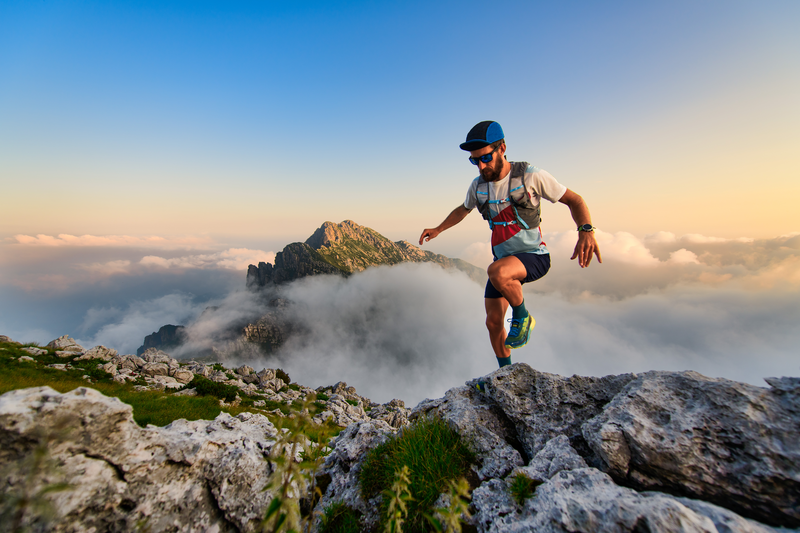
95% of researchers rate our articles as excellent or good
Learn more about the work of our research integrity team to safeguard the quality of each article we publish.
Find out more
HYPOTHESIS AND THEORY article
Front. Clim. , 17 January 2022
Sec. Climate and Decision Making
Volume 3 - 2021 | https://doi.org/10.3389/fclim.2021.781559
A changing climate makes the evaluation of human impacts on natural systems increasingly uncertain and affects the risk associated with management decisions. This influences both the achievability and meaning of marine conservation and resource management objectives. A risk-based framework that includes a risk equivalence approach in the evaluation of the potential consequences from human activity, can be a powerful tool for timely and consistent handling of environmental considerations in management advice. Risk equivalence permits a formal treatment of all sources of uncertainty, such that objectives-based management decisions can be maintained within acceptable risk levels and deliver outcomes consistent with expectations. There are two pathways to risk equivalence that can be used to account for the short-term and longer-term impacts of a changing environment: adjusting the degree of exposure to human pressure and adjusting the reference levels used to measure the risk. The first uses existing data and knowledge to derive risk conditioning factors applied to condition management advice on environmental departures from baseline conditions. The second is used to formalise the review and update of management objectives, reference levels and risk tolerances, so they remain consistent with potential consequences from human activity under new biological, ecological and socio-economic realities. A risk equivalence approach is about adapting existing practice to frame environmental considerations within objectives-based risk frameworks, systematically exploring alternative scenarios and assumptions, and conditioning management advice on environmental status. It is applicable to the management of all human activities impacting biological and ecological systems. Concepts of risk, risk conditioning factors, and incremental changes in risk, provide a common currency for the inclusion and communication of environmental effects into advice. Risk equivalence can ensure timely delivery of robust management advice accounting for demonstrated, anticipated or projected environmental effects. This can guide management decisions in a changing world, and greatly facilitate the implementation of an ecosystem approach for the management of human activities.
Climate-related changes increase uncertainty in the assessment of human impacts on marine resources and ecosystems, and in scientific advice on managing or mitigating such impacts. If not accounted for, this uncertainty alters the risk of biological, ecological and socio-economic consequences from management actions (or lack thereof). Accounting for such changes in risk is essential to inform robust management decision-making in a changing environment.
Risk occurs as a result of incomplete knowledge and information, erroneous or incorrect uses of the available data and information, and the inherent stochasticity and non-stationarity of natural systems and processes. In the marine realm, there is pervasive uncertainty in the evaluation of the state of ecosystems and ecosystem components, their responses to human-induced pressures, and how such responses are likely to evolve into the future and in a changing environment. There is also increasing evidence of shifting means and variances in oceanographic variables and of the increasing frequency of extreme events such as marine heat waves (Frölicher and Laufkötter, 2018; Oliver et al., 2018; IPCC, 2019, 2021). This makes risk assessment methods and risk management frameworks highly relevant to ocean governance (Burgman, 2005; Levin et al., 2009; Burgman and Yemshanov, 2013; Plagányi et al., 2013; Holsman et al., 2017; Hodgson et al., 2019; Ono et al., 2019; Haas et al., 2021). Risk assessments are conducted to evaluate and communicate the possible consequences of uncertainty on management outcomes (Francis and Shotton, 1997; Harwood, 2000). Risk management is the process of decision-making under uncertainty, which is informed by the risk assessment and conducted in policy environments providing guidance and direction on how to evaluate and manage the risks (Simonovic, 1997; Sethi, 2010; Hobday et al., 2011; Astles, 2015; Gibbs and Browman, 2015). Risk equivalence is about consistency in risk management i.e., maintaining a level of risk considered acceptable in management decisions. The definition of risk equivalence will vary depending on the resource and risk that are being managed, and the chosen risk tolerance. For example, in the Australian Commonwealth Fisheries Harvest Strategy Policy, risk equivalency is defined as “ensuring that a fish stock stays above the limit biomass level at least 90% of the time” (Fulton et al., 2016). In this case the fish stock is the resource, the limit biomass level is used to evaluate the risk of biological harm to the stock, and the 90% probability is the risk tolerance. Where management objectives are specified, risk equivalent management options can be formulated considering factors or circumstances that have been demonstrated or hypothesised to affect the risk associated with management decisions. The distinction between demonstrated and hypothesised or anticipated effects is important. Hence, a risk equivalence approach can formalise and facilitate the consideration and handling of quantifiable as well as yet unquantified or unquantifiable sources of uncertainty in the risk assessment.
While not often discussed or explicitly included in conventional risk management frameworks e.g., ISO (2018), risk equivalence is implicit, expected and applicable whenever scientific advice is formulated to inform risk-based management decisions. The precautionary approach (FAO, 2003) is a form of risk equivalence: when implemented systematically, its aim is to maintain a consistently low risk of negative impacts from human activities, notwithstanding the evidence basis available and used to assess the risk (i.e., differences in data and information types, their quantity and quality, and thus, levels of quantified vs. unquantified uncertainty). Similarly, where environmental changes contribute additional uncertainty in the assessment of the state and response of marine resources to human pressures, risk equivalent management options can be identified that allow human activities to continue within acceptable risk levels despite shifting or novel conditions.
Approaches for handling environmental variation and change in marine resources management have been generally focused on the development of analytical methods allowing to quantify and propagate uncertainty. This is often done without a clear understanding of the mechanistic relationships leading to that uncertainty, i.e., without specifying or exploring the mechanisms linking environmental drivers, human pressures and resources or ecosystems state. In this context, limits of applicability of the analytical methods remain unknown. While complex models with random effects can be useful in propagating error, they often result in inflated parameter variance and are mostly applicable only in data-rich circumstances. Analytical models accounting for environmental effects remain relatively rare and mostly available in only a few, high economic value, commercial fish species (King et al., 2015; Sagarese et al., 2015; Skern-Mauritzen et al., 2016; Marshall et al., 2019; Satterthwaite et al., 2020). The majority of marine resources and ecosystems affected by human activity remain data-limited or data-rich but information poor (DRIP) (Wilding et al., 2017), thus requiring alternative methods to take climate and environmental effects into account. A risk equivalence approach that builds on existing risk management practice can be used to handle the uncertainty contributed by a changing environment in marine resources and ecosystems management, regardless of the state of knowledge and data developments.
In this paper, we illustrate the relevance of risk equivalence for the management of human activities under climate change. We propose a flexible risk assessment and management framework that incorporates the explicit distinction between hypothesised and demonstrated effects (i.e., quantifiable and unquantifiable uncertainty) via the application of risk equivalence. This framework is amenable to science and technical developments while providing a decision-support process (application of risk equivalence) that can facilitate context-specific decision-making in a changing world (Jones et al., 2014). We show how risk equivalence can be used to incorporate ecosystem and environmental considerations in scientific advice for fisheries management over the data and process-knowledge continua. We demonstrate how the risk equivalence approach provides a unifying concept for handling the short-term aspects of environmental change on the probability of achieving (or not achieving) management objectives, and longer-term aspects relating to the specification of the objectives and the safe operating space within which the risk is managed. Finally, we discuss how this approach can be extended to marine resources and ecosystems management more broadly, whereby risk provides a common currency for handling and communicating all sources and types of uncertainty in a transparent and explicit manner.
For clarity and inclusiveness, throughout this paper, we use the word “environment” to encompass all external factors, drivers or indicators (e.g., ecosystem, climate, and physico-chemical variables) that are beyond the control of management systems but likely to affect the risk associated with management decisions.
In natural resources management, risk assessment methods differ in their scope, handling of uncertainty, and level of connexion to management outcomes (Hodgson et al., 2019). As a result, the term “risk” is given a variety of meanings that are often confounded and confounding. In this paper, we define risk in the context of objectives-based management (Box 1), where the link between risk and management outcomes is explicit, as is the quantification and propagation of uncertainty.
Box 1. Risk in objectives-based management.
In objectives-based risk frameworks, risk is the probability of a consequence occurring:
Risk = Pr(Consequence)
The consequences of human activities on natural systems can be biological, ecological, social and/or economic. The probability of occurrence and severity of a consequence is influenced by resource or ecosystem sensitivity and exposure to human-induced pressures:
Risk = Pr(Consequence) = Sensitivity x Exposure x Consequence
Sensitivity expresses resource or ecosystem resilience and adaptive capacity determined by structural and dynamic aspects (e.g., productivity traits, community composition, trophic structure). Exposure captures the various aspects of human activity that can be managed, as well as aspects of resource or ecosystem vulnerability to human activity (e.g., spatial extent or distribution, habitat use or heterogeneity).
Consequences are expressed as biological, ecological, and socio-economic thresholds (or reference levels) delineating the boundaries of the safe operating space used to manage the risk.
Risk is the probability of exceeding reference levels expressing potential consequences
The definition of reference levels requires an investigation of resource or ecosystem sensitivity and exposure within a specific context or baseline, often corresponding to long-term observations within a particular area. This makes reference levels and the evaluation of potential consequences from human activity conditional on one or more assumptions about the state of the environment and socio-ecological system, the spatial structure of the resource or ecosystem that is being managed, etc.
Climate change affects the sensitivity and exposure components of risk, and ultimately the consequence meaning of reference levels used to measure the risk
Objectives-based risk management consists of defining a “safe operating space” or “sustainability domain” bounded by reference levels and objectives (each with a specified risk tolerance), and choosing management options that are as robust as possible for maintaining the system within the safe operating space, taking uncertainties into account. The risk assessment has a crucial role in linking these steps. Objectives-based risk assessments involve (1) identifying the pressure(s) resulting from human activity and their potential biological, ecological, social, and economic consequence(s) (risk identification); (2) estimating the probability that pressures will lead to consequences of varying severity given sensitivity and exposure (risk analysis); and (3) evaluating the incremental changes in risk and possible trade-offs among management options in relation to acceptable risk levels (risk evaluation) (Figure 1). The risk identification step can be informed by contextual risk assessments, which are commonly used to prioritise species, ecosystem components and/or human activities for management actions, without explicit quantification of uncertainty and without consideration of management outcomes (Dulvy et al., 2004; Smith et al., 2007; Hobday et al., 2011; Gaichas et al., 2014, 2018; Cotter et al., 2015; Knights et al., 2015; Hare et al., 2016; Pedreschi et al., 2019; Muffley et al., 2021). Contextual risk assessments involve identifying relevant pressures arising from human activity, mapping how they relate to individual ecosystem components or ecological characteristics (pressure-effects pathways) and using available knowledge and information to identify and rank relative risks. Contextual risk assessments can inform the definition of potential consequences from human activities and associated biological, ecological, and socio-economic thresholds or reference levels. Such reference levels are used to measure the risk in objectives-based risk frameworks, where risk is evaluated as the probability of exceeding reference levels tied to potential consequences and management objectives representing long-term aspirational goals for the state of the resource, ecosystem and/or human activity sector and societal welfare. The specification of objectives is aspirational and guided by science, policy, regulations and legislation, addressing considerations such as maximising benefits from resource use to human societies and minimising harm to the resource, the ecosystem service, or the entire ecosystem (Barber and Taylor, 1990). The assessment of the risk associated with not meeting the objectives given the uncertainties encountered in managing human activities, is operational. All natural resource use and conservation management is ultimately objectives-based, addressing considerations such as maximising benefits from resource use to human societies and minimising harm to the resource, the ecosystem service, or the entire ecosystem (Barber and Taylor, 1990; Rice, 1999; McDaniels, 2000; Game et al., 2013).
Figure 1. A framework for objectives-based assessment and management of the risks posed by human activities to natural systems, with explicit consideration and application of risk equivalence. Management objectives are identified in relation to potential consequences from human-induced pressures. The risk assessment involves risk identification (pressures and potential consequences definition), risk analysis (estimation of the probability that pressures lead to consequences), risk evaluation (exploration of alternative management options in relation to acceptable risk levels, potential trade-offs, and alternative assumptions). There are two pathways to formulating risk equivalent management options (red boxes and arrows): conditioning the exposure and conditioning the reference levels and objectives. Note that a single human activity will usually generate multiple pressures, each with potential biological, ecological, and socio-economic consequences for various ecosystem components. Components of the risk assessment (risk identification, analysis and evaluation) were adapted from ISO (2018).
Objectives-based risk assessments are used to quantify and propagate uncertainty in performance evaluation of alternative management strategies. Key biological and ecological processes relevant to resource and ecosystem management are often poorly understood or can be described adequately by multiple rival models (Harwood and Stokes, 2003). Uncertainty in various aspects of sensitivity and exposure is quantified and propagated in the risk analysis (Figure 1). Yet no matter how intensively sampling is done and however sophisticated our understanding of the system and analytical methods, there are usually sources of uncertainty in the risk assessment that cannot be easily quantified. These include, for example, institutional and implementation uncertainty influencing decision making and associated risks subsequent to the risk assessment and formulation of scientific advice for management (Francis and Shotton, 1997; Simonovic, 1997). Similarly, the uncertainty contributed by a changing climate is rarely quantifiable within the timeframes available for providing robust management advice. Failure to recognise that a non-negligible fraction of uncertainty is not captured in standard risk assessment practice, can lead to unexpected and undesirable outcomes from management decisions.
A risk equivalence approach can facilitate the consideration and handling of quantifiable as well as unquantifiable uncertainty in risk evaluation and management (Figure 1). The application of risk equivalence formalises a process for exploring the influence of factors or circumstances that have been demonstrated, hypothesised or projected to affect the level of risk in management decisions. A framework for objectives-based risk assessment and management that includes the application of risk equivalence, can be used to inform consistent and accountable decision making under a changing environment (Figure 1). Such framework can be developed and tailored to all human activity sectors, whereas risk offers a common currency that can facilitate the communication of uncertainty and uptake of scientific results and conclusions at the science-policy interface, especially in situations involving complex and incompletely known cause-effects pathways (Kaplan and Garrick, 1981; Fu et al., 2018; Stelzenmüller et al., 2018; Hodgson et al., 2019).
Objectives-based risk management is well-exemplified in fisheries, where structured frameworks for considering risk in fisheries advice and decision-making have been adopted in several jurisdictions e.g., Canada (DFO, 2006), ICES/EU (ICES, 2021a), New Zealand (Ministry of Fisheries, 2008), and the US (USDC, 2007). Fisheries risk assessments are used for exploring and identifying management options enabling fishery objectives to be met at the acceptable risk level, given quantifiable scientific uncertainty (namely, observation, and process error variability). Fishing exerts multiple pressures on marine ecosystems, a primary one being the selective removal and possible depletion of targeted fish stocks. Depleting a fish stock to a level of impaired productivity is a potential biological consequence of fishing. This consequence is operationalised as a limit biomass reference point such as Blim. The limit reference point delineates the lower boundary of the safe operating space for the fishery, below which there is a risk of biological harm to the stock. This risk is estimated as the probability of stock size (usually spawning stock biomass, SSB) falling below Blim. It is adjusted by considering stock sensitivity to fishing pressure (e.g., how productivity attributes and dynamics including individual growth, natural mortality and recruitment vary with SSB) and after applying management options corresponding to varying degrees of exposure to fishing pressure (e.g., varying effort and harvest levels). If the management aim is to avoid potentially serious or irreversible biological consequences to the stock (e.g., inability to recover to previously observed levels), the risk should be kept low (e.g., <5%), corresponding to a low risk tolerance or high risk aversion.
Sets of reference levels expressing potential biological, ecological and socio-economic consequences of varying severity are used in fisheries risk frameworks. These include biological and exposure limits intended to delimit the true domain of sustainability [e.g., Blim and Flim (ICES, 2021a), Canada's Lower Reference Point LRP (DFO, 2006), and the Overfishing Level OFL in the US (USDC, 2007)], as well as precautionary, early-warning or trigger reference points taking into account uncertainty in limit estimates [e.g., Bpa and Fpa (ICES, 2021a)]. Upper reference levels also may be defined that correspond to potential economic consequences from missed fishing opportunities, and reflect long-term management objectives for the state of the stock and fishery [e.g., FMSY and BMSY (USDC, 2007; Ministry of Fisheries, 2008; ICES, 2021a) and Canada's Upper Stock Reference USR (DFO, 2006)]. Upper reference levels may also be defined as limits for biological and ecological consequences on other species or ecosystem components within a viability kernel/ecosystem approach (Cury et al., 2005). Together, measurable reference levels are used to delimit the safe operating space within which fishery managers can make decisions for maintaining both healthy and economically viable fisheries.
International guidance specifies an expectation that fisheries are managed sustainably and in accordance with the precautionary approach (FAO, 2020) to avoid serious or irreversible harm, both among stocks within a jurisdiction and over time for individual stocks. One way to achieve this is through the application of risk equivalence. Risk equivalence can ensure a consistently low risk of stocks being depleted below a limit reference point or not being maintained at a target reference point, regardless of differences in data availability and assessment methods (Fulton et al., 2016), but also changes in productivity dynamics and external factors. Fisheries reference levels are estimated using historical (sometimes truncated) time series of fish productivity dynamics that are assumed to reflect stock responses to long term average “baseline” environmental conditions (Smith et al., 1993; Schijns and Pauly, 2021). Deviations from baseline conditions, including directional or trending environmental change, contribute additional uncertainty that may or may not be readily captured in the conventional fisheries risk assessment. In this context, the application of risk equivalence permits a formal treatment of all sources and types of uncertainty, to ensure that management decisions remain within specified risk aversion, acceptability or tolerance.
Risk equivalence is a fundamental concept in risk evaluation and management that has been largely overlooked to date. Practical examples of the application of risk equivalence are found in fisheries management jurisdictions that have implemented data and assessment tiers and associated buffer systems to ensure comparable risks among data-rich and data-limited stocks (Smith et al., 2009; Punt et al., 2012; Dichmont et al., 2016; Fulton et al., 2016; ICES, 2017; Mildenberger et al., 2021). For stocks with comparable life histories, fisheries assessments based on fewer data are more uncertain than data-rich assessments. Low information stocks have high levels of unquantified uncertainty and may exhibit greater inaccuracies in estimates of variance, kurtosis or cumulative probability density functions. Thus, if results from low-information assessments are used to inform decision-making in the same way as results from high-information assessments, there is an increased risk that decisions would allow serious or irreversible consequences from fisheries in data-limited situations. If not accounted for, this difference in risk equates to different degrees of precaution (or risk tolerance) in the management of data-rich and data-limited stocks, with the management of data-limited stocks being less precautionary. To avoid this, precautionary buffers are applied to the advised property (usually but not necessarily the catch advice or harvest control rule) to reduce fishery exposure in data-limited situations. Precautionary buffers are adjustment factors that can be applied to any advised property used to manage the degree of exposure to fishing pressure, in order to recalibrate the risk and maintain a comparable level of precaution among data tiers or stock categories. They can be expressed numerically as the ratio of the adjusted advice to the status quo advice. Applied at a jurisdictional scale, buffers are intended to ensure that all fisheries are managed consistently, i.e., that all fish stocks have a comparably low probability of biological harm, despite differences in assessment methods and data availability. The delineation of buffers can be subjective or partly quantitative. Those currently in use have been simulation tested and found to be appropriate for formulating risk equivalent advice for fisheries (Fulton et al., 2016; ICES, 2017; Mildenberger et al., 2021). Most importantly, even buffers largely based on expert opinion have been demonstrated to be an improvement over the assumption that additional, unquantified uncertainty does not exist (Punt et al., 2012).
The buffer approach can be extended to handle external factors that are beyond the control of the decision makers but likely to affect the risk associated with management decisions and thus, management outcomes. Like precautionary buffers, ecosystem or environmental conditioning factors (ECFs) can be developed to measure the change in exposure to human pressures required to maintain a comparable risk in an increasingly variable or changing environment (Figure 2). Adjusting the degree of exposure to human pressures (conditioning of exposure) is one pathway to achieving risk equivalence in a changing world. A second pathway consists of adjusting the safe operating space used to manage the risk (conditioning of objectives) (Figure 1). Both pathways are complementary and can be implemented sequentially to handle the short-term (tactical) and longer-term (strategic) aspects of natural resources management in a changing environment (Box 2).
Figure 2. Illustration of risk equivalence in resource status evaluation in response to a human pressure (modified from Fay et al., 2012). The red dashed line is a limit reference level for resource status. Given the same degree of exposure to the pressure, the risk of resource status being below the limit increases with decreasing data availability and quality or an increasingly variable environment away from baseline conditions (from left to right). Precautionary buffers or environmental conditioning factors (ECFs), expressing the change in exposure required to maintain a comparable risk, can be developed and applied to deliver risk equivalent outcomes i.e., the grey dots corresponding to resource status estimates having the same probability of crossing the reference level, despite differences in the evidence base for assessment and environmental departures from baseline conditions. The adjusted exposure (not showed) is the risk equivalent management option. The numerical value of the buffer or ECF is the ratio of the adjusted to status quo exposure.
Box 2. Risk equivalence driving tactical decisions vs risk equivalence guiding when and how to alter risk management frameworks.
There are two pathways to formulating risk equivalent advice for objectives-based management of human activities:
Conditioning of exposure consists of adjusting the degree of exposure to a human pressure considering factors or circumstances that have been demonstrated or hypothesised to affect the degree of certainty of the advice. This includes differences in resource sensitivity and in the evidence basis for the assessment (e.g., data availability and assessment methods), and external factors such as socio-economics and environmental and ecosystem drivers. Conditioning of exposure involves risk adjustment factors expressing the change in exposure required to maintain a comparable risk under alternative assumptions. Risk equivalent options achieved by adjusting the exposure to human pressure can ensure that management decisions remain consistent with objectives and acceptable risk levels over the lifespan of the advice.
Conditioning of objectives consists of adjusting the reference levels and/or risk tolerances used to measure the risk, considering persistent, directional change in external factors (e.g., socio-economics or environmental and ecosystem drivers) affecting the definition of the potential consequences from human pressures on a resource or ecosystem. Conditioning of objectives on new environmental or socio-ecological realities, serves to maintain the safe operating space for managing risks consistent with the desired outcomes from management decisions.
Together, conditioning of exposure to human pressure and conditioning of objectives on alternative assumptions about the state of the environment, provide complementary and incremental mechanisms to account for the short-term and longer-term impacts of a changing climate in scientific advice for the management of human activities.
Conditioning of exposure involves investigating and applying ecosystem or environmental conditioning factors (ECFs) to formulate risk equivalent management options under alternative assumptions about the state of the environment. Like precautionary buffers, the ECFs measure the change in exposure to a human pressure that is required to maintain a comparable risk, and are mathematically equivalent to the ratio of the advice adjusted for environmental change to the status quo advice assuming a constant or randomly varying environment. ECFs can be investigated in risk analyses considering resource or ecosystem sensitivity to environmental change and the magnitude of departures from baseline environmental conditions. Most importantly, ECFs can be operationalised across the data and process-knowledge continuum (Figure 3). They provide a common metric for handling the uncertainty arising from observed/demonstrated and/or potential/anticipated environmental effects on the advice. In data and process-knowledge rich situations, where environmental signals can be reliably incorporated within the analytical model used for providing advice, ECFs can be estimated by forecasting the dynamic responses of the resource or ecosystem to varying degrees of exposure to human pressure under observed and/or projected environmental change scenarios, using tools such as management strategy evaluation (MSE) (Plagányi et al., 2013) and/or models of intermediate complexity (MICE) (Plagányi et al., 2014). In circumstances where data are available but the mechanistic understanding for environmental effects remains limited, ECFs can be investigated and estimated in an empirical process that is parallel and external to the assessment (Duplisea et al., 2021). In situations where both data and process knowledge are limiting, ECFs can be approximated as relative risk scores using qualitative or semi-quantitative information and scoring methods, and applied a posteriori to condition the advice on external conditions not considered within the assessment e.g., Dorn and Zador (2020). This later approach recognises that where there is evidence of departures from known or baseline environmental conditions, even qualitative ECFs based on expert knowledge can be an improvement over the assumption that the environment is stationary. The idea is to use existing data and knowledge to explore alternative assumptions about environmental status, and formulate risk equivalent options that explicitly consider these alternative assumptions in relation to the status quo (e.g., stationary, baseline average, or randomly varying environment within the range of existing observations). ECFs can have positive or negative values, indicating potential for increases in resource use when conditions are favourable, or decreases when conditions are unfavourable.
Figure 3. Degree of quantified uncertainty in Environmental Conditioning Factors (ECFs) as a function of the evidence base for environmental effects. Where data and mechanistic knowledge are limited, ECFs can be approximated as relative risk scores using qualitative and experiential information (e.g., expert knowledge) and empirical evidence such as results from laboratory studies or information inferred from other relevant studies. Where there is sufficient empirical or mechanistic evidence, ECFs can be derived empirically or analytically as the ratio of management options that can meet objectives in model scenarios with environmental change, to the management option that can meet the same objectives in a model scenario assuming a constant or randomly varying environment within known bounds. In the absence of mechanistic understanding, empirical models can be used to explore risk equivalent management options and ECFs values. Full quantification of uncertainty is only achievable where there is mechanistic evidence for both human pressure and environmental effects implemented in an analytical model.
Persistent directional environmental change will alter the meaning of the reference levels used to measure the risk. For example, a biological limit used to evaluate the risk of serious biological harm to a species under the present environmental norm, may no longer represent serious harm under persistent warming leading to increasingly favourable environmental conditions for the species. Similarly, a target used to evaluate the risk of lost economic opportunities under the present conditions, may no longer be achievable if conditions deteriorate to a point where previous levels of human activities are no longer possible. Conditioning of objectives on a new environmental norm is then required to maintain risk equivalence. This concerns the delineation of the “safe operating space” used for providing risk-based advice. Conditioning of objectives can range from updating the reference levels (e.g., biological and ecological limits for sustainability) to ensure the advice remains precautionary, to reviewing and adjusting an appropriate combination of management objectives, reference levels, and risk tolerances, to reflect new biological, ecological and/or socio-economic realities.
Conditioning of objectives will ensure that management decisions continue to deliver outcomes consistent with expectations i.e., allow avoiding negative/unwanted consequences and achieving long-term aspirational goals for the resource, ecosystem and human activity sector, attuned to the current state of the environment and socio-ecological system. In complex, multi-objectives management frameworks, new environmental conditions may require different prioritisations and trade-offs among objectives. Seeking risk equivalence under new environmental realities by adjusting the safe operating space used to inform risk-based decisions in the past, allows discussions not just of whether resources now need to be managed at a higher or lower status given expanded or reduced opportunities for human activities, but whether the risks to the resource, ecosystem and communities of users have changed. Exploring risk equivalence in this way may allow a much more constructive public policy dialogue.
A risk equivalence approach is applicable to all scientific advice aimed at assessing potential impacts from human activities and evaluating the risks associated with different management strategies and options. Conditioning of advice on environmental status can relax the short-term need to assume a greater mechanistic understanding of environmental effects than actually is known, instead focusing on the uncertainty and incremental risks contributed by a changing environment, their magnitude and direction, temporal patterns, and directionality. This can facilitate timely delivery of robust management advice in the absence of complete knowledge and understanding. A risk equivalent approach meets the call for more flexible and adaptable management and policy frameworks in a changing world (Schindler and Hilborn, 2015).
Pathways to risk equivalence can be implemented sequentially. Conditioning of exposure on environmental status requires routine monitoring and consideration of environmental conditions likely affecting the response of resources or ecosystems to human pressures. This can improve the detection of repeated environmental deviations from background conditions and incremental changes in risk. Repeated adjustments in the degree of exposure to human pressure in response to demonstrated or plausible environmental effects, can serve as a trigger for conditioning the objectives. For example, a management system can define a maximal recurrence or amplitude of environmental deviations into improbable risk space, or maximal incremental change in risk, beyond which a review and update of the reference levels and objectives is necessary for management decisions to remain risk equivalent. Alternatively, the point at which no level of exposure to human pressure can achieve one or more reference levels and objectives within the acceptable risk level, can be the point at which conditioning the objectives on a new reality is required to continue formulating robust advice for feasible actions. In recurring advice, the exploration and provision of risk equivalent management options can be iterative and adaptive, and allow to consistently operate within acceptable risk levels whilst improving the evidence basis for environmental effects for the management of human activities (Figure 4).
Figure 4. A risk equivalence approach accounting for the influence of environmental variation and change in scientific advice for the management of human activities. Conditioning the degree of exposure to human pressures on environmental status requires to monitor and prioritise environmental indicators most relevant to the resource or ecosystem and human activity under assessment. Depending on the evidence basis for environmental effects, ECFs expressing the change in exposure required to maintain a comparable risk, will be used to formulate risk equivalent management options accounting for yet unquantified, partly quantified or fully quantified and propagated uncertainty linked to a changing environment. In recurring advice, routine conditioning of exposure can assist with incrementally building the knowledge basis and mechanistic understanding required to fully quantify environmental effects on management advice. It can also assist with detecting directional change leading to a new environmental reality requiring to review and update the management objectives, reference levels, and risk tolerances (conditioning of objectives) for advice to remain consistent with the anticipated consequences from management decisions and long-term aspirational goals for the state of the resource, ecosystem and human activity sector.
Fisheries have long sought to achieve risk equivalence between current and future stock status by regulating the exposure to fishing pressure with the intent of maintaining risks within acceptable levels. This is operationalised in advice that is updated annually or on short multiyear cycles, by adjusting the advised catch (or some other component of fishery exposure that is subject to management actions), such that biological or fishery reference points are met within a specified risk tolerance. This strategy de facto treats the uncertainties quantified in the analytical (or other) assessment as fully capturing the risks. However, most conventional stock assessment methods do not account for environmental departures from reference or baseline conditions. Such departures will affect the dynamic responses of fish stocks to fishing pressure, through changes in stock productivity affecting stock sensitivity to fishing, and/or changes in fishing practice, spatial distribution, or the timing of seasonal or ontogenetic migrations, affecting stock exposure to fishing. These changes are increasingly common (McFarlane et al., 2000; Pecl et al., 2017; Cheung, 2018; Schickele et al., 2021). However, most stock assessments still lump any changes in stock status that have resulted from productivity responses to environmental conditions with overall “uncertainty,” without taking into account the change in risks of fishery management decisions that may result from operating outside the domain of known environmental conditions.
A changing climate requires the development of robust mechanistic understanding of the complex relationships among fish stocks, fishing pressure and environmental factors, and the use of heavy-tailed probability distributions (Anderson et al., 2017). Stock assessment models using random processes or time-varying parameters can be used to provide fishery options that consider changing stock productivity (Thorson and Minto, 2015; Miller et al., 2016; Holt and Michielsens, 2020; Stock and Miller, 2021). These models are relatively simple to develop and implement because they avoid the need to specify a mechanistic relationship for the effects of one or more environmental variables on stock productivity. However, projecting such models to investigate future plausible environmental status will typically result in a variance window so large as to make all future outcomes plausible (Duplisea et al., 2021). Hence, there is a sweet spot in defining model complexity in the face of uncertainty (Collie et al., 2016). Regular updating of fishery objectives and associated reference levels (e.g., FMSY and Blim) can be used to keep fisheries management decisions attuned to current environmental status and short-term variation in stock productivity, for example as examined through the benchmarking process in ICES (ICES, 2013). However, such a strategy requires both an analytical model that reliably links stock productivity to environmental conditions, and an efficient consultation and policy decision process for altering objectives and reference points. Currently, neither are readily feasible nor widely applicable because the majority of fishery resources remain data-limited and do not have a robust analytical assessment (FAO, 2020). Few assessment models treat environmental influences on stocks and fisheries dynamically, and consultation processes can be lengthy and cumbersome when dealing with areas of high knowledge uncertainty.
The formulation of risk equivalent management options for fisheries offers a practical, broadly applicable, and readily operational approach. Manageable aspects of fishery exposure can be readily conditioned on environment status in a process that is transparent, adaptive and iterative, allowing for timely delivery of reliable advice consistent with fishery objectives and risk tolerances. Ecosystem or environmental conditioning factors (ECFs) can be derived for all fish stocks using existing knowledge and information, and used to explore productivity scenarios and harvest strategies consistent with acceptable risk levels, as proposed by King and McFarlane (2006) and King et al. (2015). Existing tools such as random process models can be used to develop ECFs, for example as the ratio of the advised property (e.g., recommended harvest level) in a scenario fitted with time-varying productivity, to the same property in a scenario fitted assuming a constant productivity. This would enable investigation and communication of the change in risk that can be expected under alterative assumptions for environment effects, although not solving the problem of inflated variance in mid- to long-term projections. A multi-model approach focusing on ensemble means and variance within and between models may be used to explore risk equivalent management strategies under climate forcing (Hollowed et al., 2020). Another alternative is to estimate ECF values and risk equivalent options in an empirical process that is parallel and external to the stock assessment. Duplisea et al. (2021) used an empirical model of stock productivity dependence on an environmental variable to investigate the incremental change in risk that can be expected under environmental change scenarios, and the adjustments to fishery exposure (ECFs) that would be required to maintain a comparable risk of not achieving a fishery objective. This approach has the advantage over the use of random processes to enable exploration of a range of falsifiable hypotheses for environmental effects onto fish stocks. Similar empirical models could be developed to explore the ECFs required to maintain a comparable risk when environmental change alters the spatial distribution of a fish stock and thus, its availability to the fishing gear. In data-limited fisheries, existing data and information on stock sensitivity and exposure to changes in environmental variables can be used to approximate an ECF as a relative risk score, which is then applied to the status quo advice or management strategy (e.g., previous years catch or harvest control rule) to provide a risk equivalent option (see example in Supplementary Material).
An important distinction between an ECF and precautionary buffer is that the ECF can both increase and decrease fishery exposure, thus increasing fishing opportunities when environmental conditions are good, and reducing them when conditions are poor. In both cases, the ECFs will serve to maintain a consistently high probability of achieving economic targets, and a consistently low risk of depleting fish stocks. ECFs may similarly accommodate other types of targets that may be adopted in the future, such as employment or livelihoods supported targets. Systematic conditioning of fisheries advice to account for the uncertainty arising from plausible or demonstrated environmental effects can ensure the advice remains robust, defendable and reproducible in a changing environment.
Practical examples of the application of risk equivalence to account for environmental considerations in fisheries advice have emerged in recent years. Plagányi et al. (2013) combined a qualitative risk assessment using expert knowledge with a spatial management strategy evaluation (MSE) tool to evaluate the performance of alternative harvest strategies in meeting objectives under posited climate change relative to equivalent no-fishing, no-climate change scenarios. The MSE simulations served to quantify and communicate anticipated climate change impacts on fisheries production as incremental changes in risk. Other examples include the risk table framework implemented in the USA to adjust fisheries harvest recommendations for concerns external to the stock assessment (Dorn and Zador, 2020), and the Feco approach proposed for including ecosystem considerations in fisheries management advice in the Irish Sea (Bentley et al., 2021; Howell et al., 2021). The risk table framework (Dorn and Zador, 2020) is an extension of the existing buffer system. It uses a scoring approach to evaluate whether environmental factors not explicitly considered in the stock assessment would justify a further reduction of the acceptable biological catch (ABC) to account for additional uncertainty and risk. This approach is consistent with approximating ECFs in data and process knowledge limited space (Figure 3), and making use of the best available scientific understanding and information to formulate risk equivalent fishery options. The risk table framework has only been applied unidirectionally to ensure that the advice remains precautionary when environmental conditions are unfavourable. The Feco approach, by contrast, relies on the status of a relevant ecosystem indicator in relation to its long-term range to condition the advice in both directions, accounting for favourable as well as unfavourable conditions (Bentley et al., 2021). The Feco is set in the lower Fmsy range when conditions are poor and in the upper range when conditions are good. The Fmsy range is estimated by the stock assessment model, while a whole-ecosystem model (EwE) is used to identify an ecosystem indicator relevant to the stock trajectory in a process that is parallel to the stock assessment (Howell et al., 2021). This approach is consistent with exploring model-based ECFs using external forecasting (in this case in empirical, multivariate space) (Figure 3), in order to provide a presumptively risk equivalent option (Feco) that takes into account ecosystem information. Bentley et al. (2021) used retrospective simulations to demonstrate that adjusting fisheries advice to Feco could have supported more productive cod and whiting stocks in the Irish Sea, in comparison with status quo advice (median Fmsy). These results suggest that implementing risk equivalent options for fisheries that take into account the plausible effects of environmental variation, may hold both short and longer-term benefits for fisheries management.
Climate change will eventually see fishery management systems striving to achieve static objectives which are no longer attainable. This includes exposure (fishing mortality-based) reference points that may allow excessive removals or be overly restrictive as environmental changes make stocks less or more productive; and stock status (biomass-based) reference points that could be either not risk averse enough (e.g. in cases where a larger biomass is needed to produce adequate eggs and larvae) or too restrictive (e.g. if the suitable habitat has been reduced so overall carrying capacity is now lower and can be saturated by a smaller spawner population). Correspondingly, substantial effort is currently being directed at developing methods that can better account for biological and environmental realism in fisheries reference points estimation. This includes recalculating reference points values with more recent data, estimation of time-varying or “dynamic” references points, and other analytical methods for propagating model, estimation, and process error uncertainties in reference points estimation (O'Leary et al., 2020). Evidence of a stable and lasting change in the environment is difficult to assemble and establish. Using transient reference points in a system in transition may not result in consistent or effective risk management (Travers-Trolet et al., 2020), but may be better suited to simulation testing management strategies (Berger, 2019). Amending objectives too often or without clear rationales for actual biological, ecological or socio-economic changes, undermines both the effectiveness and credibility of entire objectives-based fisheries management plans. When management objectives do change, they must reflect decisions to maintain all dimensions of fishing practice (biological, ecological, and socio-economic) in line with the potential consequences from fishing activity and long-term sustainability goals, and not merely a wish to either avoid substantial harvest reductions (which will result in chasing stocks decline) or maintain a specific stock biomass in a changing ecosystem. Routine conditioning of fishery exposure and provision of risk equivalent advice that account for environmental variation and change, can assist with determining when adjusting fishery reference points is necessary, either because the objectives are no longer achievable or because the search for risk equivalence has indicated recurring departures into improbable (and possibly unacceptable) risk spaces.
The management of all human activities affecting marine resources and ecosystems involves dealing with uncertainty in resource and impact evaluation (whether quantifiable or not) and increasingly includes objectives, which are either proposed or rooted in policy (Table 1). Although these objectives may not be expressed explicitly in some governance settings, advisory and decision processes should apply consistent, objectives-based risk management in accordance with jurisdictional policy. This requires specification of not only the objectives but also the biological, ecological and socio-economic reference levels associated with the objectives and their acceptable risk level. As objectives-based management frameworks mature, they make a risk equivalence approach appropriate and applicable for managing human activities in a changing ocean. We illustrate this in two hypothetical examples below. In all cases, a risk equivalent approach will involve:
1) Specifying objectives, reference levels, and acceptable risk tolerances i.e., delineating a safe operating space for assessing the performance of alternative management options in meeting objectives and formulating robust and consistent management decisions under uncertainty.
2) Mapping all relevant sources and types of uncertainty (quantifiable and unquantifiable) in the assessment of human pressure effects on entire ecosystems or ecosystem components
3) Conditioning of exposure: formulating risk equivalent management options that enable objectives to be met at the acceptable risk level, either by (a) estimating and propagating quantifiable uncertainty linked to environmental variables in the assessment; (b) quantifying the same uncertainty in an empirical process parallel to the assessment; or (c) approximating the relative change in risk that can arise from changes in environmental variables considering resource sensitivity and exposure to such variables (i.e., suspected but as yet unquantified uncertainty).
4) Conditioning of objectives: Reviewing and potentially updating the reference levels, objectives and risk tolerances used to measure the risk, to enable continued formulation of robust management decisions consistent with the anticipated consequences from human pressures and desired management outcomes, in the context of persistent environmental change.
Table 1. Examples of human activities, selected manageable pressures, potential biological or ecological consequences from such pressures, and examples of management objectives, as would be applicable in objectives-based risk management frameworks.
Further developments of a risk equivalent approach should focus on retrospective studies to investigate how routine conditioning of management advice on a changing climate (i.e., implementation of risk equivalent management options in decision-making) will affect the state of marine resources and ecosystems over time, and opportunities for human activity sectors. Limitations are expected to be context-specific and mainly associated with institutional and implementation constraints in adapting existing practice.
Human impacts on fish habitat are typically assessed on a project by project basis and tools such as habitat compensation may be offered as means to offset the risk of exceeding levels of allowable harm. Although not defined, provisions for habitat compensation imply that the risk tolerance associated with allowable harm is not zero. For example, we may consider a projected bridge construction that will permanently alter the flow on a salmon river. There is a limit reference level for the occurrence of low river flow below which harmful impacts to salmon can be expected, and a management objective to prevent damage to salmon habitat. The limit reference was estimated in relation to long-term average environmental variation. The risk associated with constructing the bridge can be evaluated in relation to this reference level, considering intrinsic properties of the river system (sensitivity) and bridge design aspects and proposed compensation measures (exposure factors). However, climate change leading to increasingly variable amounts of local precipitations may alter this risk over time. Unless this is accounted for in the advice, the decision leading to infrastructure development may not be accountable over the next decade(s), i.e., it might allow irreparable harm to the salmon population. Conditioning of exposure to formulate risk equivalent options that account for potential climate change impacts would consist of identifying additional compensation measures and design options to alleviate a future increase in risk. Conditioning of objectives would consist of revisiting and perhaps modifying the reference level to ensure it remains relevant to the river system, salmon population and anticipated impacts from infrastructure development under a new environmental reality.
MPAs are management tools aimed at achieving a number of potentially competing conservation objectives, educational and awareness-raising objectives, and local socio-economic objectives. Some of these objectives are achieved through granting access to various human activities (e.g., ecotourism, resource extraction and shipping) and managing the degree of exposure and potential impacts from such activities. Consistent risk management in this context requires consideration of the hierarchy of objectives (whether implicit or explicit) and their respective risk tolerances. For example, consider an MPA with a conservation objective of protecting a seabird colony, using a target of maintaining a minimum number of breeding pairs with a high (e.g., 90%) probability. A key ecosystem consideration for the colony is the dynamics of a forage fish population which serves as a main food supply (keystone prey) to the seabirds. If the productivity of the forage fish population declines in response to warming surface waters, its exposure to a human pressure (e.g., fishing activity) could be curtailed by conditioning of fishery exposure through spatial or seasonal closures or effort restrictions. This would provide risk equivalent options aimed at preventing a reduction in the forage fish population and prey availability to seabirds, and thus maintaining a consistently low risk of failing to achieve the breeding pair target at the specified risk tolerance (<10% risk) when ecosystem conditions are potentially unfavourable to the seabird colony. However, under sustained warming, there will be a point at which no further reduction in exposure to fishing or other pressures resulting from human activity can prevent a decline in the forage fish population and allow the seabird breeding pair objective to be achieved locally, either because the total number of breeding pairs has decreased to a lower level (population-level consequence), or because some breeding pairs have permanently shifted their distribution beyond the MPA boundaries, to areas where prey availability and quality remain favourable (spatial consequence). At this point, conditioning the objective could involve revising the target number of local breeding pairs or, if protecting the seabird colony is the primary conservation objective for the MPA, reassessing and potentially adjusting or displacing the MPA boundaries. The latter points to the need for a clear hierarchy of objectives, as this will ultimately determine pathways for achieving risk equivalence in MPA management.
There is a pressing need to account for the increasing uncertainty contributed by a changing environment in marine resources and ecosystems management, regardless of the state of knowledge and data developments. Objectives-based risk management with application of risk equivalence, provides a practical framework for decision-making in a changing world. Risk equivalence is a powerful approach for operationalising available data and information in timely and systematic conditioning of management advice on demonstrated, potential or projected environmental change. The implementation of risk equivalence consists of adapting existing practice in order to frame environmental considerations within objectives-based risk frameworks; systematically explore and formulate risk equivalent management options accounting for environmental variation and change; and formalise strategic reviews and updating of entire risk management frameworks under new environmental realities. Risk equivalent advice can guide consistent, robust and accountable management decision-making in a changing world, even in the absence of perfect knowledge. It is applicable to all human activity sectors and can be extended to handle variation and change in economics and governance aspects of management systems, as human societies adapt to change. Concepts of risk and risk equivalence provide a common language and understanding for consistent communication of environmental and other considerations in management advice as incremental changes in risk.
Publicly available datasets were analysed in the Supplementary Material. This data can be found at: https://waves-vagues.dfo-mpo.gc.ca/Library/40976993.pdf.
M-JR led the writing of the manuscript, with contributions and edits from DD, JR, and KH. All authors were involved in the development of research and ideas brought forward in the manuscript.
Funding provided under the DFO Aquatic Climate Change Adaptation Services Program (ACCASP) in 2018 and 2019, allowed the authors to meet in person to work on the ideas and concepts developed in this manuscript.
The authors declare that the research was conducted in the absence of any commercial or financial relationships that could be construed as a potential conflict of interest.
All claims expressed in this article are solely those of the authors and do not necessarily represent those of their affiliated organizations, or those of the publisher, the editors and the reviewers. Any product that may be evaluated in this article, or claim that may be made by its manufacturer, is not guaranteed or endorsed by the publisher.
Constructive feedbacks on ideas presented in this manuscript and supplementary material were received during the DFO peer review meeting on incorporating climate change in stock assessment advice in May 2018, the DFO Technical Expertise in Stock Assessment (TESA) Workshop on Uncertainty, Risk and Stock Summaries in January 2020, and the peer review meeting on the assessment of northern shrimp stocks in the Estuary and Gulf of Saint-Lawrence, also in January 2020. H. Bourdages provided northern shrimp data and information. M-JR wishes to thank D. Bowden for constructive comments and encouragements in completing this manuscript.
The Supplementary Material for this article can be found online at: https://www.frontiersin.org/articles/10.3389/fclim.2021.781559/full#supplementary-material
Anderson, S. C., Branch, T. A., Cooper, A. B., and Dulvy, N. K. (2017). Black-swan events in animal populations. Proc. Nat. Acad. Sci. U.S.A. 114, 3252–3257. doi: 10.1073/pnas.1611525114
Astles, K. (2015). Linking risk factors to risk treatment in ecological risk assessment of marine biodiversity. ICES J. Mar. Sci. 72, 1116–1132. doi: 10.1093/icesjms/fsu207
Barber, W. E., and Taylor, J. N. (1990). The importance of goals, objectives, and values in the fisheries management process and organization: a review. North Am. J. Fish. Manag. 10, 365–373.
Bentley, J. W., Lundy, M. G., Howell, D., Beggs, S. E., Bundy, A., De Castro, F., et al. (2021). Refining fisheries advice with stock-specific ecosystem information. Front. Mar. Sci. 8:346. doi: 10.3389/fmars.2021.602072
Berger, A. M. (2019). Character of temporal variability in stock productivity influences the utility of dynamic reference points. Fish. Res. 217, 185–197. doi: 10.1016/j.fishres.2018.11.028
Burgman, M. (2005). Risks and decisions for conservation and environmental management. Cambridge: Cambridge University Press.
Burgman, M. A., and Yemshanov, D. (2013). Risks, decisions, and biological conservation. Divers. Distrib. 19, 485–489. doi: 10.1111/ddi.12060
Cheung, W. (2018). The future of fishes and fisheries in the changing oceans. J. Fish Biol. 92, 790–803. doi: 10.1111/jfb.13558
Collie, J. S., Botsford, L. W., Hastings, A., Kaplan, I. C., Largier, J. L., Livingston, P. A., et al. (2016). Ecosystem models for fisheries management: finding the sweet spot. Fish Fish. 17, 101–125. doi: 10.1111/faf.12093
Cotter, J., Lart, W., de Rozarieux, N., Kingston, A., Caslake, R., Le Quesne, W., et al. (2015). A development of ecological risk screening with an application to fisheries off SW England. ICES J. Mar. Sci. 72, 1092–1104. doi: 10.1093/icesjms/fsu167
Cury, P. M., Mullon, C., Garcia, S. M., and Shannon, L. J. (2005). Viability theory for an ecosystem approach to fisheries. ICES J. Mar. Sci. 62, 577–584. doi: 10.1016/j.icesjms.2004.10.007
DFO (2006). “A harvest strategy compliant with the precautionary approach,” in Can. Sci. Advis. Sec. Sci. Advis. Rep., ed. DFO(Ottawa, ON: Canadian Science Advisory Secretariat), 1–7.
Dichmont, C. M., Punt, A. E., Dowling, N., De Oliveira, J. A., Little, L. R., Sporcic, M., et al. (2016). Is risk consistent across tier-based harvest control rule management systems? a comparison of four case-studies. Fish Fishe. 17, 731–747. doi: 10.1111/faf.12142
Dorn, M. W., and Zador, S. G. (2020). A risk table to address concerns external to stock assessments when developing fisheries harvest recommendations. Ecosyst. Health Sustain. 6:1813634. doi: 10.1080/20964129.2020.1813634
Dulvy, N. K., Ellis, J. R., Goodwin, N. B., Grant, A., Reynolds, J. D., and Jennings, S. (2004). Methods of assessing extinction risk in marine fishes. Fish Fish. 5, 255–276. doi: 10.1111/j.1467-2679.2004.00158.x
Duplisea, D. E., Roux, M.-J., Hunter, K. L., and Rice, J. (2021). Fish harvesting advice under climate change: a risk-equivalent empirical approach. PLoS ONE 16:e0239503. doi: 10.1371/journal.pone.0239503
FAO (2003). The ecosystem approach to fisheries. FAO Technical Guidelines for Responsible Fisheries, Vol.4. (New York, NY: FAO). p.12. Available online at: https://www.fao.org/in-action/globefish/publications/details-publication/en/c/346126/
Fay, G., Little, L. R., Tuck, G. N., Haddon, M., and Klaer, N. L. (2012). Maintaining risk equivalency among fishery harvest control rules in the SESSF. Document prepared for the Southern and Eastern Scalefish and Shark Fishery RAG, February 2013. (Sydney, VA: CSIRO). p.22.
Fisheries and Oceans Canada (2019). Fish and Fish Habitat Protection Policy Statement. Ottawa, ON: Fisheries and Oceans Canada.
Francis, R., and Shotton, R. (1997). “Risk” in fisheries management: a review. Can. J. Fish. Aquat. Sci. 54, 1699–1715. doi: 10.1139/f97-100
Frölicher, T. L., and Laufkötter, C. (2018). Emerging risks from marine heat waves. Nat. Commun. 9, 1–4. doi: 10.1038/s41467-018-03163-6
Fu, C., Travers-Trolet, M., Velez, L., Grüss, A., Bundy, A., Shannon, L. J., et al. (2018). Risky business: the combined effects of fishing and changes in primary productivity on fish communities. Ecol. Modell. 368, 265–276. doi: 10.1016/j.ecolmodel.2017.12.003
Fulton, E. A., Punt, A. E., Dichmont, C. M., Gorton, R., Sporcic, M., Dowling, N., et al. (2016). Developing risk equivalent data-rich and data-limited harvest strategies. Fish. Res. 183, 574–587. doi: 10.1016/j.fishres.2016.07.004
Gaichas, S., Link, J., and Hare, J. (2014). A risk-based approach to evaluating northeast US fish community vulnerability to climate change. ICES J. Mar. Sci. 71, 2323–2342. doi: 10.1093/icesjms/fsu048
Gaichas, S. K., DePiper, G. S., Seagraves, R. J., Muffley, B. W., Sabo, M. G., Colburn, L. L., et al. (2018). Implementing ecosystem approaches to fishery management: risk assessment in the US Mid-Atlantic. Front. Mar. Sci. 5:442. doi: 10.3389/fmars.2018.00442
Game, E. T., Kareiva, P., and Possingham, H. P. (2013). Six common mistakes in conservation priority setting. Conserv. Biol. 27, 480–485. doi: 10.1111/cobi.12051
Gibbs, M. T., and Browman, H. I. (2015). Risk assessment and risk management: a primer for marine scientists. ICES J. Mar. Sci. 72, 992–996. doi: 10.1093/icesjms/fsu232
Haas, B., Mackay, M., Novaglio, C., Fullbrook, L., Murunga, M., Sbrocchi, C., et al. (2021). The future of ocean governance. Rev. Fish Biol. Fish. 1–18. doi: 10.1007/s11160-020-09631-x
Hare, J. A., Morrison, W. E., Nelson, M. W., Stachura, M. M., Teeters, E. J., Griffis, R. B., et al. (2016). A vulnerability assessment of fish and invertebrates to climate change on the Northeast US Continental Shelf. PLoS ONE 11:e0146756. doi: 10.1371/journal.pone.0146756
Harwood, J. (2000). Risk assessment and decision analysis in conservation. Biol. Conserv. 95, 219–226. doi: 10.1016/S0006-3207(00)00036-7
Harwood, J., and Stokes, K. (2003). Coping with uncertainty in ecological advice: lessons from fisheries. Trends Ecol. Evol. 18, 617–622. doi: 10.1016/j.tree.2003.08.001
Hobday, A., Smith, A., Stobutzki, I., Bulman, C., Daley, R., Dambacher, J., et al. (2011). Ecological risk assessment for the effects of fishing. Fish. Res. 108, 372–384. doi: 10.1016/j.fishres.2011.01.013
Hodgson, E. E., Essington, T. E., Samhouri, J. F., Allison, E. H., Bennett, N. J., Bostrom, A., et al. (2019). Integrated risk assessment for the blue economy. Front. Mar. Sci. 6:609. doi: 10.3389/fmars.2019.00609
Hollowed, A. B., Holsman, K. K., Haynie, A. C., Hermann, A. J., Punt, A. E., Aydin, K., et al. (2020). Integrated modeling to evaluate climate change impacts on coupled social-ecological systems in Alaska. Front. Mar. Sci. 6:775. doi: 10.3389/fmars.2019.00775
Holsman, K., Samhouri, J., Cook, G., Hazen, E., Olsen, E., Dillard, M., et al. (2017). An ecosystem-based approach to marine risk assessment. Ecosyst. Health Sustain. 3:e01256. doi: 10.1002/ehs2.1256
Holt, C. A., and Michielsens, C. G. (2020). Impact of time-varying productivity on estimated stock–recruitment parameters and biological reference points. Can. J. Fish. Aquat. Sci. 77, 836–847. doi: 10.1139/cjfas-2019-0104
Howell, D., Schueller, A. M., Bentley, J. W., Buchheister, A., Chagaris, D., Cieri, M., et al. (2021). Combining ecosystem and single-species modeling to provide ecosystem-based fisheries management advice within current management systems. Front. Mar. Sci. 7:1163. doi: 10.3389/fmars.2020.607831
ICES (2013). Benchmarks at ICES [Online]. Available online at: https://www.ices.dk/community/Documents/Advice/Introduction%20to%20Benchmarks%20at%20ICES.pdf (accessed september 15, 2021).
ICES (2017). Report of the Workshop on the Development of the ICES Approach to Providing MSY Advice for Category 3 and 4 Stocks (WKMSYCat34), 6-10 March 2017. Copenhagen: ICES.
ICES (2021a). Advice on Fishing Opportunities. Report of the ICES Advisory Committee, 2021. ICES Advice 2021, Section 1.1.1. Copenhagen: ICES.
ICES (2021b). Workshop on Methods and Guidelines to Link Human Activities, Pressures and State of the Ecosystem in Ecosystem Overviews (WKTRANSPARENT; outputs from 2020 meeting). ICES Scientific Reports. Copenhagen: ICES.
IPCC (2019). “Summary for policymakers,” in IPCC Special Report on the Ocean and Cryosphere in a Changing Climate, eds H.-O. Pörtner, D. C. Roberts, V. Masson-Delmotte, P. Zhai, M. Tignor, E. Poloczanska, K. Mintenbeck, A. Alegría, M. Nicolai, A. Okem, J. Petzold, B. Rama, and N.M. Weyer (Geneva: IPCC), p. 1–35.
IPCC (2021). Climate Change 2021: The Physical Science Basis. Contribution of Working Group I to the Sixth Assessment Report of the Intergovernmental Panel on Climate Change. Cambridge: Cambridge University Press.
ISO (2018). Risk management–Principles and Guidelines [Online]. Available online at: https://www.iso.org/obp/ui/#iso:std:iso:31000:ed-2:v1:en (accessed December 14, 2021).
Jones, R. N., Patwardhan, A., Cohen, S. J., Dessai, S., Lammel, A., Lempert, R. J., et al. (2014). “Foundations for decision making,” in Climate Change 2014: Impacts, Adaptation, and Vulnerability. Part A: Global and Sectoral Aspects. Contribution of Working Group II to the Fifth Assessment Report of the Intergovernmental Panel on Climate Change, eds V. R. Barros, D. J. Dokken, K. J. Mach, M. D. Mastrandrea, T. E. Bilir, M. Chatterjee, K. L. Ebi, Y. O. Estrada, R. C. Genova, B. Girma, E. S. Kissel, A. N. Levy, S. MacCracken, P. R. Mastrandrea, L. L. White, and C. B. Field (Cambridge and New York, NY: Cambridge University Press), 195–228.
Kaplan, S., and Garrick, B. J. (1981). On the quantitative definition of risk. Risk Anal. 1, 11–27. doi: 10.1111/j.1539-6924.1981.tb01350.x
King, J., and McFarlane, G. (2006). A framework for incorporating climate regime shifts into the management of marine resources. Fish. Manag. Ecol. 13, 93–102. doi: 10.1111/j.1365-2400.2006.00480.x
King, J. R., McFarlane, G. A., and Punt, A. E. (2015). Shifts in fisheries management: adapting to regime shifts. Philos. Trans. R. Soc. B Biol. Sci. 370:20130277. doi: 10.1098/rstb.2013.0277
Knights, A. M., Piet, G. J., Jongbloed, R. H., Tamis, J. E., White, L., Akoglu, E., et al. (2015). An exposure-effect approach for evaluating ecosystem-wide risks from human activities. ICES J. Mar. Sci. 72, 1105–1115. doi: 10.1093/icesjms/fsu245
Levin, P. S., Fogarty, M. J., Murawski, S. A., and Fluharty, D. (2009). Integrated ecosystem assessments: developing the scientific basis for ecosystem-based management of the ocean. PLoS Biol. 7:e1000014. doi: 10.1371/journal.pbio.1000014
Marshall, K. N., Koehn, L. E., Levin, P. S., Essington, T. E., and Jensen, O. P. (2019). Inclusion of ecosystem information in US fish stock assessments suggests progress toward ecosystem-based fisheries management. ICES J. Mar. Sci. 76, 1–9. doi: 10.1093/icesjms/fsy152
McDaniels, T. L. (2000). Creating and using objectives for ecological risk assessment and management. Environ. Sci. Policy 3, 299–304. doi: 10.1016/S1462-9011(00)00108-8
McFarlane, G. A., King, J. R., and Beamish, R. J. (2000). Have there been recent changes in climate? ask the fish. Progr. Oceanogr. 47, 147–169. doi: 10.1016/S0079-6611(00)00034-3
Mildenberger, T. K., Berg, C. W., Kokkalis, A., Hordyk, A. R., Wetzel, C., Jacobsen, N. S., et al. (2021). Implementing the precautionary approach into fisheries management: biomass reference points and uncertainty buffers. Fish Fish. doi: 10.1111/faf.12599 [Epub ahead of print].
Miller, T. J., Hare, J. A., and Alade, L. A. (2016). A state-space approach to incorporating environmental effects on recruitment in an age-structured assessment model with an application to southern New England yellowtail flounder. Can. J. Fish. Aquat. Sci. 73, 1261–1270. doi: 10.1139/cjfas-2015-0339
Ministry of Fisheries (2008). Harvest Strategy Standard for New Zealand Fisheries. Wellington: New Zealand Government Ministry of Fisheries.
Muffley, B., Gaichas, S., DePiper, G., Seagraves, R., and Lucey, S. (2021). There is no I in EAFM adapting integrated ecosystem assessment for mid-atlantic fisheries management. Coast. Manag. 49, 90–106. doi: 10.1080/08920753.2021.1846156
O'Leary, C. A., Thorson, J. T., Miller, T. J., and Nye, J. A. (2020). Comparison of multiple approaches to calculate time-varying biological reference points in climate-linked population-dynamics models. ICES J. Mar. Sci. 77, 930–941. doi: 10.1093/icesjms/fsz215
Oliver, E. C., Donat, M. G., Burrows, M. T., Moore, P. J., Smale, D. A., Alexander, L. V., et al. (2018). Longer and more frequent marine heatwaves over the past century. Nat. Commun. 9, 1–12. doi: 10.1038/s41467-018-03732-9
Ono, K., Langangen, Ø., and Stenseth, N. C. (2019). Improving risk assessments in conservation ecology. Nat. Commun. 10, 1–7. doi: 10.1038/s41467-019-10700-4
Pecl, G. T., Araújo, M. B., Bell, J. D., Blanchard, J., Bonebrake, T. C., Chen, I.-C., et al. (2017). Biodiversity redistribution under climate change: Impacts on ecosystems and human well-being. Science 355:eaai9214. doi: 10.1126/science.aai9214
Pedreschi, D., Bouch, P., Moriarty, M., Nixon, E., Knights, A. M., and Reid, D. G. (2019). Integrated ecosystem analysis in Irish waters; providing the context for ecosystem-based fisheries management. Fish. Res. 209, 218–229. doi: 10.1016/j.fishres.2018.09.023
Plagányi, É. E., Punt, A. E., Hillary, R., Morello, E. B., Thébaud, O., Hutton, T., et al. (2014). Multispecies fisheries management and conservation: tactical applications using models of intermediate complexity. Fish Fish. 15, 1–22. doi: 10.1111/j.1467-2979.2012.00488.x
Plagányi, É. E., Skewes, T. D., Dowling, N. A., and Haddon, M. (2013). Risk management tools for sustainable fisheries management under changing climate: a sea cucumber example. Clim. Change 119, 181–197. doi: 10.1007/s10584-012-0596-0
Punt, A. E., Siddeek, M., Garber-Yonts, B., Dalton, M., Rugolo, L., Stram, D., et al. (2012). Evaluating the impact of buffers to account for scientific uncertainty when setting TACs: application to red king crab in Bristol Bay, Alaska. ICES J. Mar. Sci. 69, 624–634. doi: 10.1093/icesjms/fss047
Rice, J. (1999). How Complex Should Operational Ecosystem Objectives be? Ecosystem Management–Can We Make It Operational. Copenhagen: ICES.
Sagarese, S. R., Bryan, M. D., Walter, J. F., Schirripa, M., Grüss, A., and Karnauskas, M. (2015). Incorporating Ecosystem Considerations Within the Stock Synthesis Integrated Assessment Model for Gulf of Mexico Red Grouper (Epinephelus morio). North Charleston, SC: Southeast Data Assessment and Review.
Satterthwaite, W. H., Andrews, K. S., Burke, B. J., Gosselin, J. L., Greene, C. M., Harvey, C. J., et al. (2020). Ecological thresholds in forecast performance for key United States West Coast Chinook salmon stocks. ICES J. Mar. Sci. 77, 1503–1515. doi: 10.1093/icesjms/fsz189
Schickele, A., Goberville, E., Leroy, B., Beaugrand, G., Hattab, T., Francour, P., et al. (2021). European small pelagic fish distribution under global change scenarios. Fish Fish. 22, 212–225. doi: 10.1111/faf.12515
Schijns, R., and Pauly, D. (2021). Management implications of shifting baselines in fish stock assessments. Fish. Manag. Ecol. doi: 10.1111/fme.12511. [Epub ahead of print].
Schindler, D. E., and Hilborn, R. (2015). Prediction, precaution, and policy under global change. Science 347, 953–954. doi: 10.1126/science.1261824
Sethi, S. A. (2010). Risk management for fisheries. Fish Fish. 11, 341–365. doi: 10.1111/j.1467-2979.2010.00363.x
Simonovic, S. P. (1997). “Risk in sustainable water resources management,” in Proceedings of Rabat Symposium ‘Sustainable Water Resources Under Increasing Uncertainty (Rabat: IAHS Publ.).
Skern-Mauritzen, M., Ottersen, G., Handegard, N. O., Huse, G., Dingsør, G. E., Stenseth, N. C., et al. (2016). Ecosystem processes are rarely included in tactical fisheries management. Fish Fish. 17, 165–175. doi: 10.1111/faf.12111
Smith, A., Fulton, E., Hobday, A., Smith, D., and Shoulder, P. (2007). Scientific tools to support the practical implementation of ecosystem-based fisheries management. ICES J. Mar. Sci. 64, 633–639. doi: 10.1093/icesjms/fsm041
Smith, D., Punt, A., Dowling, N., Smith, A., Tuck, G., and Knuckey, I. (2009). Reconciling approaches to the assessment and management of data-poor species and fisheries with Australia's harvest strategy policy. Mar. Coast. Fish. Dyn. Manag. Ecosyst. Sci. 1, 244–254. doi: 10.1577/C08-041.1
Smith, S. J., Hunt, J. J., and Rivard, D. (1993). Risk Evaluation and Biological Reference Points for Fisheries Management. Ottawa, ON: NRC Research Press.
Stelzenmüller, V., Coll, M., Mazaris, A. D., Giakoumi, S., Katsanevakis, S., Portman, M. E., et al. (2018). A risk-based approach to cumulative effect assessments for marine management. Sci. Total Environ. 612, 1132–1140. doi: 10.1016/j.scitotenv.2017.08.289
Stock, B. C., and Miller, T. J. (2021). The Woods Hole Assessment Model (WHAM): a general state-space assessment framework that incorporates time-and age-varying processes via random effects and links to environmental covariates. Fish. Res. 240:105967. doi: 10.1016/j.fishres.2021.105967
Thorson, J. T., and Minto, C. (2015). Mixed effects: a unifying framework for statistical modelling in fisheries biology. ICES J. Mar. Sci. 72, 1245–1256. doi: 10.1093/icesjms/fsu213
Travers-Trolet, M., Bourdaud, P., Genu, M., Velez, L., and Vermard, Y. (2020). The risky decrease of fishing reference points under climate change. Front. Mar. Sci. 7:850. doi: 10.3389/fmars.2020.568232
Wilding, T. A., Gill, A. B., Boon, A., Sheehan, E., Dauvin, J. C., Pezy, J.-P., et al. (2017). Turning off the DRIP (‘Data-rich, information-poor')–rationalising monitoring with a focus on marine renewable energy developments and the benthos. Renew. Sustain. Energy Rev. 74, 848–859. doi: 10.1016/j.rser.2017.03.013
Keywords: risk assessment, climate change, risk conditioning factors, management advice, uncertainty communication, ecosystem-based management (EBM)
Citation: Roux M-J, Duplisea DE, Hunter KL and Rice J (2022) Consistent Risk Management in a Changing World: Risk Equivalence in Fisheries and Other Human Activities Affecting Marine Resources and Ecosystems. Front. Clim. 3:781559. doi: 10.3389/fclim.2021.781559
Received: 22 September 2021; Accepted: 07 December 2021;
Published: 17 January 2022.
Edited by:
Peter Haas, University of Massachusetts Amherst, United StatesReviewed by:
Ronald Mitchell, University of Oregon, United StatesCopyright © 2022 Roux, Duplisea, Hunter and Rice. This is an open-access article distributed under the terms of the Creative Commons Attribution License (CC BY). The use, distribution or reproduction in other forums is permitted, provided the original author(s) and the copyright owner(s) are credited and that the original publication in this journal is cited, in accordance with accepted academic practice. No use, distribution or reproduction is permitted which does not comply with these terms.
*Correspondence: Marie-Julie Roux, bWFyaWUtanVsaWUucm91eEBkZm8tbXBvLmdjLmNh
Disclaimer: All claims expressed in this article are solely those of the authors and do not necessarily represent those of their affiliated organizations, or those of the publisher, the editors and the reviewers. Any product that may be evaluated in this article or claim that may be made by its manufacturer is not guaranteed or endorsed by the publisher.
Research integrity at Frontiers
Learn more about the work of our research integrity team to safeguard the quality of each article we publish.