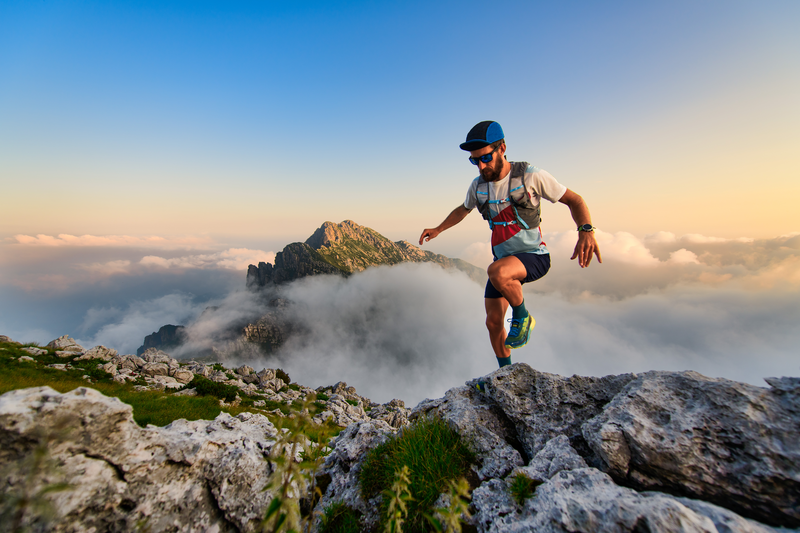
95% of researchers rate our articles as excellent or good
Learn more about the work of our research integrity team to safeguard the quality of each article we publish.
Find out more
PERSPECTIVE article
Front. Clim. , 24 August 2021
Sec. Carbon Dioxide Removal
Volume 3 - 2021 | https://doi.org/10.3389/fclim.2021.684063
This article is part of the Research Topic Governing Carbon Dioxide Removal View all 22 articles
This Perspective explores the local governance of ocean-based carbon dioxide removal (CDR). Proposals to enhance the ability of oceans and marine ecosystems to absorb atmospheric CO2 are often discussed as examples of “geoengineering,” but this framing obscures the site-specific nature of most of the suggested interventions. The Perspective outlines some of the key local dimensions of marine CDR as currently imagined, and suggests a framework for increasing local participation in its assessment. Robust processes of local participation are essential to address the place-based conflicts that are bound to emerge if any of the proposed methods of CO2 removal is ever deployed at scale.
Failure to reduce greenhouse gas emissions at a rate compatible with climate stability has accelerated the search for ways of removing CO2 from the atmosphere. Some proposed interventions involve manipulating oceans and marine ecosystems to increase, or radically enhance, their ability to absorb CO2. “Marine carbon dioxide removal” (CDR) is a fluid category that currently encompasses a highly heterogeneous set of options, from the conservation or restoration of vegetated coastal habitats (“blue carbon”), to alterations in the chemistry of the oceans to boost CO2 uptake (as in artificial ocean alkalinization or ocean iron fertilization). Some of these options, particularly ocean iron fertilization, have a track record of small-scale (and controversial) field experimentation, but the majority remain for the moment limited to preliminary technical assessments and ingenious modeling exercises. Methods such as artificial ocean upwelling and downwelling, or the direct capture of CO2 from seawater, are currently grounded in speculative technological scenarios and have undergone very limited practical assessment (see Gattuso et al., 2021 for a recent review of the field).
Yet we are at an important juncture in the development of marine CDR. Recent policy initiatives suggest growing interest in creating the scientific and technical infrastructures that would make large-scale marine CDR a realistic proposition. In the United States, the National Academies of Sciences, Engineering, and Medicine is preparing a consensus report on CDR and sequestration in coastal and open ocean waters, while governments in Europe and elsewhere are funding the assessment of detailed deployment scenarios. Research consortia and philanthropic initiatives are planning pilot studies, including offshore mesocosm experiments to characterize the ecological impacts of artificial ocean alkalinization (by the European Union-funded OceanNETs consortium, for example), or the spreading of ground olivine on beaches to increase coastal carbon capture (as in the initiatives sponsored by the non-profit Project Vesta).
These initiatives have generated a lively debate over the appropriate governance mechanisms for marine CDR (McGee et al., 2018; Webb, 2020; Boettcher et al., 2021; Cox et al., 2021). While it is difficult to define principles applicable across such a diverse range of potential interventions, it is urgent that we do so. The history of ocean iron fertilization experiments has bequeathed us a very limited range of conceptual tools and governance criteria; it has also consolidated the view of marine CDR as an “oceanic” or “planetary” mode of action, paradigmatic of the fraught moral issues pertaining to “geoengineering,” and best addressed through international regulatory mechanisms. While this framing has served to highlight some of the legal and ethical dimensions of the problem, it obscures the fact that marine CDR, as currently imagined, will in many and relevant ways be site-specific: that it represents a localized form of climate action, affecting coastal communities and environments most immediately, and presenting them with geographically specific balances of risks and benefits. International governance principles and national regulatory frameworks thus need to be complemented with governance processes oriented toward the place-based dimensions of these novel forms of CO2 capture.
In what follows, I review briefly how the controversies surrounding ocean iron fertilization have shaped our understanding of marine CDR governance, tilting it toward planetary considerations. To counterbalance this emphasis, I go on to discuss the site-specific nature of proposed marine CDR methods, with a focus on artificial ocean alkalinization. In the final part of the Perspective I discuss possible ways of tackling the local governance of marine CDR, emphasizing its crucial participatory dimensions—that is, the need to establish mechanisms that would allow those constituencies most directly affected by any decision-making process to shape its outcome. National and international legal frameworks tend to devolve key decisions, such as the definition of what constitutes “legitimate scientific research” or the calculation of the relevant “environmental risks,” to technical experts, and offer limited opportunities for public consultation and review. The legitimacy of marine CDR will require a more inclusive approach, however, able to tackle the local geographies at stake.
Beginning in the late 1990s, a series of ocean iron fertilization experiments crystallized initial positions on the desirability of marine CDR. Expressions of concern about the potential impact of ocean fertilization activities were issued by, among others, the United Nations General Assembly, the United Nations Conference on Sustainable Development, the Conference of the Parties to the Convention on Biological Diversity, and the Intergovernmental Oceanographic Commission of UNESCO. In 2008, the Conference of the Parties to the Convention on Biological Diversity urged national governments “to ensure that ocean fertilization activities do not take place until there is an adequate scientific basis on which to justify such activities, including an assessment of associated risks, and a global, transparent, and effective control and regulatory mechanism is in place for these activities” [Conference on Biological Diversity (CBD), 2008; see also Strong et al., 2009]. That same year, the London Convention and the London Protocol on the Prevention of Marine Pollution by Dumping of Wastes and Other Matter included iron fertilization activities under the scope of its provisions (resolution LC-LP.1 2008). In 2010, the Contracting Parties to the London Convention and London Protocol adopted an “assessment framework for scientific research involving ocean fertilization” that included criteria for the definition of acceptable research activities, and the characterization of attendant environmental risks (LC 32/15, Annex 6).
In 2012, the discharge of iron sulfate and iron oxide by the Haida Salmon Restoration Corporation (HSRC) around the islands of Haida Gwaii, off the coast of British Columbia, triggered a new round of public controversy. Extrapolating from the case of ocean fertilization, the Parties to the Convention on Biological Diversity declared that “there is no single geoengineering approach that currently meets basic criteria for effectiveness, safety, and affordability, and that approaches may prove difficult to deploy or govern” [Conference on Biological Diversity (CBD), 2012]. In 2013, the contracting parties to the London Convention and the London Protocol adopted a resolution (not yet in force) to introduce an amendment “to regulate the placement of matter for ocean fertilization and other marine geoengineering activities” (LC 35/15, Annex 4 (my emphasis); see Webb et al., 2021 for an up-to-date discussion of international law in this area).
What is striking about these debates is the extent to which proponents and opponents alike framed the issue in planetary terms. For the proponents of these ocean fertilization activities, their goal was to assess the potential of induced phytoplankton growth “to influence the carbon budget of our planet” (Assmy et al., 2006). They sought to gather empirical data that would strengthen global models of ocean biochemistry and CO2 uptake. For the critics, the experiments were dangerous, regardless of their scale or immediate scientific purpose, because they “give the wrong signal to the geo-engineers who would like to re-engineer our planet for profit” (ETC Group, 2009; see also Fuentes-George, 2017). Yet, by subsuming these experiments under the rubric of “geoengineering,” the discussions elided crucial local dimensions. This was particularly evident in the case of the fertilization activities in Haida Gwaii. The decision by the Haida community of Old Massett to sponsor HSRC's activities in their coastal waters was driven by a host of complex considerations, including a desire to replenish depleted salmon runs and the prospect of direct financial returns through the sale of carbon credits. It also reflected a very specific experience of vulnerability to climate risk, and of neglect by national policy-makers. As Gannon and Hulme point out “when the HSRC is discursively situated within local histories and geographies of (post)colonial indigenous subjugation, resource extraction and Haida battles to restore political autonomy, it is easy to understand how this proposal gained traction within Old Massett” (Gannon and Hulme, 2018, p. 2). Once the debate was framed as a matter of “geoengineering,” however, these “local histories and geographies” became peripheral. Actors with global reach and purposes—scientific consortia, environmental campaign organizations, international policy-makers—moved to center stage, while constituencies whose interests and concerns were oriented primarily to their specific socio-ecological context were marginalized (see Buck, 2018).
The local character of marine CDR is difficult to visualize when removal practices are imagined in oceanic terms. Graphic depictions of “marine geoengineering” often revolve around lone ships discharging minerals into ocean eddies (as with ocean alkalinity enhancement or iron fertilization), or present free-floating biochemical processes presumably unfolding somewhere in the high seas (as in many illustrations of ocean upwelling and downwelling). Localizing marine CDR is nevertheless crucial if we want to characterize the governance challenge, as it is a necessary condition for identifying the collectives and environments that will be most directly affected by its deployment. It is also crucial for designing mechanisms capable of mitigating the place-based conflicts that are bound to emerge if any of these options is used at scale.
The local nature of marine CDR is most obvious in the case of “blue carbon” strategies, which involve the conservation or restoration of vegetated coastal habitats with high rates of carbon sequestration (e.g., seagrass meadows, mangrove forests, tidal marshes). These strategies are by definition site-specific, and tend to build on existing marine and coastal conservation efforts. While it is difficult to argue a priori against any attempt to protect coastal ecosystems, the history of marine conservation suggests the difficulty of anticipating the full range of social, economic, and environmental impacts at the local level. A large body of social scientific literature on marine protected areas indicates the potential for conflict with residents whose livelihoods and cultural resources are directly or indirectly impacted by conservation efforts, and the challenge of devising interventions that operate synergically (McClanahan et al., 2005; Walley, 2010; Jentoft et al., 2012; Pascual-Fernández et al., 2018; Sowman and Sunde, 2018). A recent analysis of “blue carbon” strategies in Tanzania and Mozambique suggests, for example, multiple points of friction with a wide range of subsistence activities—from the reliance on mangrove forests for fuelwood, to small-scale trawling for fish, and crustaceans in seagrass meadows (Gullström et al., 2021; see also Veitayaki et al., 2017). When placed within what Carton and colleagues call “the long history of carbon removal” (Carton et al., 2020), “blue carbon” represents a new chapter to the genealogy of contentious carbon sequestration. Of particular relevance here is what Ehrenstein calls the “metrological inclusiveness” of carbon sink geopolitics; that is, who is in a position to produce globally accepted evidence of removal and sequestration, and how the uneven distribution of this ability to generate facts shapes the political ecology of the areas tasked with locking up carbon (Ehrenstein, 2018; see also Leach et al., 2012). Rather than being seen as a self-evident public good, the design of sustainable “blue carbon” initiatives requires detailed interdisciplinary research, and a robust process of stakeholder engagement (Thomas, 2014).
Local impacts are bound to be more apparent and less nuanced in the case of ocean afforestation and large-scale seaweed cultivation. Here, fast-growing macroalgae are grown at scale to remove CO2 from the atmosphere through photosynthesis, with the carbon then sequestered through sinking or used to generate “carbon negative” products, as in the production of bioenergy with carbon capture and storage (BECCS). While terrestrial BECCS is probably the best understood (or at least the most extensively modeled) of all proposed large-scale CDR options, we have a very limited sense of how the marine versions of this approach might impact local communities and ecosystems. The experience with farming seaweeds for biofuels and other forms of large-scale mariculture suggests a significant risk of detrimental local environmental impacts (Duarte et al., 2017). Calls to investigate the full range of consequences that BECCS might carry for specific communities are even more pertinent for marine applications of this type of climate mitigation strategy (Buck, 2019a).
The localized character of non-biological forms of marine CDR is more difficult to grasp. This is partly due to the fact that their assessment has so far relied on theoretical models and speculative scenarios that tend to be insensitive toward regional-level dynamics. Models of artificial ocean alkalinization, for example, tend to estimate the “global effectiveness” of the intervention—in terms of the total amount of CO2 extracted from the atmosphere—and assume an even distribution of the added alkalinity across the surface layer of the world's oceans. When they look at specific oceanic regions, they conclude that the site of intervention is immaterial to the scale of CO2 removal, provided enough alkalinity is added (Lenton et al., 2018).
Yet artificial ocean alkalinization at scale, if it ever comes to pass, will be geographically specific in ways that will matter a great deal to its governance. For one, it will require extensive land-based infrastructures for the extraction, processing, and transportation of the required materials. Ocean liming, for example, involves the mining, grinding, and calcination of limestone (plus the capture and storage of most of the resulting CO2, if the process is to result in a net reduction of atmospheric greenhouse gases). Given that transportation will represent a significant proportion of the cost (in both monetary and carbon terms), these infrastructures are likely to be located in or near coastal areas, often in close proximity to ancillary industrial activities (Renforth and Henderson, 2017). In fact, most scenarios for ocean alkalinity enhancement capitalize on already existing industrial activities. Alkalinity enhancement through the addition of magnesium oxide derived from reject brines, for example, implies a co-location with desalination plants (Davies, 2015). The hydrochloric acid that would be generated in the process (which is defined as a hazardous material in most jurisdictions) is also likely to be stored near shore (Webb et al., 2021). The kind of coastal spreading of olivine currently being investigated by Project Vesta would be much more economical if conducted in conjunction with beach nourishment efforts, a kind of “soft” coastal engineering with significant, if poorly understood, impacts on marine environments (Staudt et al., 2021). The point here is that the infrastructures required for artificial ocean alkalinization will likely be built on top of already existing industrial operations on or near shore, potentially intensifying their local environmental impacts even if they were to contribute to a net reduction of atmospheric CO2. It is important to keep in mind, moreover, that most scenarios for artificial ocean alkalinization anticipate decades, if not centuries, of mineral production and discharge if levels of greenhouse gas emissions remain high (Keller et al., 2014).
Comparable co-location effects are evident in early modeling of CO2 stripping. Although some of the proposed scenarios present self-supporting, stand-alone deployments (e.g., “clusters of marine-based floating islands, on which photovoltaic cells convert sunlight into electrical energy to produce H2 and to extract CO2 from seawater,” as in Patterson et al., 2019), the truth of the matter is that these will be, once again, coastal interventions linked to extensive land-based infrastructures, including those required for the transportation and storage of the extracted CO2 (La Plante et al., 2021). Artificial upwelling is often depicted as an untethered process merely replicating the natural circulation of water between ocean layers (and, because it does not require deliberately adding new materials to the sea, falling outside the purview of the London Convention and the London Protocol). Yet for this type of intervention to have any discernible impact on the climate it would involve deploying millions of devices (plastic pipes, pumps, swimming platforms) across large areas of the oceans. Recent field trials reveal the significant infrastructural preconditions for this sort of “non-invasive” climate action (Fan et al., 2020).
There is, in essence, no free-floating marine CDR. Even if key operations take place relatively far from shore and “out of sight,” they are unlikely to be of no concern to coastal actors and communities. Social scientific research on the public acceptability of offshore wind energy, marine oil, and gas extraction, or subsea CO2 storage makes clear that, far from being distant activities unfolding in unpopulated spaces, these industrial activities tend to be seen as directly impacting human landscapes, often as a new chapter in long histories of local resource exploitation and environmental destruction (Firestone and Kempton, 2007; Mabon et al., 2014; Günel, 2019; see also Bertram and Merk, 2020).
Neither an “oceanic” solution nor exclusively land-based, most marine CDR will represent a new kind of inshore practice, a compendium of littoral climate technologies with the potential to reshape the way we relate to the seas. Linking up multiple onshore and offshore activities, the impact of marine CDR will be felt most directly in coastal environments and by nearshore communities. Basic economics suggests that these operations will tend to be co-located with already existing extractive, processing, and transportation activities, potentially exacerbating environmental strains in already vulnerable areas. Optimizing the deployment of marine CDR and characterizing its potential net environmental gain thus requires greater attention to the local and regional scale of assessment.
Yet current discussions of marine CDR governance continue to be framed in planetary terms. This is true of most scientific assessments, which adopt spatially homogeneous deployment scenarios with low regional resolution. It is also true of the legal and policy initiatives that emerged in the wake of the controversies over ocean iron fertilization, which address marine CDR as a form of geoengineering and emphasize the role of international regulatory tools in mitigating transnational risks (Buck, 2019b). While this oceanic understanding of marine CDR reveals key aspects of the problem at hand, it is of little help in navigating the complex place-based governance challenges that are bound to emerge at smaller geographical scales.
Most immediately, this suggests the need to think more rigorously about local participation in the assessment of marine CDR experiments. International governance mechanisms like the London Convention and the London Protocol hinge on the demarcation of “legitimate scientific research,” but they leave the decision of whether any given study has the “proper scientific attributes” to national or international expert bodies (London Convention 32/15). They offer little guidance on how to design a robust participatory process that is attentive to local expectations and concerns beyond the scientific qualities of a proposed experiment. National jurisdictions possess many laws and regulations with potential applicability to experimental marine CDR activities, but the manner in which they should be applied remains uncertain (Webb, 2020), and they allow limited opportunities for local participation in the decision-making process. As a result, the scientific consortia and non-profit initiatives currently planning marine CDR experiments are essentially forced to invent their own, ad hoc approaches to public participation.
A participatory turn in the assessment of marine CDR experimentation must start by expanding the range of actors and factors included in these discussions, as has been argued for greenhouse gas removal technologies more generally (Forster et al., 2020). One possible way to do this would be to consider CDR proposals within existing frameworks for marine spatial planning (MSP). The key advantages of MSP is that it operates at the ecosystem level, takes into account land–sea interactions, and makes explicit the tensions—and also any potential synergies—between alternative uses of marine space. Moreover, in some jurisdictions MSP is supported by legally-binding frameworks that include explicit mandates for transparency, participation, and accountability (as in the EU Marine Spatial Planning Directive).
Incorporating marine CDR into institutionalized spatial planning processes is obviously no guarantee of good governance or of meaningful public participation; the struggle to make MSP planning a properly “public” process, not subordinated to elite interests, remains as urgent as ever (Gopnik et al., 2012; Smith, 2018; Twomey and O'Mahony, 2019). But at least MSP would embed participatory practices within a reasoned consideration of the medium-term socio-ecological impacts of marine CDR. It would visualize potential conflicts with other uses of the marine environment, and help define criteria for their co-existence.
“Blue carbon” provides an obvious starting point for such an approach, as it builds on decades of experience—good and bad—in the governance of coastal conservation areas and carbon sinks. Some regional-level “blue carbon” audits and action plans already draw on the participation of a wide range of stakeholders (Porter et al., 2020), or integrate “blue carbon” into sub-national climate strategies that sanction the involvement of a diverse set of local actors (Wedding et al., 2021; see also Duarte et al., 2017). The road is less clear for marine CDR options with a more oblique link to conservation, and were the potential for far-reaching environmental impacts is much more significant but also much more uncertain. Part of the problem here is that it is more difficult to articulate—let alone quantify—the potential benefits that might accrue at the local or regional level from any given CDR intervention. In this regard, a formal MSP process can be a useful forum to discuss the direct economic benefits that might derive from hosting particular CDR infrastructures, or the allocation of any potential monetary carbon credits associated with CO2 removals.
In sum, tackling marine CDR as a local governance challenge will necessarily shifts the parameters of the discussion. While the oceans trigger understandings of planetary fragility and demands for the protection of the global commons, coastal environments, and the communities they support are exposed to more proximate versions of climate risk and must contend with a complex mix of demands upon marine space. Under these circumstances, the governance of marine CDR becomes a vital local matter that cannot be delegated to international agreements or expert working groups. It also becomes entangled with geographically specific imaginaries of climate action and economic development, giving marine CDR a broader range of connotations than those implicit in the concept of “geoengineering.” If, as Bellamy and Geden (2019) have argued, the governance of carbon dioxide removal should be tackled “from the ground up,” marine CDR governance should be understood “from the coast out,” placing the interests, expectations, and concerns of coastal actors at its center.
The original contributions presented in the study are included in the article/supplementary material, further inquiries can be directed to the corresponding author/s.
The author confirms being the sole contributor of this work and has approved it for publication.
This work has been supported by the European Commission's Horizon 2020 Framework Programme through the project OceanNETs (Ocean-based Negative Emissions Technologies), grant agreement No 869357. It has also received support from the ClimateWorks Foundation as part of the project Greenhouse Gas Removal: Governance and Standards for Carbon Neutrality (GASCON), grant 19-1501.
The author declares that the research was conducted in the absence of any commercial or financial relationships that could be construed as a potential conflict of interest.
All claims expressed in this article are solely those of the authors and do not necessarily represent those of their affiliated organizations, or those of the publisher, the editors and the reviewers. Any product that may be evaluated in this article, or claim that may be made by its manufacturer, is not guaranteed or endorsed by the publisher.
Assmy, P., Cisewski, B., Henjes, J., Klaas, C., Sachs, O., Smetacek, V., et al. (2006). Plankton Rain in the Southern Ocean: The European Iron Fertilization Experiment EIFEX. Das AWI in den Jahren 2004 und 2005-Report 2004, 38–41.
Bellamy, R., and Geden, O. (2019). Govern CO2 removal from the ground up. Nat. Geosci. 12, 874–876. doi: 10.1038/s41561-019-0475-7
Bertram, C., and Merk, C. (2020). Public perceptions of ocean-based carbon dioxide removal technologies: the nature-engineering divide. Front. Clim. 2:31. doi: 10.3389/fclim.2020.594194
Boettcher, M., Brent, K., Buck, H. J., Low, S., McLaren, D., and Mengis, N. (2021). Navigating potential hype and opportunity in governing marine carbon removal. Front. Clim. 3:47. doi: 10.3389/fclim.2021.664456
Buck, H. J. (2018). “Village science meets global discourse: the Haida salmon restoration corporation's ocean iron fertilization experiment,” in Geoengineering Our Climate?: Ethics, Politics, and Governance, eds J. J. Blackstock and S. Low (London: Earthscan-Routledge). 107–112.
Buck, H. J. (2019a). Challenges and opportunities of bioenergy with carbon capture and storage (BECCS) for communities. Curr. Sustain. Renew. Energy Rep. 6, 124–130. doi: 10.1007/s40518-019-00139-y
Buck, H. J. (2019b). After Geoengineering: Climate Tragedy, Repair, and Restoration. London: Verso Trade.
Carton, W., Asiyanbi, A., Beck, S., Buck, H. J., and Lund, J. F. (2020). Negative emissions and the long history of carbon removal. Wiley Interdiscipl. Rev. Clim. Change 11:e671. doi: 10.1002/wcc.671
Conference on Biological Diversity (CBD) (2012) Decision adopted by the Conference of the Parties to the Convention on Biological Diversity at its Eleventh Meeting. 8–19 October 2012 (Hyderabad).
Conference on Biological Diversity (CBD). (2008). Decision adopted by the Conference of the Parties to the Convention on Biological Diversity at its Ninth Meeting. 19–30 May 2008 (Bonn).
Cox, E., Boettcher, M., Spence, E., and Bellamy, R. (2021). Casting a wider net on ocean NETs. Front. Clim. 3:4. doi: 10.3389/fclim.2021.576294
Davies, P. A. (2015). Solar thermal decomposition of desalination reject brine for carbon dioxide removal and neutralisation of ocean acidity. Environ. Sci. Water Res. Technol. 1, 131–137. doi: 10.1039/C4EW00058G
Duarte, C. M., Wu, J., Xiao, X., Bruhn, A., and Krause-Jensen, D. (2017). Can seaweed farming play a role in climate change mitigation and adaptation?. Front. Mar. Sci. 4:100. doi: 10.3389/fmars.2017.00100
Ehrenstein, V. (2018). Carbon sink geopolitics. Econ. Soc. 47, 162–186. doi: 10.1080/03085147.2018.1445569
ETC Group. Throwing Precaution (and iron) to the Wind (and waves). News Release (2009). Available online at: https://www.etcgroup.org/content/lohafex-update-throwing-precaution-and-iron-wind-and-waves (accessed on August 7, 2021).
Fan, W., Zhang, Z., Yao, Z., Xiao, C., Zhang, Y., Zhang, Y., et al. (2020). A sea trial of enhancing carbon removal from Chinese coastal waters by stimulating seaweed cultivation through artificial upwelling. Appl. Ocean Res. 101:102260. doi: 10.1016/j.apor.2020.102260
Firestone, J., and Kempton, W. (2007). Public opinion about large offshore wind power: underlying factors. Energy Policy 35, 1584–1598. doi: 10.1016/j.enpol.2006.04.010
Forster, J., Vaughan, N. E., Gough, C., Lorenzoni, I., and Chilvers, J. (2020). Mapping feasibilities of greenhouse gas removal: key issues, gaps and opening up assessments. Glob. Environ. Change 63:102073. doi: 10.1016/j.gloenvcha.2020.102073
Fuentes-George, K. (2017). Consensus, certainty, and catastrophe: discourse, governance, and ocean iron fertilization. Glob, Environ. Polit. 17, 125–143. doi: 10.1162/GLEP_a_00404
Gannon, K. E., and Hulme, M. (2018). Geoengineering at the “Edge of the World”: exploring perceptions of ocean fertilisation through the Haida Salmon Restoration Corporation. Geo Geogr. Environ. 5:e00054. doi: 10.1002/geo2.54
Gattuso, J. P., Williamson, P., Duarte, C. M., and Magnan, A. K. (2021). The potential for ocean-based climate action: negative emissions technologies and beyond. Front. Clim. 2:37. doi: 10.3389/fclim.2020.575716
Gopnik, M., Fieseler, C., Cantral, L., McClellan, K., Pendleton, L., and Crowder, L. (2012). Coming to the table: early stakeholder engagement in marine spatial planning. Mar. Policy 36, 1139–1149. doi: 10.1016/j.marpol.2012.02.012
Gullström, M., Dahl, M., Lindén, O., Vorhies, F., Forsberg, S., Ismail, R. O., et al. (2021). Coastal Blue Carbon Stocks in Tanzania and Mozambique. IUCN, Global Marine and Polar Programme.
Günel, G. (2019). Spaceship in the Desert: Energy, Climate Change, and Urban Design in Abu Dhabi. https://www.google.com/search?rlz=1C1CHWA_enIN952IN954&sxsrf=ALeKk00_w0j9-GLSCSL6sQF-zt_iGGCKCw:1627746975962&q=Durham,+North+Carolina&stick=H4sIAAAAAAAAAOPgE-LSz9U3MLIwS4qvUOIEsQ2NMyrKtLSyk63084vSE_MyqxJLMvPzUDhWGamJKYWliUUlqUXFi1jFXEqLMhJzdRT88otKMhScE4vyczLzEnewMgIAr2hEP2EAAAA&sa=X&ved=2ahUKEwjX36SC1o3yAhVvgdgFHcCQBCkQmxMoATApegQIMxAD NC: Duke University Press. doi: 10.1215/9781478002406
Jentoft, S., Pascual-Fernandez, J. J., De la Cruz Modino, R., Gonzalez-Ramallal, M., and Chuenpagdee, R. (2012). What stakeholders think about marine protected areas: case studies from Spain. Hum. Ecol. 40, 185–197. doi: 10.1007/s10745-012-9459-6
Keller, D. P., Feng, E. Y., and Oschlies, A. (2014). Potential climate engineering effectiveness and side effects during a high carbon dioxide-emission scenario. Nat. Commun. 5, 1–11. doi: 10.1038/ncomms4304
La Plante, E. C., Simonetti, D. A., Wang, J., Al-Turki, A., Chen, X., Jassby, D., et al. (2021). Saline water-based mineralization pathway for gigatonne-scale CO2 management. ACS Sustain. Chem. Eng. 9, 1073–1089. doi: 10.1021/acssuschemeng.0c08561
Leach, M., Fairhead, J., and Fraser, J. (2012). Green grabs and biochar: revaluing African soils and farming in the new carbon economy. J. Peas. Stud. 39, 285–307. doi: 10.1080/03066150.2012.658042
Lenton, A., Matear, R. J., Keller, D. P., Scott, V., and Vaughan, N. E. (2018). Assessing carbon dioxide removal through global and regional ocean alkalinization under high and low emission pathways. Earth Syst. Dyn. 9, 339–357. doi: 10.5194/esd-9-339-2018
Mabon, L., Shackley, S., and Bower-Bir, N. (2014). Perceptions of sub-seabed carbon dioxide storage in Scotland and implications for policy: a qualitative study. Mar. Policy 45, 9–15. doi: 10.1016/j.marpol.2013.11.011
McClanahan, T., Davies, J., and Maina, J. (2005). Factors influencing resource users and managers' perceptions towards marine protected area management in Kenya. Environ. Conserv. 32, 42–49. doi: 10.1017/S0376892904001791
McGee, J., Brent, K., and Burns, W. (2018). Geoengineering the oceans: an emerging frontier in international climate change governance. Aust. J. Marit. Ocean Aff. 10, 67–80. doi: 10.1080/18366503.2017.1400899
Pascual-Fernández, J. J., De la Cruz Modino, R., Chuenpagdee, R., and Jentoft, S. (2018). Synergy as strategy: learning from La Restinga, Canary Islands. Marit. Stud. 17, 85–99. doi: 10.1007/s40152-018-0091-y
Patterson, B. D., Mo, F., Borgschulte, A., Hillestad, M., Joos, F., Kristiansen, T., et al. (2019). Renewable CO2 recycling and synthetic fuel production in a marine environment. Proc. Nat. Acad. Sci. U.S.A. 116, 12212–12219. doi: 10.1073/pnas.1902335116
Porter, J., Austin, W., Burrows, M., Clarke, D., Davies, G., Kamenos, N., et al. (2020). Blue Carbon Audit of Orkney Waters. Aberdeen: Marine Scotland Science.
Renforth, P., and Henderson, G. (2017). Assessing ocean alkalinity for carbon sequestration. Rev. Geophys. 55, 636–674. doi: 10.1002/2016RG000533
Smith, G. (2018). Good governance and the role of the public in Scotland's marine spatial planning system. Mar. Policy 94, 1–9. doi: 10.1016/j.marpol.2018.04.017
Sowman, M., and Sunde, J. (2018). Social impacts of marine protected areas in South Africa on coastal fishing communities. Ocean Coast. Manage. 157, 168–179. doi: 10.1016/j.ocecoaman.2018.02.013
Staudt, F., Gijsman, R., Ganal, C., Mielck, F., Wolbring, J., Hass, H. C., et al. (2021). The sustainability of beach nourishments: a review of nourishment and environmental monitoring practice. J. Coast. Conserv. 25, 1–24. doi: 10.1007/s11852-021-00801-y
Strong, A., Chisholm, S., Miller, C., and Cullen, J. (2009). Ocean fertilization: time to move on. Nature 461, 347–348. doi: 10.1038/461347a
Thomas, S. (2014). Blue carbon: knowledge gaps, critical issues, and novel approaches. Ecol. Econ. 107, 22–38. doi: 10.1016/j.ecolecon.2014.07.028
Twomey, S., and O'Mahony, C. (2019). “Stakeholder processes in marine spatial planning: ambitions and realities from the European Atlantic experience,” in Maritime Spatial Planning, eds J. Zaucha and K. Gee (Cham: Palgrave Macmillan) 295–325. doi: 10.1007/978-3-319-98696-8_13
Veitayaki, J., Waqalevu, V., Varea, R., and Rollings, N. (2017). “Mangroves in small island development states in the Pacific: an overview of a highly important and seriously threatened resource,” in Participatory Mangrove Management in a Changing Climate, eds R. DasGupta and S. Rajib (Tokyo: Springer), 303–327. doi: 10.1007/978-4-431-56481-2_19
Walley, C. J. (2010). Rough Waters: Nature and Development in an East African Marine Park. Princeton, NJ: Princeton University Press.
Webb, R. (2020). The Law of Enhanced Weathering for Carbon Dioxide Removal. New York, NY: Sabin Center for Climate Change Law, Columbia Law School.
Webb, R., Silverman-Roati, K., and Gerrard, M. (2021). Removing Carbon Dioxide Through Ocean Alkalinity Enhancement and Seaweed Cultivation: Legal Challenges and Opportunities. Sabin Center for Climate Change Law, Columbia Law School, Columbia Public Law Research Paper Forthcoming.
Keywords: carbon dioxide removal, geoengineering, governance, marine environment, ocean-based carbon dioxide removal, ocean alkalinity enhancement, ocean iron fertilization
Citation: Lezaun J (2021) Hugging the Shore: Tackling Marine Carbon Dioxide Removal as a Local Governance Problem. Front. Clim. 3:684063. doi: 10.3389/fclim.2021.684063
Received: 22 March 2021; Accepted: 28 July 2021;
Published: 24 August 2021.
Edited by:
Emily Cox, Cardiff University, United KingdomReviewed by:
Rafael Mattos Dos Santos, University of Guelph, CanadaCopyright © 2021 Lezaun. This is an open-access article distributed under the terms of the Creative Commons Attribution License (CC BY). The use, distribution or reproduction in other forums is permitted, provided the original author(s) and the copyright owner(s) are credited and that the original publication in this journal is cited, in accordance with accepted academic practice. No use, distribution or reproduction is permitted which does not comply with these terms.
*Correspondence: Javier Lezaun, amF2aWVyLmxlemF1bkBpbnNpcy5veC5hYy51aw==
Disclaimer: All claims expressed in this article are solely those of the authors and do not necessarily represent those of their affiliated organizations, or those of the publisher, the editors and the reviewers. Any product that may be evaluated in this article or claim that may be made by its manufacturer is not guaranteed or endorsed by the publisher.
Research integrity at Frontiers
Learn more about the work of our research integrity team to safeguard the quality of each article we publish.