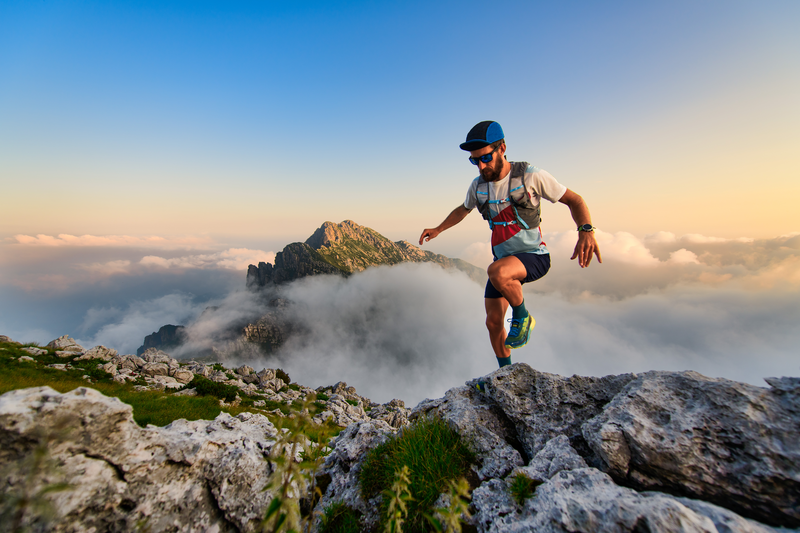
95% of researchers rate our articles as excellent or good
Learn more about the work of our research integrity team to safeguard the quality of each article we publish.
Find out more
ORIGINAL RESEARCH article
Front. Cell. Infect. Microbiol. , 20 February 2025
Sec. Veterinary and Zoonotic Infection
Volume 15 - 2025 | https://doi.org/10.3389/fcimb.2025.1544775
Ovine babesiosis, caused by Babesia ovis, is a significant tick-borne disease affecting sheep globally, with severe economic implications for sheep farming, particularly in Türkiye. Babesia ovis is transmitted exclusively by adult Rhipicephalus bursa ticks, but the potential role of infected larval stages in modulating disease severity has remained unclear. This study investigated whether infestation with B. ovis-infected R. bursa larvae reduces the severity of babesiosis following subsequent exposure to infected adult ticks. Three experimental sheep were infested with B. ovis-infected larvae, while three control sheep were infested with Babesia-free larvae. Both groups were subsequently exposed to B. ovis-infected adult R. bursa. Daily clinical, molecular, and serological monitoring revealed no clinical signs of babesiosis or B. ovis infection following larval infestation. However, all sheep developed severe clinical babesiosis after exposure to infected adult ticks. No significant differences in disease severity, parasitemia levels, or clinical outcomes were observed between the experimental and control groups, indicating that larval infestation does not confer protection or lead to milder disease courses. These findings confirm the exclusive role of adult R. bursa in B. ovis transmission and emphasize the critical need for vector control strategies targeting adult tick populations during peak activity. This study highlights the importance of understanding stage-specific transmission barriers and their implications for vector-borne disease management. Future research should explore the molecular mechanisms limiting pathogen transmission by immature ticks and investigate comparative transmission dynamics across Babesia species to inform targeted control interventions.
Ovine babesiosis, caused by several Babesia species, is a significant tick-borne disease that affects sheep. The primary Babesia species known to infect sheep include Babesia ovis, B. motasi, B. crassa, B. taylori, B. foliate, Babesia sp. Xinjiang, B. motasi Lintanensis, and B. motasi Hebeinensis (Ceylan et al., 2021; Schnittger et al., 2022). Despite the presence of around eight species, B. ovis is recognized as the most pathogenic and potentially lethal species for sheep (Ceylan et al., 2021; Firat et al., 2024). It has been reported in several countries, including Türkiye (Ceylan and Sevinc, 2020; Ulucesme et al., 2023; Bozan et al., 2024), Pakistan (Iqbal et al., 2011), Iran (Gh et al., 2020), Spain (Ferrer et al., 1998), Portugal (Horta et al., 2014), Egypt (Hussein et al., 2017), Palestine (Azmi et al., 2016), Israel (Pipano, 2022), Greece (Theodoropoulos et al., 2006), Italy (Savini et al., 1999), Nigeria (Adewumi et al., 2022), Uganda (Tumwebaze et al., 2020), Algeria (Aouadi et al., 2017), and Tunisia (Rjeibi et al., 2016). However, in many of these regions, little is known about the mortality rates or economic impact of the disease on sheep farming. As a result, ovine babesiosis is often classified as a neglected disease, particularly when compared to bovine babesiosis (Ozubek et al., 2020).
In Türkiye, B. ovis is a significant concern in sheep farming, where the disease can result in mortality rates as high as 50% (Aktas et al., 2007; Ceylan et al., 2021). The economic implications of this disease are substantial, including costs related to medication and losses from sheep mortalities (Sevinc et al., 2007; Sevinç and Xuenan, 2015). Babesia ovis is transmitted by the two-host tick Rhipicephalus bursa, with the adult stage of the tick serving as the primary vector for B. ovis transmission (Altay et al., 2008; Erster et al., 2016; Antunes et al., 2018). The larvae of R. bursa remain inactive during the hot, dry summer months and begin to climb plants in early October, peaking in activity by December and subsiding by February. Larvae and nymphs enter a state of diapause during summer and winter, respectively, and adult ticks become active once temperatures exceed 18°C during the day and 12°C at night. There is approximately a six-month interval between the host-seeking activities of immature and mature ticks (Yeruham, 1995; Yeruham et al., 1998, 2000). This natural temporal separation between immature and adult ticks is relevant for understanding the transmission dynamics of B. ovis, particularly in assessing whether infestation with B. ovis-infected larvae has any impact on the severity of subsequent infections caused by adult ticks.
While R. bursa larvae and nymphs can be infected with B. ovis, these immature stages are incapable of transmitting the pathogen. Transmission occurs exclusively during the adult stage of the tick (Erster et al., 2016; Firat et al., 2024). Despite this, some studies suggest that infestation with B. ovis-infected larvae may have an immunizing effect, leading to milder infections when adult ticks transmit the pathogen later in life (Yeruham, 1995; Yeruham et al., 1998; Erster et al., 2016). However, the clinical and immunological implications of this interaction remain poorly understood, particularly regarding whether prior exposure to infected larvae influences the severity or resilience of sheep during later infections.
This study aimed to investigate whether infestation with B. ovis-infected R. bursa larvae alters the clinical course of babesiosis in sheep, contributing to the broader understanding of ovine babesiosis. By focusing on this aspect, our research goes beyond merely demonstrating the inability of larvae to directly transmit the pathogen, instead exploring their potential indirect effects on host-pathogen dynamics. Understanding these interactions is critical for improving control strategies, especially in endemic regions like Türkiye, where the economic impact of babesiosis on sheep farming is significant. Our findings aim to provide novel insights into the clinical outcomes of larval feeding, addressing critical gaps in the global understanding of vector-host interactions in babesiosis.
This study was carried out according to the regulations of animal and welfare issued by the Turkish legislation for the protection of animals. All animal experiments were approved by the Firat University, Animal Experiment Ethic Committee, protocol number 2023/12-05. To minimize stress during the acclimatization period, all sheep were housed individually in a quiet and temperature-controlled environment, with daily health checks performed by trained personnel.
Seven clinically healthy Akkaraman sheep, aged between 5 and 8 months, were included in the study. These animals were selected after a rigorous screening process to confirm their freedom from infections with Babesia, Theileria, and Anaplasma species. Molecular analyses were performed using nested PCR (nPCR), targeting conserved genomic regions specific to these pathogens. Primer pairs used included Ec9/Ec12A (Kawahara et al., 2006) and 16S8FE/B-GA1B (Bekker et al., 2002) for Anaplasma spp., Nbab1F/Nbab1R (Oosthuizen et al., 2008) and RLBF2/RLBR2 (Georges et al., 2001) for Babesia/Theileria spp (Supplementary Table 1). Blood samples testing negative in these assays were further analyzed for the presence of antibodies against B. ovis using an in-house developed indirect ELISA (iELISA) targeting the recombinant B. ovis Secreted Antigen-1 (rBoSA1), as described by Sevinc et al. (2015). The rBoSA1 protein was expressed and purified using recombinant DNA technology in Escherichia coli. For the assay, 96-well ELISA microplates were coated with 50 µl of rBoSA1 at a concentration of 2 μg/ml, prepared in carbonate-bicarbonate buffer (pH=9.6). After an overnight incubation at 4°C, the plates were washed and blocked to prevent non-specific binding. Sheep sera were diluted at 1:100 in blocking buffer and incubated in the coated wells, followed by the addition of horseradish peroxidase-conjugated secondary antibodies (peroxidase-conjugated anti-sheep IgG; Sigma-Aldrich). The reaction was developed using a chromogenic substrate, and optical densities (OD) were measured at 450 nm. The cutoff value was calculated as the mean OD of negative control sera plus three times the standard deviation, ensuring accurate differentiation between positive and negative samples (Sevinc et al., 2015; Firat et al., 2024). Due to the lack of commercially available ELISA kits for ovine Theileria and Anaplasma species, no serological testing was performed for these pathogens. Based on the molecular and serological analyses, seven sheep were confirmed to be free of Babesia, Theileria, and Anaplasma infections. These animals were then transported to the Elazig Veterinary Control Institute Directorate, where they were housed individually in tick-free and biosecure compartments. The sheep were provided with ad libitum access to food and water throughout the study period. To ensure acclimatization and minimize stress, the animals were allowed to rest for seven days before the experiments began (Figure 1).
Figure 1. Selection of sheep for experimental infection study. All sheep were tested for Babesia spp., Theileria spp., and Anaplasma spp. using nPCR and screened for B. ovis using iELISA. Only those negative in both tests were included in the study. Figure was created using Biorender.com.
The sheep were assigned to two experimental groups. One sheep (#242) was designated for the production of B. ovis-infected R. bursa larvae, while the remaining six were randomly divided into an experimental group (#001, #357, #663) and a control group (#576, #639, #987). The sample size, consisting of three animals per group, was determined in accordance with ethical guidelines, emphasizing the principle of using the minimum number of animals necessary to achieve valid scientific results. This approach aligns with previous experimental infection studies involving Babesia species, where three animals per group have been widely adopted as standard practice (Alzan et al., 2021; Bastos et al., 2022; Silva et al., 2023; Firat et al., 2024).
To produce B. ovis-infected R. bursa larvae, the designated sheep (#242) underwent splenectomy. Splenectomy is a widely adopted method in Babesia infection studies as it suppresses the host’s immune clearance mechanisms, thereby facilitating higher parasitemia and improving the efficiency of vector infection (Sevinc et al., 2007). Following the surgical operation, the splenectomized sheep was intravenously inoculated with 15 mL of cryopreserved B. ovis Alacakaya stabilate containing 5% parasitized erythrocytes (PPE). This stabilate, derived from a naturally infected sheep, had been purified through transovarial passage in R. bursa ticks to eliminate co-infections. It was cryopreserved with 10% dimethyl sulfoxide (DMSO) as a cryoprotectant and stored in liquid nitrogen at -196°C until use.
On the first day post-inoculation (DPI 1), 25 adult R. bursa ticks (10 females and 15 males), reared in a laboratory colony (Firat et al., 2024; Ulucesme et al., 2024), were attached to the splenectomized sheep using EVA foam feeding capsules (Almazán et al., 2018). These capsules, affixed to the shaved thoracic region of the sheep, allowed for controlled tick feeding. Parasitemia in the splenectomized sheep was monitored daily, and engorged female ticks were collected on days 9 and 10 post-infection (DPI 9 and 10). The collected ticks were incubated under controlled conditions (27°C and 85% relative humidity) to produce larvae. The larvae (500 larvae from each female tick) were tested using nPCR to confirm B. ovis infection, and only those confirmed to be positive were used for subsequent infestations (Figure 2).
Figure 2. Experimental timeline for obtaining B. ovis-infected R. bursa larvae. Sheep #242 was inoculated with the B. ovis-Alacakaya stabilate on day 0. Unfed adult ticks (10 ♂ and 15 ♀) from a Babesia-free R. bursa (F2 generation) were placed on the sheep on DPI 1. The timeline shows daily post-infection days (DPI) up to day 10, highlighting days 5 to 10 where parasitemia was observed. Engorged females were collected on DPI 9 and 10 and placed in an incubator for breeding to produce B. ovis-infected (F3 generation) larvae. Figure was created using Biorender.com.
At the beginning of the experiment, sheep in the experimental group (#001, #357, #663) were infested with 0.1 grams of R. bursa larvae infected with B. ovis, while those in the control group (#576, #639, #987) were infested with the same quantity of Babesia-free R. bursa larvae (Firat et al., 2024; Ulucesme et al., 2024). This initial infestation ensured uniform exposure across both groups, enabling the evaluation of the effects of B. ovis-infected larvae compared to uninfected larvae. Following infestation, the larvae were monitored daily, and engorged nymphs were collected from each sheep and placed in separate plastic containers for incubation. Between days 20 and 24 of the experiment, these nymphs were incubated under controlled conditions at a temperature of 27°C and 85% relative humidity. This incubation facilitated the successful molting of the nymphs into adult ticks, which were subsequently used in the next phase of the study.
To confirm the presence of B. ovis, 30 adult ticks (15 females, 15 males) were collected from each sheep in the experimental group (#001, #357, #663). Ticks were organized into 10 pools per sheep, comprising 5 pools of females (3 female ticks per pool) and 5 pools of males (3 male ticks per pool). Each pool was analyzed individually by nPCR to verify infection status (Aktaş et al., 2005), and only positive pools were used for subsequent infestations (Figure 3).
Figure 3. Experimental design for assessing the impact of B. ovis-infected R. bursa larvae on babesiosis severity in sheep. The experimental group (sheep #001, #357, #663) was infested with 0.1 g of B. ovis-infected R. bursa larvae on Day 0, while the control group (sheep #575, #639, #987) received an equivalent weight of Babesia-free R. bursa larvae. On Day 20-24, engorged nymphs were collected from both groups. Subsequently, On day 52, both groups were challenged with B. ovis-infected adult R. bursa ticks (50♂ and 50♀) to evaluate the clinical course of babesiosis. Figure was created using Biorender.com.
The sheep were monitored daily for clinical signs of babesiosis, including fever, anemia, jaundice, and hemoglobinuria. Fever was measured using a digital rectal thermometer, while anemia was assessed by analyzing hematocrit (HCT) level using an automated hematology analyzer (Mindray BC-5000 Vet; Bio-Medical Electronics Co. Ltd., Shenzhen, China). Jaundice was evaluated visually by inspecting the mucous membranes for yellow discoloration, and hemoglobinuria was identified by observing the urine for dark red or brown pigmentation. Blood samples were collected in EDTA and serum tubes daily for molecular and serological analysis. Giemsa-stained blood smears were examined microscopically under a 100X oil immersion objective lens to identify the presence of parasitized erythrocytes, and the percentage of parasitized erythrocytes (PPE) was calculated based on at least 20 microscopic fields (Ozubek and Aktas, 2017).
Genomic DNA was extracted from both blood and tick samples using the PureLink™ Genomic DNA Mini Kit (Invitrogen, USA), following the manufacturer’s instructions. For blood samples, 200 µL of whole blood was processed according to the kit protocol to ensure efficient DNA isolation. For tick samples, female carcasses were bisected using a sterile scalpel after the oviposition period. One half of each carcass was placed into an eppendorf tube, crushed in liquid nitrogen to ensure thorough homogenization, and stored at -20°C until further processing. Approximately 500 larvae from each female tick were also selected, homogenized in liquid nitrogen, and stored under the same conditions. Genomic DNA was then isolated from these prepared samples, ensuring high-quality DNA suitable for downstream molecular analysis. Nested PCR assays were conducted for the detection of Babesia, Theileria, Anaplasma, and Ehrlichia species, as well as B. ovis, using previously established protocols (Georges et al., 2001; Bekker et al., 2002; Aktaş et al., 2005; Kawahara et al., 2006; Oosthuizen et al., 2008). For the first round of PCR, each reaction was prepared in a total volume of 25 μL, containing 2.5 μL of 10X PCR buffer (VitaTaq, Procomcure Biotech, Australia), 2.5 μL of each dNTP (1.25 mM), 0.1 μL of Taq DNA polymerase (5 U/μL), 0.5 μL of each outer primer specific to the target pathogen (20 pmol/μL), 2.5 μL of template DNA, and 16.4 μL of nuclease-free water. For the nested PCR, 1 μL of the first-round PCR product was used as the template, with the reaction mixture and cycling conditions consistent with the first round. Each PCR reaction included positive controls (genomic DNA of B. ovis GenBank accession number EF092454.1; Anaplasma ovis GenBank accession number MG642087.1) and negative controls (DNase/RNase-free water) to ensure the accuracy and reliability of the results. Amplifications were carried out in an automated DNA thermal cycler (Sensequest Labcycler Gradient, Göttingen, Germany). PCR products (10 μL) were electrophoresed on 1.4% agarose gels in TBE buffer for 30 minutes under a constant voltage, and the bands were visualized using the Quantum Vilber Lourmat Gel Imaging System (Marne-la-Vallée, France). Amplification bands corresponding to expected product sizes were clearly identified (Supplementary Table 1).
Descriptive statistics, including the calculation of mean values and standard deviations, were performed to analyze temperature, HCT, and parasitized erythrocyte percentage (PPE) data. Graphical representations, such as line charts and scatter plots (Figures 4–8), were generated using GraphPad Prism software version 8 (GraphPad Software, San Diego, CA).
Figure 4. Temporal dynamics of infection and treatment in splenectomized sheep (#242) experimentally infected with B. ovis Alacakaya stabilate to produce R. bursa larvae infected with B. ovis. On day 1 post-infection (DPI 1), sheep #242 was infested with Babesia-free R. bursa female and male ticks. All engorged female ticks were collected on DPI 10 and left in the incubator to produce infected larvae. Post-collection, the sheep was treated with imidocarb dipropionate (1.2 mg/kg; IM).
Figure 5. Monitoring of temperature, hematocrit (HCT), and parasitemia (PPE) levels in experimental group sheep (#576, #639, #987) after infestation with Babesia-free R. bursa larvae. Engorged nymphs were collected on Days 20–23 (purple dashed line), and adult R. bursa ticks infected with B. ovis were infested on Day 52 (black dashed line). Sheep marked with * succumbed to babesiosis during the study.
Figure 6. Monitoring of temperature, hematocrit (HCT), and parasitemia (PPE) levels in control group sheep (#576, #639, #987) after infestation with Babesia-free R. bursa larvae. Engorged nymphs were collected on Days 20–24 (purple dashed line), and adult R. bursa ticks infected with B. ovis were infested on Day 52 (black dashed line). Sheep marked with * succumbed to babesiosis during the study.
Figure 7. Monitoring of control and experimental group sheep from the start of larval infestation through to the end of the study for B. ovis detection via nPCR. Both groups were tested daily for B. ovis infection. The blue dashed line indicates the point at which the sheep were infested with adult R. bursa ticks infected with B. ovis. nPCR results are indicated as: -: nPCR negative, +: nPCR positive. Sheep marked with * succumbed to babesiosis during the study.
Figure 8. Monitoring of B. ovis BoSA1-specific antibody responses in control and experimental group sheep using iELISA. Antibody levels were measured periodically from the start of larval infestation through to the end of the study. Engorged nymphs were collected on Days 20–24 (purple dashed line), and adult R. bursa ticks infected with B. ovis were infested on Day 52 (black dashed line). The red line on the y-axis represents the cutoff value for the iELISA assay. Sheep marked with * succumbed to babesiosis during the study.
The splenectomized sheep (#242) was monitored daily to track the progression of B. ovis infection and to produce infected R. bursa larvae. After the administration of the B. ovis Alacakaya stabilate, the sheep’s body temperature began to rise on day 5 post-infection (DPI 5), reaching a peak of 42°C on DPI 8. Concurrently, the percentage of parasitized erythrocytes (PPE) increased steadily, peaking at 0.8% on DPI 9, which marked the acute phase of the infection (Figure 4). During this period, clinical signs of babesiosis, including high fever, anemia, jaundice, and lethargy, were observed. These clinical manifestations were managed with the administration of 1.2 mg/kg imidocarb dipropionate (IMDP) on DPI 10, which helped alleviate symptoms and allowed for controlled recovery.
On DPI 10, all engorged female ticks were collected from the sheep and incubated under controlled conditions (27°C, 85% relative humidity). Larvae began to emerge within 30–34 days, and the emergence period lasted 7–14 days. Nested PCR confirmed that all engorged female ticks and their larvae were infected with B. ovis (Supplementary Figure 1). These larvae were subsequently used for the infestation of experimental sheep, forming a critical part of the study’s transmission model.
Following the infestation of the experimental group with B. ovis-infected larvae and the control group with Babesia-free larvae, no clinical signs of babesiosis were observed in either group during the larval infestation period. Both molecular and serological analyses consistently showed negative results for B. ovis in all sheep during this stage (Figures 5, 6). Engorged nymphs collected between DPI 12 and DPI 24 successfully molted into adult ticks within 18–29 days post-collection. Before the DPI 52 infestation with adult ticks, all adult ticks derived from the experimental group were tested in pools, and all pools were confirmed positive for B. ovis via nPCR. This confirmed the infection status of the larvae used in the initial infestation. On DPI 52, all sheep were infested with adult ticks derived from nymphs of the experimental group. This adult tick infestation marked the onset of clinical signs of babesiosis, with clear differences in disease progression between the experimental and control groups.
In the experimental group, clinical signs of babesiosis appeared within a prepatent period of 5 to 8 days following adult tick infestation. Sheep #001 and #357 developed severe disease, exhibiting sustained fever ranging from 39.4°C to 42°C for 10 to 11 days. Maximum parasitemia levels reached 0.4% and 0.5%, respectively, accompanied by severe anemia with HCT levels dropping to a minimum of 14%. Hemoglobinuria was also observed. Molecular detection using nested PCR (nPCR) confirmed the presence of Babesia ovis DNA by day 6 post-infestation (DPI) (Figure 7), while serological analysis using iELISA detected antibodies by DPI 12 to 13 (Figure 8). Despite supportive care, both sheep succumbed to the infection due to the severity of the disease. Sheep #663, also in the experimental group, displayed a shorter prepatent period of 5 DPI and reached the highest parasitemia observed in the study, at 1.8%. Although this sheep experienced fever and anemia, the clinical signs were notably less severe compared to Sheep #001 and #357. Fever persisted for 5 days, and HCT levels dropped to 24%, indicating moderate anemia. Molecular detection of B. ovis was achieved at DPI 8 (Figure 7), and antibodies were confirmed by iELISA at DPI 12 (Figure 8).
In the control group, clinical outcomes were similarly severe in two of the three sheep, but with notable differences in disease progression. Sheep #576 reached a parasitemia peak of 0.6% and exhibited clinical signs of severe anemia, fever, and hemoglobinuria. Molecular detection of B. ovis was achieved by DPI 8 (Figure 7), and antibodies were detected via iELISA at DPI 13 (Figure 8). Sheep #987 displayed the most rapid disease progression among all sheep, succumbing on the second day of parasitemia. This sheep had a maximum parasitemia of 0.3% and exhibited severe anemia and fever. However, antibodies were not detected by iELISA due to the rapid progression of the disease (Figure 8), which left insufficient time for a detectable immune response to develop. Sheep #639, the sole survivor in the control group, displayed milder clinical signs compared to the others. Maximum parasitemia in this sheep reached 0.3%, with fever persisting for 4 days. The HCT level dropped to 27%, indicating mild anemia. Molecular detection of B. ovis DNA occurred at DPI 7 (Figure 7), and antibodies were confirmed by iELISA at DPI 11 (Figure 8). This sheep demonstrated a less severe disease progression compared to Sheep #576 and #987.
This study sought to evaluate whether infestation with B. ovis-infected R. bursa larvae could lead to a milder form of babesiosis compared to sheep infested with larvae. Contrary to the hypothesis that infected larval infestation might induce a protective immune response against subsequent exposure to infected adult ticks, our findings conclusively demonstrated that B. ovis-infected larvae neither cause disease nor confer any protective effect.
Our results showed no evidence of B. ovis infection or clinical babesiosis following infestation with infected larvae, as confirmed by negative nPCR and iELISA results in all sheep. These findings align with previous studies, such as Erster et al. (2016), which established that larvae of R. bursa are incapable of transmitting B. ovis. Furthermore, no seroconversion or parasitemia was observed in the experimental group, even after prolonged monitoring up to day 52, further supporting the notion that larval stages do not play a role in the transmission dynamics of B. ovis. This contrasts with earlier suggestions by Yeruham et al. (1998), which proposed a delayed immune response following larval infestation. Our findings provide robust evidence against this hypothesis and suggest that any reported serological responses in previous studies might be attributed to differences in experimental conditions, diagnostic methods, or host variability. The inability of larvae to transmit B. ovis could reflect biological barriers such as insufficient pathogen replication, absence of salivary components required for transmission, or host-specific immune factors at the larval feeding stage.
In stark contrast to the larval stage, adult R. bursa ticks were confirmed to be the primary vectors for B. ovis transmission. Following infestation with B. ovis-infected adult ticks, all sheep developed clinical babesiosis, characterized by fever, anemia, and hemoglobinuria. No significant differences in disease severity were observed between the experimental and control groups. For instance, PPE levels peaked at 0.4%–0.5% in sheep #001 and #357 (experimental group) and at 0.6% in sheep #576 (control group). This indicates that prior exposure to infected larvae did not mitigate the severity of the disease caused by adult ticks. Interestingly, individual variability in disease progression was evident. Sheep #987 exhibited rapid disease progression, succumbing on the second day of parasitemia, whereas sheep #639 displayed a milder disease course with lower parasitemia levels and faster recovery. Although temporal differences in disease progression between groups were not observed in this study, further investigation into this aspect could help clarify whether prior larval infestation has subtle immunomodulatory effects that are not apparent in small sample sizes. These differences suggest that host-specific factors, such as genetic predisposition, immune competence, or other physiological traits, may influence the clinical outcome of B. ovis infection. Identifying these factors could provide valuable insights into host-pathogen interactions and inform selective breeding programs to enhance disease resistance in livestock.
The differential ability of tick life stages to transmit Babesia spp. reveals a fascinating interplay of biological, ecological, and epidemiological factors that shape parasite-vector-host dynamics. In our study, we observed that immature stages of R. bursa, particularly larvae, could acquire B. ovis but were unable to transmit the parasite effectively. This finding aligns with previous research highlighting stage-specific barriers in tick-borne pathogen transmission (Erster et al., 2016). Such stage-specific dynamics are not unique to R. bursa but are a common feature in the transmission cycles of various Babesia species, emphasizing the complex evolutionary adaptations of both the parasite and its vector. For instance, only larval stages of Rhipicephalus microplus transmit Babesia bovis, whereas transmission of Babesia bigemina is restricted to nymphs and adults (Dalgliesh and Stewart, 1983). This stage-specificity may be attributed to differences in host attachment preferences, feeding behavior, or immune responses encountered by ticks during their development. A contrasting example is Babesia divergens, which displays a broader transmission capacity, as all life stages larvae, nymphs, and adults can effectively transmit the parasite. Notably, engorging female ticks play a pivotal role in acquiring the infection, which is subsequently transmitted transovarially to their progeny, maintaining the parasite within tick populations and across hosts. This highlights a unique interplay between transstadial and transovarial transmission mechanisms in perpetuating B. divergens (Donnelly and Peirce, 1975; Lewis and Young, 1980). Similarly, Babesia canis demonstrates stage-specific transmission patterns with its vector, Dermacentor reticulatus. Adults are the primary transmitters due to their feeding preferences on canine hosts, while immature stages fail to transmit the pathogen effectively. The inability of immature ticks to act as competent vectors may reflect barriers in parasite acquisition during earlier stages or limitations in pathogen development within the vector (Ristic, 1988). These observations underscore the importance of studying the immune responses elicited by immature tick feeding. Investigating whether larval or nymphal feeding primes the host’s immune system in ways that could influence subsequent pathogen transmission remains an important avenue for future research.
To summarize, this study confirms that the transmission of B. ovis is restricted to adult R. bursa ticks, while larvae, although capable of acquiring the parasite, do not induce clinical or immunological effects in their hosts. These findings reaffirm the critical role of adult ticks as the primary vectors for B. ovis, consistent with previous studies. However, a key insight from this study is that larval feeding does not influence the clinical outcomes of subsequent infections with adult ticks, suggesting that subclinical infections or milder disease courses observed in natural settings are unlikely to be related to larval feeding. This finding highlights the importance of focusing control strategies on adult tick populations, particularly during periods of peak activity, to effectively mitigate the spread of ovine babesiosis. Beyond the implications for B. ovis, these findings raise broader questions about the potential epidemiological role of immature tick stages in other tick-borne disease systems. Although immature ticks may not directly transmit pathogens, they can serve as reservoirs, maintaining infections within tick populations through transstadial transmission. Such dynamics have been observed in B. bovis and B. bigemina, where immature stages acquire the pathogen and ensure its persistence until competent stages emerge. Future studies should explore the molecular and physiological mechanisms underlying the inability of immature ticks to transmit B. ovis. Investigating whether larval and nymphal feeding induces subtle immunomodulatory effects or primes host immunity could reveal important host-pathogen-vector interactions. Moreover, the application of longitudinal immune profiling and gene expression analyses could clarify whether prior larval infestation affects the host’s susceptibility to subsequent infections. Investigating the barriers that prevent transmission in immature stages, such as developmental or molecular limitations, could uncover new insights into the complex life cycles of Babesia parasites and their vectors. By exploring these dynamics, future research could identify novel strategies for disrupting the parasite’s life cycle at multiple stages of the tick’s development, enhancing vector control efforts.
In conclusion, our study demonstrates that the transmission of B. ovis occurs predominantly through adult R. bursa, with the larval stage playing no significant role in the transmission cycle. Furthermore, exposure to B. ovis infected larvae does not result in milder disease outcomes or provide any protective immunity against subsequent infections by adult ticks. These findings emphasize the importance of prioritizing the control of adult R. bursa populations to effectively manage the spread of ovine babesiosis. Future research should aim to uncover the molecular and physiological mechanisms that limit the ability of immature ticks to transmit B. ovis. Such insights could pave the way for innovative strategies to disrupt the parasite’s life cycle and improve vector management practices. Additionally, comparative studies across different Babesia species and their vectors could offer valuable perspectives on the evolutionary and ecological factors influencing stage-specific transmission dynamics.
The raw data supporting the conclusions of this article will be made available by the authors, without undue reservation.
The animal study was approved by Firat University, Animal Experiment Ethic Committee, protocol number 2023/12-05. The study was conducted in accordance with the local legislation and institutional requirements.
SO: Conceptualization, Investigation, Methodology, Project administration, Writing – original draft, Writing – review & editing. MU: Conceptualization, Methodology, Project administration, Writing – original draft, Writing – review & editing. OC: Formal analysis, Writing – original draft, Writing – review & editing. FS: Formal analysis, Investigation, Writing – original draft, Writing – review & editing. MA: Conceptualization, Investigation, Methodology, Project administration, Writing – original draft, Writing – review & editing.
The author(s) declare financial support was received for the research, authorship, and/or publication of this article. This study was supported financially by the Comprehensive Research Project (VF.24.30) from the Scientific Research Project, Firat University.
We are grateful to Aleyna Karoglu, Arda Eyvaz, Havva Nur Cevik and Halil Unal for the excellent technical and administrative support. We would like to acknowledge the use of OpenAI’s ChatGPT-4o for assistance with language editing.
The authors declare that the research was conducted in the absence of any commercial or financial relationships that could be construed as a potential conflict of interest.
The author(s) declared that they were an editorial board member of Frontiers, at the time of submission. This had no impact on the peer review process and the final decision.
The author(s) declare that Generative AI was used in the creation of this manuscript. Generative AI was not used for content generation, data analysis, or results interpretation in this manuscript. OpenAI’s ChatGPT-4o was only used for language editing.
All claims expressed in this article are solely those of the authors and do not necessarily represent those of their affiliated organizations, or those of the publisher, the editors and the reviewers. Any product that may be evaluated in this article, or claim that may be made by its manufacturer, is not guaranteed or endorsed by the publisher.
The Supplementary Material for this article can be found online at: https://www.frontiersin.org/articles/10.3389/fcimb.2025.1544775/full#supplementary-material
Adewumi, T. S., Takeet, M. I., Akande, F. A., Sonibare, A. O., Okpeku, M. (2022). Prevalence and molecular characterization of Babesia ovis infecting sheep in Nigeria. Sustainability 14, 16974. doi: 10.3390/su142416974
Aktas, M., Altay, K., Dumanli, N. (2007). Determination of prevalence and risk factors for infection with Babesia ovis in small ruminants from Turkey by polymerase chain reaction. Parasitol. Res. 100, 797–802. doi: 10.1007/s00436-006-0345-2
Aktaş, M., Altay, K., Dumanli, N. (2005). Development of a polymerase chain reaction method for diagnosis of Babesia ovis infection in sheep and goats. Vet. Parasitol. 133, 277–281. doi: 10.1016/j.vetpar.2005.05.057
Almazán, C., Bonnet, S., Cote, M., Slovák, M., Park, Y., Šimo, L. (2018). A versatile model of hard tick infestation on laboratory rabbits. J. Vis. Exp. Jove. 140, 57994. doi: 10.3791/57994-v
Altay, K., Aktas, M., Dumanli, N. (2008). Detection of Babesia ovis by PCR in Rhipicephalus bursa collected from naturally infested sheep and goats. Res. Vet. Sci. 85, 116–119. doi: 10.1016/j.rvsc.2007.08.002
Alzan, H. F., Bastos, R. G., Ueti, M. W., Laughery, J. M., Rathinasamy, V. A., Cooke, B. M., et al. (2021). Assessment of Babesia bovis 6cys A and 6cys B as components of transmission blocking vaccines for babesiosis. Parasitol. Vectors 14, 210. doi: 10.1186/s13071-021-04712-7
Antunes, S., Couto, J., Ferrolho, J., Rodrigues, F., Nobre, J., Santos, A. S., et al. (2018). Rhipicephalus bursa sialotranscriptomic response to blood feeding and Babesia ovis infection: identification of candidate protective antigens. Front. Cell. Infect. Microbiol. 8, 116. doi: 10.3389/fcimb.2018.00116
Aouadi, A., Leulmi, H., Boucheikhchoukh, M., Benakhla, A., Raoult, D., Parola, P. (2017). Molecular evidence of tick-borne hemoprotozoan-parasites (Theileria ovis and Babesia ovis) and bacteria in ticks and blood from small ruminants in Northern Algeria. Comp. Immunol. Microbiol. Infect. Dis. 50, 34–39. doi: 10.1016/j.cimid.2016.11.008
Azmi, K., Ereqat, S., Nasereddin, A., Al-Jawabreh, A., Baneth, G., Abdeen, Z. (2016). Molecular detection of Theileria, Babesia, and Hepatozoon spp. in ixodid ticks from Palestine. Ticks Tick-Borne Dis. 7, 734–741. doi: 10.1016/j.ttbdis.2016.03.003
Bastos, R. G., Laughery, J. M., Ozubek, S., Alzan, H. F., Taus, N. S., Ueti, M. W., et al. (2022). Identification of novel immune correlates of protection against acute bovine babesiosis by superinfecting cattle with in vitro culture attenuated and virulent Babesia bovis strains. Front. Immunol. 13, 1045608. doi: 10.3389/fimmu.2022.1045608
Bekker, C. P., De Vos, S., Taoufik, A., Sparagano, O. A., Jongejan, F. (2002). Simultaneous detection of Anaplasma and Ehrlichia species in ruminants and detection of Ehrlichia ruminantium in Amblyomma variegatum ticks by reverse line blot hybridization. Vet. Microbiol. 89, 223–238. doi: 10.1016/S0378-1135(02)00179-7
Bozan, M., Ulucesme, M. C., Eyvaz, A., Ceylan, O., Sevinc, F., Aktas, M., et al. (2024). Serological and molecular survey of Babesia ovis in healthy sheep in Türkiye. Parasitologia 4, 162–171. doi: 10.3390/parasitologia4020014
Ceylan, O., Sevinc, F. (2020). Endemic instability of ovine babesiosis in Turkey: A country-wide sero-epidemiological study. Vet. Parasitol. 278, 109034. doi: 10.1016/j.vetpar.2020.109034
Ceylan, O., Xuan, X., Sevinc, F. (2021). Primary tick-borne protozoan and rickettsial infections of animals in Turkey. Pathogens 10, 231. doi: 10.3390/pathogens10020231
Dalgliesh, R. J., Stewart, N. P. (1983). The use of tick transmission by Boophilus microplus to isolate pure strains of Babesia bovis, Babesia bigemina and Anaplasma marginale from cattle with mixed infections. Vet. Parasitol. 13, 317–323. doi: 10.1016/0304-4017(83)90047-X
Donnelly, J., Peirce, M. A. (1975). Experiments on the transmission of Babesia divergens to cattle by the tick Ixodes ricinus. Int. J. Parasitol. 5, 363–367. doi: 10.1016/0020-7519(75)90085-5
Erster, O., Roth, A., Wolkomirsky, R., Leibovich, B., Savitzky, I., Shkap, V. (2016). Transmission of Babesia ovis by different Rhipicephalus bursa developmental stages and infected blood injection. Ticks Tick-Borne Dis. 7, 13–19. doi: 10.1016/j.ttbdis.2015.07.017
Ferrer, D., Castellà, J., Gutierrez, J. F. (1998). Seroprevalence of Babesia ovis in sheep in Catalonia, northeastern Spain. Vet. Parasitol. 79, 275–281. doi: 10.1016/S0304-4017(98)00175-7
Firat, R., Ulucesme, M. C., Aktaş, M., Ceylan, O., Sevinc, F., Bastos, R. G., et al. (2024). Role of Rhipicephalus bursa larvae in transstadial transmission and endemicity of Babesia ovis in chronically infected sheep. Front. Cell. Infect. Microbiol. 14. doi: 10.3389/fcimb.2024.1428719
Georges, K., Loria, G. R., Riili, S., Greco, A., Caracappa, S., Jongejan, F., et al. (2001). Detection of haemoparasites in cattle by reverse line blot hybridisation with a note on the distribution of ticks in Sicily. Vet. Parasitol. 99, 273–286. doi: 10.1016/S0304-4017(01)00488-5
Gh, H., Sepahvand-Mohammadi, E., Afshari, A., Bozorgi, S. (2020). Molecular detection of Theileria spp. and Babesia ovis Infection in Sheep in Baneh, Iran. Arch. Razi Inst. 75, 289. doi: 10.22092/ari.2019.125136.1297
Horta, S., Barreto, M. C., Pepe, A., Campos, J., Oliva, A. (2014). Highly sensitive method for diagnosis of subclinical B. ovis infection. Ticks Tick-Borne Dis. 5, 902–906. doi: 10.1016/j.ttbdis.2014.07.005
Hussein, N. M., Mohammed, E. S., Hassan, A. A., El-Dakhly, K. M. (2017). Distribution pattern of Babesia and Theileria species in sheep in Qena Province, Upper Egypt. Arch. Parasitol. 1, 1–4.
Iqbal, F., Fatima, M., Shahnawaz, S., Naeem, M., Shaikh, R. S., Ali, M., et al. (2011). A study on the determination of risk factors associated with babesiosis and prevalence of Babesia sp., by PCR amplification, in small ruminants from Southern Punjab (Pakistan). Parasite J. Société Fr. Parasitol. 18, 229. doi: 10.1051/parasite/2011183229
Kawahara, M., Rikihisa, Y., Lin, Q., Isogai, E., Tahara, K., Itagaki, A., et al. (2006). Novel genetic variants of Anaplasma phagocytophilum, Anaplasma bovis, Anaplasma centrale, and a novel Ehrlichia sp. in wild deer and ticks on two major islands in Japan. Appl. Environ. Microbiol. 72 (2), 1102–1109. doi: 10.1128/AEM.72.2.1102-1109.2006
Lewis, D., Young, E. R. (1980). The transmission of a human strain of Babesia divergens by Ixodes ricinus ticks. J. Parasitol. 66, 359–360. doi: 10.2307/3280841
Oosthuizen, M. C., Zweygarth, E., Collins, N. E., Troskie, M., Penzhorn, B. L. (2008). Identification of a Novel Babesia sp. from a Sable Antelope (Hippotragus Niger Harris 1838). J. Clin. Microbiol. 46, 2247–2251. doi: 10.1128/JCM.00167-08
Ozubek, S., Aktas, M. (2017). Molecular and parasitological survey of ovine piroplasmosis, including the first report of Theileria annulata (Apicomplexa: Theileridae) in sheep and goats from Turkey. J. Med. Entomol. 54, 212–220. doi: 10.1093/jme/tjw134
Ozubek, S., Bastos, R. G., Alzan, H. F., Inci, A., Aktas, M., Suarez, C. E. (2020). Bovine babesiosis in Turkey: Impact, current gaps, and opportunities for intervention. Pathogens 9, 1041. doi: 10.3390/pathogens9121041
Pipano, E. (2022). One hundred years of veterinary parasitology in the land of Israel. Isr. J. Vet. Med. 77, 3.
Rjeibi, M. R., Darghouth, M. A., Gharbi, M. (2016). Prevalence of Theileria and Babesia species in Tunisian sheep. Onderstepoort J. Vet. Res. 83, 6. doi: 10.4102/ojvr.v83i1.1040
Savini, G., Conte, A., Semproni, G., Scaramozzino, P. (1999). Tick-borne diseases in ruminants of Central and Southern Italy: epidemiology and case reports. Parassitologia 41 Suppl 1, 95–100.
Schnittger, L., Ganzinelli, S., Bhoora, R., Omondi, D., Nijhof, A. M., Florin-Christensen, M. (2022). The Piroplasmida Babesia, Cytauxzoon, and Theileria in farm and companion animals: Species compilation, molecular phylogeny, and evolutionary insights. Parasitol. Res. 121, 1207–1245. doi: 10.1007/s00436-022-07424-8
Sevinc, F., Cao, S., Xuan, X., Sevinc, M., Ceylan, O. (2015). Identification and expression of Babesia ovis secreted antigen 1 and evaluation of its diagnostic potential in an enzyme-linked immunosorbent assay. J. Clin. Microbiol. 53, 1531–1536. doi: 10.1128/JCM.03219-14
Sevinc, F., Turgut, K., Sevinc, M., Ekici, O. D., Coskun, A., Koc, Y., et al. (2007). Therapeutic and prophylactic efficacy of imidocarb dipropionate on experimental Babesia ovis infection of lambs. Vet. Parasitol. 149, 65–71. doi: 10.1016/j.vetpar.2007.07.014
Sevinç, F., Xuenan, X. (2015). Major tick-borne parasitic diseases of animals: A frame of references in Turkey. Eurasian J. Vet. Sci. 31, 132–142. doi: 10.15312/EurasianJVetSci.2015310969
Silva, M. G., Bastos, R. G., Laughery, J. M., Alzan, H. F., Rathinasamy, V. A., Cooke, B. M., et al. (2023). Vaccination of cattle with the Babesia bovis sexual-stage protein HAP2 abrogates parasite transmission by Rhipicephalus microplus ticks. NPJ Vaccines 8, 140. doi: 10.1038/s41541-023-00741-8
Theodoropoulos, G., Gazouli, M., Ikonomopoulos, J. A., Kantzoura, V., Kominakis, A. (2006). Determination of prevalence and risk factors of infection with Babesia in small ruminants from Greece by polymerase chain reaction amplification. Vet. Parasitol. 135, 99–104. doi: 10.1016/j.vetpar.2005.07.021
Tumwebaze, M. A., Byamukama, B., Tayebwa, D. S., Byaruhanga, J., Angwe, M. K., Galon, E. M., et al. (2020). First molecular detection of Babesia ovis, Theileria spp., Anaplasma spp., and Ehrlichia ruminantium in goats from western Uganda. Pathogens 9, 895. doi: 10.3390/pathogens9110895
Ulucesme, M. C., Ozubek, S., Aktas, M. (2024). Incompetence of vector capacity of Rhipicephalus bursa to transmit Babesia aktasi following feeding on clinically infected goat with high level of parasitemia. Vet. Sci. 11, 309. doi: 10.3390/vetsci11070309
Ulucesme, M. C., Ozubek, S., Karoglu, A., Turk, Z. I., Olmus, I., Irehan, B., et al. (2023). Small Ruminant Piroplasmosis: high prevalence of Babesia aktasi n. sp. in goats in Türkiye. Pathogens 12, 514. doi: 10.3390/pathogens12040514
Yeruham, I. (1995). Babesiosis in sheep: the ecology of the tick vector Rhiphicepalus bursa, transmission of Babesia ovis, immunological, clinical and clinico-pathological aspects. . PhD thesis. (Israel: The Hebrew University of Jerusalem).
Yeruham, I., Hadani, A., Galker, F. (1998). Some epizootiological and clinical aspects of ovine babesiosis caused by Babesia ovis—A review. Vet. Parasitol. 74, 153–163. doi: 10.1016/S0304-4017(97)00143-X
Keywords: Babesia ovis, experimental infestation, ovine babesiosis, Rhipicephalus bursa, transmission dynamics
Citation: Ozubek S, Ulucesme MC, Ceylan O, Sevinc F and Aktas M (2025) The impact of Babesia ovis-infected Rhipicephalus bursa larvae on the severity of babesiosis in sheep. Front. Cell. Infect. Microbiol. 15:1544775. doi: 10.3389/fcimb.2025.1544775
Received: 17 December 2024; Accepted: 31 January 2025;
Published: 20 February 2025.
Edited by:
Sandra Antunes, NOVA University of Lisbon, PortugalReviewed by:
Ana Gonçalves Domingos, New University of Lisbon, PortugalCopyright © 2025 Ozubek, Ulucesme, Ceylan, Sevinc and Aktas. This is an open-access article distributed under the terms of the Creative Commons Attribution License (CC BY). The use, distribution or reproduction in other forums is permitted, provided the original author(s) and the copyright owner(s) are credited and that the original publication in this journal is cited, in accordance with accepted academic practice. No use, distribution or reproduction is permitted which does not comply with these terms.
*Correspondence: Sezayi Ozubek, c296dWJla0BmaXJhdC5lZHUudHI=
Disclaimer: All claims expressed in this article are solely those of the authors and do not necessarily represent those of their affiliated organizations, or those of the publisher, the editors and the reviewers. Any product that may be evaluated in this article or claim that may be made by its manufacturer is not guaranteed or endorsed by the publisher.
Research integrity at Frontiers
Learn more about the work of our research integrity team to safeguard the quality of each article we publish.