- 1Department of Space, Earth and Environment, Chalmers University of Technology, Gothenburg, Sweden
- 2Department of Economics, Gothenburg University, Gothenburg, Sweden
Pulp mills, as large biogenic CO2 point sources, could adopt Bio Energy Carbon Capture and Storage (BECCS) through retrofitting carbon capture. These existing carbon sources constitute a great potential to roll out BECCS on commercial scale. Yet, despite political targets for negative emission production in Sweden, no incentive schemes were thus far enacted. While previous proposals focus on governmental compensation, the aim of this work is to set BECCS into the supply chain of a wide array of consumer products and thereby find alternative or complementary, business-driven, ways to incentivise BECCS when applied to the pulp and paper industry. In this work, we assess a “value proposition” for low-carbon products in supply chains linked to the pulp and paper industry. By projecting the costs and negative emissions related to BECCS from the pulp mill to typical consumer products, as exemplified by three case study products, we show how BECCS can substantially reduce the carbon footprint of the consumer products, while only marginally increasing their cost. Additional price premiums could shorten the payback period of the initial investment in BECCS. The developed business case presents how actors along the supply chain for pulp and paper products can collectively contribute to securing financing and to mitigating investment risks. The results challenge the private sector, i.e., the companies along the pulp-and-paper supply chain to commit considerable investments also in the case without or with too weak direct political incentives. We conclude by discussing the governance implications on corporate and public level to enable the collaborative “bottom-up” adoption of BECCS.
Introduction
Limiting the average global temperature increase to “well below 2°C” requires a move to net-zero emissions of greenhouse gases by around 2050. In addition to massive decarbonisation in all sectors, this will require the application of negative-emission technologies (NETs), which enable Carbon Dioxide Removal (CDR) from the atmosphere (IPCC, 2018; Luderer et al., 2018; Kaya et al., 2019). CDR could contribute to offsetting hard-to-mitigate emissions, compensating for an emission overshoot, reducing uncertainty in earth system development or limiting the overall costs of climate change mitigation (Fuss et al., 2018; Rogelj et al., 2018; Bednar et al., 2019; Geden et al., 2019).
While previous research largely focused on the global potential and technical development of NETs, in order to reduce costs and improve performance (supply-side), there has been less emphasis on the adoption of NETs (demand-side) (Nemet et al., 2018). This is why many studies have called for research, investments and demonstration projects to embark on the scaling up of NETs (Fuss et al., 2018; van Vuuren et al., 2018). Fuss et al. (2018) discuss the path to negative emissions, suggesting to start with NETs that are immediately available including nature based approaches like afforestation, reforestation and soil carbon sequestration, while developing technology based approaches with more reliable, long term geological storage, like Bio-Energy Carbon Capture and Storage (BECCS) and Direct Air Capture and Storage. Indeed, BECCS is the NET that has hitherto received the most attention (Minx et al., 2018; The Royal Society, 2018; Rickels et al., 2019), but even though the Carbon Capture and Storage (CCS) technology can be seen as mature (Bui et al., 2018; IEA, 2020), actual practical implementation falls short of previous expectations (IEA, 2019).
As main barrier to CDR, studies bring forward their minor role in the political debates (Geden et al., 2019; Fuss et al., 2020) and the lack of political will to engage and lead the development of NETs and the surrounding governance structures (Fuss et al., 2016; Peters and Geden, 2017; Fridahl and Bellamy, 2018; Geden et al., 2019). For CCS and specifically BECCS this translates to a lack of economic incentives (Fridahl and Lehtveer, 2018; Nemet et al., 2018); legal uncertainties, e.g., related to storing CO2 (Heffron et al., 2018); unclear CO2 accounting rules for captured biogenic emissions (Zakkour et al., 2014; Torvanger, 2019); and accordingly, a lack of interest from the private sector, due to the unclear market potential (Platt et al., 2018).
UN, European as well as Swedish policies have thus far failed to incentivise BECCS, yet removed legal barriers to its implementation (Fridahl and Bellamy, 2018; Heffron et al., 2018; Rickels et al., 2020). However, the EU and Sweden plan the deployment of NETs in order to reach their carbon neutrality targets for 2050 and 2045, respectively, combining both nature-based approaches and technology-based approaches. The minor role of CDR in the EU is so far focused on nature based NETs with slight non-nature-based CDR aspirations (Geden and Schenuit, 2020), yet without existing plans to incentivise BECCS or the like (Rickels et al., 2020). The Swedish climate policy framework places an emphasis on negative emissions after 2045. An extensive strategy and action plan, developed for the government, published in early 2020, suggests the deployment of a minimum of 1.8 Mt CDR though BECCS by 2030 and 3–10 Mt CO2 in 2045 (Klimatpolitiska vägvalsutredningen, 2020). To incentivise BECCS deployment the plan suggests reverse auctioning, allowing installations to bid for the minimum acceptable compensation per stored tonne of CO2. However, final decisions are yet to be taken (cf. Bellamy et al., 2021).
Proposals on how to create a demand for NETs have considered different forms of carbon pricing (Nemet et al., 2018; Zetterberg et al., 2019; Rickels et al., 2020), liabilities to provide negative emission certificates if fossil CO2 is emitted (Zetterberg et al., 2019), carbon utilisation as a niche market (Nemet et al., 2018), the “Sustainable Development Mechanism” under the Paris agreement (Honegger and Reiner, 2018), and connecting the co-benefits of NETs to other fields of policymaking (Cox and Edwards, 2019). However, with the exception of Platt et al. (2018), who assessed different business models for negative emissions and associated revenues, and Bellamy and Geden (2019), who called on policy makers to support NETs emerging “bottom-up” at the company or regional level, the role of the private sector in creating a demand for NETs remains largely unexplored.
The prevailing perspective, as discussed above, limits BECCS incentives to governmental compensation of actors that could directly deploy BECCS. With this study we want to challenge this perspective of BECCS deployment as sole political task. Instead we formulate BECCS as an innovation initiative within companies' own supply chains. This calls for Corporate Governance which involves multiple stakeholders (stakeholder approach) and lays an emphasis on the firms contribution to society (political Corporate Social Responsibility) (Scherer and Voegtlin, 2020). Existing decarbonisation initiatives in energy-intensive industries show the importance of this perspective as innovation driver in companies (Knoop et al., 2019).
In the present study, the aim was to explore the prospects for the pulp and paper industry (PPI) to adopt BECCS. The PPI processes large volumes of biomass and pulp mills could be retrofitted with a capturing plant. The PPIs annual BECCS potential was estimated to 60 MtCO2 in Europe (Jönsson and Berntsson, 2012) and 20 MtCO2 in Sweden (Hansson et al., 2017; Rootzén et al., 2018). The Swedish PPI, the largest pulp producer in Europe (CEPI, 2017) with CO2 emissions that are mainly of biogenic origin (97%) (Rootzén et al., 2018), is used as a case study. However, currently no BECCS plant is in operation within the Swedish PPI and the industry is reluctant to drive BECCS adoption since they do not see a suitable and profitable business case (Rodriguez et al., 2020).
The actors in the PPI do not see a market demand for negative emissions (Rodriguez et al., 2020) and as basic material producer it will be difficult for the PPI to create a market for more expensive pulp produced with BECCS. Therefore, we argue that the supply chain needs to be included to assess the market potential. Our analysis thus presents the impact on carbon footprint and costs that BECCS has on consumer products, i.e., end-use products of pulp and paper production, exemplified by three case study products. The customers' willingness-to-pay for these products could be increased, rewarding the climate change mitigation, as discussed in more detail below. Hence, we also analysed the effects on the involved actors of introducing a price premium on the products' retail prices. The price premium, higher than the additional cost, could create a revenue stream to incentivise BECCS adoption. The revenue can be used to compensate for risk-taking and to shorten the payback period of the original BECCS investment.
With the new perspective we present a vision for BECCS commercialisation which could be applied as an alternative or complementary to political incentives, i.e., a way forward to private-sector demand-pull. We discuss proactive corporate governance as innovation driver and how low carbon products could be a core of the BECCS business model.
Method
A pulp mill operator who decides to integrate carbon capture in the pulp mill will increase the production costs and will need to increase the price of pulp to recoup the investment. However, at the same time, negative emissions are “created.” This work proposes that the negative emissions are attributed to the product—the pulp—and that these emission reductions and their associated costs can be transferred through the supply chain so that the end-products of the pulp can be sold at a corresponding higher price. We refer to pulp associated with net-negative CO2 emissions as “CDR pulp.”
Consumer products that use such “CDR pulp” could allow for the introduction of a price premium. This is because a value proposition for a product is multi-dimensional (Rintamäki and Kirves, 2017), and can be increased by improvements to the sustainability performance (Lacoste, 2016; Bangsa and Schlegelmilch, 2020). These include, as examples, lowering the carbon footprint and contributing to the development of BECCS as such. The revenue of the price premium could contribute to de-risking and incentivising the commitment to BECCS.
BECCS in Pulp Production
The sulphate pulping process is deemed most suitable for a BECCS retrofit (Jönsson and Berntsson, 2012). Pulp fibres are thereby obtained by dissolving the non-fibre material of pulpwood, which is thereafter combusted to generate energy. This combustion and a chemical cleaning are the main CO2 sources in a sulphate pulp mill, both emitting CO2 of biogenic origin (cf. Onarheim et al., 2017a). The retrofitting potential was studied in several techno economic analyses (Möllersten, 2002; Hektor and Berntsson, 2007, 2009; Hektor, 2008; McGrail et al., 2012; Jönsson et al., 2013; Garðarsdóttir et al., 2014, 2018; Hedström, 2014; Onarheim et al., 2017a,b; Skagestad et al., 2018; Kuparinen et al., 2019; Nwaoha and Tontiwachwuthikul, 2019).
As a reference for BECCS retrofitting in sulphate pulp mills, we use the recent techno-economic evaluations carried out by Onarheim et al. (2017a,b) and Skagestad et al. (2018) (see Appendix A for a comparison of the studies and their assumptions). The basis for our analysis are their estimates of negative emissions produced per air dry tonne of pulp (negative emissions per ADt of pulp) and the corresponding cost for capture, compression, transport and storage (additional costs per ADt of pulp). We take the average of those technical set-ups in which more than 60% of the total emissions are captured (resulting in an average capture rate of 70%, see Supplementary Table 2) and assume an allocation of costs and negative emissions to all the produced pulp. The cost of pulp production increases then by CostBECCS,pulp= 110 EUR (range, 75–170 EUR) per air-dried tonne, and per air-dried tonne of pulp EBECCS,pulp= 1.6 tonnes (range, 1.4–2.3 tonnes) of biogenic CO2 emissions can be captured (combining stand-alone pulp mills and integrated pulp and paper mills). The corresponding cost for negative emissions would be approximately 70 EUR per tonne of captured and stored biogenic CO2. This is significantly higher than the carbon prices in the emissions trading systems currently in force (e.g., the EU Emissions Trading Scheme; EU ETS).
In the subsequent discussions and analysis we assume that CO2 emissions of biogenic origin during the production, i.e., the wood combusted in pulp mills, are carbon-neutral (O'Sullivan et al., 2016), assuming a managed forest landscape that maintains or increases the carbon stock (Cintas et al., 2016). Correspondingly, all captured and stored biogenic emissions are assumed to be negative emissions, i.e., reducing the CO2 concentration in the atmosphere. Additionally, we assume no leakage in transport and storage.
BECCS on the Consumer Product Level
This section describes how we estimate the pass-through of negative emissions and costs throughout the supply chain of pulp, from the pulp mill to the retailing of the consumer product. Three case products are selected. They represent three of five end-use categories that use sulphate pulp and that are produced in large volumes (for a characterisation of paper grades and their supply chain see Appendix B):
• Paperboard packaging (Case: Liquid packaging board);
• Corrugated board packaging (Case: Moving boxes);
• Graphical wood-free paper (Case: Hardcover book);
• Wrapping paper; and
• Tissue paper.
The negative emissions associated with each tonne of pulp (EBECCS,pulp) and the corresponding cost (CostBECCS,pulp), as presented in the previous section, provide the basis for the analysis. However, during paper production, non-fibrous materials (e.g., fillers, coatings, and chemical additives) can be added to the pulp fibres. For the packaging material and tissue paper, fillers were not usually added, whereas for graphical paper and other papers the shares of fillers increased (Suhr et al., 2015). In addition, pulp is measured as having 10% moisture content, while paper has 6% moisture content (Suhr et al., 2015). Assuming that no fillers are added to the paper, we used the following adjusted estimates in the analysis:
• The amount of negative emissions per mass unit paper, EBECCS, is assumed to be equal to 1.7 tonnes of negative emissions per air-dried tonne of paper (with a range of 1.5–2.4 tCO2/t paper).
• The cost increase per mass unit paper produced, CostBECCS, is assumed to be equal to 117 EUR per air-dried tonne (with a range of 85–180 EUR).
The carbon footprint reduction ΔCFP (g captured and stored biogenic CO2) is calculated based on the mass of virgin sulphate paper in the products mPaper (g paper) and the amount of negative emissions, i.e., captured biogenic emissions per mass unit paper, denoted as EBECCS (g captured and stored biogenic CO2/g paper).
Similarly, the cost impact Δcost (EUR) is calculated based on the cost increase per mass unit of produced paper, denoted as CostBECCS (EUR/g paper).
The cost and amount of negative emissions are calculated under a ceteris paribus assumption, relating both to the current retail price and the carbon footprint. Furthermore, perfect cost pass-through of the additional cost to the end-consumer is assumed.
Revenues and Profits Linked to a Price Premium on Consumer Products
We assume that that a “buyers' coalition” consortium or a vertical joint venture of actors is formed to share the financial and entrepreneurial risks among the actors along the supply chain and connect the investment in BECCS directly to the consumer products. Thus, while the pulp mill company will have to make the investment in the CCS plant, the remaining partners along the supply chain will have to make binding commitments to purchase a certain volume of CDR pulp.
Including an additional price premium to the buyers' coalition consortium set-up [compare supply chain pricing (Voeth and Herbst, 2006)], a break-even analysis for the case of liquid packaging board is performed. We assume a pulp mill with 700,000 tonnes capacity, use the CAPEX and OPEX estimates of Onarheim et al. (2017a,b) and Skagestad et al. (2018) (Supplementary Table 2), and the revenues from price premiums of 1 cent and 4 cents (EUR, before taxes) on the retail price of single consumer liquid packaging board products. The investigated premiums are chosen randomly, but in an actual implementation they could be linked to insights about the respective willingness-to-pay by consumers of different products. Nevertheless, from a practical point of view a premium of 1 cent is the lowest possible price increase of a single product.
For this calculation, we furthermore assume a risk and revenue allocation of 60% to the pulp/paper producer and 40% to the other actors, e.g., the paper-converting and paper-using companies or other transaction costs, although the design of the corresponding partnerships is a matter for agreement. The assumption means that 0.6 cent/package out of the 1 cent price premium reaches the pulp mill. Without this risk sharing the break-even point could even be reached earlier than presented here. However, we introduced risk and revenue sharing to include potential interests of the members of the buyers' coalition, i.e., the consortium of pulp producer, paper converter and paper user, who ensure this payment. If they do not have other costs, they could therefore also earn a profit once they had achieved sufficient sales to cover the guaranteed payment. The remaining 40% could be understood as a buffer to the required market size that they need to provide, i.e., if there is only one partner between the pulp mill and customers that partner only needs to sell 60% of the products with the premium to cover the payment. In a real-life case, the principle applied for risk and revenue sharing along the supply chain needs to be negotiated.
Selection and Analysis of the Case Study Products
The case study products were selected to cover the different paper grades, as well as to represent a variety of end-use categories, with varying cost and price structures and end-use purposes.
The basis for the product analysis is an understanding of the supply chain processes, from production to retail stages, and their contributions to the cost composition and the carbon footprint of the consumer product.
The carbon footprint of a product (E), i.e., the sum of emissions (e) caused by the processes and inputs (j) over the life-stages of the product (Wiedmann and Minx, 2008), is calculated as follows:
As retrofitting BECCS in pulp mills does not change the physical properties of the pulp or the biomass sourcing, the processes in the use and end-of-life phases are assumed to remain unchanged. The baseline for the carbon footprint for the investigated products is, therefore, evaluated by consulting the relevant Life Cycle Assessment (LCA) studies on a cradle-to-gate basis.
Similarly, the cost composition of the consumer product is estimated based on available market statistics on key processes and inputs, deriving from the retail price. Thus, the retail price of the product (C) is assumed to reflect the cost (c) of the processes and inputs (k) involved in the production and sale of the product.
In the following section, the selected case study products are introduced. The ambition here is to provide a magnitude estimate of the changes related to the cost composition and the carbon footprint of the selected consumer product rather than exact values. A detailed description of the current carbon footprint and cost composition are provided in Appendix C.
(A) Case: Liquid packaging board—Oat drink
The first case product is a 1-litre aseptic oat drink in a carton package made of liquid packaging board, which is sold for 1.70 EUR. The carbon footprint estimation is based on an assessment made by CarbonCloud (2019), combined with data describing the climate impact arising from the production of an oat drink obtained from Florén et al. (2013) and data on packaging from Markwardt et al. (2017). We assume the usage of 100% virgin sulphate pulp in the production of the liquid packaging board.
(B) Case: Corrugated board packaging—Moving boxes
In the second case, we investigate a set of corrugated board boxes, with a total weight of 1.87 kg, which are sold as a set of two moving boxes for 2.99 EUR. The carbon footprint estimate is based on an assessment carried out by the European Federation of Corrugated Board Manufacturers (FEFCO, 2019) using data from their European database, focusing on the paper production and conversion (FEFCO, 2018). Corrugated board is a composite of an outside layer of paper (called “liner”) and an internal layer of paper (called “fluting”), which is corrugated and glued to the outside layers. In line with FEFCO (2018), we assume that the moving boxes are made of corrugated boards that comprise 9.5% virgin sulphate paper (Kraftliner).
(C) Case: Graphical wood-free paper—Hardcover book
The third case product is a hardcover book with 300 pages, comprising inner sheets and an outside cover. We assume a retail price of 13 EUR, corresponding to the average prices for entertainment books in Sweden and Germany (Börsenverein des Deutschen Buchhandels, 2015; Wikberg, 2018). The carbon footprint estimation is based on an assessment performed by Pihkola et al. (2010), corresponding to conditions in Finland. These conditions give the mass share of the different paper grades as: 17% cover, 79% inner sheets, 2% end paper, and 2% jacket. The book weighs 500 g (after 28% maculature), of which 100 g are softwood sulphate pulp and 220 g are hardwood sulphate pulp, with the remainder comprising binders and fillers.
Results
Impacts on Carbon Footprint and Cost at the Product Level
Figure 1 shows the current carbon footprint (left side) for each of the three case study products and the different cost components share of the retail price (right side). The comparison shows that for the corrugated board boxes and the hardback book, the production of paper accounts for a relatively high share of the carbon footprint, while the economic value is relatively low. In the case of the oat drink, the shares of paper in the carbon footprint and cost are relatively low. Here, the major contributors to both the carbon footprint and cost are content production and upstream processes related to the production of input materials and electricity.
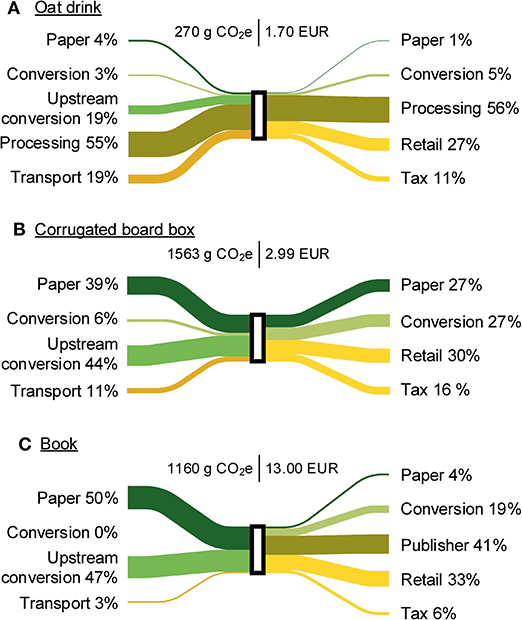
Figure 1. Current composition of the carbon footprint (left) and cost distribution (right) for the three case study products. “Paper” refers to the paper production, “Conversion” refers to the conversion of the paper to packaging or a book, “Upstream conversion” refers to emissions related to the conversion process, i.e., electricity generation or the production of chemicals/ glue, “Transport” refers to the transport-related emissions, and “Content” refers to content production.
Figure 2 shows the estimated cost and carbon footprint impact of BECCS implementation in the PPI for each of the three case study products. The carbon footprints decrease by 14–60%, while the costs increase by up to 0.7%. The oat drinks packaging uses 21.6 g of paper, which results in a cost increase of 0.003 EUR (+0.15%) while the carbon footprint decreases by 37 g (−14%). The corrugated board boxes use 177 g of virgin Kraftliner, which results in a cost increase of 0.021 EUR (+0.7%), while the carbon footprint decreases by 300 g (−19%). The hardback book uses 409 g of sulphate paper (softwood and hardwood), which results in a cost increase of 0.048 EUR (+0.37%), while the carbon footprint decreases by 696 g (−60%). Both changes need to be considered against the background that we assumed an average capture rate of 70% (see Supplementary Table 2) and that an average of the BECCS costs estimates is used, rather than the cheapest options.
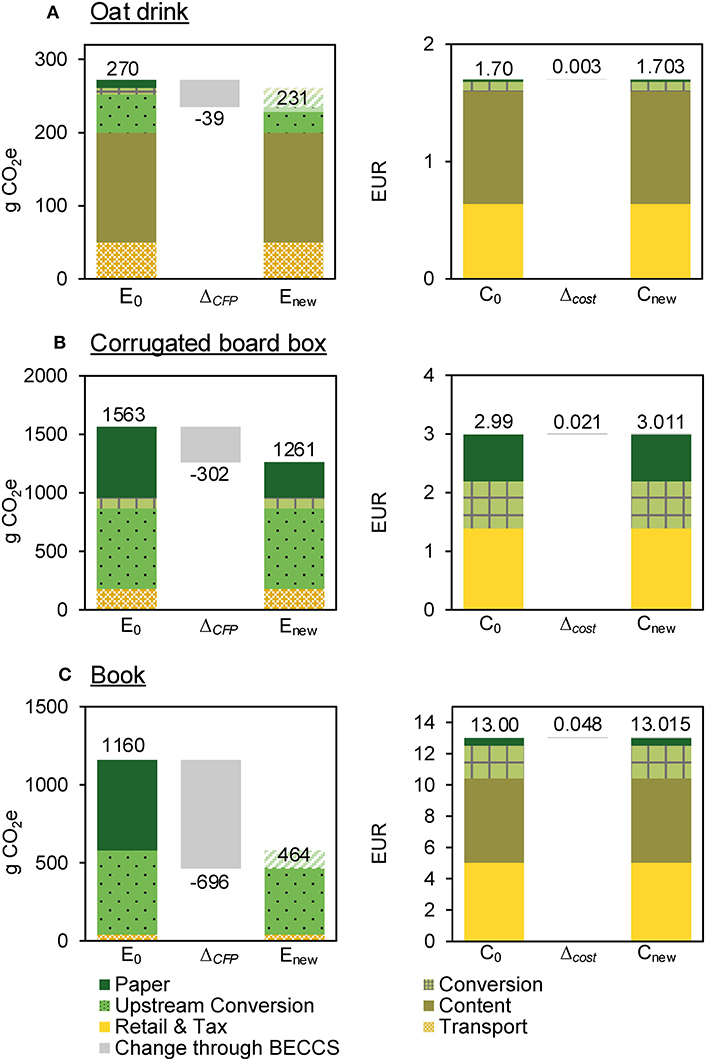
Figure 2. Current carbon footprint E0 and cost C0 compositions of the three case study products, with the potential carbon footprint reduction ΔCFP (left) achieved through applying BECCS at a pulp mill, and the corresponding cost increases Δcost (right). Enew and Cnew. represent the compositions of the carbon footprint and costs after BECCS application.
The results show that, in the case of the oat drink and the hardback book, BECCS can offset more emissions than those originating from paper production. In both cases, we assumed that all the pulp was produced with BECCS. For the corrugated board, only the virgin sulphate paper could be produced with BECCS. The highest relative cost increase linked to BECCS implementation is for the set of moving boxes, which consist entirely of paper. If the same corrugated board was not purchased as a moving box, but instead as packaging for products such as electrical appliances or furniture, the cost increase of 0.02 EUR for the two packaging boxes would be negligible.
The central assumptions made in this study relate to the amount of captured and stored biogenic CO2 per tonne of paper produced (EBECCS) and the cost of captured and storage (CostBECCS). Figure 3 shows the effect of varying both, EBECCS and CostBECCS. The factor used for the captured emissions of 1.7 tCO2/ADt paper is altered ±0.5 (to 1.2 and 2.2 tCO2/ADt paper), and the cost increase of 117 EUR/ ADt paper is altered ±50% (to 58.50 and 175.50 EUR/ADt paper). The results illustrate their linear dependence on the factors and the unchanged magnitude difference of the results. While the carbon footprints decrease by 10%−80%, the costs increase only by up to 1%.
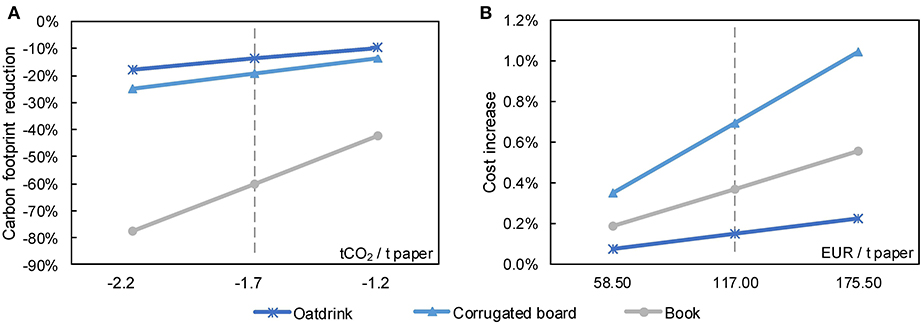
Figure 3. Sensitivity analysis showing: (A) how changes in the amount of captured CO2 per tonne of paper produced (EBECCS) influence the carbon footprint reduction; and (B) how changes in the cost of capture (CostBECCS) influence the estimated cost increase.
Revenue Streams and Profits
Figure 4 shows how a price premium of 0.01 and 0.04 EUR per package of liquid packaging board increases the revenue and shortens the payback period of the BECCS investment. The results show that the higher premium of 0.04 EUR per package allows the pulp producer to break even within the first year of operation. The low premium of 0.01 EUR per package would allow the operator to break even within the first 3 years of operation. This is a large reduction, compared to the assumed operational life-times of 15 years (Onarheim et al., 2017a,b) and 22 years (Skagestad et al., 2018) of the reference BECCS studies (see Supplementary Table 1).
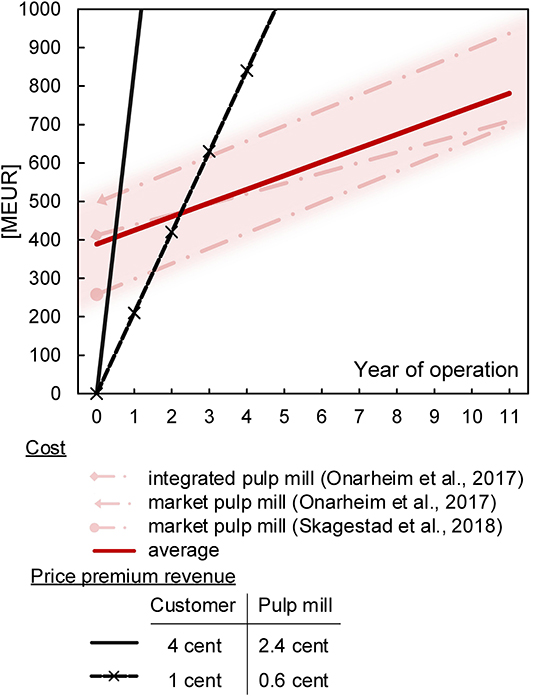
Figure 4. Break-even analysis for a BECCS plant with revenues of a 1 cent and 4 cents price premium on products that use CDR pulp, exemplified by the oat drink case study.
The short payback period becomes feasible due to the large market on which the revenue could be generated and the increased economic value of the paper. While the initial cost of liquid packaging board is 0.02 EUR and the cost of BECCS for the oat drink is 0.003 EUR, the investigated price premiums significantly increase the cash flow of the pulp producer.
Discussion
Setting BECCS deployment into the context of carbon lean production shifts BECCS from a mitigation option at the industrial stacks to being part of a production system of low-carbon products. This incorporates BECCS in the existing field of basic material decarbonisation (Wesseling et al., 2017), Corporate Governance and responsible innovation (von Schomberg, 2012; Scherer and Voegtlin, 2020), sustainable finance (Friede et al., 2015) and corporate and technology forecast (Gordon et al., 2020). This allows to draw comparisons and benefit from a wider field of research and experience, instead of only treating BECCS as a new and unique governance challenge.
Value of Products with BECCS
We suggest that the consumer willingness-to-pay for low-carbon products exceeds the cost of decarbonising pulp production. We investigated this proposition by assuming that the usage of CDR pulp increases the intangible value of consumer products by decreasing the products' climate footprint and by contributing to the development of BECCS as such. However, even though the cost increase for the consumer products may be considered as marginal, the price premium must be borne by the customer. A lack of willingness-to-pay is often presented as a barrier for private led decarbonisation (Wesseling et al., 2017). Examining the extensive field of sustainable-consumption consumer decision-making the willingness-to-pay barrier should be analysed context specifically (Bangsa and Schlegelmilch, 2020). For example, Breustedt (2014) found a substantial willingness-to-pay to offset the carbon footprints of milk and juice products. In line with these arguments, the results of a recent survey of the Swedish population indicate that 70% of Swedes would pay up to 5% more for a company's product if they knew that the company was working on its emissions performance (The Swedish EPA, 2018).
To communicate the reduced carbon footprint to the consumer, the products could be labelled with their carbon footprint or a label stating that the production of the product involved negative emissions. Research around carbon labelling is extensive and can be used to increase the effectiveness, applicability, and practicality of labelling (Liu et al., 2016). If applied in a BECCS financing context, the label would then be used to justify the price premium to the customer and encourage other supply chains to also adopt BECCS in pulp production. Further, while labels will not directly lead to “climate friendly consumption”, a beneficial usage could increase the customers “climate literacy” (Soneryd and Uggla, 2015; Boström and Klintman, 2019). The design of an impactful labelling and communication scheme for the supply chain driven BECCS implementation and its products climate benefit, also including the communication to investors, implies an interaction between Private Actors, Civil Society, and Governments (cf. Lambin and Thorlakson, 2018).
In fact, a carbon-neutral office paper is already being offered by the pulp and paper producer Stora Enso, and the diary company Arla Foods offers all its ecological milk in Sweden with a net-zero-carbon footprint (Arla, 2019; Stora Enso, 2019), indicating that there is a market for such products. In these cases, however, “carbon neutrality” is achieved through the purchase of carbon emissions reduction certificates from voluntary carbon offsetting programs, which are being criticised for the difficulties associated with their verifiability (Lovell and Liverman, 2010; Schneider et al., 2020), for lacking legitimacy, and for being used as a smokescreen by rich countries and companies that are trying to stall their own efforts to reduce emissions (Blum and Lövbrand, 2019). In this context, CCS with standardised mechanisms for storage monitoring should have a much higher degree of verifiability (Allen et al., 2020), yet also faces challenges of public acceptance (Bui et al., 2018; Bellamy et al., 2019). Additionally, the notion of climate neutral biomass is controversial. The net effect of biomass use depends on the assumptions and needs to be analysed context specifically, incorporating for example related land-use change emissions (cf. Creutzig et al., 2015; Cintas et al., 2016).
Political Corporate Social Responsibility as BECCS Incentive
Companies of different sectors increasingly commit to carbon neutral targets, so far more than 1,500 companies with an aggregated revenue exceeding US$ 11.4 trillion adopted these targets (Data-Driven EnviroLab, and NewClimate Institute, 2020). Even though different definitions and strategies can be translated into different relations to carbon dioxide removal (Allen et al., 2020; Data-Driven EnviroLab, and NewClimate Institute, 2020; Science Based Targets Initiative, 2020), this indicates a shifting of corporate governance towards more focus on climate impact—even detached from the product level as discussed here. This includes also Swedish and Finnish PPI companies. Rodriguez et al. (2020) find a willingness of these companies to contribute to BECCS development, yet they do not feel the responsibility to financial commitment. Defining BECCS within the supply chain has therefore the potential to include a wider range of companies into its commercialisation, i.e., in its innovation ecosystem (Adner, 2017; Walrave et al., 2018).
Existing decarbonisation activities in the energy intensive industry already name Corporate Social Responsibility as an important driver (Knoop et al., 2019; Tönjes et al., 2020).
Required Market Size
The “buyers' coalition” consortium as presented here needs to ensure a market for CDR pulp before committing to an investment in BECCS. Using the case study products and assuming respective realistic pulp mill capacity, we estimated the amount of resulting low carbon products. Comparing these estimations with the current market situation reveals that, depending on the sector, single companies would be able to purchase all the CDR pulp from a pulp producer. However, several similar companies should be involved in the consortium in case the required market size exceeds the paper demand of single companies, or their access to the market segment that accepts low carbon products. Please refer to the Appendix D for more details.
Another option to ensure a market for CDR pulp is to reduce the required market size by allocating the climate benefit and cost impact only to a share x% of the produced pulp. The climate benefit and cost of the remaining CDR pulp would then increase inversely by x−1 %. Assuming as an example an allocation to 50% of the pulp, the carbon footprint would decrease by 27%, 39% and 121% for the Oatdrink, Moving box and Hardcover book, respectively. The cost increase would simultaneously double to +0.30%, +1.39%, and +0.74%. In the light of Life Cycle Assessment (LCA) standards, Prado et al. (2020) discussed a similar system of cost and emissions allocation to business units to incentivise investments in environmental improvement in the chemical industry, particularly emphasising the importance of clearly communicating that method. To mitigate the risk of transferring the entire existing production volume into a new market, Pinkse and Kolk (2010) suggested this “hybridisation” approach of offering conventional and improved products in parallel. Furthermore, instead of taking allocation as a means to reduce the market risk, it could also be seen as a possibility to achieve higher offsets or even a negative carbon footprint.
The Investment in BECCS
Besides technological and regulatory challenges previous work often raise economic uncertainties as major barrier to the demonstration and commercialisation of low-carbon technologies (Polzin, 2017). Levihn et al. (2019), for example, have described how economic uncertainties are regarded as the largest barrier to BECCS application at a combined heat and power plant in Stockholm. The investments into pulp mills similarly poses a considerable financial and entrepreneurial risk with investment costs in the range of 43–500 MEUR for a 700,000 tonne/year pulp mill. Therefore, a solid risk management and ownership structure is needed to form a viable business model [see Durusut and Mattos (2018) for the different business model elements, risk forms and the business models of existing industrial CCS installations].
By introducing a small price premium on the consumer products, our analysis confirms the possibility to break-even in the first few years of operation. Building the business model around low carbon products as revenue model and funding source, as suggested here, carries the risk whether a market for low carbon products can be created. To reduce this risk a consortium of actors in the supply chain could be formed (Tönjes et al., 2020). This buyers' coalition consortium, as suggested here, would agree on a purchase agreement for the more-expensive CDR pulp. Similar corporate purchase agreements are exemplified by Apple in the ELYSIS consortium for carbon free aluminium production (Bataille, 2019), the development of a hydrogen and e-fuel production facility backed by several commercial customers (Ørsted, 2020), and is already common within renewable energy development (Miller et al., 2018).
Moreover, political support, i.e., the recognition of and involvement in climate mitigation opportunities that emerge “bottom up” in companies, are of key importance for their success (Pinkse and Kolk, 2010; Bellamy and Geden, 2019; Söderholm et al., 2019; Tönjes et al., 2020). Thus, we do not envision a process without governmental involvement and support, but such should collaboratively support the value proposition of CDR pulp and not only be limited to compensate carbon dioxide removal “at the stack.” Kolster (2019) has highlighted the role of public policies in insuring the financial risk of the investment and ensuring the infrastructure (e.g., for the transportation and long-term storage of CO2), so as to reduce the associated costs of CCS. In fact, the risk management of most current CCS projects is characterised by considerable political involvement, including the public underwriting of risks or loan guarantees (Durusut and Mattos, 2018). However, while the costs are considerable, they should also be interpreted in the context of the already capital-intensive PPI. In the period 2016–2018, single Swedish investments in new facilities and machines were in the range of 400–800 MEUR, comparable to the cost of a full-sized CCS plant. In total, the investments in the Swedish PPI during that period amounted to 4.2 Billion EUR (Skogsindustrierna, 2019).
The investment cost estimates from Onarheim et al. (2017b) and Skagestad et al. (2018), which have been used as the basis for our analysis, are in line with estimates of investment costs for CCS applications in other industries (Garðarsdóttir, 2017). Post-combustion CO2 capture, as applied in this analysis, can be considered to be a mature technology and can also be applied to the PPI (Onarheim et al., 2017b; Bui et al., 2018). However, the presented investment costs are taken from studies that assumed the installation of a Nth-of-a-kind plant. As there is currently no CCS plant operating in a pulp mill, the cost for a first large-scale project is likely to be higher (van der Spek et al., 2019). This includes the development of pilot and demonstration plants, which would likely require public funding to offer important learnings (Mossberg et al., 2020).
Conclusions
This work investigates the potential for “climate-friendly” consumer products to act as enablers of BECCS in the pulp and paper industry. This involves estimating how passing on the costs and negative emissions associated with BECCS would influence the carbon footprints and costs of a selection of consumer products. We show how cooperation between stakeholders in the supply chain could enable the production of products with a substantially reduced carbon footprint (by 14–60%), while increasing the final costs of the products only marginally (<0.7%). We therefore suggest that the consumer willingness-to-pay for low-carbon products could exceed the cost of decarbonising pulp production.
Furthermore, assuming that the value of the products increases more than their cost increases, we investigate the effects of introducing a price premium, which would create a revenue stream that could shorten the pay-back period and generate a profit from BECCS. The results of the break-even analysis show that the BECCS plant can be profitable within the first few years of operation, depending on the premium applied. This means that a minimal charge for single consumer products could enable the implementation of BECCS in pulp production, assuming that the market for such products is sufficiently large.
Even though the possibility to realise the suggested type of buyers' coalition under real market conditions remains uncertain, we believe that the conceptual framework will shed new light on (1) how new forms of proactive corporate governance can lead to collaborations that contribute towards unlocking investments in BECCS and (2) how governments could support “bottom up” emerging BECCS deployment, led by companies.
The set-ups for buyers' coalitions (cf. Bataille, 2019), as suggested here or through transformation funds (Rootzén and Johnsson, 2017), are examples of cross-industry collaboration for low-carbon innovation in energy intensive industries (cf. Tönjes et al., 2020). This new concept allows actors along the supply chains for basic materials, such as pulp and paper, steel and cement, to contribute collectively to securing financing and de-risking investments in low-, zero-, or negative-emission technologies, especially in the scale-up and roll-out phases of new technologies. We conclude that the elaboration and evaluation of such collaboration and financing concepts, which could complement existing climate policy measures and contribute to speeding up the technical transformation of the basic material industry also towards NET deployment, deserves more attention and provides fruitful avenues for future research.
Data Availability Statement
The original contributions presented in the study are included in the article/Supplementary Material, further inquiries can be directed to the corresponding author/s.
Author Contributions
JK and JR together laid out the methodology. JK conducted the analysis and drafted the manuscript under the supervision of JR, FN, and FJ. All authors contributed to manuscript revision and conceptualized the work.
Funding
This work was co-funded by the Swedish innovation agency, Vinnova, and the Swedish Foundation for Strategic Environmental Research, Mistra.
Conflict of Interest
The authors declare that the research was conducted in the absence of any commercial or financial relationships that could be construed as a potential conflict of interest.
Acknowledgments
Valuable comments from two reviewers are greatly appreciated. We also thank Hans Hellsmark for his valuable input to the work.
Supplementary Material
The Supplementary Material for this article can be found online at: https://www.frontiersin.org/articles/10.3389/fclim.2021.615578/full#supplementary-material
References
Allen, M., Axelsson, K., Caldecott, B., Hale, T., Hepburn, C., Hickey, C., et al. (2020). The Oxford Principles for Net Zero Aligned Carbon Offsetting 2020, (Oxford: Smith School of Enterprise and the Environment, University of Oxford).
Arla (2019). Nu har alla våra Arla Ko® EKO-produkter netto noll klimatavtryck. Available online at: https://www.arla.se/om-arla/vart-ansvar/ekologiskt/netto-noll-klimatavtryck/ (accessed November 12, 2019).
Bangsa, A. B., and Schlegelmilch, B. B. (2020). Linking sustainable product attributes and consumer decision-making: insights from a systematic review. J. Clean. Prod. 245:118902. doi: 10.1016/j.jclepro.2019.118902
Bataille, C. (2019). “Low and zero emissions in the steel and cement industries: Barriers, technologies and policies,” in Issue Paper Conference version (Paris).
Bednar, J., Obersteiner, M., and Wagner, F. (2019). On the financial viability of negative emissions. Nat. Commun. 10:1783. doi: 10.1038/s41467-019-09782-x
Bellamy, R., Fridahl, M., Lezaun, J., Palmer, J., Rodriguez, E., Lefvert, A., et al. (2021). Incentivising bioenergy with carbon capture and storage (BECCS) responsibly: Comparing stakeholder policy preferences in the United Kingdom and Sweden. Environ. Sci. Policy 116, 47–55. doi: 10.1016/j.envsci.2020.09.022
Bellamy, R., and Geden, O. (2019). Govern CO2 removal from the ground up. Nat. Geosci. 12, 874–876. doi: 10.1038/s41561-019-0475-7
Bellamy, R., Lezaun, J., and Palmer, J. (2019). Perceptions of bioenergy with carbon capture and storage in different policy scenarios. Nat. Commun. 10:743. doi: 10.1038/s41467-019-08592-5
Blum, M., and Lövbrand, E. (2019). The return of carbon offsetting? The discursive legitimation of new market arrangements in the Paris climate regime. Earth System Governance 2:100028. doi: 10.1016/j.esg.2019.100028
Börsenverein des Deutschen Buchhandels (2015). Buchkäufer und -leser 2015: Profile, Motive, Einstellungen. Available online at: https://www.boersenverein.de/markt-daten/marktforschung/studien-umfragen/buchkaeufer-und-buchleser-studie-2015/ (accessed November 18, 2019).
Boström, M., and Klintman, M. (2019). Can we rely on ‘climate-friendly' consumption? J. Consumer Cult. 19, 359–378. doi: 10.1177/1469540517717782
Breustedt, G. (2014). Demand for Carbon-Neutral Food—Evidence From a Discrete Choice Experiment for Milk and Apple Juice, Kiel. doi: 10.22004/ag.econ.169748
Bui, M., Adjiman, C. S., Bardow, A., Anthony, E. J., Boston, A., Brown, S., et al. (2018). Carbon capture and storage (CCS): the way forward. Energy Environ. Sci. 11, 1062–1176. doi: 10.1039/C7EE02342A
CarbonCloud, A. B. (2019). Report: The Climate Footprint of enriched Oat Drink Ambient: Oat drink Sweden: Oatly, 1.5%. Available online at: https://www.oatly.com/uploads/attachments/ck16jh9jt04k9bggixfg6ssrn-report-the-climate-footprint-of-enriched-oat-drink-ambient-carboncloud-20190917.pdf
CEPI (2017). Key Statistics 2017: European Pulp & Paper industry. Available online at: http://www.cepi.org/keystatistics2017 (accessed January 30, 2019).
Cintas, O., Berndes, G., Cowie, A. L., Egnell, G., Holmström, H., and Ågren, G. I. (2016). The climate effect of increased forest bioenergy use in Sweden: evaluation at different spatial and temporal scales. WIREs Energy Environ. 5, 351–369. doi: 10.1002/wene.178
Cox, E., and Edwards, N. R. (2019). Beyond carbon pricing: policy levers for negative emissions technologies. Climate Policy 19, 1144–1156. doi: 10.1080/14693062.2019.1634509
Creutzig, F., Ravindranath, N. H., Berndes, G., Bolwig, S., Bright, R., Cherubini, F., et al. (2015). Bioenergy and climate change mitigation: an assessment. GCB Bioenergy 7, 916–944. doi: 10.1111/gcbb.12205
Data-Driven EnviroLab and NewClimate Institute. (2020). Accelerating Net Zero: Exploring Cities, Regions, and Companies' Pledges to Decarbonise.
Durusut, E., and Mattos, A. (2018). Industrial carbon Capture Business Models: Report for Department for Business, Energy and Industrial Strategy, Cambridge.
FEFCO (2019). Ecodesign. Available online at: http://www.fefco.org/circular-by-nature/ecodesign
Florén, B., Nilsson, K., and Wallman, M. (2013). LCA på färsk och aseptisk havredryck, (Gothenburg).
Fridahl, M., and Lehtveer, M. (2018). Bioenergy with carbon capture and storage (BECCS): global potential, investment preferences, and deployment barriers. Energy Res. Soc. Sci. 42, 155–165. doi: 10.1016/j.erss.2018.03.019
Fridahl, M., and Bellamy, R. (2018). “Multilevel Policy Incentives for BECCS in Sweden,” in Bioenergy With Carbon Capture and Storage: From Global Potentials to Domestic Realities, ed. M. Fridahl (Stockholm: European Liberal Forum), 57–67.
Friede, G., Busch, T., and Bassen, A. (2015). ESG and financial performance: aggregated evidence from more than 2000 empirical studies. J. Sust. Finance Invest. 5, 210–233. doi: 10.1080/20430795.2015.1118917
Fuss, S., Canadell, J. G., Ciais, P., Jackson, R. B., Jones, C. D., Lyngfelt, A., et al. (2020). Moving toward net-zero emissions requires new alliances for carbon dioxide removal. One Earth 3, 145–149. doi: 10.1016/j.oneear.2020.08.002
Fuss, S., Jones, C. D., Kraxner, F., Peters, G., Smith, P., Tavoni, M., et al. (2016). Research priorities for negative emissions. Environ. Res. Lett. 11:115007. doi: 10.1088/1748-9326/11/11/115007
Fuss, S., Lamb, W. F., Callaghan, M. W., Hilaire, J., Creutzig, F., Amann, T., et al. (2018). Negative emissions—Part 2: costs, potentials and side effects. Environ. Res. Lett. 13, 63002. doi: 10.1088/1748-9326/aabf9f
Garðarsdóttir, S. Ó. (2017). Technical and economic conditions for efficient implementation of CO2 capture: Process design and operational strategies for power generation and process industries (Thesis for the Degree of Doctor of Philosophy). Chalmers University of Technology, Department of Space, Earth and Environment, Göteborg, Sweden.
Garðarsdóttir, S. Ó., Normann, F., Andersson, K., and Johnsson, F. (2014). Process evaluation of CO2 capture in three industrial case studies. Energy Procedia 63, 6565–6575. doi: 10.1016/j.egypro.2014.11.693
Garðarsdóttir, S. Ó., Normann, F., Skagestad, R., and Johnsson, F. (2018). Investment costs and CO2 reduction potential of carbon capture from industrial plants—A Swedish case study. Int. J. Greenhouse Gas Control 76, 111–124. doi: 10.1016/j.ijggc.2018.06.022
Geden, O., Peters, G., and Scott, V. (2019). Targeting carbon dioxide removal in the European Union. Climate Policy 19, 487–494. doi: 10.1080/14693062.2018.1536600
Geden, O., and Schenuit, F. (2020). Unconventional Mitigation: Carbon Dioxide Removal as a New Approach in EU Climate Policy. Berlin: German Institute for International and Security Affairs.
Gordon, A. V., Ramic, M., Rohrbeck, R., and Spaniol, M. J. (2020). 50 Years of corporate and organizational foresight: looking back and going forward. Technol. Forecast. Soc. Change 154:119966. doi: 10.1016/j.techfore.2020.119966
Hansson, J., Hackl, R., Taljegard, M., Brynolf, S., and Grahn, M. (2017). The potential for electrofuels production in sweden utilizing fossil and biogenic CO2 point sources. Front. Energy Res. 5:133. doi: 10.3389/fenrg.2017.00004
Hedström, J. (2014). Simulation and Assessment of Carbon Capture Processes Applied to a Pulp Mill (Master of Science Thesis). Chalmers University of Technology, Department of Energy and Environment Division of Energy Technology, Göteborg, Sweden.
Heffron, R. J., Downes, L., Bysveen, M., Brakstad, E. V., Mikunda, T., Neele, F., et al. (2018). Three layers of energy law for examining CO2 transport for carbon-capture and storage. J. World Energy Law Business 11, 93–115. doi: 10.1093/jwelb/jwx035
Hektor, E. (2008). Post-combustion CO2 Capture In Kraft Pulp and Paper Mills: Technical, Economic and System Aspects. Doktorsavhandlingar. Göteborg: Chalmers University of Technology, Department of Heat & Power Technology.
Hektor, E., and Berntsson, T. (2007). Future CO2 removal from pulp mills—Process integration consequences. Energy Conver. Manag. 48, 3025–3033. doi: 10.1016/j.enconman.2007.06.043
Hektor, E., and Berntsson, T. (2009). Reduction of greenhouse gases in integrated pulp and paper mills: possibilities for CO2 capture and storage. Clean Techn. Environ. Policy 11, 59–65. doi: 10.1007/s10098-008-0166-3
Honegger, M., and Reiner, D. (2018). The political economy of negative emissions technologies: consequences for international policy design. Climate Policy 18, 306–321. doi: 10.1080/14693062.2017.1413322
IEA (2019). CCUS in Industry and Transformation: CCUS in Industry and Transformation. Available online at: https://www.iea.org/tcep/industry/ccus/ (accessed September 24, 2019).
IEA (2020). ETP Clean Energy Technology Guide. IEA. Available online at: https://www.iea.org/articles/etp-clean-energy-technology-guide (accessed September 17, 2019).
IPCC (2018). “Summary for Policymakers,” in Global Warming of 1.5°C: An IPCC Special Report on the Impacts of Global Warming of 1.5°C Above Pre-Industrial Levels and Related Global Greenhouse Gas Emission Pathways, in the Context of Strengthening the Global Response to the Threat of Climate Change, Sustainable Development, and Efforts to Eradicate Poverty, eds. V. Masson-Delmotte, P. Zhai, H.-O. Pörtner, D. Roberts, J. Skea, P.R. Shukla, et al. (Geneva: Intergovernmental Panel on Climate Change), 93–174.
Jönsson, J., and Berntsson, T. (2012). Analysing the potential for implementation of CCS within the European pulp and paper industry. Energy 44, 641–648. doi: 10.1016/j.energy.2012.05.028
Jönsson, J., Pettersson, K., Berntsson, T., and Harvey, S. (2013). Comparison of options for utilization of a potential steam surplus at kraft pulp mills-Economic performance and CO 2 emissions. Int. J. Energy Res. 37, 1017–1035. doi: 10.1002/er.2905
Kaya, Y., Yamaguchi, M., and Geden, O. (2019). Towards net zero CO2 emissions without relying on massive carbon dioxide removal. Sustain. Sci. 354, 182. doi: 10.1007/s11625-019-00680-1
Klimatpolitiska vägvalsutredningen, M. (2020). Vägen till en Klimatpositiv Framtid: The pathway to a Climate Positive Future—Strategy and Action Plan for Achieving Negative Greenhouse Gas Emissions After, 2045.
Knoop, K., Lechtenböhmer, T., Mölter, H., Tönjes, A., and Witte, K. (2019). Drivers of Low-Carbon Innovation: Deliverable 3.6., (Wuppertal: REINVENT).
Kolster, C. (2019). Economic, process and reservoir modelling for evaluating large-scale deployment of carbon capture and storage (Ph.D. Thesis). Imperial College London, Faculty of Natural Sciences, London, United Kingdom.
Kuparinen, K., Vakkilainen, E., and Tynjäl,ä, T. (2019). Biomass-based carbon capture and utilization in kraft pulp mills. Mitig. Adapt Strateg. Glob. Change 10:2491. doi: 10.1007/s11027-018-9833-9
Lacoste, S. (2016). Sustainable value co-creation in business networks. Industrial Market. Manag. 52, 151–162. doi: 10.1016/j.indmarman.2015.05.018
Lambin, E. F., and Thorlakson, T. (2018). Sustainability standards: interactions between private actors, civil society, and governments. Annu. Rev. Environ. Resour. 43, 369–393. doi: 10.1146/annurev-environ-102017-025931
Levihn, F., Linde, L., Gustafsson, K., and Dahlen, E. (2019). Introducing BECCS through HPC to the research agenda: the case of combined heat and power in Stockholm. Energy Rep. 5, 1381–1389. doi: 10.1016/j.egyr.2019.09.018
Liu, T., Wang, Q., and Su, B. (2016). A review of carbon labeling: standards, implementation, and impact. Renew. Sust. Energy Rev. 53, 68–79. doi: 10.1016/j.rser.2015.08.050
Lovell, H., and Liverman, D. (2010). Understanding carbon offset technologies. New Political Eco. 15, 255–273. doi: 10.1080/13563460903548699
Luderer, G., Vrontisi, Z., Bertram, C., Edelenbosch, O. Y., Pietzcker, R. C., Rogelj, J., et al. (2018). Residual fossil CO2 emissions in 1.5–2 °C pathways. Nat. Clim. Chang. 8, 626–633. doi: 10.1038/s41558-018-0198-6
Markwardt, S., Wellenreuther, F., Drescher, A., Harth, J., and Busch, M. (2017). Comparative Life Cycle Assessment of Tetra Pak® Carton Packages and Alternative Packaging Systems for Liquid Food on the Nordic Market: Final Report. Commissioned. Heidelberg: Tetra Pak International SA.
McGrail, B. P., Freeman, C. J., Brown, C. F., Sullivan, E. C., White, S. K., Reddy, S., et al. (2012). Overcoming business model uncertainty in a carbon dioxide capture and sequestration project: case study at the boise white paper mill. Int. J. Greenhouse Gas Control 9, 91–102. doi: 10.1016/j.ijggc.2012.03.009
Miller, L., Carriveau, R., and Harper, S. (2018). Innovative financing for renewable energy project development—recent case studies in North America. Int. J. Environ. Studies 75, 121–134. doi: 10.1080/00207233.2017.1403758
Minx, J. C., Lamb, W. F., Callaghan, M. W., Fuss, S., Hilaire, J., Creutzig, F., et al. (2018). Negative emissions—Part 1: research landscape and synthesis. Environ. Res. Lett. 13:63001. doi: 10.1088/1748-9326/aabf9b
Möllersten, K. (2002). Opportunities for CO2 Reductions and CO2-Lean Energy Systems in Pulp and Paper Mills (Doctoral Thesis). Royal Institute of Technology, Dept. Of Chemical Engineering and Technology/Energy Processes, Stockholm, Sweden.
Mossberg, J., Frishammar, J., Söderholm, P., and Hellsmark, H. (2020). Managerial and organizational challenges encountered in the development of sustainable technology: Analysis of Swedish biorefinery pilot and demonstration plants. J. Clean. Prod, 124150. doi: 10.1016/j.jclepro.2020.124150
Nemet, G. F., Callaghan, M. W., Creutzig, F., Fuss, S., Hartmann, J., Hilaire, J., et al. (2018). Negative emissions—Part 3: innovation and upscaling. Environ. Res. Lett. 13:63003. doi: 10.1088/1748-9326/aabff4
Nwaoha, C., and Tontiwachwuthikul, P. (2019). Carbon dioxide capture from pulp mill using 2-amino-2-methyl-1-propanol and monoethanolamine blend: techno-economic assessment of advanced process configuration. Appl. Energy 250, 1202–1216. doi: 10.1016/j.apenergy.2019.05.097
Onarheim, K., Santos, S., Kangas, P., and Hankalin, V. (2017a). Performance and costs of CCS in the pulp and paper industry part 1: performance of amine-based post-combustion CO 2 capture. Int. J. Greenhouse Gas Control 59, 58–73. doi: 10.1016/j.ijggc.2017.02.008
Onarheim, K., Santos, S., Kangas, P., and Hankalin, V. (2017b). Performance and cost of CCS in the pulp and paper industry part 2: economic feasibility of amine-based post-combustion CO 2 capture. Int. J. Greenhouse Gas Control 66, 60–75. doi: 10.1016/j.ijggc.2017.09.010
Ørsted (2020). Danish companies plan 1.3 GW electrolyser using offshore wind. Fuel Cells Bull. 2020, 8–9. doi: 10.1016/S1464-2859(20)30244-3
O'Sullivan, O. S., Hopkinson, L., Crockatt, M. E., Laird, P., and Bebber, D. P. (2016). Stable forest carbon stocks support current assumption of biogenic carbon neutrality in the case of European-manufactured beverage cartons. Int. J. Life Cycle Assess 21, 118–128. doi: 10.1007/s11367-015-0989-1
Peters, G., and Geden, O. (2017). Catalysing a political shift from low to negative carbon. Nat. Clim Change 7, 619–621. doi: 10.1038/nclimate3369
Pihkola, H., Nors, M., Kujanpää, M., Helin, T., Kariniemi, M., Pajula, T., et al. (2010). Carbon Footprint and Environmental Impacts of Print Products From Cradle to Grave: Results from the LEADER project (Part 1). Helsinki; Espoo: VTT Technical Research Centre of Finland.
Pinkse, J., and Kolk, A. (2010). Challenges and trade-offs in corporate innovation for climate change. Bus. Strat. Env. 28, n/a-n/a. doi: 10.1002/bse.677
Platt, D., Workman, M., and Hall, S. (2018). A novel approach to assessing the commercial opportunities for greenhouse gas removal technology value chains: developing the case for a negative emissions credit in the UK. J. Clean. Prod. 203, 1003–1018. doi: 10.1016/j.jclepro.2018.08.291
Polzin, F. (2017). Mobilizing private finance for low-carbon innovation—A systematic review of barriers and solutions. Renew. Sust. Energy Rev. 77, 525–535. doi: 10.1016/j.rser.2017.04.007
Prado, V., Glaspie, R., Waymire, R., and Laurin, L. (2020). Energy apportionment approach to incentivize environmental improvement investments in the chemical industry. J. Clean. Prod. 257:120550. doi: 10.1016/j.jclepro.2020.120550
Rickels, W., Merk, C., Reith, F., Keller, D. P., and Oschlies, A. (2019). (Mis)conceptions about modeling of negative emissions technologies. Environ. Res. Lett. 14:104004. doi: 10.1088/1748-9326/ab3ab4
Rickels, W., Proelß, A., Geden, O., Burhenne, J., and Fridahl, M. (2020). The Future of (negative) Emissions Trading in the European Union. Kiel Working Paper, No. 2164. Kiel: Institute for the World Economy (IfW).
Rintamäki, T., and Kirves, K. (2017). From perceptions to propositions: profiling customer value across retail contexts. J. Retail. Consumer Serv. 37, 159–167. doi: 10.1016/j.jretconser.2016.07.016
Rodriguez, E., Lefvert, A., Fridahl, M., Grönkvist, S., Haikola, S., and Hansson, A. (2020). Tensions in the energy transition: Swedish and Finnish company perspectives on bioenergy with carbon capture and storage. J. Clean. Prod. 124527. doi: 10.1016/j.jclepro.2020.124527
Rogelj, J. D., Shindell, K., Jiang, S., Fifita, P., Forster, V., et al. (2018). “Mitigation pathways compatible with 1.5°c in the context of sustainable development,” in Global Warming of 1.5°C: An IPCC Special Report on the Impacts of Global Warming of 1.5°C Above Pre-Industrial Levels and Related Global Greenhouse Gas Emission Pathways, in the Context of Strengthening the Global Response to the Threat of Climate Change, Sustainable Development, and Efforts to eradicate poverty, eds. V. Masson-Delmotte, P. Zhai, H.-O. Pörtner, D. Roberts, J. Skea, P.R. Shukla, et al. (Geneva: Intergovernmental Panel on Climate Change), 3–24.
Rootzén, J., and Johnsson, F. (2017). “Technologies and policies for GHG emission reductions along the supply chains for the Swedish construction industry,” in Consumption, Efficiency & Limits, ed. T. Lindström (Stockholm: Eceee Secretariat), 1401–1407.
Rootzén, J., Kjärstad, J., Johnsson, F., and Karlsson, H. (2018). “Deployment of BECCS in Basic Industry: A Swedish Case Study.
Scherer, A. G., and Voegtlin, C. (2020). Corporate governance for responsible innovation: approaches to corporate governance and their implications for sustainable development. AMP 34, 182–208. doi: 10.5465/amp.2017.0175
Schneider, L., Healy, S., Fallasch, F., León, F., de Rambharos, M., Schallert, B., et al. (2020). What Makes a High-Quality Carbon Credit? (World Wildlife Fund (WWF-US), Environmental Defense Fund (EDF) and Oeko-Institut).
Science Based Targets Initiative (2020). Foundations for Science-Based Net-Zero Target Setting in the Corporate Sector.
Skagestad, R., Garðarsdóttir, S., Normann, F., Anheden, M., and Wolf, J. (2018). A case study of partial capture of CO2 from a pulp mill - the effect on CO2 capture cost. 14th Greenhouse Gas Control Technologies Conference Melbourne, (GHGT-14) 21–26. doi: 10.2139/ssrn.3366243
Skogsindustrierna (2019). Marknadsrapport: Så går det för skogsindustrin Februari 2019 (Stockholm: Skogsindustrierna).
Söderholm, P., Hellsmark, H., Frishammar, J., Hansson, J., Mossberg, J., and Sandström, A. (2019). Technological development for sustainability: the role of network management in the innovation policy mix. Technol. Forecast. Soc. Change 138, 309–323. doi: 10.1016/j.techfore.2018.10.010
Soneryd, L., and Uggla, Y. (2015). Green governmentality and responsibilization: new forms of governance and responses to ‘consumer responsibility'. Env. Polit 24, 913–931. doi: 10.1080/09644016.2015.1055885
Stora Enso (2019). Multicopy Zero. Avilable online at: https://www.storaenso.com/en/products/paper/office-papers/multicopy-zero (accessed May 27, 2019).
Suhr, M., Klein, G., Kourti, I., Gonzalo, M. R., Santonja, G. G., Roudier, S., et al. (2015). Best Available Techniques (BAT) Reference Document for the Production of Pulp, Paper and Board: Industrial Emissions Directive 2010/75/EU (Integrated Pollution Prevention and Control). Luxembourg: Publications Office of the European Union.
The Royal Society (2018). Greenhouse Gas Removal. London: The Royal Society; Royal Academy of Engineering.
The Swedish EPA (2018). Allmänheten om klimatet 2018: En kvantitativ undersökning om den svenska allmänhetens syn på lösningar för klimatet. Available online at: https://www.naturvardsverket.se/attityd-klimat-2018 (accessed February 26, 2019)
Tönjes, A., Lechtenböhmer, T., Witte, K., Knoop, K., and Mölter, H. (2020). “Dynamics of cross-industry low-carbon innovation in energy-intensive industries,” in ECEEE Industrial Summer Study proceedings: Industrial Efficiency 2020: Decarbonise industry! (Wuppertal), 467–476.
Torvanger, A. (2019). Governance of bioenergy with carbon capture and storage (BECCS): accounting, rewarding, and the Paris agreement. Climate Policy 19, 329–341. doi: 10.1080/14693062.2018.1509044
van der Spek, M., Roussanaly, S., and Rubin, E. S. (2019). Best practices and recent advances in CCS cost engineering and economic analysis. Int. J. Greenhouse Gas Control 83, 91–104. doi: 10.1016/j.ijggc.2019.02.006
van Vuuren, D. P., Stehfest, E., Gernaat, D. E. H. J., van den Berg, M., Bijl, D. L., Boer, H. S., et al. (2018). Alternative pathways to the 1.5 °C target reduce the need for negative emission technologies. Nat. Clim Change 8, 391–397. doi: 10.1038/s41558-018-0119-8
Voeth, M., and Herbst, U. (2006). Supply-chain pricing—A new perspective on pricing in industrial markets. Indust. Mark. Manag. 35, 83–90. doi: 10.1016/j.indmarman.2005.08.010
von Schomberg, R. (2012). “Prospects for technology assessment in a framework of responsible research and innovation,” in Technikfolgen abschätzen lehren: Bildungspotenziale transdisziplinärer Methoden, eds. M. Dusseldorp, and R. Beecroft (Wiesbaden: Springer VS), 39–61.
Walrave, B., Talmar, M., Podoynitsyna, K. S., Romme, A. G. L., and Verbong, G. P. J. (2018). A multi-level perspective on innovation ecosystems for path-breaking innovation. Technol. Forecast. Soc. Change 136, 103–113. doi: 10.1016/j.techfore.2017.04.011
Wesseling, J. H., Lechtenböhmer, S., Åhman, M., Nilsson, L. J., Worrell, E., and Coenen, L. (2017). The transition of energy intensive processing industries towards deep decarbonization: characteristics and implications for future research. Renew. Sust. Energy Rev. 79, 1303–1313. doi: 10.1016/j.rser.2017.05.156
Wiedmann, T., and Minx, J. C. (2008). “A Definition of ‘Carbon Footprint’,” in Ecological Economics Research Trends, ed. C. C. Pertsova (Hauppauge, NY: Nova Science Publishers Incorporated), 1–11.
Wikberg, E. (2018). Boken 2018:—marknaden, trender och analyser. Available online at:https://www.forlaggare.se/vara-rapporter (accessed November 18, 2019).
Zakkour, P., Kemper, J., and Dixon, T. (2014). Incentivising and accounting for negative emission technologies. Energy Procedia 63, 6824–6833. doi: 10.1016/j.egypro.2014.11.716
Keywords: negative emission technologies (NET), commercialisation, low carbon innovation, BECCS, corporate governance, value proposition, pulp and paper industry
Citation: Klement J, Rootzén J, Normann F and Johnsson F (2021) Supply Chain Driven Commercialisation of Bio Energy Carbon Capture and Storage. Front. Clim. 3:615578. doi: 10.3389/fclim.2021.615578
Received: 09 October 2020; Accepted: 26 January 2021;
Published: 24 February 2021.
Edited by:
Emily Margaret Cox, Cardiff University, United KingdomReviewed by:
Mathias Fridahl, Linköping University, SwedenJohn L. Field, Colorado State University, United States
Copyright © 2021 Klement, Rootzén, Normann and Johnsson. This is an open-access article distributed under the terms of the Creative Commons Attribution License (CC BY). The use, distribution or reproduction in other forums is permitted, provided the original author(s) and the copyright owner(s) are credited and that the original publication in this journal is cited, in accordance with accepted academic practice. No use, distribution or reproduction is permitted which does not comply with these terms.
*Correspondence: Filip Johnsson, filip.johnsson@chalmers.se