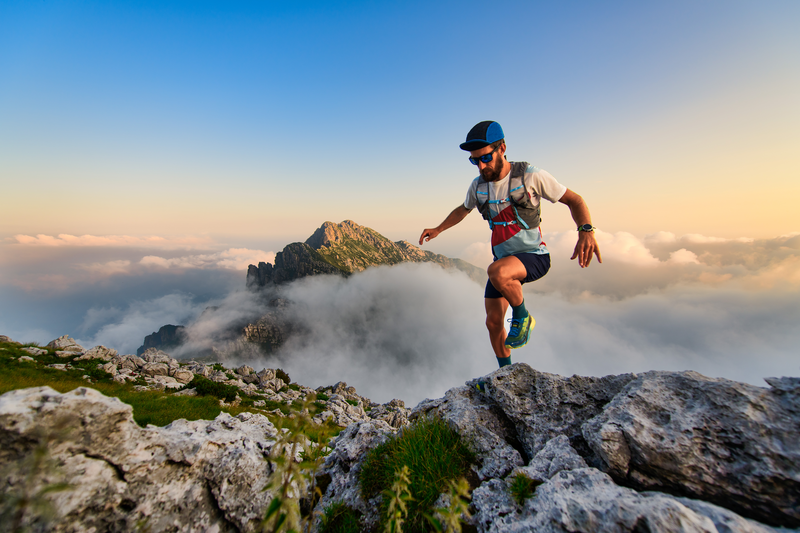
95% of researchers rate our articles as excellent or good
Learn more about the work of our research integrity team to safeguard the quality of each article we publish.
Find out more
EDITORIAL article
Front. Cell. Infect. Microbiol. , 06 June 2024
Sec. Intestinal Microbiome
Volume 14 - 2024 | https://doi.org/10.3389/fcimb.2024.1413473
This article is part of the Research Topic The interplay of gut-microbiome between infection and inflammation View all 18 articles
Editorial on the Research Topic
The interplay of gut-microbiome between infection and inflammation
Because of its varied makeup and role in regulating human health and disease, the gut microbiome has drawn huge attention from the scientific community in recent years. As a result of scientific advancements including high-throughput genome sequencing and transcriptome analysis, the gut microbiome has become a viable therapeutic target for both infectious and autoimmune diseases. In particular, it has been demonstrated that respiratory infections, such as COVID-19 and tuberculosis, significantly influence the pathogenesis of the disease progression due to gut dysbiosis, which in turn impacts the regulation of the gut-lung axis and advances the disease (Baindara et al., 2021). Conversely, it has been noted that gut dysbiosis plays a significant role in several chronic conditions, such as cancer, diabetes, obesity, inflammatory bowel disease, neurological disorders, cardiovascular complications, biliary diseases, colitis, appendicitis, and metabolic disorders (De Vos et al., 2022; Hou et al., 2022). Despite the acknowledged involvement of the gut microbiome in various pathologies, it is currently unclear how the gut microbiota contributes to the development and regulation of various diseases. Infection-associated inflammation or sterile inflammation and its molecular interaction with gut microbiota and gut dysbiosis, along with overall disease progression is one of the exciting research areas that requires further detailed investigation to understand the unanswered questions. Furthermore, understanding and methods to overcome gut dysbiosis, gut microbiome transfer, gut microbiota-based host-directed therapies, understanding the impact of conventional antibiotics on the gut microbiome, and drug-repurposing techniques to alter the gut microbiome are key underexplored Research Topics on the path to scientific advancement in the role and regulation of gut microbiome research.
Acting as a bridge between the liver and the intestine, the biliary system demonstrates proximity to the gut microbiome. Importantly, the biliary system plays an essential role in metabolic regulation, especially in lipid metabolism in the bile duct. The occurrence of biliary system diseases has rapidly increased in recent years, but the involvement of the microbiota has not yet been fully explored in this regard. In a recent systemic review, Wang et al. reviewed the associated microbial diversity in patients with gallstones (GS), primary sclerosing cholangitis (PSC), primary biliary cholangitis (PBC), and biliary tract cancer (BTC). Systemic analysis revealed that the prevalence of the genus Faecalibacterium was decreased in GS, PSC, PBC, and BTC patients, whereas the abundance of the genera Veillonella, Lactobacillus, Streptococcus, and Enterococcus was noticeably increased in the cases of PSC, PBC, and BTC-associated patients. Remarkably, the diversity of the genus Clostridium was found to be decreased in GS, PBC, and BTC patients while it was generally increased in PSC patients. Another study by Chen et al. explored the microbiome associated with biliary obstruction in patients infected with Clonorchis sinensis. An increase in Proteobacteria and a decrease in Firmicutes were reported in the biliary microbiome of C. sinensis-infected patients at the phylum level. Also, a significant increase in the genera Pseudomonas and Staphylococcus was observed along with a noticeable reduction in Enterococcus bacteria at the genus level in infected patients. Furthermore, lying within proximity, the gut microbiome was found to have a significant role in the liver environment via the gut-liver axis. Any changes in the gut microbiota can induce liver inflammation, which can further result in chronic liver diseases (CLDs) such as liver cirrhosis (Figure 1). Next, Wu et al. studied the distribution of the gut microbiome in patients with liver cirrhosis by employing 16S rRNA gene sequencing of stool samples. The results demonstrated a prominently reduced microbial diversity and diminished abundance of typical SCFA-producing bacterial groups including Firmicutes, Coprococcus, and Clostridium IV, along with an enhanced burden of pathogenic bacterial groups, Gammaproteobacteria, Veillonella, and Bacilli in cirrhotic patients. It has been suggested that probiotics may be used as an adjuvant therapy to boost SCFA-producing bacteria in patients with liver cirrhosis.
A gut infection caused by Clostridioides difficile is a major cause of nosocomial diarrhea. A microbial profiling study by Vázquez-Cuesta et al. reported an increased burden of Fusobacterium, and a reduction of Collinsella, Senegalimassilia, Prevotella, and Ruminococcus in recurrent C. difficile infection (R-CDI). Interestingly, the rate of R-CDI was significantly correlated with increased levels of calprotectin (Figure 1). The authors suggested a predictive model for R-CDI, employing dominant microbial genera and calprotectin levels.
In addition to infection-associated gut dysbiosis and involvement in the inflammatory response, the gut microbiome has also been reported to play an important role in the progression of cancers such as colorectal cancer (CRC). A study by Bucher-Johannessen et al. showed an abundance of Phascolarctobacterium succinatutens, Bifidobacterium, and Lachnospiraceae spp. associated with the patient samples diagnosed with CRC and high-risk adenoma (HRA). In another study, Xu et al. evaluated the dynamic changes of migratory microbial components in colonic tissue using an LPS-induced rat model of sepsis. The results of this study determined the temporal dynamics of bacteria and bacterial translocation from various sources to colonic tissues at different time points of sepsis progression (Figure 1). In conclusion, the identified bacterial migrants may serve as potential biomarkers that directly or indirectly alter the structure and function of the colonic microbiota, during the pathophysiology of sepsis.
It is a known fact that during COVID-19, gut microbiota played an important role in disease regulation by modulating the gut-lung axis. However, children showed only mild symptoms. To uncover the protective effect of the gut microbiome in children during COVID-19, Marzano et al. performed a metaproteome analysis of the gut microbiota in pediatric patients. Univariate analysis revealed that the pediatric gut microbiota played a protective role by being involved in metabolic processes such as tryptophan, butanoate, fatty acids, and bile acid biosynthesis along with antibiotic resistance and virulence. Another, meta-analysis by Reuben et al. analyzed the oral and nasopharyngeal or gut microbiome in COVID-19 patients and found a prominent reduction in the gut microbial diversity but not in the respiratory microbiome. Furthermore, Petakh et al. reported on the role of gut microbiota in COVID-19 progression in Type 2 diabetes (T2D) patients (Figure 1). The results showed a positive correlation between specific gut microbiota (Klebsiella spp. and Enterococcus spp.) and C-reactive protein levels and length of stay in COVID-19 patients with or without T2D. Overall, this study revealed the potential role of specific gut microbiota in COVID-19 patients with T2D, which can be used to develop treatment strategies for COVID-19 and associated T2D.
In the recent past, the impact of the gut microbiota on cardiovascular disease has also been demonstrated. An interesting review by Wang et al. explored the gut-heart axis and its role in myocarditis along with detailing the cross-linking of the immune system with the gut microbiota, and its metabolites. Next, Zhang et al. studied the gut microbiome in infants with congenital heart disease and showed the association between heart failure and the gut microbiome. The results suggested an abundance of Firmicutes, Actinobacteria, Proteobacteria, and Bacteroidetes at the phylum level and Enterococcus, Bifidobacterium, Subdoligranulum, Shigella, and Streptococcus at the genus level in the heart failure group of infants (Figure 1). Moreover, a noticeable reduction was observed in the alpha and beta diversity of the gut microbiome in the heart failure group in comparison to the control group along with a downregulation of the retinol metabolism. Overall, it is suggested that the gut microbiome could serve as a potential biomarker for the early diagnosis of heart disease.
The azomethane-dextran sodium sulfate (AOM-DSS) mouse model is well-established for the induction of acute colitis, but the success rate has always been an issue. A study of the gut microbiome associated with the AOM-DSS mouse model by Sun et al. revealed the important role of gut microbiota at the early stage of model development to improve the success rate of model construction. Gut microbiome analysis revealed uncontrolled proliferation of Pseudescherichia, Turicibacter, and Clostridium XVIII leading to death. A significant reduction was observed in the genera Ligilactobacillus, Lactobacillus, and Limosilactobacillus while Akkermansia and Ruthenibacterium were found to be increased in the gut of live AOM-DSS mice. The involvement of F. nucleatum in the progression of colitis has also been reported in the recent past. Taking this further, Duan et al. explored the role of fucose in the mice treated with F. nucleatum or fucose-treated F. nucleatum followed by colitis induction using DSS. The results suggested a protective role for fucose in colitis as the group of mice injected with fucose-treated F. nucleatum showed a reduced inflammatory response. It is revealed that the metabolic pathways of F. nucleatum are altered upon fucose treatment resulting in reduced pro-inflammatory properties of F. nucleatum. Overall it is suggested that fucose could be used as a functional food or prebiotic for the treatment of F. nucleatum-induced colitis. Next, a two-sample Mendelian Randomization (MR) study by Zhang et al. explored the relationship between gastroduodenal ulcers and gut microbiota employing a genome-wide association study (GWAS). The results demonstrated a negative correlation of gastroduodenal ulcers with the prevalence of Enterobacteriaceae, Butyricicoccus, Candidatus soleaferrea, Lachnospiraceae NC2004, Peptococcus, and Enterobacteriales while a positive correlation was observed with the abundance of Streptococcaceae, Lachnospiraceae UCG010, Marvinbryantia, Roseburia, Streptococcus, Mollicutes RF9, and NB1n. Overall, this study provides an important perspective for the gut microbiota-based therapeutic approaches for gastroduodenal ulcers. Similarly, another two-sample MR study by Wang et al. investigated the relationship between appendicitis and gut microbiota using GWAS. Their analysis revealed an inverse association of appendicitis risk with the prevalence of the genera Deltaproteobacteria, Christensenellaceae, Desulfovibrionaceae, Eubacteriumruminantium, Lachnospiraceae NK4A136, Methanobrevibacter, Desulfovibrionales, and Euryarchaeota while a positive correlation was found with the increased number of Family XIII, Howardella, and Veillonella which are associated with appendicitis susceptibility (Figure 1).
Interestingly, the gut microbiota has also been reported to be involved in dysbiosis-associated depression, but the detailed mechanism is unknown. An exciting study by Huang et al. examined chronic unpredictable mild stress (CUMS) and its association with the gut microbiome and NLRP3 inflammasome using fecal transplantation (FMT). It was found that FMT from CUMS rats to the antibiotic-treated rats showed enhanced NLRP3 inflammasomes along with increased levels of inflammatory cytokines. Moreover, probiotic treatment successfully balanced the altered gut microbiome induced by CUMS treatment and resulted in a diminished inflammatory response. Gut dysbiosis is also known to be associated with autoimmune diseases and associated inflammation (De Luca and Shoenfeld, 2019; Baindara, 2024). Chang and Choi recently reviewed the correlation of the gut microbiome with autoimmune diseases, especially focusing on Sjögren’s syndrome (SS), systemic lupus erythematosus (SLE), rheumatoid arthritis (RA), and multiple sclerosis (MS). The study suggests that gut dysbiosis in autoimmune diseases plays an essential role in the immune response by maintaining gut homeostasis, and so associated gut microbiota could be used as biomarkers for the treatment and early diagnosis of autoimmune diseases (Figure 1).
Overall, these studies have demonstrated the interplay of gut microbiota and defined microbial biomarkers in multiple infectious and autoimmune diseases (Figure 1). It is strongly recommended that specific gut microbiota could be used as therapeutic biomarkers in the early diagnosis of associated diseases. In addition, a general enrichment of the gut microbiome using probiotics could help reduce the incidence of diseases.
Figure 1 The gut microbiome in human health and disease. The upper panel shows the involvement of gut microbiota in the regulation of infectious and autoimmune diseases including cancer. The lower panel shows a schematic representation of the inverse relationship between inflammation, gut dysbiosis, and infection. CUMS, Chronic unpredictable mild stress; T2D, Type 2 diabetes; GS, Gallstone; PSC, Primary sclerosing cholangitis; PBC, Primary biliary cholangitis; BTC, Biliary tract cancer; R-CDI, C. difficile infection; CRC, Colorectal cancer; and HRA, High-risk adenoma.
PB: Conceptualization, Investigation, Project administration, Supervision, Validation, Visualization, Writing – original draft, Writing – review & editing. SM: Investigation, Supervision, Validation, Writing – review & editing.
The authors declare that the research was conducted in the absence of any commercial or financial relationship that could be construed as a potential conflict of interest.
All claims expressed in this article are solely those of the authors and do not necessarily represent those of their affiliated organizations, or those of the publisher, the editors and the reviewers. Any product that may be evaluated in this article, or claim that may be made by its manufacturer, is not guaranteed or endorsed by the publisher.
Baindara, P. (2024). Targeting interleukin-17 in radiation-induced toxicity and cancer progression. Cytokine Growth Factor Rev 75, 31–39. doi: 10.1016/j.cytogfr.2024.01.001
Baindara, P., Chakraborty, R., Holliday, Z. M., Mandal, S. M., Schrum, A. G. (2021). Oral probiotics in coronavirus disease 2019: connecting the gut–lung axis to viral pathogenesis, inflammation, secondary infection and clinical trials. New Microbes New Infect. 40, 100837. doi: 10.1016/j.nmni.2021.100837
De Luca, F., Shoenfeld, Y. (2019). The microbiome in autoimmune diseases. Clin. Exp. Immunol. 195, 74–85. doi: 10.1111/cei.13158
De Vos, W. M., Tilg, H., Van Hul, M., Cani, P. D. (2022). Gut microbiome and health: mechanistic insights. Gut 71, 1020–1032. doi: 10.1136/gutjnl-2021-326789
Keywords: gut-microbiome, infection, inflammation, autoimmune diseases, cancer
Citation: Baindara P and Mandal SM (2024) Editorial: The interplay of gut-microbiome between infection and inflammation. Front. Cell. Infect. Microbiol. 14:1413473. doi: 10.3389/fcimb.2024.1413473
Received: 07 April 2024; Accepted: 16 April 2024;
Published: 06 June 2024.
Edited and Reviewed by:
Benoit Chassaing, Institut National de la Santé et de la Recherche Médicale (INSERM), FranceCopyright © 2024 Baindara and Mandal. This is an open-access article distributed under the terms of the Creative Commons Attribution License (CC BY). The use, distribution or reproduction in other forums is permitted, provided the original author(s) and the copyright owner(s) are credited and that the original publication in this journal is cited, in accordance with accepted academic practice. No use, distribution or reproduction is permitted which does not comply with these terms.
*Correspondence: Piyush Baindara, piyush.baindara@gmail.com
†Present address: Santi M. Mandal, Department of Chemistry and Biochemistry, University of California San Diego, La Jolla, CA, United States
Disclaimer: All claims expressed in this article are solely those of the authors and do not necessarily represent those of their affiliated organizations, or those of the publisher, the editors and the reviewers. Any product that may be evaluated in this article or claim that may be made by its manufacturer is not guaranteed or endorsed by the publisher.
Research integrity at Frontiers
Learn more about the work of our research integrity team to safeguard the quality of each article we publish.