- 1Institute of Bacterial Infections and Zoonoses, Friedrich-Loeffler-Institut – Federal Research Institute for Animal Health (FLI), Jena, Germany
- 2Institute of Infectious Diseases and Infection Control, Jena University Hospital, Jena, Germany
- 3Institute of Diagnostic Virology, Friedrich-Loeffler-Institut – Federal Research Institute for Animal Health (FLI), Greifswald-Insel Riems, Germany
- 4Institute of Epidemiology, Friedrich-Loeffler-Institut – Federal Research Institute for Animal Health (FLI), Greifswald-Insel Riems, Germany
Introduction: Tick-borne pathogens, such as Borreliella spp., Rickettsia spp., and Anaplasma spp., are frequently detected in Germany. They circulate between animals and tick vectors and can cause mild to severe diseases in humans. Knowledge about distribution and prevalence of these pathogens over time is important for risk assessment of human and animal health.
Methods: Ixodes ricinus nymphs were collected at different locations in 2009/2010 and 2019 in Germany and analyzed for tick-borne pathogens by real-time PCR and sequencing.
Results: Borreliella spp. were detected with a prevalence of 11.96% in 2009/2010 and 13.10% in 2019 with B. afzelii and B. garinii as dominant species. Borrelia miyamotoi was detected in seven ticks and in coinfection with B. afzelii or B. garinii. Rickettsia spp. showed a prevalence of 8.82% in 2009/2010 and 1.68% in 2019 with the exclusive detection of R. helvetica. The prevalence of Anaplasma spp. was 1.00% in 2009/2010 and 7.01% in 2019. A. phagocytophilum was detected in seven tick samples. None of the nymphs were positive for C. burnetii.
Discussion: Here, observed changes in prevalence were not significant after a decade but require longitudinal observations including parameters like host species and density, climatic factors to improve our understanding of tick-borne diseases.
1 Introduction
Tick-borne diseases represent a severe medical problem in many regions. In Europe, Lyme borreliosis (LB) and tick-borne encephalitis are of special importance with very high incidence rates of >100/100,000 population or >15/100,000 in some European countries (Burn et al., 2023; Van Heuverswyn et al., 2023). Furthermore, other bacterial, viral, and protozoal pathogens can be transmitted by ticks and cause diseases in humans and animals. In Europe, these infections are mainly transmitted by the hard tick Ixodes (I.) ricinus. This tick species has a wide host spectrum, and tick activity can be observed in association with a broad range of biotic and abiotic factors, for example, between 3°C and 28°C air temperature and between 35% and 95% air humidity (Gethmann et al., 2020).
Rising temperatures (climate change), land management, and acaricide resistance are possible drivers of the spread of ticks into new territories or increased tick populations (Nuttall, 2022; Vanwambeke et al., 2024). These ticks may carry new pathogens and represent a financial burden to the public health system and veterinary public health system. Therefore, monitoring of ticks and tick-borne pathogens is essential to understand transmission dynamics, to raise awareness, and to adjust counter measures (treatments, vaccines, and acaricides) (Harrington et al., 2020). It has been estimated from available epidemiological data of some federal states and national health insurances that LB constitutes between 60,000 and >200,000 human infections in Germany every year (Wilking et al., 2023). The Borrelia burgdorferi sensu lato (s.l.) group, recently phylogenetically reclassified as Borreliella (B.) spp., includes at least 20 genospecies, and three further genospecies were under discussion (Gupta, 2019; Wolcott et al., 2021). According to present knowledge, only infections with B. burgdorferi sensu stricto (s.s.), B. garinii, B. afzelii, B. spielmanii, and, since 2009, B. bavariensis (former B. garinii OspA-type 4) cause clinical symptoms in humans (Margos et al., 2009; Rauer et al., 2018).
Borrelia miyamotoi, genetically more closely related to B. burgdorferi s.l. than to the relapsing fever (RF) spirochetal group, was first detected in 1994 in ticks in Japan. Now, it occurs in the northern hemisphere and co-circulates with B. burgdorferi s.l., the causative agent of LB with overlapping vertebrate and tick hosts. Human cases caused by Borrelia miyamotoi are rare and mostly present as influenza-like illness, with RF in sporadic cases (Cutler et al., 2019).
Another group of emerging tick-borne pathogens are bacteria of the order Rickettsiales. They are widespread, obligate intracellular and host-adapted bacteria. Among other genera of the order Rickettsia and Anaplasma are of human public health and agricultural interest. They are maintained between the animal host and tick vector.
Rickettsia spp. cause febrile illness of varying severity in humans. The bacteria are maintained in small mammals, e.g., rodents and the tick vector. Humans are accidental hosts where the bacteria preferentially replicate within endothelial cells of blood vessels causing vascular inflammation and a typical rash or eschar at the side of inoculation. If untreated, the infection may progress to meningoencephalitis and multiorgan failure (Helminiak et al., 2022). Most prevalent species in Northern Europe and Germany belong to the spotted fever group (SFG) rickettsiae, such as R. helvetica and R. monacensis (Scheid et al., 2016; Guccione et al., 2023). The pathogenic potential of R. helvetica varies from mild to severe and may progress to chronic perimyocarditis and meningitis (Scarpulla et al., 2018). The pathogenicity of R. monacensis is unknown, and clinical cases are rarely described (Jado et al., 2007). Both species are transmitted by I. ricinus, and a high prevalence has been reported for R. helvetica in ticks with up to 12% in Germany (Wolfel et al., 2006).
Anaplasma can affect animals as well as humans and replicate within cells of the hematopoietic system. The distribution is closely related to the tick vector with domestic and wild animals as reservoir. Three Anaplasma species have been reported in Europe, whereof A. phagocytophilum is the only species with a high zoonotic potential (Hofmann-Lehmann et al., 2004; Bauer et al., 2021; Knoll et al., 2021b). It is the etiological agent of human granulocytic anaplasmosis (HGA), and clinical symptoms vary from mild to severe febrile illness with headache, malaise, and myalgia (MacQueen and Centellas, 2022). Domestic animals such as dogs, cats, and horses may develop granulocytic anaplasmosis with various clinical symptoms (Hofmann-Lehmann et al., 2004; Jensen et al., 2007; Schafer et al., 2022b). In cattle and sheep, an infection with A. phagocytophilum causes tick-borne fever indicated by high fever, anorexia, and signs of depression. In cattle, a decreased milk yield and abortions are observed (Woldehiwet, 2006). A. phagocytophilum is transmitted by I. ricinus, the main vector in Europe, whereas Dermacentor spp. ticks seem to play a lesser role. The prevalence in vertebrate hosts varies among European countries with up to 34% (Stuen et al., 2013).
Coxiella burnetii is the etiological agent of Q (query) fever causing acute flu-like illness in humans, which can become chronic and life-threatening. It is endemic worldwide except New Zealand. It has a very broad host spectrum, and domestic ruminants are considered as the main reservoir. C. burnetii was originally isolated from a D. andersoni tick in Montana, USA, and, since then, the role of ticks in transmission of C. burnetii is controversially discussed. Especially, with the discovery of Coxiella-like endosymbionts (CLEs), the relevance of ticks in the epidemiology of Q fever is debatable (Korner et al., 2021).
In this study, we investigated bacterial tick-borne diseases at seven sites in Germany. Nine to 10 years later, the same sites were re-investigated, and changes of the infection rates were discussed.
2 Materials and methods
2.1 Study area and tick collection
Tick sampling was carried out at seven different sites located in seven German federal states from April until July in 2010 and in 2019, respectively. Only samples from Thuringia were collected from April until July in 2009 and 2019 (Supplementary Table 1; Figure 1). Ticks were collected by flagging with a cotton blanket of 1-m2 size in deciduous forest habitats with close proximity to hiking trails. After transfer to the laboratory, ticks were sorted as adult female or adult male ticks, nymphs, or larvae by morphological criteria. Species determination was conducted for all ticks according to Estrada-Peña et al. (2004).
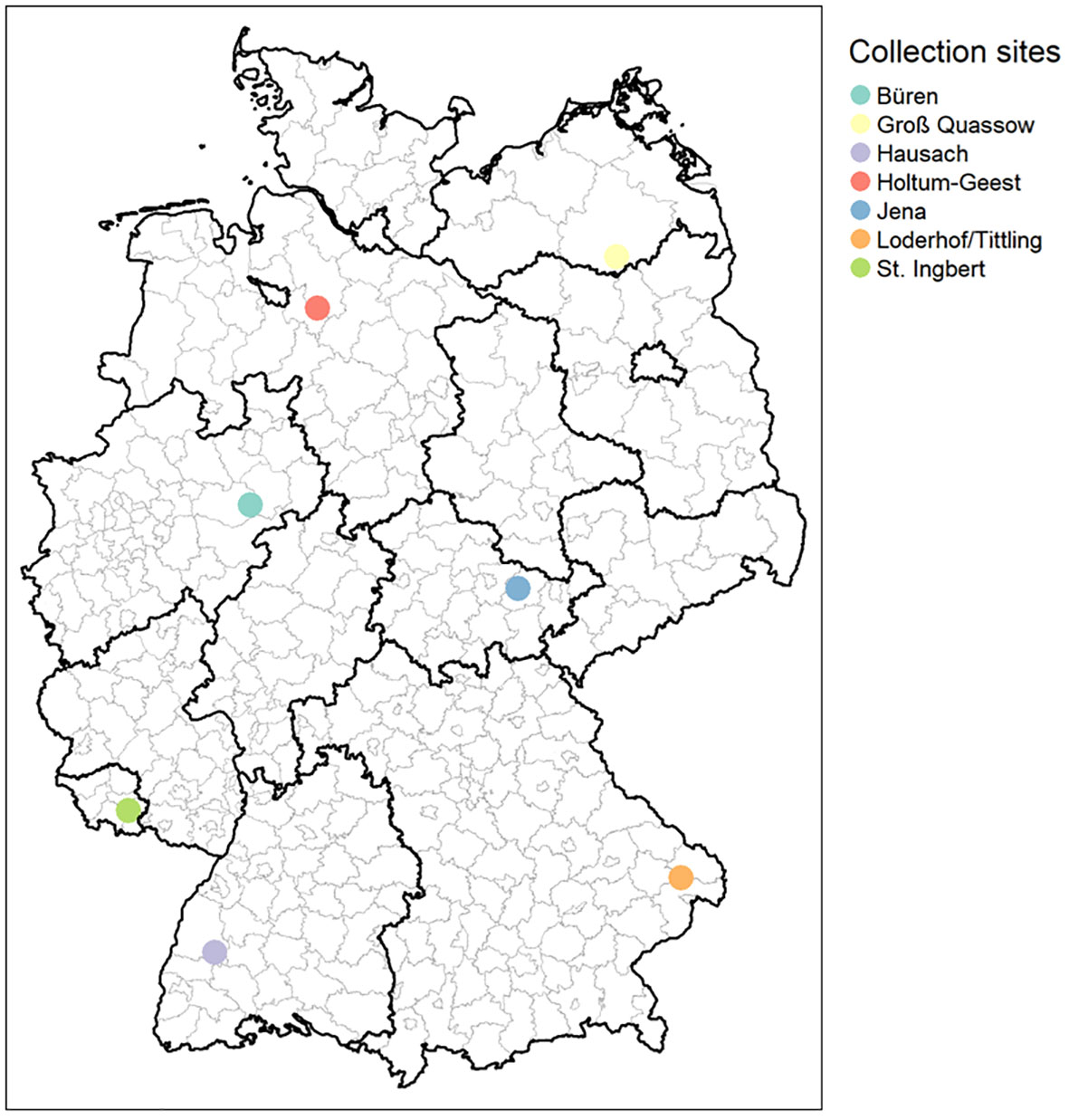
Figure 1 Geographical location of tick collection sites. Büren, North Rhine-Westphalia (NW); Groß Quassow, Mecklenburg-West Pomerania (MV); Hausch, Baden-Wuerttemberg (BW); Holtum-Geest, Lower Saxony (NI); Jena, Thuringia (TH); Loderhof/Tittling, Bavaria (BY); and St. Ingberg, Saarland (SL). Figure created with Maps © Mapbox (www.mapbox.com/about/maps) and © OpenStreetMap (www.openstreetmap.org/about).
2.2 DNA extraction
From all collection sites, a total of 1,608 Ixodes ricinus nymphs with an average of 115 nymphs per site and year were individually processed for DNA extraction using the NucleoSpin RNA/DNA kit (Macherey-Nagel, Düren, Germany) according to the manufacturer’s instructions. Briefly, ticks were ground individually in a mixer mill (Retsch, Haan, Germany) with three stainless steel beads in 350 of µl RA buffer for 2 min at 30 Hz. Tick debris were removed by centrifugation (11.000 x g, 1 min) and the lysate used for DNA extraction as indicated by the supplier’s instructions. All samples were stored at −80°C until further analysis.
2.3 Detection of Borreliella spp., Borrelia spp., and species differentiation
The ticks were examined for Borreliella spp. and Borrelia spp. DNA by real-time PCR targeting the 5S-23S intergenic spacer as described elsewhere (Strube et al., 2010). Detection of Borrelia miyamotoi was carried out by real-time PCR targeting the flaB gene as previously described (Venczel et al., 2016). Genospecies identification was carried out by ospA amplification and sequencing as described elsewhere (Rauter et al., 2002). Briefly, amplified ospA PCR fragments of 296 bp were separated by agarose gel electrophoresis and extracted (QIAquick Gel Extraction Kit, Qiagen, Hilden, Germany), and both strands were sequenced (Eurofins, Ebersberg, Germany). Raw data were trimmed with the Geneious Prime software (version 2021.0.1, Biomatters Ltd., Boston, USA), and data were analyzed using the online blastn tool (https://blast.ncbi.nlm.nih.gov/Blast.cgi) (Zhang et al., 2000). Samples used for species differentiation of Borreliella spp. and Borrelia miyamotoi could not be further investigated for other pathogens due to limited amount of DNA.
2.4 Detection of Rickettsia spp., Anaplasma spp., and Coxiella burnetii
Tick samples were screened by real-time quantitative PCR (qPCR) for Rickettsia spp. (gltA), Anaplasma spp. (16S rRNA), and A. phagocytophilum (msp2) and C. burnetii (IS1111), as described elsewhere (Courtney et al., 2004; Panning et al., 2008; Wolfel et al., 2008; Hurtado et al., 2015; Del Cerro et al., 2022). For species differentiation of rickettsiae, the rrs, gltA, and ompB genes were amplified and sequenced as described previously (Weisburg et al., 1991; Roux and Raoult, 2000; Labruna et al., 2004). Nucleotide sequences of single genes or concatemers were aligned to representative sequences of rickettsial species available from National Library of Medicine (NLM) GenBank (https://www.ncbi.nlm.nih.gov/genbank/) using MAFFT (v7.450) implemented in Geneious Prime. Alignments were manually trimmed and phylogenetic trees constructed using the neighbor-joining method with the genetic distance model Tamura-Nei and bootstrap tests with 1,000 replicates. Ehrlichia chaffeensis was set as outgroup for rrs and gltA and R. bellii for ompB. Phylogenetic trees were visualized using FigTree v1.4.3 (http://tree.bio.ed.ac.uk/software/figtree/).
2.5 Statistical analysis
To evaluate if there is a difference in the number of positive ticks between the Federal States (collection sites) and the two time points, we used the Fisher exact test (Fisher, 1922). All tests were carried out using the Statistical software R (R Core Team, 2021).
3 Results
3.1 Overall prevalence of tick-borne pathogens
The spatial and temporal distribution of tick-borne bacterial pathogens in Germany was examined. A total of 1,608 questing I. ricinus nymphs were collected from seven different locations representing seven federal states in 2009/2010 (n = 807) or 2019 (n = 801) (Figure 1). All nymphs were individually processed and screened by qPCR for bacterial pathogens.
From all collected nymphs, 88/736 (11.96%) were positive for Borreliella spp., including Borrelia miyamotoi, 62/703 (8.82%) for Rickettsia spp., 7/703 (1%) for Anaplasma spp., and 0/424 (0%) for Coxiella burnetii in 2009 and 2010. No significant differences were observed for ticks collected in 2019 with 93/710 (13.10%) nymphs positive for Borreliella spp. including Borrelia miyamotoi, 50/708 (7.01%) for Rickettsia spp., 12/713 (1.68%) for Anaplasma spp., and 0/176 (0%) for C. burnetii (Supplementary Table 1). The overall results are summarized in Figure 2.
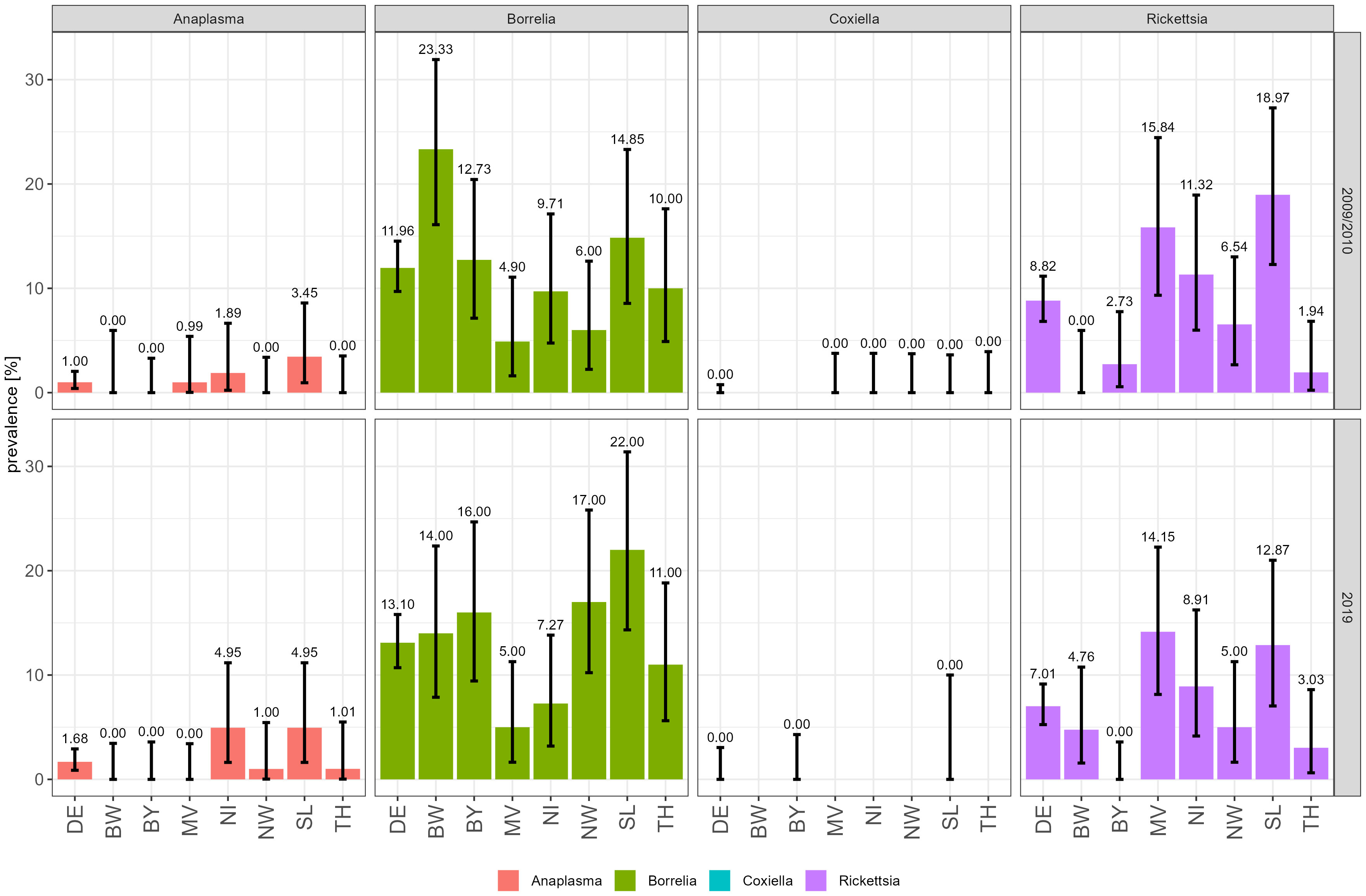
Figure 2 Prevalence of tick-borne pathogens in 2009/2010 (upper panel) and 2019 (lower panel) according to sampling site. Prevalence of Anaplasma spp. and Borreliella spp. including B. miyamotoi, C. burnetii, and Rickettsia spp. is indicated as bars with lower and higher confidence intervals. DE, Germany; BW, Baden-Wuerttemberg; BY, Bavaria; NI, Lower Saxony; MV, Mecklenburg-West Pomerania; NW, North Rhine-Westphalia; SL, Saarland; TH, Thuringia.
3.2 Detection of Borreliella spp. and Borrelia miyamotoi
For Borreliella spp., on average, 100 nymphs per collection site and year were screened (n = 1,446). The overall prevalence for Borreliella spp. including Borrelia miyamotoi in nymphs increased slightly from 11.96% (88/736) in 2009/2010 to 13.10% (93/710) in 2019 (Figure 2). However, there are some remarkable differences between the seven sites: In North Rhine-Westphalia (NW), Borreliella spp. including Borrelia miyamotoi prevalence in I. ricinus nymphs increased from 6.00% (6/100) in 2009/2010 to 17.00% (17/100) in 2019 and, in Saarland (SL), from 14.85% (15/101) to 22.00% (22/100). In Baden-Wurttemberg (BW), the prevalence decreased from 23.33% (28/120) in 2009/2010 to 14% (14/100) in 2019. At all other sites, the prevalence of Borreliella spp. including Borrelia miyamotoi remained nearly the same in 2009/2010 and 2019 (Table 1; Figure 2). Observed differences were statistically significant (Fisher test) in NW between 2009/2010 and 2019 only (Table 2).
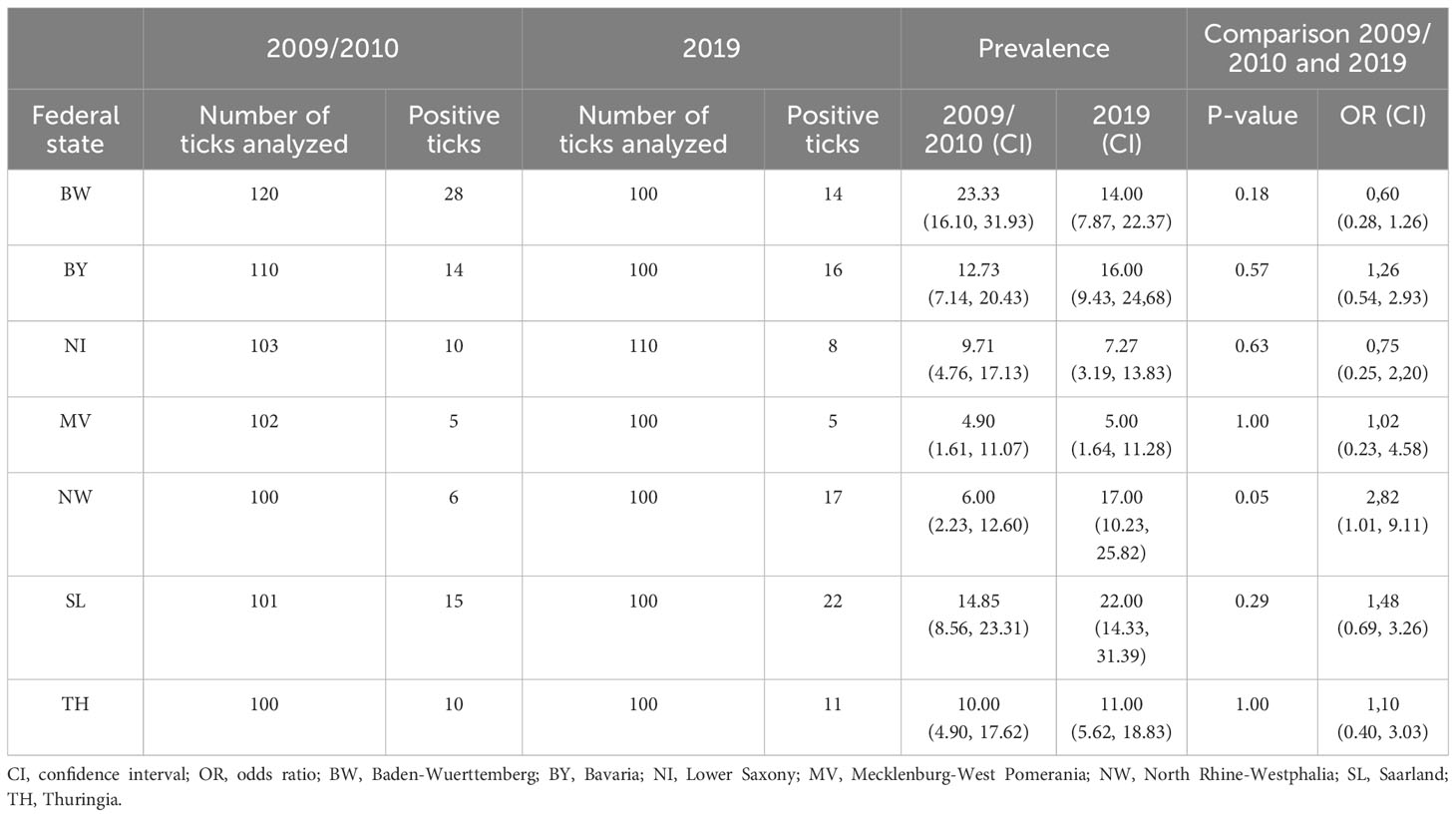
Table 1 Prevalence of Borreliella spp. and B. miyamotoi in Ixodes ricinus nymphs in Germany in 2009/2010 compared to 2019.
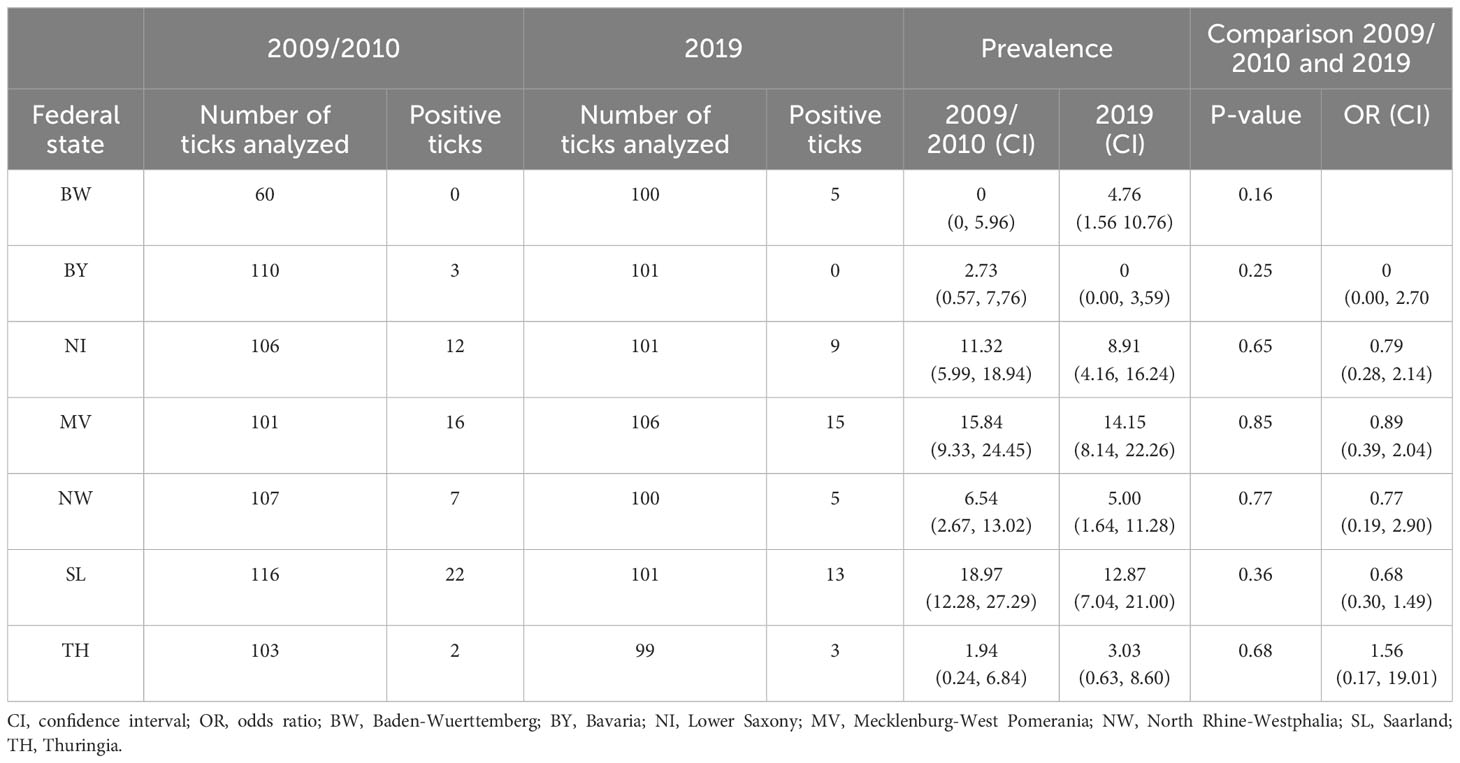
Table 2 Prevalence of Rickettsia spp. in Ixodes ricinus nymphs in Germany in 2009/2010 compared to 2019.
Positive samples (n = 181) were subjected to species differentiation, whereof 57 resulted in no detectable PCR product and 38 were not further analyzed due to screening for other pathogens or poor DNA quality. In 79 samples, B. garinii, B. afzelii, B. bavariensis, B. valaisiana, B. burgdorferi s.s., and Borrelia miyamotoi were detected. The most frequently detected species were B. afzelii and B. garinii with 15.47% (n = 28) and 17.68% (n = 32), respectively. The other four species were detected remarkably less frequently in all Borreliella spp.–positive samples (Figure 3). Mixed infections of Borrelia miyamotoi and B. afzelii or Borrelia miyamotoi and B. garinii were detected in three ticks. For one tick, sample differentiation between B. garinii and B. bavariensis was not possible (Figure 3). Detailed information is presented in Supplementary Figure 1; Supplementary Table 1.
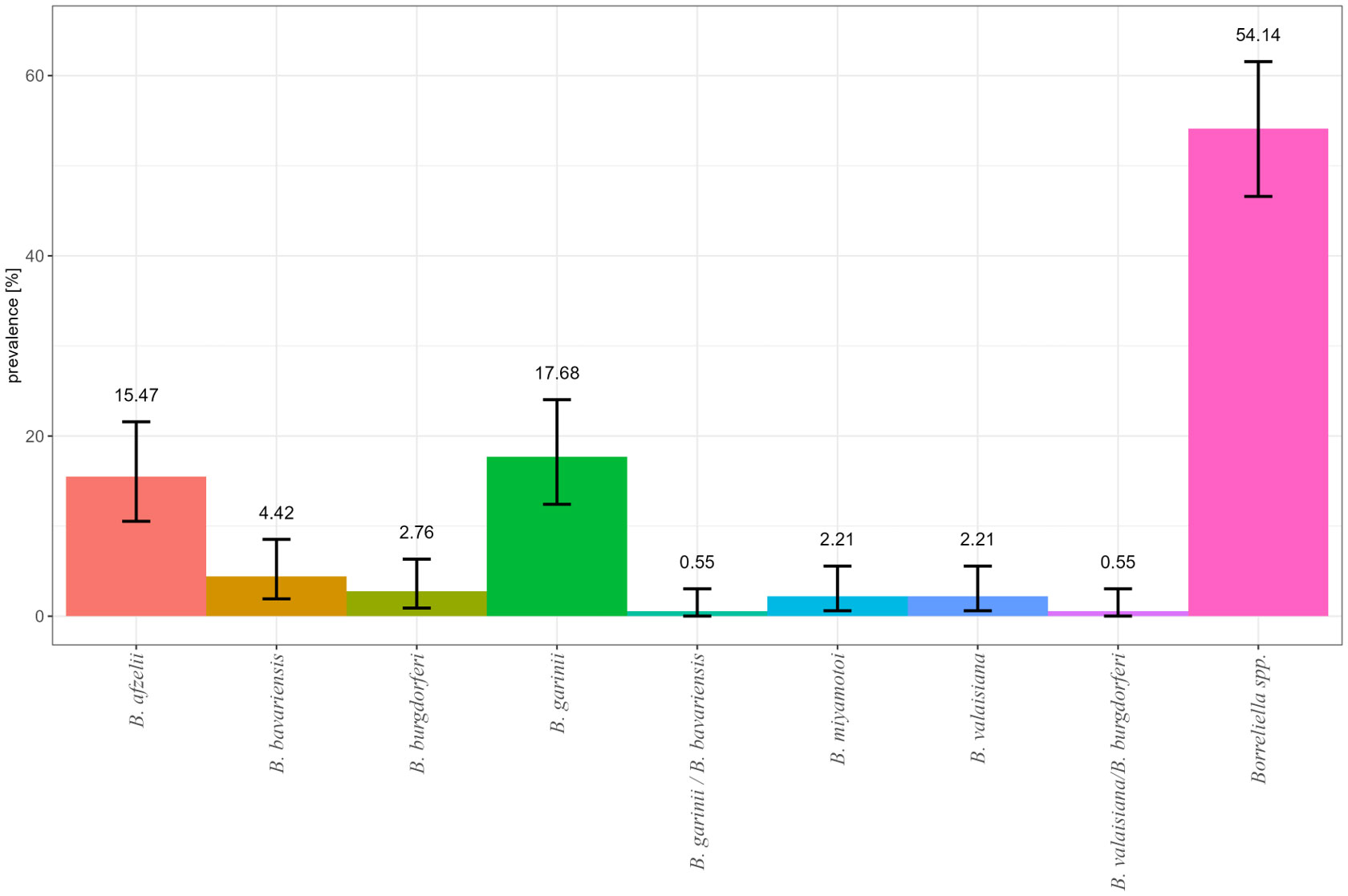
Figure 3 Relative frequency of Borreliella spp. and Borrelia miyamotoi in infected I. ricinus nymphs by real-time PCR detection in Germany in 2009/2010 and 2019.
3.3 Detection of Rickettsia spp.
On average, 100 I. ricinus nymphs were tested for Rickettsia spp. per location and time point (n = 1,411), except for BW, 60 nymphs were tested in 2009/2010 only (Table 2; Supplementary Table 1). The overall prevalence in 2009/2010 was 8.82% (62/703) with a slight but statistically insignificant decrease to 7.06% (50/708) in 2019. Rickettsiae were most frequently detected in SL with 18.97% (22/116), in Mecklenburg-West Pomerania (MV) with 15.84% (16/101) and Lower Saxony (NI) with 11.32% (12/106) in 2009/2010. Similar results were obtained for samples taken in 2019 with 12.87% (13/101) positive ticks in SL, 14.15% (15/106) in MV, and 8.91% (9/101) in NI. In all other locations, none or ≤ 5 samples tested positive for Rickettsia spp. (Table 1).
For species differentiation, all 112 Rickettsia spp.–positive samples were subjected to PCR amplification and sequencing of rrs, gltA, and ompB gene fragments. Overall, from 100, 88, and 103 samples, a rrs gene fragment, a gltA gene fragment, and an ompB gene fragment were generated, respectively. All three gene fragments were obtained from 79 samples. All retrieved sequences from the rrs and gltA gene fragments were identical (Figures 4A, B). Four sequence types of ompB (Figure 4C) were observed and six sequence types when all three gene fragments were aligned as concatemers (Figure 4D). All positive (108/112) samples clustered together with spotted fever (SFG) group rickettsiae and were identified as R. helvetica.
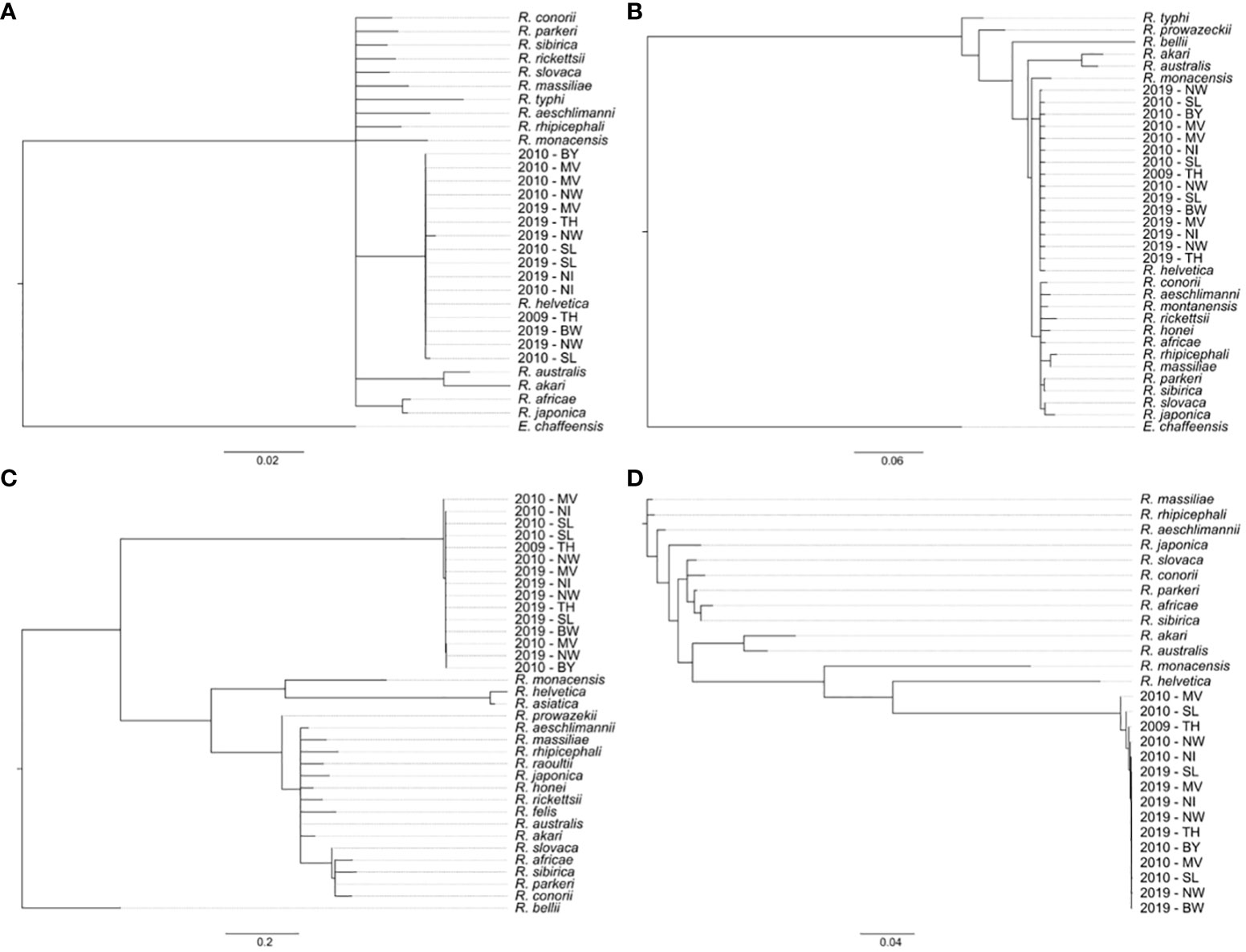
Figure 4 Phylogenetic tree based on (A) rrs, (B) gltA, and (C) ompB partial fragments or (D) rrs-gltA-ompB-concatemers from Rickettsia spp. reference strains and representative samples per sampling location and time. Trees were built using the neighbor-joining method with the genetic distance model Tamura-Nei and bootstrap tests with 1,000 replicates. Bar indicates nucleotide distance. Nucleotide accession numbers of Rickettsia spp. reference genes are listed in Supplementary Table 2; BW, Baden-Wuerttemberg; BY, Bavaria; NI, Lower Saxony; MV, Mecklenburg-West Pomerania; NW, North Rhine-Westphalia; SL, Saarland; TH, Thuringia.
3.4 Detection of Anaplasma spp.
Anaplasma were detected in 19 out of 1,416 tested I. ricinus nymphs (Table 3). There was no statistically significant difference between sampling sites and time with 1.00% (7/703) in 2009/2010 and 1.68% (12/713) in 2019. Most Anaplasma positive ticks were found in SL and NI with a slight increase in prevalence from 3.45% (4/116) to 4.95% (5/101) or 1.89% (2/106) to 4.95% (5/101). Of these, seven [0.469% (0.200, 1.021)] tested positive for A. phagocytophilum collected in MV in 2010 (n = 1), in NI in 2019 (n = 1), in SL in 2010 (n = 3), and 2019 (n = 2).
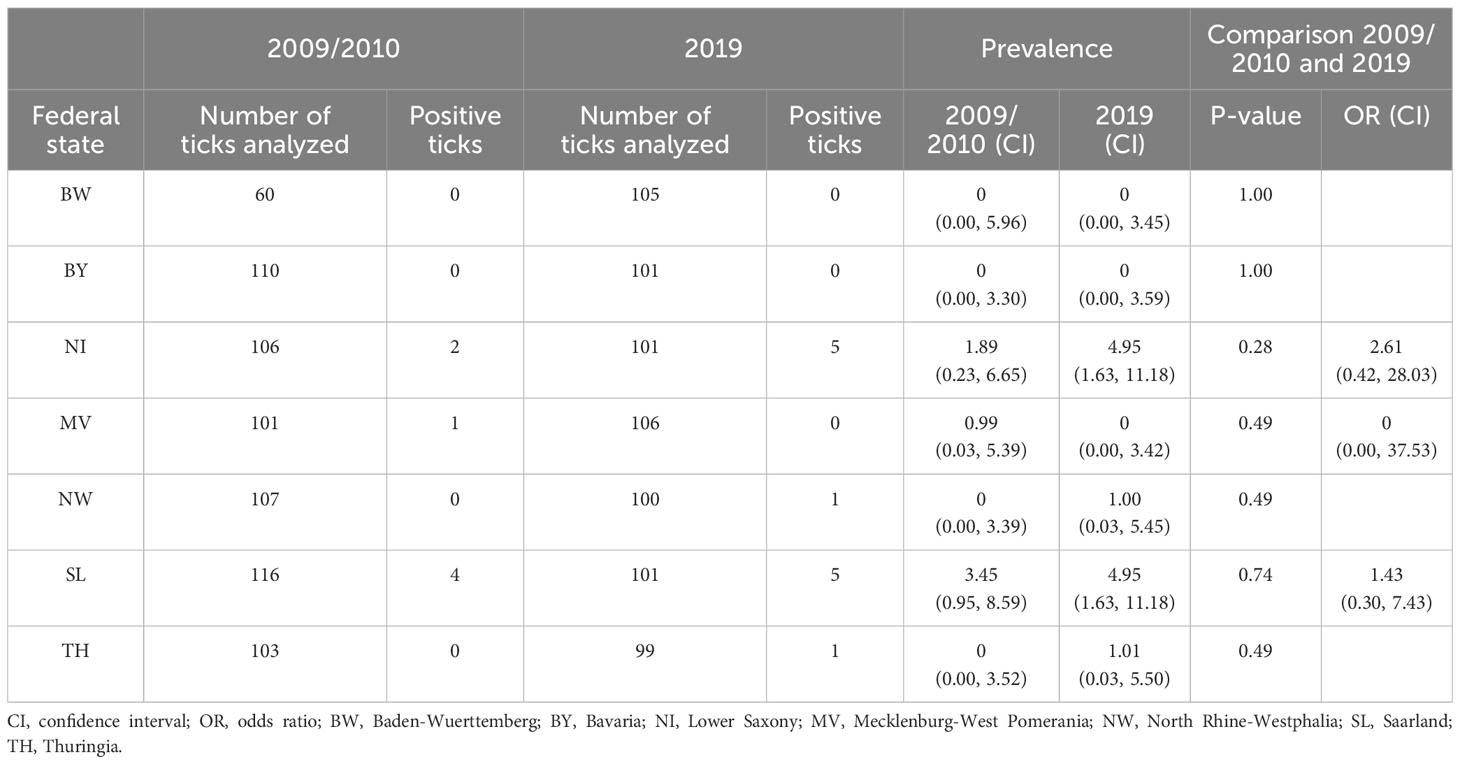
Table 3 Prevalence of Anaplasma spp. in Ixodes ricinus nymphs in Germany in 2009/2010 compared to 2019.
3.5 Detection of Coxiella burnetii
Nearly 600 I. ricinus nymphs collected in 2009/2010 (n = 424) from five different locations and in 2019 (n = 176) from two different locations were analyzed for C. burnetii. All samples tested negative for the IS1111 element. Because of the overall negative results, further testing of samples was neglected.
4 Discussion
Tick-borne diseases are of high interest in human and veterinary medicine. Observations about their spread in space and time can help to assess the epidemiological situation in a given area. The vector I. ricinus has a broad range of host species including mammals, birds, and reptiles. The compilation of a high-resolution density map of unfed nymphal I. ricinus allows an insight into the broad distribution of this important disease vector in Germany depending on bioclimatic variables and land cover (Brugger et al., 2016). In our study, the prevalence for Borreliella spp. including Borrelia miyamotoi in nymphal I. ricinus was 11.96% in 2009/2010 and 13.10% 10 years later (Table 1). This is in accordance with many other studies, e.g., with prevalence of 10%, between 5% and 12% or 12.9% in nymphs (Wilske et al., 1987; Kampen et al., 2004; Fingerle et al., 2008). A higher prevalence in nymphs was found in South Germany (15%) by Raileanu et al. (2021), in Hanover in Northwest Germany (19.8%) by Blazejak et al. (2018) and in North Germany (28.6%) by Knoll et al. (2021a) with prevalence between 18.6% and 45.7% in nymphs at different places and habitats. Prevalence for B. burgdorferi s.l. in Europe was reported between 2% and 43% for nymphs, with a mean average of 10.8% (Hubalek and Halouzka, 1998). A meta-analysis of studies in Europe between 2010 and 2016 showed an average Borreliella prevalence of 15.6% (Strnad et al., 2017).
Comparable results were shown by Kampen et al. (2004) at three sites in the region Siebengebirge in West Germany. They found a higher prevalence of 5.5%, 15.8%, and 21.8% at these locations in 2001 compared to 1987–1989 with 1.1% to 15.4% (Kurtenbach et al., 1995; Kampen et al., 2004). This change might be caused by presently unknown changes, e.g., in ecological conditions or wildlife management. Schwarz et al. (2012) detected an increasing B. burgdorferi s.l. prevalence in the same region over the last two decades (Schwarz et al., 2012). In our study, only at one site, a significant higher prevalence was observed (NW) after 10 years.
The detected differences may be caused by changes in ecological conditions or wildlife management, like it was assumed by Kampen et al. (2004) for three sites in the Siebengebirge in West Germany. In Hanover in Northwest Germany, it was assumed that the reason for decreased B. afzelii detection over 10 years could be caused by changed reservoir host population (Blazejak et al., 2018). It is suggested that the bird population could be responsible for local changes of Borreliella spp. prevalence like in the here presented study (Klaus et al., 2016). Birds, especially blackbirds (Turdus merula) and song thrushes (Turdus philomelos), play an important role in the distribution of Borreliella spp (Humair et al., 1993; Olsen et al., 1995; Taragel’ova et al., 2008; Klaus et al., 2016). They can also serve as reservoir hosts (Lommano et al., 2014), but there are no data available about bird populations at the sampling sites visited in the here presented study. The population size of abundant and common bird species increased generally from 2009 until 2018, but trends are different according to habitat and nest sides (Kamp et al., 2021). The observed increase of overall prevalence of Borreliella spp. might reflect the general increase of birds as tick hosts, but this is only speculative.
In our study, in 2009/2010, the dominant species was rodent-associated B. afzelii with up to 40% in SL and TH whose prevalence declined to 27.27% or 9.09% in 2019 (Supplementary Figure S1). The same high percentage (43.1%) for B. afzelii was found in Bavaria in 2010 (Vogerl et al., 2012). In 2019, the bird-associated species B. garinii increased at all sampling sites except BW and became the dominant species (Supplementary Figure S1). The reason is not quite clear. It is possible that such changes can be introduced by birds as transportation tool for ticks and reservoir hosts for B. burgdorferi s.l. species. A shift in B. burgdorferi s.l. species over a time span was observed not only by Blazejak et al. (2018) in Hanover, Germany, but also by Okeyo et al. (2020) during a longitudinal study between 1999 and 2010 in Latvia in one of three investigated habitats. In a former study, in Germany, it became evident that not only sedentary birds like blackbirds (Turdus merula) were the most frequently tick infested species, but also short- and long-distance bird species were more suitable to transfer Borreliella spp. strains to new sites (Klaus et al., 2016). However, the rodent-associated species B. afzelii can be harbored in ticks infesting birds and can be transported in this way as well (Olsen et al., 1995; Geller et al., 2013; Klaus et al., 2016). A study of Norte et al. (2020) in 11 European countries showed that 28 bird species transported three tick species. Among the ticks, 37% were infected with various B. burgdorferi s.l. species, especially bird-associated B. garinii (61%), but, among the species, also rodent-associated B. afzelii (9%). In our study, other B. burgdorferi s.l. species were found very rarely, i.e., B. bavariensis (4.42%), B. valaisiana (0.55%), and B. burgdorferi s.s. (2.76%) (Figure 3). Other studies showed higher prevalence for Borreliella spp., e.g., 9.7% for B. valaisiana and 9.9% B. burgdorferi s.s. in I. ricinus collected in northern Germany or 14.7% for B. valaisiana and 6.3% for B. burgdorferi s.s. in I. ricinus collected in southern Germany, respectively (Vögerl et al., 2012; Tappe et al., 2014). These studies analyzed all tick developmental stages, and the overall prevalence was reported. This might reflect that tick developmental stages differ in Borreliella spp. prevalence and might explain the here observed lower prevalence in nymphs.
A special remark should be made about Borrelia miyamotoi (2.21%) that was found in NW and SL in 2009/2010 and in Lower Saxony and Thuringia in 2019. Reports show that this species is established in Germany although at a very low level of 1.2% to 3.5% prevalence in I. ricinus ticks (Richter et al., 2003; Sinski et al., 2016; Szekeres et al., 2017). The clinical relevance of this Borrelia species should not be underestimated (Cutler et al., 2019; Kubiak et al., 2021). Clinical reports are rare but severe with central nervous system infections as chronic manifestations in immunocompromised persons (Gugliotta et al., 2013; Hovius et al., 2013; Boden et al., 2016). An association between the history of non-Hodgkin lymphoma and rituximab treatment, which causes conditional immunosuppression, was made in these patients. However, serological assays for lyme disease fail to detect B. miyamotoi infections and clinicians should rely on patient’s history, clinical examination, and routine cerebrospinal fluid (CSF) analysis. Classical dark-field microscopy or acrinidine orange staining and 16S RNA sequencing of CSF supports detection of spirochetes and B. miyamotoi (Boden et al., 2016).
Rickettsiae are distributed worldwide and cause mild to severe, sometimes life-threatening diseases in humans. Several Rickettsia spp. have been reported in Germany, but there is limited information available on their distribution and genetic diversity. Almost all Rickettsia spp. positive tick samples could be identified as R. helvetica (108/112 samples) in the here reported study. This species was discovered in 1979 in Switzerland and has since been reported in Germany in I. ricinus ticks with a prevalence up to 13.4% and in small rodents with up to 33.3% (Burgdorfer et al., 1979; Silaghi et al., 2011; Obiegala et al., 2016). The highest prevalence of Rickettsia spp. in I. ricinus ticks was recorded for the area of Hamburg in North Germany with 52.5%. Of all positive samples 25.6% were subjected to species differentiation with R. helvetica as the only occurring species (May and Strube, 2014). Other studies demonstrated a prevalence of 12% for Rickettsia spp. in I. ricinus and exclusively detected R. helvetica in South Germany (Wolfel et al., 2006). Monitoring of ticks for Rickettsia spp. over a 15-year period in Northern Germany in the region of Hannover showed significant fluctuations between sampling years, with a general increase from 2005 with 33.3% until 2020 with 36.0% and a peak of 50.8% in 2015 (Glass et al., 2022). This demonstrates, that the here reported prevalence from approximately 2% to 19% for some federal states and an overall prevalence of 8.82% for 2009/2010 or 7.01% for 2019 for Rickettsia spp. and the exclusively detected species R. helvetica in I. ricinus is comparable with former studies.
Albeit I. ricinus is considered as the main vector for R. helvetica, other rickettsiae, e.g., the highly pathogenic R. conorii have been detected in this vector (Sprong et al., 2009). Interestingly, R. helvetica was also detected in a botfly larvae from a roe deer questioning the animal reservoir (Scheid et al., 2016). It is assumed that small mammals function as animal reservoir for R. helvetica. Several studies show a high positivity of wild and companion animals by DNA detection or serological examination. Particulary, rodents and shrews carry R. helvetica as dominant occurring species and less frequent R. felis and R raoultii. The prevalence ranged from 6.8% in MV, 7.0% in NW, and 8.0% in TH (Fischer et al., 2018). Dogs as companion animals show a high seroprevalence of 93.9% for Rickettsia spp. antibodies using a micro-immunofluorescence assay. These dogs were not imported or have left Germany. Of all serological positive samples, 66.0% could be determined as specific for R. helvetica, 2.8% for R. raoulti, and 1.6% for R. slovaca (Wachter et al., 2015). Companion animals are in close contact with their owners and living area. Therefore, the pathogenic potential of R. helvetica and other Rickettsia spp. present in Germany and neighboring countries should not be underestimated. New tick species have been reported in Germany, such as Hyalomma spp. carrying the human pathogenic species R. slovaca and R. aeschlimanii (Chitimia-Dobler et al., 2019, 2024). Also, migrating birds carry ticks and their pathogenic cargo. One study discovered in addition to R. helvetica another species Candidatus R. vini in I. arboricola and I. lividus ticks for the first time in Germany. This species is closely related to the highly human pathogenic species R. japonica and R. heilongjiangensis (Wimbauer et al., 2022).
The prevalence of Rickettsia spp. and Anaplasma spp. is often analyzed and reported together. The here detected prevalence for Anaplasma spp. from approximately 2% to 5% for some federal states or 1.00 and 1.68% for 2009/2010 and 2019, respectively, is comparable to previously reported prevalence in I. ricinus ticks of 1.9% to 6.4% in different parts of Germany (Hartelt et al., 2004; Hildebrandt et al., 2010; Tappe and Strube, 2013; Knoll et al., 2021b). Differences in prevalence may be caused by variable geographical host densities, e.g., for wild ruminants and rodents (De la Fuente et al., 2005; Stuen et al., 2013).
No significant changes were observed between 2009/2010 and 2019. Of all Anaplasma positive ticks seven nymphs were positive for the zoonotic pathogen A. phagocytophilum. Reported co-infection rates of A. phagocytophilum and Rickettsia spp. are low with approximately 1% (Hildebrandt et al., 2010; Tappe and Strube, 2013). Co-infection was detected in two I. ricinus nymphs from 2010 or 2019 in NI only. However, A. phagocytophilum is an important pathogen affecting humans as well as companion animals like horses, cats, and dogs (Kohn et al., 2011; Langenwalder et al., 2020; Schafer et al., 2022a, b, 2023).
Only a few studies compare prevalence of tick-borne pathogens over time with contradictory results. Reports from the region of Hannover in northern Germany detected statistically significant increase for A. phagocytophilum and Rickettsia spp. positive ticks in 2010 compared to 2005 (Tappe and Strube, 2013). In contrast, a significant decrease in prevalence was detected in north-west Germany in 2019 compared to 2018 (Knoll et al., 2021b). Another study reported a stagnating prevalence for A. phagocytophilum but a significant increase for Rickettsia spp. in I. ricinus over a 10-year monitoring period (Blazejak et al., 2017). These data are comparable with the here reported prevalence for these pathogens with no significant changes after a decade.
Comprehensive studies on the prevalence of rickettsial diseases or HGA in Germany are missing. There are a few reports available showing a high seroprevalence for Rickettsia spp. in forestry workers or homeless people, which are more likely to be exposed to arthropods. Among homeless people 7% (10/147) tested serological positive for R. conorii, whereof only two originated not from Germany or neighboring countries (Heinrich et al., 2023). Further forestry workers show a high seroprevalence for SFG rickettsiae of 27.5% with 9.7% for R. helvetica, 5% R. raoultii, 2.7% R. felis, 0.5% R. monacensis, and 0.5% R. slovaca (Wolfel et al., 2017). Similar, the seroprevalence for A. phagocytophilum in forestry workers with previous tick exposure was reported as 4.51% and 1.20% in the control group (Kowalski et al., 2006). Reports on clinical cases are rare. This suggests that rickettsial diseases and HGA might be rare events in Germany or underdiagnosed.
Historically, Q fever in humans and Coxiellosis in ruminants was associated with a high abundance of the sheep tick D. marginatus in southern Germany. It was assumed that C. burnetii is either transmitted by the tick bite or excreted and spread by tick feces (Liebisch, 1977). Vector competence for two in Germany most abundant tick species in Germany, I. ricinus and D. marginatus, was shown recently. Both tick species can take up the bacteria with the blood meal and excrete them with feces. Transstadial transmission from nymphs to adult ticks was demonstrated but transovarial transmission seems unlikely (Korner et al., 2020; Bauer et al., 2023). However, several studies reporting an overall negative or very low prevalence for C. burnetii in ticks in Germany. A few reports are available targeting Dermacentor spp. ticks and small rodents in Q fever outbreak areas in southern Germany, but none of the tested samples were Coxiella-positive by PCR (Hartelt et al., 2008; Pluta et al., 2010). A small-scale study carried out in western Germany detected no C. burnetii–positive I. ricinus ticks (n = 52) (Henning et al., 2006). Only one study reported a small portion of C. burnetii–positive I. ricinus (1.9%) for a region in East Germany (Hildebrandt et al., 2011). Therefore, the prevalence of C. burnetii in I. ricinus and Dermacentor spp. ticks is generally assumed to be very low or negative in Germany and is in accordance with the here reported data.
The prevalence of C. burnetii in ticks increases in eastern and southern European countries, e.g., high prevalence for C. burnetii in D. marginatus from Serbia (22%) (Tomanovic et al., 2013) or France (2% to 13%) (Bonnet et al., 2013; Michelet et al., 2014) was reported. Prevalence of C. burnetii in I. ricinus varies with approximately 16% in Poland (Szymańska-Czerwińska et al., 2013) and negative results for Spain (Astobiza et al., 2011) or France (Michelet et al., 2014). This implies that foci in which ticks may play a role in transmission of C. burnetii do exist. However, all results need to be evaluated with care, because most studies do not employ methods for differentiation between C. burnetii and CLEs. The abundance of certain tick species seems to play a role in the spread of pathogens. High prevalence of C. burnetii in species of the genera Hyalomma spp. and Rhipicephalus spp. with up to 54% in Spain or 22% in Italy was reported (Mancini et al., 2014; Gonzalez et al., 2020), and vector competence was shown for H. aegypticum (Široký et al., 2010). Climate change might influence the distribution of tick species and Hyalomma spp. was already detected and shown to develop from nymphs to adult ticks in Germany (Chitimia-Dobler et al., 2019, 2024). However, Hyalomma ticks (n = 18) collected in Germany and examined for tick-borne pathogen were negative for C. burnetii, but the number of ticks examined was very low and may not be representative (Chitimia-Dobler et al., 2019).
5 Conclusions
Considering the high importance of bacterial tick-borne infections for human health, it is recommended to examine changes of pathogens in ticks as vector in space and time. Especially, longitudinal studies at the same places are rare because of high costs and technical problems. Here, we gave an insight at seven sites in Germany 9 to 10 years after the first examination with very different aspects at the single sites but no general increase of tick-borne pathogens during this time span. This may be caused by the multifactorial conditions at the sites, e.g., climate conditions and especially host population. These factors were not monitored in the here presented study but are necessary to understand which factors influence the prevalence of tick-borne pathogens, tick density, and transmission dynamics. Longitudinal observations are recommended by including such parameters like host species and density to improve our understanding of tick-borne diseases.
Data availability statement
The datasets presented in this study can be found in online repositories. The names of the repository/repositories and accession number(s) can be found in the article/Supplementary Material.
Ethics statement
The manuscript presents research on animals that do not require ethical approval for their study.
Author contributions
KM: Data curation, Formal Analysis, Investigation, Methodology, Project administration, Resources, Supervision, Validation, Writing – original draft, Writing – review & editing. BH: Formal Analysis, Investigation, Resources, Writing – review & editing. JG: Data curation, Formal Analysis, Investigation, Resources, Validation, Visualization, Writing – review & editing. HB: Formal Analysis, Writing – review & editing. MP: Writing – review & editing. CK: Conceptualization, Data curation, Formal Analysis, Investigation, Methodology, Project administration, Resources, Supervision, Validation, Writing – original draft, Writing – review & editing.
Funding
The author(s) declare financial support was received for the research, authorship, and/or publication of this article.
Acknowledgments
The authors are thankful to Hannes Axt, Fiona Balzer, Nadine Engelhardt, Christian Korthase, Nico Rabold, Birgit Schikowski, Petra Sippach, and Johannes Solle for their excellent technical assistance.
Conflict of interest
The authors declare that the research was conducted in the absence of any commercial or financial relationships that could be construed as a potential conflict of interest.
Publisher’s note
All claims expressed in this article are solely those of the authors and do not necessarily represent those of their affiliated organizations, or those of the publisher, the editors and the reviewers. Any product that may be evaluated in this article, or claim that may be made by its manufacturer, is not guaranteed or endorsed by the publisher.
Supplementary material
The Supplementary Material for this article can be found online at: https://www.frontiersin.org/articles/10.3389/fcimb.2024.1429667/full#supplementary-material
References
Astobiza, I., Barral, M., Ruiz-Fons, F., Barandika, J. F., Gerrikagoitia, X., Hurtado, A., et al. (2011). Molecular investigation of the occurrence of Coxiella burnetii in wildlife and ticks in an endemic area. Vet. Microbiol. 147, 190–194. doi: 10.1016/j.vetmic.2010.05.046
Bauer, B. U., Knittler, M. R., Andrack, J., Berens, C., Campe, A., Christiansen, B., et al. (2023). Interdisciplinary studies on Coxiella burnetii: From molecular to cellular, to host, to one health research. Int. J. Med. Microbiol. 313, 151590. doi: 10.1016/j.ijmm.2023.151590
Bauer, B. U., Raileanu, C., Tauchmann, O., Fischer, S., Ambros, C., Silaghi, C., et al. (2021). Anaplasma phagocytophilum and Anaplasma ovis-Emerging Pathogens in the German Sheep Population. Pathogens 10, 15. doi: 10.3390/pathogens10101298
Blazejak, K., Janecek, E., Strube, C. (2017). A 10-year surveillance of Rickettsiales (Rickettsia spp. and Anaplasma phagocytophilum) in the city of Hanover, Germany, reveals Rickettsia spp. as emerging pathogens in ticks. Parasit Vectors 10, 588. doi: 10.1186/s13071-017-2537-2
Blazejak, K., Raulf, M. K., Janecek, E., Jordan, D., Fingerle, V., Strube, C. (2018). Shifts in Borrelia burgdorferi (s.l.) geno-species infections in Ixodes ricinus over a 10-year surveillance period in the city of Hanover (Germany) and Borrelia miyamotoi-specific Reverse Line Blot detection. Parasit Vectors 11, 304. doi: 10.1186/s13071-018-2882-9
Boden, K., Lobenstein, S., Hermann, B., Margos, G., Fingerle, V. (2016). Borrelia miyamotoi-associated neuroborreliosis in immunocompromised person. Emerg. Infect. Dis. 22, 1617–1620. doi: 10.3201/eid2209.152034
Bonnet, S., de la Fuente, J., Nicollet, P., Liu, X., Madani, N., Blanchard, B., et al. (2013). Prevalence of tick-borne pathogens in adult Dermacentor spp. ticks from nine collection sites in France. Vector Borne Zoonotic Dis. 13, 226–236. doi: 10.1089/vbz.2011.0933
Brugger, K., Boehnke, D., Petney, T., Dobler, G., Pfeffer, M., Silaghi, C., et al. (2016). A density map of the tick-borne encephalitis and lyme borreliosis vector ixodes ricinus (Acari: ixodidae) for Germany. J. Med. Entomol 53, 1292–1302. doi: 10.1093/jme/tjw116
Burgdorfer, W., Aeschlimann, A., Peter, O., Hayes, S. F., Philip, R. N. (1979). Ixodes ricinus: vector of a hitherto undescribed spotted fever group agent in Switzerland. Acta Trop. 36, 357–367.
Burn, L., Tran, T. M. P., Pilz, A., Vyse, A., Fletcher, M. A., Angulo, F. J., et al. (2023). Incidence of lyme borreliosis in Europe from national surveillance systems, (2005-2020). Vector Borne Zoonotic Dis. 23, 156–171. doi: 10.1089/vbz.2022.0071
Chitimia-Dobler, L., Schaper, S., Riess, R., Bitterwolf, K., Frangoulidis, D., Bestehorn, M., et al. (2019). Imported hyalomma ticks in Germany in 2018. Parasit Vectors 12, 134. doi: 10.1186/s13071-019-3380-4
Chitimia-Dobler, L., Springer, A., Lang, D., Lindau, A., Fachet, K., Dobler, G., et al. (2024). Molting incidents of Hyalomma spp. carrying human pathogens in Germany under different weather conditions. Parasit Vectors 17, 70. doi: 10.1186/s13071-024-06175-y
Courtney, J. W., Kostelnik, L. M., Zeidner, N. S., Massung, R. F. (2004). Multiplex real-time PCR for detection of Anaplasma phagocytophilum and Borrelia burgdorferi. J. Clin. Microbiol. 42, 3164–3168. doi: 10.1128/JCM.42.7.3164-3168.2004
Cutler, S., Vayssier-Taussat, M., Estrada-Pena, A., Potkonjak, A., Mihalca, A. D., Zeller, H. (2019). A new Borrelia on the block: Borrelia miyamotoi - a human health risk? Euro Surveill 24, 14. doi: 10.2807/1560-7917.ES.2019.24.18.1800170
De la Fuente, J., Naranjo, V., Ruiz-Fons, F., Höfle, U., Fernandez de Mera, I. G., Villanua, D., et al. (2005). Potential Vertebrate Reservoir Hosts and Invertebrate Vectors of Anaplasma marginale and A. phagocytophilum in Central Spain. Vector-Borne Zoonotic Dis. 5, 390–401. doi: 10.1089/vbz.2005.5.390
Del Cerro, A., Oleaga, A., Somoano, A., Barandika, J. F., Garcia-Perez, A. L., Espi, A. (2022). Molecular identification of tick-borne pathogens (Rickettsia spp., Anaplasma phagocytophilum, Borrelia burgdorferi sensu lato, Coxiella burnetii and piroplasms) in questing and feeding hard ticks from North-Western Spain. Ticks Tick Borne Dis. 13, 101961. doi: 10.1016/j.ttbdis.2022.101961
Estrada-Peña, A., Mihalca, A. D., Petney, T. (2017). Ticks of Europe and North Africa. Springer International Publishing.
Fingerle, V., Schulte-Spechtel, U. C., Ruzic-Sabljic, E., Leonhard, S., Hofmann, H., Weber, K., et al. (2008). Epidemiological aspects and molecular characterization of Borrelia burgdorferi s.l. from southern Germany with special respect to the new species Borrelia spielmanii sp. nov. Int. J. Med. Microbiol. 298, 279–290. doi: 10.1016/j.ijmm.2007.05.002
Fischer, S., Spierling, N. G., Heuser, E., Kling, C., Schmidt, S., Rosenfeld, U. M., et al. (2018). High prevalence of Rickettsia helvetica in wild small mammal populations in Germany. Ticks Tick Borne Dis. 9, 500–505. doi: 10.1016/j.ttbdis.2018.01.009
Fisher, R. A. (1922). On the interpretation of χ2 from contingency tables, and the calculation of P. J. R. Stat. Soc. 85, 87–94. doi: 10.2307/2340521
Geller, J., Nazarova, L., Katargina, O., Leivits, A., Jarvekulg, L., Golovljova, I. (2013). Tick-borne pathogens in ticks feeding on migratory passerines in Western part of Estonia. Vector Borne Zoonotic Dis. 13, 443–448. doi: 10.1089/vbz.2012.1054
Gethmann, J., Hoffmann, B., Kasbohm, E., Suss, J., Habedank, B., Conraths, F. J., et al. (2020). Research paper on abiotic factors and their influence on Ixodes ricinus activity-observations over a two-year period at several tick collection sites in Germany. Parasitol. Res. 119, 1455–1466. doi: 10.1007/s00436-020-06666-8
Glass, A., Springer, A., Strube, C. (2022). A 15-year monitoring of Rickettsiales (Anaplasma phagocytophilum and Rickettsia spp.) in questing ticks in the city of Hanover, Germany. Ticks Tick Borne Dis. 13, 101975. doi: 10.1016/j.ttbdis.2022.101975
Gonzalez, J., Gonzalez, M. G., Valcarcel, F., Sanchez, M., Martin-Hernandez, R., Tercero, J. M., et al. (2020). Prevalence of Coxiella burnetii (Legionellales: Coxiellaceae) Infection Among Wildlife Species and the Tick Hyalomma lusitanicum (Acari: Ixodidae) in a Meso-Mediterranean Ecosystem. J. Med. Entomol 57, 551–556. doi: 10.1093/jme/tjz169
Guccione, C., Colomba, C., Iaria, C., Cascio, A. (2023). Rickettsiales in the WHO European Region: an update from a One Health perspective. Parasit Vectors 16, 41. doi: 10.1186/s13071-022-05646-4
Gugliotta, J. L., Goethert, H. K., Berardi, V. P., Telford, S. R., 3rd (2013). Meningoencephalitis from Borrelia miyamotoi in an immunocompromised patient. N Engl. J. Med. 368, 240–245. doi: 10.1056/NEJMoa1209039
Gupta, R. S. (2019). Distinction between Borrelia and Borreliella is more robustly supported by molecular and phenotypic characteristics than all other neighbouring prokaryotic genera: Response to Margos’ et al. “The genus Borrelia reloaded” (PLoS ONE 13(12): e0208432). PloS One 14, e0221397. doi: 10.1371/journal.pone.0221397
Harrington, L. C., Foy, B. D., Bangs, M. J. (2020). Considerations for human blood-feeding and arthropod exposure in vector biology research: an essential tool for investigations and disease control. Vector Borne Zoonotic Dis. 20, 807–816. doi: 10.1089/vbz.2020.2620
Hartelt, K., Oehme, R., Frank, H., Brockmann, S. O., Hassler, D., Kimmig, P. (2004). Pathogens and symbionts in ticks: prevalence of Anaplasma phagocytophilum (Ehrlichia sp.), Wolbachia sp., Rickettsia sp., and Babesia sp. in Southern Germany. Int. J. Med. Microbiol. 293 Suppl 37, 86–92. doi: 10.1016/S1433-1128(04)80013-5
Hartelt, K., Pluta, S., Oehme, R., Kimmig, P. (2008). Spread of ticks and tick-borne diseases in Germany due to global warming. Parasitol. Res. 103 Suppl 1, S109–S116. doi: 10.1007/s00436-008-1059-4
Heinrich, F., Rauch, J., Bertram, F., Kempf, V. A. J., Besier, S., Kuta, P., et al. (2023). Seroprevalence of arthropod-borne bacterial infections in homeless individuals in Hamburg in 2020. Infection 51, 1819–1822. doi: 10.1007/s15010-023-02059-y
Helminiak, L., Mishra, S., Kim, H. K. (2022). Pathogenicity and virulence of rickettsia. Virulence 13, 1752–1771. doi: 10.1080/21505594.2022.2132047
Henning, K., Greiner-Fischer, S., Hotzel, H., Ebsen, M., Theegarten, D. (2006). Isolation of Spiroplasma sp. from an Ixodes tick. Int. J. Med. Microbiol. 296 Suppl 40, 157–161. doi: 10.1016/j.ijmm.2006.01.012
Hildebrandt, A., Kramer, A., Sachse, S., Straube, E. (2010). Detection of Rickettsia spp. and Anaplasma phagocytophilum in Ixodes ricinus ticks in a region of Middle Germany (Thuringia). Ticks Tick Borne Dis. 1, 52–56. doi: 10.1016/j.ttbdis.2009.11.005
Hildebrandt, A., Straube, E., Neubauer, H., Schmoock, G. (2011). Coxiella burnetii and coinfections in Ixodes ricinus ticks in Central Germany. Vector Borne Zoonotic Dis. 11, 1205–1207. doi: 10.1089/vbz.2010.0180
Hofmann-Lehmann, R., Meli, M. L., Dreher, U. M., Gonczi, E., Deplazes, P., Braun, U., et al. (2004). Concurrent infections with vector-borne pathogens associated with fatal hemolytic anemia in a cattle herd in Switzerland. J. Clin. Microbiol. 42, 3775–3780. doi: 10.1128/JCM.42.8.3775-3780.2004
Hovius, J. W., de Wever, B., Sohne, M., Brouwer, M. C., Coumou, J., Wagemakers, A., et al. (2013). A case of meningoencephalitis by the relapsing fever spirochaete Borrelia miyamotoi in Europe. Lancet 382, 658. doi: 10.1016/S0140-6736(13)61644-X
Hubalek, Z., Halouzka, J. (1998). Prevalence rates of Borrelia burgdorferi sensu lato in host-seeking Ixodes ricinus ticks in Europe. Parasitol. Res. 84, 167–172. doi: 10.1007/s004360050378
Humair, P. F., Turrian, N., Aeschlimann, A., Gern, L. (1993). Ixodes ricinus immatures on birds in a focus of Lyme borreliosis. Folia Parasitol. (Praha) 40, 237–242.
Hurtado, A., Barandika, J. F., Oporto, B., Minguijon, E., Povedano, I., Garcia-Perez, A. L. (2015). Risks of suffering tick-borne diseases in sheep translocated to a tick infested area: a laboratory approach for the investigation of an outbreak. Ticks Tick Borne Dis. 6, 31–37. doi: 10.1016/j.ttbdis.2014.09.001
Jado, I., Oteo, J. A., Aldamiz, M., Gil, H., Escudero, R., Ibarra, V., et al. (2007). Rickettsia monacensis and human disease, Spain. Emerg. Infect. Dis. 13, 1405–1407. doi: 10.3201/eid1309.060186
Jensen, J., Simon, D., Murua Escobar, H., Soller, J. T., Bullerdiek, J., Beelitz, P., et al. (2007). Anaplasma phagocytophilum in dogs in Germany. Zoonoses Public Health 54, 94–101. doi: 10.1111/j.1863-2378.2007.01028.x
Kamp, J., Franj, C., Trautmann, S., Busch, M., Dröschmeister, R., Flade, M., et al. (2021). Population trends of common breeding birds in Germany 1990–2018. J. Ornithology 162, 1–15. doi: 10.1007/s10336-020-01830-4
Kampen, H., Rotzel, D. C., Kurtenbach, K., Maier, W. A., Seitz, H. M. (2004). Substantial rise in the prevalence of Lyme borreliosis spirochetes in a region of western Germany over a 10-year period. Appl. Environ. Microbiol. 70, 1576–1582. doi: 10.1128/AEM.70.3.1576-1582.2004
Klaus, C., Gethmann, J., Hoffmann, B., Ziegler, U., Heller, M., Beer, M. (2016). Tick infestation in birds and prevalence of pathogens in ticks collected from different places in Germany. Parasitol. Res. 115, 2729–2740. doi: 10.1007/s00436-016-5022-5
Knoll, S., Springer, A., Hauck, D., Schunack, B., Pachnicke, S., Fingerle, V., et al. (2021a). Distribution of Borrelia burgdorferi s.l. and Borrelia miyamotoi in Ixodes tick populations in Northern Germany, co-infections with Rickettsiales and assessment of potential influencing factors. Med. Vet. Entomol 35, 595–606. doi: 10.1111/mve.12537
Knoll, S., Springer, A., Hauck, D., Schunack, B., Pachnicke, S., Strube, C. (2021b). Regional, seasonal, biennial and landscape-associated distribution of Anaplasma phagocytophilum and Rickettsia spp. infections in Ixodes ticks in northern Germany and implications for risk assessment at larger spatial scales. Ticks Tick Borne Dis. 12, 101657. doi: 10.1016/j.ttbdis.2021.101657
Kohn, B., Silaghi, C., Galke, D., Arndt, G., Pfister, K. (2011). Infections with Anaplasma phagocytophilum in dogs in Germany. Res. Vet. Sci. 91, 71–76. doi: 10.1016/j.rvsc.2010.08.008
Korner, S., Makert, G. R., Mertens-Scholz, K., Henning, K., Pfeffer, M., Starke, A., et al. (2020). Uptake and fecal excretion of Coxiella burnetii by Ixodes ricinus and Dermacentor marginatus ticks. Parasit Vectors 13, 75. doi: 10.1186/s13071-020-3956-z
Korner, S., Makert, G. R., Ulbert, S., Pfeffer, M., Mertens-Scholz, K. (2021). The prevalence of coxiella burnetii in hard ticks in Europe and their role in Q fever transmission revisited-A systematic review. Front. Vet. Sci. 8. doi: 10.3389/fvets.2021.655715
Kowalski, J., Hopfenmuller, W., Fingerle, V., Malberg, H., Eisenblatter, M., Wagner, J., et al. (2006). Seroprevalence of human granulocytic anaplasmosis in Berlin/Brandenburg, Germany: an 8-year survey. Clin. Microbiol. Infect. 12, 924–927. doi: 10.1111/j.1469-0691.2006.01490.x
Kubiak, K., Szczotko, M., Dmitryjuk, M. (2021). Borrelia miyamotoi-an emerging human tick-borne pathogen in Europe. Microorganisms 9, 13. doi: 10.3390/microorganisms9010154
Kurtenbach, K., Kampen, H., Dizij, A., Arndt, S., Seitz, H. M., Schaible, U. E., et al. (1995). Infestation of rodents with larval Ixodes ricinus (Acari: Ixodidae) is an important factor in the transmission cycle of Borrelia burgdorferi s.l. in German woodlands. J. Med. Entomol 32, 807–817. doi: 10.1093/jmedent/32.6.807
Labruna, M. B., Whitworth, T., Horta, M. C., Bouyer, D. H., McBride, J. W., Pinter, A., et al. (2004). Rickettsia species infecting Amblyomma cooperi ticks from an area in the state of Sao Paulo, Brazil, where Brazilian spotted fever is endemic. J. Clin. Microbiol. 42, 90–98. doi: 10.1128/JCM.42.1.90-98.2004
Langenwalder, D. B., Schmidt, S., Silaghi, C., Skuballa, J., Pantchev, N., Matei, I. A., et al. (2020). The absence of the drhm gene is not a marker for human-pathogenicity in European Anaplasma phagocytophilum strains. Parasit Vectors 13, 238. doi: 10.1186/s13071-020-04116-z
Liebisch, A. (1977). Das Q-fieber als naturherdinfektion in süddeutschland. Bundesgesundheitsblatt 20, 185–191.
Lommano, E., Dvorak, C., Vallotton, L., Jenni, L., Gern, L. (2014). Tick-borne pathogens in ticks collected from breeding and migratory birds in Switzerland. Ticks Tick Borne Dis. 5, 871–882. doi: 10.1016/j.ttbdis.2014.07.001
MacQueen, D., Centellas, F. (2022). Human granulocytic anaplasmosis. Infect. Dis. Clin. North Am. 36, 639–654. doi: 10.1016/j.idc.2022.02.008
Mancini, F., Di Luca, M., Toma, L., Vescio, F., Bianchi, R., Khoury, C., et al. (2014). Prevalence of tick-borne pathogens in an urban park in Rome, Italy. Ann. Agric. Environ. Med. 21, 723–727. doi: 10.5604/12321966.1129922
Margos, G., Vollmer, S. A., Cornet, M., Garnier, M., Fingerle, V., Wilske, B., et al. (2009). A new Borrelia species defined by multilocus sequence analysis of housekeeping genes. Appl. Environ. Microbiol. 75, 5410–5416. doi: 10.1128/AEM.00116-09
May, K., Strube, C. (2014). Prevalence of Rickettsiales (Anaplasma phagocytophilum and Rickettsia spp.) in hard ticks (Ixodes ricinus) in the city of Hamburg, Germany. Parasitol. Res. 113, 2169–2175. doi: 10.1007/s00436-014-3869-x
Michelet, L., Delannoy, S., Devillers, E., Umhang, G., Aspan, A., Juremalm, M., et al. (2014). High-throughput screening of tick-borne pathogens in Europe. Front. Cell Infect. Microbiol. 4. doi: 10.3389/fcimb.2014.00103
Norte, A. C., Margos, G., Becker, N. S., Albino Ramos, J., Nuncio, M. S., Fingerle, V., et al. (2020). Host dispersal shapes the population structure of a tick-borne bacterial pathogen. Mol. Ecol. 29, 485–501. doi: 10.1111/mec.15336
Nuttall, P. A. (2022). Climate change impacts on ticks and tick-borne infections. Biologia 77, 1503–1512. doi: 10.1007/s11756-021-00927-2
Obiegala, A., Oltersdorf, C., Silaghi, C., Kiefer, D., Kiefer, M., Woll, D., et al. (2016). Rickettsia spp. in small mammals and their parasitizing ectoparasites from Saxony, Germany. Vet. Parasitol. Reg. Stud. Rep. 5, 19–24. doi: 10.1016/j.vprsr.2016.08.008
Okeyo, M., Hepner, S., Rollins, R. E., Hartberger, C., Straubinger, R. K., Marosevic, D., et al. (2020). Longitudinal study of prevalence and spatio-temporal distribution of Borrelia burgdorferi sensu lato in ticks from three defined habitats in Latvia 1999-2010. Environ. Microbiol. 22, 5033–5047. doi: 10.1111/1462-2920.15100
Olsen, B., Jaenson, T. G., Bergstrom, S. (1995). Prevalence of Borrelia burgdorferi sensu lato-infected ticks on migrating birds. Appl. Environ. Microbiol. 61, 3082–3087. doi: 10.1128/aem.61.8.3082-3087.1995
Panning, M., Kilwinski, J., Greiner-Fischer, S., Peters, M., Kramme, S., Frangoulidis, D., et al. (2008). High throughput detection of Coxiella burnetii by real-time PCR with internal control system and automated DNA preparation. BMC Microbiol. 8, 77. doi: 10.1186/1471-2180-8-77
Pluta, S., Hartelt, K., Oehme, R., Mackenstedt, U., Kimmig, P. (2010). Prevalence of Coxiella burnetii and Rickettsia spp. in ticks and rodents in southern Germany. Ticks Tick Borne Dis. 1, 145–147. doi: 10.1016/j.ttbdis.2010.04.001
Raileanu, C., Silaghi, C., Fingerle, V., Margos, G., Thiel, C., Pfister, K., et al. (2021). Borrelia burgdorferi sensu lato in questing and engorged ticks from different habitat types in southern Germany. Microorganisms 9, 13. doi: 10.3390/microorganisms9061266
Rauer, S., Kastenbauer, S., et al. (2018). Neuroborreliose, S3-Leitlinie (Deutsche Gesellschaft für Neurologie). Available online at: www.dgn.org/leitlinien (Accessed 04.03.2024).
Rauter, C., Oehme, R., Diterich, I., Engele, M., Hartung, T. (2002). Distribution of clinically relevant Borrelia genospecies in ticks assessed by a novel, single-run, real-time PCR. J. Clin. Microbiol. 40, 36–43. doi: 10.1128/JCM.40.1.36-43.2002
Richter, D., Schlee, D. B., Matuschka, F. R. (2003). Relapsing fever-like spirochetes infecting European vector tick of Lyme disease agent. Emerg. Infect. Dis. 9, 697–701. doi: 10.3201/eid0906.020459
Roux, V., Raoult, D. (2000). Phylogenetic analysis of members of the genus Rickettsia using the gene encoding the outer-membrane protein rOmpB (ompB). Int. J. Syst. Evol. Microbiol. 50 Pt 4, 1449–1455. doi: 10.1099/00207713-50-4-1449
Scarpulla, M., Barlozzari, G., Salvato, L., De Liberato, C., Lorenzetti, R., Macri, G. (2018). Rickettsia helvetica in Human-Parasitizing and Free-Living Ixodes ricinus from Urban and Wild Green Areas in the Metropolitan City of Rome, Italy. Vector Borne Zoonotic Dis. 18, 404–407. doi: 10.1089/vbz.2017.2235
Schafer, I., Kohn, B., Muller, E. (2022a). Anaplasma phagocytophilum in domestic cats from Germany, Austria and Switzerland and clinical/laboratory findings in 18 PCR-positive cats, (2008-2020). J. Feline Med. Surg. 24, 290–297. doi: 10.1177/1098612X211017459
Schafer, I., Kohn, B., Silaghi, C., Fischer, S., Marsboom, C., Hendrickx, G., et al. (2023). Molecular and serological detection of anaplasma phagocytophilum in dogs from Germany, (2008-2020). Anim. (Basel) 13. doi: 10.3390/ani13040720
Schafer, I., Silaghi, C., Fischer, S., Marsboom, C., Hendrickx, G., Gehlen, H., et al. (2022b). Detection of Anaplasma phagocytophilum in horses from Germany by molecular and serological testing, (2008-2021). Vet. Parasitol. 312, 109840. doi: 10.1016/j.vetpar.2022.109840
Scheid, P., Speck, S., Schwarzenberger, R., Litzinger, M., Balczun, C., Dobler, G. (2016). Detection of Rickettsia helvetica in Ixodes ricinus infesting wild and domestic animals and in a botfly larva (Cephenemyia stimulator) infesting roe deer in Germany. Ticks Tick Borne Dis. 7, 1268–1273. doi: 10.1016/j.ttbdis.2016.07.002
Schwarz, A., Honig, V., Vavruskova, Z., Grubhoffer, L., Balczun, C., Albring, A., et al. (2012). Abundance of Ixodes ricinus and prevalence of Borrelia burgdorferi s.l. in the nature reserve Siebengebirge, Germany, in comparison to three former studies from 1978 onwards. Parasit Vectors 5, 268. doi: 10.1186/1756-3305-5-268
Silaghi, C., Hamel, D., Thiel, C., Pfister, K., Pfeffer, M. (2011). Spotted fever group rickettsiae in ticks, Germany. Emerg. Infect. Dis. 17, 890–892. doi: 10.3201/eid1705.101445
Sinski, E., Welc-Faleciak, R., Zajkowska, J. (2016). Borrelia miyamotoi: A human tick-borne relapsing fever spirochete in Europe and its potential impact on public health. Adv. Med. Sci. 61, 255–260. doi: 10.1016/j.advms.2016.03.001
Široký, P., Kubelová, M., Modrý, D., Erhart, J., Literák, I., Špitalská, E., et al. (2010). Tortoise tick Hyalomma aEgyptium as long term carrier of Q fever agent Coxiella burnetii—evidence from experimental infection. Parasitol. Res. 107, 1515–1520. doi: 10.1007/s00436-010-2037-1
Sprong, H., Wielinga, P. R., Fonville, M., Reusken, C., Brandenburg, A. H., Borgsteede, F., et al. (2009). Ixodes ricinus ticks are reservoir hosts for Rickettsia helvetica and potentially carry flea-borne Rickettsia species. Parasit Vectors 2, 41. doi: 10.1186/1756-3305-2-41
Strnad, M., Honig, V., Ruzek, D., Grubhoffer, L., Rego, R. O. M. (2017). Europe-Wide Meta-Analysis of Borrelia burgdorferi Sensu Lato Prevalence in Questing Ixodes ricinus Ticks. Appl. Environ. Microbiol. 83, 16. doi: 10.1128/AEM.00609-17
Strube, C., Montenegro, V. M., Epe, C., Eckelt, E., Schnieder, T. (2010). Establishment of a minor groove binder-probe based quantitative real time PCR to detect Borrelia burgdorferi sensu lato and differentiation of Borrelia spielmanii by ospA-specific conventional PCR. Parasit Vectors 3, 69. doi: 10.1186/1756-3305-3-69
Stuen, S., Granquist, E. G., Silaghi, C. (2013). Anaplasma phagocytophilum–a widespread multi-host pathogen with highly adaptive strategies. Front. Cell Infect. Microbiol. 3. doi: 10.3389/fcimb.2013.00031
Szekeres, S., Lugner, J., Fingerle, V., Margos, G., Foldvari, G. (2017). Prevalence of Borrelia miyamotoi and Borrelia burgdorferi sensu lato in questing ticks from a recreational coniferous forest of East Saxony, Germany. Ticks Tick Borne Dis. 8, 922–927. doi: 10.1016/j.ttbdis.2017.08.002
Szymańska-Czerwińska, M., Galińska, E. M., Niemczuk, K., Zasępa, M. (2013). Prevalence of Coxiella burnetii infection in foresters in the south-eastern Poland and comparison of diagnostic methods. Ann. Agric. Environ. Med. 20, 699–704.
Tappe, J., Jordan, D., Janecek, E., Fingerle, V., Strube, C. (2014). Revisited: Borrelia burgdorferi sensu lato infections in hard ticks (Ixodes ricinus) in the city of Hanover (Germany). Parasites Vectors 7, 441. doi: 10.1186/1756-3305-7-441
Tappe, J., Strube, C. (2013). Anaplasma phagocytophilum and Rickettsia spp. infections in hard ticks (Ixodes ricinus) in the city of Hanover (Germany): revisited. Ticks Tick Borne Dis. 4, 432–438. doi: 10.1016/j.ttbdis.2013.04.009
Taragel’ova, V., Koci, J., Hanincova, K., Kurtenbach, K., Derdakova, M., Ogden, N. H., et al. (2008). Blackbirds and song thrushes constitute a key reservoir of Borrelia garinii, the causative agent of borreliosis in Central Europe. Appl. Environ. Microbiol. 74, 1289–1293. doi: 10.1128/AEM.01060-07
R Core Team (2021). “R: A language and environment for statistical computing.” (Vienna, Austria: R Foundation for Statistical Computing). R.C. Team.
Tomanovic, S., Chochlakis, D., Radulovic, Z., Milutinovic, M., Cakic, S., Mihaljica, D., et al. (2013). Analysis of pathogen co-occurrence in host-seeking adult hard ticks from Serbia. Exp. Appl. Acarol 59, 367–376. doi: 10.1007/s10493-012-9597-y
Van Heuverswyn, J., Hallmaier-Wacker, L. K., Beaute, J., Gomes Dias, J., Haussig, J. M., Busch, K., et al. (2023). Spatiotemporal spread of tick-borne encephalitis in the EU/EEA 2012 to 2020. Euro Surveill 28, 12. doi: 10.2807/1560-7917.ES.2023.28.11.2200543
Vanwambeke, S. O., Lambin, E. F., Meyfroidt, P., Asaaga, F. A., Millins, C., Purse, B. V. (2024). Land system governance shapes tick-related public and animal health risks. J. Land Use Sci. 19, 78–96. doi: 10.1080/1747423X.2024.2330379
Venczel, R., Knoke, L., Pavlovic, M., Dzaferovic, E., Vaculova, T., Silaghi, C., et al. (2016). A novel duplex real-time PCR permits simultaneous detection and differentiation of Borrelia miyamotoi and Borrelia burgdorferi sensu lato. Infection 44, 47–55. doi: 10.1007/s15010-015-0820-8
Vogerl, M., Zubrikova, D., Pfister, K. (2012). Prevalence of Borrelia burgdorferi s. l. in Ixodes ricinus ticks from four localities in Bavaria, Germany. Berl Munch Tierarztl Wochenschr 125, 401–406.
Vögerl, M., Zubrikova, D., Pfister, K. (2012). Prevalence of Borrelia burgdorferi s. l. in Ixodes ricinus ticks from four localities in Bavaria, Germany. Berliner und Münchener Tierärztliche Wochenschrift 125, 401–406. doi: 10.2376/0005-9366-125-401
Wachter, M., Wolfel, S., Pfeffer, M., Dobler, G., Kohn, B., Moritz, A., et al. (2015). Serological differentiation of antibodies against Rickettsia helvetica, R. raoultii, R. slovaca, R. monacensis and R. felis in dogs from Germany by a micro-immunofluorescent antibody test. Parasit Vectors 8, 126. doi: 10.1186/s13071-015-0745-1
Weisburg, W. G., Barns, S. M., Pelletier, D. A., Lane, D. J. (1991). 16S ribosomal DNA amplification for phylogenetic study. J. Bacteriol 173, 697–703. doi: 10.1128/jb.173.2.697-703.1991
Wilking, H., Beermann, S., Boone, I., Dreesman, J., Fingerle, V., Gethmann, J., et al. (2023). [Bacterial zoonoses of public health importance in Germany-incidence, distribution, and modes of transmission]. Bundesgesundheitsblatt Gesundheitsforschung Gesundheitsschutz 66, 617–627. doi: 10.1007/s00103-023-03703-6
Wilske, B., Steinhuber, R., Bergmeister, H., Fingerle, V., Schierz, G., Preac-Mursic, V., et al. (1987). [Lyme borreliosis in South Germany. Epidemiologic data on the incidence of cases and on the epidemiology of ticks (Ixodes ricinus) carrying Borrelia burgdorferi]. Dtsch Med. Wochenschr 112, 1730–1736. doi: 10.1055/s-2008-1068320
Wimbauer, M., Bakkes, D. K., Wolfel, S., Broker, M., Schaper, S., Riess, R., et al. (2022). Rickettsia spp. in ticks (Acari: Ixodidae) from wild birds: First detection of Candidatus Rickettsia vini in Hesse, Germany. Ticks Tick Borne Dis. 13, 101908. doi: 10.1016/j.ttbdis.2022.101908
Wolcott, K. A., Margos, G., Fingerle, V., Becker, N. S. (2021). Host association of Borrelia burgdorferi sensu lato: A review. Ticks Tick Borne Dis. 12, 101766. doi: 10.1016/j.ttbdis.2021.101766
Woldehiwet, Z. (2006). Anaplasma phagocytophilum in ruminants in Europe. Ann. N Y Acad. Sci. 1078, 446–460. doi: 10.1196/annals.1374.084
Wolfel, R., Essbauer, S., Dobler, G. (2008). Diagnostics of tick-borne rickettsioses in Germany: A modern concept for a neglected disease. Int. J. Med. Microbiol. 298, 6. doi: 10.1016/j.ijmm.2007.11.009
Wolfel, S., Speck, S., Essbauer, S., Thoma, B. R., Mertens, M., Werdermann, S., et al. (2017). High seroprevalence for indigenous spotted fever group rickettsiae in forestry workers from the federal state of Brandenburg, Eastern Germany. Ticks Tick Borne Dis. 8, 132–138. doi: 10.1016/j.ttbdis.2016.10.009
Wolfel, R., Terzioglu, R., Kiessling, J., Wilhelm, S., Essbauer, S., Pfeffer, M., et al. (2006). Rickettsia spp. in Ixodes ricinus ticks in Bavaria, Germany. Ann. N Y Acad. Sci. 1078, 509–511. doi: 10.1196/annals.1374.133
Keywords: Borreliella spp., Rickettsia spp., Anaplasma phagocytophilum, Coxiella burnetii, Ixodes ricinus, prevalence
Citation: Mertens-Scholz K, Hoffmann B, Gethmann JM, Brangsch H, Pletz MW and Klaus C (2024) Prevalence of tick-borne bacterial pathogens in Germany—has the situation changed after a decade? Front. Cell. Infect. Microbiol. 14:1429667. doi: 10.3389/fcimb.2024.1429667
Received: 08 May 2024; Accepted: 25 June 2024;
Published: 18 July 2024.
Edited by:
Ceferino M. López Sández, University of Santiago de Compostela, SpainReviewed by:
Benjamin Cull, University of Minnesota Twin Cities, United StatesJana Mojzisova, University of Veterinary Medicine and Pharmacy in Košice, Slovakia
Copyright © 2024 Mertens-Scholz, Hoffmann, Gethmann, Brangsch, Pletz and Klaus. This is an open-access article distributed under the terms of the Creative Commons Attribution License (CC BY). The use, distribution or reproduction in other forums is permitted, provided the original author(s) and the copyright owner(s) are credited and that the original publication in this journal is cited, in accordance with accepted academic practice. No use, distribution or reproduction is permitted which does not comply with these terms.
*Correspondence: Katja Mertens-Scholz, a2F0amEubWVydGVucy1zY2hvbHpAZmxpLmRl