- 1Clinical Microbiology Laboratory, Shanghai Center for Clinical Laboratory, Shanghai, China
- 2Laboratory Medicine Center, Second Affiliated Hospital, Nanjing Medical University, Nanjing, China
- 3Department of Laboratory Medicine, Shanghai Children’s Medical Center, Shanghai Jiao Tong University School of Medicine, Shanghai, China
- 4Department of Laboratory Medicine, Zhujiang Hospital, Southern Medical University, Guangzhou, China
Introduction: Methicillin-resistant Staphylococcus aureus (MRSA) exhibits diverse genotypes with varying virulence and resistance profiles, particularly in the context of bloodstream infections (BSI). This study investigates the prevalence of the sasX, mupA, and qacA/B genes among MRSA isolates from bloodstream infections in southern China and analyzes their genetic relatedness.
Methods: A polymerase chain reaction (PCR) assay was developed to detect the presence of the sasX gene, which is associated with nasal colonization, immune evasion, and virulence, the mupirocin resistance gene mupA, and the chlorhexidine tolerance gene qacA/B in a total of 77 MRSA isolates. Multilocus sequence typing (MLST) was performed to determine the sequence types (STs) and assess the genetic relatedness of the isolates. The resistance of these strains to 16 antibiotics was also analyzed. The distribution of these genes and their association with epidemic STs were analyzed.
Results: A total of 26 STs were identified, with notable prevalence in five epidemic clones: ST59, ST5, and ST764. The prevalence of the sasX, mupA, and qacA/B genes across all isolates was 23.4%, 33.8%, and 79.2%, respectively. Specifically, the frequency of the sasX gene was highest in ST59 (29.4%), ST239 (100%), and ST764 (37.5%); mupA was most prevalent in ST5 (66.7%), ST59 (17.6%), ST764 (37.5%), and ST15 (100%); qacA/B was predominantly found in ST59 (88.2%), ST5 (66.7%), ST398 (85.7%), ST764 (50.0%), and ST239 (100%). The gene distribution patterns revealed that sasX+ qacA/B+ mupA+ strains were closely associated with epidemic clones ST6290 and ST88, whereas sasX+ qacA/B+ mupA- strains were linked to ST59, ST239, and ST764.
Discussion: Notably, forty-seven (61%) MRSA BSI strains were multidrug-resistant, with the majority exhibiting resistance to penicillin, erythromycin, and clindamycin. Major MRSA clones in southern China include ST59, ST5, ST764, and ST398. In this study, sasX, mupA and qacA/B genes were present in the MRSA isolates, with the mupA gene being the most prevalent. Variations in the prevalence of virulence and resistance genes among these epidemic strains underscore the need for targeted infection control measures. These findings contribute to a better understanding of the genetic epidemiology of MRSA in the region, facilitating the development of effective prevention and control strategies for BSI.
1 Introduction
Staphylococcus aureus, a major Gram-positive coagulase-positive pathogen, is a spherical bacterium approximately 1 μm in diameter that forms grape-like clusters (Lakhundi and Zhang, 2018). This bacterium possesses a range of virulence factors and has the capability to develop resistance to most antibiotics and antiseptics, further compounded by the continual emergence of new clones. The clinical use of methicillin has led to the rise of methicillin-resistant S. aureus (MRSA). MRSA can adapt its genetic content and expression to generate new strains with enhanced virulence and colonization abilities. As a highly virulent and challenging pathogen, MRSA is a prevalent cause of both community-acquired and hospital-associated infections (Lakhundi and Zhang, 2018). Colonization with MRSA is a critical step in the pathogenesis of active infection and a key factor in the epidemiology of MRSA infections. Colonizing strains can act as endogenous reservoirs for overt clinical infections or spread to other patients, potentially leading to serious bacterial infections (Abad et al., 2013).
The novel Staphylococcus aureus cell wall-anchored protein gene, sasX, plays a critical role in enhancing nasal colonization, immune evasion, and overall virulence (Li et al., 2012). In addition to its role in nasal colonization, sasX is involved in biofilm formation and mechanisms of immune evasion. MRSA strains carrying sasX gene have been identified as potential causes of severe diseases, including pulmonary infections and abscess formation (Alam et al., 2022). The SasX protein, which is carried on the φSPβ-like prophage, was initially identified in the sequence type (ST) 239 TW20 isolated in the UK (Holden et al., 2010; Baines et al., 2015; Harris et al., 2010); However, sasX has also been detected in other STs, suggesting its association with diverse phenotypic expressions (Li et al., 2012; Holden et al., 2010; Wang et al., 2014).
Mupirocin, a topical antibiotic produced by Pseudomonas fluorescens, is used for the decolonization of both methicillin-susceptible S. aureus (MSSA) and MRSA in patients and healthcare personnel. It exhibits high efficacy against staphylococci, streptococci, and certain Gram-negative bacteria, including Haemophilus influenzae and Neisseria gonorrhoeae (Sutherland et al., 1985). Mupirocin is commonly employed to treat local skin and soft tissue infections caused by S. aureus and streptococcal species. Resistance to mupirocin is associated with the presence of the plasmid-encoded mupA gene, which is linked to high-level mupirocin resistance and therapeutic failure (Udo et al., 2001; Simor et al., 2007; Chaves et al., 2004; Cadilla et al., 2011). High-level mupirocin resistance, defined as a minimum inhibitory concentration (MIC) ≥512 mg/L, is mediated by the mupA gene that encodes an alternate isoleucyl-tRNA synthetase (IleRS-2) (Hetem et al., 2016), located on mobile genetic elements (Hetem and Bonten, 2013; Hodgson et al., 1994).
Staphylococcus aureus strains have shown decreased sensitivity to chlorhexidine, an antiseptic solution used globally since the 1950s (Milstone et al., 2008). This resistance is attributed to the presence of the qacA/B genes, which encodes proton-motive force-dependent export pumps (Mayer et al., 2001). The qacA/B genes are typically located on multiresistance plasmids and can co-exist with antimicrobial resistance genes, contributing to the persistence and survival of qacA/B-positive MRSA strains (Sidhu et al., 2002).The presence of the qacA/B genes is associated with elevated minimum bactericidal concentrations of chlorhexidine and failures in MRSA decolonization protocols (Warren et al., 2016).
Multilocus sequence typing (MLST) has been employed to study the genetic relatedness among MRSA isolates from clinical specimens. Among 119 European MRSA isolates, only two harbored sasX, and both were of the ST239 type (De Backer et al., 2019). MRSA ST239 is known for producing exotoxins that cause a range of severe infections (Abimanyu et al., 2012). The recent dissemination of sasX from ST239 to other invasive clones indicates that this ST shift may be a significant factor driving the MRSA epidemic in Asia (Li et al., 2012).
Detecting the sasX, qacA/B, and mupA genes, along with identifying circulating MRSA strains that carry these specific genes, has significant implications for nasal decolonization of S. aureus, reducing the infection rate, and guiding rational drug use in clinical practice. It provides crucial insights for optimizing therapeutic strategies and informs the development of more effective decontamination protocols currently in place.
2 Materials and methods
2.1 Collection and detection of MRSA isolates
The sample from three tertiary first-class hospitals, consisting of 77 non-duplicated convenience clinical MRSA isolates from patients in southern China collected between 2012 and 2020, provides a snapshot of the MRSA sequence type population in China. These strains were isolated from hospitalized patients with BSI. The identification of the MRSA isolates was performed based on colony morphology, antibiotic susceptibility testing, and mecA gene detection by PCR, as previously described (Kondo et al., 2007). Bacterial identification and oxacillin resistance (susceptibility testing) were performed using the Vitek 2 Compact Automated Microbiology System (BioMérieux, Durham, NC, USA) following the manufacturer’s instructions in a clinical microbiology laboratory. A total of 16 drugs were tested: penicillin, erythromycin, oxacillin, clindamycin, ciprofloxacin, moxifoxacin, levofloxacin, tetracycline, gentamicin, rifampicin, trimethoprim–sulfamethoxazole, quinupristin/dalfopristin, linezolid, tigecycline, vancomycin and cefoxitin. Results were interpreted according to the recommendations and definitions of the Clinical and Laboratory Standards Institute [CLSI]. S. aureus ATCC 29213 was used for quality control in antimicrobial susceptibility testing. All MRSA strains were stored at −80°C until use.
2.2 Genomic DNA extraction
All isolates were cultured on blood agar (CHROMagar) and incubated overnight at 37°C. Bacterial DNA was isolated using a TIANamp Bacteria DNA Kit (TIANGEN, Beijing, China) according to the manufacturer’s instructions and used as the template for all PCRs.
2.3 Gene typing
MLST was performed on all isolates by amplifying and sequencing seven internal housekeeping gene fragments—arcC, aroE, glpF, gmk, pta, tpi, and yqi—each approximately 450 bp in length (Enright et al., 2000), using the Mastercycler Nexus Gradient Thermal Cycler (Eppendorf). The sequence profile and ST of each allele were determined according to the MLST database (http://saureus.mlst.net). The allelic profiles were assigned by comparing the sequences at each locus with those of the known alleles in the S. aureus MLST database, and the profiles were then defined as the STs. Each sequence contig was submitted to the Staphylococcus aureus webpage on PubMLST (https://pubmlst.org/organisms/staphylococcus-aureus) for allelic profiles and ST characterization. In our study, primers were designed manually and obtained commercially. All oligonucleotide primers used were synthesized by Sangon Biotech (Shanghai, China).
2.4 Analysis of phylogenetic
GrapeTree (Zhou et al., 2018) implements a novel minimum spanning tree algorithm (MSTree V2) to reconstruct genetic relationships from allelic profiles as a minimum spanning tree. Distances between isolates are calculated based on the number of shared multilocus sequence typing (MLST) alleles. Additionally, the Interactive Tree of Life (iTOL) (Letunic and Bork, 2021) generates neighbor-joining phylogenies for visualization, incorporating annotations for virulence factors, MLST, and the geographic locations of isolates. Both the GrapeTree and iTOL plugins are hosted on the PubMLST website (https://pubmlst.org/).
2.5 PCR assay for gene detection
Detection of the sasX, mupA and qacA/B genes was conducted using published protocols (Monecke et al., 2014; Noguchi et al., 2005; Yun et al., 2003).The specific primers used for the identification of the respective targets —sasX, mupA, and qacA/B genes are listed in Table 1.
The sasX, mupA, and qacA/B genes were amplified using a S1000 thermal cycler (Bio-Rad, Hercules, California, USA) under the following conditions: 5 minutes of denaturation at 94°C, followed by 32 cycles consisting of 45 seconds at 94°C, 30 seconds at 56°C, and 1 minute at 72°C, with a final 7-minute elongation step at 72°C. The amplified PCR products were analyzed by agarose gel electrophoresis on a 1.2% gel in 1×TAE buffer at 110V for 42 minutes, then stained with ethidium bromide and exposed to UV light for visualization. If the bands were not completely clear, the experiment was repeated to confirm reproducibility. The gel was also stained with Florosafe (Apical Scientific Sdn Bhd, Selangor, Malaysia) and visualized using a gel imaging system (AlphaImager; Alpha Innotec, Kasendorf, Germany). The PCR products were sent for sequencing analysis (Apical Scientific Sdn Bhd, Selangor, Malaysia), which was performed bidirectionally. In addition to the genes associated with MRSA decolonization protocols, we also tested adhesion genes (sdrC, sdrD, sdrE, icaA, and clfA), as previously described (Peacock et al., 2002; Campbell et al., 2008). All primers are listed in Supplementary Table S2.
2.6 Statistical analysis
Statistical analyses were performed using GraphPad Prism 9.0 (GraphPad Software Inc.; San Diego, CA, USA) and IBM SPSS Statistics (SPSS Inc., Chicago, IL, USA). All graphs related to the study were constructed and described in terms of percentages and frequencies. Pearson’s chi-square test or Fisher’s exact test was used to determine whether differences in the frequency of sasX, mupA, and qacA/B genes exist among isolates with different ST types. Statistical significance was set at a P-value of <0.05. The descriptive data were presented as percentages for the categorical data.
3 Results
A total of 77 MRSA isolates were analyzed, revealing the presence of sasX, mupA, and qacA/B genes across various STs, as detailed in Table 2. PCR results indicated that 18 isolates (23.4%) were positive for the sasX gene, 26 isolates (33.8%) for the mupA gene, and 61 isolates (79.2%) for the qacA/B genes. The predominant STs with sasX were ST59 (5 isolates, 6.5%), ST239 (3 isolates, 3.9%), and ST764 (3 isolates, 3.9%). For mupA, the most common STs were ST5 (6 isolates, 7.8%), ST59 (3 isolates, 3.9%), and ST764 (3 isolates, 3.9%). The most frequent STs for qacA/B were ST59 (15 isolates, 19.5%), ST5 (6 isolates, 7.8%), ST398 (6 isolates, 7.8%), and ST764 (4 isolates, 5.2%). Co-existence of sasX and qacA/B genes was observed exclusively in 6.5% (n = 5) of MRSA ST59, and in 3.9% (n = 3) of MRSA ST239 and ST764. The simultaneous presence of sasX, qacA/B, and mupA genes occurred in 1.3% (n = 1) of MRSA ST6290 and 1.3% (n = 1) of MRSA ST88 (see Table 3).
The proportional distribution of CA-MRSA and HA-MRSA strains across various specimens is shown in Figure 1. No significant differences were observed in the detection rates of individual genes between hospital-acquired and community-acquired MRSA. The detection frequencies of the three genes in adults and children are presented in Figure 2, where the detection rate of mupA in adults was higher than that in children (P < 0.001).
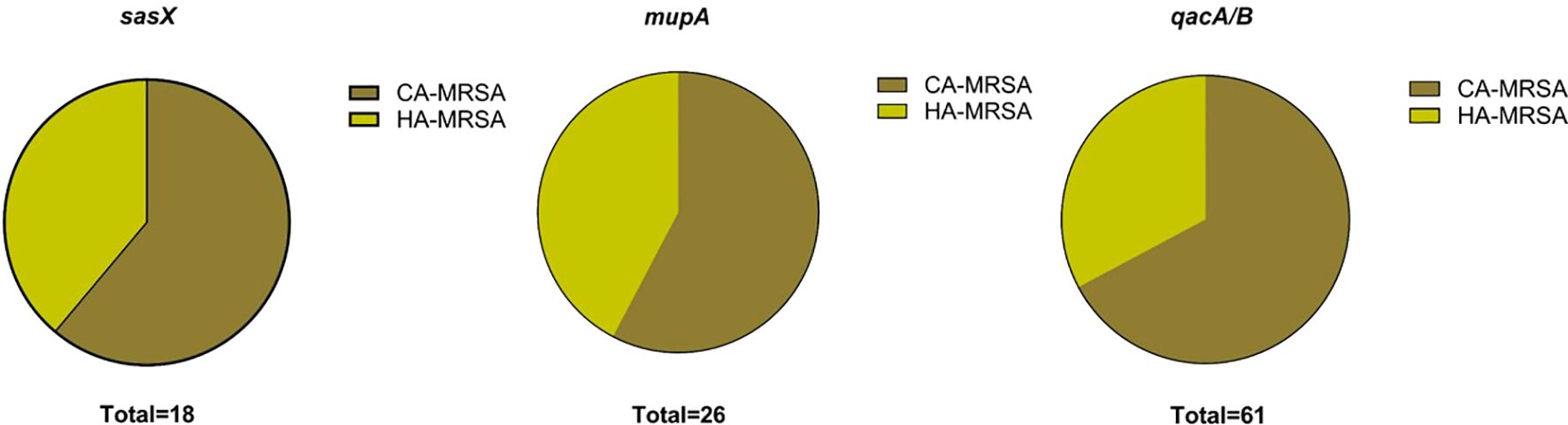
Figure 1. Charts show the distribution of HA-MRSA and CA-MRSA based on the presence of the respective target genes: sasX, mupA, and qacA/B.
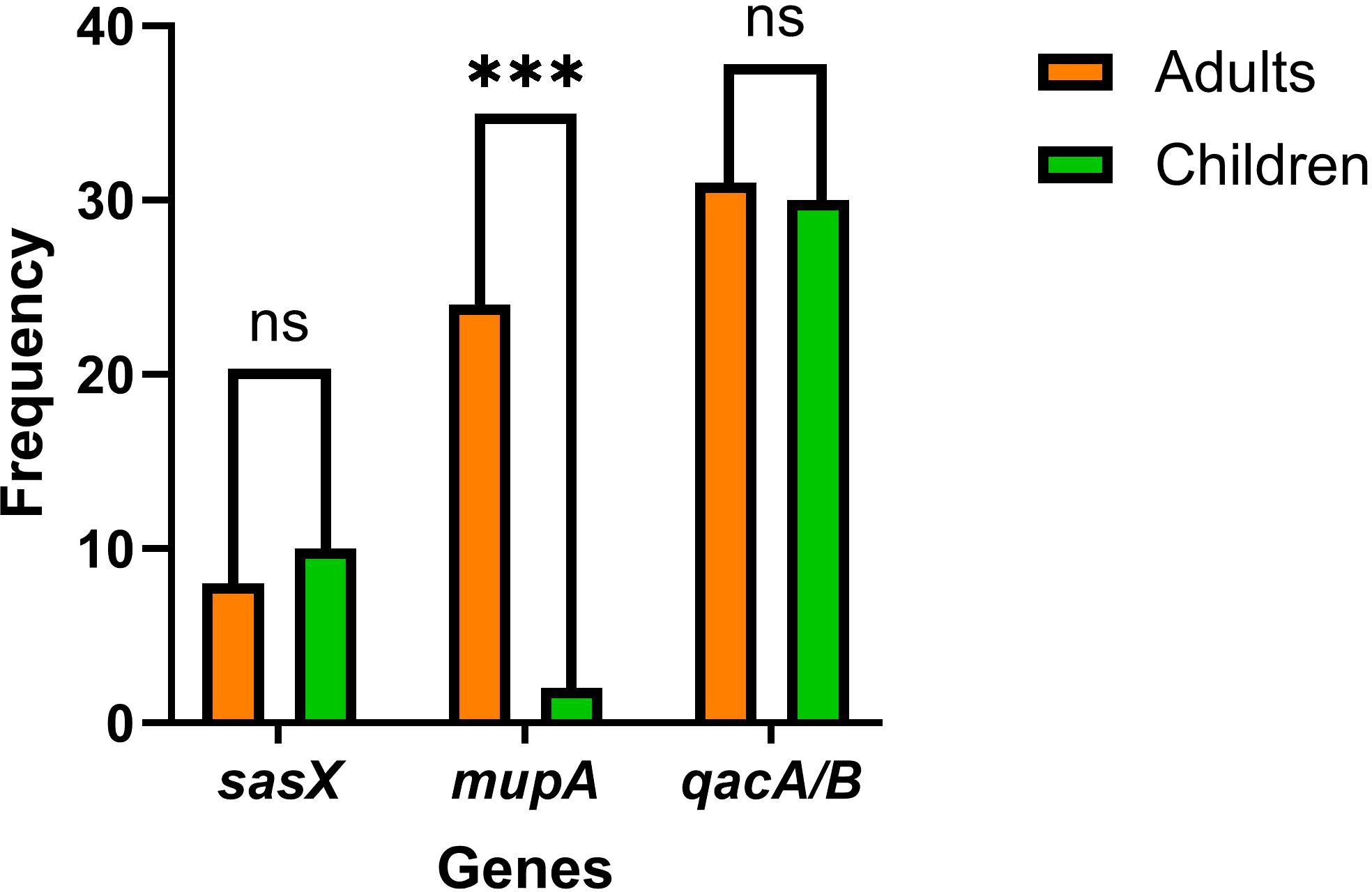
Figure 2. The graph shows the frequencies of sasX, qacA/B, and mupA genes in MRSA isolates from adults and children. *** indicates p < 0.001, and “ns (no significance)”, indicates p > 0.05.
The icaA and clfA genes were the most frequently detected adhesion genes, with detection rates of 100% and 96.1%, respectively. However, the detection rate of sdrD was only 64.9% (Supplementary Table S1). The sdrC, sdrD, sdrE, icaA, and clfA genes were detected in all ST1-MRSA isolates and most ST5-MRSA isolates. Relatively low detection rates of sdrD were observed among ST59-MRSA and ST398-MRSA isolates. Only two MRSA isolates from ST398 harbored the sdrE gene, as shown in Supplementary Table S1.
The antimicrobial resistance profiles of 77 S. aureus isolates are displayed in Supplementary Figure S1. All strains were resistant to penicillin, oxacillin, and cefoxitin, but fully susceptible to several tested antibiotics, including quinupristin/dalfopristin, linezolid, vancomycin, and tigecycline. Notably, forty-seven (61%) S. aureus BSI strains were multidrug-resistant, with the majority being resistant to penicillin, oxacillin, erythromycin, and clindamycin.
Overall, 26 STs were identified among the MRSA strains. Phylogenetic analysis revealed significant genetic variability among the MRSA isolates, as shown in Figure 3. Three predominant clones—ST59, ST5, and ST764—were identified. The phylogenetic tree demonstrated that MRSA isolates obtained from clinical blood culture samples were clonal. The phylogram, reflecting the evolutionary relationships among clinical epidemic MRSA isolates from southern China (n = 77), is based on MLST homology (Figure 3).
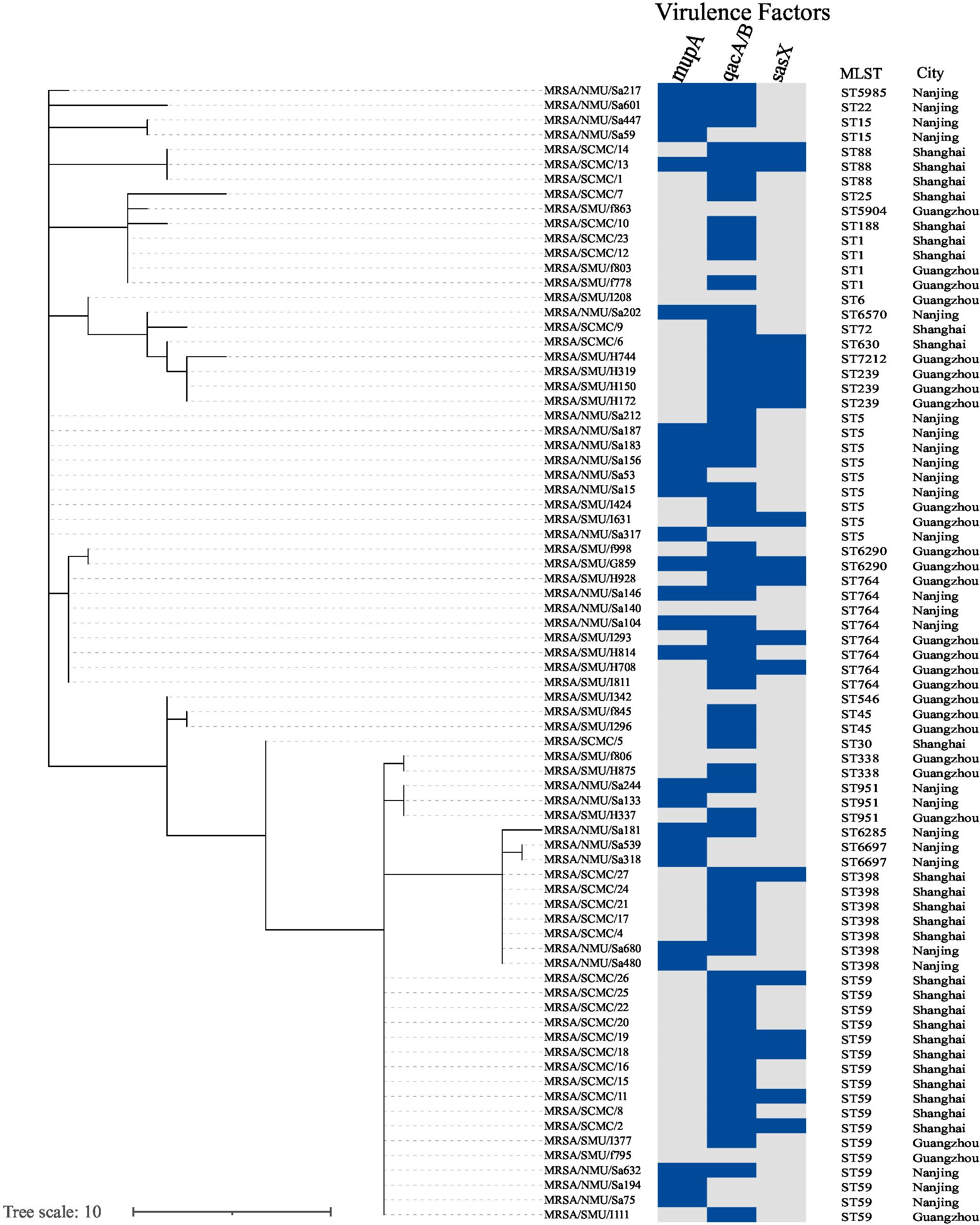
Figure 3. Phylogenetic tree shows the genetic relatedness of isolates (n = 77). Phylogenetic tree inferred by MSTree V2. The tree was constructed using MLST reference genomes (n = 77) and was labeled with the metadata using iTOL v.6. Columns represent, respectively, the isolate ID, sequence type (ST), hospital city location. The presence of various antimicrobial resistance genes in the genomes is indicated as a blue box.
4 Discussion
MRSA remains one of the most successful contemporary pathogens, demonstrating remarkable adaptability as both a commensal organism and a leading cause of severe infections in healthcare and community settings. It is responsible for a broad spectrum of clinical manifestations, including bacteremia, endocarditis, skin and soft tissue infections, bone and joint infections, and hospital-acquired infections (Turner et al., 2019). MRSA colonization significantly increases the risk of developing ventilator-associated pneumonia following intubation, particularly in intensive care units, and is associated with elevated mortality rates among hospitalized patients (Percival et al., 2015). Patients who have MRSA nasal colonization are at high risk of developing subsequent infections. The epidemiology of MRSA is characterized by the serial emergence of epidemic strains, posing a persistent clinical threat with consistently high morbidity and mortality rates (Turner et al., 2019). Analyzing the distribution of sasX, qacA/B, and mupA genes in MRSA clones is crucial for understanding the pathogen’s evolution and dissemination across different regions.
The sasX gene, although rarely detected in clinical strains, has been identified at varying frequencies across different studies. Nakaminami et al. reported a sasX prevalence of only 0.3% among isolated strains (Nakaminami et al., 2017), while Kong et al. detected it in 5.8% of their isolates (Kong et al., 2018). Surprisingly, sasX was relatively frequently identified in the study by Zieliński W et al., with 69% of sasX-positive isolates (75 out of 108) being S. aureus. A study conducted in a Malaysian hospital detected the sasX gene in 14.9% of cases (Alam et al., 2022), with one case involving ST4649 and the remainder (n = 13) exclusively from ST239 (Alam et al., 2022). This finding is consistent with a previous study (Zarizal et al., 2018). Another study conducted in a Chinese hospital found sasX in 36.7% of cases (Song et al., 2013). Despite the common association of the sasX gene with ST239, Nair et al. reported in 2013 that the sasX gene was not detected in any MRSA isolates, including those of ST239, which may reflect its low prevalence in the Mongolian hospital studied (Nair et al., 2013). Moreover, recent evidence suggests that sasX has spread from ST239 to other invasive clones across different sequence types (Li et al., 2012). In our study, 23.4% (n = 18) of MRSA isolates carried the sasX gene. Among these, five were from ST59 (5/17, 29.4%), three from ST239 (3/3, 100%), and three from ST764 (3/8, 37.5%) strains. Notably, all three ST239 strains in this study contained the sasX gene, making ST239 the dominant lineage among sasX-positive isolates, with a 100% detection rate within this sequence type. The sasX gene, a colonization-virulence factor, likely contributes to the success of MRSA ST239 in Asia (De Backer et al., 2019). Our data underscore the dissemination of sasX to non-ST239 sequence types, highlighting its broader impact on MRSA epidemiology.
Additionally, antiseptic resistance genes qacA/B were detected in 79.2% (n = 61) of MRSA isolates in our study. This finding aligns with the report by Shamsudin MN et al., who in 2012 observed a high prevalence of qacA/B-positive MRSA at 83.3% (50 out of 60) in Malaysian isolates (Shamsudin et al., 2012). However, a more recent study from Malaysia reported a much lower prevalence, with qacA/B genes detected in only 7.4% (n = 7) of MRSA isolates. These findings, including those from our study and others, suggest significant regional differences in the prevalence of qacA/B genes among MRSA isolates. Our results also indicate a notable association between qacA/B genes and specific sequence types. For example, qacA/B genes were frequently present in ST59 (15/17, 88.2%), ST5 (6/9, 66.7%), ST398 (6/7, 85.7%), ST764 (4/8, 50.0%), and ST239 (3/3, 100%) isolates. This observation is consistent with a report by Lu et al., who found that 88.0% (22/25) of ST239 MRSA isolates were qacA/B-positive (Lu et al., 2015). Similarly, Ho et al. reported a high frequency of qacA/B genes (88.9%) in ST239 isolates (Ho and Branley, 2012). Conversely, Kong H et al. observed that the highest incidence of qacA/B genes was in ST5 clones (34.1%), which were the predominant clone in their MRSA isolates from the region (Kong et al., 2018). In Taiwan, several studies screening MRSA isolates from various hospitals over different periods consistently found that the genotypic resistance rate to chlorhexidine, attributed to the presence of qacA/B genes, ranged from 35.4% to 55.4% (Sheng et al., 2009; Wang et al., 2008; Lee et al., 2022). Among different sequence types, ST239 MRSA isolates exhibited the highest resistance to both chlorhexidine and other antimicrobial agents (Sheng et al., 2009; Lee et al., 2022), likely due to the widespread use of chlorhexidine in hospital settings (Wang et al., 2008).
Previous studies have also reported an increased incidence of mupirocin resistance in MRSA, leading to the failure of decolonization treatments (Lu et al., 2015). Several MRSA decolonization studies have shown that 6.9%–10.9% of MRSA isolates were mupirocin-resistant, and all carried the mupA gene (Warren et al., 2016; Hayden et al., 2016; Muñoz-Gallego et al., 2016). Notably, no mupirocin-resistant MRSA isolates were reported in Taiwan before 2010 (Chen et al., 2014); however, by later years, 91.5% of MRSA isolates harbored the mupA gene, despite mupirocin not being routinely used for MRSA decolonization in the country (Lee et al., 2022).
In our study, the mupA gene was detected in 26 (33.8%) of the MRSA isolates. This gene was predominantly found in ST5 (6/9, 66.7%), ST59 (3/17, 17.6%), ST764 (3/8, 37.5%), and ST15 (2/2, 100%) isolates. Notably, all ST15 isolates exhibited a 100% detection rate for the mupA gene, whereas none of the ST239 isolates harbored the mupA gene. This contrasts with an earlier Malaysian study, which reported that 70% (11 out of 16) of ST239 isolates were positive for the mupA gene (Ghasemzadeh-Moghaddam et al., 2014). In contrast, studies by Alam NNNB et al. and Nejabat et al. found no mupA gene presence in any of their MRSA isolates (Alam et al., 2022). Our study underscores the importance of MLST data analysis in assessing the genetic variability of MRSA isolates, investigating the association between the sasX, qacA/B, and mupA genes with different STs, and examining the genetic relatedness among MRSA strains from different regions. Notably, this study revealed the co-existence of the sasX, qacA/B, and mupA genes in MRSA isolates, with this gene co-existence occurring exclusively in ST6290 and ST88 isolates. The gene distribution pattern from all of the isolates showed that sasX+ qacA/B+ mupA- was closely associated with epidemic clones ST59, ST239, and ST764. Both sasX and qacA/B genes were detected in ST239, consistent with previous studies (Alam et al., 2022; Song et al., 2013).
Obtaining relevant information about the sasX, qacA/B, and mupA genes is greatly helpful for nasal decolonization of S. aureus, reducing the infection rate, and guiding rational drug use in clinical practice. The involvement of adhesion genes is also important in contributing to the severity of infections and the pathogen’s endemicity. Adhesion genes were detected in the majority of MRSA isolates. The positivity rates for the icaA and clfA genes were high in our study, and all ST1-MRSA isolates carried the five genes with a 100% detection rate, which were consistent with previous findings (Wang et al., 2018; Foster et al., 2020). However, some findings differ from previous reports. For example, the icaA gene was detected in all isolates except for ST188 (Liu et al., 2023). Only 16.7% (8/48) of isolates harbored the icaA gene (Zamani et al., 2022). The carrying rates for the sdrC, sdrD, and sdrE genes were 76.7%, 20.0%, and 91.7%, respectively (Yang et al., 2017).Phylogenetic analysis revealed genetic diversity among MRSA isolates. The most common MRSA clones in southern China were ST59, ST5, ST764, and ST398, as identified from blood samples. Notably, 47 (61%) MRSA BSI strains were multidrug-resistant, with the highest resistance observed against penicillin, erythromycin, and clindamycin. These findings are consistent with those reported in previous studies (Wang et al., 2018).
In clinical practice, one of the critical hygienic measures to prevent the spread of S. aureus is the decontamination of potentially contaminated rooms, utensils, and colonized patients. Therefore, the isolation of S. aureus strains carrying antiseptic resistance genes from clinical samples is concerning (Kong et al., 2018). Our findings could be instrumental in developing more effective control and prevention strategies for nosocomial MRSA infections. However, this study has limitations. We did not perform a correlation validation between laboratory results and clinical outcomes, which would be a valuable direction for future research. Additionally, differences in study approaches and sample sizes limit the comparability of our data with that of other studies.
5 Conclusion
In conclusion, we analyzed a total of 77 MRSA isolates from a healthcare setting, revealing the presence of the sasX, mupA, and qacA/B genes, which are associated with increased mortality rates in hospitalized patients. The detection rates of these genes across all isolates were 23.4%, 33.8%, and 79.2%, respectively. Our findings demonstrated that circulating MRSA genotypes exhibit varying virulence and resistance determinants, with significant differences in gene prevalence among STs. Notably, ST59 (5/17), ST239 (3/3), and ST764 (3/8) isolates had the highest prevalence of sasX, while ST59, ST398, and ST239 showed the highest rates of qacA/B. Additionally, ST5 and ST15 isolates exhibited higher incidences of the mupA gene compared to other STs. Despite these findings, our understanding of the distribution and associations of sasX, qacA/B, and mupA genes in MRSA isolates remains limited, highlighting the need for ongoing, intensive research. Continued studies are crucial to further elucidate the genetic diversity, evolution, and epidemiology of MRSA.
Data availability statement
The original contributions presented in the study are included in the article/Supplementary Material. Further inquiries can be directed to the corresponding authors.
Author contributions
RZ: Investigation, Writing – original draft. BD: Writing – original draft. LH: Writing – original draft. CL: Writing – original draft. FX: Writing – original draft. XW: Resources, Writing – review & editing. CJ: Resources, Writing – original draft. JW: Writing – review & editing, Funding acquisition. YZ: Conceptualization, Writing – review & editing.
Funding
The author(s) declare financial support was received for the research, authorship, and/or publication of this article. This work was supported by the National Natural Science Foundation of China (Grant Nos. 81802071); Three-Year Initiative Plan for Strengthening Public Health System Construction in Shanghai (GWVI-3).
Acknowledgments
We are grateful to all patients who contributed their specimens and clinical data to this study. We express our sincere gratitude toward The Second Affiliated Hospital of Nanjing Medical University, Shanghai Children’s Medical Center, and Zhujiang Hospital of Southern Medical University for providing clinical isolates and data. We are also thankful for the guidance and help provided by the Wutai Lab Center of the Second Affiliated Hospital of Nanjing Medical University.
Conflict of interest
The authors declare that the research was conducted in the absence of any commercial or financial relationships that could be construed as a potential conflict of interest.
Publisher’s note
All claims expressed in this article are solely those of the authors and do not necessarily represent those of their affiliated organizations, or those of the publisher, the editors and the reviewers. Any product that may be evaluated in this article, or claim that may be made by its manufacturer, is not guaranteed or endorsed by the publisher.
Supplementary material
The Supplementary Material for this article can be found online at: https://www.frontiersin.org/articles/10.3389/fcimb.2025.1491658/full#supplementary-material
Abbreviations
ST, sequence type; MLST, multilocus sequence typing; PCR, polymerase chain reaction; MRSA, methicillin-resistant Staphylococcus aureus; MSSA, methicillin-susceptible Staphylococcus aureus; S. aureus, Staphylococcus aureus.
References
Abad, C. L., Pulia, M. S., Safdar, N. (2013). Does the nose know? An update on MRSA decolonization strategies. Curr. Infect. Dis. Rep. 15, 455–464. doi: 10.1007/s11908-013-0364-y
Abimanyu, N., Murugesan, S., Krishnan, P. (2012). Emergence of methicillin-resistant staphylococcus aureus ST239 with high-level mupirocin and inducible clindamycin resistance in a tertiary care center in Chennai, South India. J. Clin. Microbiol. 50, 3412–3413. doi: 10.1128/jcm.01663-12
Alam, N., Hassan, S. A., Harun, A., Foo, P. C., Ismadi, Y. K. M., Chan, Y. Y., et al (2022). Distribution of sasX, qacA/B and mupA genes and determination of genetic relatedness of methicillin-resistant Staphylococcus aureus among clinical isolates and nasal swab samples from the same patients in a hospital in Malaysia. Singapore Med. J. 63 (6), 335–341. doi: 10.11622/smedj.2020166
Baines, S. L., Holt, K. E., Schultz, M. B., Seemann, T., Howden, B. O., Jensen, S. O., et al. (2015). Convergent adaptation in the dominant global hospital clone ST239 of methicillin-resistant staphylococcus aureus. mBio. 6 (2), e00080. doi: 10.1128/mBio.00080-15
Cadilla, A., David, M. Z., Daum, R. S., Boyle-Vavra, S. (2011). Association of high-level mupirocin resistance and multidrug-resistant methicillin-resistant Staphylococcus aureus at an academic center in the midwestern United States. J. Clin. Microbiol. 49, 95–100. doi: 10.1128/JCM.00759-10
Campbell, S. J., Deshmukh, H. S., Nelson, C. L., Bae, I. G., Stryjewski, M. E., Federspiel, J. J., et al. (2008). Genotypic characteristics of Staphylococcus aureus isolates from a multinational trial of complicated skin and skin structure infections. J. Clin. Microbiol. 46, 678–684. doi: 10.1128/JCM.01822-07
Chaves, F., Garcia-Martinez, J., de Miguel, S., Otero, J. R. (2004). Molecular characterization of resistance to mupirocin in methicillin-susceptible and -resistant isolates of Staphylococcus aureus from nasal samples. J. Clin. Microbiol. 42, 822–824. doi: 10.1128/JCM.42.2.822-824.2004
Chen, Y. H., Liu, C. Y., Ko, W. C., Liao, C. H., Lu, P. L., Huang, C. H., et al. (2014). Trends in the susceptibility of methicillin-resistant Staphylococcus aureus to nine antimicrobial agents, including ceftobiprole, nemonoxacin, and tyrothricin: results from the Tigecycline In Vitro Surveillance in Taiwan (TIST) study 2006–2010. Eur. J. Clin. Microbiol. Infect. Dis. 33, 233–239. doi: 10.1007/s10096-013-1949-y
De Backer, S., Xavier, B. B., Vanjari, L., Coppens, J., Lammens, C., Vemu, L., et al. (2019). Remarkable geographical variations between India and Europe in carriage of the staphylococcal surface protein-encoding sasX/sesI and in the population structure of methicillin-resistant Staphylococcus aureus belonging to clonal complex 8. Clin. Microbiol. Infect. 25, 628 e1–628.e7. doi: 10.1016/j.cmi.2018.07.024
Enright, M. C., Day, N. P., Davies, C. E., Peacock, S. J., Spratt, B. G. (2000). Multilocus sequence typing for characterization of methicillin-resistant and methicillin-susceptible clones of Staphylococcus aureus. J. Clin. Microbiol. 38, 1008–1015. doi: 10.1128/JCM.38.3.1008-1015.2000
Foster, C. E., Kok, M., Flores, A. R., Minard, C. G., Luna, R. A., Lamberth, L. B., et al. (2020). Adhesin genes and biofilm formation among pediatric Staphylococcus aureus isolates from implant-associated infections. PLoS One 15, e0235115. doi: 10.1371/journal.pone.0235115
Ghasemzadeh-Moghaddam, H., van Belkum, A., Hamat, R. A., van Wamel, W., Neela, V. (2014). Methicillin-susceptible and -resistant Staphylococcus aureus with high-level antiseptic and low-level mupirocin resistance in Malaysia. Microb. Drug Resist. 20, 472–477. doi: 10.1089/mdr.2013.0222
Harris, S. R., Feil, E. J., Holden, M. T., Quail, M. A., Nickerson, E. K., Chantratita, N., et al. (2010). Evolution of MRSA during hospital transmission and intercontinental spread. Science. 327, 469–474. doi: 10.1126/science.1182395
Hayden, M. K., Lolans, K., Haffenreffer, K., Avery, T. R., Kleinman, K., Li, H., et al. (2016). Chlorhexidine and mupirocin susceptibility of methicillin-resistant staphylococcus aureus isolates in the REDUCE-MRSA trial. J. Clin. Microbiol. 54, 2735–2742. doi: 10.1128/jcm.01444-16
Hetem, D. J., Bonten, M. J. (2013). Clinical relevance of mupirocin resistance in Staphylococcus aureus. J. Hosp. Infect. 85, 249–256. doi: 10.1016/j.jhin.2013.09.006
Hetem, D. J., Bootsma, M. C., Bonten, M. J. (2016). Prevention of surgical site infections: decontamination with mupirocin based on preoperative screening for staphylococcus aureus carriers or universal decontamination? Clin. Infect. Dis. 62, 631–636. doi: 10.1093/cid/civ990
Ho, J., Branley, J. (2012). Prevalence of antiseptic resistance genes qacA/B and specific sequence types of methicillin-resistant Staphylococcus aureus in the era of hand hygiene. J. Antimicrob. Chemother. 67, 1549–1550. doi: 10.1093/jac/dks035
Hodgson, J. E., Curnock, S. P., Dyke, K. G., Morris, R., Sylvester, D. R., Gross, M. S. (1994). Molecular characterization of the gene encoding high-level mupirocin resistance in Staphylococcus aureus J2870. Antimicrob. Agents Chemother. 38, 1205–1208. doi: 10.1128/AAC.38.5.1205
Holden, M. T. G., Lindsay, J. A., Corton, C., Quail, M. A., Cockfield, J. D., Pathak, S., et al. (2010). Genome sequence of a recently emerged, highly transmissible, multi-antibiotic- and antiseptic-resistant variant of methicillin-resistant staphylococcus aureus, sequence type 239 (TW). J. Bacteriol. 192, 888–892. doi: 10.1128/jb.01255-09
Kondo, Y., Ito, T., Ma, X. X., Watanabe, S., Kreiswirth, B. N., Etienne, J., et al. (2007). Combination of multiplex PCRs for staphylococcal cassette chromosome mec type assignment: rapid identification system for mec, ccr, and major differences in junkyard regions. Antimicrob. Agents Chemother. 51, 264–274. doi: 10.1128/AAC.00165-06
Kong, H., Fang, L., Jiang, R., Tong, J. (2018). Distribution of sasX, pvl, and qacA/B genes in epidemic methicillin-resistant Staphylococcus aureus strains isolated from East China. Infection Drug resistance. 11, 55–59. doi: 10.2147/IDR.S153399
Lakhundi, S., Zhang, K. (2018). Methicillin-resistant staphylococcus aureus: molecular characterization, evolution, and epidemiology. Clin. Microbiol. Rev. 31 (4), e00020-18. doi: 10.1128/CMR.00020-18
Lee, C. Y., Fang, Y. P., Wu, T. H., Chang, Y. F., Sung, C. H. (2022). Sequence types 8, 59, and 45 methicillin resistant Staphylococcus aureus as the predominant strains causing skin and soft tissue infections in Taiwan's prisons and jails. J. Microbiology Immunol. Infection. 55, 1239–1245. doi: 10.1016/j.jmii.2021.08.013
Letunic, I., Bork, P. (2021). Interactive Tree Of Life (iTOL) v5: an online tool for phylogenetic tree display and annotation. Nucleic Acids Res. 49, W293–W296. doi: 10.1093/nar/gkab301
Li, M., Du, X., Villaruz, A. E., Diep, B. A., Wang, D., Song, Y., et al. (2012). MRSA epidemic linked to a quickly spreading colonization and virulence determinant. Nat. Med. 18, 816–819. doi: 10.1038/nm.2692
Liu, H., Ji, X., Wang, H., Hou, X., Sun, H., Billington, C., et al. (2023). Genomic epidemiology and characterization of Staphylococcus aureus isolates from raw milk in Jiangsu, China: emerging broader host tropism strain clones ST59 and ST398. Front. Microbiol. 14. doi: 10.3389/fmicb.2023.1266715
Lu, Z., Chen, Y., Chen, W., Liu, H., Song, Q., Hu, X., et al. (2015). Characteristics of qacA/B-positive Staphylococcus aureus isolated from patients and a hospital environment in China. J. Antimicrob. Chemother. 70, 653–657. doi: 10.1093/jac/dku456
Mayer, S., Boos, M., Beyer, A., Fluit, A. C., Schmitz, F. J. (2001). Distribution of the antiseptic resistance genes qacA, qacB and qacC in 497 methicillin-resistant and -susceptible European isolates of Staphylococcus aureus. J. Antimicrob. Chemother. 47, 896–897. doi: 10.1093/jac/47.6.896
Milstone, A. M., Passaretti, C. L., Perl, T. M. (2008). Chlorhexidine: expanding the armamentarium for infection control and prevention. Clin. Infect. Dis. 46, 274–281. doi: 10.1086/524736
Monecke, S., Muller, E., Dorneanu, O. S., Vremera, T., Ehricht, R. (2014). Molecular typing of MRSA and of clinical staphylococcus aureus isolates from Iaşi, Romania. PLoS One 9 (5), e97833. doi: 10.1371/journal.pone.0097833
Muñoz-Gallego, I., Infiesta, L., Viedma, E., Perez-Montarelo, D., Chaves, F. (2016). Chlorhexidine and mupirocin susceptibilities in methicillin-resistant Staphylococcus aureus isolates from bacteraemia and nasal colonisation. J. Global Antimicrobial Resistance. 4, 65–69. doi: 10.1016/j.jgar.2015.11.005
Nair, R., Hanson, B. M., Kondratowicz, K., Dorjpurev, A., Davaadash, B., Enkhtuya, B., et al. (2013). Antimicrobial resistance and molecular epidemiology of Staphylococcus aureus from Ulaanbaatar, Mongolia. PeerJ. 1, e176. doi: 10.7717/peerj.176
Nakaminami, H., Ito, T., Han, X., Ito, A., Matsuo, M., Uehara, Y., et al. (2017). First report of sasX-positive methicillin-resistant Staphylococcus aureus in Japan. FEMS Microbiol. Lett. 364 (16). doi: 10.1093/femsle/fnx171
Noguchi, N., Suwa, J., Narui, K., Sasatsu, M., Ito, T., Hiramatsu, K., et al. (2005). Susceptibilities to antiseptic agents and distribution of antiseptic-resistance genes qacA/B and smr of methicillin-resistant Staphylococcus aureus isolated in Asia during 1998 and 1999. J. Med. Microbiol. 54, 557–565. doi: 10.1099/jmm.0.45902-0
Peacock, S. J., Moore, C. E., Justice, A., Kantzanou, M., Story, L., Mackie, K., et al. (2002). Virulent combinations of adhesin and toxin genes in natural populations of Staphylococcus aureus. Infect. Immun. 70, 4987–4996. doi: 10.1128/IAI.70.9.4987-4996.2002
Percival, S. L., Suleman, L., Vuotto, C., Donelli, G. (2015). Healthcare-associated infections, medical devices and biofilms: risk, tolerance and control. J. Med. Microbiol. 64, 323–334. doi: 10.1099/jmm.0.000032
Shamsudin, M. N., Alreshidi, M. A., Hamat, R. A., Alshrari, A. S., Atshan, S. S., Neela, V. (2012). High prevalence of qacA/B carriage among clinical isolates of meticillin-resistant Staphylococcus aureus in Malaysia. J. Hosp. Infect. 81, 206–208. doi: 10.1016/j.jhin.2012.04.015
Sheng, W.-H., Wang, J.-T., Lauderdale, T.-L., Weng, C.-M., Chen, D., Chang, S.-C. (2009). Epidemiology and susceptibilities of methicillin-resistant Staphylococcus aureus in Taiwan: emphasis on chlorhexidine susceptibility. Diagn. Microbiol. Infect. Dis. 63, 309–313. doi: 10.1016/j.diagmicrobio.2008.11.014
Sidhu, M. S., Heir, E., Leegaard, T., Wiger, K., Holck, A. (2002). Frequency of disinfectant resistance genes and genetic linkage with β-lactamase transposon tn552 among clinical staphylococci. Antimicrobial Agents Chemotherapy. 46, 2797–2803. doi: 10.1128/aac.46.9.2797-2803.2002
Simor, A. E., Stuart, T. L., Louie, L., Watt, C., Ofner-Agostini, M., Gravel, D., et al. (2007). Mupirocin-resistant, methicillin-resistant Staphylococcus aureus strains in Canadian hospitals. Antimicrob. Agents Chemother. 51, 3880–3886. doi: 10.1128/AAC.00846-07
Song, Y., Du, X., Li, T., Zhu, Y., Li, M. (2013). Phenotypic and molecular characterization of Staphylococcus aureus recovered from different clinical specimens of inpatients at a teaching hospital in Shanghai between 2005 and 2010. J. Med. Microbiol. 62, 274–282. doi: 10.1099/jmm.0.050971-0
Sutherland, R., Boon, R. J., Griffin, K. E., Masters, P. J., Slocombe, B., White, A. R. (1985). Antibacterial activity of mupirocin (pseudomonic acid), a new antibiotic for topical use. Antimicrob. Agents Chemother. 27, 495–498. doi: 10.1128/AAC.27.4.495
Turner, N. A., Sharma-Kuinkel, B. K., Maskarinec, S. A., Eichenberger, E. M., Shah, P. P., Carugati, M., et al. (2019). Methicillin-resistant Staphylococcus aureus: an overview of basic and clinical research. Nat. Rev. Microbiol. 17, 203–218. doi: 10.1038/s41579-018-0147-4
Udo, E. E., Jacob, L. E., Mathew, B. (2001). Genetic analysis of methicillin-resistant Staphylococcus aureus expressing high- and low-level mupirocin resistance. J. Med. Microbiol. 50, 909–915. doi: 10.1099/0022-1317-50-10-909
Wang, X., Liu, Q., Zhang, H., Li, X., Huang, W., Fu, Q., et al. (2018). Molecular characteristics of community-associated staphylococcus aureus isolates from pediatric patients with bloodstream infections between 2012 and 2017 in Shanghai, China. Front. Microbiol. 9, 1211. doi: 10.3389/fmicb.2018.01211
Wang, J. T., Sheng, W. H., Wang, J. L., Chen, D., Chen, M. L., Chen, Y. C., et al. (2008). Longitudinal analysis of chlorhexidine susceptibilities of nosocomial methicillin-resistant Staphylococcus aureus isolates at a teaching hospital in Taiwan. J. Antimicrob. Chemother. 62, 514–517. doi: 10.1093/jac/dkn208
Wang, Z., Zhou, H., Wang, H., Chen, H., Leung, K. K., Tsui, S., et al. (2014). Comparative genomics of methicillin-resistant Staphylococcus aureus ST239: distinct geographical variants in Beijing and Hong Kong. BMC Genomics 15 (1), 529. doi: 10.1186/1471-2164-15-529
Warren, D. K., Prager, M., Munigala, S., Wallace, M. A., Kennedy, C. R., Bommarito, K. M., et al. (2016). Prevalence of qacA/B Genes and Mupirocin Resistance Among Methicillin-Resistant Staphylococcus aureus (MRSA) Isolates in the Setting of Chlorhexidine Bathing Without Mupirocin. Infect. Control Hosp. Epidemiol. 37, 590–597. doi: 10.1017/ice.2016.1
Yang, X., Qian, S., Yao, K., Wang, L., Liu, Y., Dong, F., et al. (2017). Multiresistant ST59-SCCmec IV-t437 clone with strong biofilm-forming capacity was identified predominantly in MRSA isolated from Chinese children. BMC Infect. Dis. 17, 733. doi: 10.1186/s12879-017-2833-7
Yun, H. J., Lee, S. W., Yoon, G. M., Kim, S. Y., Choi, S., Lee, Y. S., et al. (2003). Prevalence and mechanisms of low- and high-level mupirocin resistance in staphylococci isolated from a Korean hospital. J. Antimicrob. Chemother. 51, 619–623. doi: 10.1093/jac/dkg140
Zamani, S., Mohammadi, A., Hajikhani, B., Abiri, P., Fazeli, M., Nasiri, M. J., et al. (2022). Mupirocin-resistant staphylococcus aureus in Iran: A biofilm production and genetic characteristics. BioMed. Res. Int. 2022, 7408029. doi: 10.1155/2022/7408029
Zarizal, S., Yeo, C. C., Faizal, G. M., Chew, C. H., Zakaria, Z. A., Jamil Al-Obaidi, M. M., et al. (2018). Nasal colonisation, antimicrobial susceptibility and genotypic pattern of Staphylococcus aureus among agricultural biotechnology students in Besut, Terengganu, east coast of Malaysia. Trop. Med. Int. Health 23, 905–913. doi: 10.1111/tmi.13090
Keywords: MRSA, MLST, bloodstream infections, sasX, mupA, qacA/B, sequence type
Citation: Zhao R, Du B, Hu L, Li C, Xue F, Wang X, Jiang C, Wang J and Zhao Y (2025) Distribution of sasX, mupA, and qacA/B genes and determination of genetic relatedness of epidemic methicillin-resistant Staphylococcus aureus strains associated with bloodstream infections in southern China. Front. Cell. Infect. Microbiol. 15:1491658. doi: 10.3389/fcimb.2025.1491658
Received: 05 September 2024; Accepted: 13 January 2025;
Published: 30 January 2025.
Edited by:
Amanda Claire Brown, Tarleton State University, United StatesReviewed by:
William R. Schwan, University of Wisconsin–La Crosse, United StatesCemal Sandalli, Recep Tayyip Erdoğan University, Türkiye
Copyright © 2025 Zhao, Du, Hu, Li, Xue, Wang, Jiang, Wang and Zhao. This is an open-access article distributed under the terms of the Creative Commons Attribution License (CC BY). The use, distribution or reproduction in other forums is permitted, provided the original author(s) and the copyright owner(s) are credited and that the original publication in this journal is cited, in accordance with accepted academic practice. No use, distribution or reproduction is permitted which does not comply with these terms.
*Correspondence: Yanfeng Zhao, emhhb3lhbmZlbmdAbmptdS5lZHUuY24=; Jinghua Wang, d2FuZ2ppbmdodWFAc2NjbC5vcmcuY24=
†These authors have contributed equally to this work and share first authorship