- 1Department of Food Science and Nutrition, The Hong Kong Polytechnic University, Hong Kong, Hong Kong SAR, China
- 2Department of Health Technology and Informatics, The Hong Kong Polytechnic University, Hong Kong, Hong Kong SAR, China
- 3Research Centre for Chinese Medicine Innovation, The Hong Kong Polytechnic University, Hong Kong, Hong Kong SAR, China
- 4Department of Clinical Pathology, Pamela Youde Nethersole Eastern Hospital, Hong Kong, Hong Kong SAR, China
- 5AI Farming Company (Hong Kong) Limited, Hong Kong, Hong Kong SAR, China
- 6Research Institute for Future Food, The Hong Kong Polytechnic University, Hong Kong, Hong Kong SAR, China
- 7NICM Health Research Institute, Western Sydney University, Penrith, NSW, Australia
Introduction: Vibrio alginolyticus is a Gram-negative, rod-shaped bacterium belonging to the family of Vibrionaceae, a common pathogen in aquaculture animals, However, studies on its impact on Scylla serrata (mud crabs) are limited. In this study, we isolated V. alginolyticus SWS from dead mud crab during a disease outbreak in a Hong Kong aquaculture farm, which caused up to 70% mortality during summer.
Methods: Experimental infection and histopathology were used to investigate the pathogenicity of V. alginolyticus SWS in S. serrata and validate Koch’s postulates. Comprehensive whole-genome analysis and phylogenetic analysis antimicrobial susceptibility testing, and biochemical characterization were also performed.
Results: Our findings showed that V. alginolyticus SWS caused high mortality (75%) in S. serrata with infected individuals exhibiting inactivity, loss of appetite, decolored and darkened hepatopancreas, gills, and opaque muscle in the claw. Histopathological analysis revealed tissue damage and degeneration in the hepatopancreas, gills, and claw muscle suggesting direct and indirect impacts of V. alginolyticus SWS infection.
Conclusions: This study provides a comprehensive characterization of V. alginolyticus SWS as an emerging pathogen in S. serrata aquaculture. Our findings underscore the importance of ongoing surveillance, early detection, and the development of targeted disease management strategies to mitigate the economic impact of vibriosis outbreaks in mud crab aquaculture.
1 Introduction
Mud crabs is a collective term referring to four different crabs’ species, namely, Scylla serrata, Scylla olivacea, Scylla paramamosain, and Scylla tranquebarica, which belong to the family Portunidae (Fazhan et al., 2017). Among these species, S. serrata is the most widely distributed species of mud crabs and is commonly cultivated (Paran et al., 2022). They inhabit estuaries and mangrove areas and are regarded as one of the most popular and valuable seafood globally due to their nutritional value, palatability, and high market demand (Bhuiyan et al., 2021). In recent decades, the demand for mud crabs has continuously increased in the world, coinciding with a decline in natural population due to overfishing. Hence, aquaculture has become an increasingly prevalent means of meeting the global demand, with worldwide production of mud crabs rising from 10.7 kt in 2000 to 248.8 kt in 2020 (FAO, 2022). While the aquaculture industry for mud crabs is expected to continue growing in response to the market demand, sustainability and profitability are hindered by high mortality rate due to cannibalism and diseases (Alberts-Hubatsch et al., 2016; Ly Van et al., 2022). The management of disease outbreak in Scylla spp. aquaculture remains a challenging task for the industry; the emergence of new diseases, highly contagious characteristics, and pathogen resistance to drugs can lead to substantial economic loss (Hu et al., 2021; Apine et al., 2023). Therefore, it is crucial to study the pathogenicity and profile of pathogens in Scylla spp. especially in aquaculture.
Currently, there are approximately 30 documented infectious diseases found to afflict mud crab caused by bacteria, viruses, parasites, and fungi (Jithendran et al., 2011). Among them, viral and bacterial infections are the most common with typically pathogens, such as the white spot syndrome virus and chitin-digesting bacteria, including Vibrio spp., Aeromonas spp., Pseudomonas spp., and Spirillium spp (Jithendran et al., 2011). Notably, several Vibrio species, including V. vulnificus, V. splendidus, V. orientalis, V. alginolyticus, V. harveyi, and V. parahemolyticus, have been reported to cause high mortality in Scylla spp. due to shell disease and milky disease (Lavilla-Pitogo et al., 2001; Sarjito et al., 2018; Gunasekaran et al., 2019; Cheng et al., 2020). However, while most studies investigating diseases of mud crab focus on V. parahemolyticus and white spot syndrome virus, the pathogenicity of V. alginolyticus in mud crab, particularly S. serrata, remains underexplored.
V. alginolyticus is a species of Gram-negative, rod-shaped bacteria belonging to the family Vibrionaceae. It is the second most common Vibrio species found in coastal, marine, and aquatic environments (Yu et al., 2022). Increasing number of studies have reported that V. alginolyticus is a pathogen affecting a variety of aquatic animals, including fish, shrimp, and oyster, resulting in high mortality and substantial economic loss in aquaculture (George et al., 2005; Cao et al., 2018; Yang et al., 2021). V. alginolyticus is also well known to cause seafood poisoning and systemic infection in humans (Li et al., 2009; Hong et al., 2014). However, in the context of mud crab, V. alginolyticus has only been recognized for causing shell disease, and the pathogenicity and characteristics of V. alginolyticus isolated from S. serrata (mud crab) have not been well studied (Gunasekaran et al., 2019).
Recently, S. serrata aquaculture in Hong Kong experienced a significant mortality rate, with up to 70% of crabs dying during the summer period due to unknown causes. Site investigations found that all of the water parameters on the farm were normal, and the use of antibiotics was strictly prohibited according to farm practices. Based on these observations, we hypothesized that the outbreak might be caused by a bacterial agent. In this study, pure V. alginolyticus SWS was isolated from a crab that died on the aquaculture. Experimental infection was then conducted on S. serrata to assess its impact on mortality, pathogenicity, and disease manifestation. In addition, to characterize this strain of bacterium, we performed whole-genome sequencing (WGS), biochemical tests, and antibiotic susceptibility test (AST). Results from our crab infection model indicate that V. alginolyticus SWS is a strain of deadly pathogen to S. serrata causing infected crabs to become inactive, lose appetite, and lose normal appearance of the muscle, gills, and hepatopancreas, and induce tissue damage and degeneration in the hepatopancreas, gills, and claw muscle in the infected crab. Our current study provides useful information for disease surveillance and formulation of effective disease prevention measurements in mud crab aquaculture.
2 Materials and methods
2.1 Bacterial isolation
Vibrio species were isolated from two diseased S. serrata supplied by AI Farming Company (Hong Kong) Limited. Samples were swabbed from the inner shell of the diseased mud crab into sterilized phosphate-buffered saline (PBS) followed by streaking on LB agar with 3% NaCl and incubated at 37°C for 24 h. After 24h, a single colony was picked and further streaked on trypticase soy agar (TSA) with 8% NaCl at 37°C for 24 h. Eventually, a single colony was picked and further streaked on thiosulfate–citrate–bile salt–sucrose (TCBS) agar. The pure isolates were either routinely sub-cultured for sequent analysis or stored at −80°C in tryptic soy broth (TSB) with an 8% salt supplement containing 15% glycerol. The isolated colonies from two disease crabs were tentatively identified by mass spectrometry matrix-assisted laser desorption ionization-time of flight (MALDI-TOF) mass spectrometry system (Microflex LT with MALDI Biotyper Sirius one RUO system, Bruker Daltonics, Bremen, Germany) equipped with FlexControl software, version 3.3 (Bruker Daltonics) MALDI‐TOF (2.4.2), and their morphology was also observed.
2.2 Experimental infection
Eighteen healthy mud crab S. serrata (112.5 ± 17.7 g) were kindly provided by AI Farming Company (Hong Kong) Limited. Crabs were maintained in 22–23 ppt (part per thousand) brackish water with 6.8–7.2 pH at 26 ± 2°C in AI farming. Before challenging with bacterial isolate, crabs were acclimatized in 23 ppt brackish water for 30 min. The crabs were divided into two groups, the infection group, and the control group, consisting of 8 and 10 mud crabs, respectively. They were maintained in two plastic tanks (100 L, 117 × 43 × 20 cm3) with 70 L of filtered artificial seawater at 21.2–23 ppt salinity at 23°C, proper aeration, and 12h light/dark cycle. Crabs were fed with commercial pellet feed and fish meat, approximately 10% of their weight. The infection group was injected with 500 μl of bacterial suspension in sterile 0.85% saline with a final concentration of 2.5 × 105 CFU/ml at the base of their right fourth walking leg using a 25G needle. Similarly, the control group was injected with 500 μl of 0.85% sterile saline. The mortalities and symptoms were monitored daily for 7 days. V. alginolyticus SWS was re-isolated from the inner crab shell and hemolymph in infected crab, but not found in healthy control crab, which meets the criteria of Koch’s postulates. Hepatopancreases, gills, and muscles from claws were collected for histology.
2.3 Histopathological examination
The tissues (hepatopancreas, gill, and claw muscle) of infected crabs and a control crab were subjected to routine crustacean tissue-processing procedures adapted from “Histological techniques for marine bivalve mollusks and crustaceans, second edition” (Howard, 2004). In short, the tissues collected were first fixed in 40% formalin followed by storage in 75% ethanol. Then, the tissues were dissected to an appropriate thickness of approximately 0.5 cm and subjected to automated tissue processing under vacuum using Excelsior AS Tissue Processor (Epredia). During the tissue processing, the tissues were dehydrated by a series of graded ethanol, cleared by three changes of xylene, and infiltrated with three changes of paraffin wax, followed by manual embedding. The blocks were sectioned at 6 µm, and then hematoxylin and eosin (H&E) staining was performed on the sections to visualize histological details under light microscopy using Eclipse Ci Microscope (Nikon) with different magnifications (×100, ×200, and ×400).
2.4 Molecular biological species identification
2.4.1 Bacterial genomic DNA extraction and purification
Prior to genomic DNA (gDNA) extraction for WGS, four single colonies from each TCBS agars were picked to have tentative identification using MALDI Biotyper (RUO) MALDI-TOF Mass Spectrometry Based Microorganism Identification System (Bruker Daltonics, USA). After that, tentative identified single colonies were picked, and Genomic DNA was extracted and purified from it using a QIAamp® BiOstic® Bacteremia DNA kit (Qiagen, Valencia, CA) with reference to the manufacturer’s protocol. The concentration of the purified genomic DNA was determined using a NanoDrop One Microvolume UV-Vis Spectrophotometer (Thermo Scientific, USA). All DNA was stored at −80°C prior to further analysis.
2.4.2 Tentative identification using mass spectrometry MALDI‐TOF
For bacterial identification, colonies freshly picked from TCBS agar, isolated from deceased mud crabs, were utilized. Each colony was mixed with 1 µl of matrix solution and subsequently dried on a stainless-steel target plate. Identification was performed using MALDI-TOF. The acquired spectra were analyzed and compared against the Bruker Biotyper database using the MBT Compass Library (version 12.0.0.0).
2.4.3 Genome sequencing and bioinformatics analysis (annotation of genome assembly)
Bacterial whole-genome sequencing of V. alginolyticus SWS was sent to Azenta Life Sciences to perform sequencing by Illumina NovaSeq 6000 in PE150 mode. The Raw DNA-Seq data were deposited to the US National Center for Biotechnology Information (NCBI) database under accession number PRJNA1101690. For construction of complete-genome sequence, the qualified DNA reads were assembled using the Assembly RAST (ARAST) service with auto mode in Pathosystems Resource Integration Center (PATRIC) server comprehensive genome analysis function (Wattam et al., 2016). The genome Annotation was performed by Rapid Annotation using Subsystem Technology (RAST), which is annotated using genetic code 11. Subsystem Analysis of the genomic was also performed using PATRIC. Specialty genes, including transporter virulence factors, drug targets, and antibiotic resistance genes were also annotated with reference to specific source databases using PATRIC. Antimicrobial resistance (AMR) genes was also predicted by utilizing k-mer-based AMR gene detection method in PATRIC, while a virulence factors database (http://www.mgc.ac.cn/VFs/main.htm) was used to predict the virulence factors in V. alginolyticus SWS (Liu et al., 2019, 2022). Phylogenetic analysis was also performed with the aid of PATRIC that selects the closest reference and representative genomes by Mash/MinHash algorithm; PATRIC global protein families were selected to determine the phylogenetic placement of the isolated genome. The protein sequences were aligned with MUSCLE algorithm (multiple sequence alignment with high accuracy and high throughput), and the nucleotides for each of those sequences were mapped to the protein alignment. The joint set of amino acid and nucleotide alignments were concatenated into a data matrix, and Randomized Axelerated Maximum Likelihood (RaxML) was used to analyze the matrix with fast bootstrapping method. Genome similarity was studied by calculating the pairwise average nucleotide identity (ANI) based on BLAST+ (ANIb) and MUMmer (ANIm) using JSpeciesWS (version 4.1.1, https://jspecies.ribohost.com/jspeciesws/#home) (Richter et al., 2015).
2.5 Characterization of isolated bacteria
2.5.1 Salt tolerance test
A single colony of V. alginolyticus SWS was picked from freshly streaked TCBS and inoculated into nutrient broth (NB) supplementary with different percentages of salt, 0%, 1%, 6%, and 8% at 37°C, for 24 h for evaluating the salt requirements of crab isolate.
2.5.2 Antimicrobial susceptibilities
Antibiotic susceptibility testing was conducted on V. alginolyticus SWS using the disk diffusion method, as per the guidelines established by the Clinical and Laboratory Standards Institute (CLSI). The test employed commercially available antibiotic disks from Oxoid (UK) and Liofilchem (Italy). A fresh isolate from crab was prepared by inoculation into 0.85% saline solution to match a 0.5-McFarland standard turbidity. This suspension was uniformly streaked onto Mueller–Hinton agar plates. Subsequently, antibiotic discs were applied to the inoculated plates. The antibiotics tested included cefoxitin (FOX, 30 µg), ceftriaxone (CRO, 30 µg), cefepime (FEP, 30 µg), ampicillin (AMP,10 µg), chloramphenicol (C, 30 µg), ciprofloxacin (CIP, 5 µg), colistin (CS, 10 µg), gentamycin (CN, 10 µg), nalidixic acid (NA, 30 µg), meropenem (MEM, 10 µg), imipenem (IMP, 10 µg), ertapenem (EPT, 10 µg), doripenem (DOR, 10 µg), tetracyclin (TE, 30 µg), trimethoprim/sulfamethoxazole (SXT, 25 µg), cefotaxime (CTX, 30µg), cefotaxime + clavulanic acid (CTL, 30 + 10 = 40 µg), ceftazidime (CAZ) (30 µg), ceftazidime + clavulanic acid (CAL, 30 + 10 = 40 µg), and amoxicillin–clavulanic acid (AUG/AMC, 30 µg). The inoculated plates were incubated at 35 ± 2°C for 16–18 h. Post-incubation, zones of inhibition were measured and interpreted using the BIOMIC V3 Microbiology System (Giles Scientific, USA), with reference values from both CLSI and the European Committee on Antimicrobial Susceptibility Testing (EUCAST).
2.5.3 Metabolic/biochemical characterization
Vitek 2 Compact (bioMérieux. Marcy l’Etoile, France) Gram-Negative Identification (GNI) cards were used to determine the metabolic/biochemical characterization of crab isolate with reference to the manufacturer’s guideline. In brief, three to four fresh colonies were suspended in sterilized 0.85% saline and thoroughly mixed to obtain 0.5 McFarland standard. A 5-μl suspension of crab isolate was loaded into Vitek 2 GNI cards to perform 48 biochemical tests. The VITEK-2 system reported the results automatically with installed version 9.02.3.
2.5.4 Optimal temperature and pH
The optimal growth condition of V. alginolyticus SWS, in terms of temperature and pH, was determined using the method of Norfolks et al. with slight adjustment (Norfolk et al., 2023). The isolate was subcultured from the TSB stored at –80°C (Section 2.1) at least twice. Then, a single colony of the isolate was inoculated into approximately 5 ml of LB broth with 3% NaCl (w/v) (LBS), followed by a 4h shaking incubation at 230 rpm and 37°C to reach stationary phases. After that, the culture was diluted by 10-fold to OD600 of approximately 0.1. Then, 2 µl of the culture was added to 148 µl of LBS with pH adjusted to 4, 5, 6, 7, 8, 9, and 10 ( ± 0.1) in a 96-well plate. The bacterial growth at different pH values under 26°C, 30°C, and 37°C was measured in terms of OD600 at regular intervals of 15 min for 14 h. The readings of OD600 were subjected to analysis using Dashing Growth Curves (Reiter and Vorholt, 2024). The readings were blanked using the average OD600 values of three wells with 150 µl of LBS at each temperature. The tight logistic model was used for growth curve fitting and computational determination of the exponential phase, while the growth curve was smoothed using rolling windows of 10. The application returned the doubling time for each well, which was then used to determine the optimal growth condition.
3 Results
3.1 Clinical signs of the naturally infected mud crab from a local crab farm
The clinical signs of infected S. serrata from aquaculture were observed after dissection. Infected S. serrata had a darkened hepatopancreas with flaccid and atrophied appearance and decolorized gills (Figure 1A).
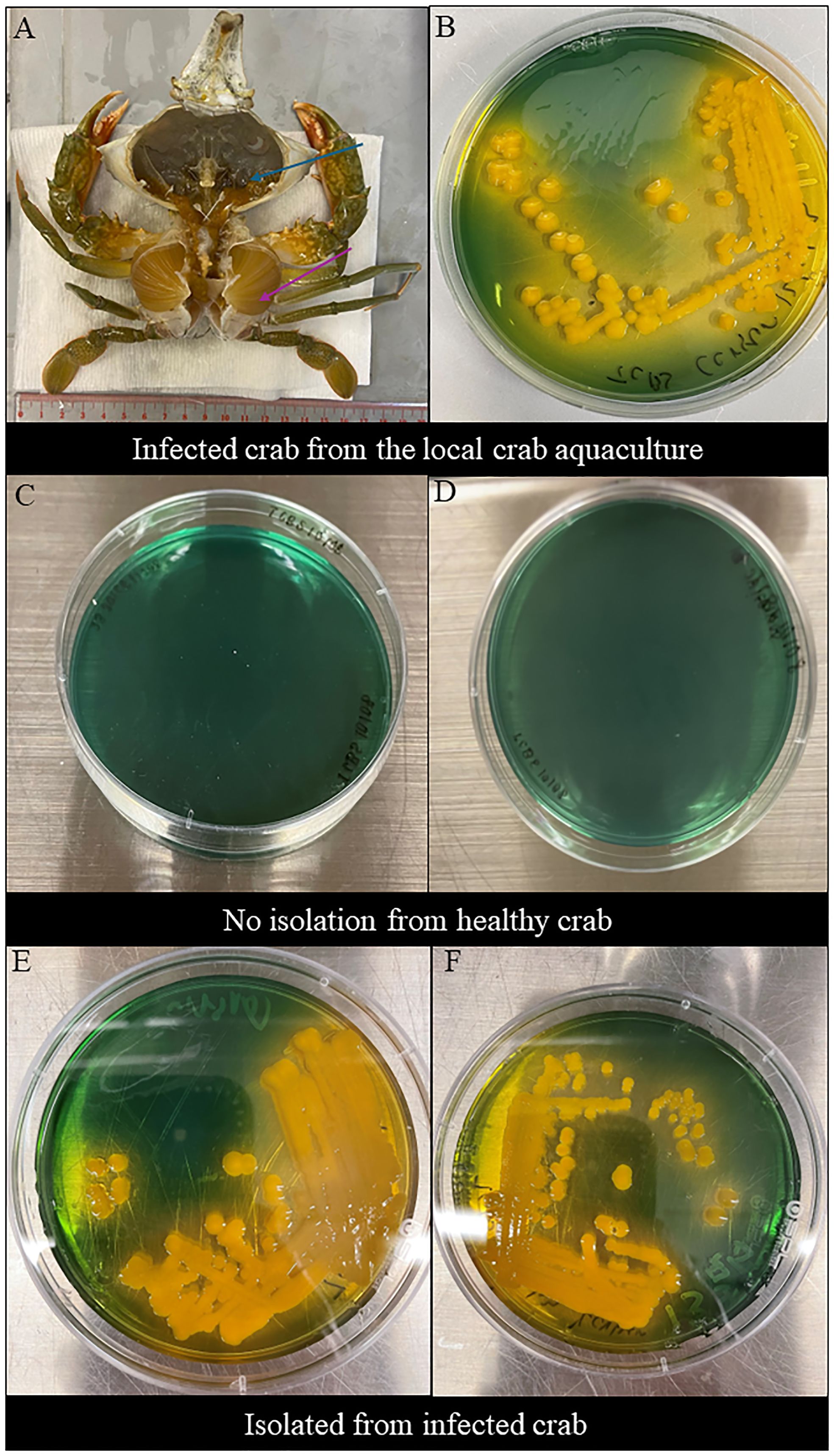
Figure 1 Clinical sign of infected mud crab and morphological observations in TCBS agar. (A) Clinical sign of naturally infected S. serrata from the mud crab farm with decolorized darkened hepatopancreas with flaccid and atrophied appearance (blue arrow) and decolorized gills (purple arrow). (B) Morphological observations of V. alginolyticus SWS isolated from infected Scylla serrata from the local crab aquaculture formed large, round, mucoid, convex, yellow colonies in TCBS. (C, D) No Vibrio isolated from the inner crab shell and hemolymph of healthy crabs. (E, F) Morphological observations of V. alginolyticus SWS reisolated from the inner crab shell in TCBS agar showed the formation of large, round, mucoid, convex, yellow colonies (E) and hemolymph (F) of V. alginolyticus SWS-infected crabs.
3.2 Morphological observation of isolated bacteria and tentative identification
V. alginolyticus SWS formed large, round, mucoid, convex, yellow colonies on TCBS agar (Figure 1B), which indicated that they could ferment sucrose.
The tentative identification of this V. alginolyticus SWS was performed by MALDI-TOF. Results revealed that the bacteria isolated from two dead crabs probably were Vibrio genus, although the species identification was identified as V. alginolyticus. The score value was arranged from 1.82 to 1.99 implying the species identification is in low confidence, which required a method with higher differentiating power (Mougin et al., 2020).
Swabs were retrieved from the inner shell of infected and healthy mud crab and their hemolymph to isolate bacteria. No bacteria were isolated from healthy crabs (Figures 1C, D), while V. alginolyticus SWS were again isolated from experimentally infected crabs (Figures 1E, F). These imply that the bacteria used in the infection experiment was the causative infectious agents and met the criteria of Koch’s postulates suggesting that V. alginolyticus SWS is a pathogen to S. serrata.
3.3 V. alginolyticus SWS in S. serrata infection model
3.3.1 Mud crab survival
The 7 days survival curve of mud crabs challenged with or without V. alginolyticus SWS is illustrated in Figure 2A. Throughout the experimental infections, the control/mock crab (PBS group) remained healthy, while the infected crab began to die after 1 day of V. alginolyticus SWS injection and reached a 75% cumulative mortality rate on day 4 (Figure 2A). This suggested that V. alginolyticus SWS was highly pathogenic to S. serrata.
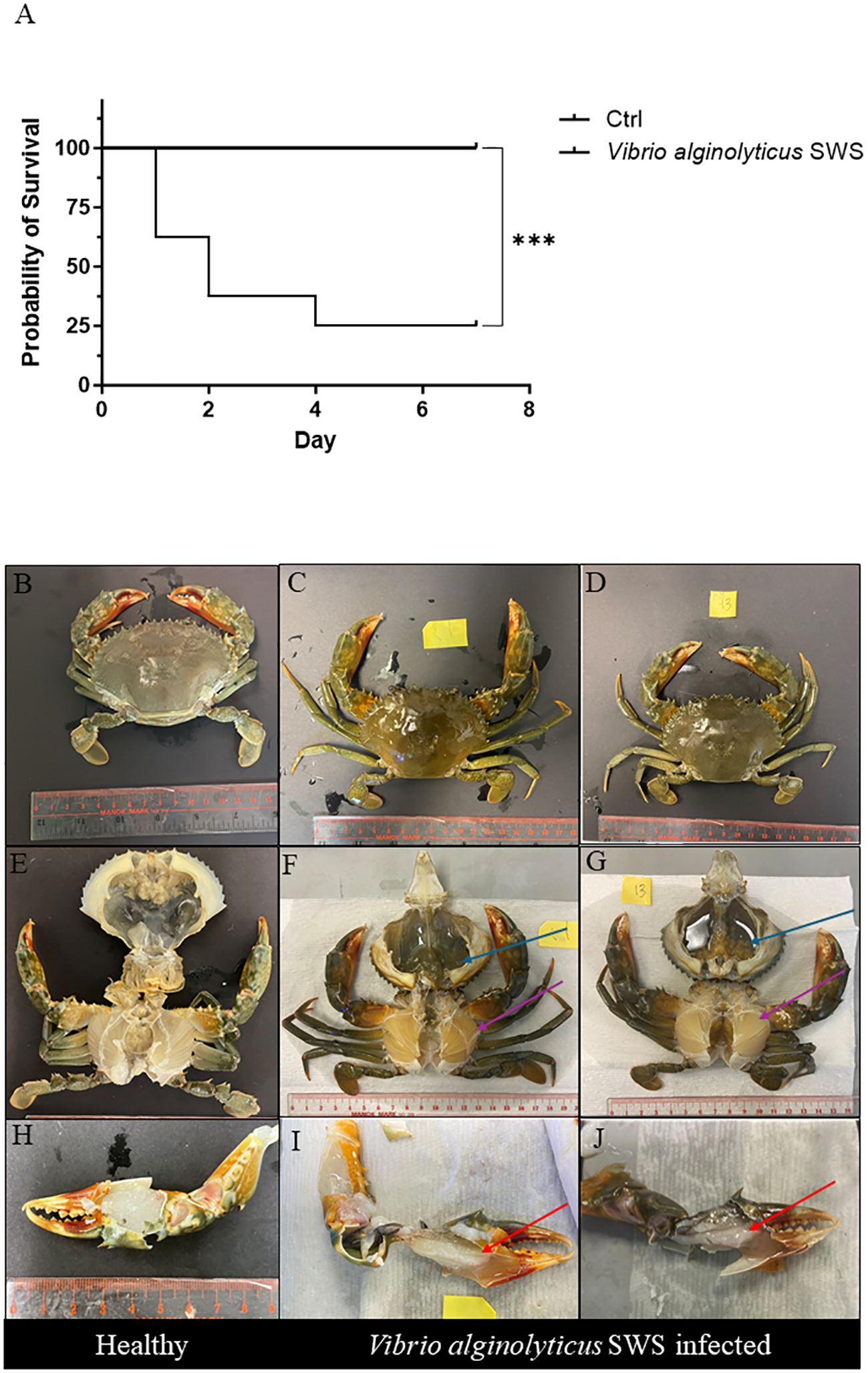
Figure 2 Effect of Vibrio alginolyticus SWS on survival and induced symptoms in S. serrata. (A) Survival curve of mud crab in the experimental infection of V. alginolyticus SWS (infected group = 8, control = 10); log-rank (Mantel–Cox) test was used to compared the difference between two groups, ***p < 0.001 versus control. (B–D) Comparison between the exoskeleton of healthy mud crab (B) and V. alginolyticus SWS-infected mud crab (C, D). (E) Healthy crab without clinical signs. (F, G) V. alginolyticus SWS-infected S. serrata with decolorized darkened hepatopancreas with flaccid and atrophied appearance (blue arrow) and decolorized gills (purple arrow). (H) Healthy S. serrata with a transparent white muscle in the claw. (I, J) V. alginolyticus SWS-infected S. serrata with opaque white muscle in the claw (red arrow).
3.3.2 Clinical signs of diseased mud crab
Infected S. serrata was monitored daily; visual inspection on exoskeleton and necropsy was carried on infected mud crab and healthy mud crab to observe lesions. Infected crabs had low appetite and were inactive before their death. There was no obvious lesion on the external shell of infected mud crabs (Figures 2C–F) and control crabs (Figure 2B). In contrast, morphological observations from necropsy of both healthy crabs (Figure 2E) and infected crabs (Figures 2F, G) showed that infected crabs had decolorized and darkened hepatopancreas with flaccid and atrophied appearance (dark green, Figure 2F; pale brown, Figure 2G) and decolorized gills (Figures 2F, G). When comparing the muscle in the claw of healthy crabs (Figure 2H), infected crabs had an opaque muscle with milky appearance (Figures 2I, J).
3.3.3 Histopathology of infected S. serrata
Histopathology was performed to study the impact of V. alginolyticus SWS on S. serrata. The impact of V. alginolyticus SWS infection on the gills of crabs was obvious. In healthy crabs, the lamellae structures of the gills are well organized (Figure 3A). The lamellae consist of nodules of hemocytes in the gill cavities and thin epithelial cells enclosing the cavities (Figure 3B). In the crabs with most severe tissue damage, the basic lamellae structures of the gills were destroyed (Figure 3C). On a higher magnification (×400), necrotic features were found with eosinophilia and plenty of cell debris. The spindle-shaped nuclei of epithelial cells are rounded (Figure 3D). Hemocytes, which present as a group of few inside the gill cavity normally, may heavily infiltrate into the gill cavities of infected crabs (Figure 3F). The histological damage was weaker in some other crabs. The lamellae may still degenerate, but to a smaller extent (Figures 3E, G). Vacuolation may occur in the epithelial cells. The cavities, though observable, started to fill with cell debris (Figure 3H).
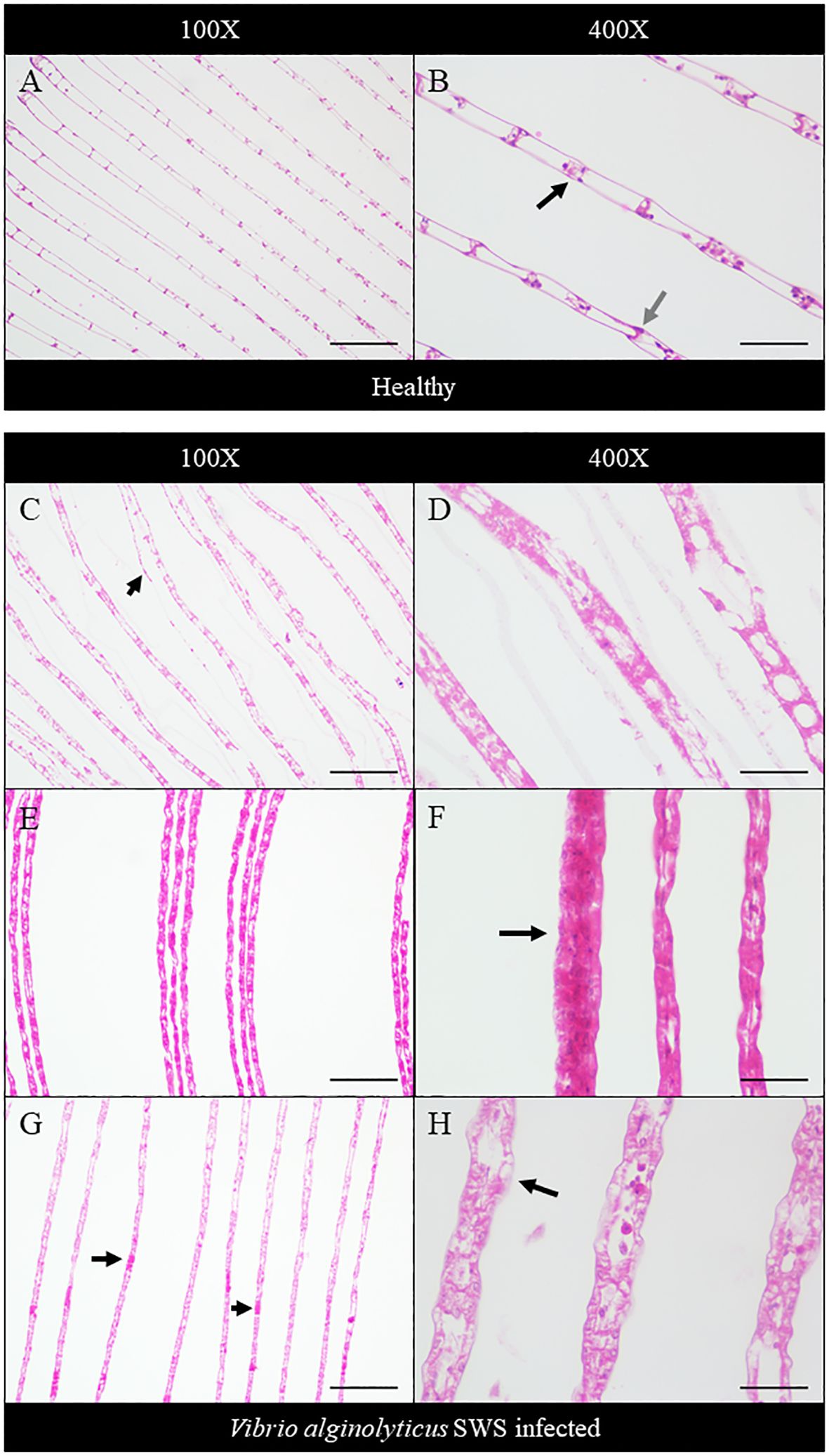
Figure 3 Histopathological analysis of the gills under ×100 (A, C, E, G) and ×400 magnification (B, D, F, H). In the control crabs, basic lamellae structures were seen (A), with the presence of hemocyte nodules (black arrow) in the cavity of the gills and spindle-shaped nuclei of epithelial cells (gray arrow) (B). In heavily infected crabs, the lamellae structure of the gills was destroyed with exfoliated epithelial cells (black arrow) (C), and eosinophilia was seen with much cell debris and rounded nuclei of epithelial cells (D). In moderately infected crabs, the lamellae start to degenerate with gross eosinophilia (E), while heavy infiltration of hemocytes was also seen (black arrow) (F). In less-infected crabs, the lamellae remained rather intact with the presence of eosinophilic masses within the cavity (black arrows) (G), and vacuolation occurred in the epithelial cells (black arrow), with leakage of cell debris into the cavities, which were still observable (H). Scale bars represented 200 µm in ×100 magnification and 50 µm in ×400 magnification, respectively.
The extent of hepatopancreatic degeneration also varied among crabs. The hepatopancreas of healthy crabs demonstrated round tubular structures with star-shaped lumens (Figure 4A). The spaces between the tubules are filled with interstitial tissues (Figure 4B). Severe necrosis of hepatopancreas was observed with the loss of its characteristic tubular structure. Tubular cells detached, and the lumen widened (Figure 4C). On the cellular level, nuclear pyknosis occurred with eosinophilic and fragmented cytoplasm. Together with the indistinguishable cell boundaries, all of these showed typical necrotic features (Figure 4D). In some other crabs, the tubular degeneration became milder, with cells presenting inflammatory response (Figures 4E, F). Also, heavy infiltration of hemocytes was found in the hepatopancreas of another crab (Figure 4G). The tubular lumen was fused (Figure 4H), and a granuloma-like structure was found (Figure 4I).
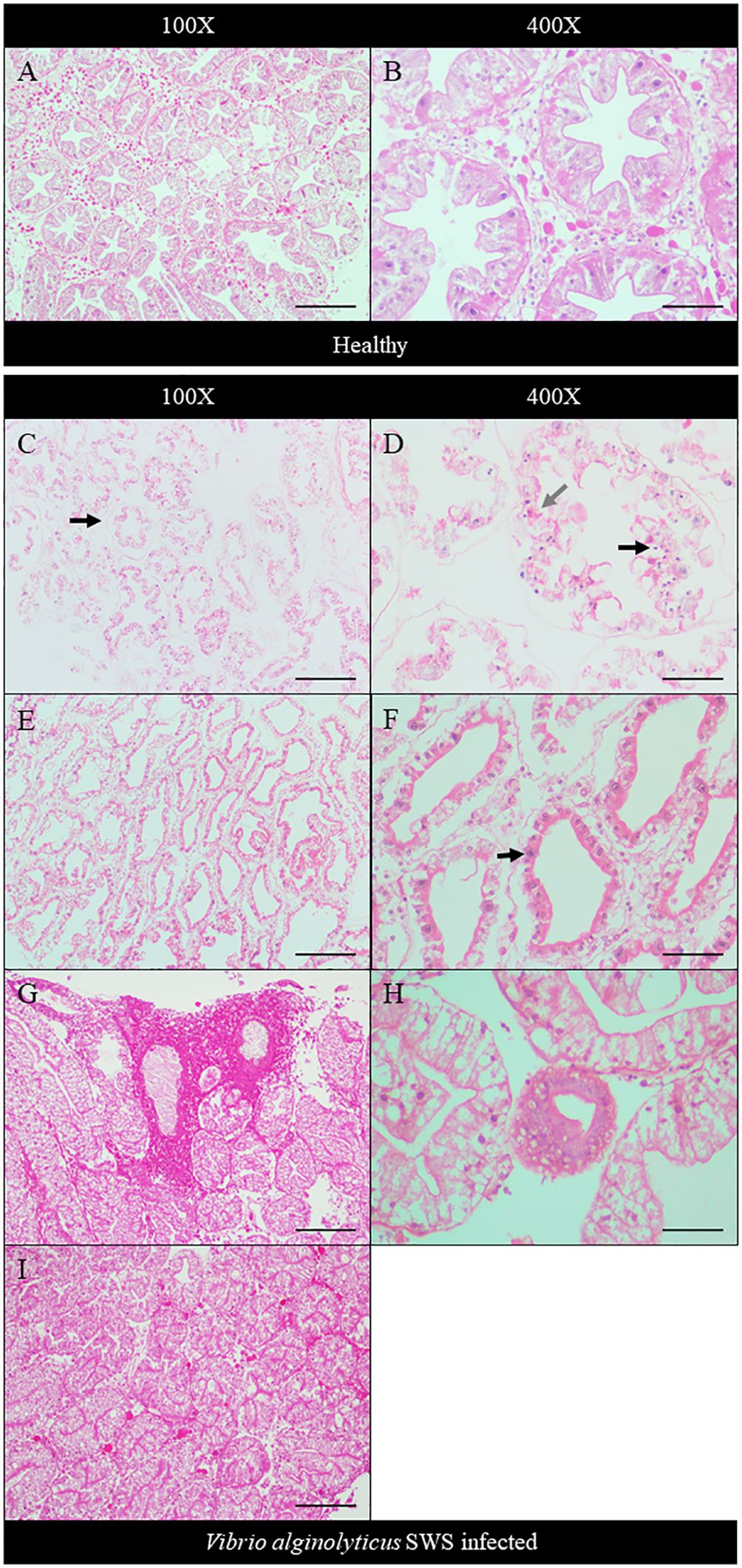
Figure 4 Histopathological analysis of hepatopancreas under ×100 (A, C, E, G, I) and ×400 magnification (B, D, F, H). In control crabs, tubular structure and star-shaped lumen were present with interstitial tissue filling between tubules (A, B). In heavily infected crabs, the tubular degenerated with the detachment of cells from the basement membrane (black arrow) and no observable interstitial tissues (C), while severe cell necrosis was seen with pyknotic nuclei (black arrow), eosinophilic and fragmented cytoplasm (gray arrow), and indistinguishable cell boundaries (D). In moderately infected crabs, the tubule degeneration was milder with widened lumen and shrinkage of tubular cells (E). Strong eosinophilia was found in tubular cells, with chromatin margination and prominent nucleoli seen (black arrow) (F). In less-infected crab, heavy infiltration of hemocytes was seen (G). Granuloma-like structure was observed (H), and the tubular lumen shrank or even fused (I). Scale bars represented 200 µm in ×100 magnification and 50 µm in ×400 magnification, respectively.
In the infected crabs, degeneration of muscles also occurred. Compared to the healthy muscle of crabs which showed tightly packed muscle fibers (Figures 5A, B), both the transverse and longitudinal organizations of muscle fibers were affected. Muscular atrophy was found in the tissues of infected crabs. The normal structure of muscles was disrupted with amorphous masses and derangement of muscle fibers seen (Figures 5C–H). A granuloma-like tissue was also found (Figure 5F).
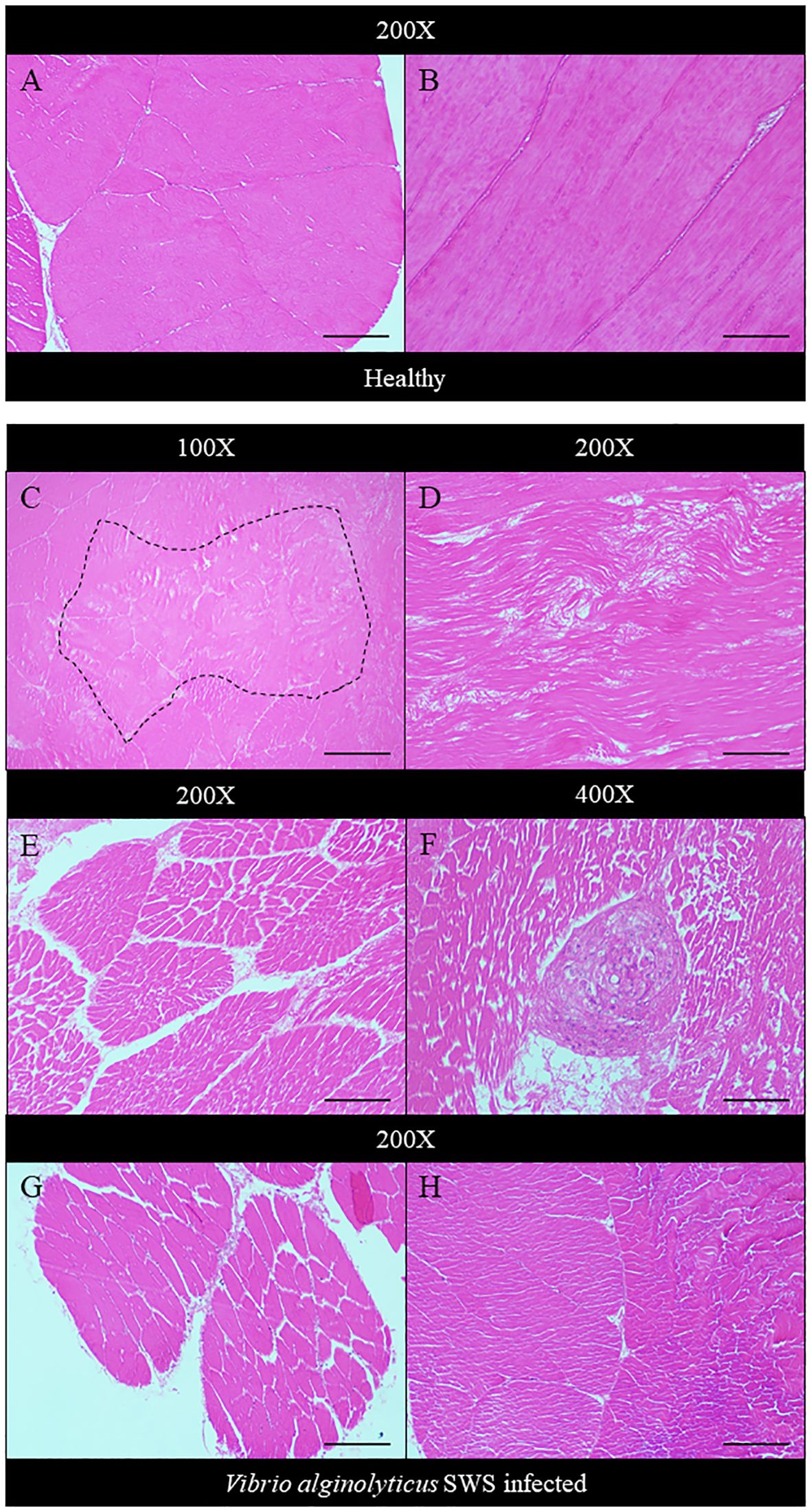
Figure 5 Histopathological analysis of muscle under ×100 (C), ×200 (A, B, D, E, G, H), and ×400 magnification (F). In control crabs, muscle fibers were tightly packed and well organized in transverse (A) and longitudinal sections (B). In heavily infected crabs, an amorphous mass of muscle tissue was seen (dotted box) (C), and muscle fiber derangement occurs with disrupted organization (D). Muscular atrophy started to occur with gaps present between fibers of a single muscle bundle (E). A granuloma-like structure was also seen (F). The extent of muscular atrophy decreases in moderately infected (G) and least infected crabs (H). Scale bars represented 200 µm in ×100 magnification, 100 µm in ×200 magnification, and 50 µm in ×400 magnification.
3.4 Molecular identification and annotation of genome
3.4.1 Comprehensive genome analysis and annotation of genome assembly
Comprehensive genome analysis service at PATRIC was used to assemble the genome of V. alginolyticus SWS. The assembled genome features of V. alginolyticus SWS are summarized in Supplementary Table 1. In brief, the assembled genome of V. alginolyticus SWS had 87 Contigs and 44.61% average G + C content with an estimated genome length of 5,215,511 bp.
Annotation of the genome of V. alginolyticus SWS was done by performing RAST tool kit in PATRIC. The results showed that the genome of V. alginolyticus SWS had 4,876 protein coding sequences (CDS), 78 transfer RNA (tRNA) genes, and 3 ribosomal RNA (rRNA) genes (Supplementary Table 2). The annotation further revealed that the protein features of V. alginolyticus SWS include 1,128 hypothetical proteins and 3,748 proteins with functional assignments (Supplementary Table 3). Among proteins that had functional assignments, 1,087 had Enzyme Commission (EC) number assignments, 910 with gene ontology (GO) assignments, and 802 with gene and genome pathway mapping. In addition, the antimicrobial resistance (AMR) genes annotated and their corresponding mechanism are summarized in Supplementary Table 3.The annotated genome of V. alginolyticus SWS is plotted as a circular graph (Supplementary Figure 1A), and the subsystem analysis of the genomes showed that majority of genes were linked to subsystem (a group of proteins that create structural complex or work together to carry out a specific biological activity) related to metabolism, stress response, defense, virulence, and protein processing (Supplementary Figure 1B). Virulence factor (VF) database revealed that V. alginolyticus SWS might possess 151 VF-related genes from different classes of VF including adherence, anti-phagocytosis, chemotaxis and motility, iron uptake and quorum sensing, secretion system, toxin, and biofilm formation (Supplementary Table 4).
3.4.2 Phylogenetic analysis and genome similarity
Phylogenetic analysis of V. alginolyticus SWS was performed in PATRIC service by comparing PATRIC global protein families from genomes of closest reference and representative genomes identified by Mash/MinHash. The result of phylogenetic analysis showed that V. alginolyticus SWS clustered with V. alginolyticus NBRC 15630 (ATCC 17749) (Figure 6), thus confirming the species identification of the isolate as V. alginolyticus. Genome similarity of V. alginolyticus SWS among 15 V. alginolyticus species was calculated by ANIb and ANIm utilizing JSpeciesWS. Supplementary Table 5 shows that V. alginolyticus SWS and the other 15 V. alginolyticus had ANI values higher than 98% suggesting they were highly similar.
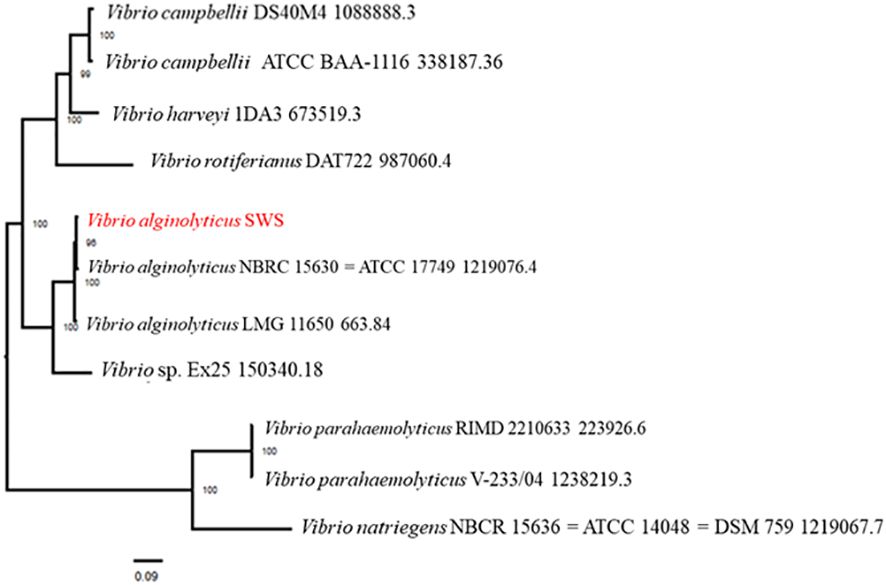
Figure 6 Phylogenetic analysis of Vibrio alginolyticus SWS. V. alginolyticus SWS was clustered to V. alginolyticus ATCC 17749.
3.5 Characterization of the V. alginolyticus SWS
3.5.1 Salt-tolerant V. alginolyticus SWS
V. alginolyticus SWS was inoculated into nutrient broth (NB) with different NaCl % to investigate its salt tolerance characteristics. It was unable to grow in NB with no salt; in contrast, V. alginolyticus SWS grew in NB ranging from 1% to 8% NaCl (Table 1), where V. alginolyticus SWS grow much better in higher salt content (6% and 8% NaCl, Supplementary Figure 2). This indicated that crab isolate had moderately halophilic bacterium as a previous study defined that moderately halophilic bacterium grew optimally in media containing 3%–15% (w/v) salt (Ventosa et al., 1998).
3.5.2 Antimicrobial susceptibilities
Antimicrobial susceptibility test was conducted to investigate the antimicrobial-resistant profile of V. alginolyticus SWS. The antimicrobial-resistant profile of V. alginolyticus SWS is illustrated in Table 2. V. alginolyticus SWS was resistant to two antibiotics, including colistin and ampicillin among 20 tested antibiotics/antibiotic combinations, while sensitive to the remaining 18 antibiotic agents except 6 antibiotics that do not have official interpretation. These 12 antibiotic agents were highly effective in inhibiting V. alginolyticus SWS, which were amoxicillin–clavulanic acid (AUG/AMC), cefepime (FEP), cefotaxime (CTX), cefoxitin (FOX), ceftazidime (CAZ), chloramphenicol (C), ciprofloxacin (CIP), gentamycin (CN), imipenem (IMP), meropenem (MEM), tetracyclin (TE), and trimethoprim/sulfamethoxazole (SXT). However, there is no official reference from the European Committee on Antimicrobial Susceptibility Testing and Clinical and Laboratory Standards Institute for the zone of inhibition of ceftriaxone (CRO), ertapenem (EPT), doripenem (DOR), cefotaxime + clavulanic acid (CTL), ceftazidime + clavulanic acid (CAL), and nalidixic acid (NA) (Clinical and Laboratory Standards Institute, 2016).
3.5.3 Metabolic/biochemical characterization
Metabolic/biochemical characterization of V. alginolyticus SWS was evaluated by Vitek 2 GN cards. The summary of metabolic/biochemical characteristics are shown in Table 3. V. alginolyticus SWS was identified as V. alginolyticus and had positive results on 11 biochemical tests, including ala-phe-pro-arylamidase (APPA), L-pyrrolydonyl-arylamidase (PyrA), beta-n-acetyl-glucosaminidase (BNAG), phosphatase (PHOS), glycine arylamidase (GlyA), coumarate (CMT), Glu-Gly-Arg-arylamidase (GGAA), D-glucose (dGLU), fermentation/glucose (OFF), L-proline arylamidase (ProA), and tyrosine arylamidase (TyrA).
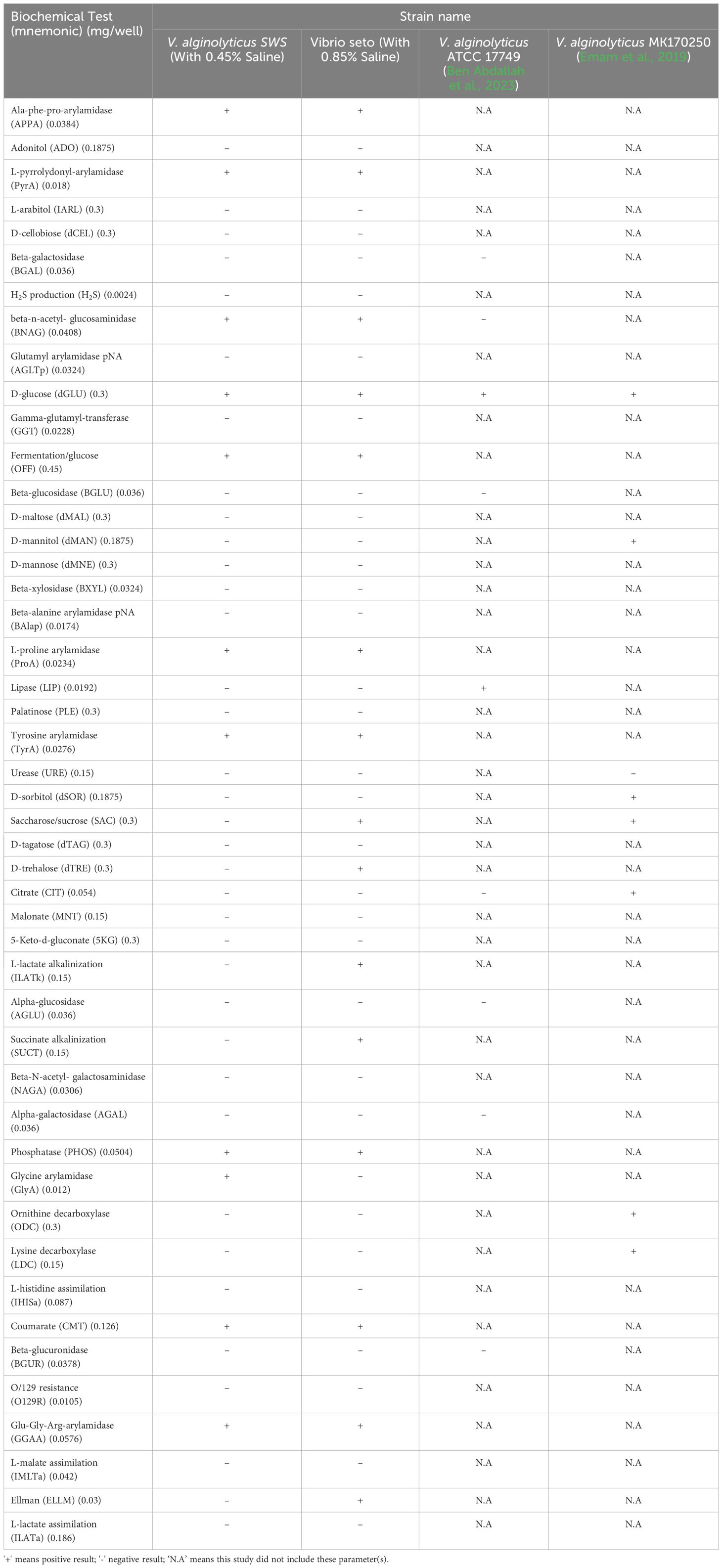
Table 3 Summary of metabolic and physiological characterization of isolated Vibrio alginolyticus SWS reported in this study and other V. alginolyticus strains in other studies.
3.5.4 Optimal temperature and pH
The growth kinetics of V. alginolyticus SWS at different pH and temperature, in terms of doubling time, was determined (Figure 7). The optimal temperature for the growth of this strain is 37°C with the lowest doubling times at pH 6–10, while the doubling times at 26°C were slightly longer than that, followed by those at 30°C. On the other hand, the isolate failed to grow at pH 4 at all tested temperatures, and the growth at pH 5 was insufficient to achieve the stationary phase within 14 h, with the OD600 values below 1.0 (data not shown). From pH 6–10, the optimal pH for the growth of the isolate depended on the temperature. Generally, the isolate grew better at a pH range of 8–10. At 37°C, the optimal pH range was 8–10 since the doubling times at these pH values were similar and generally lower than those at pH 6–7. When the temperature decreased to 30°C, the doubling times at pH 9–10 were lower than those at pH 6–8. Yet, the doubling times at 26°C fluctuated as the pH increased. While the optimal pH is 9, the doubling time increased significantly when the pH increased to 10.
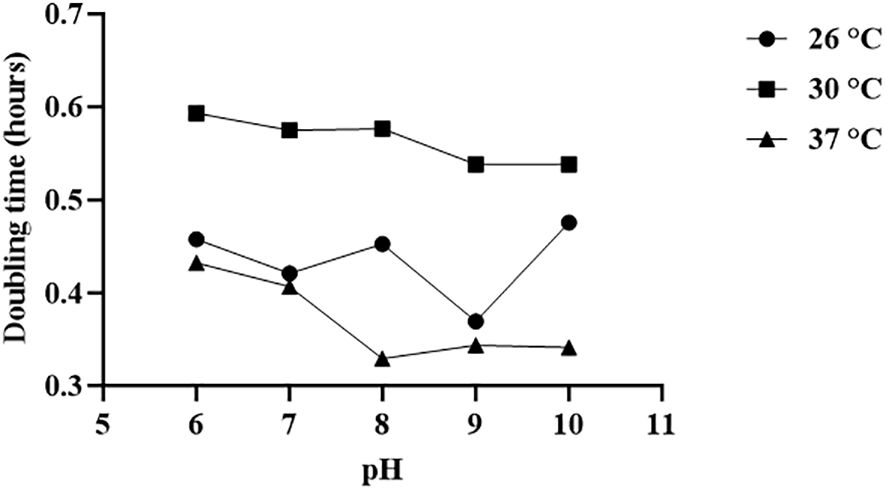
Figure 7 Optimal temperature and pH for the growth of Vibrio alginolyticus SWS. The optimal temperature for the growth of Vibrio alginolyticus SWS is 37°C, while the optimal pH for the growth of V. alginolyticus SWS depended on the temperature. Generally, the isolate grew better at the pH range of 8–10.
4 Discussion
The demand for mud crab is an increasing trend due to its high nutritional value and palatability. However, increasing the supply of mud crab in aquaculture are mainly constrained by cannibalism and disease outbreak (Liew et al., 2023). Unlike cannibalism that can be easily managed via improving knowledge and experience of the farmer, outbreak of diseases remains the biggest challenge in mud crab aquaculture. This is because the diseases can result in high mortality with no effective treatment, and some diseases have causative pathogens that may be unknown (Coates and Rowley, 2022). In addition, the use of antibiotics is not recommended in aquaculture because of the concern of developing antibiotic resistance bacteria eventually affecting humans (Hoseinifar et al., 2024). Hence, the investigation of the disease outbreaks in mud crab aquaculture to formulate effective preventative control measures for preventing the recurrence of disease outbreaks is important to maintain the sustainability of mud crab aquaculture. In this study, V. alginolyticus SWS was isolated from local crab aquaculture responsible for causing high morality, which showed high pathogenicity in experimental infection of S. serrata.
There is only a single report showing that V. alginolyticus could cause shell disease in mud crabs, but experimental infection was not used to validate it, and the mortality rate and pathogenicity as well as characteristics were not investigated in detail (Gunasekaran et al., 2019). Based on the current understanding of mud crab diseases, they are mainly caused by pathogens, including mud crab reovirus, mud crab dicistrovirus, mud crab tombus-like virus (MCTV), V. parahaemolyticus, and Aquimarina hainanensis (Coates and Rowley, 2022). Furthermore, there has been an increase in outbreaks of disease causing high mortality in aquatic animals, for instance, fish, oysters, and shrimp associated with V. alginolyticus (George et al., 2005; Cao et al., 2018; Yang et al., 2021), with unknown mortality in crabs. Therefore, V. alginolyticus could be an emerging pathogen in mud crab aquaculture. Our result suggested that the disease outbreak accounted for 70% of mortality resulting from V. alginolyticus infection, which leads to excessive economic loss to the aquaculture.
Experimental infection has been commonly employed to validate whether the isolated causative agents in diseases meet the criteria of Koch’s postulates and to investigate its pathogenicity (Xie et al., 2021). Therefore, this study also performed experimental infection to determine whether V. alginolyticus SWS was a novel causative agent in the recent local massive death of S. serrata aquaculture. V. alginolyticus SWS was only reisolated from experimentally infected S. serrata (Figures 1C-F), and the same clinical symptoms were observed in infected mud crabs from farm and experimental infected S. serrata implying that V. alginolyticus SWS was the causative agent. Results from experimental infection showed that V. alginolyticus SWS could result in high mortality (75%) after 4 days of infection. Previously, V. alginolytigus was found to cause shell disease in S. serrata with obvious shell lesions or induce whitish muscle syndrome (Gunasekaran et al., 2019; Hou et al., 2023). However, V. alginolyticus SWS did not induce these symptoms; instead, it caused inactivity, low appetite, and decolorization of the hepatopancreas, gills, and opaque muscle. Furthermore, in the present study, 2.5 × 105 CFU/ml of V. alginolyticus SWS induced 75% mortality after 4 days of infection in S. serrata, while other studies required much higher CFU of V. alginolytigus to induce a similar mortality rate in oysters and shrimps, at 5 × 107 and 4 × 108 CFU/ml, respectively (Chun-Hung et al., 2004; Yang et al., 2021). This indicated that S. serrata were more vulnerable than oysters and shrimps to V. alginolytigus probably because it affected different parts of mud crabs. The occurrence of V. alginolytigus SWS outbreak in mud crabs might increase.
Histopathology was employed to understand the negative impact of V. alginolyticus SWS on mud crabs. The significant histological differences in the hepatopancreas, gills, and muscles between healthy and infected mud crabs provided clues about how the infection of this new isolate of Vibrio caused the death of mud crabs. Some typical histological features indicated inflammation in the tissues observed, such as eosinophilia and infiltration of hemocytes. Targeting pathogens, hemocyte plays a crucial role in the innate immunity of crustaceans (Zhang et al., 2019). As infiltration of hemocytes was only seen in the hepatopancreas and gills of the infected crabs, but not in the muscles, it was possible that the infection primarily affects the former two organs, while the muscle was subjected to the secondary impact of infection. Besides, various cellular features suggested that the tissues also experienced necrosis of different extents, including nuclear rounding, pyknosis, and loss of histological architecture. Heavy necrosis in the three types of tissues suggested that the infection could cause systemic damage to the organs of the crabs. The histological observation of the hepatopancreas and muscles resonates with previous studies (Xie et al., 2021; John et al., 2024). For example, in the infection of S. paramamosain by Photobacterium sp., similar pathologies were observed in the hepatopancreas with tissue necrosis, widened lumen, and detachment of epithelial cells from basement membrane (Xie et al., 2021), while the muscles fibers were disorganized, and similar muscular atrophy was observed (Xie et al., 2021). When Photobacterium species infects shrimps, similar hepatopancreatic damage was seen with widened lumen and damaged epithelial cells (Liu et al., 2016). Besides, in the co-infection of S. serrata by mud crab reovirus and Staphylococcus saprophyticus, hemocyte infiltration and degradation of interstitial tissues were also seen. The lumen of the hepatopancreatic tubules reduced in size, which was also observed in the less infected crabs (John et al., 2024). The extent of damage in the present study was larger compared to these previous studies suggesting a high virulence of this strain that might be due to its virulence factor-related genes.
Molecular identification of bacteria is commonly performed by 16S rRNA gene sequencing; however, 16S sequencing is unable to identify the species level between Vibrio species due to a high similarity in the 16S rRNA region of their genomes (Mougin et al., 2020). A recent study misidentified species and strains in Vibrio species because of using 16S rRNA sequencing only or multilocus sequence analysis (MLSA) of four genes. The same study suggested at least performing MLSA of eight genes or even more advanced techniques to achieve reliable identification up to the strain level (Jiang et al., 2021). In this study, we first tentatively identified the V. alginolyticus SWS isolated from natural infected crab by MALDI-TOF MS as V. alginolytigus, then WGS was performed and submitted to the PATRIC server for comprehensive genome analysis to confirm the species. The genome similarity of V. alginolyticus SWS was also calculated by ANI using JSpeciesWS.
Regarding the metabolic/biochemical characteristics, our study aligned with the findings of a previous study of V. alginolyticus ATCC 17749 showing that V. alginolyticus SWS could not grow in salt-free environment (Farid and Larsen, 1981). Here, in our study, we found that some of the metabolic/biochemical characteristics of V. alginolyticus SWS were different from those of V. alginolyticus ATCC 17749 that has been reported to be positive to lipase, negative to beta-n-acetyl- glucosaminidase (Ben Abdallah et al., 2023), while V. alginolyticus SWS was also found to be different from V. alginolyticus MK170250 isolated from stingray (Emam et al., 2019), which had positive result on lysine decarboxylase, ornithine decarboxylase, citrate, mannitol, and D-sorbitol.
Antibiotic resistance in vibrio species is a major obstacle in the prevention and management of disease outbreak that can result in huge economic loss. A recent study reported that 43 out of 104 V. alginolyticus isolates from diseased aquaculture in China exhibited multiple antimicrobial resistance to at least four kinds of antibiotics, in which all of them are resistant to ampicillin (Yu et al., 2022). Our findings were aligned with the previous study, which also showed that V. alginolyticus SWS is resistant to ampicillin. These results could provide fundamentals for designing prevention and management control of the occurrence of V. alginolyticus SWS in aquaculture, particularly mud crab. Ideally, alternatives should be considered rather than using antibiotics such as using Chinese herbal medicines that have served as a good replacement for antibiotics to treat bacterial infection in mud crabs (Wu et al., 2021).
5 Conclusion
In summary, our study identified that V. alginolyticus SWS was responsible for a recent outbreak in S. serrata aquaculture with validation on experiential infection. Results from experimental infection and histopathological analysis implied that V. alginolyticus SWS was highly pathogenic and lethal to S. serrata. Genome features of V. alginolyticus SWS and its antimicrobial resistance (AMR) genes and virulence factor-related genes were also studied in our study by comprehensive genome analysis and were predicted in a virulence factor database. Our results also showed that V. alginolyticus SWS was clustered to V. alginolyticus ATCC 17749, but some of the metabolic/biochemical characteristics were different. Antimicrobial susceptibility tests in this study indicated that V. alginolyticus SWS was resistant to ampicillin. The discovery of the emerging pathogen V. alginolyticus SWS in S. serrata in this study highlights the importance of identifying emerging pathogens and formulating feasible management to reduce economic loss in mud crab agriculture.
Data availability statement
The datasets presented in this study can be found in online repositories. The names of the repository/repositories and accession number(s) can be found in the article/Supplementary Material.
Ethics statement
The use of invertebrates in research or teaching activities does not require ethical approval from ethics committee. The studies were conducted in accordance with the local legislation and institutional requirements.
Author contributions
CK: Data curation, Formal analysis, Investigation, Methodology, Writing – original draft. RCWY: Data curation, Formal analysis, Investigation, Methodology, Writing – original draft. PTH: Data curation, Methodology, Writing – review & editing. KC: Data curation, Methodology, Writing – review & editing. IN: Data curation, Formal analysis, Methodology, Writing – review & editing. JF: Data curation, Formal analysis, Methodology, Writing – review & editing. IW: Data curation, Formal analysis, Methodology, Writing – review & editing. MCYY: Data curation, Formal analysis, Methodology, Writing – review & editing. WML: Writing – review & editing. HKK: Methodology, Resources, Supervision, Validation, Writing – review & editing. GKHS: Methodology, Project administration, Resources, Supervision, Writing – review & editing. FWNC: Conceptualization, Methodology, Project administration, Resources, Supervision, Writing – review & editing. SWS: Conceptualization, Funding acquisition, Project administration, Resources, Supervision, Writing – review & editing.
Funding
The author(s) declare financial support was received for the research, authorship, and/or publication of this article. This work is partly supported by a research donation from AI Farming HK Ltd. S-WS is supported by The Hong Kong Polytechnic University General Research Fund (Project No.: P0036597) and Research Centre for Chinese Medicine Innovation, The Hong Kong Polytechnic University (Project No.: P0041138). FWNC is supported by PolyU Start-up Fund for RAPs under the Strategic Hiring Scheme (P0038407) and partially by Research Grant Council—General Research Fund (15100322).
Acknowledgments
The authors would like to thank Mr. Tsz-Chung Yeung and Ching-Ngai Li for their technical support in this study. The authors would like to also thank Ms. Wu Lai Han, Daphne, for her advice on histopathology. In addition, the authors would like to express their deepest gratitude to AI Farming HK Ltd. for providing the crab samples.
Conflict of interest
Author W-ML was employed by the company AI Farming Company Hong Kong Limited. The authors declare that this study received funding from AI Farming HK Ltd. The funder had the following involvement in the study: provided technical support and revised manuscript.
The author(s) declared that they were an editorial board member of Frontiers, at the time of submission. This had no impact on the peer review process and the final decision.
Publisher’s note
All claims expressed in this article are solely those of the authors and do not necessarily represent those of their affiliated organizations, or those of the publisher, the editors and the reviewers. Any product that may be evaluated in this article, or claim that may be made by its manufacturer, is not guaranteed or endorsed by the publisher.
Supplementary material
The Supplementary Material for this article can be found online at: https://www.frontiersin.org/articles/10.3389/fcimb.2024.1425104/full#supplementary-material
Supplementary Figure 1 | Results of the comprehensive genome analysis of Vibrio alginolyticus SWS (A) Circular graphical assembly of the genome of Vibrio alginolyticus SWS, from outer to inner rings, the contigs, CDS on the forward strand, CDS on the reverse strand, RNA genes, CDS with homology to known antimicrobial resistance genes, CDS with homology to known virulence factors, GC content and GC skew; The colours of the CDS on the forward and reverse strand indicate the subsystem (refer to B) that these genes belong to. (B) Subsystem analysis of the genome of Vibrio alginolyticus SWS.
Supplementary Figure 2 | Salt-tolerant of Vibrio alginolyticus SWS in different percentage of salt in nutrient broth. V. alginolyticus SWS could not grow in NB with no salt, in contrast, V. alginolyticus SWS grew in NB ranging from 1% to 8% NaCl.
References
Alberts-Hubatsch, H., Lee, S. Y., Meynecke, J.-O., Diele, K., Nordhaus, I., Wolff, M. (2016). Life-history, movement, and habitat use of Scylla serrata (Decapoda, Portunidae): current knowledge and future challenges. Hydrobiologia 763, 5–21. doi: 10.1007/s10750-015-2393-z
Apine, E., Ramappa, P., Bhatta, R., Turner, L. M., Rodwell, L. D. (2023). Challenges and opportunities in achieving sustainable mud crab aquaculture in tropical coastal regions. Ocean Coast. Manage. 242, 106711. doi: 10.1016/j.ocecoaman.2023.106711
Ben Abdallah, L., Tapia Paniagua, S. T., Turki, Y., Medina, A., Mehri, I., Morinigo, M., et al. (2023). Intraspecific characterization of Vibrio alginolyticus isolates recovered from aquaculture systems and marine biotopes in Tunisia by PCR-RFLP, ECP and OMPs profiliranja. Acta Adriat. 64, 113–124. doi: 10.32582/aa.64.2.3
Bhuiyan, M. S., Shamsuzzaman, M. M., Hossain, M. M., Mitu, S. J., Mozumder, M. M. H. (2021). Mud crab (Scylla serrata Forsskal 1775) value chain analysis in the Khulna region of Bangladesh. Aquac. Fish. 6, 330–336. doi: 10.1016/j.aaf.2021.01.004
Cao, J., Zhang, J., Ma, L., Li, L., Zhang, W., Li, J. (2018). Identification of fish source Vibrio alginolyticus and evaluation of its bacterial ghosts vaccine immune effects. Microbiologyopen 7, e00576. doi: 10.1002/mbo3.576
Cheng, C.-H., Ma, H.-L., Deng, Y.-Q., Feng, J., Jie, Y.-K., Guo, Z.-X. (2020). Effects of Vibrio parahaemolyticus infection on physiological response, histopathology and transcriptome changes in the mud crab (Scylla paramamosain). Fish Shellf. Immunol. 106, 197–204. doi: 10.1016/j.fsi.2020.07.061
Chun-Hung, L., Winton, C., Jung-Ping, H., Jiann-Chu, C. (2004). Vibrio alginolyticus infection in the white shrimp Litopenaeus vannamei confirmed by polymerase chain reaction and 16S rDNA sequencing. Dis. Aquat. Organ. 61, 169–174. doi: 10.3354/dao061169
Clinical and Laboratory Standards Institute (2016). Methods for antimicrobial dilution and disk susceptibility testing of infrequently isolated or fastidious bacteria. 3rd ed (Pennaylvania, USA: Clinical and Laboratory Standards Institute).
Coates, C. J., Rowley, A. F. (2022). Emerging diseases and epizootics in crabs under cultivation. Front. Mar. Sci. 8. doi: 10.3389/fmars.2021.809759
Emam, A. M., Hashem, M., Gadallah, A. O., Haridy, M. (2019). An outbreak of Vibrio alginolyticus infection in aquarium-maintained dark-spotted (Himantura uarnak) and Tahitian (H. fai) stingrays. Egypt. J. Aquat. Res. 45, 153–158. doi: 10.1016/j.ejar.2019.05.003
FAO (2022). The state of world fisheries and aquaculture 2022: towards blue transformation. state World fish. aquacult., I–236. doi: 10.4060/cc0461en
Farid, A. F., Larsen, J. L. (1981). Growth of Vibrio alginolyticus: Interacting effects on pH, temperature, salt concentration, and incubation time. Zentralblatt für Bakteriologie Mikrobiologie und Hyg.: I. Abt. Originale C: Allgemeine angewandte und ökologische Mikrobiologie 2, 68–75. doi: 10.1016/S0721-9571(81)80019-1
Fazhan, H., Waiho, K., Ikhwanuddin, M. (2017). Non-indigenous giant mud crab, Scylla serrata (Forskål 1775) (Crustacea: Brachyura: Portunidae) in Malaysian coastal waters: a call for caution. Mar. Biodivers. Rec. 10, 26. doi: 10.1186/s41200-017-0128-8
George, M. R., John, K. R., Iyappan, T., Jeyaseelan, M. J. P. (2005). Genetic heterogeneity among Vibrio alginolyticus isolated from shrimp farms by PCR fingerprinting. Lett. Appl. Microbiol. 40, 369–372. doi: 10.1111/j.1472-765X.2005.01675.x
Gunasekaran, T., Gopalakrishnan, A., Deivasigamani, B., Muhilvannan, S., Kathirkaman, P. (2019). Vibrio alginolytigus causing shell disease in the mud crab Scylla serrata (Forskal 1775). Indian J. Geo-Mar. Sci. 48, 1359–1363.
Hong, G.-L., Dai, X.-Q., Lu, C.-J., Liu, J.-M., Zhao, G.-J., Wu, B., et al. (2014). Temporizing surgical management improves outcome in patients with Vibrio necrotizing fasciitis complicated with septic shock on admission. Burns 40, 446–454. doi: 10.1016/j.burns.2013.08.012
Hoseinifar, S. H., Ashouri, G., Marisaldi, L., Candelma, M., Basili, D., Zimbelli, A., et al. (2024). Reducing the use of antibiotics in European aquaculture with vaccines, functional feed additives and optimization of the gut microbiota. J. Mar. Sci. Eng. 12, 204. doi: 10.3390/jmse12020204
Hou, D., Lian, T., Guo, G., Gong, H., Wu, C., Han, P., et al. (2023). Integration of microbiome and Koch’s postulates to reveal multiple bacterial pathogens of whitish muscle syndrome in mud crab, Scylla paramamosain. Microbiome 11, 155. doi: 10.1186/s40168-023-01570-6
Howard, D. W. (2004). Histological techniques for marine bivalve mollusks and crustaceans (Maryland, USA: NOAA, National Ocean Service, National Centers for Coastal Ocean Service, Center for Coastal Environmental Health and Biomolecular Research, Cooperative Oxford Laboratory).
Hu, F., Zhong, H., Wu, C., Wang, S., Guo, Z., Tao, M., et al. (2021). Development of fisheries in China. Reprod. Breed. 1, 64–79. doi: 10.1016/j.repbre.2021.03.003
Jiang, C., Tanaka, M., Nishikawa, S., Mino, S., Romalde, J. L., Thompson, F. L., et al. (2021). Vibrio Clade 3.0: New Vibrionaceae evolutionary units using genome-based approach. Curr. Microbiol. 79, 10. doi: 10.1007/s00284-021-02725-0
Jithendran, K. P., Poornima, M., Balasubramanian, C. P., Kulasekarapandian, S. (2011). Diseases of mud crabs (Scylla spp.): an overview. Indian J. Fish. 57, 55–63.
John, A. S., Gopalakrishnan, A., Sravani, S., Dewangan, N. K., Seralathan, M. V., Prabhu, N. M. (2024). Co-infection of mud crab Reovirus (MCRV) and Staphylococcus saprophyticus in giant mud crab, Scylla serrata (Forsskal 1775). Aquaculture 579, 740199. doi: 10.1016/j.aquaculture.2023.740199
Lavilla-Pitogo, C., Marcial, H., Pedrajas, S., Quinitio, E., Millamena, O. (2001). Problems associated with tank-held mud crab (Scylla spp.) broodstock. Asian Fish. Sci. 14, 217–224. doi: 10.33997/j.afs.2001.14.2.012
Li, X. C., Xiang Zhen, Y., Xu Xiao, M., Yan Wei, H., Ma Jian, M. (2009). Endophthalmitis caused by Vibrio alginolyticus. J. Clin. Microbiol. 47, 3379–3381. doi: 10.1128/JCM.00722-09
Liew, K.-S., Yong, F. K.-B., Lim, L.-S. (2023). An overview of the major constraints in Scylla mud crabs grow-out culture and its mitigation methods. Aquac. Stud. 24, AQUAST993. doi: 10.4194/2618-6381
Liu, B., Zheng, D., Jin, Q., Chen, L., Yang, J. (2019). VFDB 2019: a comparative pathogenomic platform with an interactive web interface. Nucleic Acids Res. 47, D687–D692. doi: 10.1093/nar/gky1080
Liu, B., Zheng, D., Zhou, S., Chen, L., Yang, J. (2022). VFDB 2022: a general classification scheme for bacterial virulence factors. Nucleic Acids Res. 50, D912–D917. doi: 10.1093/nar/gkab1107
Liu, F., Liu, G., Li, F. (2016). Characterization of two pathogenic Photobacterium strains isolated from Exopalaemon carinicauda causing mortality of shrimp. Aquaculture 464, 129–135. doi: 10.1016/j.aquaculture.2016.06.019
Ly Van, K., Arsa, C. P., Nguyen Thi, N. A., Tran Ngoc, H. (2022). Use of different seaweeds as shelter in nursing mud crab, Scylla paramamosain: effects on water quality, survival, and growth of crab. J. World Aquac. Soc 53, 485–499. doi: 10.1111/jwas.12830
Mougin, J., Flahaut, C., Roquigny, R., Bonnin-Jusserand, M., Grard, T., Le Bris, C. (2020). Rapid Identification of Vibrio species of the Harveyi clade Using MALDI-TOF MS profiling with main spectral profile database implemented with an in-house database: Luvibase. Front. Microbiol. 11. doi: 10.3389/fmicb.2020.586536
Norfolk, W. A., Shue, C., Henderson, W. M., Glinski, D. A., Lipp, E. K. (2023). Vibrio alginolyticus growth kinetics and the metabolic effects of iron. Microbiol. Spectr. 11, e02680–e02623. doi: 10.1128/spectrum.02680-23
Paran, B. C., Jeyagobi, B., Kizhakedath, V. K., Antony, J., Francis, B., Anand, P. S. S., et al. (2022). Production of juvenile mud crabs, Scylla serrata: captive breeding, larviculture and nursery production. Aquac. Rep. 22, 101003. doi: 10.1016/j.aqrep.2021.101003
Reiter, M. A., Vorholt, J. A. (2024). Dashing Growth Curves: a web application for rapid and interactive analysis of microbial growth curves. BMC Bioinf. 25, 67. doi: 10.1186/s12859-024-05692-y
Richter, M., Rosselló-Móra, R., Oliver Glöckner, F., Peplies, J. (2015). JSpeciesWS: a web server for prokaryotic species circumscription based on pairwise genome comparison. Bioinformatics 32, 929–931. doi: 10.1093/bioinformatics/btv681
Sarjito, D., Haditomo, A. H. C., Prayitno, S. B. (2018). The bacterial diversity associated with bacterial diseases on mud crab (Scylla serrata Fab.) from Pemalang Coast, Indonesia. J. Phys.: Conf. Ser. 1025, 12076. doi: 10.1088/1742-6596/1025/1/012076
Ventosa, A., Márquez, M. C., Garabito, M. J., Arahal, D. R. (1998). Moderately halophilic gram-positive bacterial diversity in hypersaline environments. Extremophiles 2, 297–304. doi: 10.1007/s007920050072
Wattam, A. R., Davis, J. J., Assaf, R., Boisvert, S., Brettin, T., Bun, C., et al. (2016). Improvements to PATRIC, the all-bacterial bioinformatics database and analysis resource center. Nucleic Acids Res. 45, D535–D542. doi: 10.1093/nar/gkw1017
Wu, Q., Jiang, Y., Chen, E., Mu, C., Waiho, K. (2021). Chinese gallnut (Galla chinensis) against Vibrio parahaemolyticus: In vitro activity and the use of medicated bath method to treat infected mud crab Scylla paramamosain. Aquaculture 539, 736632. doi: 10.1016/j.aquaculture.2021.736632
Xie, J., Mei, H., Jin, S., Bu, L., Wang, X., Wang, C., et al. (2021). First report of Photobacterium damselae subsp. damselae infection in the mud crab Scylla paramamosain cultured in China. Aquaculture 530, 735880. doi: 10.1016/j.aquaculture.2020.735880
Yang, B., Zhai, S., Li, X., Tian, J., Li, Q., Shan, H., et al. (2021). Identification of Vibrio alginolyticus as a causative pathogen associated with mass summer mortality of the Pacific Oyster (Crassostrea gigas) in China. Aquaculture 535, 736363. doi: 10.1016/j.aquaculture.2021.736363
Yu, Y., Li, H., Wang, Y., Zhang, Z., Liao, M., Rong, X., et al. (2022). Antibiotic resistance, virulence and genetic characteristics of Vibrio alginolyticus isolates from aquatic environment in costal mariculture areas in China. Mar. pollut. Bull. 185, 114219. doi: 10.1016/j.marpolbul.2022.114219
Keywords: pathogen, Vibrio alginolyticus, mud crab, Scylla serrata, disease, histopathology, infection, aquaculture
Citation: Kwok CT-K, Yu RC-W, Hau P-T, Cheung KY-C, Ng IC-F, Fung J, Wong IT-F, Yau MC-Y, Liu W-M, Kong H-K, Siu GK-H, Chow FW-N and Seto S-W (2024) Characteristics and pathogenicity of Vibrio alginolyticus SWS causing high mortality in mud crab (Scylla serrata) aquaculture in Hong Kong. Front. Cell. Infect. Microbiol. 14:1425104. doi: 10.3389/fcimb.2024.1425104
Received: 29 April 2024; Accepted: 26 June 2024;
Published: 23 July 2024.
Edited by:
Xihui Shen, Northwest A&F University, ChinaReviewed by:
Anbarasu Kumarasamy, Bharathidasan University, IndiaHeyu Lin, Queensland University of Technology, Australia
Copyright © 2024 Kwok, Yu, Hau, Cheung, Ng, Fung, Wong, Yau, Liu, Kong, Siu, Chow and Seto. This is an open-access article distributed under the terms of the Creative Commons Attribution License (CC BY). The use, distribution or reproduction in other forums is permitted, provided the original author(s) and the copyright owner(s) are credited and that the original publication in this journal is cited, in accordance with accepted academic practice. No use, distribution or reproduction is permitted which does not comply with these terms.
*Correspondence: Sai-Wang Seto, c2Fpd2FuZy5zZXRvQHBvbHl1LmVkdS5oaw==; Franklin Wang-Ngai Chow, ZnJhbmtsaW4uY2hvd0Bwb2x5dS5lZHUuaGs=
†These authors have contributed equally to this work