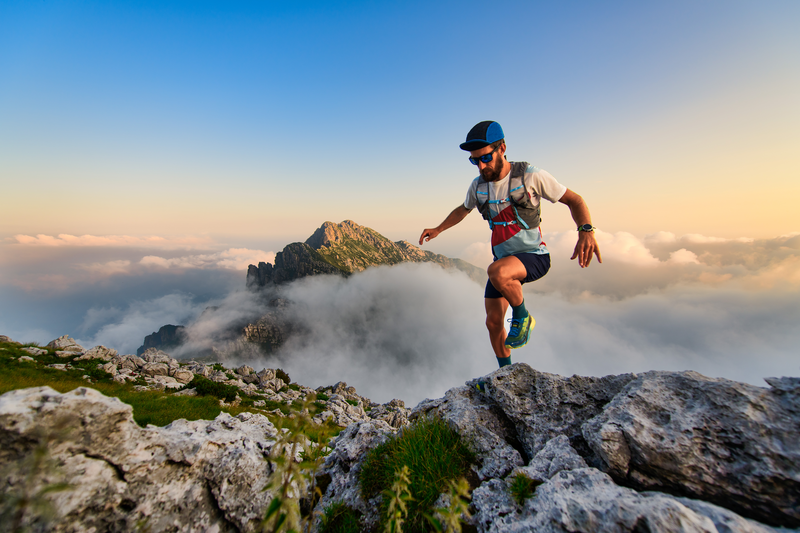
95% of researchers rate our articles as excellent or good
Learn more about the work of our research integrity team to safeguard the quality of each article we publish.
Find out more
ORIGINAL RESEARCH article
Front. Cell. Infect. Microbiol. , 20 March 2025
Sec. Veterinary and Zoonotic Infection
Volume 15 - 2025 | https://doi.org/10.3389/fcimb.2025.1528408
This article is part of the Research Topic Viral Diseases in Swine View all 7 articles
This study investigates the plasmid sequences of porcine O139:H1 Shiga toxin-producing Escherichia coli (STEC) responsible for Edema Disease (ED). Whole-genome analysis reveals significant similarities between these strains and known plasmids, notably pW1316-2, which harbors key virulence genes like hemolysin (hlyA, hlyB) and adhesion factors (aidA-I, faeE). These genes contribute to the cytotoxicity and host colonization associated with ED. Additionally, similarities to plasmids from Shigella flexneri 2a highlight potential associations in virulence gene regulation, particularly via the Hha-H-NS complex. The identification of sequences resembling plasmid pB71 raises serious concerns about the emergence of highly pathogenic strains, as it includes tetracycline resistance genes (tetA, tetC, tetR). This research emphasizes the role of plasmid-like sequences in ED pathogenesis, indicating important implications for swine industry management and public health.
Edema Disease (ED) is a sudden and severe form of toxemia caused by Shiga toxin-producing Escherichia coli (STEC) strains (Moxley, 2000). These isolates produce F18 fimbrial adhesins, α-hemolysin, and Shiga toxin 2e (stx2e), primarily affecting healthy, rapidly growing nursery pigs (Fairbrother and Nadeau, 2019). Following adhesion of STEC to the intestinal mucosa mediated by F18, stx2e enters the bloodstream, leading to vascular damage in various organs, including the brain and gastrointestinal tract (Marques et al., 1987). ED poses a significant economic burden on the pig industry, and its treatment is often ineffective due to the disease’s sudden onset and rapid progression (Gale and Velazquez, 2020).
The prevalent STEC serogroups associated with ED include O138, O139, and O141, with a notable presence of serotypes O139:K82:H1, O141:K85:H4, and O138:K81:NM (Perrat et al., 2022). Key virulence factors, beside the F18 fimbriae, contributing to ED development include Stx2e and α-hemolysin (Gale and Velazquez, 2020). Their coding genes are typically harbored on plasmids, except for the stx2e gene, which is commonly integrated into the chromosome within a prophage (Denamur et al., 2021; Gigliucci et al., 2021). The initial colonization of the porcine intestine by STEC causing ED is primarily mediated by F18 (F18ab or F18ac) and F4 (K88) adhesins (DebRoy et al., 2009). Additionally, bacterial AIDA (adhesin involved in diffuse adherence) also contributes to the initial phases of the pathogenic process (Gale and Velazquez, 2020). These adhesins are also encoded by genes described in plasmids of Enterobacterales members other than E. coli, particularly Shigella spp. and Salmonella enterica, indicating a potential mechanism of acquisition of these adhesins by STEC causing ED from these species (Keshmiri et al., 2022; Badouei et al., 2023). Horizontal transfer of virulence plasmids among STEC pathotypes is described and could be a mean to enhance the pathogenicity of ED strains and eventually leading to the emergence of hybrid pathotypes (Cointe et al., 2018; Tomeh et al., 2024). Characterizing these strains is therefore crucial for assessing their virulence potential, facilitating the development of detection methods, and understanding their evolution, to unravel potential implications for public health (Nemati et al., 2024).
Plasmid-encoded genes of porcine O139 STEC strains thus can influence various stages of ED pathophysiology, including those related with adhesion, invasion, colonization, and modulation of host immune responses (Fairbrother and Nadeau, 2019). Additionally, food contamination with STEC may pose significant concerns for food safety and public health (Tseng et al., 2014). In this study, we conducted a whole-genome sequencing-based study on a collection of Italian O139 STEC strains isolated from pigs with ED to investigate the structure of their plasmid-like sequences and to elucidate the possible ways such a plasmid was acquired by ED-associated STEC as well as the possibility to identify new relevant virulence factors carried on these sequences.
We analyzed a collection of 53 STEC O139:H1 strains isolated from pigs affected by ED in Italy, maintained in the National Reference Laboratory for E. coli collections at the Istituto Superiore di Sanità. Additionally, we included in this study 83 more STEC O139:H1 genomes retrieved from the GenBank and the European Nucleotide Archive (ENA) databases, from strains isolated from pigs or other sources (Supplementary Tables 1, 2).
To conduct whole genome sequencing (WGS), total DNA was extracted from a 2 mL overnight TSB culture of each strain grown at 37°C using the GRS Genomic DNA Kit Bacteria (GRISP Research Solutions, Porto, Portugal). The majority of sequences were generated using Ion Torrent sequencing technology (Thermo Fisher Scientific, MA, USA). Sequencing libraries of approximately 400 bp were prepared from 100 ng of total DNA using the NEBNext Fast DNA Fragmentation & Library Prep Set for Ion Torrent (New England BioLabs, MA, USA). These libraries were then processed through emulsion PCR and enrichment on the Ion OneTouch 2 System, followed by sequencing on an Ion Torrent S5 platform (Thermo Fisher Scientific, MA, USA) using the ION 520/530 KIT-OT2 (Thermo Fisher Scientific, MA, USA) according to the manufacturer’s instructions. All genomic sequences are accessible at the GenBank (BioProject: PRJNA1152229).
Most of the bioinformatic analyses to characterize the genomes were carried out using the tools available on the Galaxy public server ARIES (Istituto Superiore di Sanità, https://www.iss.it/site/aries) (Knijn et al., 2020).
Single-end reads from the Ion Torrent S5 platform were assembled using SPADES version 3.12.0 with default parameters (Bankevich et al., 2012), and filtered using the Filter SPAdes repeats tool (https://github.com/phac-nml/galaxy_tools) with default settings to eliminate repeated contigs or those <1,000 bases in length. Paired-end reads were trimmed, filtered using the Extended Randomized Numerical alignEr–filter (Del Fabbro et al., 2013), and de novo assembled using SPAdes version 3.10.0 (Bankevich et al., 2012).
Multilocus sequence typing was performed using the MentaLiST tool version 0.2.3 (Feijao et al., 2018), following the scheme developed by Wirth et al (Wirth et al., 2006). The assembled contigs were analyzed using BLAST (http://blast.ncbi.nlm.nih.gov/Blast.cgi) and the blastn algorithm version 2.7.1 using different databases. Serotyping was determined by aligning the contigs with reference sequences for the O and H antigen genes (Joensen et al., 2015). Subtyping of stx was performed using the Shiga toxin typer tool v2.0 (https://github.com/aknijn/shigatoxin-galaxy), which conducts an optimized blastn search against the sequence database of stx subtypes developed by the Statens Serum Institut (https://bitbucket.org/genomicepidemiology/virulencefinder_db/src/master/stx.fsa).
BLAST + blastn was also used to detect the presence of plasmid-related sequences and their replicon types within the whole genome of the O139:H1 strains using the PlasmidFinder and PLSDB tools, with a minimum identity of 95% and minimum coverage of 60%. Additionally, we retrieved the most closely related plasmid sequences available online from the National Center for Biotechnology Information (NCBI) nucleotide collection database (accessed June 1, 2024). The focus of this analysis was on determining the similarity of these identified sequences to known reference plasmid sequences.
The identification of resistance genes was conducted by submitting the complete reference plasmid nucleotide sequence to the ResFinder web server with default parameters, which required a minimum identity of 90% and minimum coverage of 60% (http://genepi.food.dtu.dk/resfinder) (Zankari et al., 2012). Similarly, the reference plasmid was submitted to the VFDB web server via the BLAST sequence-similarity (setB), also with default parameters, to identify virulence factors (http://www.mgc.ac.cn/VFs/search_VFs.htm) (Liu et al., 2022).
The Prokka tool (Galaxy Version 1.14.5) (Seemann, 2014) was used to perform functional annotation on the assembled sequences of the O139:H1 strains and reference plasmids, utilizing the E. coli-specific gene database and default parameters. Additionally, the Blast Ring Image Generator (BRIG) software v0.95 (Alikhan et al., 2011) was used with default parameters to compare and visually represent these plasmid-like sequences in relation to reference plasmids, highlighting regions of similarity and potential functional significance.
The associations and clustering between the virulence genes identified on the reference plasmids were assessed using the STRING database (https://string-db.org/). The analyses were carried out following the updated instructions (The STRING database in 2023) (Szklarczyk et al., 2023).
We performed the analysis using core genome multilocus sequence typing (cgMLST) with the chewBBACA tool and the INNUENDO project’s scheme, available on the Galaxy public server ARIES, which includes 2,360 loci (Llarena et al., 2018; Silva et al., 2018). Pairwise comparisons were deemed reliable when more than 80% of loci for each sample were assigned to an allele. The distances between strains were calculated by comparing allelic profiles pairwise, using the chewTree tool on the ARIES webserver. For each sample pair, alleles that were missing, partially identified, or incorrectly assigned to any locus were excluded. The resulting dendrogram was visualized using Newick Display on Galaxy Version 1.6 (Junier and Zdobnov, 2010).
Among the genomes of the 53 Italian strains examined, 50 were classified as ST1, and 3 as ST955. All strains were identified as O139:H1 serotype, with all the 53 strains carrying the stx2 gene only, specifically subtype stx2e.
Among the additional 83 genomes retrieved from the public domain, 79 were classified as ST1, two as ST955, one as ST10859, and one as ST114. Of these, 79 strains carried the stx2 gene, subtype stx2e, while the stx2 subtypes in four isolates were not identified (Supplementary Tables 1, 2).
Three different reference plasmids were identified in the genome of studied isolates based on the similarity between plasmid-like sequences in the O139:H1 strains and the identified reference plasmid sequences according to the PLSDB (Figure 1): Escherichia coli O139:H1 strain W13-16 plasmid pW1316-2 (Accession number: NZ_CP080237.1), Shigella flexneri 2a strain ATCC 29903 (Accession number: CP026790.1), and Salmonella enterica subsp. enterica serovar Typhimurium strain 21G7 isolate B71 plasmid pB71 (Accession number: NZ_KP899806.1). Moreover, some genome returned hits against Escherichia coli O111:H- str. 11128 plasmid pO111_1 (Accession number: NC_013365.1), Escherichia coli strain 15OD0495 plasmid p15ODAR (Accession number: NZ_MG904995.1), Escherichia coli strain ESBL3153 plasmid pESBL3153-IncX4 (Accession number: NZ_MW390521.1), and Escherichia coli strain 20Ec-P-124 plasmid pMRY16-002_3 (Accession number: NZ_AP017613.1) plasmids (Figure 1).
Figure 1. The identified reference plasmids in the genomes of the 53 Italian STEC strains which included: pW1316-2 (37/53, 69.8%), Shigella flexneri 2a (16/53, 30.1%), pB71 (15/53, 28.3%), pO111_1 (1/53, 1.8%), p15ODAR (1/53, 1.8%), pESBL3153-IncX4 (1/53, 1.8%), and pMRY16-002_3 (1/53, 1.8%).
We identified seven different replicon types among the plasmid-related sequences in the O139:H1 genomes based on the PlasmidFinder which included: IncI1-I(Alpha), IncI2, IncFIA(HI1), IncHI1B(R27), IncFII, IncX1, IncX4 (Figures 2, 3).
Figure 2. Different plasmid replicon types detected among the identified plasmid-related sequences in the genomes of the 53 Italian STEC strains which included: IncI1-I(Alpha) (16/53, 30.1%), IncI2 (4/53, 7.5%), IncFIA(HI1) (16/53, 30.1%), IncHI1B(R27) (16/53, 30.1%), IncFII (24/53, 45.2%), IncX1 (17/53, 32.0%), and IncX4 (2/53, 3.7%).
Figure 3. Heatmap generated according to the co-occurrence between identified reference plasmids and different detected replicon types among the genomes of the 53 Italian STEC strains.
As far as the additional genomes included in the study are concerned, the plasmids Escherichia coli O139:H1 strain W13-16 plasmid pW1316-2 (Accession number: NZ_CP080237.1), Shigella flexneri 2a strain ATCC 29903 (Accession number: CP026790.1), and Salmonella enterica subsp. enterica serovar Kentucky plasmid pCS0010A_9 (Accession number: NC_019104.1) were the most frequently identified reference plasmids to which the genomes demonstrated similarity. Moreover, the replicon types IncFII, IncX1, IncI1-I(Alpha), and IncHI1B(R27) were the most frequently detected replicon sequences (Supplementary Tables 1, 2).
Overall, the most frequent plasmid signatures identified in all strains (O139:H1 STEC isolates of our and other studies), were to pW1316-2, Shigella flexneri 2a, and pB71 plasmids.
Our analysis using BRIG software revealed the presence of hha, yhcR, finO, aidA-I, tibC, rhaR_1, tpx, rhaR_2, faeE, elfC, bin3, pir, dnaT, hlyD, hlyB, hlyA, hlyC, and topB genes on the pW1316-2 plasmid (Figure 4).
Figure 4. Whole-genome comparison of Blast Ring Image Generated for Escherichia coli O139:H1 pW1316-2 (NZ_CP080237.1) plasmid in O139:H1 STEC strains isolated from pigs affected by Edema disease in Italy.
Shigella flexneri 2a plasmid, harbored speE, higB_1, RepB_1, RepB_2, dam, noc, traC, virB, parM, uvrD, tus, hha, repE, hns, smc, dcm, umuD, umuC, dsbC, and resA genes (Figure 5).
Figure 5. Whole-genome comparison of Blast Ring Image Generated for Shigella flexneri 2a (CP026790.1) plasmid in O139:H1 STEC strains isolated from pigs affected by Edema disease in Italy.
Ultimately, in pB71 plasmid, the genes uvrD, tus, hha, repE, hns, smc, dcm, yhcR, cynR, gltS, yqjZ, tetR, tetA, tetC, folP, emrE, ant1, xerC, hin, cat, catM, ifcA, proP, umuD, umuC, dsbC, resA, corA, higB-1, repB_2, dam, repB_1, traC, virB, and parM were present (Figure 6). Most of the genes detected were present in all the reference plasmid sequences identified in the studied genomes (Figures 4-6).
Figure 6. Whole-genome comparison of Blast Ring Image Generated for Salmonella enterica pB71 plasmid in O139:H1 STEC strains isolated from pigs affected by Edema disease in Italy.
The virulence factors hlyA, hlyB, aidA-I, and faeE were identified on pW1316-2 plasmid and hns, traC and hha were detected both on the Shigella flexneri 2a and pB71 plasmids according to the VFDB web server (Table 1).
Table 1. Genomic characterization of the reference plasmids pW1316-2, Shigella flexneri 2a, and pB71 in the studied O139:H1 STEC genomes.
Based on the ResFinder analysis on the reference plasmids, the pW1316-2 and Shigella flexneri 2a plasmids had no known antibiotic resistance genes; and in the pB71 plasmid, we detected tet genes (tetA, tetC, and tetR) responsible for Tetracycline antibiotic resistance (Table 1).
Amid the identified plasmids, we observed functional and regulatory associations among the genes encoded on the reference plasmid derived from the Shigella flexneri 2a (Figure 7). The core of these clustering is centered around the Hha-H-NS complex, the functions of which ultimately lead to the production of α-hemolysin, an important virulence factor in ED (Details of associations are provided in tabular form in Supplementary Table 3).
Figure 7. Functional and regulatory associations of genes encoded on the Shigella flexneri 2a reference plasmid were examined using the STRING database in O139:H1 STEC strains isolated from pigs affected by Edema disease in Italy. The genes analyzed include ECs1739 (hns), ECs0125 (speE), ECs1679 (umuC), ECs4229 (dam), ECs0513 (hha), ECs2699 (dcm), ECs3765 (dsbC), ECs2316 (tus), ECs4743 (uvrD), and ECs1678 (umuD).
To explore the phylogenetic relationships and their association with plasmid characteristics, we conducted a cluster analysis using cgMLST for comparative purposes. In addition, we included 28 STEC O139:H1 genomes from various global sources, retrieved from GenBank and ENA databases (Supplementary Table 2). We then calculated the number of allelic differences between strains (Supplementary Table 4). The analysis grouped the strains into nine main clades (Figure 8). Clades 1-2 and 6-9 exhibited significantly lower allelic distances (AD) compared to the other clades, all showing fewer than 90 AD. The majority of the 28 additional strains from external studies were incorporated into clade 5, which was phylogenetically close to clade 3, with most strains showing fewer than 30 AD relative to clade 3 (Figure 8). The most genetically distant strains were found in clade 4 (Figure 8). Notably, a remarkable correlation was observed between allelic differences in the cgMLST analysis and plasmid characteristics, with strains carrying specific plasmids and replicon types clustering into particular phylogenetic clades (Figure 8).
Figure 8. Phylogenetic analysis of O139:H1 STEC strains isolated from pigs affected by Edema disease in Italy. The analysis also included 28 additional O139:H1 STEC genomes from different studies retrieved from public repositories (GenBank and ENA databases). Each entry on the phylogenetic tree shows the strain name, the clade number, the corresponding plasmid, and replicon type, with different colors representing plasmid and replicon types. The scale bar reflects the number of allelic differences.
The understanding of ED pathogenesis is crucial due to its significant impact on the swine industry (Imberechts et al., 1992; Gale and Velazquez, 2020). This study undertook a whole genome analysis of O139 STEC isolates collected from pigs with ED to elucidate the role of virulence plasmids on ED development, including adhesion, invasion, and colonization.
The predominance of the reference plasmid pW1316-2, identified through its similarity with genomes, indicates that it is a well-represented plasmid among porcine O139:H1 STEC strains, highlighting its importance in the pathogenesis of ED in pigs. This plasmid, harboring genes encoding hemolysin toxin (hlyA and hlyB) and adhesins (aidA-I, faeE), highlights its importance in the pathogenicity (Gale and Velazquez, 2020; Perrat et al., 2022). The presence of hlyA and hlyB, related with the potent hemolytic activity characteristic of STEC strains associated with ED, emphasizes the cytotoxic potential of pW1316-2 and its implication in the progression of the disease (Holland et al., 1990; Menestrina et al., 1994; Welch, 2005). Furthermore, the involvement of aidA-I and faeE in adhesion mechanisms crucial for host colonization underscores the importance of pW1316-2 in establishing STEC infections in porcine hosts (Craig, 1906; Niewerth et al., 2001). The assignment of replicon types IncFII/IncX1 to pW1316-2 reference plasmid aligns with previous reports, suggesting the stability and widespread distribution of this plasmid among O139:H1 STEC strains (Nagy et al., 1997; Perrat et al., 2022). Notably, the absence of identified resistance genes on pW1316-2 indicates that this plasmid may not contribute to the dissemination of antibiotic resistance in O139:H1 STEC populations.
The detection of similarity within the genomes to a plasmid present in Shigella flexneri 2a among porcine O139:H1 STEC strains indicates intriguing insights into the potential interplay between Shigella virulence factors and STEC pathogenesis. This plasmid, harboring genes such as hns, traC, and hha, known for their roles in gene regulation, conjugative transfer, and toxin production, respectively, presents a unique molecular landscape contributing to STEC pathogenesis (Nieto et al., 1991; Schandel et al., 1992; Müller et al., 2006). The association between hns gene and the upregulation of key virulence factors, including α-hemolysin and fimbriae, indicates its significance in driving the progression of porcine ED (Müller et al., 2006). Additionally, the presence of traC gene, responsible for the synthesis and assembly of the F pilus, highlights the potential for horizontal transfer of this plasmid among O139:H1 strains, potentially contributing to the dissemination of virulence traits via conjugation mediated by F pili (Schandel et al., 1992). Our investigation utilizing the String server has elucidated associations between the hns and hha genes, indicating their collaborative involvement in regulating the expression of the hly operon (Madrid et al., 2002). The Hha-H-NS complex is important in controlling the production of α-hemolysin, which is crucial in diseases caused by STEC, like ED in pigs (Nieto et al., 2000). The replicon IncHI1B(R27) was found in the Shigella flexneri 2a reference plasmid, a type frequently seen in such plasmids (Beloin and Dorman, 2003; Wei et al., 2003). Interestingly, there appears to be a connection between IncHI1B(R27), hns, and hha genes, as the Shigella flexneri 2a plasmid containing the IncHI1B(R27) replicon also houses the hns and hha genes (Doyle and Dorman, 2006). The absence of antimicrobial resistance genes in the Shigella flexneri 2a reference plasmid suggests that, akin to pW1316-2, it may not contribute to the emergence of antibiotic-resistant O139:H1 STEC strains (Beloin and Dorman, 2003; Wei et al., 2003). Moreover, the absence of IpaH family genes in the Shigella flexneri 2a reference plasmid aligns with the observation that ED-associated E. coli strains are typically non-invasive (Tabaran and Tabaran, 2019). Together, these findings reveal the associations and clustering involved in the pathogenicity of porcine O139:H1 STEC strains and emphasize the need for additional research to understand how the Shigella flexneri 2a plasmid interacts with STEC virulence.
The identification of similarity to the reference plasmid pB71 among porcine O139:H1 STEC strains reveals a novel dimension in the virulence and resistance profile of these pathogens. Our findings suggest that pB71, which shares virulence genes hns, traC, and hha with plasmid Shigella flexneri 2a, may contribute to the pathogenicity of STEC isolates by enhancing the manifestations of ED in pigs (Forns et al., 2005). Moreover, conjugative plasmids within the IncHI1 group play a significant role in disseminating antibiotic resistance among Salmonella enterica (Kubasova et al., 2016; Hounmanou et al., 2021). The identification of the IncFIA(HI1) replicon within pB71 suggests its potential involvement in the transmission of antibiotic resistance. This is supported by our finding of tetracycline resistance genes tetA, tetC, and tetR on this plasmid (Bryan et al., 2004; Olowe et al., 2013; Shi et al., 2021). The widespread use of tetracycline in pig farming raises worries about antibiotic resistance in the swine industry due to the rise of pB71-carrying STEC strains resistant to tetracycline (Herrero-Fresno et al., 2017; Abubakar et al., 2019; Græsbøll et al., 2019). Overall, the presence of pB71 reference plasmid underscores the complex interplay between virulence and resistance mechanisms in O139:H1 STEC strains, emphasizing the need for continued surveillance and intervention strategies to mitigate the risk of antimicrobial resistance in swine populations.
Within the various clades identified in the phylogenetic tree from the cgMLST analysis, the pW1316-2 plasmid and the IncFII replicon type were commonly found in the strains, suggesting that this plasmid may have been selected and stabilized within the O139:H1 populations (Perrat et al., 2022). Additionally, the O139:H1 STEC isolates from this study, along with others harboring extra plasmids such as Shigella flexneri 2a and pB71, formed a distinct population of STEC strains. With few exceptions, these strains grouped together in clades with small allelic differences and were separate from strains that only had the pW1316-2 plasmid. This suggests that these strains might belong to different lineages, which possibly emerged after the pW1316-2 plasmid spread within the O139 STEC population.
This study provides new data that augment our understanding of the role of virulence plasmids in the pathogenesis of ED, attributed to porcine O139:H1 STEC strains, showing how important this is for swine industry. The high prevalence of reference plasmid pW1316-2 emphasizes its crucial involvement in causing ED because it carries virulence genes, particularly those related to adhesion mechanisms important for host colonization. The detection of Shigella flexneri 2a reference plasmid presents interesting perspectives on possible associations in virulence, while the identification of reference plasmid pB71 reveals a novel dimension in virulence and resistance profiles of O139:H1 STEC strains, raising concerns about antibiotic resistance dissemination in pig farming.
The datasets presented in this study can be found in online repositories. The names of the repository/repositories and accession number(s) can be found in the article/Supplementary Material.
The animal studies were approved by Istituto Superiore di Sanità ethics committee. The studies were conducted in accordance with the local legislation and institutional requirements. Written informed consent was obtained from the owners for the participation of their animals in this study.
AN: Conceptualization, Data curation, Methodology, Software, Visualization, Writing – original draft, Writing – review & editing. FG: Conceptualization, Formal analysis, Investigation, Methodology, Software, Writing – review & editing. SM: Conceptualization, Funding acquisition, Project administration, Resources, Supervision, Writing – review & editing. MB: Investigation, Writing – review & editing.
The author(s) declare that financial support was received for the research and/or publication of this article. Funding was provided by intramural funds of Istituto Superiore di Sanità.
We wish to thank our colleagues and technicians, especially Paola Chiani at the European Union Reference Laboratory for Escherichia coli, for her assistance in this research project. We also thank the Scientific Service Team at Istituto Superiore di Sanità for performing the whole-genome sequencing of the swine strains isolated in Italy.
The authors declare that the research was conducted in the absence of any commercial or financial relationships that could be construed as a potential conflict of interest.
The author(s) declare that no Generative AI was used in the creation of this manuscript.
All claims expressed in this article are solely those of the authors and do not necessarily represent those of their affiliated organizations, or those of the publisher, the editors and the reviewers. Any product that may be evaluated in this article, or claim that may be made by its manufacturer, is not guaranteed or endorsed by the publisher.
The Supplementary Material for this article can be found online at: https://www.frontiersin.org/articles/10.3389/fcimb.2025.1528408/full#supplementary-material
ED, Edema Disease; STEC, Shiga toxin-producing Escherichia coli; stx2e, Shiga toxin 2e; NM, Non-motile; AIDA, Adhesin Involved in Diffuse Adherence; TSB, Tryptic Soy Broth.
Abubakar, R. H., Madoroba, E., Adebowale, O., Fasanmi, O. G., Fasina, F. O. (2019). Antimicrobial usage in pig production: Effects on Escherichia coli virulence profiles and antimicrobial resistance. Onderstepoort J. Vet. Res. 86, 1–11. doi: 10.4102/ojvr.v86i1.1743
Alikhan, N.-F., Petty, N. K., Ben Zakour, N. L., Beatson, S. A. (2011). BLAST Ring Image Generator (BRIG): simple prokaryote genome comparisons. BMC Genomics 12, 402. doi: 10.1186/1471-2164-12-402
Badouei, M. A., Taban, H., Nemati, A., dos Santos, L. F. (2023). Molecular serotyping of Shiga toxin-producing Escherichia coli (STEC) of animal origin in Iran reveals the presence of important non-O157 seropathotypes. Vet. Res. Forum 14, 267–274. doi: 10.30466/vrf.2022.550618.3416
Bankevich, A., Nurk, S., Antipov, D., Gurevich, A. A., Dvorkin, M., Kulikov, A. S., et al. (2012). SPAdes: A new genome assembly algorithm and its applications to single-cell sequencing. J. Comput. Biol. 19, 455–477. doi: 10.1089/cmb.2012.0021
Beloin, C., Dorman, C. J. (2003). An extended role for the nucleoid structuring protein H-NS in the virulence gene regulatory cascade of Shigella flexneri. Mol. Microbiol. 47, 825–838. doi: 10.1046/j.1365-2958.2003.03347.x
Bryan, A., Shapir, N., Sadowsky, M. J. (2004). Frequency and distribution of tetracycline resistance genes in genetically diverse, nonselected, and nonclinical Escherichia coli strains isolated from diverse human and animal sources. Appl. Environ. Microbiol. 70, 2503–2507. doi: 10.1128/AEM.70.4.2503-2507.2004
Cointe, A., Birgy, A., Mariani-Kurkdjian, P., Liguori, S., Courroux, C., Blanco, J., et al. (2018). Emerging multidrug-resistant hybrid pathotype shiga toxin–producing Escherichia coli O80 and related strains of clonal complex 165, Europe. Emerg. Infect. Dis. 24, 2262–2269. doi: 10.3201/eid2412.180272
DebRoy, C., Roberts, E., Scheuchenzuber, W., Kariyawasam, S., Jayarao, B. M. (2009). Comparison of genotypes of Escherichia coli strains carrying F18ab and F18ac fimbriae from pigs. J. Vet. Diagn. Investig. 21, 359–364. doi: 10.1177/104063870902100310
Del Fabbro, C., Scalabrin, S., Morgante, M., Giorgi, F. M. (2013). An extensive evaluation of read trimming effects on illumina NGS data analysis. PLoS One 8, e85024. doi: 10.1371/journal.pone.0085024
Denamur, E., Clermont, O., Bonacorsi, S., Gordon, D. (2021). The population genetics of pathogenic Escherichia coli. Nat. Rev. Microbiol. 19, 37–54. doi: 10.1038/s41579-020-0416-x
Doyle, M., Dorman, C. J. (2006). Reciprocal transcriptional and posttranscriptional growth-phase-dependent expression of sfh, a gene that encodes a paralogue of the nucleoid-associated protein H-NS. J. Bacteriol. 188, 7581–7591. doi: 10.1128/JB.00685-06
Fairbrother, J. M., Nadeau, É. (2019). “Colibacillosis,” in Diseases of Swine (Hoboken, New Jersey, U.S.: Wiley), 807–834. doi: 10.1002/9781119350927.ch52
Feijao, P., Yao, H.-T., Fornika, D., Gardy, J., Hsiao, W., Chauve, C., et al. (2018). MentaLiST – A fast MLST caller for large MLST schemes. Microb. Genomics 4, e000146. doi: 10.1099/mgen.0.000146
Forns, N., Baños, R. C., Balsalobre, C., Juárez, A., Madrid, C. (2005). Temperature-dependent conjugative transfer of R27: role of chromosome- and plasmid-encoded Hha and H-NS proteins. J. Bacteriol. 187, 3950–3959. doi: 10.1128/JB.187.12.3950-3959.2005
Gale, C., Velazquez, E. (2020). Oedema disease: a review of the disease and control and preventative measures. Livestock 25, 142–147. doi: 10.12968/live.2020.25.3.142
Gigliucci, F., van Hoek, A. H. A. M., Chiani, P., Knijn, A., Minelli, F., Scavia, G., et al. (2021). Genomic Characterization of hlyF -positive Shiga Toxin–Producing Escherichia coli, Italy and the Netherlands 2000–2019. Emerg. Infect. Dis. 27, 853–861. doi: 10.3201/eid2703.203110
Græsbøll, K., Larsen, I., Clasen, J., Birkegård, A. C., Nielsen, J. P., Christiansen, L. E., et al. (2019). Effect of tetracycline treatment regimens on antibiotic resistance gene selection over time in nursery pigs. BMC Microbiol. 19, 269. doi: 10.1186/s12866-019-1619-z
Herrero-Fresno, A., Zachariasen, C., Nørholm, N., Holm, A., Christiansen, L. E., Olsen, J. E. (2017). Effect of different oral oxytetracycline treatment regimes on selection of antimicrobial resistant coliforms in nursery pigs. Vet. Microbiol. 208, 1–7. doi: 10.1016/j.vetmic.2017.07.005
Holland, I. B., Kenny, B., Blight, M. (1990). Haemolysin secretion from E coli. Biochimie 72, 131–141. doi: 10.1016/0300-9084(90)90138-7
Hounmanou, Y. M. G., Bortolaia, V., Dang, S. T. T., Truong, D., Olsen, J. E., Dalsgaard, A. (2021). ESBL and ampC β-lactamase encoding genes in E. coli from pig and pig farm workers in Vietnam and their association with mobile genetic elements. Front. Microbiol. 12. doi: 10.3389/fmicb.2021.629139
Imberechts, H., De Greve, H., Lintermans, P. (1992). The pathogenesis of edema disease in pigs. A review. Vet. Microbiol. 31, 221–233. doi: 10.1016/0378-1135(92)90080-D
Joensen, K. G., Tetzschner, A. M. M., Iguchi, A., Aarestrup, F. M., Scheutz, F. (2015). Rapid and easy in silico serotyping of Escherichia coli isolates by use of whole-genome sequencing data. J. Clin. Microbiol. 53, 2410–2426. doi: 10.1128/JCM.00008-15
Junier, T., Zdobnov, E. M. (2010). The Newick utilities: high-throughput phylogenetic tree processing in the Unix shell. Bioinformatics 26, 1669–1670. doi: 10.1093/bioinformatics/btq243
Keshmiri, M. A., Nemati, A., Badouei, A. M., Tamai, A. I., Salehi, Z. T. (2022). Clonal relatedness and antimicrobial susceptibility of Salmonella serovars isolated from humans and domestic animals in Iran: a one health perspective. Iran. J. Vet. Res. 23, 104–110. doi: 10.22099/IJVR.2022.40594.5881
Knijn, A., Michelacci, V., Orsini, M., Morabito, S. (2020). Advanced research infrastructure for experimentation in genomicS (ARIES): A lustrum of galaxy experience. bioRxiv 2020, 5.14.095901. doi: 10.1101/2020.05.14.095901
Kubasova, T., Cejkova, D., Matiasovicova, J., Sekelova, Z., Polansky, O., Medvecky, M., et al. (2016). Antibiotic resistance, core-genome and protein expression in IncHI1 plasmids in salmonella typhimurium. Genome Biol. Evol. 8, 1661–1671. doi: 10.1093/gbe/evw105
Liu, B., Zheng, D., Zhou, S., Chen, L., Yang, J. (2022). VFDB 2022: a general classification scheme for bacterial virulence factors. Nucleic Acids Res. 50, D912–D917. doi: 10.1093/nar/gkab1107
Llarena, A., Ribeiro-Gonçalves, B. F., Nuno Silva, D., Halkilahti, J., MaChado, M. P., Da Silva, M. S., et al. (2018). INNUENDO: A cross-sectoral platform for the integration of genomics in the surveillance of food-borne pathogens. EFSA Support. Publ. 15, 1498E. doi: 10.2903/sp.efsa.2018.EN-1498
Müller, C. M., Dobrindt, U., Nagy, G., Emödy, L., Uhlin, B. E., Hacker, J. (2006). Role of histone-like proteins H-NS and StpA in expression of virulence determinants of uropathogenic Escherichia coli. J. Bacteriol. 188, 5428–5438. doi: 10.1128/JB.01956-05
Madrid, C., Nieto, J. M., Paytubi, S., Falconi, M., Gualerzi, C. O. (2002). Temperature- and H-NS-dependent regulation of a plasmid-encoded virulence operon expressing Escherichia coli hemolysin. J. Bacteriol. 184, 5058–5066. doi: 10.1128/JB.184.18.5058-5066.2002
Marques, L. R. M., Peiris, J. S. M., Cryz, S. J., O’Brien, A. D. (1987). Escherichia coli strains isolated from pigs with edema disease produce a variant of Shiga-like toxin II. FEMS Microbiol. Lett. 44, 33–38. doi: 10.1111/j.1574-6968.1987.tb02237.x
Menestrina, G., Moser, C., Pellet, S., Welch, R. (1994). Pore-formation by Escherichia coli hemolysin (HlyA) and other members of the RTX toxins family. Toxicology 87, 249–267. doi: 10.1016/0300-483X(94)90254-2
Moxley, R. A. (2000). Edema disease. Vet. Clin. North Am. Food Anim. Pract. 16, 175–185. doi: 10.1016/S0749-0720(15)30142-0
Nagy, B., Whipp, S. C., Imberechts, H., Bertschinger, H. U., Dean-Nystrom, E. A., Casey, T. A., et al. (1997). Biological relationship between F18ab and F18ac fimbriae of enterotoxigenic and verotoxigenicEscherichia colifrom weaned pigs with oedema disease or diarrhoea. Microb. Pathog. 22, 1–11. doi: 10.1006/mpat.1996.0085
Nemati, A., Askari Badouei, M., Hashemi Tabar, G., Morabito, S., Dadvar, A. (2024). Molecular and in silico analyses for detection of Shiga toxin-producing Escherichia coli (STEC) and highly pathogenic enterohemorrhagic Escherichia coli (EHEC) using genetic markers located on plasmid, O Island 57 and O Island 71. BMC Vet. Res. 20, 413. doi: 10.1186/s12917-024-04251-0
Nieto, J. M., Carmona, M., Bolland, S., Jubete, Y., de la Cruz, F., Juárez, A. (1991). The hha gene modulates haemolysin expression in Escherichia coli. Mol. Microbiol. 5, 1285–1293. doi: 10.1111/j.1365-2958.1991.tb01902.x
Nieto, J. M., Madrid, C., Prenafeta, A., Miquelay, E., Balsalobre, C., Carrascal, M., et al. (2000). Expression of the hemolysin operon in Escherichia coli is modulated by a nucleoid-protein complex that includes the proteins Hha and H-NS. Mol. Gen. Genet. MGG 263, 349–358. doi: 10.1007/s004380051178
Niewerth, U., Frey, A., Voss, T., Bouguénec, L., Baljer, G., Franke, S., et al. (2001). The AIDA autotransporter system is associated with F18 and Stx2e in Escherichia coli isolates from pigs diagnosed with edema disease and postweaning diarrhea. Clin. Diagn. Lab. Immunol. 8, 143–149. doi: 10.1128/CDLI.8.1.143-149.2001
Olowe, O. A., Idris, O. J., Taiwo, S. S. (2013). Prevalence of TET genes mediating tetracycline resistance in Escherichia coli clinical isolates in Osun State, Nigeria. Eur. J. Microbiol. Immunol. 3, 135–140. doi: 10.1556/EuJMI.3.2013.2.7
Perrat, A., Branchu, P., Decors, A., Turci, S., Bayon-Auboyer, M.-H., Petit, G., et al. (2022). Wild boars as reservoir of highly virulent clone of hybrid shiga toxigenic and enterotoxigenic Escherichia coli responsible for edema disease, France. Emerg. Infect. Dis. 28, 382–393. doi: 10.3201/eid2802.211491
Schandel, K. A., Muller, M. M., Webster, R. E. (1992). Localization of TraC, a protein involved in assembly of the F conjugative pilus. J. Bacteriol. 174, 3800–3806. doi: 10.1128/jb.174.11.3800-3806.1992
Seemann, T. (2014). Prokka: rapid prokaryotic genome annotation. Bioinformatics 30, 2068–2069. doi: 10.1093/bioinformatics/btu153
Shi, Y., Zhang, Y., Wu, X., Zhang, H., Yang, M., Tian, Z. (2021). Potential dissemination mechanism of the tetC gene in Aeromonas media from the aerobic biofilm reactor under oxytetracycline stresses. J. Environ. Sci. 105, 90–99. doi: 10.1016/j.jes.2020.12.038
Silva, M., MaChado, M. P., Silva, D. N., Rossi, M., Moran-Gilad, J., Santos, S., et al. (2018). chewBBACA: A complete suite for gene-by-gene schema creation and strain identification. Microb. Genomics 4, e000166. doi: 10.1099/mgen.0.000166
Szklarczyk, D., Kirsch, R., Koutrouli, M., Nastou, K., Mehryary, F., Hachilif, R., et al. (2023). The STRING database in 2023: protein–protein association networks and functional enrichment analyses for any sequenced genome of interest. Nucleic Acids Res. 51, D638–D646. doi: 10.1093/nar/gkac1000
Tabaran, F., Tabaran, A. (2019). Edema disease of swine: a review of the pathogenesis. Porcine Res. 9, 7–14. Available online at: http://www.porc.bioflux.com.ro/7 (Accessed October 1, 2024).
Tomeh, R., Nemati, A., Hashemi Tabar, G., Tozzoli, R., Badouei, M. A. (2024). Antimicrobial resistance, β-lactamase genotypes, and plasmid replicon types of Shiga toxin-producing Escherichia coli isolated from different animal hosts. J. Appl. Microbiol. 135, lxae059. doi: 10.1093/jambio/lxae059
Tseng, M., Fratamico, P. M., Manning, S. D., Funk, J. A. (2014). Shiga toxin-producing Escherichia coli in swine: the public health perspective. Anim. Heal. Res. Rev. 15, 63–75. doi: 10.1017/S1466252313000170
Wei, J., Goldberg, M. B., Burland, V., Venkatesan, M. M., Deng, W., Fournier, G., et al. (2003). Complete genome sequence and comparative genomics of shigella flexneri serotype 2a strain 2457T. Infect. Immun. 71, 2775–2786. doi: 10.1128/IAI.71.5.2775-2786.2003
Welch, R. A. (2005). The Escherichia coli hemolysin. EcoSal Plus 1, 10–1128. doi: 10.1128/ecosalplus.8.7.2
Wirth, T., Falush, D., Lan, R., Colles, F., Mensa, P., Wieler, L. H., et al. (2006). Sex and virulence in Escherichia coli : an evolutionary perspective. Mol. Microbiol. 60, 1136–1151. doi: 10.1111/j.1365-2958.2006.05172.x
Keywords: plasmid, O139:H1, Shiga toxin-producing Escherichia coli, STEC, Edema disease
Citation: Nemati A, Gigliucci F, Morabito S and Badouei MA (2025) Virulence plasmids in edema disease: Insights from whole-genome analysis of porcine O139:H1 Shiga toxin-producing Escherichia coli (STEC) strains. Front. Cell. Infect. Microbiol. 15:1528408. doi: 10.3389/fcimb.2025.1528408
Received: 14 November 2024; Accepted: 04 March 2025;
Published: 20 March 2025.
Edited by:
Muddassar Hameed, Virginia Tech, United StatesReviewed by:
Jorge Blanco, University of Santiago de Compostela, SpainCopyright © 2025 Nemati, Gigliucci, Morabito and Badouei. This is an open-access article distributed under the terms of the Creative Commons Attribution License (CC BY). The use, distribution or reproduction in other forums is permitted, provided the original author(s) and the copyright owner(s) are credited and that the original publication in this journal is cited, in accordance with accepted academic practice. No use, distribution or reproduction is permitted which does not comply with these terms.
*Correspondence: Ali Nemati, YmFjLmZ1bUBnbWFpbC5jb20=
Disclaimer: All claims expressed in this article are solely those of the authors and do not necessarily represent those of their affiliated organizations, or those of the publisher, the editors and the reviewers. Any product that may be evaluated in this article or claim that may be made by its manufacturer is not guaranteed or endorsed by the publisher.
Research integrity at Frontiers
Learn more about the work of our research integrity team to safeguard the quality of each article we publish.