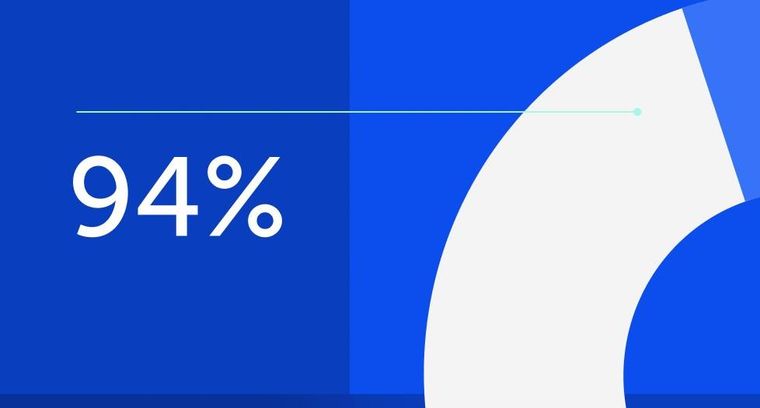
94% of researchers rate our articles as excellent or good
Learn more about the work of our research integrity team to safeguard the quality of each article we publish.
Find out more
ORIGINAL RESEARCH article
Front. Clim., 14 December 2020
Sec. Climate Services
Volume 2 - 2020 | https://doi.org/10.3389/fclim.2020.571836
This article is part of the Research TopicClimate Science Advances to Address 21st Century Weather and Climate ExtremesView all 6 articles
A heatwave that blanketed the northeast Pacific Ocean in 2013–2015 had severe impacts on the marine ecosystem through altered species composition and survival. A direct result of this marine heatwave was a sustained, record-setting harmful algal bloom (HAB), caused by the toxigenic diatom, Pseudo-nitzschia, that led to an unprecedented delay in harvest opportunity for commercial Dungeness crab (Metacarcinus magister) and closure of other recreational, commercial and tribal shellfish harvest, including razor clams. Samples collected during a cruise in summer 2015, showed the appearance of a highly toxic “hotspot” between Cape Mendocino, CA and Cape Blanco, OR that was observed again during cruises in the summers of 2016–2018. The transport of toxic cells from this retentive site northward during wind relaxations or reversals associated with storms resulted in economically debilitating delay or closure of Dungeness crab harvest in both northern California and Oregon in 2015–2019. Analyses of historic large-scale Pseudo-nitzschia HABs have shown that these events occur during warm periods such as El Niño, positive phases of the Pacific Decadal Oscillation, or the record-setting marine heatwave. In order to reduce the impacts of large-scale HABs along the west coast of North America, early warning systems have been developed to forewarn coastal managers. These early warning systems include the Pacific Northwest and California HAB Bulletins, both of which have documented elevated domoic acid and increased risk associated with the northern California hotspot. These early warnings enable mitigative actions such as selective opening of safe harvest zones, increased harvest limits during low risk periods, and early harvest in anticipation of impending HAB events. The aims of this study are to show trends in nearshore domoic acid along the US west coast in recent years, including the recent establishment of a new seed bed of highly-toxic Pseudo-nitzschia, and to explore how early warning systems are a useful tool to mitigate the human and environmental health and economic impacts associated with harmful algal blooms.
The effects of climate change on marine ecosystems will be long lasting. The impacts of climate change on marine biota, including the shifting range of marine species to higher latitudes, have decreased food security, and enhanced the decline of coral reefs by 70–90% at 1.5°C warming (IPCC, 2018). The International Panel on Climate Change report documented a high confidence of increased frequency and range of harmful algal blooms (HAB) in coastal areas since the 1980s, attributed partly to the effects of ocean warming and marine heatwaves (Collins et al., 2019). The probability of recent high-impact marine heatwaves has increased by more than 20-fold as a result of climate change (Laufkötter et al., 2020). The 2013–2015 marine heatwave that caused large-scale marine ecosystem impacts in the northeast Pacific was five times more likely to occur due to anthropogenic forcing over natural variability alone. This was determined through fraction of attributable risk analysis which compared observed trends and anomalies with model predictions (Weller et al., 2015; Di Lorenzo and Mantua, 2016) to assess the probability of extreme events occurring with and without anthropogenic influences (Stott et al., 2004). A direct result of this marine heatwave was a sustained, record-setting HAB, caused by the toxigenic diatom, Pseudo-nitzschia. The toxin, domoic acid (DA), produced by this diatom, accumulated in shellfish, resulting in an unprecedented delay in harvest opportunity for the commercial Dungeness crab (Metacarcinus magister) and closure of other recreational, commercial and tribal shellfish harvest (McCabe et al., 2016; McKibben et al., 2017).
Entanglements of mostly humpback whales (Megaptera novaeangliae), were an indirect result of the 2015 HAB event because the delay of the crab season opener resulted in higher numbers of crab pot lines than usual along the whale migration routes (NOAA Fisheries, 2018; Saez et al., 2020; Santora et al., 2020). A record number of illnesses and deaths of marine mammals (McCabe et al., 2016) and seabirds (Piatt et al., 2020) were caused both by food web transfer of HAB toxins (McCabe et al., 2016) and habitat compression into a geographically reduced coastal zone of food availability (Santora et al., 2020). An analysis of the historical record of large-scale Pseudo-nitzschia HABs has demonstrated that these events tend to occur during periods of anomalously warm ocean conditions such as El Niño, positive phases of the Pacific Decadal Oscillation (PDO), or the record-setting marine heatwave (McCabe et al., 2016; McKibben et al., 2017). Anomalous poleward currents together with fewer reversals (Sanford et al., 2019), facilitated the northward advection of a species of Pseudo-nitzschia australis, not usually found northward of California in the springtime (McCabe et al., 2016). Low nutrients were associated with the warm water mass that hosted the Pseudo-nitzschia population that subsequently bloomed when advected toward the coast into the nutrient-rich upwelled water (Trainer et al., 2020).
This observation of P. australis (McCabe et al., 2016) further north than usual in 2015, exemplified a range extension that included marine fish, mollusks, tunicates, bryozoans, cnidarians, crustaceans, and echinoderms (Sanford et al., 2019). In particular, northern California is a key transition zone between the milder temperate taxa found within the southern California Current and the cooler temperate species found further north, making this region an “ideal barometer” for observing such geographical range expansion in response to warming events (Sanford et al., 2019). Here we analyze annual trends in nearshore DA that were altered by the 2013–2015 marine heatwave by establishing a new seed bed of highly-toxic Pseudo-nitzschia, and explore how early warning systems are a useful tool to mitigate the human and environmental health and economic impacts associated with harmful algal blooms.
Whole seawater was collected from 3 to 5 m depth on research cruises by Niskin bottle or scientific seawater supply in 1998 (described in Trainer et al., 2000); Niskin bottle (NOAA Ship Bell M. Shimada, 2012; 2016; 2018) and via the scientific seawater system aboard the NOAA Ship Miller Freeman (2009) and NOAA Ship Bell M. Shimada (2015, described in McCabe et al. (2016); also 2017; 2019).
Analysis of satellite-derived sea surface temperature for the region of interest utilized the Multi-Scale Ultra-high Resolution Sea Surface Temperature (MURSST) product MUR-JPL-L4-GLOB-v4.1 (JPL MUR MEaSUREs Project, 2015). This is a global, gap-free, gridded, daily 1 km Sea Surface Temperature (SST) dataset created by merging multiple Level-2 satellite SST datasets. For this analysis monthly averages were created by averaging the months September-November 2002–2013 and 2014–2019, and the difference was calculated by subtracting the 2014–2019 average from the 2002–2013 average.
Particulate (cellular) DA was analyzed by filtering 1L seawater collected from surface waters onboard cruises using a bucket or Niskin bottle and analyzed using the [3H]kainic acid-based receptor binding assay for the 1998 cruise (Trainer et al., 2000), or the Biosense or PNW enzyme-linked immunosorbent assay for all other cruises (Eberhart et al., 2012).
DA in Dungeness crab (Metacarcinus magister) was measured using standard high-performance liquid chromatography (Wekell et al., 1994). These data were kindly provided by the OR Department of Agriculture and the California Department of Public Health.
Transition plans for the conversion of HAB Bulletins from research to operations have been developed for many regions of the US (https://coastalscience.noaa.gov/research/stressor-impacts-mitigation/hab-monitoring-system/). Some forecasts are fully operational (e.g., Lake Erie); others are in the process of transition. Successful transition will depend on collaborative approaches to ensure full operational support, including partnerships with the Integrated Ocean Observing System (Anderson et al., 2019), state monitoring organizations such as the Olympic Region Harmful Algal Bloom partnership and health departments (Trainer and Suddleson, 2005) as well as private companies (e.g., https://www.oceanaero.com/) to ensure the availability of advanced technologies to improve forecasts.
Toxigenic Pseudo-nitzschia blooms are known to initiate in highly retentive regions, designated as HAB “hotspots” along the west coast, including the Juan de Fuca eddy, Heceta Bank, Monterey Bay, and Point Conception (Trainer et al., 2001, 2012). However, the 2015 bloom was different. The warm water anomaly was found to be a source of Pseudo-nitzschia that were delivered to the coast after a series of spring storms, where these cells grew rapidly to bloom proportions when fueled by nutrients from upwelled waters close to shore (McCabe et al., 2016; Trainer et al., 2020). Among the locations that showed record levels of cellular (particulate) DA was a site near the California and Oregon border (McCabe et al., 2016). The emergence of this new toxin source region is also evident from both a compilation of historical observations collected from ships of opportunity and shore stations (Figure 1), as well as from the California Harmful Algae Risk Mapping (C-HARM) predictive statistical model (Anderson et al., 2016). Both the observations and the model identified the Humboldt (Trinidad Head) region as particularly toxic during 2015, with maximum total DA (particulate plus dissolved) exceeding 15,000 ng/L and DA in razor clams at a record level of 340 ppm (McClatchie et al., 2016). The C-HARM model continued to predict elevated probabilities of HABs in the region through 2018 (Wells et al., 2017; Thompson et al., 2018).
Figure 1. Sea surface temperature from the Multi-scale Ultra-High Resolution Sea Surface Temperature (MURSST) monthly product for September–November is shown for 2002–2013 (A) and 2014–2019 (B). The difference (B–A) is shown in panel (C). A large dataset of particulate domoic acid (pDA), collated from multiple programs along the US west coast, includes shore-based observations. Data from 2002–2013 (D) and 2014–2019 (E) were spatially interpolated using DIVA in OceanDataView. The northern California region of enhanced toxicity is prominent in the 2014–2019 dataset, and consistent with the expected optimal temperature for DA production (Ryan et al., 2017; Kudela et al., 2020).
The coastwide 2015 bloom appears to have “seeded” Pseudo-nitzschia cells to a site near Trinidad, California where high concentrations of DA were measured (Figure 2, red circled area). Cruises of opportunity prior to 2015, including summer cruises in 1998, 2009, and 2012, showed low concentrations of particulate DA near the California/Oregon border (Figure 2), suggesting that either lower abundances or less toxic species of Pseudo-nitzschia were found at this site prior to 2015. During a cruise from 7 to 10 August 1997 (data not shown), low concentrations of particulate DA, ranging from 200 to 500 ng/L, were measured at several stations between the Cape Mendocino and the CA/OR border. However, during the cruise in summer 2015 (McCabe et al., 2016), a more toxic DA “hotspot” was observed between Cape Mendocino, CA and Cape Blanco, OR that remained evident over 3–4 subsequent summers (Figures 2, 3). These data suggest that this site was freshly seeded with the more toxigenic species, P. australis, promoting higher DA toxicity in filter-feeding organisms in the region. In fact, Pseudo-nitzschia measured at this physically-retentive site have impacted Dungeness crab harvest in both CA and OR where frequent DA closures or harvest delays were first observed in 2015 and continued through early 2019 (Table 1), with Humboldt County (Figure 4) the last county in California to open for harvesting in 2015.
Figure 2. Domoic acid first appeared at the “hotspot” site between Cape Blanco (CB) in OR and Cape Mendocino (CM) in 2015 (red circle). Particulate domoic acid concentrations (ng/L; see legend inside the 1998 map) at stations sampled during summer cruises in 1998, 2008, 2012, 2015, 2016, 2017, 2018, 2019. The dates when stations in the red circled area that is centered around Trinidad, California, were sampled are shown on each panel.
Figure 3. Maximum DA concentrations in Dungeness crab viscera in northern California and southern, central and northern Oregon. The regulatory closure level of 30 ppm DA is shown as a horizontal line on each crab panel. Dotted vertical lines show first appearance of DA above 30 ppm in southern OR crab (which follows DA above 30 ppm in northern CA in all years except 2017). Numbers on the northern CA panel show values of crab DA exceeding the y-axis scale. The north-south component of low pass filtered winds (m s−1), filtered with a 15-day Hanning window, is shown from buoy 46027-6 (location shown in Figure 4). Linear regressions with other nearby wind sources were used to fill gaps in the wind time series. Map of regions used for calculation of maximum DA in Dungeness crab, also showing locations of Cape Mendocino (CM) and Cape Blanco (CB). Humboldt County, CA, is found in Figure 4.
Table 1. Oregon State harvest actions due to elevated domoic acid in Dungeness crab viscera (2015–2019).
Figure 4. Geographical regions of average domoic acid in Dungeness crab shown in Figure 3 and reference locations on land for crab closures shown in Table 1. Humboldt County, CA, is shown as a dotted line on land. The location of buoy 46027-6, from which wind data in Figure 3 were retrieved, is shown with an X.
Time series data from 2015, 2017, 2018 demonstrate that northern CA crab were first reported to be toxic (above the regulatory limit of 30 ppm DA), with southern and central OR crab becoming toxic about 1 week later (Figure 3). This is consistent with northward transport of toxigenic cells from the CA hotspot site during periods of relaxations or northward wind reversals associated with storm events near the time of the fall transition (Kosro, 1987; Send et al., 1987). A site of high DA between Cape Blanco and Cape Mendocino was not observed in summer 2019 (Figure 3). However, a single station with elevated particulate DA was noted just south of Cape Mendocino.
The northern CA region has been called an “ideal barometer” (Sanford et al., 2019) for documenting geographic range expansion and shifts in nearshore community composition. This region is an important transition zone where the poleward migration of marine species in response to warming events has been documented (Sagarin et al., 1999; Sanford et al., 2019). During 2014–2016, alongshore currents exhibited anomalously higher poleward flows which were estimated to have transported plankton over maximum distances of ~500 km in a month (Sanford et al., 2019). Plankton and marine larvae were transported northward over such large distances, with fewer reversals to facilitate their return southward (Sanford et al., 2019), allowing them to be established as new populations in more northern ecosystems. This diffusive transport was enhanced by retentive features or offshore mesoscale eddies especially after the spring transition when mean flow in the California Current System typically is equatorward. The intensified upwelling at coastal headlands (Davis, 1985; Kelly, 1985), including Cape Blanco (Barth et al., 2000) and Cape Mendocino (Largier et al., 1993) provides nutrients to fuel phytoplankton blooms, including HABs (Kudela et al., 2005). Areas on the lee side of coastal headlands are often associated with retentive eddies (Largier et al., 1993; Barth et al., 2000). To summarize, the anomalous poleward flows, such as those observed during 2014–2016, resulted in a higher probability of northward transport of particles (Sanford et al., 2019) such as phytoplankton, and their increased retention near coastal headlands. Indeed, P. australis, a highly toxic species typically observed only as far north in the springtime as central California, was detected in northern CA, OR and WA in the spring 2015 (McCabe et al., 2016). This provided an opportunity for this high DA-producing species to be “seeded” to the retentive site near the CA/OR border for future initiation of Pseudo-nitzschia blooms. During the 2015 event, P. australis emerged from a background of multiple Pseudo-nitzschia species, and dominated along almost the entire U.S. west coast, whereas during other years, less toxic species such as P. delicatissima have dominated, resulting in large blooms but with lower toxicity (Bowers et al., 2018; Smith et al., 2018).
Pseudo-nitzschia species are a major component of marine snow due to their ability to form long chains that can become intertwined while they sink to depths. This shower of organic material that cascades from surface waters to depth can transport DA to the benthos where it has been measured in benthic organisms, including bottom dwelling fish, worms, crustaceans (Vigilant and Silver, 2005, 2007; Kvitek et al., 2008) and Dungeness crab (Taylor, 1993). Toxigenic Pseudo-nitzschia and their inherent DA can sink to as deep as 800 m, providing a source of toxin to benthic filter feeders (Schnetzer et al., 2007; Sekula-Wood et al., 2009, 2011). In the Gulf of Mexico, ~50% of Pseudo-nitzschia cells present in the water column sank into sediment traps with ~10% as live cells (Dortch et al., 1997). Pseudo-nitzschia found in sediments were intact, contained pigment and maintained their chain formation (Sekula-Wood et al., 2009). The flux of Pseudo-nitzschia to sediments can be as high as 3.2 × 108 cells/m−2/day (Sekula-Wood et al., 2011). More than 80% of DA contained in a surface bloom is estimated to reach sediments >500 m deep (Schnetzer et al., 2017) and previous studies off OR have demonstrated that floc collected from the bottom boundary layer can seed a surface bloom of diatoms, including Pseudo-nitzschia, under simulated upwelling conditions (Wetz et al., 2004). Furthermore, sediment trap data suggests that once Pseudo-nitzschia are seeded into a retentive region, blooms will be frequent and increasing in intensity. A 15-year time series of sediment from the Santa Barbara Channel indicates that this region was seeded with Pseudo-nitzschia in the mid-1990s with increasingly toxic blooms occurring annually (Sekula-Wood et al., 2011). Therefore, the surficial sediment is a source of Pseudo-nitzschia and DA available to initiate the next bloom during upwelling conditions (Seegers et al., 2015).
Dungeness crab fishing generates the most income and has the highest vessel participation of all fisheries on the U.S. west coast (Fuller et al., 2017). The harvest yield of the Dungeness crab fishery and the reliability of the timely opening of crab season determines the livelihood of many fishers in rural communities (Ritzman et al., 2018). The Daily Astorian newspaper stated on 23 April 2018, that “HABs have complicated commercial Dungeness crab harvest on the OR coast for the past 3 seasons, threatening the viability of the state's most valuable fishery.” The commercial season in northern CA, OR, and WA typically opens December 1 and ends July 15. Unfortunately, after the delay of the crab opener until 15 January 2019 due to high levels of DA, the opportunity for CA crabbers was further limited by statewide fishery closure on April 15, almost 3 months earlier than normal, as stipulated under the terms of a settlement agreement reached between the California Department of Fish and Wildlife (CDFW) and the Centers for Biological Diversity (CBD) to limit whale entanglements in crab nets. The agreement requires CDFW to monitor areas off the central and northern CA coasts where whales feed along their spring migration route (Dillman, 2019). If more than 20 whales are observed in an area, fishery managers must order an immediate closure. Thus, the compression of the crab season by DA events, exacerbated by anomalous ocean warming, will have long-lasting impacts on the accessibility to this fishery and the resulting economic benefits to many rural communities. Small vessel fishers may trade their fishing effort disproportionately to access other fisheries when the crab season is canceled, causing concern for resource managers (Saez et al., 2020). Climate change and related warming have compressed habitats for many organisms (Santora et al., 2020). Both habitat compression and intensified Pseudo-nitzschia HABs with range expansion are a result of anomalously warm temperatures in the coastal ocean. In the future, the predicted increased intensity of these HABs (McCabe et al., 2016; McKibben et al., 2017) will reduce fishing opportunity directly due to the presence of toxins in seafood or indirectly through ecosystem impacts such as whale entanglement which may shorten the crab harvest season. Newly seeded HAB retentive sites, resulting from anthropogenic climate change, will challenge managers to ensure the safety of our seafood while having cascading impacts on the health of marine animals and economic welfare of coastal communities.
Early warning systems that anticipate HAB risk at the new hotspot site in northern CA as well as the other known hotspot sites on the US west coast include the Pacific Northwest (PNW) HAB Bulletin (Figure 5; http://depts.washington.edu/orhab/pnw-hab-bulletin/) and the California HAB Bulletin (https://sccoos.org/california-hab-bulletin/). Both Bulletins synthesize biological data from coastal and offshore monitoring of HABs and their toxins, a suite of physical environmental data, and output from realistic numerical models, including the LiveOcean model (https://faculty.washington.edu/pmacc/LO/LiveOcean.html) and the California Regional Ocean Modeling System (Anderson et al., 2019), to forecast potential HAB events for coastal managers. The PNW HAB Bulletin is provided to managers prior to razor clam and Dungeness crab openers and can be helpful in pinpointing areas of the coast that are high risk for HABs. When available, data collected from advanced technologies, such as the Environmental Sample Processor (ESP, Doucette et al., 2009; Scholin et al., 2009; Anderson et al., 2019) and autonomous ocean-going vessels (e.g., http://www.nanoos.org/news/index.php?item=Submaran180924) are also implemented in the forecasting Bulletins. While regionally specific information is utilized in each Bulletin, the PNW HAB Bulletin also tracks larger scale indices such as the Pacific Decadal Oscillation and El Niño, which have been shown to correlate with DA events throughout the PNW (McCabe et al., 2016; McKibben et al., 2017). In the case of the PNW HAB Bulletin, a summary narrative and a HAB risk assessment (low, medium, high; shown as “traffic light” symbols) are provided as a synthesis of results. The early warnings provided by the Bulletins enable mitigative actions such as selective opening of safe shellfish harvest zones, increased harvest limits during low risk periods, and early harvest in anticipation of upcoming toxin outbreaks. The timely and spatially-refined identification of toxin risk will allow managers to define areas of targeted closure, making these early warning Bulletins potentially more cost-effective than other mitigation methods (Holland and Leonard, 2020). In cases when predictions indicate an increasing risk for toxins, managers often modify workflows in order to collect additional shellfish samples (Dungeness crab, clams, and mussels) to ensure human and marine animal safety.
Figure 5. The PNW HAB Bulletin from 4 October 2018 (http://www.nanoos.org/products/habs/forecasts/bulletins/pnw_hab_bulletin-20181004.pdf (accessed 22 Nov 2020)) communicating elevated seawater particulate domoic acid off of Bandon, OR (492 ng/L), Brookings, OR (875 ng/L), and Humboldt, CA (>800 ng/L) in late September 2018. Pseudo-nitzschia australis was dominant across those sites at that time.
The new northern CA hotspot has been a repeated feature since the west coast wide 2015 DA event (McCabe et al., 2016), often leading to shellfish harvest closures in southern OR. The higher concentrations of DA in crab in southern OR compared to northern OR in late 2015, 2017, and 2018 (Figure 3), suggest that DA originated from northern CA (Figure 3, compare crab DA in CA North to OR South and OR North). By contrast, in late 2016, lower concentrations of DA in northern CA crab compared to OR crab suggest that toxigenic Pseudo-nitzschia originated from a northern source. Measurement of DA in razor clams provided additional insights regarding the geographical source of toxin. In fact, this northern source of DA in 2016 is supported by the cancellation of razor clam digs in southern WA (https://www.dailyastorian.com/news/local/washington-approves-razor-clam-digs-at-two-beaches-long-beach-still-closed/article_ad972fac-0cd4-5059-a845-fb2b25bb5818.html) and all of OR (DA in razor clams was above the regulatory level of 20 ppm in September and continued to increase, reaching a maximum of 360 ppm on the central OR coast in Dec 2016 [OR Department of Agriculture Food Safety data]; https://www.seattletimes.com/sports/entire-oregon-coast-closed-for-razor-clam-digging-after-marine-toxin-levels-skyrocket/ in the fall due to high DA.
The strong influence of the northern CA hotspot site on OR shellfish safety was documented in several PNW HAB Bulletins that prominently featured the fact that southern OR razor clams, mussels, and crabs all contained elevated DA during fall 2018 (e.g., Figure 3). In September 2018, razor clam DA concentrations as high as 180 ppm were measured at Gold Beach, OR, well above the 20 ppm regulatory limit for that species (in contrast to Dungeness crab which have a 30 ppm regulatory limit). Mussels collected off Humboldt, CA, were similarly over the regulatory limit at that same time, suggesting an ongoing toxin event spanning the northern CA/southern OR hotspot. As discussed in the 04-Oct-2018 PNW HAB Bulletin (Figure 5; http://www.nanoos.org/products/habs/forecasts/bulletins.php), scanning electron microscopy of Pseudo-nitzschia samples collected in late September, confirmed the presence of P. australis off both Bandon, OR, and Humboldt, CA. A resurgence of razor clam DA concentrations (from 39 to 140 ppm) at Gold Beach, OR, as well as elevated DA in crab viscera (40 ppm) in early May 2019 indicated the continued risk posed by this new hotspot, even though high particulate DA was not measured in this region in the few samples collected onboard the July 2019 cruise. However, DA poisoning was again a potential risk in October 2019 as seawater particulate DA was documented at an extremely high 5,005 ng/L at Brookings, OR (19-Oct-2019 PNW HAB Bulletin). Crab closures in CA were reported again in 2020 (CDPH report, 2020) demonstrating the persistence of this toxic feature.
PNW HAB Bulletins are emailed to fisheries managers and posted on the ORHAB and NANOOS websites (http://depts.washington.edu/orhab/pnw-hab-bulletin/ and http://www.nanoos.org/products/habs/forecasts/bulletins.php).
There are plans for HAB forecasts across the entire US west coast region to be coordinated through integration with regional Ocean Observing Systems (Anderson et al., 2019) and through the NOAA West Coast Operational Forecast System (WCOFS) effort, currently in development, which eventually will provide consistent ocean modeling across the entire west coast (https://tidesandcurrents.noaa.gov/ofs/dev/wcofs/wcofs_info.html). This dedicated, persistent, and comprehensive monitoring at key transition sites to quantify ocean changes will provide early warning of HABs, allowing management of important resources to promote the health of ocean ecosystems while assisting with safe harvest of seafood.
The raw data supporting the conclusions of this article will be made available by the authors, without undue reservation.
VT conceived of this article and wrote the draft based on input from all authors. RK created Figure 1. NA created Figures 2, 4. NA and RM created Figure 3. MH and NA created Table 1. All authors contributed to editing and improving the final manuscript.
This study was supported by a grant from the NOAA National Centers for Coastal Ocean Science's Monitoring and Event Response to Harmful Algal Blooms (MERHAB) program (NA16NOS4780189), Northwest Fisheries Science Center base funding, funding from the Central and Northern California Ocean Observing System (CeNCOOS; NA16NOS0120021) for toxin analysis from the Trinidad Head region, and by the Joint Institute for the Study of the Atmosphere and Ocean (JISAO) under NOAA Cooperative Agreement NA15OAR4320063. This is MERHAB contribution number 227, and JISAO contribution number 2020-1109.
The reviewer, EB, declared a past co-authorship with two of the authors, VT and RK, to the handling Editor.
The remaining authors declare that the research was conducted in the absence of any commercial or financial relationships that could be construed as a potential conflict of interest.
We thanked the captains and crews of the R/V New Horizon, NOAA Ship Miller Freeman, R/V Shana Rae, CA Fish and Wildlife Patrol Boat Bluefin, NOAA Ship Bell M. Shimada, and the following volunteers who have been invaluable in helping us over the years with HAB sampling: Tracie Barry, Monica Baze, Brian Bill, Julia Clemons, Madison Drescher, Naomi Estrada, Jennifer Hagen, Alexander Islas, Jessica Knoth, John Kim, Dan Lomax, Anthony Odell, O. Paul Olson, Aaron Parker, Lynne Scamman, Kathleen Schacht, Carla Stehr, Tom Werth. This project contributes to the implementation of the GlobalHAB Programme areas focusing on climate change, human health, and fisheries.
Anderson, C. R., Berdalet, E., Kudela, R. M., Cusack, C. K., Silke, J., O'Rourke, E., et al. (2019). Scaling up from regional case studies to a global harmful algal bloom observing system. Front. Marine Sci. 6:250. doi: 10.3389/fmars.2019.00250
Anderson, C. R., Kudela, R. M., Kahru, M., Chao, Y., Rosenfeld, L. K., Bahr, F. L., et al. (2016). Initial skill assessment of the California Harmful Algae Risk Mapping (C-HARM) system. Harmful Algae 59, 1–18. doi: 10.1016/j.hal.2016.08.006
Barth, J. A., Pierce, S. D., and Smith, R. L. (2000). A separating coastal upwelling jet at Cape Blanco, Oregon and its connection to the California Current System. Deep Sea Res. Pt. II 47, 783–810. doi: 10.1016/S0967-0645(99)00127-7
Bowers, H. A., Ryan, J. P., Hayashi, K., Woods, A. L., Marin, R., Smith, G. J., et al. (2018). Diversity and toxicity of Pseudo-nitzschia species in Monterey Bay: perspectives from targeted and adaptive sampling. Harmful Algae 78, 129–141. doi: 10.1016/j.hal.2018.08.006
Collins, M., Sutherland, M., Bouwer, L., Cheong, S.-M., Frölicher, T., Combes, H., et al. (2019). “Extremes, abrupt changes and managing risk,” in IPCC Special Report on the Ocean and Cryosphere in a Changing Climate, eds H.-O. Pörtner, D.C. Roberts, V. Masson-Delmotte, P. Zhai, M. Tignor, E. Poloczanska, et al. (in press). Available online at: https://www.ipcc.ch/srocc/chapter/chapter-6/ (accessed November 29, 2019).
Davis, R. E. (1985). Drifter observations of coastal surface currents during CODE-The statistical and dynamical views. J. Geophys. Res. Oceans 90, 4756–4772. doi: 10.1029/JC090iC03p04756
Di Lorenzo, E., and Mantua, N. (2016). Multi-year persistence of the 2014/15 North Pacific marine heatwave. Nat. Climate Change 6, 1042–1047. doi: 10.1038/nclimate3082
Dortch, Q., Robichaux, R., Pool, S., Milsted, D., Mire, G., Rabalais, N. N., et al. (1997). Abundance and vertical flux of Pseudo-nitzschia in the northern Gulf of Mexico. Mar. Ecol. Prog. Ser. 146, 249–264. doi: 10.3354/meps146249
Doucette, G. J., Mikulski, C. M., Jones, K. L., King, K. L., Greenfield, D. I., Marin, R., et al. (2009). Remote, subsurface detection of the algal toxin domoic acid onboard the Environmental Sample Processor: assay development and field trials. Harmful Algae 8, 880–888. doi: 10.1016/j.hal.2009.04.006
Eberhart, B. T. L., Bill, B. D., and Trainer, V. L. (2012). Remote sampling of harmful algal blooms: a case study on the Washington State coast. Harmful Algae 19, 39–45. doi: 10.1016/j.hal.2012.05.005
Fuller, E. C., Samhouri, J. F., Stoll, J. S., Levin, S. A., and Watson, J. R. (2017). Characterizing fisheries connectivity in marine social-ecological systems. ICES J. Mar. Sci. 74, 2087–2096. doi: 10.1093/icesjms/fsx128
Holland, D. S., and Leonard, J. (2020). Is a delay a disaster? Evaluation of the economic impacts of the delay of the California Dungeness crab fishery due to a harmful algal bloom. Harmful Algae 98:101904. doi: 10.1016/j.hal.2020.101904
IPCC (2018). Global Warming of 1.5°C. An IPCC Special Report on the impacts of global warming of 1.5°C above pre-industrial levels and related global greenhouse gas emission pathways, in the context of strengthening the global response to the threat of climate change, sustainable development, and efforts to eradicate poverty, eds. V. Masson-Delmotte, P. Zhai, H.-O. Pörtner, D. Roberts, J. Skea, P.R. Shukla, et al. (in press). Available online at: https://www.ipcc.ch/sr15/ (accessed November 22, 2020).
JPL MUR MEaSUREs Project (2015). GHRSST Level 4 MUR Global Foundation Sea Surface Temperature Analysis (v4.1). NASA PO.DAAC. Available online at: http://podaac.jpl.nasa.gov/dataset/MUR-JPL-L4-GLOB-v4.1 (accessed October 29, 2019).
Kelly, K. A. (1985). The influence of winds and topography on the sea-surface temperature patterns over the northern California slope. J. Geophys. Res. Oceans 90, 11783–11798. doi: 10.1029/JC090iC06p11783
Kosro, P. M. (1987). Structure of the coastal current field off northern California during the Coastal Ocean Dynamics Experiment. J. Geophys. Res. Oceans 92, 1637–1654. doi: 10.1029/JC092iC02p01637
Kudela, R., Pitcher, G., Probyn, T., Figueiras, F., Moita, T., and Trainer, V. L. (2005). Harmful algal blooms in coastal upwelling systems. Oceanography 18, 184–197. doi: 10.5670/oceanog.2005.53
Kudela, R. M., Hayashi, K., and Caceres, C. G. (2020). Is San Francisco Bay resistant to Pseudo-nitzschia and domoic acid? Harmful Algae 92:101617. doi: 10.1016/j.hal.2019.05.010
Kvitek, R. G., Goldberg, J. D., Smith, G. J., Doucette, G. J., and Silver, M. W. (2008). Domoic acid contamination within eight representative species from the benthic food web of Monterey Bay, California, USA. Mar. Ecol. Prog. Ser. 367, 35–47. doi: 10.3354/meps07569
Largier, J. L., Magnell, B. A., and Winant, C. D. (1993). Subtidal circulation over the northern California shelf. J. Geophys. Res. Oceans 98, 18147–18179. doi: 10.1029/93JC01074
Laufkötter, C., Zscheischler, J., and Frolicher, T. L. (2020). High-impact marine heatwaves attributable to human-induced global warming. Science 369, 1621–1625. doi: 10.1126/science.aba0690
McCabe, R. M., Hickey, B. M., Kudela, R. M., Lefebvre, K. A., Adams, N. G., Bill, B. D., et al. (2016). An unprecedented coastwide toxic algal bloom linked to anomalous ocean conditions. Geophys. Res. Lett. 43, 10366–10376. doi: 10.1002/2016GL070023
McClatchie, S., Goericke, R., Leising, A., Auth, T. D., Bjorkstedt, E., Robertson, R. R., et al. (2016). State of the California current 2015-16: comparisons with the 1997-98 El Niño. CalCOFI Reports 57, 1–61. Available online at: http://calcofi.org/publications/calcofireports/v57/Vol57-SOTCC_pages.5-61.pdf (accessed November 22, 2020).
McKibben, S. M., Peterson, W., Wood, A. M., Trainer, V. L., Hunter, M., and White, A. E. (2017). Climatic regulation of the neurotoxin domoic acid. Proc. Natl. Acad. Sci. U.S.A. 114, 239–244. doi: 10.1073/pnas.1606798114
NOAA Fisheries (2018). 2017 National Report on Large Whale Entanglements. Available online at: https://www.fisheries.noaa.gov/resource/document/national-report-large-whale-entanglements-2017 (accessed November 22, 2020).
Piatt, J. F., Parrish, J. K., Renner, H. M., Schoen, S. K., Jones, T. T., Arimitsu, M. L., et al. (2020). Extreme mortality and reproductive failure of common murres resulting from the northeast Pacific marine heatwave of 2014-2016. PLoS ONE 15:e0226087. doi: 10.1371/journal.pone.0226087
Ritzman, J., Brodbeck, A., Brostrom, S., McGrew, S., Dreyer, S., Klinger, T., et al. (2018). Economic and sociocultural impacts of fisheries closures in two fishing-dependent communities following the massive 2015 US West Coast harmful algal bloom. Harmful Algae 80, 35–45. doi: 10.1016/j.hal.2018.09.002
Ryan, J. P., Kudela, R. M., Birch, J. M., Blum, M., Bowers, H. A., Chavez, F. P., et al. (2017). Causality of an extreme harmful algal bloom in Monterey Bay, California, during the 2014–2016 northeast Pacific warm anomaly. Geophys. Res. Lett. 44, 5571–5579. doi: 10.1002/2017GL072637
Saez, L., Lawson, D., and DeAngelis, M. (2020). Large Whale Entanglements off the U.S. West Coast, from 1982-2017. NOAA Tech. Memo. NMFS-OPR-63, 48. Available online at: https://www.fisheries.noaa.gov/resource/document/large-whale-entanglements-us-west-coast-1982-2017 (accessed November 22, 2020).
Sagarin, R. D., Barry, J. P., Gilman, S. E., and Baxter, C. H. (1999). Climate-related change in an intertidal community over short and long time scales. Ecol. Monogr. 69, 465–490. doi: 10.1890/0012-9615(1999)069[0465:CRCIAI]2.0.CO;2
Sanford, E., Sones, J. L., García-Reyes, M., Goddard, J. H. R., and Largier, J. L. (2019). Widespread shifts in the coastal biota of northern California during the 2014–2016 marine heatwaves. Sci. Rep. 9:4216. doi: 10.1038/s41598-019-40784-3
Santora, J. A., Mantua, N. J., Schroeder, I. D., Field, J. C., Hazen, E. L., Bograd, S. J., et al. (2020). Habitat compression and ecosystem shifts as potential links between marine heatwave and record whale entanglements. Nat. Commun. 11:536. doi: 10.1038/s41467-019-14215-w
Schnetzer, A., Lampe, R. H., Benitez-Nelson, C. R., Marchetti, A., Osburn, C. L., and Tatters, A. O. (2017). Marine snow formation by the toxin-producing diatom, Pseudo-nitzschia australis. Harmful Algae 61, 23–30. doi: 10.1016/j.hal.2016.11.008
Schnetzer, A., Miller, P. E., Schaffner, R. A., Stauffer, B. A., Jones, B. H., Weisberg, S. B., et al. (2007). Blooms of Pseudo-nitzschia and domoic acid in the San Pedro Channel and Los Angeles harbor areas of the Southern California Bight, 2003-2004. Harmful Algae 6, 372–387. doi: 10.1016/j.hal.2006.11.004
Scholin, C., Doucette, G., Jensen, S., Roman, B., Pargett, D., Marin, R. I., et al. (2009). Remote detection of marine microbes, small invertebrates, harmful algae, and biotoxins using the Environmental Sample Processor (ESP). Oceanography 22, 158–167. doi: 10.5670/oceanog.2009.46
Seegers, B. N., Birch, J. M., Marin, R., Scholin, C. A., Caron, D. A., Seubert, E. L., et al. (2015). Subsurface seeding of surface harmful algal blooms observed through the integration of autonomous gliders, moored environmental sample processors, and satellite remote sensing in southern California. Limnol. Oceanogr. 60, 754–764. doi: 10.1002/lno.10082
Sekula-Wood, E., Benitez-Nelson, C., Morton, S., Anderson, C., Burrell, C., and Thunell, R. (2011). Pseudo-nitzschia and domoic acid fluxes in Santa Barbara Basin (CA) from 1993 to 2008. Harmful Algae 10, 567–575. doi: 10.1016/j.hal.2011.04.009
Sekula-Wood, E., Schnetzer, A., Benitez-Nelson, C. R., Anderson, C., Berelson, W. M., Brzezinski, M. A., et al. (2009). Rapid downward transport of the neurotoxin domoic acid in coastal waters. Nature Geosci. 2, 272–275. doi: 10.1038/ngeo472
Send, U., Beardsley, R. C., and Winant, C. D. (1987). Relaxation from upwelling in the Coastal Ocean Dynamics experiment. J. Geophys. Res. Oceans 92, 1683–1698. doi: 10.1029/JC092iC02p01683
Smith, J., Connell, P., Evans, R. H., Gellene, A. G., Howard, M. D. A., Jones, B. H., et al. (2018). A decade and a half of Pseudo-nitzschia spp. and domoic acid along the coast of southern California. Harmful Algae 79, 87–104. doi: 10.1016/j.hal.2018.07.007
Stott, P. A., Stone, D. A., and Allen, M. R. (2004). Human contribution to the European heatwave of 2003. Nature 432, 610–614. doi: 10.1038/nature03089
Taylor, F. J. R. (1993). British Columbia: implications of the North American west coast experience. Harmful Algae News 6, 2–3.
Thompson, A. R., Schroeder, I. D., Bograd, S. J., Hazen, E. L., Jacox, M. G., Leising, A., et al. (2018). State of the California Current 2017-18: still not quite normal in the north and getting interesting in the south. CalCOFI Reports 59, 1–66. Available online at: https://calcofi.org/publications/calcofireports/v59/Vol59-SOTC2018_1-66.pdf (accessed November 22, 2020).
Trainer, V. L., Adams, N. G., Bill, B. D., Stehr, C. M., Wekell, J. C., Moeller, P., et al. (2000). Domoic acid production near California coastal upwelling zones, June 1998. Limnol. Oceanogr. 45, 1818–1833. doi: 10.4319/lo.2000.45.8.1818
Trainer, V. L., Adams, N. G., and Wekell, J. C. (2001). “Domoic acid-producing Pseudo-nitzschia species off the US west coast associated with toxification events.” in Harmful Algal Blooms 2000, eds G. M. Hallegraeff, S. I. Blackburn, C. J. Bolch and R. J. Lewis (Paris: UNESCO), 46–49.
Trainer, V. L., Bates, S. S., Lundholm, N., Thessen, A. E., Cochlan, W. P., Adams, N. G., et al. (2012). Pseudo-nitzschia physiological ecology, phylogeny, toxicity, monitoring and impacts on ecosystem health. Harmful Algae 14, 271–300. doi: 10.1016/j.hal.2011.10.025
Trainer, V. L., Moore, S. K., Hallegraeff, G., Kudela, R. M., Clement, A., Mardones, J. I., et al. (2020). Pelagic harmful algal blooms and climate change: lessons from nature's experiments with extremes. Harmful Algae 91:101591. doi: 10.1016/j.hal.2019.03.009
Trainer, V. L., and Suddleson, M. (2005). Approaches for early warning of domoic acid events in Washington state. Oceanography 18, 228–237. doi: 10.5670/oceanog.2005.56
Vigilant, V., and Silver, M. W. (2005). “Domoic acid in the fat innkeeper worm, Urechis caupo, at Elkhorn Slough, CA,” in 3rd Symposium for Harmful Algae in the US (Monterey, CA), 152. Available online at: https://www.whoi.edu/cms/files/Abstracts_-_3rd_Symposium_24225.pdf (accessed November 22, 2019).
Vigilant, V. L., and Silver, M. W. (2007). Domoic acid in benthic flatfish on the continental shelf of Monterey Bay, California, USA. Mar. Biol. 151, 2053–2062. doi: 10.1007/s00227-007-0634-z
Wekell, J. C., Gauglitz, E. J., Barnett, H. J., Hatfield, C. L., and Eklund, M. (1994). The occurrence of domoic acid in razor clams (Siliqua patula), dungeness crab (Cancer magister), and anchovies (Engraulis mordax). J. Shellfish Res. 13, 587–593.
Weller, E., Min, S. K., Lee, D., Cai, W. J., Yeh, S. W., and Kug, J. S. (2015). Human contribution to the 2014 record high sea surface temperatures over the Western Tropical and Northeast Pacific Ocean. Bull. Amer. Meteor. Soc. 96, 100–104. doi: 10.1175/BAMS-D-15-00055.1
Wells, B. K., Schroeder, I. D., Bograd, S. J., Hazen, E. L., Jacox, M. G., Leising, A., et al. (2017). State of the California current 2016-17: still anything but “normal” in the north. CalCOFI Reports 58, 1–55. Available online at: http://calcofi.org/publications/calcofireports/v58/Vol58-State_of_the_Current_pages_1-55.pdf (accessed November 22, 2020).
Keywords: climate extremes, weather extremes, climate change, climate services, early warning, harmful algal blooms, domoic acid
Citation: Trainer VL, Kudela RM, Hunter MV, Adams NG and McCabe RM (2020) Climate Extreme Seeds a New Domoic Acid Hotspot on the US West Coast. Front. Clim. 2:571836. doi: 10.3389/fclim.2020.571836
Received: 12 June 2020; Accepted: 13 November 2020;
Published: 14 December 2020.
Edited by:
Chris C. Funk, United States Geological Survey (USGS), United StatesReviewed by:
Ladislaus Benedict Chang'A, Tanzania Meteorological Agency, TanzaniaCopyright © 2020 Trainer, Kudela, Hunter, Adams and McCabe. This is an open-access article distributed under the terms of the Creative Commons Attribution License (CC BY). The use, distribution or reproduction in other forums is permitted, provided the original author(s) and the copyright owner(s) are credited and that the original publication in this journal is cited, in accordance with accepted academic practice. No use, distribution or reproduction is permitted which does not comply with these terms.
*Correspondence: Vera L. Trainer, dmVyYS5sLnRyYWluZXJAbm9hYS5nb3Y=
Disclaimer: All claims expressed in this article are solely those of the authors and do not necessarily represent those of their affiliated organizations, or those of the publisher, the editors and the reviewers. Any product that may be evaluated in this article or claim that may be made by its manufacturer is not guaranteed or endorsed by the publisher.
Research integrity at Frontiers
Learn more about the work of our research integrity team to safeguard the quality of each article we publish.