J. Petzold, B. Rama, and N. M. Weyer (Geneva: World Meteorological Organization), 589–655.
Coughlan de Perez, E., van den Hurk, B., van Aalst, M. K., Jongman, B., Klose, T., and Suarez, P. (2015). Forecast-based financing: an approach for catalyzing humanitarian action based on extreme weather and climate forecasts. Nat. Hazards Earth Syst. Sci. 15, 895–904. doi: 10.5194/nhess-15-895-2015
Cutler, E. M., Albert, M. R., and White, K. D. (2020). Tradeoffs between beach nourishment and managed retreat: Insights from dynamic programming for climate adaptation decisions. Environ. Modell. Softw. 125:104603. doi: 10.1016/j.envsoft.2019.104603
David, C. G., Hennig, A., Ratter, B., Roeber, V., and Zahid Schlurmann, T. (2021). Considering socio-political framings when analyzing coastal climate change effects can prevent maldevelopment on small islands. Nat. Commun. 12:5882. doi: 10.1038/s41467-021-26082-5
De Ruiter, M. C., Couasnon, A., Van den Homberg, M. J. C., Daniell, J. E., Gill, J. C., and Ward, P. J. (2020). Why we can no longer ignore consecutive disasters. Earths Fut. 8:e2019EF001425. doi: 10.1029/2019EF001425
De Ruiter, M. C., Couasnon, A., and Ward, P. J. (2021b). Breaking the Silos: an online serious game for multi-risk disaster risk reduction (DRR) management. Geosci. Commun. 4, 383–397. doi: 10.5194/gc-4-383-2021
De Ruiter, M. C., de Bruijn, J. A., Englhardt, J., Daniell, J. E., de Moel, H., and Ward, P. J. (2021a). The asynergies of structural disaster risk reduction measures: Comparing floods and earthquakes. Earths Fut. 9:e2020EF001531. doi: 10.1029/2020EF001531
Defrance, D., Ramstein, G., Charbit, S., Vrac, M., Famein, A. M., Sultan, B., et al. (2017). Consequences of rapid ice sheet melting on the Sahelian population vulnerability. PNAS 114, 6533–6538. doi: 10.1073/pnas.1619358114
Donatti, C. I., Harvey, C. A., Hole, D., Panfill, S. N., and Schurman, H. (2020). Indicators to measure the climate change adaptation outcomes of ecosystem-based adaptation. Clim. Change 158, 413–433. doi: 10.1007/s10584-019-02565-9
Doshi, A., Pascoe, S., Thébaud, O., Thomas, C. R., Setiasih, N., Hong, J. T. C., et al. (2012). “Loss of economic value from coral bleaching in S.E. Asia,” in Proceedings of the 12th International Coral Reef Symposium (Cairns, QLD).
Emerton, R., Cloke, H. L., Stephens, E. M., Zsoter, E., Woolnough, S. J., and Pappenberger, F. (2017). Complex picture for likelihood of ENSO-driven flood hazard. Nat. Commun. 8:14796. doi: 10.1038/ncomms14796
Ensor, J., Tuhkanen, H., Boyland, M., Salamanca, A., Johnson, K., Thomalla, F., et al. (2021). Redistributing resilience? Deliberate transformation and political capabilities in post-Haiyan Tacloban. World Dev. 140:105360. doi: 10.1016/j.worlddev.2020.105360
European Environment Agency (2021). Nature-Based Solutions in Europe: Policy, Knowledge and Practice for Climate Change Adaptation and Disaster Risk Reduction. EEA Report No 1/2021. Copenhagen: European Environment Agency.
Fakhruddin, B. S., and Schick, L. (2019). Benefits of economic assessment of cyclone early warning systems-a case study on cyclone evan in Samoa. Progr. Disaster Sci. 2:100034. doi: 10.1016/j.pdisas.2019.100034
Fazey, I., Carmen, E., Ross, H., Rao-Williams, J., Hodgson, A., Searle, B. A., et al. (2021). Social dynamics of community resilience building in the face of climate change: the case of three Scottish communities. Sust. Sci. 16, 1731–1747. doi: 10.1007/s11625-021-00950-x
Fedele, G., Donatti, C. I., Harvey, C. A., Hannah, L., and Hole, D. G. (2019). Transformative adaptation to climate change for sustainable social-ecological systems. Env. Sci. Policy 101, 116–125. doi: 10.1016/j.envsci.2019.07.001
Fitzpatrick, D., and Compton, C. (2019). “Disaster risk reduction and the State: the failure of no-build zones after Typhoon Haiyan,” in The Cambridge Handbook of Disaster Risk Reduction and International Law (Cambridge: Cambridge University Press), 295–312. doi: 10.1017/9781108564540.017
Forester, J. (1999). The Deliberative Practitioner. Encouraging Participatory Planning Processes. Cambridge, MA: MIT Press.
Freestone, D. (2018). Filling the Gaps in Ocean Governance. Available online at: https://www.researchgate.net/publication/329354868_Filling_the_Gaps_in_Ocean_Governance (accessed January 03, 2022).
Frölicher, T. L, Fischer, E. M., and Gruber, N. (2018). Marine heatwaves under global warming. Nature 560, 360–364. doi: 10.1038/s41586-018-0383-9
GEF (2016). Governance Challenges, Gaps and Management Opportunities in Areas Beyond National Jurisdiction. Washington, DC: Global Environment Facility. GEF/STAP/C.51/Inf.02. Available online at: https://www.thegef.org/sites/default/files/council-meeting-documents/EN_GEF.STAP_.C.51.Inf_.02_Governance_Report.pdf
Grilli, N. M., Andrade, M. M., Xavier, L. Y., Santos, C. R., Stori, F. T., Carrilho, C. D., et al. (2021). Step by step: a participatory action-research framework to improve social participation in coastal systems. Ambiente Soc. 24:e02551. doi: 10.1590/1809-4422asoc20190255r1vu2021l1ao
Gruber, N., Boyd, P. W., Frölicher, T. L., and Vogt, M. (2021). Biogeochemical extremes and compound events in the ocean. Nature 600, 395–407. doi: 10.1038/s41586-021-03981-7
Haas, B., Mackay, M., Novaglio, C., Fullbrook, L., Murunga, M., Sbrocchi, C., et al. (2021). The future of ocean governance. Rev. Fish Biol. Fisher. 12, 1–18. doi: 10.1007/s11160-020-09631-x
Hall, T. M., and Sobel, A. H. (2013). On the impact angle of hurricane sandy's New Jersey landfall. Geophys. Res. Lett. 40, 2312–2315. doi: 10.1002/grl.50395
Handmer, J., Honda, Y., Kundzewicz, Z. W., Arnell, N., Benito, G., Hatfield, J., et al. (2012). “Changes in impacts of climate extremes: human systems and ecosystems,” in Managing the Risks of Extreme Events and Disasters to Advance Climate Change Adaptation. A Special Report of Working Groups I and II of the Intergovernmental Panel on Climate Change, Chapter 4, ed C. B. Field (Cambridge, New York, NY: Cambridge University Press), 231–290. doi: 10.1017/CBO9781139177245.007
Hay, J. E., Manley, M., Lal, P., Bennett, C., Chong, J., Campbell, J., et al. (2018). Climate Change and Disaster Risk Reduction: Research Synthesis Report. Submitted to the New Zealand Ministry of Foreign Affairs and Trade, Wellington, New Zealand. Available Online at: https://www.mfat.govt.nz/assets/Aid-Prog-docs/Research/Climate-Change-and-Disaster-Risk-Reduction-Research-Synthesis-Report.pdf
Hobday, A. J., Alexander, L. V., Perkins, S. E., Smale, D. A., Straub, S. C., Oliver, E. C. J., et al. (2016). A hierarchical approach to defining marine heatwaves. Prog. Oceanogr. 141, 227–238. doi: 10.1016/j.pocean.2015.12.014
Holbrook, N. J., Sen Gupta, A., Oliver, E. C. J., Hobday, A. J., Benthuysen, J. A., Scannell, H. A., et al. (2020). Keeping pace with marine heatwaves. Nat. Rev. 1, 482–493. doi: 10.1038/s43017-020-0068-4
Hughes, T. P., Kerry, J. T., Baird, A. H., Connolly, S. R., Dietzel, A., Eakin, C. M., et al. (2018). Global warming transforms coral reef assemblages. Nature 556, 492–496. doi: 10.1038/s41586-018-0041-2
IPCC (2012). Managing the Risks of Extreme Events and Disasters to Advance Climate Change Adaptation. A Special Report of Working Groups I and II of the Intergovernmental Panel on Climate Change. Cambridge, New York, NY: Cambridge University Press. Available online at: https://archive.ipcc.ch/report/srex/
IPCC (2019). Special Report on the Ocean and Cryosphere in a Changing Climate, eds H. O. Pörtner, D. C. Roberts, V. Masson-Delmotte, P. Zhai, M. Tignor, E. Poloczanska, K. Mintenbeck, A. Alegría, M. Nicolai, A. Okem, J. Petzold, B. Rama, N. M. Weyer. Available online at: https://www.ipcc.ch/srocc/
Johnson, L. E., Cifelli, R., and White, A. (2020). Benefits of an advanced quantitative precipitation information system. J. Flood Risk Manage. 13:e12573. doi: 10.1111/jfr3.12573
Khanam, M., Sofia, G., Koukoula, M., Lazin, R., Nikolopoulos, E. I., Shen, X., et al. (2021). Impact of compound flood event on coastal critical infrastructures considering current and future climate. Nat. Hazards Earth Syst. Sci. 21, 587–605. doi: 10.5194/nhess-21-587-2021
King, M. P., Yu, E., and Sillmann, J. (2020). Impact of strong and extreme El Niños on European hydroclimate. Tellus A Dynam. Meteorol. Oceanogr. 72, 1–10. doi: 10.1080/16000870.2019.1704342
Klein, S. G., Geraldi, N. R., Anton, A., Schmidt-Roach, S., Ziegler, M., and Cziesielski, M. J. (in press). Projecting coral responses to intensifying marine heatwaves under ocean acidification. Glob. Change Biol. 1–13. doi: 10.1111/gcb.15818.
Koks, E., Pant, R., Thacker, S., and Hall, J. W. (2019). Understanding business disruption and economic losses due to electricity failures and flooding. Int. J. Disaster Risk Sci. 10, 421–438. doi: 10.1007/s13753-019-00236-y
Kopits, E., Marten, A., and Wolverton, A. (2014). Incorporating 'catastrophic' climate change into policy analysis. Clim. Pol. 14, 637–664. doi: 10.1080/14693062.2014.864947
Kopp, R. E., Hayhoe, K., Easterling, D. R., Hall, T., Horton, R., Kunkel, K. E., et al. (2017). “Potential surprises – compound extremes and tipping elements,” in Climate Science Special Report: Fourth National Climate Assessment, Volume I, eds D. J. Wuebbles, D. W. Fahey, K. A. Hibbard, D. J. Dokken, B. C. Stewart, and T. K. Maycock (Washington, DC: U.S. Global Change Research Program), 411–429. doi: 10.7930/J0GB227J
Kossin, J. P., Knapp, K. R., Olander, T. L., and Velden, C. S. (2020). “Global increase in major tropical cyclone exceedance probability over the past four decades,” in Proceedings of the National Academy of Sciences, 117, 11975–11980. doi: 10.1073/pnas.1920849117
Kuntz, J. R. C., and Gomes, J. F. S. (2012). Transformational change in organisations: a self-regulation approach. J. Organ. Chang. Manag. 25, 143–162. doi: 10.1108/09534811211199637
Lancaster, J. T., Swanson, B. J., Lukashov, S. G., Oakley, N. S., Lee, J. B., Spangler, E. R., et al. (2021). Observations and analyses of the 9 January 2018 debris-flow disaster, Santa Barbara County, California. Environ. Eng. Geosci. 27, 3–27. doi: 10.2113/EEG-D-20-00015
Laufkötter, C., Zscheischler, J., and Frölicher, T. (2020). High-impact marine heatwaves attributable to human-induced global warming. Science 369, 1621–1625. doi: 10.1126/science.aba0690
Le Grix, N., Zscheischler, J., Laufkötter, C., Rousseaux, C. S., and Frölicher, T. L. (2021). Compound high-temperature and low-chlorophyll extremes in the ocean over the satellite period. Biogeosciences 18, 2119–2137. doi: 10.5194/bg-18-2119-2021
Lejano, R. P., Tan, J. M., and Wilson, A. M. W. (2016). A textual processing model of risk communication: lessons from typhoon haiyan. Weather Clim. Soc. 8, 447–463. doi: 10.1175/WCAS-D-16-0023.1
Leonard, M., Westra, S., Phatak, A., Lambert, M., Hurt, B., Mclines, K., et al. (2013). A compound event framework for understanding extreme impacts. Wi Res. Clim. Change. 5, 113–128. doi: 10.1002/wcc.252
Lin, Y. C., Jenkins, S. F., Chow, J. R., Biass, S., Woo, G., and Lallemant, D. (2020). Modeling downward counterfactual events: unrealized disasters and why they matter. Front. Earth Sci. 8:575048. doi: 10.3389/feart.2020.575048
Link, P. M., and Tol, R. S. (2009). Economic impacts on key Barents Sea fisheries arising from changes in the strength of the Atlantic thermohaline circulation. Global Environ. Change 19, 422–433. doi: 10.1016/j.gloenvcha.2009.07.007
Link, P. M., and Tol, R. S. (2011). Estimation of the economic impact of temperature changes induced by a shutdown of the thermohaline circulation: an application of FUND. Clim. Change, 104, 287–304. doi: 10.1007/s10584-009-9796-7
Liu, G., Heron, S. F., Eakin, C. M., Muller-Karger, F. E., Vega-Rodriguez, M., Guild, L. S., et al. (2014). Reef-scale thermal stress monitoring of coral ecosystems: New 5-km global products from NOAA coral reef watch. Remote Sensing 6, 11579–11606. doi: 10.3390/rs61111579
Mach, K. J., and Siders, A. R. (2021). Reframing strategic, managed retreat for transformative climate adaptation. Science 372, 1294–1299. doi: 10.1126/science.abh1894
Mallette, A., Smith, T. F., Elrick-Barr, C., Blythe, J., and Plummer, R. (2021). Understanding preferences for coastal climate change adaptation: a systematic literature review. Sustainability 13:8594. doi: 10.3390/su13158594
McMichael, C., Kothari, U., McNamara, K. E., and Arnall, A. (2021). Spatial and temporal ways of knowing sea level rise: bringing together multiple perspectives. WIREs Clim. Change 12:e703. doi: 10.1002/wcc.703
Melet, A., Almar, R., Hemer, M., Le Cozannet, G., Meyssignac, B., and Ruggiero, P. (2020). Contribution of wave setup to projected coastal sea level changes. J. Geophys. Res. Ocean 125:e2020JC016078. doi: 10.1029/2020JC016078
Morim, J., Hemer, M., Wang, X. L., Cartwright, N., Trenham, C., Semedo, A., et al. (2019). Robustness and uncertainties in global multivariate wind-wave climate projections. Nat. Clim. Chang. 9, 711–718. doi: 10.1038/s41558-019-0542-5
Nalau, J., Becken, S., and Mackey, B. (2018). Ecosystem-based adaptation: a review of the constraints. Environ. Sci. Policy 89, 357–364. doi: 10.1016/j.envsci.2018.08.014
Nalau, J., and Verrall, B. (2021). Mapping the evolution and current trends in climate change adaptation science. Clim. Risk Manage. 32:100290. doi: 10.1016/j.crm.2021.100290
O'Brien, K. (2012). Global environmental change II: from adaptation to deliberate transformation. Prog. Hum. Geogr. 36, 667–676. doi: 10.1177/0309132511425767
Oliver, E.C.J. (2019). Mean warming not variability drives marine heatwave trends. Clim. Dyn. 53, 1653–1659. doi: 10.1007/s00382-019-04707-2
Olsson, P., Folke, C., and Hughes, T. P. (2008). Navigating the transition to ecosystem-based management of the Great Barrier Reef, Australia. PNAS 105, 9489–94. doi: 10.1073/pnas.0706905105
Olsson, P., Gunderson, L. H., Carpenter, S. R., Ryan, P., Lebel, L., Folke, C., et al. (2006). Shooting the rapids: navigating transitions to adaptive governance of social-ecological systems. Ecol. Soc. 11:18. doi: 10.5751/ES-01595-110118
Piatt, J. F., Parrish, J. K., Renner, H. M., Schoen, S. K., Jones, T. T., Arimitsu, M. L., et al. (2020). Extreme mortality and reproductive failure of common murres resulting from the northeast Pacific marine heatwave of 2014-2016. PLoS ONE 15:e0226087. doi: 10.1371/journal.pone.0226087
Pidgeon, N. (2020). Engaging publics about environmental and technology risks: frames, values and deliberation. J. Risk Res. 19, 1–20. doi: 10.1080/13669877.2020.1749118
Porto de Albuquerque, J., Anderson, L., Calvillo, N., Coaffee, J., Cunha, M. A., Degrossi, L. C., et al. (2021). The role of data in transformations to sustainability: a critical research agenda. Curr. Opin. Environ. Sust. 49, 153–163. doi: 10.1016/j.cosust.2021.06.009
Ratter, B., and Leyshon, C. (2021). Perceptions of and Resilience to Coastal Climate Risks. Oxford Research Encyclopedia of Climate Science. Available online at: https://oxfordre.com/climatescience/view/10.1093/acrefore/9780190228620.001.0001/acrefore-9780190228620-e-819 (accessed May 14, 2020).
Reichstein, M., Riede, F., and Frank, D. (2021). More floods, fires and cyclones - plan for domino effects on sustainability goals. Nature 592, 347–349. doi: 10.1038/d41586-021-00927-x
Ritchie, P. D. L., Smith, G. S., Davis, K. J., Fezzi, C., Halleck-Vega, S., Harper, A. B., et al. (2020). Shifts in national land use and food production in Great Britain after a climate tipping point. Nature Food 1, 76–83. doi: 10.1038/s43016-019-0011-3
Rodrigues, R. R., Taschetto, A. S., Sen Gupta, A., and Foltz, G. R. (2019). Common cause for severe droughts in South America and marine heatwaves in the South Atlantic. Nat. Geosci. 12, 620–626. doi: 10.1038/s41561-019-0393-8
Santidrián Tomillo, P., Fonseca, L. G., Ward, M., Tankersley, N., Robinson, N. J., Orrego, C. M., et al. (2020). The impacts of extreme El Niño events on sea turtle nesting populations. Clim. Change 159, 163–176. doi: 10.1007/s10584-020-02658-w
Schaper, J., Ulm, M., Arns, A., Jensen, J., Ratter, B., and Weisse, R. (2019). Transdisziplinäres Risikomanagement im Umgang mit extremen Nordsee-Sturmfluten: Vom Modell zurWissenschafts-Praxis-Kooperation. Die Kuste, 87, 75–114. doi: 10.18171/1.087112
Scolobig, A., Komendantova, N., and Mignan, A. (2017). Mainstreaming multi-risk approaches into policy. Geosciences 7:129. doi: 10.3390/geosciences7040129
Seager, R., Hoerling, M., Schubert, S., Wang, H., Lyon, B., Kumar, A., et al. (2015). Causes of the 2011-14 California drought. J. Clim. 28, 6997–7024. doi: 10.1175/JCLI-D-14-00860.1
Sen Gupta, A., Thomsen, M., Benthuysen, J. A., Hobday, A. J., Oliver, E., Alexander, L. V., et al. (2020). Drivers and impacts of the most extreme marine heatwave events. Sci. Rep. 10:19359. doi: 10.1038/s41598-020-75445-3
Shi, H., García-Reyes, M., Jacox, M. G., Rykaczewski, R. R., Black, B. A., Bograd, S. J., et al. (2021). Co-occurrence of California drought and northeast Pacific marine heatwaves under climate change. Geophys. Res. Lett. 48:e2021GL092765. doi: 10.1029/2021GL092765
Shi, L., and Moser, S. (2021). Transformative climate adaptation in the United States: trends and prospects. Science 372:eabc8054. doi: 10.1126/science.abc8054
Smale, D. A., Wernberg, T., Oliver, E. C. J., Thomsen, M., Harvey, B. P., Straub, S. C., et al. (2019). Marine heatwaves threaten global biodiversity and the provision of ecosystem services. Nat. Clim. Chang. 9, 306–312. doi: 10.1038/s41558-019-0412-1
Sutton, R. T. (2019). Climate science needs to take risk assessment much more seriously. Bull. Am. Meteorol. Soc. 100, 1637–1642. doi: 10.1175/BAMS-D-18-0280.1
Tang, Y., Zhang, R. H., Liu, T., Duan, W., Yang, D., Zheng, F., et al. (2018). Progress in ENSO prediction and predictability study. Natl. Sci. Rev. 5, 826–839. doi: 10.1093/nsr/nwy105
Thomas, A., Theokritoff, E., Lesnikowski, A., Reckien, D., Jagannathan, K., Cremades, R., et al. (2021). Global evidence of constraints and limits to human adaptation. Reg. Environ. Change 21:85. doi: 10.1007/s10113-021-01808-9
UN (2018). Transforming our World: The 2030 Agenda for Sustainable Development. A New Era in Global Health. New York, NY: UN.
UNFCCC (2012). Report of the Conference of the Parties on Its Seventeenth Session, Held in Durban from 28 November to 11 December 2011. Addendum. Part Two: Action Taken by the Conference of the Parties at Its Seventeenth Session. Bonn: UNFCCC.
Van Assche, K., Hornidge, A. K., Schlüter, A., and Vaidiano, N. (2020). Governance and the coastal condition: new modes of observation, adaptation and integration. Mar. Policy J. 112:103413. doi: 10.1016/j.marpol.2019.01.002
Viglioni, G. (2021). Fevers are plaguing the oceans — and climate change is making them worse. Nature 593, 26–28. doi: 10.1038/d41586-021-01142-4
Wang, S., and Toumi, R. (2021). Recent migration of tropical cyclones toward coasts. Science 371, 514–517. doi: 10.1126/science.abb9038
Weijer, W., Cheng, W., Garuba, O. A., Hu, A., and Nadiga, B. T. (2020). CMIP6 models predict significant 21st century decline of the atlantic meridional overturning circulation. Geophys. Res. Lett. 47:E2019GL086075. doi: 10.1029/2019GL086075
Weinkle, J., Landsea, C., Collins, D., Musulin, R., Crompton, R. P., Klotzbach, P. J., et al. (2018). Normalized hurricane damage in the continental United States 1900–2017. Nat. Sust. 1:808. doi: 10.1038/s41893-018-0165-2
Weisse, R., Grabemann, I., Gaslikova, L., Meyer, E., Tinz, B., Fery, N., et al. (2019). Extreme Nordseesturmfluten und mögliche auswirkungen: das EXTREMENESS projekt. Die Kuste 87, 39–45. doi: 10.18171/1.087110
Wernberg, T., Bennett, S., Babcock, R. C., De Bettignies, T., Cure, K., Depczynski, M., et al. (2016). Climate-driven regime shift of a temperate marine ecosystem. Science 353:eaad8745. doi: 10.1126/science.aad8745
Yamaguchi, M., Chan, J. C. L., Moon, I. J., Yoshida, K., and Mizuta, R. (2020). Global warming changes tropical cyclone translation speed. Nat. Commun. 11, 47–52. doi: 10.1038/s41467-019-13902-y
Zhang, G., Murakami, H., Knutson, T.R., Mizuta, R., and Yoshida, K. (2020). Tropical cyclone motion in a changing climate. Sci. Adv. 6:eaaz7610. doi: 10.1126/sciadv.aaz7610
Zhang, S., Wang, H., Jiang, H., and Ma, W. (2021). Evaluation of ENSO prediction skill changes since 2000. Based on Multimodel Hindcasts. Atmosphere 12:365. doi: 10.3390/atmos12030365
Zhou, X., Chen, Q., Li, Y., Yang, Y., Zhang, S., Zhao, Y., et al. (2021). The North american spring coldness response to the persistent weak stratospheric vortex induced by extreme el niño events. Front. Earth Sci. 9:626244. doi: 10.3389/feart.2021.626244
Zscheischler, J., Martius, O., Westra, S., Bevacqua, E., Raymond, C., Horton, R. M., et al. (2020). A typology of compound weather and climate events. Nat. Rev. Earth Environ. 1, 333–347. doi: 10.1038/s43017-020-0060-z
Keywords: adaptation, risk management, extremes, abrupt, compound event, transformation, IPCC
Citation: Bouwer LM, Cheong S-M, Jacot Des Combes H, Frölicher TL, McInnes KL, Ratter BMW and Rivera-Arriaga E (2022) Risk Management and Adaptation for Extremes and Abrupt Changes in Climate and Oceans: Current Knowledge Gaps. Front. Clim. 3:785641. doi: 10.3389/fclim.2021.785641
Received: 29 September 2021; Accepted: 06 December 2021;
Published: 25 January 2022.
Edited by:
Matthew Collins, University of Exeter, United KingdomReviewed by:
Marleen De Ruiter, VU Amsterdam, NetherlandsGregg Garfin, University of Arizona, United States
Copyright © 2022 Bouwer, Cheong, Jacot Des Combes, Frölicher, McInnes, Ratter and Rivera-Arriaga. This is an open-access article distributed under the terms of the Creative Commons Attribution License (CC BY). The use, distribution or reproduction in other forums is permitted, provided the original author(s) and the copyright owner(s) are credited and that the original publication in this journal is cited, in accordance with accepted academic practice. No use, distribution or reproduction is permitted which does not comply with these terms.
*Correspondence: Laurens M. Bouwer, bGF1cmVucy5ib3V3ZXJAaGVyZW9uLmRl