- 1Jiangsu Key Laboratory of Zoonosis/Jiangsu Co-Innovation Center for Prevention and Control of Important Animal Infectious Diseases and Zoonoses, Yangzhou University, Yangzhou, China
- 2Key Laboratory of Prevention and Control of Biological Hazard Factors (Animal Origin) for Agrifood Safety and Quality, Ministry of Agriculture of China, Yangzhou University, Yangzhou, China
Tigecycline serves as a last-resort antimicrobial agent against severe infections caused by multidrug-resistant bacteria. Tet(X) and its numerous variants encoding flavin-dependent monooxygenase can confer resistance to tigecycline, with tet(X4) being the most prevalent variant. This study aims to investigate the prevalence and characterize tigecycline resistance gene tet(X) in E. coli isolates from various origins in Yangzhou, China, to provide insights into tet(X) dissemination in this region. In 2022, we tested the presence of tet(X) in 618 E. coli isolates collected from diverse sources, including patients, pig-related samples, chicken-related samples, and vegetables in Yangzhou, China. The antimicrobial susceptibility of tet(X)-positive E. coli isolates was conducted using the agar dilution method or the broth microdilution method. Whole genome sequencing was performed on tet(X)-positive strains using Illumina and Oxford Nanopore platforms. Four isolates from pig or pork samples carried tet(X4) and exhibited resistance to multiple antimicrobial agents, including tigecycline. They were classified as ST542, ST10, ST761, and ST48, respectively. The tet(X4) gene was located on IncFIA8-IncHI1/ST17 (n=2), IncFIA18-IncFIB(K)-IncX1 (n=1), and IncX1 (n=1) plasmids, respectively. These tet(X4)-carrying plasmids exhibited high similarity to other tet(X4)-bearing plasmids with the same incompatible types found in diverse sources in China. They shared related genetic environments of tet(X4) associated with ISCR2, as observed in the first identified tet(X4)-bearing plasmid p47EC. In conclusion, although a low prevalence (0.65%) of tet(X) in E. coli strains was observed in this study, the horizontal transfer of tet(X4) among E. coli isolates mediated by pandemic plasmids and the mobile element ISCR2 raises great concerns. Thus, heightened surveillance and immediate action are imperative to curb this clinically significant resistance gene and preserve the efficacy of tigecycline.
Introduction
Globally, the rapid increase of multidrug-resistant (MDR) bacteria poses a serious threat to clinical therapy and public health, a concern underscored by the World Health Organization (WHO, 2014). Tigecycline, a semisynthetic glycylcycline approved by the US Food and Drug Administration (FDA) in 2005, is considered as a last-resort antimicrobial agent for treating severe infections caused by MDR bacteria, particularly carbapenem-resistant Enterobacteriaceae (Pournaras et al., 2016; Yaghoubi et al., 2022). It acts by binding reversibly to the 30S ribosomal subunit, impeding the entry of tRNA into the A site of the ribosome and thereby preventing the elongation of peptide chains (Pournaras et al., 2016; Yaghoubi et al., 2022; Stein and Babinchak, 2012). In contrast to first and second generation tetracyclines (e.g., tetracycline, doxycycline, and minocycline), tigecycline can evade classical resistance mechanisms of tetracyclines, such as tetracyclines efflux pump Tet(A) and the ribosomal protection protein Tet(M) (Pournaras et al., 2016; Yaghoubi et al., 2022). However, the clinical use of tigecycline has led to the gradual emergence of strains resistant to tigecycline (Deng et al., 2014; Pournaras et al., 2016; Yaghoubi et al., 2022).
Overexpression of efflux pumps (AcrAB-TolC and OqxAB), mutations in the plasmid-mediated tetracycline efflux pump gene tet(A), and chromosomally located monooxygenase gene tet(X) and its variant tet(X2) can result in low-level tigecycline resistance (Moore et al., 2005; Yaghoubi et al., 2022). However, the recent discovery of novel plasmid-borne tigecycline resistance genes conferring high-level resistance, namely tet(X3) in Acinetobacter baumannii and tet(X4) in Escherichia coli from animals in China in 2019, has significantly compromised the clinical efficacy of tigecycline (He et al., 2019). Following the identification of tet(X3) and tet(X4), a variety of tet(X) variants associated with tigecycline resistance have been successively unveiled, with tet(X4) emerging as the predominant variant (Fang et al., 2020; Rueda Furlan et al., 2023). The mobile tet(X4) gene has increasingly been detected in E. coli from various sources, including animals, food products, humans, and the environment, primarily in China (He et al., 2019; Fang et al., 2020; Li et al., 2020b, 2023; Dong et al., 2022; Chen et al., 2019b; Li et al., 2020a). Its sporadic presence has also been reported in countries beyond China, such as Thailand, Pakistan, Vietnam, Singapore, the United Kingdom, and Norway (Ding et al., 2020; Li et al., 2022, 2023; Marathe et al., 2021; Martelli et al., 2022);. Previous research has indicated that mobile element ISCR2 and various conjugative and mobilizable plasmids (e.g., IncQ, IncX1, IncFIB, and IncHI1) facilitate the horizontal transfer of tet(X4) in E. coli (He et al., 2019; Fang et al., 2020; Liu et al., 2022). ISCR2 belongs to the ISCR elements, which can transpose adjacent DNA sequences via rolling-circle (RC) transposition, mediated by a single copy of the element (Toleman et al., 2006). ISCR2 has been identified as a key factor in the dissemination of tet(X4) between plasmids and chromosomes across various species and hosts, forming the conserved transposable element ISCR2-tet(X4)-catD (Liu et al., 2022).
Although tigecycline is not approved for use in animals, the emergence of tet(X) and tigecycline resistance in farmed animals may be linked to the long-term and extensive use of tetracycline drugs, such as oxytetracycline and doxycycline in veterinary medicine (He et al., 2019). The rise and prevalence of the horizontally transferable tigecycline resistance gene tet(X4) in E. coli derived from animals and food sources raises concerns, due to the limited therapeutic options available. Consequently, there is an urgent need to assess and monitor the prevalence of tet(X) in pathogens. This study aims to investigate the prevalence and characterize tet(X4) in E. coli isolates from various origins in Yangzhou, China, to provide insights into the dissemination of tet(X4) in this region.
Materials and methods
Isolates and tet(X) detection
In 2022, a total of 618 E. coli isolates were collected from various sources, including patients (n=16), chicken meat (n=98), pork (n=108), chicken intestinal contents (n=86), pigs (n=241, feces and the pig farm environment), and vegetables (n=69) in Yangzhou, China (Supplementary Table S1). The samples were incubated in Luria-Bertani (LB) broth at 37°C for 18–24 h and then cultured on MacConkey agar at 37°C for 24 h. One pink colony per plate was further streaked on an eosin methylene blue (EMB) agar plate for 24 h at 37°C. One suspected E. coli isolate (metallic sheen color) was selected from each plate and subsequently confirmed by using matrix-assisted laser desorption/ionization time-of-flight mass spectrometry. The presence of the tet(X) gene was detected through PCR and Sanger sequencing using the universal primers tet(X)-F (5′-CCGTTGGACTGACTATGGC-3′) and tet(X)-R (5′-TCAACTTGCGTGTCGGTAA-3′) (Wang et al., 2019), with anticipated product of 475 bp. The tet(X) gene was amplified by PCR as follows: 94°C for 5 min; 30 cycles of penetration at 94°C for 30 s, annealing at 46°C for 30 s, and extension at 72 °for 30 s; and a 5 min final incubation at 72°C.
Antimicrobial susceptibility testing
We assessed the susceptibility of tet(X)-positive E. coli isolates to 14 antimicrobial agents, which included ampicillin, cefotaxime, meropenem, gentamicin, amikacin, streptomycin, tetracycline, tigecycline, chloramphenicol, florfenicol, nalidixic acid, ciprofloxacin, colistin and sulfamethoazole/trimethoprim. Antimicrobial susceptibility testing was conducted using the agar dilution method or the broth microdilution method (limited to tigecycline and colistin). E. coli ATCC 25922 served as the quality control strain. Results were interpreted following the guidelines of the Clinical and Laboratory Standards Institute (CLSI) M100, 32nd edition (CLSI, 2022). For tigecycline (≥ 1 mg/L), streptomycin (> 16 mg/L), and florfenicol (> 16 mg/L), interpretation was based on clinical breakpoint or epidemiological cut-off values established by the European Committee on Antimicrobial Susceptibility Testing (EUCAST; www.eucast.org).
Conjugation experiments
Conjugation experiments were conducted using tet(X)-positive E. coli strains as donor strains and E. coli C600 (with high-level streptomycin resistance) or J53 (sodium azide-resistant) as the recipient strain. In brief, donor and the recipient strains were inoculated in 2 mL of LB broth at 37°C, 180 r/min for 4 h, followed by mixing in a 1:4 ratio (v/v) and further incubation at 37°C for 24 h. Transconjugants were selected on MacConkey agar plates contained tigecycline (2 μg/mL) and streptomycin (3000 μg/mL) or sodium azide (100 μg/mL) and subsequently confirmed by detecting tet(X) through PCR as described above. Experiments were performed in triplicate.
Whole genome sequencing and analysis
The bacterial DNA from four tet(X4)-positive E. coli strains was extracted using the TIANamp Bacteria DNA Kit (Tiangen, Beijing, China). Subsequently, whole-genome sequencing was conducted using Illunina (NovaSeq) and Oxford Nanopore (MinION system) platforms. De novo hybrid assembly was carried out using Unicycler version 0.5.0 (https://github.com/rrwick/Unicycler) and further corrected using Pilon version 1.24. Genome sequences were analyzed for multilocus sequence typing (MLST), resistance genes, mutations, and plasmid replicons using the Centre for Genomic Epidemiology (CGE) pipeline (http://www.genomicepidemiology.org). Comparison of the tet(X4)-carrying plasmids with similar plasmids was conducted using BRIG (Alikhan et al., 2011).
Nucleotide sequence accession number
The whole-genome sequences of the four tet(X4)-positive isolates have been deposited in GenBank under accession numbers: PRJNA1039986.
Results
Characterization of tet(X4)-positive E. coli isolates
In the present study, we observed a low prevalence (0.65%) of tet(X) in E. coli strains obtained from diverse sources. Two strains from pigs in the same pig farm and two strains from pork obtained from the same farm′s market were positive for tet(X4), respectively (Table 1). All tet(X4)-positive isolates displayed resistance to tigecycline (MIC ≥ 8 mg/L) and also exhibited resistance to ampicillin (n=4), gentamicin (n=1), streptomycin (n=3), tetracycline (n=4), chloramphenicol (n=4), florfenicol (n=4), nalidixic acid (n=1), and sulfamethoxazole/trimethoprim (n=4) (Table 1). However, they remained susceptible to cefotaxime, meropenem, amikacin, colistin, ciprofloxacin, and fosfomycin (Table 1). In addition to tet(X4), these isolates also harbored other resistance genes, such as blaTEM-1b (n=4), aadA22 (n=2), strAB (n=1), tet(A) (n=3), qnrS1 (n=4), floR (n=4), lnu(G) (n=2), and sul3 (n=3) (Table 1). In addition, strain YZ22MPE54 had a single mutation in gyrA (S83L), which was responsible for its resistance to nalidixic acid. The presence of these resistance genes and mutation likely accounts for their multidrug resistance profiles.
Based on whole-genome sequencing, the MLST analysis revealed that four tet(X4)-positive E. coli strains belonged to sequence types ST542, ST10, ST761, and ST48, respectively (Table 1). The tet(X4) gene was identified on three different types of plasmids: IncFIA8-IncHI1/ST17 (n=2), IncFIA18-IncFIB(K)-IncX1 (n=1), and IncX1 (n=1) (Table 1). However, all tigecycline-resistant isolates failed to transfer tet(X4)-carrying plasmids to E. coli C600 and E. coli J53 through conjugation.
Characterization of the tet(X4)-carrying IncFIA8-IncHI1/ST17 plasmids pYUYZMPE6–1 and pYUYZMPE54 in E. coli strains YZ22MPE6 and YZ22MPE54
The complete genome sequences of E. coli strains YZ22MPE6 (ST542) and YZ22MPE54 (ST10) were obtained, each consisting of one chromosome (4,607,660 or 4,670,021 bp) and one to four plasmids (Supplementary Table S2). The tet(X4) gene in isolates YZ22MPE6 and YZ22MPE54 was located on IncFIA8-IncHI1/ST17 plasmids pYUYZMPE6–1 (191,776 bp) and pYUYZMPE54 (190,712 bp), respectively. Both plasmids carried five additional resistance genes including blaTEM-1b, aadA22, floR, tet(A), and qnrS1. They were almost identical to each other, except for the insertion of one copy of insertion sequence IS903 downstream of the parR gene in pYUYZMPE6–1, with 9-bp direct repeats (DR, 5’-CTCCAGTTA-3’). They also displayed high similarity (>99.9%) to other tet(X4)-carrying IncFIA8-IncHI1/ST17 plasmids isolated from various Enterobacteriaceae species in China, for instance, pPM4–5-tetX4 (E. coli, pig cecum, CP096833), pQZZ116-tetX-190K (E. fergusonii, pig feces, CP095844), pSZ6R-tetX4 (Citrobacter sp., pork, MW940627), pTECL_2–190k-tetX4 (Enterobacter cloacae, swine nasal swab, MZ773210), pXY36-tet(X4) (Morganella morganii, swine, ON390820), and pYPE10–190k-tetX4 (E. coli, pork, CP041449), and pYUGZP1-tetX (E. coli, pig, MW439255) (Figure 1A).
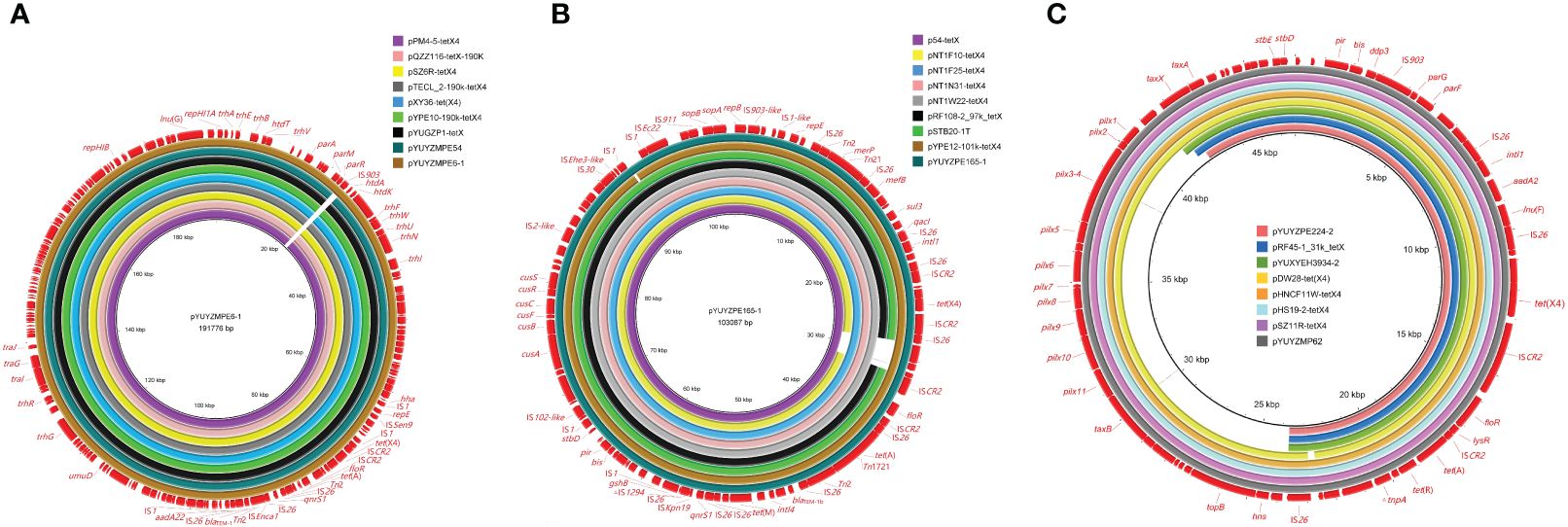
Figure 1 Sequence comparison of tet(X4)-carrying plasmids in this study with other similar plasmids using BRIG. (A) IncFIA8-IncHI1/ST17 plasmids pYUYZMPE6-1 and pYUYZMPE54 from E coli isolate YZ22MPE6 and YZ22MPE54; (B) IncFIA18-IncFIB(K)-IncX1 plasmid pYUYZPE165-1 from E. coli isolate YZ22PE165; (C) IncX1 plasmid pYUYZPE244-2 from E. coli isolate YZ22PE244. The outer circles in red with annotation are the reference plasmids pYUYZMPE6-1, pYUYZPE165-1, and pHS19-2-tetX4 (GenBank accession number MW940619), respectively.
Characterization of the tet(X4)-carrying IncFIA18-IncFIB(K)-IncX1 plasmid pYUYZPE165–1 in E. coli strain YZ22PE165
The complete genomic sequences of strain YZ22PE165 was obtained, comprising one chromosome (4,711,790 bp) and three plasmids named pYUYZPE165–1 to pYUYZPE165–3 (Supplementary Table S2). Notably, the largest plasmid, designated as pYUYZPE165–1, harbored tet(X4) and eight additional resistance genes [blaTEM-1b/tet(A)/tet(M)/qnrS1/floR/sul3/dfrA5/mef(B)]. This plasmid belonged to the hybrid IncFIA18-IncFIB(K)-IncX1 plasmid with a size of 103,087 bp. E. coli ST761 has been identified as a successful clone for transferring tet(X) associated with IncFIA18-IncFIB(K)-IncX1 plasmid across different sources in China (Li et al., 2020a, b; Li et al., 2021b; Wang et al., 2022; Zhai et al, 2022b). As shown in Figure 1B, pYUYZPE165–1 exhibited high similarity to tet(X4)-carrying IncFIA18-IncFIB(K)-IncX1 plasmids found in ST761 E. coli strains in China, with identities ranging from 99.88% to 99.98% and coverage from 96.17% too 100.00%, such as plasmids pNT1F10-tetX4 (CP075463), pNT1W22-tetX4 (CP075470), pRF108–2_97k_tetX (MT219820), and pSTB20–1T (CP050174) from pigs, p54-tetX (cow, CP041286) from a cow, and pYPE12–101k-tetX4 (CP041443) from a pork sample. Similar tet(X4)-carrying IncFIA18-IncFIB(K)-IncX1 plasmids have also been reported in other E. coli lineages, such as pNT1N31-tetX4 (pig, CP075481) and pNT1F25-tetX4 (pig, CP075471) obtained from ST716 and ST1421, respectively (Li et al., 2021b) (Figure 1B).
Characterization of the tet(X4)-carrying IncX1 plasmid pYUYZPE244–2 in E. coli strain YZ22PE244
The complete sequence of isolate YZ22PE244 was obtained. It consisted of a single chromosome (4,515,595 bp) and four plasmids, as detailed in Supplementary Table S2. Among them, tet(X4) and three additional resistance genes [aadA2, tet(A), lnu(F)] were found co-located on a 30,871 bp IncX1 plasmid designated as pYUYZPE244–2. This tet(X4)-carrying plasmid was similar to other IncX1 plasmids carrying tet(X4), such as pYUYZMP62 (MW439254, pig), pDW28-tet(X4) (ON390803, pig), pYY76–1-2 (CP040929, cow), pRF45–1_31k_tetX (MT219821, pig) and pYUXYEH3934–2 (CP110980, patient) from E. coli in China, pHNCF11W-tetX4 (CP053047, chicken) and pSZ11R-tetX4 (MW940629, pork) from Escherichia, and pHS19–2-tetX4 (MW940619, pork) from Klebsiella sp. (Figure 1C). However, pYUYZPE244–2 as well as plasmids pDW28-tet(X4), pRF45–1_31k_tetX and pYUXYEH3934–2 lacked the conjugative transfer region of IncX1 plasmids (Figure 1C), potentially explaining their failure in conjugation (Sun et al., 2023).
Related genetic environments of tet(X4) in four plasmids
Four tet(X4)-carrying plasmids in this study shared related genetic environments of tet(X4) to the first identified tet(X4)-bearing plasmid p47EC (170,312 bp, IncFIB) from E. coli strain 47EC, isolated from a fecal swab from a pig farm in China in 2018 (He et al., 2019). The tet(X4) gene was situated within a 6,456-bp region ISCR2-catD-tet(X4)-ISCR2 in p47EC, capable of forming a classical rolling circle transposable unit (RC-TU) mediated by ISCR2 (He et al., 2019). However, among the four tet(X4)-carrying plasmids in this study, intact ISCR2 was found only downstream of tet(X4), although one copy of IS26 was inserted into the origin of replication (oriIS) of ISCR2, flanked with 8-bp DRs (5’-TTTATTCT-3’) in pYUYZPE165–1(Figure 2). Upstream of catD-tet(X), the sequences were truncated by other insertion sequences, namely IS26 (pYUYZPE244–2 and pYUYZPE165–1) and IS1 (pYUYZMPE54 and pYUYZMPE6–1), resulting in a truncated ISCR2 upstream in pYUYZPE165–1 and the absence of ISCR2 in the remaining three plasmids (Figure 2). In contrast to p47EC, our four plasmids contained the florfenicol resistance gene floR downstream of the tet(X4) module, associated with an incomplete ISCR2 (Figure 2).
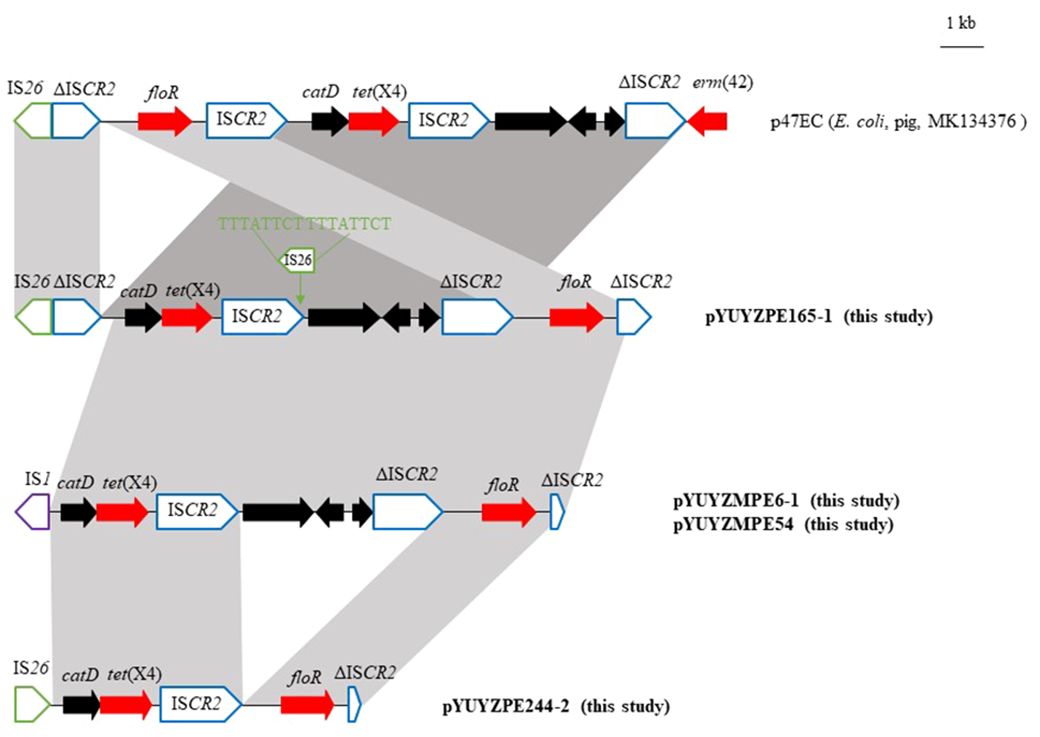
Figure 2 Genetic organization of the tet(X4) gene in this study and comparison with p47EC (MK134376). The extents and direction of antibiotic resistance (red arrows) and other genes (black arrows) are indicated. ISs are shown as boxes labeled with their name. labeled vertical arrows with IS box indicate the insertion site of IS element. Δ indicates a truncated mobile element. Direct repeats are indicated by arrows and sequences.
Discussion
Since its discovery in E. coli in 2019, the plasmid-mediated tigecycline resistance gene tet(X4) has been identified in various bacterial species, such as E. coli, Proteus, Raoultella ornithinolytica, Acinetobacter baumannii, Aeromonas caviae, Citrobacter freundii, Klebsiella pneumoniae, and Shewanella xiamenensis, with E. coli being the primary host (He et al., 2019; Dong et al., 2022; Chen et al., 2019a; Dao et al., 2022; Zeng et al., 2021; Zhai et al., 2022a). In our study, we tested tet(X4) in E. coli from diverse sources, revealing the exclusive presence of tet(X4) in E. coli originating from pigs and pork. Conversely, tet(X4) was not detected in E. coli isolates derived from other sources, including chickens, chicken meat, vegetables, and clinical specimens. However, it is imperative to acknowledge that the number of isolates from these sources was relatively limited, posing a constraint on our study. Currently, tet(X4) is commonly detected in E. coli originating from food-producing animals and food products, particularly pigs and pork, but it is infrequently reported in E. coli from humans (He et al., 2019; Sun et al., 2019a, 2020; Dong et al., 2022; Li et al., 2021a; Sun et al., 2023). The detection rate of tet(X4) in this study was relatively low (0.65%), aligning closely with the findings of Sun et al. (0.22%) (Sun et al., 2019a), Yu et al. (0.17%) (Yu et al., 2022), and Zhang et al. (0.32%) (Zhang et al., 2020) in their research on E. coli collected from China. However, in contrast, significantly higher detection rates of tet(X4) were reported in retail pork samples from Shandong and Sichuan provinces (20.6%, 7/34) (Bai et al., 2019) and across ten regions in China (39.56%, 55/139) (Li et al., 2021a).
Currently, a variety of E. coli strains with different sequence types (STs) carrying tet(X4) have been identified in animals, food, the environment, and humans (Li et al., 2020b, 2023; Sun et al., 2019b, 2020; Dong et al., 2022; Li et al., 2021a, b; Cui et al., 2022). Additionally, tet(X4)-positive plasmids with the same Inc type have been detected in E. coli strains sharing the same or different STs (Sun et al., 2019b, 2020; Li et al., 2021a; Cui et al., 2022). These observations suggest that plasmids are the primary means by which tet(X4) is disseminated among diverse E. coli strains. However, it is worth noting that the clonal spread of certain ST-type E. coli strains may also contribute to the dissemination of tet(X4). For instance, E. coli ST542, ST10, ST48, and ST761, which were identified in our study, represent common ST types known to carry tet(X4) in China and have been detected in animals, food products, the environment, and patients (Dong et al., 2022; Bai et al., 2019; Chen et al., 2019c; Zhang et al., 2020; Li et al., 2021a; Cui et al., 2022; Wang et al., 2022; Zhai et al., 2022b). Globally, ST10 and ST48 are the most prevalent clones in the tet(X)-positive E. coli isolates, detected in multiple countries and over 10 hosts (Li et al., 2023). Notably, certain tet(X)-positive E. coli isolates (e.g., ST10) from different geographical locations or hosts shared high genetic similarity (<200 SNPs), indicating possible clonal transmission (Li et al., 2023).
Plasmids are crucial vectors for tet(X4) transmission. Presently, tet(X4) is associated with diverse plasmids, such as IncX1, IncFIA/IncHI1, IncFIA18/IncFIB(K)/IncX1, IncFII, IncQ1, ColE2, IncA/C, and IncN plasmids (Fang et al., 2020; Li et al., 2020b; Li et al., 2021b; Cui et al., 2022; Wang et al., 2022). The tet(X4)-bearing plasmids identified in this study exhibit high similarity to those tet(X4)-carrying plasmids found in Enterobacteriaceae, particularly E. coli, of various origins. This observation underscores the role of horizontal transmission of prevalent plasmids in the dissemination of tet(X4) within Enterobacteriaceae. Certain plasmids with a complete conjugal transfer region carrying tet(X4) possess conjugative capabilities, allowing them to be horizontally transferred between distinct bacterial strains, greatly facilitating the widespread distribution of tet(X4). Conversely, plasmids lacking a conjugal transfer region, such as the tet(X4)-positive IncFIA18-IncFIB(K)-IncX1 plasmids in this study, are rendered non-conjugative. For instance, the IncX1 plasmid has been identified in E. coli from various sources and has demonstrated its capacity to transfer and persist within a range of Enterobacteriaceae (Li et al., 2020b; Cui et al., 2022; Chen et al., 2019c). However, our study found that the IncX1 plasmid pYUYZPE244–2 was incapable of conjugative transfer, possibly due to the absence of the conjugal transfer region compared to other tet(X4)-positive IncX1 plasmids. On the contrary, the presence of IncI2, IncX4, and IncFII conjugative plasmids carrying mcr-1 could serve as helper plasmids, enabling the conjugative transfer of non-conjugative tet(X4)-positive IncX1 or IncQ plasmids (Sun et al., 2019b; He et al., 2020). Furthermore, the non-conjugative tet(X4) plasmid can undergo homologous recombination with conjugative IncFII plasmid mediated by IS26, resulting in the formation of a novel fusion plasmid, thus, achieving the conjugative transfer of tet(X4) (Du et al., 2020). In our investigation, we also discovered that plasmids carrying tet(X4) often bear a variety of drug resistance genes, such as blaTEM-1b, qnrS1, and floR, which confer resistance to β-lactams, quinolones, and florfenicol. It suggests that these commonly used antibiotics in livestock may exert a co-selective pressure on tet(X4).
The horizontal transfer of tet(X4) between different plasmids, plasmids or chromosomes is associated with the insertion sequence ISCR2 (He et al., 2019; Chen et al., 2019b; Liu et al., 2022). ISCR2 is present upstream and/or downstream of tet(X4) and other tet(X) variants such as tet(X3), tet(X5), and tet(X6) (He et al., 2019; Wang et al., 2019; He et al., 2020; Liu et al., 2022). As revealed in prior research, ISCR2 carries adjacent regions including tet(X4) to form a 4,608 bp RC-TU, and it could also capture more resistance genes such as floR to form a larger RC-TU, although the ISCR2-tet(X4) element was frequently truncated by other insertion sequences such as IS26 (Liu et al., 2022). Additionally, the insertion sequence IS1 can also mediate the transfer of tet(X4) (Yu et al., 2022).
Conclusions
While the prevalence of tet(X4) within E. coli isolates in this study was relatively low, the horizontal transfer of this resistance gene within E. coli strains associated with pandemic plasmids (IncFIA8-IncHI1, IncFIA18-IncFIB(K)-IncX1, and IncX1) and mobile elements such as ISCR2 is a matter of great concern. Mobile elements and plasmids play a pivotal role in rapid dissemination of this clinically crucial resistance gene in E. coli originating from livestock and animal-derived food products. It has the potential for this gene to be transmitted to humans through the food chain. The detection of tet(X4) among E. coli isolates from animals and food products raises substantial public health concerns, necessitating enhanced surveillance and immediate action to control this medically significant resistance gene and uphold the efficacy of tigecycline.
Data availability statement
The datasets presented in this study can be found in online repositories. The names of the repository/repositories and accession number(s) can be found in the article/Supplementary Material.
Ethics statement
The studies involving humans were approved by Jiangsu Key Laboratory of Zoonosis, Yangzhou University. The studies were conducted in accordance with the local legislation and institutional requirements. The human samples used in this study were acquired from gifted from another research group. Written informed consent for participation was not required from the participants or the participants’ legal guardians/next of kin in accordance with the national legislation and institutional requirements. The animal studies were approved by Jiangsu Key Laboratory of Zoonosis, Yangzhou University. The studies were conducted in accordance with the local legislation and institutional requirements. Written informed consent was obtained from the owners for the participation of their animals in this study.
Author contributions
X-YF: Investigation, Writing – original draft. Y-J: Formal analysis, Investigation, Writing – review & editing. HW: Writing – original draft. JL: Investigation, Writing – review & editing. Q-YG: Investigation, Writing – review & editing. Z-YW: Formal analysis, Software, Writing – review & editing. LS: Conceptualization, Resources, Writing – review & editing. XJ: Funding acquisition, Writing – review & editing. QL: Conceptualization, Writing – review & editing. JW: Conceptualization, Formal analysis, Supervision, Writing – original draft, Writing – review & editing.
Funding
The author(s) declare financial support was received for the research, authorship, and/or publication of this article. This work was supported in part by the Training Program of Innovation for College Students in Jiangsu Province (202211117085Y), the fifth phase of the scientific research 333 project in Jiangsu Province (BRA2020002), and Key Research and Development Program (Modern Agriculture) project of Jiangsu Province (BE2021331).
Conflict of interest
The authors declare that the research was conducted in the absence of any commercial or financial relationships that could be construed as a potential conflict of interest.
Publisher’s note
All claims expressed in this article are solely those of the authors and do not necessarily represent those of their affiliated organizations, or those of the publisher, the editors and the reviewers. Any product that may be evaluated in this article, or claim that may be made by its manufacturer, is not guaranteed or endorsed by the publisher.
Supplementary material
The Supplementary Material for this article can be found online at: https://www.frontiersin.org/articles/10.3389/fcimb.2024.1399732/full#supplementary-material
References
Alikhan, N. F., Petty, N. K., Ben Zakour, N. L., Beatson, S. A. (2011). BLAST Ring Image Generator (BRIG): simple prokaryote genome comparisons. BMC Genomics 12, 402. doi: 10.1186/1471-2164-12-402
Bai, L., Du, P., Du, Y., Sun, H., Zhang, P., Wan, Y., et al. (2019). Detection of plasmid-mediated tigecycline-resistant gene tet(X4) in Escherichia coli from pork, Sichuan and Shandong Provinces, China, February 2019. Euro Surveill. 24, 1900340. doi: 10.2807/1560-7917.ES.2019.24.25.1900340
Chen, C., Chen, L., Zhang, Y., Cui, C. Y., Wu, X. T., He, Q., et al. (2019a). Detection of chromosome-mediated tet(X4)-carrying Aeromonas caviae in a sewage sample from a chicken farm. J. Antimicrob. Chemother. 74, 3628–3630. doi: 10.1093/jac/dkz387
Chen, C., Cui, C. Y., Zhang, Y., He, Q., Wu, X. T., Li, G., et al. (2019b). Emergence of mobile tigecycline resistance mechanism in Escherichia coli strains from migratory birds in China. Emerg. Microbes Infect. 8, 1219–1222. doi: 10.1080/22221751.2019.1653795
Chen, C., Wu, X. T., He, Q., Chen, L., Cui, C. Y., Zhang, Y., et al. (2019c). Complete sequence of a tet(X4)-harboring IncX1 plasmid, pYY76-1-2, in Escherichia coli from a cattle sample in China. Antimicrob. Agents Chemother. 63, e01528–e01519. doi: 10.1128/AAC.01528-19
CLSI (2022). Clinical and Laboratory Standards Institute (CLSI). PerformanceStandards for Antimicrobial Susceptibility Testing. CLSI Supplement M100, 32th Edn. (Wayne, PA: CLSI).
Cui, C. Y., Li, X. J., Chen, C., Wu, X. T., He, Q., Jia, Q. L., et al. (2022). Comprehensive analysis of plasmid-mediated tet(X4)-positive Escherichia coli isolates from clinical settings revealed a high correlation with animals and environments-derived strains. Sci. Total Environ. 806, 150687. doi: 10.1016/j.scitotenv.2021.150687
Dao, T. D., Kasuga, I., Hirabayashi, A., Nguyen, D. T., Tran, H. T., Vu, H., et al. (2022). Emergence of mobile tigecycline resistance gene tet(X4)-harbouring Shewanella xiamenensis in a water environment. J. Glob Antimicrob. Resist. 28, 140–142. doi: 10.1016/j.jgar.2021.12.022
Deng, M., Zhu, M. H., Li, J. J., Bi, S., Sheng, Z. K., Hu, F. S., et al. (2014). Molecular epidemiology and mechanisms of tigecycline resistance in clinical isolates of Acinetobacter baumannii from a Chinese university hospital. Antimicrob. Agents Chemother. 58, 297–303. doi: 10.1128/AAC.01727-13
Ding, Y., Saw, W. Y., Tan, L. W. L., Moong, D. K. N., Nagarajan, N., Teo, Y. Y., et al. (2020). Emergence of tigecycline- and eravacycline-resistant Tet(X4)-producing Enterobacteriaceae in the gut microbiota of healthy Singaporeans. J. Antimicrob. Chemother. 75, 3480–3484. doi: 10.1093/jac/dkaa372
Dong, N., Zeng, Y., Cai, C., Sun, C., Lu, J., Liu, C., et al. (2022). Prevalence, transmission, and molecular epidemiology of tet(X)-positive bacteria among humans, animals, and environmental niches in China: An epidemiological, and genomic-based study. Sci. Total Environ. 818, 151767. doi: 10.1016/j.scitotenv.2021.151767
Du, P., Liu, D., Song, H., Zhang, P., Li, R., Fu, Y., et al. (2020). Novel IS26-mediated hybrid plasmid harbouring tet(X4) in Escherichia coli. J. Glob Antimicrob. Resist. 21, 162–168. doi: 10.1016/j.jgar.2020.03.018
Fang, L. X., Chen, C., Cui, C. Y., Li, X. P., Zhang, Y., Liao, X. P., et al. (2020). Emerging high-level tigecycline resistance: novel tetracycline destructases spread via the mobile Tet(X). Bioessays 42, e2000014. doi: 10.1002/bies.202000014
He, T., Wang, R., Liu, D., Walsh, T. R., Zhang, R., Lv, Y., et al. (2019). Emergence of plasmid-mediated high-level tigecycline resistance genes in animals and humans. Nat. Microbiol. 4, 1450–1456. doi: 10.1038/s41564-019-0445-2
He, D., Wang, L., Zhao, S., Liu, L., Liu, J., Hu, G., et al. (2020). A novel tigecycline resistance gene, tet(X6), on an SXT/R391 integrative and conjugative element in a Proteus genomospecies 6 isolate of retail meat origin. J. Antimicrob. Chemother. 75, 1159–1164. doi: 10.1093/jac/dkaa012
He, T., Wei, R., Li, R., Zhang, L., Sun, L., Bao, H., et al. (2020). Co-existence of tet(X4) and mcr-1 in two porcine Escherichia coli isolates. J. Antimicrob. Chemother. 75, 764–766. doi: 10.1093/jac/dkz510
Li, R., Li, Y., Peng, K., Yin, Y., Liu, Y., He, T., et al. (2021a). Comprehensive genomic investigation of tigecycline resistance gene tet(X4)-bearing strains expanding among different settings. Microbiol. Spectr. 9, e0163321. doi: 10.1128/spectrum.01633-21
Li, R., Lu, X., Liu, Z., Liu, Y., Xiao, X., Wang, Z. (2020a). Rapid detection and characterization of tet(X4)-positive Escherichia coli strains with nanopore sequencing. J. Antimicrob. Chemother. 75, 1068–1070. doi: 10.1093/jac/dkz528
Li, R., Lu, X., Munir, A., Abdullah, S., Liu, Y., Xiao, X., et al. (2022). Widespread prevalence and molecular epidemiology of tet(X4) and mcr-1 harboring Escherichia coli isolated from chickens in Pakistan. Sci. Total Environ. 806, 150689. doi: 10.1016/j.scitotenv.2021.150689
Li, R., Lu, X., Peng, K., Liu, Z., Li, Y., Liu, Y., et al. (2020b). Deciphering the structural diversity and classification of the mobile tigecycline resistance gene tet(X)-bearing plasmidome among bacteria. mSystems 5, e00134–e00120. doi: 10.1128/mSystems.00134-20
Li, Y., Sun, X., Xiao, X., Wang, Z., Li, R. (2023). Global distribution and genomic characteristics of tet(X)-positive Escherichia coli among humans, animals, and the environment. Sci. Total Environ. 887, 164148. doi: 10.1016/j.scitotenv.2023.164148
Li, Y., Wang, Q., Peng, K., Liu, Y., Xiao, X., Mohsin, M., et al. (2021b). Distribution and genomic characterization of tigecycline-resistant tet(X4)-positive Escherichia coli of swine farm origin. Microb. Genom. 7, 667. doi: 10.1099/mgen.0.000667
Liu, D., Wang, T., Shao, D., Song, H., Zhai, W., Sun, C., et al. (2022). Structural diversity of the ISCR2-mediated rolling-cycle transferable unit carrying tet(X4). Sci. Total Environ. 826, 154010. doi: 10.1016/j.scitotenv.2022.154010
Marathe, N. P., Svanevik, C. S., Ghavidel, F. Z., Grevskott, D. H. (2021). First report of mobile tigecycline resistance gene tet(X4)-harbouring multidrug-resistant Escherichia coli from wastewater in Norway. J. Glob Antimicrob. Resist. 27, 37–40. doi: 10.1016/j.jgar.2021.07.019
Martelli, F., AbuOun, M., Cawthraw, S., Storey, N., Turner, O., Ellington, M., et al. (2022). Detection of the transferable tigecycline resistance gene tet(X4) in Escherichia coli from pigs in the United Kingdom. J. Antimicrob. Chemother. 77, 846–848. doi: 10.1093/jac/dkab439
Moore, I. F., Hughes, D. W., Wright, G. D. (2005). Tigecycline is modified by the flavin-dependent monooxygenase TetX. Biochemistry 44, 11829–11835. doi: 10.1021/bi0506066
Pournaras, S., Koumaki, V., Spanakis, N., Gennimata, V., Tsakris, A. (2016). Current perspectives on tigecycline resistance in Enterobacteriaceae: susceptibility testing issues and mechanisms of resistance. Int. J. Antimicrob. Agents. 48, 11–18. doi: 10.1016/j.ijantimicag.2016.04.017
Rueda Furlan, J. P., Fuentes-Castillo, D., Guedes Stehling, E., Lincopan, N., Sellera, F. P. (2023). The emergence of tet(X) variants highlight challenges for the global genomic surveillance of tigecycline resistance. Lancet Microbe 4, e857. doi: 10.1016/S2666-5247(23)00249-5
Stein, G. E., Babinchak, T. (2013). Tigecycline: an update. Diagn. Microbiol. Infect. Dis. 75, 331–336. doi: 10.1016/j.diagmicrobio.2012.12.004
Sun, J., Chen, C., Cui, C. Y., Zhang, Y., Liu, X., Cui, Z. H., et al. (2019b). Plasmid-encoded tet(X) genes that confer high-level tigecycline resistance in Escherichia coli. Nat. Microbiol. 4, 1457–1464. doi: 10.1038/s41564-019-0496-4
Sun, C., Cui, M., Zhang, S., Liu, D., Fu, B., Li, Z., et al. (2020). Genomic epidemiology of animal-derived tigecycline-resistant Escherichia coli across China reveals recent endemic plasmid-encoded tet(X4) gene. Commun. Biol. 3, 412. doi: 10.1038/s42003-020-01148-0
Sun, C., Cui, M., Zhang, S., Wang, H., Song, L., Zhang, C., et al. (2019a). Plasmid-mediated tigecycline-resistant gene tet(X4) in Escherichia coli from food-producing animals, China 2008-2018. Emerg. Microbes Infect. 8, 1524–1527. doi: 10.1080/22221751.2019.1678367
Sun, L., Sun, G. Z., Jiang, Y., Mei, C. Y., Wang, Z. Y., Wang, H. Y., et al. (2023). Low prevalence of mobilized resistance genes blaNDM, mcr-1, and tet(X4) in Escherichia coli from a hospital in China. Front. Microbiol. 14. doi: 10.3389/fmicb.2023.1181940
Toleman, M. A., Bennett, P. M., Walsh, T. R. (2006). ISCR elements: novel gene-capturing systems of the 21st century? Microbiol. Mol. Biol. Rev. 70, 296–316. doi: 10.1128/MMBR.00048-05
Wang, L., Liu, D., Lv, Y., Cui, L., Li, Y., Li, T., et al. (2019). Novel plasmidmediated tet(X5) gene conferring resistance to tigecycline, eravacycline, and omadacycline in a clinical Acinetobacter baumannii isolate. Antimicrob. Agents Chemother. 64, e01326–e01319. doi: 10.1128/AAC.01326-19
Wang, J., Lu, M. J., Wang, Z. Y., Jiang, Y., Wu, H., Pan, Z. M., et al. (2022). Tigecycline-resistant Escherichia coli ST761 carrying tet(X4) in a pig farm, China. Front. Microbiol. 13. doi: 10.3389/fmicb.2022.967313
WHO (2014). Antimicrobial resistance: global report on surveillance. Available online at: https://www.who.int/publications/i/item/9789241564748.
Yaghoubi, S., Zekiy, A. O., Krutova, M., Gholami, M., Kouhsari, E., Sholeh, M., et al. (2022). Tigecycline antibacterial activity, clinical effectiveness, and mechanisms and epidemiology of resistance: Narrative review. Eur. J. Clin. Microbiol. Infect. Dis. 41, 1003–1022. doi: 10.1007/s10096-020-04121-1
Yu, R., Chen, Z., Schwarz, S., Yao, H., Du, X. D. (2022). Mobilization of tet(X4) by IS1 family elements in porcine Escherichia coli isolates. Antimicrob. Agents Chemother. 66, e0159721. doi: 10.1128/AAC.01597-21
Zeng, Y., Dong, N., Liu, C., Lu, J., Zhang, R. (2021). Presence of tet(X4)-positive Citrobacter freundii in cancer patient with chemotherapy-induced persistent diarrhea. J. Glob Antimicrob. Resist. 24, 88–89. doi: 10.1016/j.jgar.2020.11.007
Zhai, W., Tian, Y., Lu, M., Zhang, M., Song, H., Fu, Y., et al. (2022a). Presence of mobile tigecycline resistance gene tet(X4) in clinical Klebsiella pneumoniae. Microbiol. Spectr. 10, e0108121. doi: 10.1128/spectrum.01081-21
Zhai, W., Wang, T., Yang, D., Zhang, Q., Liang, X., Liu, Z., et al. (2022b). Clonal relationship of tet(X4)-positive Escherichia coli ST761 isolates between animals and humans. J. Antimicrob. Chemother. 77, 2153–2157. doi: 10.1093/jac/dkac175
Keywords: tet(X4), tigecycline resistance, public health, plasmid, mobile element
Citation: Fan X-Y, Jiang Y, Wu H, Liu J, Gu Q-Y, Wang Z-Y, Sun L, Jiao X, Li Q and Wang J (2024) Distribution and spread of tigecycline resistance gene tet(X4) in Escherichia coli from different sources. Front. Cell. Infect. Microbiol. 14:1399732. doi: 10.3389/fcimb.2024.1399732
Received: 12 March 2024; Accepted: 17 June 2024;
Published: 28 June 2024.
Edited by:
Juan Carlos Rodriguez Diaz, Hospital General Universitario de Alicante, SpainReviewed by:
Yingwang Ye, Hefei University of Technology, ChinaMashkoor Mohsin, University of Agriculture, Faisalabad, Pakistan
Copyright © 2024 Fan, Jiang, Wu, Liu, Gu, Wang, Sun, Jiao, Li and Wang. This is an open-access article distributed under the terms of the Creative Commons Attribution License (CC BY). The use, distribution or reproduction in other forums is permitted, provided the original author(s) and the copyright owner(s) are credited and that the original publication in this journal is cited, in accordance with accepted academic practice. No use, distribution or reproduction is permitted which does not comply with these terms.
*Correspondence: Qiuchun Li, cWNsaUB5enUuZWR1LmNu; Jing Wang, d2oxMjMxQHl6dS5lZHUuY24=
†These authors have contributed equally to this work