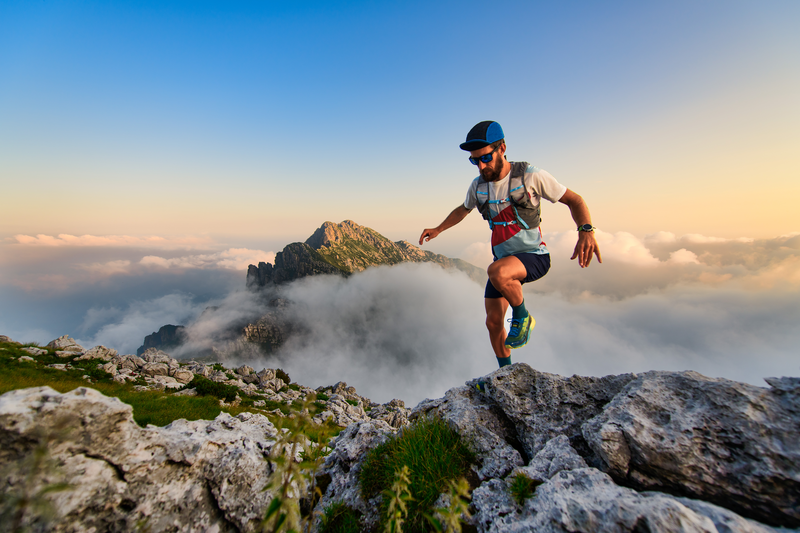
95% of researchers rate our articles as excellent or good
Learn more about the work of our research integrity team to safeguard the quality of each article we publish.
Find out more
ORIGINAL RESEARCH article
Front. Cell. Infect. Microbiol. , 15 February 2024
Sec. Intestinal Microbiome
Volume 14 - 2024 | https://doi.org/10.3389/fcimb.2024.1347110
Background: Increasing evidence suggests a close association between the intestinal microbiome and the respiratory system, drawing attention to studying the gut-lung axis. This research employs bibliometric methods to conduct a visual analysis of literature in the field of intestinal microbiota and lung diseases over the past two decades. It offers scientific foundations for research directions and critical issues in this field.
Methods: We retrieved all articles on intestinal microbiota and lung diseases from the SCI-Expanded of WoSCC on October 25, 2023. The analysis included original articles and reviews published in English from 2011 to 2023. We utilized Python, VOSviewer, and CiteSpace to analyze the retrieved data visually.
Results: A total of 794 publications were analyzed. China ranked first in the number of publications, while the United States had the highest citations and H-index. Jian Wang was the most prolific author. Zhejiang University was the institution with the highest number of publications. Frontiers in Microbiology was the journal with the most publications. Author keywords appearing more than 100 times included “intestinal microbiota/microbiome”, “microbiota/microbiome”, and “gut-lung axis”.
Conclusion: The correlation and underlying mechanisms between intestinal microbiota and lung diseases, including asthma, COPD, lung cancer, and respiratory infections, remain hot topics in research. However, understanding the mechanisms involving the gut-lung axis is still in its infancy and requires further elucidation.
The intestinal microbiota refers to the microbial ecosystem within the human gastrointestinal tract, encompassing bacteria, fungi, viruses, and other microorganisms. The gene pool of this microbiota exceeds that of the human genome by a hundredfold (O’Hara and Shanahan, 2006; Grice and Segre, 2012; Ley et al., 2006). Changes in the composition and diversity of these microorganisms can impact not only the colonized organ but also distant organs and systems (Feng et al., 2018). The intestinal microbiota is closely linked to human health and is associated with various diseases, such as gastrointestinal disorders, metabolic diseases, and immune-related illnesses (Zhou et al., 2021; de Steenhuijsen Piters et al., 2015; Clemente et al., 2012). Current research indicates that dysbiosis of the intestinal microbiota is a fundamental cause of numerous gastrointestinal and non-gastrointestinal diseases and a range of mental health conditions (Carding et al., 2015; Forsythe et al., 2016). Recent evidence suggests intestinal microbiota is intricately connected to the respiratory system, acting as a critical regulatory factor in developing lung diseases like pneumonia, asthma, and chronic obstructive pulmonary disease (COPD) (Wang et al., 2017). While research on the gut-lung axis is still in its early stages, it holds potential as a novel approach for treating pulmonary diseases (Feng et al., 2018).
Bibliometrics is a quantitative research method used to analyze and assess scientific literature’s volume, quality, distribution, and impact. It involves statistical analysis of citations, author collaborations, and journal impact factors (Ma et al., 2021). Employing bibliometric methods can reveal the latest scientific research trends, hot topics, and interdisciplinary fields, as well as evaluate the impact and quality of academic achievements, providing decision support for the advancement of scientific research (Guler et al., 2016). No study has systematically explored the interrelationship between intestinal microbiota and lung diseases using bibliometric methods. This study aims to employ bibliometric techniques to quantitatively analyze research on the intestinal microbiota and the lung, providing a scientific basis for research directions and significant issues in this field.
We systematically searched the WoSCC database, setting the search timeframe from the database’s inception to 2023, with the search completed on October 25, 2023. A total of 1364 articles were retrieved, and the selection process was finalized on October 27, 2023. The search strategy was as follows:
1: [TI=(“gut microbiota” OR “intestinal microbiota” OR “fecal microbiota” OR “gastrointestinal microbiota” OR “gut microbiome” OR “intestinal microbiome” OR “fecal microbiome” OR “gastrointestinal microbiome” OR “intestinal bacteria” OR “gut bacteria” OR “fecal bacteria” OR “gastrointestinal bacteria” OR “intestinal flora” OR “gut flora” OR “fecal flora” OR “gastrointestinal flora” OR “gut microflora” OR “intestinal microflora” OR “fecal microflora” OR “gastrointestinal microflora”)] OR AB=(“gut microbiota” OR “intestinal microbiota” OR “fecal microbiota” OR “gastrointestinal microbiota” OR “gut microbiome” OR “intestinal microbiome” OR “fecal microbiome” OR “gastrointestinal microbiome” OR “intestinal bacteria” OR “gut bacteria” OR “fecal bacteria” OR “gastrointestinal bacteria” OR “intestinal flora” OR “gut flora” OR “fecal flora” OR “gastrointestinal flora” OR “gut microflora” OR “intestinal microflora” OR “fecal microflora” OR “gastrointestinal microflora”)
2: [TI=(“pulmonary” OR “lung”)] OR AB=(“pulmonary” OR “lung”)
3: #1 AND #2
Two reviewers independently identified and discussed potential discrepancies in the data search, ultimately reaching a consensus. After excluding articles written in non-English and those whose titles and abstracts did not match the research content, and by restricting the publication type to reviews and original articles, we obtained 794 articles that met the criteria for inclusion in the analysis (Figure 1).
This approach ensured a comprehensive and focused collection of relevant literature, providing a robust foundation for the subsequent bibliometric analysis.
The original data were extracted from WoSCC database, including paper and citation numbers, publication year, country/region, affiliation, authors, journals, references, and keywords. The data were imported into VOSviewer (version 1.6.19) and CiteSpace (version 6.2.R3) for subsequent bibliometric analysis.
The Web of Science (WoS) Core Collection database, known for its rigorous journal selection, has become internationally recognized for evaluating the scientific achievements of scholars and institutions and for assessing the development of disciplines (Sun et al., 2022). Therefore, our research chose the WoS database.
CiteSpace is a software tool for visualizing and analyzing academic literature citation networks. It automatically generates citation network graphs and calculates citation frequency, keyword distribution, collaboration among authors, and displays this information in visual charts. CiteSpace also offers a range of analysis tools, such as clustering analysis and keyword co-occurrence analysis, to help researchers identify important research themes, hotspots, and influential papers in academic fields (Synnestvedt et al., 2005).
Similar to CiteSpace, VOSviewer supports visualization analysis based on literature citation networks. Using VOSviewer, researchers can easily explore citation relationships between literature and research hotspots. It aids in quickly grasping an overview of large-scale literature data, assisting in identifying field hotspots, research trends, and relevant literature resources and research directions related to specific research questions (van Eck and Waltman, 2010; Pan et al., 2018). In the generated network graphs, nodes can represent different countries, institutions, authors, or keywords. The size of a node reflects the number of publications associated with it, while different colors represent different clusters or years. Lines between nodes reveal collaboration or citation relationships.
Python is an open-source programming language known for its flexibility and productivity. Compared to traditional data visualization tools, Python offers the availability of multiple plotting and graphics libraries and the customization of various types of advanced charts (Wang and Maniruzzaman, 2022). Our study utilized Python to extract multiple key attributes from the literature, such as publication year, authors, institutions, countries, research fields, journals, references, and author keywords, followed by comprehensive statistical analysis. To visually represent the data, we also created bubble charts illustrating the annual publication trends in various research fields, journals, and author keywords. In these charts, the diameter of a bubble symbolizes the most prominent research field, journal, or author keyword in a particular year, and the number inside the bubble indicates the corresponding number of publications (Sun et al., 2022).
Before initiating the bibliometric analysis, we meticulously processed the raw data using Python. Our data cleaning process involved several key steps:
a. Country Consolidation: We merged countries that are geographically or politically part of the same entity. For example, Scotland, England, and Northern Ireland were combined under “United Kingdom”.
b. Synonym Merging for Countries: We consolidated synonyms for countries, such as replacing “Peoples R China” and “Taiwan” with “China”.
c. Standardization of Country Abbreviations: We standardized country abbreviations, for instance, converting “USA” to “United States”.
d. Keyword Standardization: To avoid loss of information due to synonyms among keywords, we deduced author keywords and replaced synonyms with standardized terms.
e. Author Identity Verification: We conducted thorough identity verification to eliminate confusion caused by authors with the same name. Besides using ORCID information for identity verification, we also referred to reliable sources such as official institutional websites.
These steps ensured the accuracy and consistency of our data, which is crucial for the integrity and reliability of our bibliometric analysis.
Figure 2 illustrates the trends in annual publication volume and citation frequency. The total number of articles surged from 3 in 2011 to 190 in 2022. Since 2011, lung and intestinal microbiota research has shown an upward trend. The annual publication volume exceeded 150 articles starting in 2021, indicating a continuous increase in the number of scholars conducting related research and a flourishing development of theories in lung and intestinal microbiota research.
Figure 2 Trends in the growth of publications and the number of citations in intestinal microbiota and lung disease.
Since the database’s inception, 5,710 authors have participated in lung and intestinal microbiota research, publishing a total of 794 articles. Table 1 displays the top 10 core authors, their publication count, total citations, and H-index. The top 10 authors published 63 articles collectively, totalling 1,750 citations. In terms of publication count, Jian Wang leads with 8 publications, followed by Derrick R Samuelson with 7 publications. Among them, 4,963 authors have published only one article, and only 23 have published five or more. Regarding total citations, Benjamin J Marsland leads with 2,522 citations, though outside the top 10 authors. Eva S Gollwitzer followed them with 2,120 citations and Aurelien Trompette with 2,052 citations. Regarding H-index, Valentin Sencio and Francois Trottein are in the leading positions. When assessing prolific authors, it is essential to consider the quantity of articles and the quality and publication timeline.
Among the top 10 authors in terms of publication volume, 5 are based in China, 3 in the United States, and 2 in France. This data indicates the concentration of research in this field in specific countries. VOSviewer software was used to depict the collaborations among authors in this field (Figures 3, 4). We set a threshold in the statistical analysis, requiring authors to have published at least 2 articles; ultimately, only 720 authors met this threshold. After removing unconnected nodes, 89 authors met the threshold. In the network visualization, the size of a node increases with the number of contributions by the author. The larger the node, the more articles the author has published.
1,431 institutions systematically published articles on the lung and intestinal microbiota. The majority of the top 10 institutions in terms of publication volume are from China. Zhejiang University in China ranks first in the number of publications and the H-index, with 26 publications, 878 total citations, and an H-index of 14. Fudan University is second with 18 publications, 297 total citations, and an H-index 8. Shanghai Jiao Tong University (17 publications, 470 total citations) and Sun Yat-sen University (17 publications, 310 total citations) are tied for third place (Table 2).
Institutional collaboration analysis was conducted using VOSviewer software, and an institutional collaboration network map was constructed (Figure 5). The minimum publication threshold was set to 5, with 66 institutions meeting this threshold. After removing unconnected nodes, 61 institutions met the criteria. As shown in Figure 5, there is close collaboration among these institutions.
Our analysis included 57 countries. The top 10 countries/regions were ranked based on the total number of publications by all authors. China had the highest publication volume, accounting for 45.2%, followed by the United States (21.8%) and France (5.3%) (Table 3, Figure 6). Only China and the United States published more than 100 articles. Although China had the highest number of publications, the United States led in total citations and H-index, indicating a higher quality of articles. The United States maintained the most vital international collaboration partnerships with other countries in this field.
794 articles related to the lung and intestinal microbiota were published across 333 journals, as detailed in Table 4. The journal with the most publications was Frontiers in Microbiology (39 articles, accounting for 4.9%), followed by Frontiers in Immunology (35 articles, 4.4%), Frontiers in Cellular and Infection Microbiology (23 articles, 2.9%), Plos One (21 articles, 2.6%), Nutrients (17 articles, 2.1%), Biomedicine & Pharmacotherapy (16 articles, 2.0%), Scientific Reports (15 articles, 1.9%), International Journal of Molecular Sciences (15 articles, 1.9%), Gut Microbes (13 articles, 1.6%), and Frontiers in Pharmacology (12 articles, 1.5%). Nature Medicine had the highest total citations (2004) but only published 3 articles.
Supplementary Figure 1 presents a bubble chart of the top 20 journals related to lung and intestinal microbiota research. The chart reveals that since 2021, Frontiers in Microbiology, Frontiers in Immunology, and Frontiers in Cellular and Infection Microbiology have been the most prolific journals in this research area. Plos One has maintained a steady publication volume since 2013, while Scientific Reports shows a general downward trend in publication volume. Specifically, Frontiers in Microbiology published 12 articles in this field in 2023.
The research on lung diseases and intestinal microbiota encompasses 64 fields, with Table 5 listing the top 20 fields in publication volume. The fields of “Microbiology” and “Immunology” lead in publication volume, with 166 and 144 papers, respectively, and rank highest in total citations, with 6483 and 5025 citations each. The field with the highest average citation number is “Virology” (109.5 citations per paper), which, despite having a smaller publication volume of only 10 papers, has garnered relatively high attention and citations.
Supplementary Figure 2 presents a bubble chart sorted by publication volume for the top 20 research fields. According to the chart, “Microbiology” and “Immunology” have been the most popular research fields since 2011. Since 2018, there has been a rising trend in the publication volume in fields such as “Pharmacology & Pharmacy,” “Oncology,” “Biochemistry & Molecular Biology,” “Respiratory System,” “Medicine, Research & Experimental,” “Multidisciplinary Sciences,” and “Cell Biology.” This trend indicates increasing attention in these areas.
An analysis was conducted on 1,046 author keywords, with “intestinal microbiota/microbiome,” “microbiota/microbiome,” and “gut-lung axis” being the keywords that appeared over 100 times (Table 6). As shown in the bubble chart of Supplementary Figure 3, since the outbreak of COVID-19/SARS-CoV-2 in 2020, it has become a focal point of interest.
Using CiteSpace software, the keywords were categorized into 11 clusters (Figure 7). Closely related keywords were automatically grouped into a cluster, with each cluster named after the keyword with the highest log-likelihood ratio (LLR). The higher the LLR, the more representative the keyword is of its cluster. The 11 clusters identified in this field are #0 asthma, #1 probiotics, #2 non-small cell lung cancer, #3 inflammation, #4 metagenomics, #5 COPD, #6 immunity, #7 gut, #8 pulmonary hypertension, #9 gut-lung axis, and #10 sepsis, with cluster #0 being the largest.
A timeline chart was created based on the first year of the appearance of the keywords (Figure 8). In this field, the earliest active cluster was #9, followed by the rise of #1. Our study identified 5 keywords with the most potent citation bursts (Figure 9), including asthma, lung, airway hyperresponsiveness, allergy, and non-small cell lung cancer.
Figure 8 The timeline of clustered network map of author keywords in intestinal microbiota and lung diseases.
Figure 9 All author keywords with the strongest citation bursts in intestinal microbiota and lung diseases.
Figure 10 displays a network visualization map of cited references, with yellow nodes representing the most recently appearing keywords, indicating these are the latest cited references in the field. Figure 11 shows 12 related clusters identified by CiteSpace: COVID-19/SARS-CoV-2, COPD, chronic pulmonary disease, immunotherapy, asthma, sepsis, tuberculosis, lung cancer, cystic fibrosis, anti-tuberculosis therapy, pulmonary hypertension, and Th1 and M1 polarization.
Figure 11 The clustered network map of co-cited references in intestinal microbiota and lung diseases.
A burst strength analysis of the references was conducted using CiteSpace to gain a comprehensive understanding of the cited references. The time frame was set from 2011 to 2023, with a one-year interval, and 40 references with significant burst strength were identified. Among these, 8 references had a burst strength more significant than 10 (Figure 12). This analysis highlights the most influential and rapidly emerging topics in the field within the specified time frame.
Figure 12 Top 40 references with the strongest citation bursts in intestinal microbiota and lung diseases.
In this study, we analyzed 794 articles from the WoSCC database in the field of lung diseases and intestinal microbiota using Python, VOSviewer, and CiteSpace. The research findings indicate a significant increase in annual publications and citation numbers starting from 2020, with a predominant contribution from China. This highlights the extensive research contributions of China in this field. Among the top 10 institutions in terms of publications, 9 are from China, including Zhejiang University, Fudan University, Shanghai Jiao Tong University, Sun Yat-sen University, Capital Medical University, Beijing University of Traditional Chinese Medicine, Chinese Academy of Sciences, Guangzhou Medical University, and Southeast University. This indicates increasing attention and emphasis on this research area in China.
In terms of publication volume, the most prolific author is Jian Wang from China. However, in terms of total citations, Benjamin J Marsland from Monash University, Australia, holds the leading position but is outside the top 10 authors. Following him are Eva S Gollwitzer from Universitaire Vaudois, Switzerland, and Aurelien Trompette. In terms of the H-index, the leading positions are occupied by Valentin Sencio and Francois Trottein. When assessing prolific authors, it is crucial to consider the quantity of their publications and the quality of the articles.
From the perspective of journals, Frontiers in Microbiology holds a leading position as the journal with the highest number of publications in this field. However, Nature Medicine has the highest total citations despite publishing only three articles. This discrepancy highlights the significant impact and high quality of the articles in Nature Medicine.
The most focused research fields are “Microbiology” and “Immunology”, which lead in both the number of publications and total citations. Despite having fewer publications, the field of “Virology” has the highest average citation count. This heightened attention and citation rate could be related to the outbreak of COVID-19/SARS-CoV-2 in 2020, which has also become a focal point of interest.
Benjamin J Marsland from Monash University is a prominent author in terms of citations in this field. His long-term research into the impact of the microbiome on human immunity and the respiratory system has been influential. His team discovered a new protective pathway within the gut-lung axis - L-tyrosine–PCS, which can be used to treat or prevent inflammatory diseases (Wypych et al., 2021). This discovery underscores the critical interplay between intestinal microbiota and lung health, revealing novel pathways for therapeutic interventions.
The lung and intestinal microbiota fields have consistently attracted attention from scholars worldwide. Current research hotspots, identified through analyses of the most-cited references, keyword co-occurrence, clustering, and emerging trends, primarily focus on the gut-lung axis, probiotics, COVID-19, asthma, and COPD. It is well-recognized that intestinal microbiota dysbiosis is associated with increased susceptibility to respiratory diseases and alterations in lung immune responses and homeostasis. Although an increasing number of epidemiological and experimental studies have highlighted the relationship between the intestinal microbiome and the lungs, referred to as the “gut-lung axis” (Bingula et al., 2017; Dang and Marsland, 2019), the mechanisms by which intestinal microbiota influence lung diseases remain unclear (Budden et al., 2017). Understanding these mechanisms is crucial for developing effective treatments and prevention strategies for respiratory diseases influenced by intestinal microbiota.
The human gut harbors a rich and diverse microbial community, predominantly constituted by the intestinal microbiota. The dominant phyla within this community include Firmicutes (e.g., Clostridia, Lactobacillus) and Bacteroidetes (e.g., Bacteroides, Prevotella) (Adak and Khan, 2019). Research into the human microbiome has shown that the intestinal microbiota plays a crucial role in maintaining homeostasis in the gastrointestinal system and distant organs by modulating immune responses. It is critical to host health and intestinal immune homeostasis (Tan et al., 2014). However, intestinal microbiota dysbiosis can impact lung diseases, such as pneumonia, asthma, lung cancer, COPD (Chronic Obstructive Pulmonary Disease), and PH (Pulmonary Hypertension). This influence is likely related to alterations in immune responses, hormonal balance, and metabolic homeostasis (Bander et al., 2020; Ma et al., 2022).
Short-chain fatty acids (SCFAs) are among the most abundant microbial metabolites in the gut, playing fundamental roles such as lowering intestinal pH and promoting mucin synthesis. These functions prevent the adherence of harmful bacteria, enhance epithelial integrity, and strengthen the host’s immune system (Ashique et al., 2022). SCFAs interact with G-protein-coupled receptors (GPCRs) and regulate the differentiation of Treg, Th1, and Th17 cells. They also inhibit histone deacetylases (HDACs), thereby controlling cytokine storms and excessive immune responses, making them essential regulators in immune responses, inflammation, and the development of lung diseases (Jardou and Lawson, 2021; Smith et al., 2013; Castro-Mejía et al., 2018; Kleiner et al., 2015; Tang et al., 2021; Brown et al., 2017). However, not all studies report the anti-inflammatory actions of SCFAs. They have also been shown to increase the production of pro-inflammatory cytokines in cells stimulated by toll-like receptors (TLRs) (Rutting et al., 2019; Mirmonsef et al., 2012). Further research by Ghorbani et al. (2015) suggests that SCFAs can have anti-inflammatory and pro-inflammatory effects on lung cells. This dual role indicates that the therapeutic value of SCFAs in treating and managing diseases, particularly those involving the lung, is a promising area for further exploration. The complex nature of SCFA interactions in the human body, especially in the context of the gut-lung axis, highlights the importance of a nuanced understanding of these metabolites in health and disease.
Research suggests that gut microbiota influence the composition of airway microbiota through their metabolic products, such as short-chain fatty acids (SCFAs), and immune cells (Reyes et al., 2012; Chakradhar, 2017; Cait et al., 2018; Steed et al., 2017; Samuelson et al., 2015; Herbst et al., 2011; Grayson et al., 2018). Compared to gut microbiota, research on airway microbiota is still in its infancy, but interest in this area is gradually increasing (Dang and Marsland, 2019). Rapid advancements in high-throughput sequencing technologies have corrected the misconception that “healthy lungs are sterile,” making airway microbiomics a focus of current research. Multiple studies have confirmed that the lungs are not a sterile environment, although the microbial load in the airways is significantly lower than in the gut (Yagi et al., 2021; Rastogi et al., 2022). A mature airway microbiome is equally important for the development and regulation of adaptive and innate immune responses (Enaud et al., 2020; Pattaroni et al., 2018). Increasingly, research is showing a link between airway microbiota and respiratory diseases (such as ventilator-associated pneumonia, asthma, chronic obstructive pulmonary disease) as well as metabolic diseases (such as obesity, diabetes) (Shah and Bunyavanich, 2021; Huang and Shi, 2019; Tiew et al., 2021; Lee et al., 2019; Martin-Loeches et al., 2020; Trompette et al., 2014; Fenn et al., 2022). Comparing the airway microbiome composition of healthy individuals and patients with diseases can reveal specific microbial communities associated with the onset and progression of diseases. Reviews by Yagi et al. (2021) suggest that the airway microbiome could become a useful biomarker for respiratory diseases in clinical settings. Therefore, investigating the role of airway microbiota dysbiosis in various lung diseases is crucial.
Researchers have delved into the functions of airway microbiota within the body. They have found that certain microbes can positively impact respiratory health by producing specific metabolic products or participating in immune responses. Additionally, airway microbiota interact with host cells, influencing disease progression by regulating host gene expression (Yuan et al., 2022; Dang and Marsland, 2019; Ver Heul et al., 2019; Yan et al., 2022; Sequeira et al., 2020; Brown et al., 2017; Subramaniam et al., 2015). With the widespread use of broad-spectrum antibiotics, increasing numbers of antibiotic-resistant strains are emerging in the airway microbiota. Researchers are studying the transmission pathways of antibiotic resistance genes in airway microbiota and the mechanisms of resistant strain formation to better manage antibiotic use and develop new treatment strategies (Lin et al., 2023; Cai et al., 2022; Lin et al., 2021; Chmiel et al., 2014; Sherrard et al., 2014; Mac Aogáin et al., 2020). Recent research indicates that the airway microbiome could spread through airborne transmission, direct contact, or other routes among people, particularly since the COVID-19 outbreak (Doggett et al., 2020). This finding is significant for disease control, as intervening in the transmission pathways of the airway microbiome could help prevent the spread of diseases.
Keyword cluster analysis has revealed that probiotics are an emerging research hotspot in the study of the relationship between intestinal bacteria and lung diseases. Probiotics, beneficial microorganisms, selectively stimulate the growth and activity of a limited number of bacteria in the gut (da Silva et al., 2021). Primarily, this refers to species within the Lactobacillus and Bifidobacterium genera in the intestine. Probiotics play multiple roles within the human body: they aid in breaking down complex substances in food, promoting digestion and absorption. Additionally, they produce enzymes and substances that enhance the utilization of nutrients in food. Probiotics can competitively occupy ecological niches in the gut, inhibiting the growth of harmful bacteria and reducing pathogenic attacks and infections on the host. They also stimulate and regulate the function of the host’s immune system, enhancing the body’s immunity and helping to fend off diseases (Reid et al., 2017; Licciardi et al., 2012). Probiotics produce beneficial metabolic products, such as short-chain fatty acids, which regulate the intestinal pH balance and maintain gut health (Kim et al., 2019; Ragan et al., 2022; Yan and Polk, 2020). Modulating the composition of the gut microbiota, adjusting the metabolic products of gut microbes, and improving intestinal barrier function are potential regulatory mechanisms of probiotics (Li et al., 2021; Wang X. et al., 2021). Professor Matsuzaki demonstrated the efficacy of probiotics as early as 1985 (Matsuzaki et al., 1985). However, current research on the role of probiotics in lung diseases is relatively limited, and more studies are needed to confirm their effects in this context.
The gut-lung axis is an emerging field with many aspects yet to be fully understood. Future research aims to delve deeper into understanding the gut microbiota and its metabolic products, airway microbiota and their metabolites, and to develop suitable bioinformatics and machine learning models. These models are intended to predict and assess various gut-lung axis diseases and health statuses, leading to the development of personalized prevention and treatment strategies (Zheng et al., 2020; Zheng et al., 2023; Liang et al., 2022). Recent discoveries indicate significant differences in the gut microbiota of lung cancer patients compared to their lung microbiome communities. Changes in the gut microbiota can affect the prognosis and treatment outcomes of lung cancer (Goto, 2022; Tsay et al., 2021; Tsay et al., 2018), making the role of the gut-lung axis in the prevention, diagnosis, and treatment of lung cancer a valuable area of exploration. Dietary habits have been found to influence the gut microbiota, thereby impacting the prevention and treatment of lung diseases (Gasmi et al., 2021; Healy et al., 2021). Understanding the relationship between nutrition and the gut-lung axis, and designing specific dietary plans, may be one of the future research directions. COVID-19, one of the most widely discussed diseases in recent years, has been shown to be influenced by the gut microbiota, which may affect the host’s infection and immune response to the virus (Wang H. et al., 2021; McGinniss and Collman, 2021; Liu et al., 2022; Ancona et al., 2023). Therefore, studying the relationship between the gut-lung axis and COVID-19 is of significant importance for the prevention and treatment of this disease. This line of research holds the potential to unlock new insights into managing not only COVID-19 but also other respiratory diseases influenced by the complex interactions within the gut-lung axis.
Our study leveraged bibliometric methods to perform a visual analysis of research on the relationship between intestinal microbiota and lung diseases, aiding scholars in better understanding the hotspots and trends in this field. However, our study also has limitations. We analyzed only articles published on WoSCC within a specific timeframe and in English, potentially overlooking research results from other databases. Despite this, using visual methods to understand the current state, hotspots, and trends in a field remains valuable.
Using Python programming, VOSviewer, and CiteSpace software, we conducted a bibliometric study on articles related to the gut microbiota and lung diseases. The results indicate an upward trend in research volume in this field since 2011, signifying the burgeoning development of theories related to the lung and gut microbiota. China has the highest total publication volume in this area, while the quality of articles from the United States is comparatively higher. The correlation and potential mechanisms between the gut microbiota and lung diseases, including asthma, COPD, lung cancer, and respiratory infections, remain hot research topics. However, the understanding of the mechanisms involved in the gut-lung axis is still in its nascent stages and requires further elucidation. Future research needs to focus more on the gut microbiota and airway microbiota to enhance our understanding of the gut-lung axis. This deeper understanding is crucial for developing new and effective treatment strategies for lung diseases. As this field continues to evolve, it’s evident that interdisciplinary research encompassing microbiology, immunology, and respiratory medicine will be pivotal in unlocking new insights into the gut-lung axis and its implications for human health.
The original contributions presented in the study are included in the article/Supplementary Material. Further inquiries can be directed to the corresponding authors.
WS: Writing – original draft. TZ: Writing – review & editing. PD: Writing – review & editing. LG: Writing – review & editing. XZ: Writing – review & editing. KL: Writing – review & editing.
The author(s) declare financial support was received for the research, authorship, and/or publication of this article. This study was supported by the Science and Technology Department of Sichuan Province (No. 2022YFS0388, 23ZYZYTS0231).
The authors declare that the research was conducted in the absence of any commercial or financial relationships that could be construed as a potential conflict of interest.
All claims expressed in this article are solely those of the authors and do not necessarily represent those of their affiliated organizations, or those of the publisher, the editors and the reviewers. Any product that may be evaluated in this article, or claim that may be made by its manufacturer, is not guaranteed or endorsed by the publisher.
The Supplementary Material for this article can be found online at: https://www.frontiersin.org/articles/10.3389/fcimb.2024.1347110/full#supplementary-material
Supplementary Figure 1 | Bubble chart of the top 20 journals by year.
Supplementary Figure 2 | Bubble chart of the top 20 research areas by year.
Supplementary Figure 3 | Bubble chart of the top 30 author keywords by year.
Adak, A., Khan, M. R. (2019). An insight into gut microbiota and its functionalities. Cell Mol. Life Sci. 76, 473–493. doi: 10.1007/s00018-018-2943-4
Ancona, G., Alagna, L., Alteri, C., Palomba, E., Tonizzo, A., Pastena, A., et al. (2023). Gut and airway microbiota dysbiosis and their role in COVID-19 and long-COVID. Front. Immunol. 14. doi: 10.3389/fimmu.2023.1080043
Ashique, S., De Rubis, G., Sirohi, E., Mishra, N., Rihan, M., Garg, A., et al. (2022). Short Chain Fatty Acids: Fundamental mediators of the gut-lung axis and their involvement in pulmonary diseases. Chem. Biol. Interact. 368, 110231. doi: 10.1016/j.cbi.2022.110231
Bander, Z., Nitert, M. D., Mousa, A., Naderpoor, N. (2020). The gut microbiota and inflammation: an overview. Int. J. Environ. Res. Public Health 17, 7618. doi: 10.3390/ijerph17207618
Bingula, R., Filaire, M., Radosevic-Robin, N., Bey, M., Berthon, J. Y., Bernalier-Donadille, A., et al. (2017). Desired turbulence? Gut-lung axis, immunity, and lung cancer. J. Oncol. 2017, 5035371. doi: 10.1155/2017/5035371
Brown, R. L., Sequeira, R. P., Clarke, T. B. (2017). The microbiota protects against respiratory infection via GM-CSF signaling. Nat. Commun. 8, 1512. doi: 10.1038/s41467-017-01803-x
Budden, K. F., Gellatly, S. L., Wood, D. L., Cooper, M. A., Morrison, M., Hugenholtz, P., et al. (2017). Emerging pathogenic links between microbiota and the gut-lung axis. Nat. Rev. Microbiol. 15, 55–63. doi: 10.1038/nrmicro.2016.142
Cai, X., Luo, Y., Zhang, Y., Lin, Y., Wu, B., Cao, Z., et al. (2022). Airway microecology in rifampicin-resistant and rifampicin-sensitive pulmonary tuberculosis patients. BMC Microbiol. 22, 286. doi: 10.1186/s12866-022-02705-9
Cait, A., Hughes, M. R., Antignano, F., Cait, J., Dimitriu, P. A., Maas, K. R., et al. (2018). Microbiome-driven allergic lung inflammation is ameliorated by short-chain fatty acids. Mucosal Immunol. 11, 785–795. doi: 10.1038/mi.2017.75
Carding, S., Verbeke, K., Vipond, D. T., Corfe, B. M., Owen, L. J. (2015). Dysbiosis of the gut microbiota in disease. Microb. Ecol. Health Dis. 26, 26191. doi: 10.3402/mehd.v26.26191
Castro-Mejía, J. L., Deng, L., Vogensen, F. K., Reyes, A., Nielsen, D. S. (2018). Extraction and purification of viruses from fecal samples for metagenome and morphology analyses. Methods Mol. Biol. 1838, 49–57. doi: 10.1007/978-1-4939-8682-8_5
Chakradhar, S. (2017). A curious connection: Teasing apart the link between gut microbes and lung disease. Nat. Med. 23, 402–404. doi: 10.1038/nm0417-402
Chmiel, J. F., Aksamit, T. R., Chotirmall, S. H., Dasenbrook, E. C., Elborn, J. S., LiPuma, J. J., et al. (2014). Antibiotic management of lung infections in cystic fibrosis. I. The microbiome, methicillin-resistant Staphylococcus aureus, gram-negative bacteria, and multiple infections. Ann. Am. Thorac. Soc. 11, 1120–1129. doi: 10.1513/AnnalsATS.201402-050AS
Clemente, J. C., Ursell, L. K., Parfrey, L. W., Knight, R. (2012). The impact of the gut microbiota on human health: an integrative view. Cell 148, 1258–1270. doi: 10.1016/j.cell.2012.01.035
Dang, A. T., Marsland, B. J. (2019). Microbes, metabolites, and the gut-lung axis. Mucosal Immunol. 2, 843–850. doi: 10.1038/s41385-019-0160-6
da Silva, T. F., Casarotti, S. N., de Oliveira, G. L. V., Penna, A. L. B. (2021). The impact of probiotics, prebiotics, and synbiotics on the biochemical, clinical, and immunological markers, as well as on the gut microbiota of obese hosts. Crit. Rev. Food Sci. Nutr. 61, 337–355. doi: 10.1080/10408398.2020.1733483
de Steenhuijsen Piters, W. A., Sanders, E. A., Bogaert, D. (2015). The role of the local microbial ecosystem in respiratory health and disease. Philos. Trans. R Soc. Lond B Biol. Sci. 370, 20140294. doi: 10.1098/rstb.2014.0294
Doggett, N., Chow, C. W., Mubareka, S. (2020). Characterization of experimental and clinical bioaerosol generation during potential aerosol-generating procedures. Chest 158, 2467–2473. doi: 10.1016/j.chest.2020.07.026
Enaud, R., Prevel, R., Ciarlo, E., Beaufils, F., Wieërs, G., Guery, B., et al. (2020). The gut-lung axis in health and respiratory diseases: A place for inter-organ and inter-kingdom crosstalks. Front. Cell Infect. Microbiol. 10. doi: 10.3389/fcimb.2020.00009
Feng, Q., Chen, W. D., Wang, Y. D. (2018). Gut microbiota: an integral moderator in health and disease. Front. Microbiol. 9. doi: 10.3389/fmicb.2018.00151
Fenn, D., Abdel-Aziz, M. I., van Oort, P. M. P., Brinkman, P., Ahmed, W. M., Felton, T., et al. (2022). Composition and diversity analysis of the lung microbiome in patients with suspected ventilator-associated pneumonia. Crit. Care 26, 203. doi: 10.1186/s13054-022-04068-z
Forsythe, P., Kunze, W., Bienenstock, J. (2016). Moody microbes or fecal phrenology: what do we know about the microbiota-gut-brain axis? BMC Med. 14, 58. doi: 10.1186/s12916-016-0604-8
Gasmi, A., Tippairote, T., Mujawdiya, P. K., Peana, M., Menzel, A., Dadar, M., et al. (2021). The microbiota-mediated dietary and nutritional interventions for COVID-19. Clin. Immunol. 226, 108725. doi: 10.1016/j.clim.2021.108725
Ghorbani, P., Santhakumar, P., Hu, Q., Djiadeu, P., Wolever, T. M., Palaniyar, N., et al. (2015). S hort-chain fatty acids affect cystic fibrosis airway inflammation and bacterial growth. Eur. Respir. J. 46, 1033–1045. doi: 10.1183/09031936.00143614
Goto, T. (2022). Microbiota and lung cancer. Semin. Cancer Biol. 86, 1–10. doi: 10.1016/j.semcancer.2022.07.006
Grayson, M. H., Camarda, L. E., Hussain, S. A., Zemple, S. J., Hayward, M., Lam, V., et al. (2018). Intestinal microbiota disruption reduces regulatory T cells and increases respiratory viral infection mortality through increased IFNγ Production. Front. Immunol. 9. doi: 10.3389/fimmu.2018.01587
Grice, E. A., Segre, J. A. (2012). The human microbiome: our second genome. Annu. Rev. Genomics Hum. Genet. 13, 151–170. doi: 10.1146/annurev-genom-090711-163814
Guler, A. T., Waaijer, C. J., Palmblad, M. (2016). Scientific workflows for bibliometrics. Scientometrics 107, 385–398. doi: 10.1007/s11192-016-1885-6
Healy, C., Munoz-Wolf, N., Strydom, J., Faherty, L., Williams, N. C., Kenny, S., et al. (2021). Nutritional immunity: the impact of metals on lung immune cells and the airway microbiome during chronic respiratory disease. Respir. Res. 22, 133. doi: 10.1186/s12931-021-01722-y
Herbst, T., Sichelstiel, A., Schär, C., Yadava, K., Bürki, K., Cahenzli, J., et al. (2011). Dysregulation of allergic airway inflammation in the absence of microbial colonization. Am. J. Respir. Crit. Care Med. 184, 198–205. doi: 10.1164/rccm.201010-1574OC
Huang, C., Shi, G. (2019). Smoking and microbiome in oral, airway, gut and some systemic diseases. J. Transl. Med. 17, 225. doi: 10.1186/s12967-019-1971-7
Jardou, M., Lawson, R. (2021). Supportive therapy during COVID-19: The proposed mechanism of short-chain fatty acids to prevent cytokine storm and multi-organ failure. Med. Hypotheses 154, 110661. doi: 10.1016/j.mehy.2021.110661
Kim, S. K., Guevarra, R. B., Kim, Y. T., Kwon, J., Kim, H., Cho, J. H., et al. (2019). Role of probiotics in human gut microbiome-associated diseases. J. Microbiol. Biotechnol. 29, 1335–1340. doi: 10.4014/jmb.1906.06064
Kleiner, M., Hooper, L. V., Duerkop, B. A. (2015). Evaluation of methods to purify virus-like particles for metagenomic sequencing of intestinal viromes. BMC Genomics 16, 7. doi: 10.1186/s12864-014-1207-4
Lee, J. T., Kim, C. M., Ramakrishnan, V. (2019). Microbiome and disease in the upper airway. Curr. Opin. Allergy Clin. Immunol. 19, 1–6. doi: 10.1097/ACI.0000000000000495
Ley, R. E., Peterson, D. A., Gordon, J. I. (2006). Ecological and evolutionary forces shaping microbial diversity in the human intestine. Cell 124, 837–848. doi: 10.1016/j.cell.2006.02.017
Li, H. Y., Zhou, D. D., Gan, R. Y., Huang, S. Y., Zhao, C. N., Shang, A., et al. (2021). Effects and mechanisms of probiotics, prebiotics, synbiotics, and postbiotics on metabolic diseases targeting gut microbiota: A narrative review. Nutrients 13, 3211. doi: 10.3390/nu13093211
Liang, M., Liu, J., Chen, W., He, Y., Kahaer, M., Li, R., et al. (2022). Diagnostic model for predicting hyperuricemia based on alterations of the gut microbiome in individuals with different serum uric acid levels. Front. Endocrinol. (Lausanne) 13. doi: 10.3389/fendo.2022.925119
Licciardi, P. V., Toh, Z. Q., Dunne, E., Wong, S. S., Mulholland, E. K., Tang, M., et al. (2012). Protecting against pneumococcal disease: critical interactions between probiotics and the airway microbiome. PloS Pathog. 8, e1002652. doi: 10.1371/journal.ppat.1002652
Lin, D., Wang, X., Li, Y., Wang, W., Li, Y., Yu, X., et al. (2021). Sputum microbiota as a potential diagnostic marker for multidrug-resistant tuberculosis. Int. J. Med. Sci. 18, 1935–1945. doi: 10.7150/ijms.53492
Lin, L., Yi, X., Liu, H., Meng, R., Li, S., Liu, X., et al. (2023). The airway microbiome mediates the interaction between environmental exposure and respiratory health in humans. Nat. Med. 29, 1750–1759. doi: 10.1038/s41591-023-02424-2
Liu, T. F. D., Philippou, E., Kolokotroni, O., Siakallis, G., Rahima, K., Constantinou, C. (2022). Gut and airway microbiota and their role in COVID-19 infection and pathogenesis: a scoping review. Infection 50, 815–847. doi: 10.1007/s15010-021-01715-5
Ma, C., Su, H., Li, H. (2021). Global research trends on prostate diseases and erectile dysfunction: A bibliometric and visualized study. Front. Oncol. 10. doi: 10.3389/fonc.2020.627891
Ma, P. J., Wang, M. M., Wang, Y. (2022). Gut microbiota: A new insight into lung diseases. BioMed. Pharmacother. 155, 113810. doi: 10.1016/j.biopha.2022.113810
Mac Aogáin, M., Lau, K. J. X., Cai, Z., Kumar Narayana, J., Purbojati, R. W., Drautz-Moses, D. I., et al. (2020). Metagenomics reveals a core macrolide resistome related to microbiota in chronic respiratory disease. Am. J. Respir. Crit. Care Med. 202, 433–447. doi: 10.1164/rccm.201911-2202OC
Martin-Loeches, I., Dickson, R., Torres, A., Hanberger, H., Lipman, J., Antonelli, M. d., et al. (2020). The importance of airway and lung microbiome in the critically ill. Crit. Care 24, 537. doi: 10.1186/s13054-020-03219-4
Matsuzaki, T., Yokokura, T., Azuma, I. (1985). Anti-tumour activity of Lactobacillus casei on Lewis lung carcinoma and line-10 hepatoma in syngeneic mice and Guinea pigs. Cancer Immunol. Immunother. 20, 18–22. doi: 10.1007/BF00199768
McGinniss, J. E., Collman, R. G. (2021). The upper airway microbiome and lung injury in COVID-19. Am. J. Respir. Crit. Care Med. 204, 1353–1355. doi: 10.1164/rccm.202109-2226ED
Mirmonsef, P., Zariffard, M. R., Gilbert, D., Makinde, H., Landay, A. L., Spear, G. T. (2012). Short-chain fatty acids induce pro-inflammatory cytokine production alone and in combination with toll-like receptor ligands. Am. J. Reprod. Immunol. 67, 391–400. doi: 10.1111/j.1600-0897.2011.01089.x
O’Hara, A. M., Shanahan, F. (2006). The gut flora as a forgotten organ. EMBO Rep. 7, 688–693. doi: 10.1038/sj.embor.7400731
Pan, X., Yan, E., Cui, M., Hua, W. (2018). Examining the usage, citation, and diffusion patterns of bibliometric mapping software: A comparative study of three tools. J. Informetrics 12, 481–493. doi: 10.1016/j.joi.2018.03.005
Pattaroni, C., Watzenboeck, M. L., Schneidegger, S., Kieser, S., Wong, N. C., Bernasconi, E., et al. (2018). Early-life formation of the microbial and immunological environment of the human airways. Cell Host Microbe 24, 857–865.e4. doi: 10.1016/j.chom.2018.10.019
Ragan, M. V., Wala, S. J., Goodman, S. D., Bailey, M. T., Besner, G. E. (2022). Next-generation probiotic therapy to protect the intestines from injury. Front. Cell Infect. Microbiol. 12. doi: 10.3389/fcimb.2022.863949
Rastogi, S., Mohanty, S., Sharma, S., Tripathi, P. (2022). Possible role of gut microbes and host’s immune response in gut-lung homeostasis. Front. Immunol. 13. doi: 10.3389/fimmu.2022.954339
Reid, G., Abrahamsson, T., Bailey, M., Bindels, L. B., Bubnov, R., Ganguli, K., et al. (2017). How do probiotics and prebiotics function at distant sites? Benef Microbes 8, 521–533. doi: 10.3920/BM2016.0222
Reyes, A., Semenkovich, N. P., Whiteson, K., Rohwer, F., Gordon, J. I. (2012). Going viral: next-generation sequencing applied to phage populations in the human gut. Nat. Rev. Microbiol. 10, 607–617. doi: 10.1038/nrmicro2853
Rutting, S., Xenaki, D., Malouf, M., Horvat, J. C., Wood, L. G., Hansbro, P. M., et al. (2019). Short-chain fatty acids increase TNFα-induced inflammation in primary human lung mesenchymal cells through the activation of p38 MAPK. Am. J. Physiol. Lung Cell Mol. Physiol. 316, L157–L174. doi: 10.1152/ajplung.00306.2018
Samuelson, D. R., Welsh, D. A., Shellito, J. E. (2015). Regulation of lung immunity and host defense by the intestinal microbiota. Front. Microbiol. 6. doi: 10.3389/fmicb.2015.01085
Sequeira, R. P., McDonald, J. A. K., Marchesi, J. R., Clarke, T. B. (2020). Commensal Bacteroidetes protect against Klebsiella pneumoniae colonization and transmission through IL-36 signalling. Nat. Microbiol. 5, 304–313. doi: 10.1038/s41564-019-0640-1
Shah, R., Bunyavanich, S. (2021). The airway microbiome and pediatric asthma. Curr. Opin. Pediatr. 33, 639–647. doi: 10.1097/MOP.0000000000001054
Sherrard, L. J., Tunney, M. M., Elborn, J. S. (2014). Antimicrobial resistance in the respiratory microbiota of people with cystic fibrosis. Lancet 384, 703–713. doi: 10.1016/S0140-6736(14)61137-5
Smith, P. M., Howitt, M. R., Panikov, N., Michaud, M., Gallini, C. A., Bohlooly, Y. M., et al. (2013). The microbial metabolites, short-chain fatty acids, regulate colonic Treg cell homeostasis. Science 341, 569–573. doi: 10.1126/science.1241165
Steed, A. L., Christophi, G. P., Kaiko, G. E., Sun, L., Goodwin, V. M., Jain, U., et al. (2017). The microbial metabolite desaminotyrosine protects from influenza through type I interferon. Science 357, 498–502. doi: 10.1126/science.aam5336
Subramaniam, R., Hillberry, Z., Chen, H., Feng, Y., Fletcher, K., Neuenschwander, P., et al. (2015). Delivery of GM-CSF to protect against influenza pneumonia. PloS One 10, e0124593. doi: 10.1371/journal.pone.0124593
Sun, G., Dong, D., Dong, Z., Zhang, Q., Fang, H., Wang, C., et al. (2022). Drug repositioning: A bibliometric analysis. Front. Pharmacol. 13. doi: 10.3389/fphar.2022.974849
Synnestvedt, M. B., Chen, C., Holmes, J. H. (2005). CiteSpace II: visualization and knowledge discovery in bibliographic databases. AMIA Annu. Symp Proc. 2005, 724–728.
Tan, J., McKenzie, C., Potamitis, M., Thorburn, A. N., Mackay, C. R., Macia, L. (2014). The role of short-chain fatty acids in health and disease. Adv. Immunol. 121, 91–119. doi: 10.1016/B978-0-12-800100-4.00003-9
Tang, J., Xu, L., Zeng, Y., Gong, F. (2021). Effect of gut microbiota on LPS-induced acute lung injury by regulating the TLR4/NF-kB signaling pathway. Int. Immunopharmacol 91, 107272. doi: 10.1016/j.intimp.2020.107272
Tiew, P. Y., Jaggi, T. K., Chan, L. L. Y., Chotirmall, S. H. (2021). The airway microbiome in COPD, bronchiectasis and bronchiectasis-COPD overlap. Clin. Respir. J. 15, 123–133. doi: 10.1111/crj.13294
Trompette, A., Gollwitzer, E. S., Yadava, K., Sichelstiel, A. K., Sprenger, N., Ngom-Bru, C., et al. (2014). Gut microbiota metabolism of dietary fiber influences allergic airway disease and hematopoiesis. Nat. Med. 20, 159–166. doi: 10.1038/nm.3444
Tsay, J. J., Wu, B. G., Badri, M. H., Clemente, J. C., Shen, N., Meyn, P., et al. (2018). Airway microbiota is associated with upregulation of the PI3K pathway in lung cancer. Am. J. Respir. Crit. Care Med. 198, 1188–1198. doi: 10.1164/rccm.201710-2118OC
Tsay, J. J., Wu, B. G., Sulaiman, I., Gershner, K., Schluger, R., Li, Y., et al. (2021). Lower airway dysbiosis affects lung cancer progression. Cancer Discovery 11, 293–307. doi: 10.1158/2159-8290.CD-20-0263
van Eck, N. J., Waltman, L. (2010). Software survey: VOSviewer, a computer program for bibliometric mapping. Scientometrics 84, 523–538. doi: 10.1007/s11192-009-0146-3
Ver Heul, A., Planer, J., Kau, A. L. (2019). The human microbiota and asthma. Clin. Rev. Allergy Immunol. 57, 350–363. doi: 10.1007/s12016-018-8719-7
Wang, J., Li, F., Tian, Z. (2017). Role of microbiota on lung homeostasis and diseases. Sci. China Life Sci. 60, 1407–1415. doi: 10.1007/s11427-017-9151-1
Wang, J., Maniruzzaman, M. (2022). A global bibliometric and visualized analysis of bacteria-mediated cancer therapy. Drug Discovery Today 27, 103297. doi: 10.1016/j.drudis.2022.05.023
Wang, H., Wang, H., Sun, Y., Ren, Z., Zhu, W., Li, A., et al. (2021). Potential associations between microbiome and COVID-19. Front. Med. (Lausanne) 22. doi: 10.3389/fmed.2021.785496
Wang, X., Zhang, P., Zhang, X. (2021). Probiotics regulate gut microbiota: an effective method to improve immunity. Molecules 26, 6076. doi: 10.3390/molecules26196076
Wypych, T. P., Pattaroni, C., Perdijk, O., Yap, C., Trompette, A., Anderson, D., et al. (2021). Microbial metabolism of L-tyrosine protects against allergic airway inflammation. Nat. Immunol. 22, 279–286. doi: 10.1038/s41590-020-00856-3
Yagi, K., Huffnagle, G. B., Lukacs, N. W., Asai, N. (2021). The lung microbiome during health and disease. Int. J. Mol. Sci. 22, 10872. doi: 10.3390/ijms221910872
Yan, Z., Chen, B., Yang, Y., Yi, X., Wei, M., Ecklu-Mensah, G., et al. (2022). Multi-omics analyses of airway host-microbe interactions in chronic obstructive pulmonary disease identify potential therapeutic interventions. Nat. Microbiol. 7, 1361–1375. doi: 10.1038/s41564-022-01196-8
Yan, F., Polk, D. B. (2020). Probiotics and probiotic-derived functional factors-mechanistic insights into applications for intestinal homeostasis. Front. Immunol. 11. doi: 10.3389/fimmu.2020.01428
Yuan, Y., Wang, C., Wang, G., Guo, X., Jiang, S., Zuo, X., et al. (2022). Airway microbiome and serum metabolomics analysis identify differential candidate biomarkers in allergic rhinitis. Front. Immunol. 12. doi: 10.3389/fimmu.2021.771136
Zheng, C., Lu, F., Chen, B., Yang, J., Yu, H., Wang, D., et al. (2023). Gut microbiome as a biomarker for predicting early recurrence of HBV-related hepatocellular carcinoma. Cancer Sci. 114, 4717–4731. doi: 10.1111/cas.15983
Zheng, Y. Y., Wu, T. T., Liu, Z. Q., Li, A., Guo, Q. Q., Ma, Y. Y., et al. (2020). Gut microbiome-based diagnostic model to predict coronary artery disease. J. Agric. Food Chem. 68, 3548–3557. doi: 10.1021/acs.jafc.0c00225
Keywords: intestinal microbiota, lung, bibliometric analysis, VOSviewer, Citespace
Citation: Sun W, Zhou T, Ding P, Guo L, Zhou X and Long K (2024) Bibliometric analysis of intestinal microbiota and lung diseases. Front. Cell. Infect. Microbiol. 14:1347110. doi: 10.3389/fcimb.2024.1347110
Received: 30 November 2023; Accepted: 09 January 2024;
Published: 15 February 2024.
Edited by:
Valeriy Poroyko, Laboratory Corporation of America Holdings (LabCorp), United StatesReviewed by:
Weiming Sun, First Affiliated Hospital of Nanchang University, ChinaCopyright © 2024 Sun, Zhou, Ding, Guo, Zhou and Long. This is an open-access article distributed under the terms of the Creative Commons Attribution License (CC BY). The use, distribution or reproduction in other forums is permitted, provided the original author(s) and the copyright owner(s) are credited and that the original publication in this journal is cited, in accordance with accepted academic practice. No use, distribution or reproduction is permitted which does not comply with these terms.
*Correspondence: Xiujuan Zhou, emhvdXhpdWp1YW5AY2R1dGNtLmVkdS5jbg==; Kunlan Long, MTkxNDMwODY1QHFxLmNvbQ==
†These authors have contributed equally to this work
Disclaimer: All claims expressed in this article are solely those of the authors and do not necessarily represent those of their affiliated organizations, or those of the publisher, the editors and the reviewers. Any product that may be evaluated in this article or claim that may be made by its manufacturer is not guaranteed or endorsed by the publisher.
Research integrity at Frontiers
Learn more about the work of our research integrity team to safeguard the quality of each article we publish.