- 1Guangdong Provincial Key Laboratory of Medical Molecular Diagnostics, School of Medical Technology, The First Dongguan Affiliated Hospital, Guangdong Medical University, Dongguan, China
- 2Department of Laboratory Medicine, Dongguan Kanghua Hospital, Dongguan, China
- 3The Fourth Affiliated Hospital of Guangzhou Medical University, Guangzhou, China
- 4Department of Laboratory Medicine, Dongguan Songshan Lake Tungwah Hospital, Dongguan, China
Introduction: Bacterial resistance is a major threat to public health worldwide. To gain an understanding of the clinical infection distribution, drug resistance information, and genotype of CRE in Dongguan, China, as well as the resistance of relevant genotypes to CAZ-AVI, this research aims to improve drug resistance monitoring information in Dongguan and provide a reliable basis for the clinical control and treatment of CRE infection.
Methods: VITEK-2 Compact automatic analyzer was utilized to identify 516 strains of CRE collected from January 2017 to June 2023. To determine drug sensitivity, the K-B method, E-test, and MIC methods were used. From June 2022 to June 2023, 80 CRE strains were selected, and GeneXpert Carba-R was used to detect and identify the genotype of the carbapenemase present in the collected CRE strains. An in-depth analysis was conducted on the CAZ-AVI in vitro drug sensitivity activity of various genotypes of CRE, and the results were statistically evaluated using SPSS 23.0 and WHONET 5.6 software.
Results: This study identified 516 CRE strains, with the majority (70.16%) being K.pneumoniae, followed by E.coli (18.99%). Respiratory specimens had highest detection rate with 53.77% identified, whereas urine specimens had the second highest detection rate with 17.99%. From June 2022 to June 2023, 95% of the strains tested using the CRE GeneXpert Carba-R assay possessed carbapenemase genes, of which 32.5% were blaNDM strains and 61.25% blaKPC strains. The results showed that CRE strains containing blaKPC had a significantly higher rate of resistance to amikacin, cefepime, and aztreonam than those harboring blaNDM.
Conclusions: The CRE strains isolated from Dongguan region demonstrated a high resistance rate to various antibiotics used in clinical practice but a low resistance rate to tigecycline. These strains produce Class A serine carbapenemases and Class B metals β-lactamases, with the majority of them carrying blaNDM and blaKPC. Notably, CRE strains with blaKPC and blaNDM had significantly lower resistance rates to tigecycline. CAZ-AVI showed a good sensitivity rate with no resistance to CRE strains carrying blaKPC. Therefore, CAZ-AVI and tigecycline should be used as a guide for rational use of antibiotics in clinical practice to effectively treat CRE.
1 Introduction
Bacterial resistance is a major threat to public health worldwide. According to the latest data released by the 2021 National Bacterial Resistance Monitoring Network (CARSS) in 2023, 71.1% of clinical pathogens will be gram-negative bacteria (China Antimicrobial Resistance Surveillance System (CARSS), 2023b). Among them, intestinal bacteria are the most common drug-resistant bacteria in clinical practice, and carbapenem antibiotics include meropenem, imipenem, and ertapenem, it is one of the most effective antibiotics for treating infections caused by multidrug-resistant Enterobacteriaceae (MDR-E) (Djukovic et al., 2022; Esemu et al., 2022; Zheng et al., 2022; Lyu et al., 2023). With the widespread application of carbapenems in clinical treatment, the detection rate of carbapenem-resistant Enterobacteria (CR-Ent) has rapidly increased. The results revealed that the most common human-source CR-Ent species in China was E. xiangfangensis (66/92, 71.93%), and the proportion of carbapenemase-producing CP-Ent in CR-Ent was higher (72/92, 78.26%) than that in other global regions (Zhu et al., 2023). It mainly causes lower respiratory tract infections (65.4%), urinary tract infections (16.6%), abdominal infections (7.7%), and bacteremia (7.7%), with a total hospital mortality rate of 33.5% (Zhang et al., 2018). Currently, over 20% of Enterobacteriaceae have developed resistance, and it has been found that the production of carbapenemases is the main mechanism of resistance to carbapenems in Enterobacteriaceae bacteria (Tzouvelekis et al., 2012; Pang et al., 2018; Wang et al., 2018; Hu et al., 2019).
Ceftazidime-avibactam (CAZ-AVI) was awarded the Qualified New Antibiotic Qualification (QIDP), approved in the United States in 2015 and the European Union in 2016, and is now available in more than 40 countries and regions worldwide. Approved by the National Drug Administration (CFDA) on May 21, 2019, for the treatment of complex intraperitoneal infections (cIAI), hospital-acquired pneumonia (HAP)/ventilator-associated pneumonia (VAP), caused by Klebsiella pneumoniae (K. pneumoniae), Enterobacter cloacae, Escherichia coli (E. coli), Proteus mirabilis and Pseudomonas aeruginosa (P. aeruginosa), aged ≥18 years. CAZ-AVI can combat infections caused by most MDR-E strains, including carbapenem-resistant Enterobacteriaceae (CRE) (Zhen and Feng, 2021). CAZ-AVI) is composed of the third-generation cephalosporin ceftazidime with the novel non-β-lactamase β-lactamase inhibitor abvibatam (AVI), which inhibits class A, C, and some class D enzymes. The essential difference between AVI and classical β-lactamase lies in the fact that the serine of β-lactamase binds to the AVI amide bond to form a covalent conjugate to obtain the enzyme inhibitor complex. This enzyme-inhibiting form does not hydrolyze AVI, and AVI can recover its activity after cycling to form a lactam ring (Sader et al., 2015; Wong and van Duin, 2017). During this process, the structure of AVI is restored through a reversible reaction, resulting in a long-term inhibitory effect on enzymes. Moreover, AVI does not induce β-lactamase production (Ehmann et al., 2013; Livermore et al., 2015), and it was also found that 1-5 AVl molecules can inhibit one β-lactamase molecules, while 55 to 214 molecules are required for trizobactam and clavulanic acid, therefore AVI has stronger antibacterial effects (Zhanel et al., 2013). In addition, clinical experimental results have shown that patients infected with CRE strains treated with CAZ-AVI after ineffective treatment with other antibiotics had a cure rate of 95% (Temkin et al., 2017). This indicates that CAZ-AVI has a strong antibacterial effect on CRE bacteria and is an effective drug for the clinical treatment of infected CRE strains. However, there is no universally effective method for rapid identification of CRE strains in clinical practice.
Therefore, this study aimed to use the GeneXpert Carba-R method to quickly and accurately identify the carbapenase genotypes of Enterobacteriaceae. The study also investigated the in vitro antibacterial activity of CAZ-AVI against different carbapenase genotypes of CRE strains through an antibiotic susceptibility test (AST), providing a new strategy and a theoretical basis for precise drug use in patients with clinical infection.
2 Experimental materials and methods
2.1 Experimental strains
From January 2017 to June 2023, 516 Dongguan were collected from Dongguan, and duplicate samples were eliminated. The quality control strain is Escherichia coli ATCC 25922. CRE strains were mainly derived from respiratory, urine, blood, ascites, pus, and other specimens.
2.2 Identification of bacterial strains and AST
Strain identification and AST were performed according to the procedures recommended in the National Clinical Testing Procedures of the VITEK-2 Compact Automated Bacterial Identification and Drug Sensitivity System Analyzer (VITEK-2 Compact automatic analyzer) (Merieux, France). AST results were strictly determined according to the standards of the Clinical Laboratory Standardization Institute (CLSI) of the United States. The disc diffusion test (K-B method) and culture medium were purchased from OXOID (UK). Tigecycline was tested for minimum inhibitory concentration (MIC) methods using an E-test strip (BioMerier, France), and the results were determined in accordance with the guidelines of the United States Food and Drug Administration (FDA).
2.3 Detection of carbapenemase gene
The GeneXpert Carba-R detection method was adopted, and the specific steps were as follows: First of all, the bacterial solution was adjusted to a turbidity of 0.5 MCG with normal saline, 10 μL bacterial solution was absorbed into the sample processing solution, and then oscillated with an oscillator for 10s. Finally, 1.7 mL of the mixed solution was added to the Carba-R reagent kit, and the Cepheid® GeneXpert® Infinity System Fully Automated Medical PCR Analysis System (Infinity-80) (Cepheid (Shanghai) Trading Co., LTD.) was used for detection.
2.4 Statistical processing
WHONET 5.6 software was used for the statistical analysis of strain distribution and AST. SPSS software (version 23.0) and Fisher’s exact probability test were used to statistically analyze the differences in drug resistance rates of CRE strains, and P<0.05 indicated that the differences were statistically significant.
3 Results
3.1 Specimen sources of CRE strains
CRE strains were mainly isolated from respiratory (278 strains, 53.77%), urine (93 strains, 17.99%), blood (67 strains, 12.96%), ascites (15 strains, 2.90%), pus (13 strains, 2.51%), and other specimens (24 strains, 4.63%) (Figure 1A).
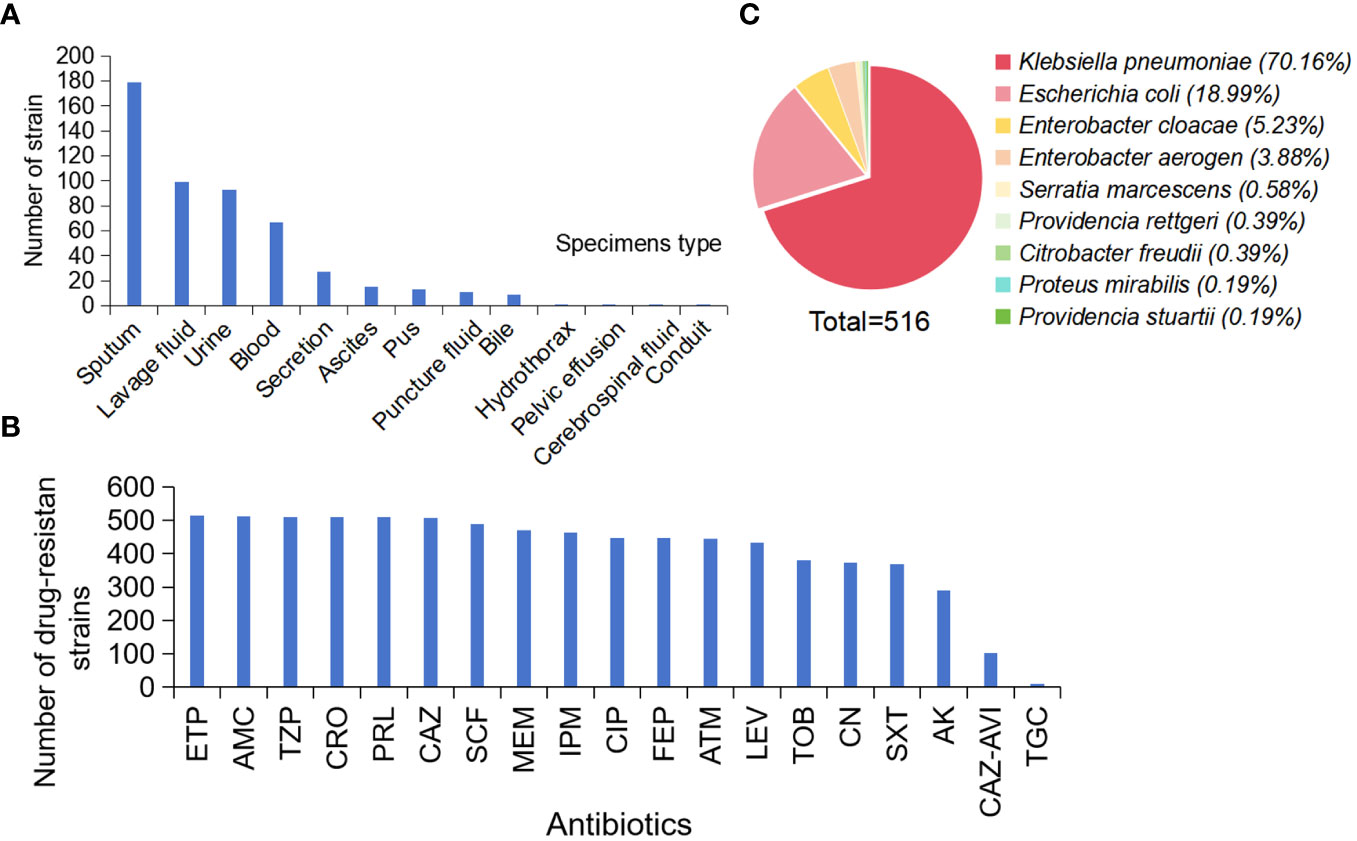
Figure 1 Distribution and AST Results of CRE Strains. (A) Sample distribution, (B) AST Results, (C) Distribution of 516 strains. (Ertapenem, ETP; Piperacillin-tazobactam, TZP; Ceftriaxone, CRO; Piperacillin, PRL; Ceftazidime, CAZ; Cefoperazone-sulbactam, SCF; Meropenem, MEM; Imipenem, IPM; Ciprofloxacin, CIP; Cefepime, FEP; Aztreonam, ATM; Levofloxacin, LEV; Tobramycin, TOB; Gentamicin, CN; Sulfamethoxazole-trimethoprim, SXT; Amikacin, AK; Tigecycline, TGC).
In addition, 49 strains of blaKPC were mainly distributed in the purum (28 strains, 57.1%), lavage fluid (seven strains, 14.3%), urine (five strains, 10.2%), blood (five strains, 10.2%), functional fluid (two strains, 4.1%), wound secrets (one strain, 2%), and bile (one strain, 2%). 27 strains of blaNDM were mainly distributed in the purum (nine strains, 33.3%), wound secrets (seven strains, 25.9%), urine (four strains, 14.8%), blood (three strains, 11.1%), bile (one strain, 3.7%), ascites (one strain, 3.7%), pelvic fusion (one strain, 3.7%), and drainage fluid (one strain, 3.7%) (Table 1).
3.2 Resistance of CRE strains to commonly used antibiotics
The AST assay of the 516 CRE strains showed that the drug resistance rate to ertapenem was the highest (99.6%). The drug resistance rate to piperacillin-tazobactam, ceftriaxone, and piperacillin was 98.8%. In addition, the drug resistance rates for ceftazidime, cefoperazone-sulbactam, meropenem, and imipenem were > 90% (98.3%, 95%, 91.3%, and 90.1%, respectively). Tigecycline resistance was the lowest (2.1%) (Figure 1B).
3.3 Sample distribution of CRE
Analysis of 516 CRE strains collected showed that 362 strains of K. pneumoniae (Vading et al., 2011; Shen et al., 2022) (70.16%), 98 strains of E. coli (18.99%), 27 strains of Enterobacter cloacae (5.23%), 20 strains of Enterobacter aerogenes and 3 other strains (3.88%), 3 strains of Serratia marcescens (0.58%). There were two strains of Providencia stuartii (0.39%), two strains of Proteus mirabilis (0.39%), one strain of Provencia steinii (0.19%), and one strain of Proteus mirabilis (0.19%) (Figure 1C).
3.4 Carbapenemase gene was detected by GeneXpert Carba-R assay
The GeneXpert Carba-R assay was used to detect 80 strains collected between June 2022 and June 2023. It was found that 76 strains (95%) carried carbapenase gene, among which 27 strains of blaNDM (32.5%) and 49 strains of blaKPC (61.25%) carried carbapenase gene. blaVIM, blaIPM and blaOXA genes were not detected, and no strains containing both blaNDM and blaKPC were detected (Table 2).
3.5 AST results of CRE strains with different carbapenemase genotypes
Because of the different clinical treatment regimens used for patients infected with different carbapenem enzyme types, we tested the AST of CRE strains carrying blaKPC and blaNDM. The results showed that the resistance rate of CRE strains to commonly used antibiotics was generally high, with CRE strains carrying the blaKPC genotype having a lower resistance rate to tigecycline (13.9%), The resistance rate of CRE strains carrying the blaNDM genotype to amikacin (22.22%) and tigecycline (7.14%) is relatively low. According to Fisher’s exact probability test, the resistance rate of CRE strains carrying the blaKPC genotype to cefepime, amikacin, and aztreonam was significantly higher than that of strains carrying the blaNDM genotype (P<0.05), whereas there was no significant difference between them in resistance rates to other antibiotics (P>0.05). In addition, we found that none of the CRE strains carrying the blaKPC genotype showed resistance to ceftazidime-avibactam, while the blaNDM genotype showed 100% resistance to ceftazidime-avibactam (Table 3).
4 Discussion
According to the Ambler molecular classification method, carbapenemases can be classified into categories A, B, and D. Among the 4 classes of β-lactamases defined by the Ambler classification system, the carbapenemas that confer carbapenem resistance in Enterobacteriaceae belong to 3 of them: Class A (K. pneumoniae carbapenemas, KPC), Class B (metallo-β-lactamases, MBL including New Delhi metallo-β-lactamases, NDM) and Class D (OXA-48 like carbapenemases) (van Duin and Doi, 2017). Class A is serine carbapenemase, mainly consisting of blaKPC (blaKPC-2-blaKPC-55)、blaSME (blaSME-1-blaSME-5)、blaIMI (blaIMI-1-blaIMI-18)、blaNMC and blaGES (blaGES-1-blaGES-43); Class B is metallo-β-lactamases, mainly blaNDM (blaNDM-1-blaNDM-29)、blaIMP (blaIMP-1-blaIMP-85)、blaVIM (blaVIM-1-blaVIM-69)、blaGIM (blaGIM-1-blaGIM-2), and blaSPM; Class D is OXA-48-like carbapenemases, mainly blaOXA-181 and blaOXA-232 (Yu et al., 2020, Yu et al., 2022). In addition to producing carbapenemases, the mechanism of carbapenem resistance in some strains is the production of ultra-broad spectrum β-lactam enzyme and/or AmpC enzyme combined with downregulation or deletion of outer membrane porin expression (Zhang et al., 2017; Fan et al., 2023).
The carbapenemases produced by CRE strains clinically isolated in China are mainly of the KPC and NDM types, with some strains producing OXA-48, IMP, and VIM carbapenemases (Zhang et al., 2017; Wang et al., 2018; Han et al., 2020). The main subtype of KPC-type carbapenemases is KPC-2, the main subtypes of NDM-type metalloenzymes are NDM-1 and NDM-5, and the main subtypes of OXA-48 type carbapenemases are OXA-181 and OXA-232 enzyme types (Yu et al., 2020, Yu et al., 2022). The CHINET surveillance of antimicrobial resistance among bacterial isolates from 2005 to 2022 showed that the resistance rate of K. pneumoniae strains isolated clinically in China to carbapenem antibiotics increased from 3% in 2005 to over 24.2% in 2022, an 8-fold increase (Hu et al., 2020; Zheng et al., 2020). According to data from the China Antimicrobial Resistance Surveillance System (CARSS) in 2018, the average resistance rate of K. pneumoniae clinically isolated from 1429 hospitals nationwide to carbapenems is 10.1% and exceeded 20% in some provinces and cities (China Antimicrobial Resistance Surveillance Network, 2020). The detection rate of carbapenem-resistant K. pneumoniae (CR-KPN) in 2021 is 11.3%, an increase of 0.4% compared to 10.9% in 2020 (China Antimicrobial Resistance Surveillance Network, 2021; China Antimicrobial Resistance Surveillance System (CARSS), 2023a). This indicates that the resistance rate of K. pneumoniae to carbapenems is gradually increasing and the situation is severe. However, there are certain differences in the carbapenem-producing enzyme types of different bacteria in different regions, which lead to different clinical treatment plans for different carbapenem-producing enzyme types. Therefore, there is an urgent need to develop new methods for the rapid and accurate identification of carbapenem-producing Enterobacteriaceae enzymes and test their drug sensitivity results to guide the clinical adoption of correct treatment plans, effectively treat patients’ infections, and save treatment time.
According to data from the International Network for Optimal Resistance Monitoring (INFORM) data, the resistance rate of meropenem-resistant E. coli to CAZ-AVI was 27% between 2015 and 2017 (Spiliopoulou et al., 2020). According to INFORM reports, the resistance rate of most Enterobacteriaceae bacteria to CAZ-AVI is low (<2.6%) (Wise et al., 2018), whereas the resistance rate of P. aeruginosa is relatively high, reaching 4%–8% (Nichols et al., 2016). Several studies conducted from 2006 to 2018 have shown that the resistance rates of most gram-negative bacteria to CAZ-AVI were below 3.7% in the United States (Senchyna et al., 2019). In Europe and the Asia-Pacific region, the resistance rates of Enterobacteriaceae to CAZ-AVI are less than 1.1% and 1.7%, respectively, and those of P. aeruginosa are less than 8.9% and 7.4%, respectively (Karlowsky et al., 2018; Kazmierczak et al., 2018). In Canada and Brazil, the rate of resistance to CAZ-AVI is generally below 5.3% (Denisuik et al., 2015; Rossi et al., 2017). Therefore, CAZ-AVI could be considered as an adequate treatment option for tract infections caused by KPC and OXA-48 producers (García-Castillo et al., 2018).
The results of this study showed that K. pneumoniae was the main CRE strain isolated from the Dongguan area, primarily from respiratory specimens. The AST assay showed that CRE strains isolated from Dongguan had the lowest tigacycline resistance. In addition, 76 strains (92.5%) carrying the carbapenemase gene were detected using the GeneXpert Carba-R method, among which 27 strains contained blaNDM gene (33.75%) and 24 strains contained blaKPC gene (61.25%). No blaVIM, blaIPM, or blaOXA genes were detected and no strains with both blaNDM and blaKPC were detected. It is speculated that CRE strains may be caused by factors such as high yield of AMPCase, ultra-broad spectrum β-lactamase, deletion of outer membrane protein, and overexpression of efflux pump (Li et al., 2012). Therefore, the results of this study indicate that CRE strains in Dongguan region were mainly serine-producing carbapenemase and B-producing metal β-lactamase.
Current studies have shown that the resistance mechanisms of CAZ-AVI are mainly the following: (1) expression of metallic β-lactamase (Lahiri et al., 2015; Aitken et al., 2016; Grupper et al., 2017); (2) promote the expression of blaKPC gene and the mutation of key sites of β-lactamase (Giddins et al., 2017; Gaibani et al., 2018); (3) porin deletion changes membrane permeability (Winkler et al., 2015; Humphries and Hemarajata, 2017; Nelson et al., 2017; Rocker et al., 2020; Guo et al., 2021); (4) promote the expression of efflux pumps (Zhang et al., 2017). One of the most common resistance mechanisms is the production of metallic β-lactamase. Class B metallic β-lactamases bind to β-lactamides substrates via zinc ions to hydrolyze all clinically used serine β-lactamase inhibitors, including avibactam (Schillaci et al., 2017), suggesting that CAZ-AVI cannot be used to treat patients infected with strains producing such enzymes. Mutations in the KPC-type carbapenemase gene are the main mechanism leading to CAZ-AVI resistance. Moreover, studies have shown that β-lactamase amino acid mutations or deletion, membrane permeability defects (i.e., changes in OmpK35, OmpK36, and OmpK37), and penicillin-binding protein mutations, and overexpression of KPC and ESBL determinants (SHV-, CTX-M-, or VEB types) are all associated with the resistance of KPC type carbapenemase strain to CAZ-AVI. In this study, we found significant differences in the resistance rates of CRE strains carrying blaKPC and blaNDM to cefepime, aztreonam, and amikacin, and no strains carrying blaKPC were found to be resistant to CAZ-AVI, suggesting a reason for the low frequency of CAZ-AVI antibiotic use in Dongguan. This study found that CRE strains carrying the blaNDM gene were all resistant to CAZ-AVI. In summary, the GeneXpert Carba-R method can rapidly detect the genotype of carbapenemase carried by CRE strains, save time for the treatment of patients with clinical emergency infections, and provide an experimental basis for the clinical use of CAZ-AVI for the treatment of infections. It is important to provide targeted and personalized treatment in clinical departments.
Finally, our study had some limitations. First, because of the large sample size of the carbapenem-resistant Enterobacteriaceae strains collected and the lack of research funding, we only used GeneXpert Carba-R to detect and identify the genotype of carbapenemase in CRE strains from 2022 to 2023. Therefore, we did not analyze all strains from 2017 to 2023. Second, this single-center study was conducted at a comprehensive tertiary hospital in Dongguan. The sample size of CRE strains was relatively small; therefore, our results cannot be extrapolated to other hospitals and regions in China. Additionally, this study lacks relevant research on the mechanisms of drug resistance is lacking. In future studies, we will conduct relevant analyses of CRE strain resistance mechanisms to gain a more comprehensive understanding of CRE strain resistance in the Dongguan region.
5 Conclusion
In summary, the CRE strains isolated from the Dongguan region demonstrated a high resistance rate to various antibiotics used in clinical practice but a low resistance rate to tigecycline. These strains produce Class A serine carbapenemases and Class B metals β-lactamases, with the majority of them carrying blaNDM and blaKPC. Notably, CRE strains with blaKPC and blaNDM had significantly lower resistance rates to tigecycline. CAZ-AVI showed a good sensitivity rate with no resistance to CRE strains carrying blaKPC. However, the CRE strains with blaNDM were not sensitive to CAZ-AVI. Therefore, CAZ-AVI and tigecycline should be used as a guide for the rational use of antibiotics in clinical practice in to effectively treat CRE. There is a need to conduct future analyses of CRE strain resistance mechanisms to gain a more comprehensive understanding of CRE strain resistance.
Data availability statement
The original contributions presented in the study are included in the article/supplementary material. Further inquiries can be directed to the corresponding authors.
Author contributions
XZ: Data curation, Formal analysis, Writing – original draft. CG: Data curation, Supervision, Visualization, Writing – original draft. SX: Investigation, Methodology, Writing – original draft. FL: Software, Writing – original draft. ZG: Project administration, Writing – original draft. SL: Data curation, Writing – original draft. CY: Investigation, Writing – original draft. ZD: Methodology, Software, Writing – original draft. SC: Conceptualization, Writing – original draft. YH: Writing – original draft. ZZ: Supervision, Writing – original draft. LL: Funding acquisition, Writing – original draft, Writing – review & editing.
Funding
The author(s) declare financial support was received for the research, authorship, and/or publication of this article. This project was supported by grant from PHD researchers of Guangdong Medical University in 2022 (GDMUB2022028/4SG23245G), 100 Youth Research Project Funding Program of Guangdong Medical University in 2023 (GDMUD2023001), Dongguan Science and Technology of Social Development Program (20211800900702, 20231800936272), Guangdong Basic and Applied Basic Research Foundation (2022A1515110158), Guangzhou Health Science and Technology Project (20241A010117) and the National Natural Science Foundation of China (32300048).
Conflict of interest
The authors declare that the research was conducted in the absence of any commercial or financial relationships that could be construed as a potential conflict of interest.
Publisher’s note
All claims expressed in this article are solely those of the authors and do not necessarily represent those of their affiliated organizations, or those of the publisher, the editors and the reviewers. Any product that may be evaluated in this article, or claim that may be made by its manufacturer, is not guaranteed or endorsed by the publisher.
References
Aitken, S. L., Tarrand, J. J., Deshpande, L. M., Tverdek, F. P., Jones, A. L., Shelburne, S. A., et al. (2016). High rates of nonsusceptibility to ceftazidime-avibactam and identification of New Delhi metallo-β-lactamase production in Enterobacteriaceae bloodstream infections at a major cancer center. Clin. Infect. Dis. 63, 954–958. doi: 10.1093/cid/ciw398
China Antimicrobial Resistance Surveillance Network (2020). 2018 national antibiotic resistance surveillance report. Chin. J. Rational Drug Use 17, 1–10. doi: 10.3969/j.issn.2096-3327.2020.1.001
China Antimicrobial Resistance Surveillance Network (2021). 2019 national antibiotic resistance surveillance report. Chin. J. Rational Drug Use 18, 1–11. doi: 10.3969/j.issn.2096-3327.2021.3.001
China Antimicrobial Resistance Surveillance System (CARSS) (2023a). Antimicrobial resistance surveillance reports from patients of out patient departments from China antimicrobial resistance surveillance systemin 2021. Chin. J. Nosocomiol. 33, 3403–3409. doi: 10.11816/cn.ni.2023-230911
China Antimicrobial Resistance Surveillance System (CARSS) (2023b) The report on China Antimicrobial Resistance Surveillance System (CARSS) in 2021. Available online at: http://www.carss.cn/ (Accessed 23 November 2023).
Denisuik, A. J., Karlowsky, J. A., Denisuik, T., Nichols, W. W., Keating., T. A., Adam, H. J., et al. (2015). In vitro activity of ceftazidime-avibactam against 338 molecularly characterized gentamicin-nonsusceptible gram-negative clinical isolates obtained from patients in Canadian hospitals. Antimicrob. Agents Chemother. 59, 3623–3626. doi: 10.1128/AAC.00364-15
Djukovic, A., Garzón, M. J., Canlet, C., Cabral, V., Lalaoui, R., García-Garcerá, M., et al. (2022). Lactobacillus supports Clostridiales to restrict gut colonization by multidrug-resistant Enterobacteriaceae. Nat. Commun. 13, 5617. doi: 10.1038/s41467-022-33313-w
Ehmann, D. E., Jahic, H., Ross, P. L., Gu, R. F., Hu, J., Durand-Réville, T. F., et al. (2013). Kinetics of avibactam inhibition against Class A, C, and D β-lactamases. J. Biol. Chem. 288, 27960–27971. doi: 10.1074/jbc.M113.485979
Esemu, S. N., Aka, T. K., Kfusi, A. J., Ndip, R. N., Ndip, L. M. (2022). Multidrug-resistant bacteria and Enterobacteriaceae count in abattoir wastes and its receiving waters in limbe municipality, Cameroon: public health implications. Biomed. Res. Int. 2022, 9977371. doi: 10.1155/2022/9977371
Fan, L., Pan, Z., Zhong, Y., Guo, J., Liao, X., Pang, R., et al. (2023). L-glutamine sensitizes Gram-positive-resistant bacteria to gentamicin killing. Microbiol. Spectr. 11, e0161923. doi: 10.1128/spectrum.01619-23
Gaibani, P., Campoli, C., Lewis, R. E., Volpe, S. L., Scaltriti, E., Giannella, M., et al. (2018). In vivo evolution of resistant subpopulations of KPC-producing Klebsiella pneumoniae during ceftazidime/avibactam treatment. J. Antimicrob. Chemother. 73, 1525–1529. doi: 10.1093/jac/dky082
García-Castillo, M., García-Fernández, S., Gómez-Gil, R., Pitart, C., Oviaño, M., Gracia-Ahufinger, I., et al. (2018). iCREST study group. Activity of ceftazidime-avibactam against carbapenemase-producing Enterobacteriaceae from urine specimens obtained during the infection-carbapenem resistance evaluation surveillance trial (iCREST) in Spain. Int. J. Antimicrob. Agents. 51, 511–515. doi: 10.1016/j.ijantimicag.2018.01.011
Giddins, M. J., Macesic, N., Annavajhala, M. K., Stump, S., Khan, S., McConville, T. H., et al. (2017). Successive emergence of ceftazidime-avibactam resistance through distinct genomic adaptations in blaKPC-2-harboring Klebsiella pneumoniae sequence type 307 isolates. Antimicrob. Agents Chemother. 62, e02101–e02117. doi: 10.1128/AAC.02101-17
Grupper, M., Sutherland, C., Nicolau, D. P. (2017). Multicenter evaluation of ceftazidime-avibactam and ceftolozane-tazobactam inhibitory activity against meropenem-nonsusceptible Pseudomonas aeruginosa from blood, respiratory tract, and wounds. Antimicrob. Agents Chemother. 61, e00875–e00817. doi: 10.1128/AAC.00875-17
Guo, Y., Liu, N., Lin, Z., Ba, X., Zhuo, C., Li, F., et al. (2021). Mutations in porin LamB contribute to ceftazidime-avibactam resistance in KPC-producing Klebsiella pneumoniae. Emerg. Microbes Infect. 10, 2042–2051. doi: 10.1080/22221751.2021.1984182
Han, R., Shi, Q., Wu, S., Yin, D., Peng, M., Dong, D., et al. (2020). China antimicrobial surveillance network (CHINET) study group. Dissemination of Carbapenemases (KPC, NDM, OXA-48, IMP, and VIM) among carbapenem-resistant Enterobacteriaceae isolated from adult and children patients in China. Front. Cell. Infect. Microbiol. 10. doi: 10.3389/fcimb.2020.00314
Hu, F., Guo, Y., Yang, Y., Zheng, Y., Wu, S., Jiang, X., et al. (2019). China antimicrobial surveillance network (CHINET) study group. Resistance reported from China antimicrobial surveillance network (CHINET) in 2018. Eur. J. Clin. Microbiol. Infect. Dis. 38, 2275–2281. doi: 10.1007/s10096-019-03673-1
Hu, F. P., Guo, Y., Zhu, D. M., Wang, F., Jiang, X. F., Xu, Y. C., et al. (2020). CHINET surveillance of bacterial resistance across tertiary hospitals in 2019. Chin. J. Infect. Chemother. 20 (03), 233–243. doi: 10.16718/j.1009-7708.2020.03.001
Humphries, R. M., Hemarajata, P. (2017). Resistance to ceftazidime-avibactam in Klebsiella pneumoniae due to porin mutations and the increased expression of KPC-3. Antimicrob. Agents Chemother. 61, e00537–e00517. doi: 10.1128/AAC.00537-17
Karlowsky, J. A., Kazmierczak, K. M., Bouchillon, S. K., de Jonge, B. L. M., Stone, G. G., Sahm, D. F. (2018). In Vitro Activity of ceftazidime-avibactam against clinical isolates of Enterobacteriaceae and Pseudomonas aeruginosa collected in Asia-Pacific countries: Results from the INFORM global surveillance program 2012 to 2015. Antimicrob. Agents Chemother. 62, e02569–e02517. doi: 10.1128/AAC.02569-17
Kazmierczak, K. M., de Jonge, B. L. M., Stone, G. G., Sahm, D. F. (2018). In vitro activity of ceftazidime/avibactam against isolates of Enterobacteriaceae collected in European countries: INFORM global surveillance 2012-15. J. Antimicrob. Chemother. 73, 2782–2788. doi: 10.1093/jac/dky266
Lahiri, S. D., Walkup, G. K., Whiteaker, J. D., Palmer, T., Mc Cormack, K., Tanudra, M. A., et al. (2015). Selection and molecular characterization of ceftazidime/avibactam-resistant mutants in Pseudomonas aeruginosa strains containing derepressed AmpC. J. Antimicrob. Chemother. 70, 1650–1658. doi: 10.1093/jac/dkv004
Li, B. B., Liu, Y. M., Li, F., Wang, S. S., Guo, P., Yang, C. X., et al. (2012). Drug resistance of gram-negative bacilli in hospital of 2000-2010. Chin. J. Nosocomiol. 22, 5379–5382. doi: 10.1126/science.282.5390.943
Livermore, D. M., Warner, M., Jamrozy, D., Mushtaq, S., Nichols, W. W., Mustafa, N., et al. (2015). In vitro selection of ceftazidime-avibactam resistance in Enterobacteriaceae with KPC-3 carbapenemase. Antimicrob. Agents Chemother. 59, 5324–5330. doi: 10.1128/AAC.00678-15
Lyu, J., Chen, H., Bao, J., Liu, S., Chen, Y., Cui, X., et al. (2023). Clinical distribution and Drug resistance of Pseudomonas aeruginosa in Guangzhou, China from 2017 to 2021. J. Clin. Med. 12, 1189. doi: 10.3390/jcm12031189
Nelson, K., Hemarajata, P., Sun, D., Rubio-Aparicio, D., Tsivkovski, R., Yang, S., et al. (2017). Resistance to ceftazidime-avibactam is due to transposition of KPC in a porin-deficient strain of Klebsiella pneumoniae with increased efflux activity. Antimicrob. Agents Chemother. 61, e00989–e00917. doi: 10.1128/AAC.00989-17
Nichols, W. W., de Jonge., B. L., Kazmierczak, K. M., Karlowsky, J. A., Sahm, D. F. (2016). In vitro susceptibility of global surveillance isolates of Pseudomonas aeruginosa to ceftazidime-avibactam (INFORM 2012 to 2014). Antimicrob. Agents Chemother. 60, 4743–4749. doi: 10.1128/AAC.00220-16
Pang, F., Jia, X. Q., Zhao, Q. G., Zhang, Y. (2018). Factors associated to prevalence and treatment of carbapenem-resistant Enterobacteriaceae infections: a seven years retrospective study in three tertiary care hospitals. Ann. Clin. Microbiol. Antimicrob. 17, 13. doi: 10.1186/s12941-018-0267-8
Rocker, A., Lacey, J. A., Belousoff, M. J., Wilksch, J. J., Strugnell, R. A., Davies, M. R., et al. (2020). Global trends in proteome remodeling of the outer membrane modulate antimicrobial permeability in Klebsiella pneumoniae. mBio 11, e00603–e00620. doi: 10.1128/mBio.00603-20
Rossi, F., Cury, A. P., Franco, M. R. G., Testa., R., Nichols., W. W. (2017). The in vitro activity of ceftazidime-avibactam against 417 gram-negative bacilli collected in 2014 and 2015 at a teaching hospital in São Paulo, Brazil. Braz. J. Infect. Dis. 21, 569–573. doi: 10.1016/j.bjid.2017.03.008
Sader, H. S., Castanheira, M., Flamm, R. K., Mendes, R. E., Farrell, D. J., Jones, R. N. (2015). Ceftazidime/avibactam tested against gram-negative bacteria from intensive care unit (ICU) and non-ICU patients, including those with ventilator-associated pneumonia. Int. J. Antimicrob. Agents. 46, 53–59. doi: 10.1016/j.ijantimicag.2015.02.022
Schillaci, D., Spano, V., Parrino, B. (2017). Pharmaceutical approaches to target antibiotic resistance mechanisms. J. Med. Chem. 60, 8268–8297. doi: 10.1021/acs.jmedchem.7b00215
Senchyna, F., Gaur, R. L., Sandlund, J., Truong, C., Tremintin, G., Kültz, D. (2019). Diversity of resistance mechanisms in carbapenem-resistant Enterobacteriaceae at a health care system in Northern California, from 2013 to 2016. Diagn. Microbiol. Infect. Dis. 93, 250–257. doi: 10.1016/j.diagmicrobio.2018.10.004
Shen, S., Tang, C., Ding, L., Han, R., Yin, D., Yang, W., et al. (2022). Identification of KPC-112 from an ST15 Klebsiella pneumoniae strain conferring resistance to ceftazidime-avibactam. mSphere 7, e0048722. doi: 10.1128/msphere.00487-22
Spiliopoulou, I., Kazmierczak, K., Stone, G. G. (2020). In vitro activity of ceftazidime/avibactam against isolates of carbapenem-non-susceptible Enterobacteriaceae collected during the INFORM global surveillance programme, (2015-2017). J. Antimicrob. Chemother. 75, 384–391. doi: 10.1093/jac/dkz456
Temkin, E., Torre-Cisneros, J., Beovic, B., Benito, N., Giannella, M., Gilarranz, R., et al. (2017). Ceftazidime-avibactam as salvage therapy for infections caused by carbapenem-resistant organisms. Antimicrob. Agents Chemother. 61, e01964–e01916. doi: 10.1128/AAC.01964-16
Tzouvelekis, L. S., Markogiannakis, A., Psichogiou, M., Tassios, P. T., Daikos, G. L. (2012). Carbapenemases in Klebsiella pneumoniae and other Enterobacteriaceae: an evolving crisis of global dimensions. Clin. Microbiol. Rev. 25(4), 682–707. doi: 10.1128/CMR.05035-11
Vading, M., Samuelsen, Ø., Haldorsen, B., Sundsfjord, A. S., Giske, C. G. (2011). Comparison of disk diffusion, E-test and VITEK2 for detection of carbapenemase-producing Klebsiella pneumoniae with the EUCAST and CLSI breakpoint systems. Clin. Microbiol. Infect. 17, 668–674. doi: 10.1111/j.1469-0691.2010.03299.x
van Duin, D., Doi, Y. (2017). The global epidemiology of carbapenemase-producing Enterobacteriaceae. Virulence 8, 460–469. doi: 10.1080/21505594.2016.1222343
Wang, Q., Wang, X., Wang, J., Ouyang, P., Jin, C., Wang, R., et al. (2018). Phenotypic and genotypic characterization of carbapenem-resistant Enterobacteriaceae: data from a longitudinal Large-scale CRE study in China, (2012-2016). Clin. Infect. Dis. 67, S196–S205. doi: 10.1093/cid/ciy660
Winkler, M. L., Papp-Wallace, K. M., Hujer, A. M., Domitrovic, T. N., Hujer, K. M., Hurless, K. N. (2015). Unexpected challenges in treating multidrug-resistant gram-negative bacteria: resistance to ceftazidime-avibactam in archived isolates of Pseudomonas aeruginosa. Antimicrob. Agents Chemother. 59, 1020–1029. doi: 10.1128/AAC.04238-14
Wise, M. G., Estabrook, M. A., Sahm, D. F., Stone, G. G., Kazmierczak, K. M. (2018). Prevalence of mcr-type genes among colistin-resistant Enterobacteriaceae collected in 2014-2016 as part of the INFORM global surveillance program. PloS One 13, e0195281. doi: 10.1371/journal.pone.0195281
Wong, D., van Duin, D. (2017). Novel β-lactamase inhibitors: unlocking their potential in therapy. Drugs 77, 615–628. doi: 10.1007/s40265-017-0725-1
Yu, H., Xu, X. S., Li, M., Yang, Q. W., Yang, Q., Zhang, R., et al. (2020). Expert consensus statement on laboratory detection and clinical report of carbapenemase among Enterobacterales. Chin. J. Infect. Chemother. 20, 671–680. doi: 10.16718/j.1009-7708.2020.06.015
Yu, H., Xu, X. S., Li, M., Yang, Q. W., Yang., Q., Zhang., R., et al. (2022). Expert consensus statement on laboratory detection and clinical report of carbapenemase among Enterobacterales (second edition). Chin. J. Infect. Chemother. 22, 463–474. doi: 10.16718/j.1009-7708.2022.04.014
Zhanel, G. G., Lawson, C. D., Adam, H., Schweizer, F., Zelenitsky, S., Lagacé-Wiens, P. R., et al. (2013). Ceftazidime-avibactam: a novel cephalosporin/β-lactamase inhibitor combination. Drugs 73, 159–177. doi: 10.1007/s40265-013-0013-7
Zhang, Y., Kashikar, A., Brown, C. A. (2017). Unusual Escherichia coli PBP 3 insertion sequence identified from a collection of carbapenem-resistant Enterobacteriaceae tested in vitro with a combination of ceftazidime-, ceftaroline-, or aztreonam-avibactam. Antimicrob. Agents Chemother. 61, e00389–e00317. doi: 10.1128/AAC.00389-17
Zhang, R., Liu, L., Zhou, H., Chan, E. W., Li, J., Fang, Y., et al. (2017). Nationwide surveillance of clinical carbapenem-resistant Enterobacteriaceae (CRE) strains in China. EBio Med. 19, 98–106. doi: 10.1016/j.ebiom.2017.04.032
Zhang, Y., Wang, Q., Yin, Y., Chen, H., Jin, L., Gu, B., et al. (2018). Epidemiology of carbapenem-resistant Enterobacteriaceae infections: report from the China CRE network. Antimicrob. Agents Chemother. 62, e01882–e01817. doi: 10.1128/AAC.01882-17
Zhen, S. S., Feng, S. Z. (2021). Updated clinical use of ceftazidime-avibactam in treating antibiotic-resistant bacterial infections. Chin. J. Infect. Chemother. 21, 752–758. doi: 10.16718/j.1009-7708.2021.06.025
Zheng, Y. G., Hu, F. P., Zhu, D. M., Guo, Y., Yang, Y., Dong, D., et al. (2020). CHINET surveillance of bacterial resistance in secondary care hospitals across China: report of results in 2019. Chin. J. Infect. Chemother. 20, 585–593. doi: 10.16718/j.1009-7708.2020.06.001
Zheng, G., Shi, Y., Cao, Y., Qian, L., Lv, H., Zhang, L., et al. (2022). Clinical feature, therapy, antimicrobial resistance gene distribution, and outcome of nosocomial meningitis induced by multidrug-resistant Enterobacteriaceae-A longitudinal cohort study from two neurosurgical centers in Northern China. Front. Cell. Infect. Microbiol. 12. doi: 10.3389/fcimb.2022.839257
Keywords: Carbapenem-resistant Enterobacteriaceae, BlaNDM, blaKPC, GeneXpert Carba-R, ceftazidime-avibactam
Citation: Zhu X, Guo C, Xu S, Lv F, Guo Z, Lin S, Yang CZ, Deng Z, Chen S, Huang Y, Zhao Z and Li L (2024) Clinical distribution of carbapenem genotypes and resistance to ceftazidime-avibactam in Enterobacteriaceae bacteria. Front. Cell. Infect. Microbiol. 14:1345935. doi: 10.3389/fcimb.2024.1345935
Received: 28 November 2023; Accepted: 08 March 2024;
Published: 20 March 2024.
Edited by:
Jean-ralph Zahar, Hôpital Avicenne, FranceCopyright © 2024 Zhu, Guo, Xu, Lv, Guo, Lin, Yang, Deng, Chen, Huang, Zhao and Li. This is an open-access article distributed under the terms of the Creative Commons Attribution License (CC BY). The use, distribution or reproduction in other forums is permitted, provided the original author(s) and the copyright owner(s) are credited and that the original publication in this journal is cited, in accordance with accepted academic practice. No use, distribution or reproduction is permitted which does not comply with these terms.
*Correspondence: Lu Li, bGlsdTI2OThAZ2RtdS5lZHUuY24=; Zuguo Zhao, emhhb3p1Z3VvQGdkbXUuZWR1LmNu; Ya Huang, aHkxODgyNTU0NjU0MUAxNjMuY29t
†These authors have contributed equally to this work