- 1School of Life Sciences and Medicine, Shandong University of Technology, Zibo, Shandong, China
- 2National Institute of Traditional Chinese Medicine Constitution and Preventive Medicine, Beijing University of Chinese Medicine, Beijing, China
- 3College of Life Science, Yangtze University, Jingzhou, Hubei, China
- 4Institute of Basic Theory for Chinese Medicine, China Academy of Chinese Medical Sciences, Beijing, China
- 5The Second Clinical Medical College, Henan University of Chinese Medicine, Zhengzhou, China
- 6Basic Medical College, Zhejiang Chinese Medical University, Hangzhou, China
Metabolic-associated fatty liver disease (MAFLD) is a chronic liver disease characterized by the excessive accumulation of fat in hepatocytes. However, due to the complex pathogenesis of MAFLD, there are no officially approved drugs for treatment. Therefore, there is an urgent need to find safe and effective anti-MAFLD drugs. Recently, the relationship between the gut microbiota and MAFLD has been widely recognized, and treating MAFLD by regulating the gut microbiota may be a new therapeutic strategy. Natural products, especially plant natural products, have attracted much attention in the treatment of MAFLD due to their multiple targets and pathways and few side effects. Moreover, the structure and function of the gut microbiota can be influenced by exposure to plant natural products. However, the effects of plant natural products on MAFLD through targeting of the gut microbiota and the underlying mechanisms are poorly understood. Based on the above information and to address the potential therapeutic role of plant natural products in MAFLD, we systematically summarize the effects and mechanisms of action of plant natural products in the prevention and treatment of MAFLD through targeting of the gut microbiota. This narrative review provides feasible ideas for further exploration of safer and more effective natural drugs for the prevention and treatment of MAFLD.
Highlights
● The gut microbiota may be a new target for MAFLD.
● Plant natural compounds can prevent and treat MAFLD by targeting the gut microbiota.
● Herb extracts could prevent and treat MAFLD by targeting the gut microbiota.
● TCM prescriptions could prevent and treat MAFLD by targeting the gut microbiota.
● Changing the structure and metabolites of the gut microbiota is the mechanism of action of plant natural products.
1 Introduction
Metabolic-associated fatty liver disease (MAFLD) is a new term for nonalcoholic fatty liver disease (NAFLD) (Eslam et al., 2020) and is a standard positive diagnosis based on metabolic factors and independent of alcohol use. Currently, the diagnostic criteria for MAFLD are based on evidence of hepatic steatosis (demonstrated by biopsy, imaging or validated serum biomarkers), in addition to one of the following criteria: overweight/obesity, type 2 diabetes mellitus, or metabolic dysregulation defined by the presence of at least two metabolic risk factors, including high waist circumference, hypertension, hypertriglyceridemia, hypo-HDL cholesterolemia, prediabetes, insulin resistance, and elevated high-sensitivity C-reactive protein levels. Recently, the prevalence rate of MAFLD has increased consistently. The global prevalence of MAFLD is already approximately 25% (Younossi et al., 2016), reaching approximately 70% in the obese population (Quek et al., 2022). MAFLD has become the most common chronic liver disease in the world. MAFLD comprises a continuous spectrum of liver diseases, including not simple fatty liver (NAFL) but also dynamic disease that can progress to steatohepatitis (NASH), decompensated cirrhosis and even hepatocellular carcinoma (HCC), and has gradually become an important cause of liver failure and liver transplantation. Moreover, the development of MAFLD often occurs in combination with various metabolic disorders and contributes to the progression of serious diseases, such as gout (Kuo et al., 2010), type 2 diabetes mellitus (T2DM) (Tanase et al., 2020), hypertension (Ma et al., 2021), and cardiovascular disease (Targher et al., 2016). Therefore, it is particularly important to find appropriate and efficient ways to control and treat MAFLD.
Currently, traditional management strategies for MAFLD largely focus on weight reduction and lifestyle modification. Lifestyle interventions consist primarily of dietary interventions and exercise interventions. Most studies of dietary weight loss have shown limited average weight loss (<5%) after 12 months of intervention, and there are some risks associated with long-term ketogenic diet interventions (Anekwe et al., 2020). For exercise interventions, it is recommend that the average adult complete at least 30 min of moderate-intensity aerobic exercise on 1 day and no less than 4 times per week for at least 16 weeks (Shojaee-Moradie et al., 2016). Both interventions (dietary and exercise interventions) are not only long-lasting and slow but also difficult to adhere to, leading to poor patient compliance. Pharmacological interventions are usually used in patients with MAFLD who fail to respond to conventional treatments. Pharmacological interventions prevent the progression of hepatitis and liver fibrosis by reducing liver fat accumulation and alleviating inflammatory damage. Studies have shown that drugs for type 2 diabetes can be used to treat patients with MAFLD (Mitrovic et al., 2022), but there are several toxic side effects. In fact, there are no Food and Drug Administration (FDA)-approved drugs for MAFLD treatment. Therefore, there is an urgent need to find safe and effective anti-MAFLD targets and drugs.
The gut microbiota plays an important role in regulating gut development, regulating host nutrient metabolism, preventing pathogenic bacterial colonization, and maintaining the gut barrier and immune function (Zhang et al., 2015). In recent years, numerous studies have shown that gut ecological dysbiosis is closely associated with the progression of obesity (Fei and Zhao, 2013; Cheng et al., 2022), type 2 diabetes (Ma et al., 2019) and cardiovascular disease (Xu H. et al., 2020). In particular, many studies have indicated that the gut microbiota is associated with the development of MAFLD (Le Roy et al., 2012). There are differences in the gut microbiota between healthy individuals and MAFLD patients, and MAFLD patients have poorer gut microbial ecological diversity and reduced bacterial abundance. Moreover, germ-free mice exhibited significantly reduced sensitivity to diet-induced hepatic steatosis (Bäckhed et al., 2004). Based on these findings, the gut microbiota has become a potential new therapeutic target for MAFLD.
A natural product is defined as “a product derived from a plant, animal or microbial source, also known as natural sources.” (Paola et al., 2023) Normally, plant natural compounds, herb extracts and traditional Chinese medicine (TCM) prescriptions have been considered the main forms of plant natural products. Historically, plant natural products, especially TCMs, have been used since ancient times and in folk medicine for the treatment of many diseases and illnesses. Recently, plant natural products have attracted much attention for the treatment of MAFLD due to their multiple targets and pathways and few side effects. However, their low bioavailability has limited their further development. Many research studies have indicated that only some plant natural products are absorbed in the small intestine, while most of these products reach the colon, where the composition of the gut microbiota can be affected, facilitating the metabolism of the microbiota and alleviating MAFLD. Recent studies have suggested that plant natural products may exert their hypolipidemic and hepatoprotective effects by altering the structure of and metabolite production by the gut microbiota (Zhang N-N. et al., 2023). However, the effects of plant natural products on MAFLD and the underlying mechanisms are also poorly understood and need to be further clarified, especially given the data from the last five years.
Based on the above information and to address the potential therapeutic role of plant natural products in MAFLD, we systematically summarize the effects and mechanisms of action of plant natural compounds, herb extracts and TCM prescriptions in the prevention and treatment of MAFLD through targeting of the gut microbiota. This review summarizes studies from the past 5 years reported in databases, including PubMed, Web of Science, Google Scholar, and X-MOL, which were filtered using the keywords “gut microbiota” and/or “MAFLD”. Those on human-modified substances, such as plant natural product-related nanoparticles or synthetic derivatives, are beyond the scope of this review. Overall, this narrative review provides feasible ideas for the further exploration of safer and more effective natural anti-MAFLD drugs.
2 A potential mechanism for the treatment of MAFLD by targeting the gut microbiota and its metabolites
The gut microbiome is a complex ecosystem composed of bacteria, archaea, fungi, protozoa and viruses that not only participates in nutrient digestion and immune regulation under host physiological and pathological conditions but also serves as a bridge between the gut and other extragut tissues (Kuziel and Rakoff-Nahoum, 2022). Thus, changes in the gut microbiome may affect the health of the host. Many studies have indicated that the gut microbiota is closely associated with the progression of MAFLD (Safari and Gérard, 2019; Hrncir et al., 2021; Shen et al., 2017; Boursier et al., 2016). For example, the ratio of Firmicutes to Bacteroides is greater in patients with MAFLD than in healthy people, and the abundances of Proteobacteria and Enterobacteriaceae increase while that of Ruminococcaceae decreases in these patients (Boursier et al., 2016). The abundance of Prevotella decreases significantly with increasing liver inflammation (Chen and Vitetta, 2020). However, the mechanism through which the gut microbiota affects MAFLD remains incompletely understood. With the development of metabolomics technology, changes in gut metabolites during the development of MAFLD have gradually been revealed (Chen and Vitetta, 2020). Among them, the roles of bile acid (BAs), lipopolysaccharides (LPSs), short-chain fatty acids (SCFAs), trimethylamine-N-oxide (TMAO) and vitamins have received widespread attention. These findings may lead to the identification of potential mechanisms of action for the treatment of MAFLD and to new feasible ideas for intervention therapy for MAFLD, as shown in Figure 1.
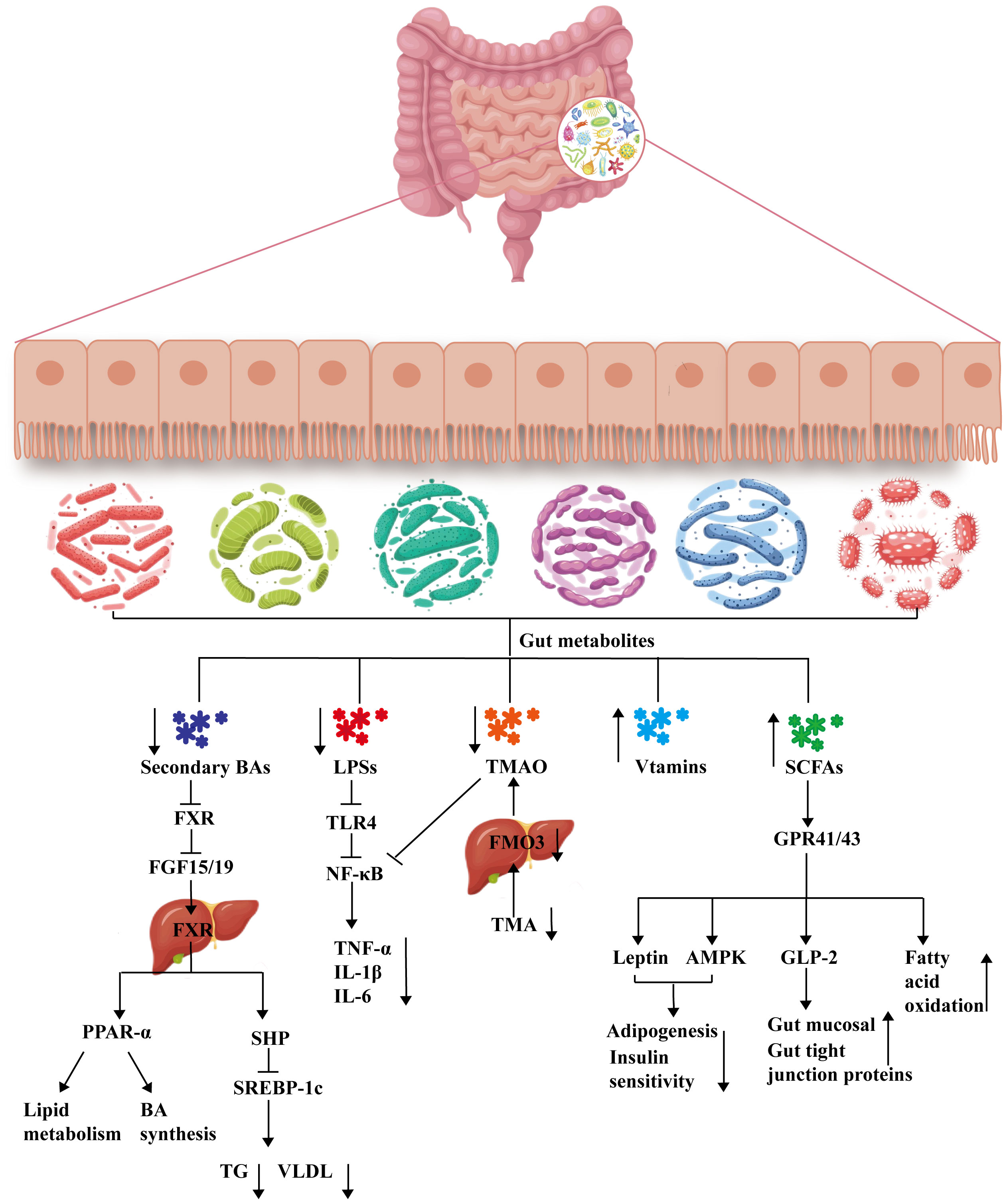
Figure 1 Mechanisms of gut metabolism involved in the treatment of MAFLD. BA, bile acid; FXR, farnesoid X receptor; FGF15/19, fibroblast growth factor 15/19; PPAR-α, peroxisome proliferator-activated receptor alpha; SHP, small heterodimer partner; SREBP-1c, sterol regulatory element binding protein-1c; TG, triglyceride; VLDL, very low-density lipoprotein; LPS, lipopolysaccharide; TLR4, Toll-like receptor-4; NF-κB, nuclear factor kappa-B; TNF-α, tumor necrosis factor alpha; IL-1β, interleukin-1β; IL-6, interleukin-6; TMAO, trimethylamine-N-oxide; TMO, trimethylamine; FMO3, flavin monooxygenase; SCFA, short-chain fatty acid; GPR41/GPR43, mammalian G protein-coupled receptor 41/43; AMPK, adenosine 5’-monophosphate (AMP)-activated protein kinase; GLP-2, glucagon-like peptide-2 receptor.
2.1 The gut microbiota may ameliorate MAFLD by regulating the BA pool composition
Bile acids (BAs) are cholesterol metabolites that play an important role in the balance of cholesterol and energy metabolism and the absorption of nutrients in the small intestine. The liver is the main site for BA synthesis. BAs are synthesized in the liver and subsequently secreted into the bile duct and stored in the gallbladder. After eating, the gallbladder contracts, and the stored BAs are expelled into the small intestine. In the small intestine, 95% of the BAs are reabsorbed by the small intestine and returned to the liver via the hepatic portal vein, with another approximately 5% exiting the body in the feces or entering systemic circulation (Di Ciaula et al., 2017). BAs act as emulsifiers to promote the absorption and transport of lipids and other substances in the small intestine and as indispensable signaling molecules that bind to a variety of receptors, including the nuclear receptor farnesoid X receptor (FXR), vitamin D receptor (VDR), pregnane X receptor (PXR) and the membrane-bound G protein-coupled receptor Takeda G protein-coupled receptor 5 (TGR5), which exert essential effects to regulate the balance of BA metabolism, glycolipid metabolism and energy metabolism (Perino and Schoonjans, 2022). Recently, much attention has been given to the interaction between BAs and the gut microbiota (Long et al., 2017). Gut microbes can directly modify BAs, such as bacteria that produce bile salt hydrolases (BSHs), which catalyze the conversion of primary bile acids to secondary bile acids, resulting in decreased levels of coupled BAs that may activate intestinal FXR expression and promote hepatic steatosis (Yang and Wu, 2022). Most studies have suggested that BA levels are elevated and that the BA composition is substantially altered in the serum and liver of MAFLD patients (Ferslew et al., 2015; Kalhan et al., 2010). It has also been established in animals that changes in the expression of BA-metabolizing enzymes and transporters that occur with the progression of NASH-related liver fibrosis lead to an increase in the plasma total bile acid (TBA) concentration (Suga et al., 2019). Based on the above information, the gut BA pathway might participate in the treatment of MAFLD.
Changes in composition are the main way that the gut microbiota alters BAs, and these changes affect the efficiency of binding of BAs to their receptors. For example, the order in which BAs bind and activate FXR is chenodeoxycholic acid > lithocholic acid > deoxycholic acid > cholic acid; the most efficacious BA ligands for TGR5 are in the order lithocholic acid > deoxycholic acid > chenodeoxycholic acid > cholic acid (Chiang, 2013). BAs regulate lipid and glucose metabolism mainly through the receptors FXR and TGR5 (Schaap et al., 2013). On the one hand, BAs can reduce triglyceride levels through the pathways of the FXR and the small heterodimer partner (SHP), as well as sterol regulatory element-binding protein 1c (SREBP-1c) (Watanabe et al., 2004). In addition, FXR activation promotes the expression of peroxisome proliferator-activated receptor alpha (PPAR-α), which facilitates the regulation of lipid metabolism, glucose homeostasis and anti-inflammatory activity (Carr and Reid, 2015; Pineda Torra et al., 2003). On the other hand, BAs not only activate TGR5 to improve insulin sensitivity and reduce obesity (Brighton et al., 2015; Thomas et al., 2009) but also inhibit the expression of cytochrome P450 7A1 (CYP7A1) by activating FXR and TGR5, thus inhibiting BA synthesis (Goodwin et al., 2000). Many studies have shown that TGR5 or FXR agonists can reduce lipogenesis, alleviate cholesterolemia, induce energy expenditure, and reduce liver inflammation (Pineda Torra et al., 2003; McMahan et al., 2013; Jadhav et al., 2018). BAs affect not only the gut-liver axis but also the gut-brain axis. BA-TGR5 signaling reduces the expression of Agouti-related protein (AgRP)/neuropeptide Y (NPY) and temporarily blocks the release of neuropeptides in AgRP/NPY neurons, which in turn inhibits feeding behavior, which may also be relevant to the treatment of MAFLD (Perino et al., 2021). Therefore, activation of FXR or TGR5 by regulating the gut microbiota to change the BA pool composition is expected to be the main therapeutic mechanism for the treatment of MAFLD.
2.2 Increased concentrations of SCFAs may ameliorate MAFLD in the gut microbiota
Under the action of anaerobic microorganisms in the mammalian colon, carbohydrates are degraded and fermented to produce large amounts of short-chain fatty acids (SCFAs), and acetic acid, propionic acid, and butyric acid make up approximately 90%-95% of the total SCFAs. SCFAs play an active role in the pathogenesis of MAFLD through portal vein entry into the liver and regulation of the inflammatory response, lipid metabolism and glucose metabolism (Zhou and Fan, 2019). SCFAs can also protect the gut barrier (Liu et al., 2020). Studies have shown that MAFLD patients have fewer SCFA-producing bacteria in the gut and decreased levels of SCFAs in the feces (Raman et al., 2013). In addition, numerous studies have demonstrated that SCFA or butyrate supplementation can repair the gut barrier and ameliorate NASH (Jin et al., 2015; Zhou et al., 2017; Gart et al., 2021; Ye et al., 2018). Therefore, the gut SCFA pathway might be a potential target for the treatment of MAFLD.
The specific receptors for SCFAs identified are mainly mammalian G protein-coupled receptor 41 (GPR41), mammalian G protein-coupled receptor 43 (GPR43), and G protein-coupled receptor 109 A (GPR109A). GPR41 and GPR43 are highly expressed in adipocytes, enteroendocrine cells and immune cells (polymorphonuclear cells and macrophages) (Maslowski et al., 2009), while GPR109A is expressed in adipocytes, hepatocytes and colon cells (Singh et al., 2014). These receptors usually mediate anti-inflammatory effects directly (Thorburn et al., 2014). Recent studies have shown that mammalian G protein-coupled receptors, especially GPR41, GPR43, and GPR109A, play important roles in metabolism, inflammation, and disease regulation and may be potential new drug targets for the treatment of certain metabolic diseases (Tan et al., 2014; Sekiguchi et al., 2015). The different SCFAs had the following order of activity against GPR43: propionic acid (C3) ≥ acetic acid (C2) = butyric acid (C4) > valeric acid (C5) > capric acid (C6) = formic acid (C1) (Brown et al., 2003; Nilsson et al., 2003). Activation of GPR43 directly inhibits lipid degradation (Hong et al., 2005; Ge et al., 2008). Thus, the gut microbiota may regulate lipid metabolism by affecting the SCFA composition and the expression of receptors in the gut. Moreover, SCFAs may inhibit adipogenesis through GPR41 or GPR43 by stimulating leptin secretion from white adipocytes in mice and suppressing appetite (Xiong et al., 2004; Zaibi et al., 2010). On the other hand, SCFAs also ameliorate gut inflammation and reduce gut mucosal injury. SCFAs may promote gut mucosal growth and development by inducing human glucagon-like peptide-2 activation; SCFAs may inhibit gut inflammation by activating GPR43 to protect the liver from portal vein-derived gut microbes while decreasing insulin sensitivity in adipose tissue and activating the hepatic adenosine 5’-monophosphate (AMP)-activated protein kinase (AMPK) signaling pathway, which directly or indirectly plays a protective role in liver health (Zhu et al., 2014). In conclusion, SCFAs and their receptors, which are regulated by the gut microbiome, play essential roles in regulating lipid metabolism and the inflammatory response, thereby improving the pathological progression of MAFLD.
2.3 The gut microbiota may ameliorate MAFLD by reducing LPS levels
LPSs are glycolipids that can be found on the surface of bacteria and are produced mainly by gram-negative bacteria. LPSs are endotoxins that are transported through the serum circulation to target tissues and are recognized by immune cells. Most LPSs activate inflammatory signaling pathways to secrete proinflammatory factors, cause the body to enter a state of chronic low-grade inflammation, induce metabolic abnormalities, and exert few anti-inflammatory effects through immune cells (Bertani and Ruiz, 2018). The gut microbiota is the main source of LPSs in healthy individuals (Candelli et al., 2021). Differences in the composition of the gut microbiota determine whether LPSs are toxic. For example, LPS produced by Bacteroides is harmless (Poli and Orange, 2017), while LPS produced by Escherichia coli is highly toxic and increases the level of fecal calprotectin, which is a marker of gut inflammation (Orivuori et al., 2015). Moreover, the serum LPS concentration was found to be positively correlated with the abundance of the aerobic bacteria Escherichia coli and Enterococcus and negatively correlated with the abundance of the anaerobic bacteria Lactobacillus, Bifidobacterium and Bacteroides, which is consistent with the results of microbiota changes in MAFLD patients (Xue et al., 2017).
Toll-like receptor 4 (TLR4) is an important member of the TLR family. Studies have shown that TLR4 is a key pattern recognition receptor for LPSs and plays an important role in the connection between the innate immune system and metabolic syndrome (Csak et al., 2011; Maddie et al., 2022; Sharifnia et al., 2015). During the progression of MAFLD, serum LPS levels and hepatic TLR4 expression were elevated in patients, while the gut microbiota diversity and biological colonization resistance of gut microorganisms were decreased (Thuy et al., 2008; Dapito et al., 2012). In addition, the correlation between hepatic TLR4 and the gut microbiota also showed the above trend. These findings suggest that LPSs and TLR4 are key molecules in the pathogenesis of MAFLD and that liver injury mediated by the LPS-TLR4 signaling pathway may be involved in the progression of MAFLD. Many studies have indicated that gut LPS and TLR4 activation is associated with diet-induced MAFLD onset, and the use of LPSs can serve as noninvasive tools for the diagnosis and grading of MAFLD severity in overweight and obese patients (Hegazy et al., 2020). Therefore, activation of TLR4 by regulating the gut microbiota to reduce LPS levels could regulate lipid metabolism and the inflammatory response and is expected to be the main therapeutic mechanism for the treatment of MAFLD.
2.4 The gut microbiota may affect MAFLD by regulating TMAO
Trimethylamine-N-oxide (TMAO) is an important product of the enterohepatic axis that is produced by the metabolism of trimethylamine (TMA) by the gut microbiota and enters the liver via the enterohepatic axis, where it is then oxidized by enzymes such as flavin-containing monooxygenase 3. Clinical studies have shown a significant increase in the serum TMA, TMAO and choline levels in patients with MAFLD compared to those in healthy individuals and that there is an association between high circulating TMAO concentrations and MAFLD as well as NASH (Theofilis et al., 2022; Flores-Guerrero et al., 2021; León-Mimila et al., 2020; Shi et al., 2022). TMAO may promote the progression of MAFLD through several mechanisms. For example, TMAO may directly enhance the development of MAFLD by affecting oxidative stress (Li X. et al., 2021); TMAO stimulation leads to increased expression of unfolded protein response-related proteins (glucose-regulated protein 78, X-box binding protein 1, and Derlin-1) (Shi et al., 2022), which may lead to hepatocyte lipid metabolism disorders and inflammation, causing the development and progression of MAFLD and ultimately to death (Lebeaupin et al., 2018; Song and Malhi, 2019). Furthermore, serum TMAO levels were found to be positively associated with total serum BA levels and hepatic CYP7A1 expression, suggesting that TMAO can increase BA synthesis and shift hepatic BA components toward FXR antagonistic activity, thereby exacerbating hepatic steatosis (Tan et al., 2019). However, it has also been shown that TMAO supplementation enhances the ability to repair tissue damage by increasing the number of endothelial cells in mice, thereby enhancing the integrity of the blood–brain barrier and protecting it from inflammatory damage, which may be beneficial in ameliorating NASH. For example, Zhou et al. reported that TMAO supplementation restored gut microbiota diversity, reduced liver fibrosis, and protected vascular function in mice, suggesting a possible protective effect of TMAO metabolic retrotransposition in the gut (Zhou et al., 2022). Therefore, reducing elevated TMAO levels by regulating the gut microbiota is expected to be the main therapeutic mechanism for the treatment of MAFLD.
2.5 The gut microbiota may affect MAFLD by regulating gut vitamins
Vitamins are essential nutrients for the normal growth of humans and animals, and deficiency and overdose can lead to metabolic disorders and reduced growth performance (Brennan, 2006). The human body cannot synthesize most vitamins and must obtain them from the diet or rely on symbiotic bacteria in the gastrointestinal tract for their synthesis (Hill, 1997). For example, Lactobacillus and Bifidobacterium species are capable of synthesizing most water-soluble B vitamins, and E. coli is capable of synthesizing vitamin K. The development of MAFLD has been shown to disrupt the gut microbiota; therefore, MAFLD may affect the synthesis of vitamins in the body through the gut microbiota. Clinical studies have indicated that vitamin D (VD) levels are markedly lower in patients with MAFLD than in those without this disease (Chung et al., 2016; Tian et al., 2020). The biological roles of VD include not only maintaining the balance of calcium and phosphorus metabolism in the body and participating in bone reconstruction but also reducing insulin resistance, modulating immunity, protecting cardiovascular health, and exerting antifibrosis and anti-inflammation effects (Chun et al., 2019; Whiting and Calvo, 2021). Numerous epidemiological investigations have shown that VD deficiency is closely associated with MAFLD, cirrhosis and obesity, and there is also evidence that VD has a protective effect against MAFLD (Tian et al., 2020; Du et al., 2023; Barchetta et al., 2011; Ciardullo et al., 2023; Barchetta et al., 2020; Barchetta et al., 2017; Pop et al., 2022). Furthermore, VD supplementation can alter the abundance and diversity of the gut microbiota. Zhang et al. reported that VD supplementation could mitigate MAFLD by increasing the relative abundances of Prevotella and Porphyromonadaceae and decreasing the relative abundances of Mucispirillum, Acetatifactor, Desulfovibrio and Oscillospira (Zhang X-L. et al., 2023). In addition to VD, numerous studies have shown that supplementation with vitamin B12 (Li L. et al., 2022), vitamin C (Lee et al., 2022), vitamin E (Perumpail et al., 2018), or a vitamin-rich diet could alleviate the progression of MAFLD. Therefore, regulating the gut microbiota by vitamin supplementation may be an additional modality for MAFLD treatment.
2.6 Other metabolites
In addition to the above metabolites, the gut microbiota secretes metabolites such as sphingolipids, amino acids, phenolic acids, and ethanol. However, the relationship between these metabolites and the progression of MAFLD requires further investigation. In summary, alterations in the gut microbiota and its metabolites may be potential therapeutic targets for MAFLD.
3 Plant natural products ameliorate MAFLD by regulating the gut microbiota
Plant natural products, including plant natural compounds, herb extracts and TCM prescriptions, have attracted much attention in the treatment of MAFLD due to their effects on multiple pathways and multiple targets and their few adverse reactions. Plant natural products can not only affect the gut microbial composition but also regulate gut microbial metabolism. This review systematically summarizes the effects and mechanisms of action of plant natural compounds, herb extracts and TCM prescriptions in the prevention and treatment of MAFLD through the targeting of the gut microbiota reported in studies published from 2017 to 2023 (Table 1).
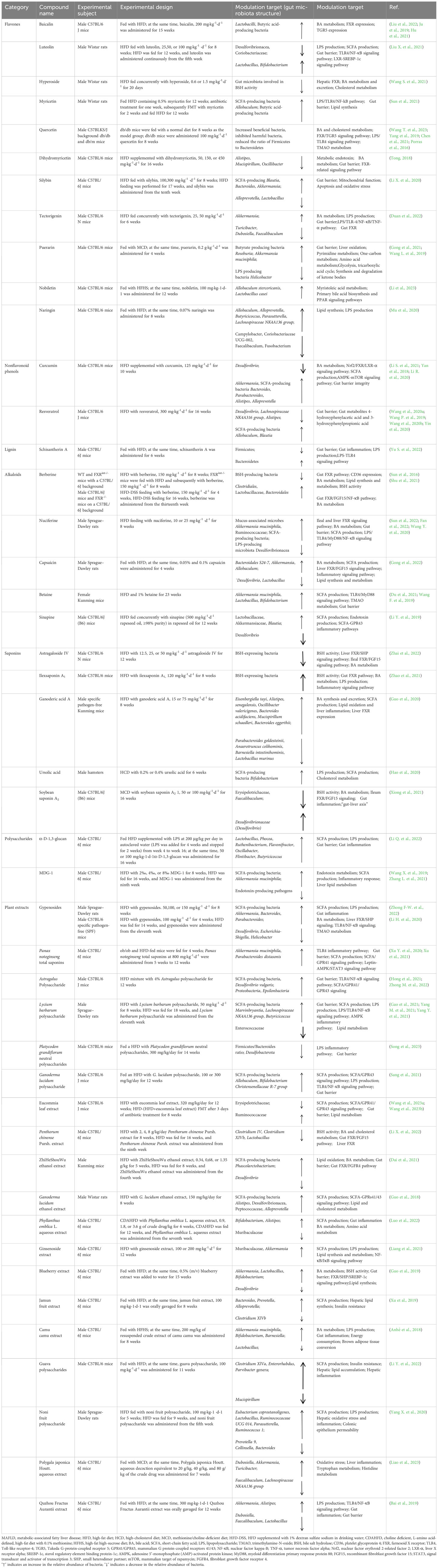
Table 1 Mechanism of action of plant natural compounds and herb extracts in the treatment of MAFLD involves modulating the gut microbiota and its metabolites.
3.1 Plant natural compounds ameliorate MAFLD by regulating the gut microbiota
Plant natural compounds mainly include active ingredients, such as polyphenols, lignans, alkaloids, saponins and polysaccharides. Polyphenols can be divided into flavonoid and nonflavonoid phenolics. Modern pharmacological studies have isolated the numerous active monomeric compounds mentioned above, as shown in Figure 2, and have shown that these active monomers can act as therapeutic agents for MAFLD by modulating the gut microbiota and its metabolites.
3.1.1 Polyphenols
3.1.1.1 Flavones
Baicalin is the main active component of the Chinese herb Scutellaria baicalensis Georgi. Recent studies have shown that baicalin is a typical multitarget and multipathway drug with hepatoprotective, antitumor, antibacterial, anti-inflammatory, antidepressant and antioxidant effects. Studies suggest that the effect of baicalin in treating MAFLD may be related to the gut microbiota. Baicalin inhibits liver fibrosis and inflammation by increasing the relative abundance of lactobacilli (Liu et al., 2022). Moreover, baicalin increases the abundance of SCFA-producing bacteria, especially butyric acid-producing bacteria, which is beneficial for improving gut homeostasis (Ju et al., 2019). On the other hand, baicalin may reduce hepatic cholestasis and alleviate cholestasis-induced liver fibrosis by increasing the activity of BA-metabolizing enzymes and key receptors such as FXR and TGR5 through targeting of the gut microbiota (Hu et al., 2021).
Luteolin (3’,4’,5,7-tetrahydroxy flavone) is a natural flavonoid that is widely found in vegetables, fruits, and natural herbs and has anti-inflammatory, antioxidant, antitumor and immunomodulatory effects. Recent studies have shown that luteolin intervention markedly regulates the gut microbial composition in MAFLD rats by decreasing the abundances of Desulfovibrionaceae and Coriobacteriaceae to reduce plasma LPS concentrations, inhibiting the TLR4/nuclear factor kappa-B (NF-κB) signaling pathway in the liver, and reducing the secretion of proinflammatory factors (Liu X. et al., 2021). Moreover, luteolin can increase the abundances of Lactobacillus and Bifidobacterium. Therefore, luteolin may alleviate MAFLD by restoring the microbiota imbalance.
Hyperoside (quercetin-3-O-galactoside) is a flavonol glycoside found in Crataegus pinnatifida Bunge., Artemisia capillaris Thunb., and Hyperlcurn perforatum L. that has anti-inflammatory, hepatoprotective, and antioxidant protective effects. Recent studies have shown that hyperoside can alter the high-fat diet (HFD)-induced gut microbiota composition. Hyperoside modulates the BA composition by inhibiting gut microbes involved in BSH activity, which increases hepatic FXR receptor activation, promotes free fatty acid β-oxidation, and inhibits de novo fatty acid synthesis (Wang S. et al., 2021). Therefore, the regulation of BA synthesis and transport by hyperoside may be the mechanism underlying the alleviation of MAFLD.
Myricetin (3,3,4,5,5,7-hexahydroxyflavone) is a flavonoid with antioxidant, anti-inflammatory, antibacterial, anticancer, antidiabetic and hepatoprotective effects (Song et al., 2020). Myricetin supplementation not only increases the abundance of SCFA-producing bacteria to reduce liver lipid synthesis and liver inflammation in HFD-induced MAFLD but also modulates insulin resistance by increasing the fecal butyric acid concentration, which may also benefit the treatment and prevention of MAFLD (Sun et al., 2021).
Quercetin (3,3,4’,5,7-pentahydroxyflavone) is an abundant polyphenolic flavonoid with a variety of bioactivities including antioxidant, anti-inflammatory, antiapoptotic, immunoprotective and anticancer properties. Quercetin can improve gut microbiota imbalance (Wang T. et al., 2023) and modulate gut microbiota metabolites to ameliorate MAFLD. On the one hand, quercetin maintains lipid homeostasis and reduces hepatic steatosis by regulating gut BA metabolism and activating FXR and TGR5 in the liver (Yang et al., 2019; Chen et al., 2021). On the other hand, quercetin can reverse gut microbiota disorders and inhibit the endotoxemia-mediated TLR-4 pathway, which in turn inhibits inflammatory vesicle responses and reticulostriatal pathway activation, leading to blocked lipid metabolism abnormalities (Porras et al., 2016).
Dihydromyricetin, the most abundant flavonoid in Ampelopsis grossedentata (Hand.-Mazz.) W.T. Wang (vine tea), has been proven to have anti-inflammatory, antioxidant, antihypertensive, hepatoprotective, lipid-modulating and antitumor effects. Studies have indicated that dihydromyricetin can prevent and ameliorate MAFLD by regulating the gut microbiota and its metabolites. Dihydromyricetin not only affects BSH activity by reducing the abundance of Lactobacillus but also enhances BA binding and BA transport in the liver and inhibits FXR-related signaling pathway-mediated BA reabsorption in the ileum. In addition, dihydromyricetin can improve gut mucosal barrier function; increase the expression of gut tight junction proteins such as zonula occludens protein 1 (ZO-1), Occludin, and Claudin1; improve gut microvillus structure; and reduce peripheral serum LPS levels (Tong, 2018).
Silybin is isolated from the seeds of Silybum marianum (L.) Gaertn. (milk thistle) and is widely used as a hepatoprotective agent (Federico et al., 2017). Studies suggest that the effect of silybin in treating liver diseases may be achieved by modulating the gut microbiota and its metabolites (Li X. et al., 2020). Mechanistic studies revealed that silybin supplementation increased the relative abundances of the SCFA-producing Blautia, Bacteroides and Akkermansia, thereby increasing the gut levels of acetate, propionate and butyrate while suppressing the levels of formate, ultimately exhibiting protective effects against MAFLD and hepatitis. In addition, silybin improved gut mucosal barrier function by increasing ZO-1 and Occludin expression. However, silybin also inhibits some known beneficial bacteria, such as Alloprevotella and Lactobacillus; therefore, the relationship between silymarin-induced alterations in the gut microbiota and the alleviation of MAFLD needs to be investigated in greater depth.
Tectorigenin, a methoxy isoflavone with three hydroxyl groups, can be isolated from many medicinal plants, such as Iris unguicularis, Belamcanda chinensis (L.) DC., and Pueraria lobata (Willd.) Ohwi. Modern medical studies have shown that tectorigenin has anti-inflammatory, antioxidative, and antidiabetic effects on oxidative stress injury. Duan et al. (Duan et al., 2022) reported that tectorigenin could restore gut barrier function by promoting the growth of Akkermansia and inhibiting Turicibacter, Dubosiella and Faecalibaculum, which in turn reduced LPS levels and inhibited the LPS/TLR-4/NF-κB/tumor necrosis factor alpha (TNF-α) pathway to alleviate liver inflammation. Furthermore, tectorigenin can also activate the expression of hepatic and gut FXR, which is involved in the reduction of serum TBA levels and the excretion of fecal BAs, to reduce lipid accumulation and bacterial translocation. Therefore, tectorigenin may potentially mediate the gut-liver axis to attenuate MAFLD.
Puerarin (7,4-dihydroxy-isoralone-8-glucoside) is an active isoflavone glycoside extracted from the dry root of P. lobata that has vasodilatory, cardioprotective, antioxidant, anti-inflammatory, and anti-insulin resistance effects. It was found that puerarin treatment could inhibit the activity of the LPS-producing Helicobacter species, promote the activity of the butyrate-producing Roseburia species, and increase the abundance of Akkermansia muciniphila (Gong et al., 2021) to increase gut expression levels of Mucin 2 and Reg3g and protect gut barrier function by increasing ZO-1 and Occludin expression in vivo and in vitro (Wang L. et al., 2019). Thus, the anti-MAFLD activity of puerarin may be closely associated with the regulation of the gut microbiota.
Nobiletin is a polymethoxyflavonoid that is the main component of Citri Reticulatae Pericarpium and has been shown to have anti-obesity (Lee et al., 2012), antihyperglycemic (Lee et al., 2010), antihypercholesterolemic and anti-MAFLD activities (Kim et al., 2021). Recently, Li et al. (Li et al., 2023) reported that nobiletin treatment not only ameliorated high-fat high-sucrose feed-induced lipid accumulation but also reversed the dysbiosis of the gut microbiota in mice with MAFLD and increased the relative abundance of Allobaculum stercoricanis and Lactobacillus casei. Moreover, untargeted metabolomics revealed that nobiletin modulated the metabolism of the long-chain fatty acid myristoleic acid and experimentally demonstrated the protective effects of fecal transplantation as well as the administration of bacteria or metabolites to treat MAFLD. These results suggest that nobiletin may be a potential treatment for MAFLD, but further studies are needed.
Naringin, a naturally occurring flavonoid found predominantly in the rinds of grapefruit and citrus fruits, has been reported to have antihyperglycemic (Ahmed et al., 2017) and antihyperlipidemic properties (Yu X. et al., 2022). Mu et al. (Mu et al., 2020) reported that naringin treatment increased the relative abundance of Allobaculum, Alloprevotella, Butyricicoccus and Parasutterella and downregulated the expression of the sterol regulatory element binding protein 1, fatty acid synthase, acetyl-CoA carboxylase 1, and stearoyl-CoA desaturase 1 proteins, effectively attenuating hepatic de novo fatty acid synthesis. On the other hand, naringin treatment reduced the relative abundance of the deleterious Campylobacter species and the proinflammatory Faecalibaculum and Fusobacterium species, which may be associated with the reduction in LPS levels in the serum and hepatic inflammatory cells of mice with MAFLD. Thus, naringin may attenuate NAFLD by preventing gut ecological dysregulation, but experimental evidence confirming that naringin attenuates MAFLD by directly modulating the gut microbiota is lacking.
3.1.1.2 Nonflavonoid phenols
Curcumin is a natural polyphenol compound isolated from Curcuma longa L. that has been proven to have antiobesity, anticancer, antioxidant, hepatoprotective and other biological activities. Studies suggest that curcumin may ameliorate MAFLD by regulating the gut microbiota through the enrichment of the SCFA-producing bacteria Bacteroides, Parabacteroides, Alistipes and Alloprevotella and a reduction in the abundance of the endotoxin-producing Desulfovibrio (Li S. et al., 2021). In addition, curcumin can inhibit hepatic lipogenesis and promote BA metabolism by regulating the nuclear factor erythroid 2-related factor 2 (Nrf2)/FXR/liver X receptor alpha (LXR-α) pathway (Yan et al., 2018). Furthermore, the results of a comparative study of curcumin and metformin showed that they had similar effects in reducing hepatic steatosis, improving gut barrier integrity and regulating the gut microbiota in rats with HFD-induced obesity and that curcumin may prove to be a novel adjuvant therapy for MAFLD (Li R. et al., 2020).
Resveratrol is a natural polyphenolic compound that is mainly isolated from Vitis vinifera L. (grape) and has anticancer, anti-inflammatory, antioxidant, and antiobesity effects. Resveratrol can attenuate HFD-induced steatosis through modulation of the gut microbiota composition (Wang et al., 2020a; Wang P. et al., 2019; Wang et al., 2020b; Yin et al., 2020). Resveratrol not only reduced the relative abundances of the harmful bacteria Desulfovibrio, Lachnospiraceae NK4A316 group and Alistipes but also increased the relative abundances of the SCFA-producing bacteria Allobaculum and Blautia (Wang et al., 2020b) and the gut metabolites 4-hydroxyphenylacetic acid and 3-hydroxyphenylpropionic acid, contributing to the improvement in lipid metabolism (Wang et al., 2020a). Furthermore, transplantation of the resveratrol-induced microbiota into HFD-fed mice also showed therapeutic effects (Wang et al., 2020a; Wang P. et al., 2019; Wang et al., 2020b; Yin et al., 2020). These results demonstrated that resveratrol has the potential to regulate the gut-liver axis to ameliorate MAFLD.
3.1.2 Lignin
Schisantherin A is an active substance isolated from the fruit of Schisandra chinensis (Turcz.) Baill., a perennial deciduous woody liana with antiparkinsonian, anti-inflammatory, hepatoprotective, ischemia–reperfusion injury prevention and osteoclast formation inhibition effects (Xiao et al., 2022). The hepatoprotective effects of schisantherin A may be achieved by improving gut inflammation and modulating the gut microbiota (Yu S. et al., 2022). On the one hand, schisantherin A treatment alleviated the HFD-induced imbalance in the gut microbiota by reducing the abundance of Firmicutes and increasing the abundance of Bacteroidetes. Furthermore, antibiotic treatment demonstrated the role of the gut microbiome in the schisantherin A-mediated improvement in liver inflammation. On the other hand, schisantherin A treatment reduced gut LPS production and release in HFD-fed mice and inhibited the LPS-TLR4 signaling pathway to ameliorate gut permeability impairment and inhibit the progression of MAFLD to NASH.
3.1.3 Alkaloids
Berberine, also known as safranin, is isolated from the root of Coptis chinensis Franch. and is traditionally used to treat diarrhea. Modern pharmacological studies have reported that berberine is beneficial for treating metabolic disorders, such as type 2 diabetes, dyslipidemia, and MAFLD/NASH. However, because the bioavailability of berberine is low, the gut microbiome and microbe-derived metabolites are thought to be key factors involved in the mechanisms of action of berberine (Cheng et al., 2021). Berberine may exert its lipid-lowering effects mainly through the regulation of BA turnover and thus through the gut FXR signaling pathway (Sun et al., 2016; Shu et al., 2021). Sun et al. reported that berberine increased taurine-coupled BA levels by reducing the relative abundance of BSH-producing bacteria, which activated the gut FXR pathway (Sun et al., 2016). Shu et al. reported that berberine alleviated NASH by modulating the gut microbiota and BA metabolism and upregulating gut FXR and recombinant fibroblast growth factor 15 (FGF15) expression and FGF15 secretion to further inhibit adipogenesis and NF-κB activation in the liver (Shu et al., 2021).
Nuciferine, the major functional aporphine alkaloid from the dried leaves of Nelumbo nucifera Gaertn., has been shown to be useful for reducing body weight, lowering serum and liver lipids, and alleviating hepatic steatosis and liver damage. Nuciferine supplementation not only reduced the abundances of BSH-producing and 7α-dehydroxylated bacteria, leading to the accumulation of coupled BAs as FXR antagonists to inhibit gut FXR signaling but also regulated the BA cycle in vivo by modulating the levels of the rate-limiting enzymes CYP7A1 and cytochrome P450 27A1 (CYP27A1) and the BA transporters bile salt export pump (BSEP) and Na+-taurocholate cotransporting polypeptide (NTCP) in the liver (Sun et al., 2022). In addition, nuciferine elevated the relative abundances of mucus-associated microbes (Akkermansia muciniphila and Ruminococcaceae) and SCFA-producing bacteria to improve gut barrier integrity and reduce liver inflammation by decreasing the abundance of LPS-producing microbes (Desulfovibrionaceae), reducing LPS production, and inhibiting the TLR4/myeloid differentiation primary response protein 88/NF-κB signaling pathway (Fan et al., 2022; Wang Y. et al., 2020).
Capsaicin (trans-8-methyl-N-vanillyl-6-nonenamide) is one of the active ingredients of Capsicum annuum L. (pepper) and has anti-statogenic, antioxidant, anti-inflammatory and antifibrotic effects. Studies have indicated that capsaicin treatment increases the relative abundances of Bacteroidales S24-7, Akkermansia and Allobaculum, leading to the accumulation of SCFAs, which in turn enhances lipid accumulation and decreases TG and TC levels. Furthermore, capsaicin decreased BSH activity by inhibiting Lactobacillus, which increased the levels of conjugated BAs, especially tauro-β-muricholic acid (T-β-MCA), which in turn inhibited the enterohepatic FXR-FGF15 axis to regulate the composition of the BA pool (Gong et al., 2022). These results suggested that capsaicin can mitigate MAFLD by regulating the gut microbiota and the composition of BAs and SCFAs.
Betaine is a quaternary ammonium-type water-soluble alkaloid with antioxidant and anti-inflammatory effects. Betaine is an amino acid (trimethylglycine) that is a necessary intermediate in the catabolism of choline. Betaine supplementation can increase the abundances of Akkermansia muciniphila, Lactobacillus and Bifidobacterium and promote the production of SCFAs (Du et al., 2021). In mice lacking a gut microbiota, the ability of betaine to prevent HFD-induced obesity and metabolic syndrome was significantly reduced, which suggested that betaine can alleviate MAFLD by regulating the gut microbiota. In addition, Wang et al. (Wang F. et al., 2019) reported that betaine can reduce liver lipid accumulation by improving gut BA and TMA-related oxidative metabolism in blunt mouth bream.
Sinapine accounts for 70-80% of rapeseed polyphenols and may have the potential to ameliorate MAFLD. It was shown that sinapine intervention could regulate the HFD-induced gut microbiota, increase the relative abundance of the probiotic bacteria Lactobacillaceae, and inhibit endotoxin production by reducing the relative abundance of Desulfovibrio. Moreover, supplementation with sinapine could increase the abundance of the SCFA-producing bacteria Akkermansiaceae and Blautia, which increase SCFA levels and upregulate the GPR43 receptor to inhibit gut inflammatory factor expression (Li Y. et al., 2019). Therefore, sinapine has a therapeutic effect on MAFLD by regulating the gut microbiota.
3.1.4 Saponins
Astragaloside IV is one of the major saponin compounds extracted from the roots of Astragalus membranaceus (Fisch.) Bge. and has anti-inflammatory, anti-liver fibrosis, antioxidative stress, anti-asthma, antidiabetic, immunomodulatory and cardioprotective effects (Li et al., 2016). Astragaloside IV inhibits gut FXR expression by reducing the abundance of BSH-expressing bacteria and decreasing BSH activity, thus increasing the level of T-β-MCA, which is often accompanied by a decrease in FGF15 and subsequent activation of hepatic FXR, leading to the inhibition of hepatic steatosis (Zhai et al., 2022). Moreover, fecal transplantation experiments further demonstrated that the action of astragaloside IV is dependent on the gut microbiota. Therefore, alterations in the gut microbiota and BAs may be involved in the mechanism of action of astragaloside IV for treating MAFLD.
Ilexsaponin A1 is one of the most abundant triterpenoid saponins and has antithrombotic and anticoagulant properties; it was isolated from Ilex chinensis Sims, a small evergreen tree with red fruit. Previous studies have shown that the combination of ilexhainanoside D and ilexsaponin A1 modulates the gut microbiota, restores gut barrier function, and ameliorates gut inflammation (Zhao et al., 2019). A separate study of ilexsaponin A1 revealed that ilexsaponin A1 may exert cholesterol-lowering and MAFLD-inhibiting effects by altering the gut microbiota and BA metabolism (Zhao et al., 2021). Ilexsaponin A1 intervention enhanced BSH activity by increasing the relative abundance of BSH-producing bacteria, which increased BA uncoupling and excretion in the ileum. Moreover, ilexsaponin A1 intervention also increased FXR and BSEP expression and decreased NTCP expression, which increased hepatic BA efflux and reduced BA uptake. In addition, intake of ilexsaponin A1 also decreased the serum LPS content and the expression of inflammatory cytokines.
Ganoderic acid A is one of the most abundant triterpenes isolated from the red fungus Ganoderma lucidum (Leyss. ex Fr.) Karst. and has antinociceptive, antioxidative and anticancer pharmacological effects. Ganoderic acid A intervention increased the relative abundances of Eisenbergiella tayi, Alistipes senegalensis, Oscillibacter valericigenes, Bacteroides acidifaciens, Mucispirillum schaedleri and Bacteroides eggerthii but significantly reduced the relative abundances of Parabacteroides goldesteinii, Anaerotruncus colihominis, Barnesiella intestinihominis, and Lactobacillus murinus, which increased the production of SCFAs in the gut. In addition, ganoderic acid A treatment can interfere with the regulation of BAs. Ganoderic acid A not only upregulated the expression of liver genes (FXR, CYP7A1 and NTCP) involved in BA homeostasis and reduced liver BA levels but also promoted the excretion of BAs through the feces (Guo et al., 2020). Therefore, the ability of ganoderic acid A to improve MAFLD may be mediated by regulation of the gut microbiota and metabolites.
Ursolic acid is a natural pentacyclic triterpene carboxylic acid that occurs naturally in various fruits and vegetables, such as apples, blueberries and cranberries, and has antioxidant, anti-inflammatory, anticancer and hepatoprotective effects. Recent studies have indicated that the addition of ursolic acid is effective at promoting the growth of Bifidobacterium, which is recognized as a genus of SCFA-producing bacteria. Bifidobacterium species, as recognized probiotics, have been shown to be effective at limiting the production of the endotoxins LPSs. In addition, ursolic acid can promote the production of SCFAs, including acetic acid, propionic acid and butyric acid, in feces (Hao et al., 2020). Therefore, ursolic acid holds promise as a potential therapeutic agent for ameliorating MAFLD, but further studies are needed.
Soyasaponins are phytochemicals found in Glycine max (L.) Merr. (soya bean) and that have antioxidant, anti-inflammatory, hypoglycemic, cholesterol-lowering, and hepatoprotective effects. Soyasaponin A2 is a monomer of soyasaponins, and Xiong et al. (Xiong et al., 2021) reported that the hepatoprotective effect of soyasaponin A2 against NASH may be achieved by modulating the gut microbiota metabolite BAs. On the one hand, soyasaponins A2 may alleviate methionine–choline-deficient (MCD) diet-induced steatohepatitis by reducing the abundance of Erysipelotrichaceae and Faecalibaculum species. On the other hand, it may not only effectively block gut-liver circulation and the reabsorption of BAs in the terminal ileum by forming mixed micelles with BAs but also directly bind to BAs and promote their excretion via feces.
3.1.5 Polysaccharides
Radix Puerariae thomsonii, the root of the botanical family Fabaceae species Pueraria montana var. thomsonii (Benth.) MR Almeida, can be used as food or medicine. Recently, an α-D-1,3-glucan isolated and purified from Radix Puerariae thomsonii was shown to not only reduce HFD-induced liver injury, inflammation, glucose metabolism, and steatosis but also regulate the gut microbiota and its metabolites (Li Q. et al., 2022). α-D-1,3-glucan treatment not only increased the relative abundance of Lactobacillus, Phocea, Ruthenibacterium, Flavonifractor, Oscillabacter, Flavinibacter, and Butyricoccus to increase butyric acid, propionic acid and acetate levels but also promoted the integrity of the gut barrier by increasing the expression of tight junction proteins (ZO-1, Occludin, and Claudin-4) and mucin (Mucin 2). In addition, α-D-1,3-glucan administration significantly reduced the serum levels of the proinflammatory factor TNF-α and LPSs and inhibited HFD-induced inflammation. In conclusion, α-D-1,3-glucan is expected to be a potential drug for alleviating MAFLD.
MDG-1, a β-D-fructose polysaccharide isolated and purified from the root of Ophiopogon japonicus (L.f.) Ker-GawL., prevents HFD-induced obesity and hyperlipidemia in mice. MDG-1 modulates the structure of the gut microbiota by promoting the growth of beneficial SCFA-producing bacteria and reducing the abundance of endotoxin-producing pathogens to inhibit the inflammatory response and liver lipid metabolism (Wang X. et al., 2019). In addition, MDG-1 intervention markedly promoted the growth of Akkermansia muciniphila, the abundance of which was inversely proportional to MAFLD (Zhang L. et al., 2021). Therefore, MDG-1 may be a potential agent for preventing and treating MAFLD by targeting the gut microbiota.
3.2 Herb extracts ameliorate MAFLD by regulating the gut microbiota
Plant natural products also include extracts with diverse functions, including total polyphenols, total saponins, and total polysaccharides. Plant natural products may act through a single compound in the total extract, but this phenomenon has not been studied at the monomer level because of certain limitations, such as those of isolation techniques. On the other hand, the complex compounds of the extracts may interact with each other and work together to exert therapeutic effects. Studies have shown that some herbal extracts can alter the gut microbiota and its metabolites to exert beneficial effects against MAFLD, as shown in Figure 3.
Gypenosides are the main components of Gynostemma pentaphyllum (Thunb.) Makino, a herb known as “southern ginseng”. These compounds are widely used as hepatoprotective agents in Asia, and their mechanism of action may be related to improvement of the gut microbiota. Gypenosides not only promoted the growth of SCFA-producing bacteria (Akkermansia, Bacteroides and Parabacteroides) and increased the content of SCFAs (acetate, propionate and butyrate) but also decreased the relative abundances of harmful bacteria (Desulfovibrio, Escherichia-Shigella and Helicobacter) to inhibit LPS production, thereby suppressing inflammation (Zhong F-W. et al., 2022). In addition, gypenosides also inhibited hepatic lipid synthesis by modulating the gut BA composition and increasing hepatic FXR and SHP levels (Li H. et al., 2020).
Panax notoginseng saponins are the main bioactive components of Panax notoginseng (Burk.) F.H. Chen, also known as “the king of ginseng”, and have been found to reduce serum lipid and liver lipid accumulation. Panax notoginseng saponins promoted the growth of Akkermansia muciniphila and Parabacteroides distasonis, which activated the leptin-AMPK/signal transducer and activator of transcription 3 signaling pathway to promote energy expenditure and reduce lipid accumulation (Xu Y. et al., 2020). In addition, Panax notoginseng saponin supplementation can increase the levels of long-chain fatty acids (oleic acid and 2-palmitoyl glycerol) and SCFAs (acetic acid, butyric acid, propionic acid, etc.) in feces, and then large amounts of SCFAs enter the liver to activate GPR41, further ameliorating steatosis and inflammation (Xu et al., 2021). Therefore, Panax notoginseng saponins may alleviate MAFLD by regulating the structure and function of the gut microbiota.
Astragalus polysaccharide is an active ingredient isolated from A. membranaceus that has antioxidant, anti-inflammatory, hypoglycemic, antiviral, hypolipidemic and immunomodulatory effects. Mechanistic studies suggest that Astragalus polysaccharide may ameliorate MAFLD by altering the gut microbiota composition. Hong et al. (Hong et al., 2021) reported that Astragalus polysaccharide can specifically enrich SCFA-producing bacteria, such as Desulfovibrio vulgaris, which can effectively produce acetic acid and inhibit the expression of liver fatty acid synthase and platelet glycoprotein 4 protein to exert anti-MAFLD effects. Zhong et al. (Zhong M. et al., 2022) reported that Mongolian Astragalus polysaccharide intervention could decrease the ratio of Firmicutes to Bacteroides and increase the abundances of Proteobacteria and Epsilonproteobacteria. In addition, Mongolian Astragalus polysaccharide supplementation may alleviate liver inflammation and lipid accumulation in MAFLD treatment through the gut microbiota by modulating TLR4-mediated inflammatory pathways and the SCFA-GPR signaling pathway.
Lycium barbarum polysaccharide is the main bioactive component of Lycium barbarum L. (goji berry) and has many pharmacological properties, such as serum glucose-lowering, serum lipid-lowering, anti-inflammatory, antioxidant and antitumor effects. Numerous studies have demonstrated that Lycium barbarum polysaccharide can alleviate MAFLD by altering the gut microbiota composition and SCFA metabolism (Gao et al., 2021; Yang M. et al., 2021; Yang Y. et al., 2021). Lycium barbarum polysaccharide intervention increased the relative abundances of the SCFA-producing bacteria Marvinbryantia, Lachnospiraceae NK4A136 group and Butyricicoccus, which increased the content of fecal SCFAs (Yang M. et al., 2021; Yang Y. et al., 2021). Moreover, it also inhibited the LPS/TLR4/NF-κB signaling pathway by inhibiting the increase in the harmful bacteria Enterococcaceae and their metabolite LPSs (Gao et al., 2021), thereby alleviating MAFLD. Therefore, Lycium barbarum polysaccharide is expected to be a promising treatment for MAFLD.
Platycodon grandiflorum neutral polysaccharides are active components isolated and purified from Platycodon grandiflorum (Jacq.) A.DC. (balloon flower) that have antioxidative stress and immunomodulatory activities. Song et al. (Song et al., 2023) studied mice with HFD-induced obesity and reported that Platycodon grandiflorum neutral polysaccharide treatment could reduce the Firmicutes/Bacteroides ratio and inhibit the release of LPSs into the gut by reducing the abundance of Desulfobacterota, preventing an inflammatory response and disrupting gut energy metabolism. In addition, Platycodon grandiflorum neutral polysaccharides can increase gut tight junction protein expression and ameliorate gut leakage. Although the lipid-lowering effect of Platycodon grandiflorum neutral polysaccharides has been confirmed, whether they can prevent MAFLD through the gut microbiota requires fecal microbiota transplantation (FMT) experiments.
G. lucidum polysaccharides are the main active components isolated from G. lucidum and have immunomodulatory, antioxidant, anti-inflammatory, antitumor, antiobesity and antidiabetic effects. Several studies have reported that G. lucidum polysaccharides can prevent obesity and MAFLD by regulating the gut microbiota (Sang et al., 2021). G. lucidum polysaccharides increased the relative abundances of SCFA-producing bacteria and potential probiotics, such as Allobaculum, Bifidobacterium and Christensenellaceae R-7 group, which activated the GPR43 receptor in adipose tissue to regulate metabolism and inhibit obesity. In addition, G. lucidum polysaccharides inhibited the HFD-induced inflammatory response by reducing the serum LPS concentration and inhibiting the TLR4/NF-κB signaling pathway. Therefore, G. lucidum polysaccharide may be a potential agent for targeting the gut microbiota to prevent obesity, hyperlipidemia and MAFLD.
Eucommia, isolated from the dry roots of Eucommia ulmoides Oliv., is a Chinese medicine and food homolog that has antiosteoporotic, anti-inflammatory, hypoglycemic and hypolipidemic effects. Recent studies have indicated that Eucommia leaves have similar active ingredients and antihyperlipidemic effects as Eucommia bark and can modulate the gut microbiota to alleviate lipid metabolism disorders (Wang et al., 2023a; Wang et al., 2023b). Eucommia leaf extract supplementation increased the relative abundance of Ruminococcaceae to promote the production of butyric acid, which upregulated the expression of GPR43 to reduce the area of adipocytes and lipid accumulation. Furthermore, it reduced the relative abundance of the harmful Erysipelotrichaceae species. Therefore, Eucommia leaf extract has the potential to alleviate MAFLD by targeting the gut microbiota.
Penthorum chinense Pursh. has been widely used as a traditional Chinese functional food to prevent and treat liver diseases. Numerous studies have demonstrated the therapeutic effects of P. chinense extract on MAFLD through alterations in the gut microbiota and its metabolite BAs (Li X. et al., 2022). P. chinense extract reduced the relative abundances of Clostridium IV, Clostridium XIVb, and Lactobacillus to inhibit BSH activity, inhibited BA uncoupling and dehydroxylation, and increased taurochenodeoxycholic acid (TCDCA) and tauroursodeoxycholic acid (TUDCA) levels. TCDCA and TUDCA, which are naturally occurring FXR antagonists, can lower cholesterol levels by inhibiting gut FXR activity, downregulating FGF15 expression, activating hepatic BA synthase activity, and promoting cholesterol conversion. In addition, P. chinense extract increased chenodeoxycholic acid production, which activated hepatic FXR expression and increased hepatic FXR-induced BA excretion levels, thus further promoting the conversion of cholesterol to BAs.
ZhiHeShouWu is obtained by processing Polygonum multiflorum Thunb. in black bean juice and is often used clinically to regulate lipid metabolism. Studies have shown that supplementation with ZhiHeShouWu ethanol extract could increase the relative abundances of the SCFA-producing Phascolarctobacterium species and reduce the relative abundance of Desulfovibrio (Dai et al., 2021). In addition, the ZhiHeShouWu ethanol extract affected BA metabolism by remodeling the gut microbiota, thus inhibiting the expression of gut FXR genes, accelerating cholesterol metabolism in vivo, and maintaining cholesterol homeostasis. Therefore, ZhiHeShouWu ethanol extract may improve MAFLD by regulating the gut microbiota composition and maintaining gut barrier function.
G. lucidum, used in TCM for thousands of years, has a variety of activities, such as hepatoprotective, antitumor and antiaging activities. As described above for G. lucidum, its hepatoprotective effect is associated with the regulation of the gut microbiota. The G. lucidum ethanol extract was also able to improve HFD-induced gut microbiota disorders due to its abundance of triterpenoids (Guo et al., 2018). The G. lucidum ethanol extract significantly elevated the relative abundances of Alistipes, Desulfovibrionaceae, Peptococcaceae, and Alloprevotella to increase gut propionic acid and butyric acid levels, which have been associated with positive effects on gut health, such as anti-inflammatory effects and improvement in glucose homeostasis and other metabolic symptoms. Therefore, the mechanism of action of the G. lucidum ethanol extract in improving MAFLD may involve regulation of the gut microbiota and SCFAs.
Phyllanthus emblica L. is an edible medicinal fruit used to treat hepatobiliary disorders, viral hepatitis, alcoholic hepatitis, MAFLD, and hepatocellular carcinoma. It has been shown that P. emblica aqueous extract can improve MAFLD by remodeling the gut microbiota (Luo et al., 2022). On the one hand, P. emblica aqueous extract elevated the relative abundances of Bifidobacterium and Alistipes and reduced the relative abundance of Muribaculaceae. Remodeling of the gut microbiota helped to increase SCFA levels, suppress gut inflammation and maintain gut homeostasis. On the other hand, P. emblica aqueous extracts affected choline-deficient, L-amino acid-defined, high-fat diet-induced metabolic disorders by modulating primary BA biosynthesis and taurine and hypotaurine metabolism.
Ginsenosides are the main bioactive components isolated from Panax ginseng C.A.Mey. (ginseng) and have many biological effects, such as antiobesity, antihyperglycemia and anti-MAFLD effects. Studies have shown that various isoforms of ginsenosides, such as Rg1 (Hou et al., 2020), Rg2, Rh1 (Wang F. et al., 2021), CK (Zhang J. et al., 2021), Rb2 (Huang et al., 2017), Rc (Yang Z. et al., 2020), and Rf (Chen et al., 2022), have the potential to alleviate MAFLD. Liang et al. (Liang et al., 2021) reported that ginsenoside extract treatment could modulate HFD-induced gut microbiota imbalance and attenuate ecological dysbiosis-mediated gut leakage and metabolic endotoxemia, which may be a potential mechanism of action for improving MAFLD. Ginsenoside extract supplementation elevated the relative abundances of Muribaculaceae and Akkermansia, which promoted the production of SCFAs in the gut, thereby providing energy to colonic epithelial cells and improving lipid accumulation and liver inflammation. In addition, ginsenoside extract treatment also reduced the serum LPS concentration and attenuated metabolic endotoxemia in HFD-fed mice.
Blueberries (Vaccinium spp.) are rich in polyphenols and are known for their antioxidant and cardiovascular protective properties (Emily et al., 2023). Studies have shown that blueberry extract may ameliorate HFD-induced obesity by modulating the composition of the gut microbiota (Guo et al., 2019). On the one hand, blueberry extract significantly increased the relative abundance of Akkermansia, Lactobacillus, and Bifidobacterium, which increased BSH activity, resulting in lower plasma and liver TG concentrations in mice. In addition, blueberry extract administration significantly reduced plasma taurine-coupled BAs, including tauro-α-muricholic acid (T-α-MCA) and T-β-MCA levels, to activate FXR in the liver, which enhanced SHP expression and further inhibited the activity of SREBP-1c and its downstream genes associated with lipid synthesis. On the other hand, blueberry extract reduced the abundance of Desulfovibrio and increased colonic Mucin 2 and ZO-1 levels, thereby attenuating gut permeability. In conclusion, blueberry extract may ameliorate MAFLD by modulating the gut microbiota and bile acid pool.
Eugenia jambolana Lam., commonly known as jamun, is an edible berry that has been shown to have antioxidant, anti-inflammatory and hepatoprotective activities (Chhikara et al., 2018). Xu et al. (Xu et al., 2019) found that jamun fruit extract not only ameliorated HFD diet-induced obesity, hepatic steatosis and insulin resistance but also regulated the gut microbiota and SCFA metabolism. Jamun fruit extract supplementation increased the relative abundance of Bacteroides, Prevotella and Alloprevotella, not only promoting the content of total SCFAs and acetic, propionic and butyric acids but also mitigating the imbalance in the major components of SCFAs, especially the propionic/n-butyric acid ratio. In addition, jamun fruit extract also reduced the level of Clostridium XlVb, which has been reported to be associated with cognitive impairment in patients with Parkinson’s disease (Qian et al., 2018). In conclusion, jamun fruit extract may ameliorate MAFLD by modulating the gut microbiota and SCFA production.
Myrciaria dubia (Kunth) McVaugh, commonly known as camu camu, is an Amazonian fruit rich in vitamin C and polyphenols and is considered a “superfruit” with various properties including antioxidant, antihyperglycemic and anti-obesity properties (García-Chacón et al., 2023). A recent study found that camu camu extract was able to significantly increase the number of Akkermansia muciniphila, Bifidobacterium and Barnesiella and decrease the abundance of Lactobacillus. Meanwhile, mice receiving camu camu extract feces showed the same trend, suggesting that Akkermansia muciniphila and Lactobacillus may be important drivers of increased energy expenditure and decreased weight gain. In addition, camu camu extract not only increased the levels of chenodeoxycholic acid, deoxycholic acid and ursodeoxycholic acid to activate TGR5, which enhances the response of brown adipocytes to thyroid hormones and activates nonshivering thermogenesis in this tissue, but also reduced IL-1β, IL-6 and LPS levels to prevent gut inflammation (Anhê et al., 2018). In conclusion, camu camu extract has the potential to prevent and treat MAFLD.
Psidium guajava L., also known as guava, is an edible fruit that is widely used in folk medicine as an adjunct in the treatment of diabetes (Jiao et al., 2017). It was found that polysaccharides extracted from guava have hypolipidemic effects, and the mechanism of action is highly correlated with its role in regulating the gut microbiota and metabolites (Li Y. et al., 2022). Supplementation with guava polysaccharides increased the relative abundance of Enterorhabdus and Clostridium XlVa, thereby contributing to the restoration of total SCFA levels and increased levels of acetic acid, propionic acid and butyric acid. The increase in SCFA levels was able to activate hepatic AMPKα and inhibit the expression of peroxisome proliferator-activated receptor γ, thereby suppressing HFD-induced hepatic steatosis and insulin resistance. In addition, guava polysaccharides reduced the relative abundance of Mucispirillum to alleviate TNF-α release and NF-κB signaling activation. In conclusion, guava polysaccharides are potential prebiotics with beneficial effects on obesity and MAFLD.
Morinda citrifolia L. (noni) is an evergreen tree or shrub of the Rubiaceae family, found primarily in tropical and subtropical regions. In recent years, it has been found that noni fruit polysaccharide not only has physiological activities, such as antitumor, antioxidant and anti-inflammatory activities, but also can play an antilipidemic role by regulating the gut microbiota (Yang X. et al., 2020; Mo et al., 2023). On the one hand, noni fruit polysaccharide increased the relative abundance of Eubacterium coprostanoligenes, Lactobacillus, Ruminococcaceae UCG 014, Parasutterella, and Ruminococcus 1 and decreased the relative abundance of Prevotella 9, Collinsella, and Bacteroides. The altered composition of the gut microbiota increased SCFA production and maintained colonic barrier permeability, thereby reducing serum LPS levels and hepatic lipid accumulation and ultimately ameliorating HFD-induced hepatic oxidative stress and inflammation (Yang X. et al., 2020). On the other hand, noni fruit polysaccharide also increased fecal excretion of fecal-coupled BAs, especially T-α-MCA and T-β-MCA, by modulating the structure of the gut microbiota and activating hepatic and colonic FXR receptors, thus increasing lipid oxidation and energy digestion (Mo et al., 2023). In summary, noni fruit polysaccharide modulates the gut microbiota and its metabolites, which may be its mechanism of action in alleviating MAFLD.
Polygala japonica Houtt. is a commonly used herbal medicine that has been shown to possess anti-obesity (Jee et al., 2021) and anti-inflammatory properties (Wang et al., 2007). In addition, Liao et al. (Liao et al., 2023) found that Polygala japonica Houtt. may alleviate NASH by regulating gut microbiota composition and amino acid metabolism. On the one hand, Polygala japonica Houtt. administration decreased the relative abundance of Faecalibaculum and Lachnospiraceae NK4A136 group and increased the relative abundance of Dubosiella, Turicibacter and Akkermansia, with the increase in Akkermansia modulating the decrease in fecal L-tryptophan, thereby ameliorating the disorders of tryptophan metabolism. In contrast, Polygala japonica Houtt. significantly reduced histamine levels as well as fecal and hepatic glutamate accumulation in NASH mice, suggesting that Polygala japonica Houtt. not only reduces inflammatory responses by modulating histamine levels but also ameliorates glutamate metabolism disorders. In conclusion, Polygala japonica Houtt. treatment significantly altered the composition of the gut microbiota as well as histidine and tryptophan metabolism, which may play a key role in the treatment of NASH.
Quzhou Fructus Aurantii is the dried unripe fruit of Citrus aurantium L that is used as a folk medicine and food for treating liver disease; it has hepatoprotective (Lan et al., 2023) and anti-inflammatory effects (Li L. et al., 2020). Recently, Bai et al. (Bai et al., 2019) found that the mechanism of action of Quzhou Fructus Aurantii extract in the treatment of liver disease may be related to its regulation of the gut microbiota. Quzhou Fructus Aurantii extract administration increased the relative abundance of Akkermansia and Alistipes, which may contribute to the reduction in gut permeability and LPS leakage. On the one hand, Quzhou Fructus Aurantii extract decreased LPS levels and effectively inhibited the TLR4/NF-κB signaling pathway and subsequent proinflammatory cytokine expression. On the other hand, it restored the expression of the gut tight junction proteins Claudin3 and Occludin and improved gut permeability. Furthermore, Quzhou Fructus Aurantii extract reduced the relative abundance of Dubosiella, Faecalibaculum and Lactobacillus. Thus, Quzhou Fructus Aurantii extract ameliorated MAFLD and gut inflammation, at least in part, by altering the gut microbiota.
3.3 TCM prescriptions treat MAFLD by regulating the gut microbiota
TCMs, which mainly originated in China, is used to prevent, diagnose and treat diseases or regulate human body functions under the guidance of TCM theories and has been widely used to treat liver diseases, including MAFLD. The treatment of MAFLD by TCM is based on liver protection via multiple mechanisms of action, such as antioxidative stress effects, regulation of lipid metabolism, anti-inflammation effects, anti-fibrosis effects, and regulation of the gut microbiota. Among them, TCM prescriptions have multicomponent and multitarget pharmacological effects, which are compatible with the complex pathogenesis of MAFLD and can alleviate the progression of MAFLD. In recent years, as the gut microbiota has gradually become a new target for the treatment of MAFLD, whether TCM prescriptions exert their therapeutic effects through the gut microbiota has also been gradually investigated. Among them, 12 TCM prescriptions, such as the Salvia-Nelumbinis naturalis formula and Qiang-Gan formula, have been shown to regulate gut microbiota metabolites to improve MAFLD, as shown in Table 2.
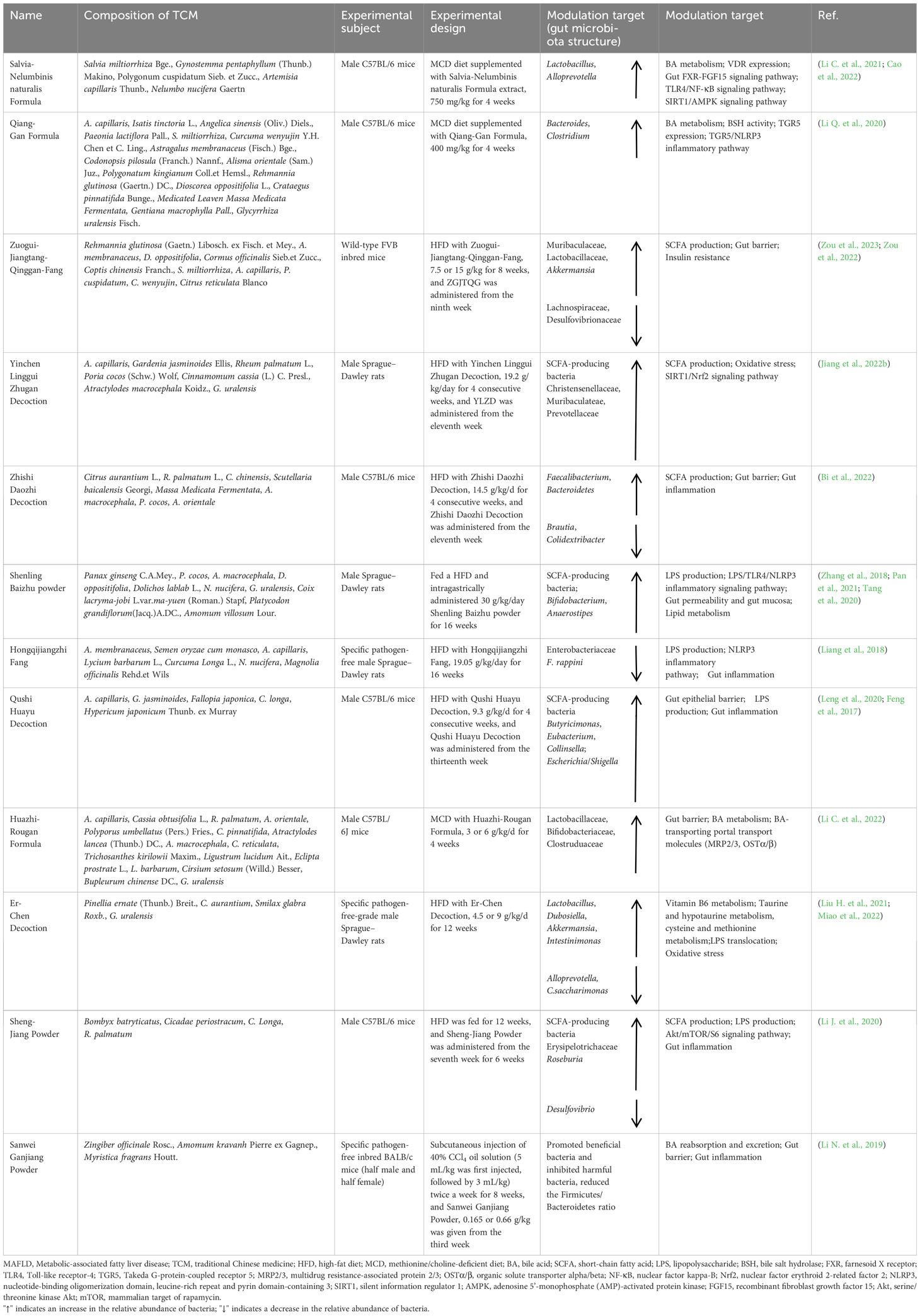
Table 2 Mechanism of action of TCM prescriptions in the treatment of MAFLD by modulating the gut microbiota and its metabolites.
The Salvia-Nelumbinis naturalis formula, initially called a lipid-lowering granule, is an herbal compound designed based on TCM theories and has been used clinically for the treatment of MAFLD and alleviation of liver steatosis with significant beneficial effects and few side effects. The alleviation of MAFLD by Salvia-Nelumbinis naturalis may be achieved by regulation of the gut microbiota and BAs (Li C. et al., 2021; Cao et al., 2022). Salvia-Nelumbinis naturalis supplementation restored the relative abundances of the beneficial bacteria Lactobacillus and Alloprevotella, increased (3α,5β,12α)-3,12-dihydroxy-24-norcholan-23-oic acid levels, and activated the FXR signaling pathway, which helped counteract LPS-induced impairment of the gut epithelial barrier and ameliorated metabolic disorders and liver disease (Li C. et al., 2021). In addition, Salvia-Nelumbinis naturalis counteracts the progression of NASH by increasing the proportion of secondary BAs and activating the endogenous vitamin D receptor (Cao et al., 2022).
The Qianggan formula is a traditional formula used in China for the treatment of liver diseases. Studies have shown that Qianggan formula treatment reverses gut microbiota disturbance and elevates the relative abundances of Bacteroides and Clostridium, which are involved in the BSH uncoupling process and therefore lead to an increase in secondary bile acid lithocholic acid (LCA) levels. In addition, the expression of TGR5 was increased in the livers of QGE-treated mice with NASH, which could be attributed to the increased LCA activation by TGR5, which ameliorated metabolic disorders and blocked nucleotide-binding oligomerization domain, leucine-rich repeat and pyrin domain-containing 3 (NLRP3) inflammasome-dependent inflammation in mice (Li Q. et al., 2020). Thus, the therapeutic effect of the Qianggan formula for MAFLD may be related to the interaction between the gut microbiota and BA metabolism.
Zuogui-Jiangtang-Qinggan-Fang, a new herbal formulation, has been clinically used to treat T2DM and fatty liver disease. Zuogui-Jiangtang-Qinggan-Fang reportedly elevated the relative abundances of Muribaculaceae and Lactobacillaceae and reduced the relative abundances of Lachnospiraceae and Desulfovibrionaceae (Zou et al., 2023). Furthermore, Zuogui-Jiangtang-Qinggan-Fang promoted the growth of the SCFA-producing bacterium Akkermansia, which repaired the gut epithelial barrier and alleviated insulin resistance by increasing the concentration of SCFAs (Zou et al., 2022). Thus, Zuogui-Jiangtang-Qinggan-Fang treatment may prevent and alleviate MAFLD by regulating the gut microbiota composition.
Yinchen Linggui Zhugan decoction is a classic combination of two well-known herbal prescriptions, namely, Linggui Zhugan and Yinchenhao decoctions, and has been used to treat hepatobiliary diseases and metabolic syndrome (Jiang et al., 2022a). Yinchen Linggui Zhugan decoction can enrich the SCFA-producing bacteria Christensenellaceae, Muribaculateae and Prevotellaceae to increase the gut SCFA content, especially Christensenellaceae, which is closely correlated with acetic acid, butyric acid and total SCFAs. The increase in butyric acid not only provides energy to gut epithelial cells but also activates the silent information regulator 1/Nrf2 signaling pathway and increases the expression of downstream antioxidant factors, which in turn ameliorates oxidative stress (Jiang et al., 2022b). These results suggest that Yinchen Linggui Zhugan decoction can alleviate MAFLD by modulating the gut microbiota and butyric acid levels.
Zhishi Daozhi decoction, a water decoction of herbs prescribed for use in Zhishi Daozhi pills, has been shown to have hepatoprotective and lipid-lowering effects. The effect of the Zhishi Daozhi decoction in the treatment of MAFLD may be achieved by modulating the gut microbiota (Bi et al., 2022). Zhishi Daozhi decoction may restore gut microbiota imbalance by promoting the growth of Faecalibacterium and Bacteroidetes and inhibiting the growth of Brautia and Colidex. The reconstituted gut microbiota also increases the amount of SCFAs in the gut, which not only reduces energy and fat deposition in the body but also provides energy to gut epithelial cells and ensures adequate expression of gut transepithelial resistance and tight junction proteins, thus improving the gut barrier and preventing gut inflammation.
Shenling Baizhu powder is a classic herb with a history of clinical use for thousands of years. Shenling Baizhu powder may alleviate MAFLD by regulating the gut microbiota. PSP not only increased the abundances of Bifidobacterium and Anaerostipes but also promoted the growth of SCFA-producing bacteria, accelerating the production of SCFAs that maintain normal gut permeability and protect the gut mucosa (Zhang et al., 2018). On the other hand, Shenling Baizhu powder can reduce LPS levels and inhibit TLR4 expression to suppress NLRP3 inflammatory vesicle activation and interleukin-1β (IL-1β) release (Pan et al., 2021). In addition, Shenling Baizhu powder can increase lipocalin levels in the liver and serum and inhibit SREBP-1c expression, thereby regulating systemic lipid metabolism and reducing hepatic lipid accumulation (Tang et al., 2020).
Hongqijiangzhi Fang is a spleen-strengthening herbal formula. Studies have shown that Hongqijiangzhi Fang can alleviate lipid metabolism disorders and reduce liver fat deposition, suggesting that it has a therapeutic effect on MAFLD. Liang et al. (Liang et al., 2018) reported that Hongqijiangzhi Fang may enhance gut barrier integrity by reducing the relative abundances of Enterobacteriaceae and F. rapa, thereby reducing LPS levels and suppressing gut inflammation. In addition, Hongqijiangzhi Fang may inhibit hepatic steatosis by reducing Enterobacteriaceae translocation and inhibiting NLRP3 inflammasome activation.
Qushi Huayu decoction is derived from Yinchenhao decoction, a classic prescription dating back to the Han Dynasty documented in the book Treatise on Cold Damage Disorders, which is one of the popular formulas for the treatment of MAFLD in China. In recent years, it has been shown that Qushi Huayu decoction can elevate the relative abundances of SCFA-producing bacteria Butyricimonas and Eubacterium to increase the SCFA content, which can provide energy to local gut epithelial cells and help maintain the gut epithelial barrier (Leng et al., 2020). Feng et al. (Feng et al., 2017) also reported that Qushi Huayu decoction treatment suppressed the HFD-induced increase in Escherichia/Shigella and elevated the relative abundance of the SCFA-producing bacteria Collinsella. In addition, Qushi Huayu decoction was able to reduce the serum LPS concentration, which may be associated with an improvement in gut inflammation. Therefore, Qushi Huayu decoction may alleviate MAFLD by repairing the gut barrier and modulating the gut microbiota composition.
The Huazhi-Rougan formula contains flavonoids, alkaloids and lactones as the main components and has been widely used to treat MAFLD and its complications. Studies have shown that Huazhi-Rougan formula treatment counteracts MCD-induced hepatic steatosis and inflammation by regulating gut ecological dysregulation and promoting fecal BA excretion (Li C. et al., 2022). The Huazhi-Rougan formula decreased the enrichment of HFD-associated bacteria and elevated the enrichment of Lactobacillaceae, Bifidobacteriaceae, and Clostridiaceae, which are involved in the uncoupling, oxidation and isomerization of BAs and contribute to the formation of secondary BAs. In addition, the Huazhi-Rougan formula alleviated gut barrier damage and alleviated MAFLD by inhibiting the expression of the intestinal BA transporter and the BA-transporting portal transport molecules multidrug resistance‐associated protein 2/3 and organic solute transporter alpha/beta, promoting fecal BA excretion and reducing secondary BA accumulation in the gut.
Er-Chen decoction, a common TCM formula originating from the Taiping Huimin Formula Bureau, is a basic herbal formula for treating dry dampness and phlegm and has been used to treat various phlegm-dampness diseases, such as MAFLD. Many studies have investigated the mechanism by which Er-Chen decoction improves lipid metabolism disorders, and Liu et al. (Liu H. et al., 2021) reported that Er-Chen decoction could reduce LPS translocation and reduce liver inflammation by reversing HFD-induced gut barrier dysfunction through regulation of the gut microbiota. Miao et al. (Miao et al., 2022) showed that Er-Chen decoction could alleviate MAFLD by inhibiting oxidative stress, reducing inflammatory responses, ameliorating gut microbiota dysbiosis, and increasing pyridoxal (vitamin B6) levels. The increase in pyridoxal concentrations not only inhibited lipid synthesis but also reduced oxidative and inflammatory stress. Thus, the effect of Er-Chen decoction on MAFLD may be achieved through the gut-liver axis pathway involving the gut microbiota.
Sheng-Jiang powder, derived from the “wan bing hui chun” compiled by Ting-Xian Gong of the Ming Dynasty, is a typical herbal formula for restoring the “ascending and descending abnormalities” of the spleen qi and has been used to treat MAFLD. Sheng-Jiang powder supplementation increased the relative abundances of the SCFA-producing bacteria Erysipelotrichaceae and Roseburia and decreased the relative abundance of the harmful bacteria Desulfovibrio (Li J. et al., 2020). These findings suggest that Sheng-Jiang powder may alleviate gut leakage by increasing SCFA levels to ameliorate gut barrier damage and may also ameliorate gut inflammation by inhibiting LPS production or entry into the gut. Thus, the protective effect of Sheng-Jiang powder against HFD-induced MAFLD may be partially attributed to its regulation of the gut microbiota.
Sanwei Ganjiang powder, also known as Jia Ga Song Tang, not only has antipyretic and antidiarrheal effects and promotes gas circulation but also has anti-inflammatory effects, protects the gastrointestinal mucosa, and enhances antioxidant and hepatoprotective pharmacological effects. Li et al. (Li N. et al., 2019) reported that Sanwei Ganjiang powder could alleviate liver injury by regulating the gut microbiota and restoring BA homeostasis. Sanwei Ganjiang powder may regulate the Firmicutes/Bacteroidetes ratio, promote beneficial bacteria and inhibit harmful bacteria. Its modifying effects on the gut microbiota lead to upregulation of the expression of specific BA metabolic enzymes, as well as reabsorption and efflux transporters, thus promoting the detoxification, reabsorption and excretion of TBAs and reversing disrupted BA homeostasis. In addition, Sanwei Ganjiang powder increased the expression of gut tight junction proteins such as ZO-1 and Occludin and repaired the gut barrier to inhibit LPS-induced gut inflammation. Therefore, treatment with Sanwei Ganjiang powder may be a potential strategy for treating MAFLD by maintaining the gut-liver axis.
4 Perspective
MAFLD has become the most common chronic liver disease in the world, and its incidence is increasing annually (Lin et al., 2021). However, due to the complexity of the pathogenesis of MAFLD, pathogenic processes such as insulin resistance, lipotoxicity, oxidative stress, altered immune or cytokine or mitochondrial function, and apoptosis, are collectively involved in the development and progression of MAFLD. At the same time, the pathogenetic process of MAFLD is often accompanied by a variety of complications; therefore, drug therapy needs to simultaneously account for the prevention and treatment of hepatic steatosis, inflammation, hepatocellular injury and fibrosis, as well as the treatment of complications, which is very difficult. Second, NASH requires a liver biopsy to determine if the patient has NASH and what stage of liver fibrosis the patient is currently in. However, liver biopsies are invasive and not easily accepted by patients. In clinical practice, it is not very feasible to repeat liver biopsies to assess whether liver injury and liver fibrosis have improved. While it is feasible that current research on NASH drug therapy focuses on improvements in MAFLD activity scores, the scores are subjective. Therefore, there is an urgent need to develop and validate a set of noninvasive methods for assessing NASH fibrosis and documenting its progression or reversal to help enhance accelerated new drug development for MAFLD and NASH. For these and other reasons, there are currently no FDA-approved therapeutic drugs. Therefore, it is essential to find safe and effective anti-MAFLD drugs. In recent years, the relationship between the gut microbiota and metabolic diseases has been widely recognized (Boulangé et al., 2016). An imbalance in the gut microbiome exacerbates disease progression, and targeted modulation of the gut microbiome has emerged as a viable strategy for the prevention and treatment of MAFLD. Plant natural products involve multiple pathways, multiple targets and few side effects. Plant natural products exert their effects in the prevention and treatment of MAFLD not only by regulating lipogenic pathways, downregulating inflammatory pathways, improving insulin sensitivity, and ameliorating oxidative stress but also by regulating the gut microbiota and its metabolites, for example, via the gut-liver axis (Wang L. et al., 2023).
Modern pharmacokinetic studies have shown that most plant natural products are difficult to absorb or have poor absorption rates. Berberine, for example, is less than 1% orally available, but it has been shown to have therapeutic activity against a variety of diseases. This may be because berberine can be converted to dihydroberberine by enzymes produced by the gut microbiota, which increases its absorption and subsequently utilization. In addition, some “zero absorption” natural ingredients whose main function may be to provide nutrients for the gut microbiota, improve the activity of the gut microbiota and exert their effects through the release of active molecules by the gut microbiota. The contribution and function of the gut microbiota through biotransformation of the components of plant natural products or through enhancement of the activity of the gut microbiota cannot be ignored. Therefore, targeting the gut microbiota for the treatment of MAFLD may be more conducive to ensuring the efficacy of plant natural products in controlling the development of this disease.
Recent research on the relationships among the gut microbiota, gut permeability and metabolic disorders has provided new perspectives on the treatment of MAFLD. In the clinical application of plant natural products for MAFLD treatment, many patients are treated based on the principle of “strengthening the spleen” from the perspective of “spleen deficiency”, which is often accompanied by the improvement of gut symptoms such as diarrhea and defecation. Numerous studies have shown that plant natural products can support the growth of beneficial bacteria in the gut, inhibit the growth of harmful bacteria, maintain the balance of beneficial and harmful bacteria, and improve gut permeability. The study of “gut-liver” axis interactions provides a reference and method for the treatment of MAFLD by plant natural products and the gut microbiota, which allows exploration of the pharmacological mechanism of plant natural products in the treatment of MAFLD from the perspective of gut microenvironment and gut barrier regulation.
Although the gut microbiome is a hot topic and a new entry point for studying the mechanism of action of plant natural products, the mechanism cannot be simply attributed to alterations in the gut microbiome. Alterations in the gut microbiota may be one result of plant natural product interventions in the organism, but not all the pathways involved in these effects are known, and additional experimental evidence needs to be obtained. Based on the above, in addition to using 16S ribosomal DNA identification to determine the changes in the gut microbiota, we need to further explore the role of the gut microbiota in the efficacy of plant natural products through FMT, testing with germ-free mice, antibiotic intervention and other methods.
In addition, the changes in the gut microbiota are unstable. The gut microbiota is easily affected by the external environment. Although the self-repair ability of the gut microbiota can restore its balance after short-term disturbance, long-term disturbance often causes gut microbiota disorders; that is, differences in experimental environments and conditions may lead to changes in the gut microbiota. Therefore, it is particularly important and more convincing to identify stable and changing bacterial species, such as pathogenic bacteria or beneficial bacteria, through the instability of the gut microbiota. Crossomics data need to be integrated and analyzed with data from additional high-throughput omics technologies, such as metagenomics, metabolomics, and bioinformatics. From the perspective of the composition and function of the gut microbiota and the interaction between the gut microbiota and host metabolism, key strains and their related metabolites will need to be explored in depth.
Numerous plant natural compounds, such as berberine, resveratrol, curcumin, and puerarin, have been found to alleviate MAFLD. However, TCM prescriptions often achieve better therapeutic effects and have the characteristics and advantages associated with the holistic use of Chinese medicine in the treatment of complex diseases. TCM prescriptions are safe and effective modern Chinese medicines with a clear mechanism of action formed on the basis of TCM enhanced by modernization and pharmacological research and represent one of the future development directions of Chinese medicine. However, there are some limitations in the current development of plant natural products for the treatment of MAFLD. One of the limitations is that the mechanisms of action of plant natural products are complex, and it is difficult to clarify the specific mechanisms of action. Second, the limitation of plant natural products emphasizes the influence of the place of origin on their quality and efficacy. Authentic herbs not only have unique environmental conditions in terms of their origin but also are subjected to specific natural factors and artificial management during their growth process, which makes them of superior quality. In conclusion, the interaction between plant natural products and the gut microbiota provides a safe, effective, and feasible therapeutic approach and modality for treating MAFLD.
5 Conclusion
This narrative review systematically summarizes the effects of plant natural products on the prevention of MAFLD by regulating the gut microbiota and its metabolite-related pathways, which provides feasible ideas for further exploring safer and more effective plant natural products as drugs for the prevention and treatment of MAFLD. Moreover, on the basis of this review, it is necessary to strengthen the study of the interactions among plant natural products, the gut microbiota and the human body. In the future, more attention should be given to the role of the gut microbiota in the pharmacodynamic effect of Chinese medicine and the contribution of the effects of the gut microbiota on host metabolism to the development of disease or pharmacodynamic effects.
Author contributions
TC: Writing – original draft, Writing – review & editing. XS: Writing – original draft, Writing – review & editing. XX: Software, Visualization, Writing – review & editing. LD: Software, Visualization, Writing – review & editing. SL: Software, Visualization, Writing – review & editing. MX: Software, Visualization, Writing – review & editing. YH: Software, Visualization, Writing – review & editing. LZ: Investigation, Resources, Writing – review & editing. TL: Investigation, Resources, Writing – review & editing. XW: Investigation, Resources, Writing – review & editing. YF: Investigation, Resources, Writing – review & editing. ZX: Conceptualization, Writing – review & editing. CW: Conceptualization, Writing – review & editing. MW: Conceptualization, Writing – review & editing. JL: Conceptualization, Writing – review & editing. YZ: Project administration, Supervision, Writing – review & editing. WS: Conceptualization, Investigation, Project administration, Supervision, Writing – review & editing. LL: Project administration, Supervision, Writing – review & editing.
Funding
The author(s) declare financial support was received for the research, authorship, and/or publication of this article. This work was supported by the University Youth Innovation Team of Shandong Province (2022KJ229), the Natural Science Foundation of Shandong Province (ZR2022MH306 and ZR2020QB004), the National Natural Science Foundation of China (82104112and 82374308), the China Postdoctoral Science Foundation (2023M733918), the Beijing Science and Technology New Star Project (Z201100000820027), the National Key Research and Development Program of China (2022YFC2010104), the Key Research and Development Program of Shandong Province (2021SFGC1205), the State Key Program of National Natural Science Foundation of China (81730112), and High level Key Discipline of National Administration of Traditional Chinese Medicine-Traditional Chinese constitutional medicine (No.zyyzdxk-2023251).
Conflict of interest
The authors declare that the research was conducted in the absence of any commercial or financial relationships that could be construed as a potential conflict of interest.
Publisher’s note
All claims expressed in this article are solely those of the authors and do not necessarily represent those of their affiliated organizations, or those of the publisher, the editors and the reviewers. Any product that may be evaluated in this article, or claim that may be made by its manufacturer, is not guaranteed or endorsed by the publisher.
Glossary
References
Ahmed, O. M., Hassan, M. A., Abdel-Twab, S. M., Abdel Azeem, M. N. (2017). Navel orange peel hydroethanolic extract, naringin and naringenin have anti-diabetic potentials in type 2 diabetic rats. Biomedicine Pharmacotherapy 94, 197–205. doi: 10.1016/j.biopha.2017.07.094
Anekwe, C. V., Chandrasekaran, P., Stanford, F. C. (2020). Ketogenic diet-induced elevated cholesterol, elevated liver enzymes and potential non-alcoholic fatty liver disease. Cureus 12 (1), e6605. doi: 10.7759/cureus.6605
Anhê, F. F., Nachbar, R. T., Varin, T. V., Trottier, J., Dudonné, S., Le Barz, M., et al. (2018). Treatment with camu camu (Myrciaria dubia) prevents obesity by altering the gut microbiota and increasing energy expenditure in diet-induced obese mice. Gut 68 (3), 453–464. doi: 10.1136/gutjnl-2017-315565
Bäckhed, F., Ding, H., Wang, T., Hooper, L. V., Koh, G. Y., Nagy, A., et al. (2004). The gut microbiota as an environmental factor that regulates fat storage. Proc. Natl. Acad. Sci. U. S. A. 101 (44), 15718–15723. doi: 10.1073/pnas.0407076101
Bai, Y.-F., Wang, S. W., Wang, X. X., Weng, Y. Y., Fan, X. Y., Sheng, H., et al. (2019). The flavonoid-rich Quzhou Fructus Aurantii extract modulates gut microbiota and prevents obesity in high-fat diet-fed mice. Nutr. Diabetes 9 (1), 30. doi: 10.1038/s41387-019-0097-6
Barchetta, I., Cimini, F., Cavallo, M. (2017). Vitamin D supplementation and non-alcoholic fatty liver disease: present and future. Nutrients 9 (9), 1015. doi: 10.3390/nu9091015
Barchetta, I., Cimini, F. A., Cavallo, M. G. (2020). Vitamin D and metabolic dysfunction-associated fatty liver disease (MAFLD): an update. Nutrients 12 (11), 3302. doi: 10.3390/nu12113302
Barchetta, I., Angelico, F., Del Ben, M., Baroni, M. G., Pozzilli, P., Morini, S., et al. (2011). Strong association between non alcoholic fatty liver disease (NAFLD) and low 25(OH) vitamin D levels in an adult population with normal serum liver enzymes. BMC Med. 9, 85. doi: 10.1186/1741-7015-9-85
Bertani, B., Ruiz, N. (2018). Function and biogenesis of lipopolysaccharides. EcoSal Plus 8 (1), 10.1128/ecosalplus.ESP-0001-2018. doi: 10.1128/ecosalplus.esp-0001-2018
Bi, C. R., Sun, J. T., Du, J., Chu, L. Y., Li, Y. J., Jia, X. Y., et al. (2022). Effects of Zhishi Daozhi Decoction on the intestinal flora of nonalcoholic fatty liver disease mice induced by a high-fat diet. Front. Cell Infect. Microbiol. 12, 1005318. doi: 10.3389/fcimb.2022.1005318
Boulangé, C. L., Neves, A. L., Chilloux, J., Nicholson, J. K., Dumas, M.-E. (2016). Impact of the gut microbiota on inflammation, obesity, and metabolic disease. Genome Med. 8 (1), 42. doi: 10.1186/s13073-016-0303-2
Boursier, J., Mueller, O., Barret, M., Machado, M., Fizanne, L., Araujo-Perez, F., et al. (2016). The severity of nonalcoholic fatty liver disease is associated with gut dysbiosis and shift in the metabolic function of the gut microbiota. Hepatology 63 (3), 764–775. doi: 10.1002/hep.28356
Brennan, C. (2006). Vitamins: their role in the human body. Int. J. Food Sci. Technol 41 (2), 221–222. doi: 10.1111/j.1365-2621.2005.01015.x
Brighton, C. A., Rievaj, J., Kuhre, R. E., Glass, L. L., Schoonjans, K., Holst, J. J., et al. (2015). Bile acids trigger GLP-1 release predominantly by accessing basolaterally located G protein-coupled bile acid receptors. Endocrinology 156 (11), 3961–3970. doi: 10.1210/en.2015-1321
Brown, A. J., Goldsworthy, S. M., Barnes, A. A., Eilert, M. M., Tcheang, L., Daniels, D., et al. (2003). The Orphan G protein-coupled receptors GPR41 and GPR43 are activated by propionate and other short chain carboxylic acids. J. Biol. Chem 278 (13), 11312–11319. doi: 10.1074/jbc.m211609200
Candelli, M., Franza, L., Pignataro, G., Ojetti, V., Covino, M., Piccioni, A., et al. (2021). Interaction between lipopolysaccharide and gut microbiota in inflammatory bowel diseases. Int. J. Mol. Sci. 22 (12), 6242. doi: 10.3390/ijms22126242
Cao, Y., Shu, X., Li, M., Yu, S., Li, C., Ji, G., et al. (2022). Jiangzhi granule attenuates non-alcoholic steatohepatitis through modulating bile acid in mice fed high-fat vitamin D deficiency diet. Biomedicine Pharmacotherapy 149, 112825. doi: 10.1016/j.biopha.2022.112825
Carr, R. M., Reid, A. E. (2015). FXR agonists as therapeutic agents for non-alcoholic fatty liver disease. Curr. Atheroscl. Rep 17 (4), 500. doi: 10.1007/s11883-015-0500-2
Chen, L., Liu, J., Mei, G., Chen, H., Peng, S., Zhao, Y., et al. (2021). Quercetin and non-alcoholic fatty liver disease: A review based on experimental data and bioinformatic analysis. Food Chem. Toxicol. 154, 112314. doi: 10.1016/j.fct.2021.112314
Chen, L., Wang, L., Ao, W., Chen, Y., Li, S., Huang, Z., et al. (2022). Bioinformatics study of the potential therapeutic effects of ginsenoside Rf in reversing nonalcoholic fatty liver disease. Biomedicine Pharmacotherapy 149, 112879. doi: 10.1016/j.biopha.2022.112879
Chen, J., Vitetta, L. (2020). Gut microbiota metabolites in NAFLD pathogenesis and therapeutic implications. Int. J. Mol. Sci. 12 (4), 541–555. doi: 10.3390/ijms21155214
Cheng, H., Liu, J., Tan, Y., Feng, W., Peng, C. (2021). Interactions between gut microbiota and berberine, a necessary procedure to understand the mechanisms of berberine. J. Pharm. Anal. 12 (4), 541–555. doi: 10.1016/j.jpha.2021.10.003
Cheng, Z., Zhang, L., Yang, L., Chu, H. (2022). The critical role of gut microbiota in obesity. Front. Endocrinol. 13, 1025706. doi: 10.3389/fendo.2022.1025706
Chhikara, N., Kaur, R., Jaglan, S., Sharma, P., Gat, Y., Panghal, A. (2018). Bioactive compounds and pharmacological and food applications of Syzygium cumini – a review. Food Funct. 9 (12), 6096–6115. doi: 10.1039/c8fo00654g
Chiang, J. Y. (2013). Bile acid metabolism and signaling. Compr. Physiol. 3, 1191–1212. doi: 10.1002/cphy.c120023
Chun, R. F., Shieh, A., Gottlieb, C., Yacoubian, V., Wang, J., Hewison, M., et al. (2019). Vitamin D binding protein and the biological activity of vitamin D. Front. Endocrinol. 10, 718. doi: 10.3389/fendo.2019.00718
Chung, G. E., Kim, D., Kwak, M. S., Yang, J. I., Yim, J. Y., Lim, S. H., et al. (2016). The serum vitamin D level is inversely correlated with nonalcoholic fatty liver disease. Clin. Mol. Hepatol 22 (1), 146–151. doi: 10.3350/cmh.2016.22.1.146
Ciardullo, S., Muraca, E., Cannistraci, R., Perra, S., Lattuada, G., Perseghin, G. (2023). Low 25 (OH) vitamin D levels are associated with increased prevalence of nonalcoholic fatty liver disease and significant liver fibrosis. Diabetes/Metabolism Res. Rev 39 (5), e3628. doi: 10.1002/dmrr.3628
Csak, T., Velayudham, A., Hritz, I., Petrasek, J., Levin, I., Lippai, D., et al. (2011). Deficiency in myeloid differentiation factor-2 and toll-like receptor 4 expression attenuates nonalcoholic steatohepatitis and fibrosis in mice. Am. J. Physiology-Gastrointestinal Liver Physiol. 300 (3), G433–G441. doi: 10.1152/ajpgi.00163.2009
Dai, X., He, L., Hu, N., Guo, C., Zhou, M., Zhao, X., et al. (2021). Polygoni multiflori radix praeparata ethanol extract exerts a protective effect against high-fat diet induced non-alcoholic fatty liver disease in mice by remodeling intestinal microbial structure and maintaining metabolic homeostasis of bile acids. Front. Pharmacol. 12, 734670. doi: 10.3389/fphar.2021.734670
Dapito, D. H., Mencin, A., Gwak, G. Y., Pradere, J. P., Jang, M. K., Mederacke, I., et al. (2012). Promotion of hepatocellular carcinoma by the intestinal microbiota and TLR4. Cancer Cell 21 (4), 504–516. doi: 10.1016/j.ccr.2012.02.007
Di Ciaula, A., Garruti, G., Lunardi Baccetto, R., Molina-Molina, E., Bonfrate, L., Wang, D. Q., et al. (2017). Bile acid physiology. Ann. Hepatol 16 (Suppl. 1: s3-105.), s4–s14. doi: 10.5604/01.3001.0010.5493
Du, J., Zhang, P., Luo, J., Shen, L., Zhang, S., Gu, H., et al. (2021). Dietary betaine prevents obesity through gut microbiota-drived microRNA-378a family. Gut Microbes 13 (1), 1–19. doi: 10.1080/19490976.2020.1862612
Du, T., Xiang, L., Zhang, J., Yang, C., Zhao, W., Li, J., et al. (2023). Vitamin D improves hepatic steatosis in NAFLD via regulation of fatty acid uptake and β-oxidation. Front. Endocrinol. 14, 1138078. doi: 10.3389/fendo.2023.1138078
Duan, R., Huang, K., Guan, X., Li, S., Xia, J., Shen, M., et al. (2022). Tectorigenin ameliorated high-fat diet-induced nonalcoholic fatty liver disease through anti-inflammation and modulating gut microbiota in mice. Food Chem. Toxicol. 164, 112948. doi: 10.1016/j.fct.2022.112948
Emily, K. W., Sylvia, Y. L., Nancy, G., Allegra, R. V., Sarah, A. J. (2023). Protective effects of blueberries on vascular function: A narrative review of preclinical and clinical evidence. Nutr. Res. 120, 20–57. doi: 10.1016/j.nutres.2023.09.007
Eslam, M., Newsome, P. N., Sarin, S. K., Anstee, Q. M., Targher, G., Romero-Gomez, M., et al. (2020). A new definition for metabolic associated fatty liver disease: an international expert consensus statement. J. Hepatol 73 (1), 202–209. doi: 10.1016/j.jhep.2020.03.039
Fan, J., Sun, J., Li, T., Yan, X., Jiang, Y. (2022). Nuciferine prevents hepatic steatosis associated with improving intestinal mucosal integrity, mucus-related microbiota and inhibiting TLR4/MyD88/NF-κB pathway in high-fat induced rats. J. Funct. Foods 88 (3), 104859. doi: 10.1016/j.jff.2021.104859
Federico, A., Dallio, M., Loguercio, C. (2017). Silymarin/silybin and chronic liver disease: A marriage of many years. Molecules 22 (2), 191. doi: 10.3390/molecules22020191
Fei, N., Zhao, L. (2013). An opportunistic pathogen isolated from the gut of an obese human causes obesity in germfree mice. ISME J. 7 (4), 880–884. doi: 10.1038/ismej.2012.153
Feng, Q., Liu, W., Baker, S. S., Li, H., Chen, C., Liu, Q., et al. (2017). Multi-targeting therapeutic mechanisms of the Chinese herbal medicine QHD in the treatment of non-alcoholic fatty liver disease. Oncotarget 8 (17), 27820–27838. doi: 10.18632/oncotarget.15482
Ferslew, B. C., Xie, G., Johnston, C. K., Su, M., Stewart, P. W., Jia, W., et al. (2015). Altered bile acid metabolome in patients with nonalcoholic steatohepatitis. Digestive Dis. Sci. 60 (11), 3318–3328. doi: 10.1007/s10620-015-3776-8
Flores-Guerrero, J. L., Post, A., van Dijk, P. R., Connelly, M. A., Garcia, E., Navis, G., et al. (2021). Circulating trimethylamine-N-oxide is associated with all-cause mortality in subjects with nonalcoholic fatty liver disease. Liver Int 41 (10), 2371–2382. doi: 10.1111/liv.14963
Gao, L.-L., Ma, J. M., Fan, Y. N., Zhang, Y. N., Ge, R., Tao, X. J., et al. (2021). Lycium barbarum polysaccharide combined with aerobic exercise ameliorated nonalcoholic fatty liver disease through restoring gut microbiota, intestinal barrier and inhibiting hepatic inflammation. Int. J. Biol. Macromolecules 183, 1379–1392. doi: 10.1016/j.ijbiomac.2021.05.066
García-Chacón, J. M., Marín-Loaiza, J. C., Osorio, C. (2023). Camu camu (Myrciaria dubia (Kunth) mcVaugh): an amazonian fruit with biofunctional properties–A review. ACS Omega 8 (6), 5169–5183. doi: 10.1021/acsomega.2c07245
Gart, E., van Duyvenvoorde, W., Toet, K., Caspers, M. P.M., Verschuren, L., Nielsen, M. J., et al. (2021). Butyrate protects against diet-induced NASH and liver fibrosis and suppresses specific non-canonical TGF-beta signaling pathways in human hepatic stellate cells. Biomedicines 9 (12), 1954. doi: 10.3390/biomedicines9121954
Ge, H., Li, X., Weiszmann, J., Wang, P., Baribault, H., Chen, J. L., et al. (2008). Activation of G protein-coupled receptor 43 in adipocytes leads to inhibition of lipolysis and suppression of plasma free fatty acids. Endocrinology 149 (9), 4519–4526. doi: 10.1210/en.2008-0059
Gong, T., Wang, H., Liu, S., Zhang, M., Xie, Y., Liu, X. (2022). Capsaicin regulates lipid metabolism through modulation of bile acid/gut microbiota metabolism in high-fat-fed SD rats. Food Nutr. Res. 66, 10.29219/fnr.v66.8289. doi: 10.29219/fnr.v66.8289
Gong, M. J., Zhu, C. Y., Zou, Z. J., Han, B., Huang, P. (2021). Therapeutic potential of puerarin against methionine-choline-deficient diet-induced non-alcoholic steatohepatitis determined by combination of (1)H NMR spectroscopy-based metabonomics and 16S rRNA gene sequencing. J. Pharm. BioMed. Anal. 197, 113964. doi: 10.1016/j.jpba.2021.113964
Goodwin, B., Jones, S. A., Price, R. R., Watson, M. A., McKee, D. D., Moore, L. B., et al. (2000). A regulatory cascade of the nuclear receptors FXR, SHP-1, and LRH-1 represses bile acid biosynthesis. Mol. Cell 6 (3), 517–526. doi: 10.1016/s1097-2765(00)00051-4
Guo, W. L., Pan, Y. Y., Li, L., Li, T. T., Liu, B., Lv, X. C. (2018). Ethanol extract of Ganoderma lucidum ameliorates lipid metabolic disorders and modulates the gut microbiota composition in high-fat diet fed rats. Food Funct. 9, 3419–3431. doi: 10.1039/c8fo00836a
Guo, J., Han, X., Tan, H., Huang, W., You, Y., Zhan, J. (2019). Blueberry Extract Improves Obesity through Regulation of the Gut Microbiota and Bile Acids via Pathways Involving FXR and TGR5. iScience 19, 676–690. doi: 10.1016/j.isci.2019.08.020
Guo, W. L., Guo, J. B., Liu, B. Y., Lu, J. Q., Chen, M., Liu, B., et al. (2020). Ganoderic acid A from Ganoderma lucidum ameliorates lipid metabolism and alters gut microbiota composition in hyperlipidemic mice fed a high-fat diet. Food Funct. 11, 6818–6833. doi: 10.1039/d0fo00436g
Hao, W., Kwek, E., He, Z., Zhu, H., Liu, J., Zhao, Y., et al. (2020). Ursolic acid alleviates hypercholesterolemia and modulates the gut microbiota in hamsters. Food Funct. 11, 6091–6103. doi: 10.1039/d0fo00829j
Hegazy, M. A., Mogawer, S. M., Alnaggar, A. R. L. R., Ghoniem, O. A., Abdel Samie, R. M. (2020). Serum LPS and CD163 biomarkers confirming the role of gut dysbiosis in overweight patients with NASH. Diabetes Metab. Syndrome Obesity: Targets Ther. 13, 3861–3872. doi: 10.2147/dmso.s249949
Hill, M. J. (1997). Intestinal flora and endogenous vitamin synthesis. Eur. J. Cancer Prev. 6 (Suppl 1), S43–S45. doi: 10.1097/00008469-199703001-00009
Hong, Y.-H., Nishimura, Y., Hishikawa, D., Tsuzuki, H., Miyahara, H., Gotoh, C., et al. (2005). Acetate and propionate short chain fatty acids stimulate adipogenesis via GPCR43. Endocrinology 146 (12), 5092–5099. doi: 10.1210/en.2005-0545
Hong, Y., Sheng, L., Zhong, J., Tao, X., Zhu, W., Ma, J., et al. (2021). Desulfovibrio vulgaris, a potent acetic acid-producing bacterium, attenuates nonalcoholic fatty liver disease in mice. Gut Microbes 13 (1), 1–20. doi: 10.1080/19490976.2021.1930874
Hou, Y., Gu, D., Peng, J., Jiang, K., Li, Z., Shi, J., et al. (2020). Ginsenoside rg1 regulates liver lipid factor metabolism in NAFLD model rats. ACS Omega 5 (19), 10878–10890. doi: 10.1021/acsomega.0c00529
Hrncir, T., Hrncirova, L., Kverka, M., Hromadka, R., Machova, V., Trckova, E., et al. (2021). Gut microbiota and NAFLD: pathogenetic mechanisms, microbiota signatures, and therapeutic interventions. Microorganisms 9 (5), 957. doi: 10.3390/microorganisms9050957
Hu, Q., Zhang, W., Wu, Z., Tian, X., Xiang, J., Li, L., et al. (2021). Baicalin and the liver-gut system: Pharmacological bases explaining its therapeutic effects. Pharmacol. Res. 165, 105444. doi: 10.1016/j.phrs.2021.105444
Huang, Q., Wang, T., Yang, L., Wang, H.-Y. (2017). Ginsenoside rb2 alleviates hepatic lipid accumulation by restoring autophagy via induction of sirt1 and activation of AMPK. Int. J. Mol. Sci. 18 (5), 1063. doi: 10.3390/ijms18051063
Jadhav, K., Xu, Y., Xu, Y., Li, Y., Xu, J., Zhu, Y., et al. (2018). Reversal of metabolic disorders by pharmacological activation of bile acid receptors TGR5 and FXR. Mol. Metab. 9, 131–140. doi: 10.1016/j.molmet.2018.01.005
Jee, W., Lee, S. H., Ko, H. M., Jung, J. H., Chung, W. S., Jang, H. J. (2021). Anti-Obesity Effect of Polygalin C Isolated from Polygala japonica Houtt. via Suppression of the Adipogenic and Lipogenic Factors in 3T3-L1 Adipocytes. Int. J. Mol. Sci. 22 (19), 10405. doi: 10.3390/ijms221910405
Jiang, H., Mao, T., Liu, Y., Tan, X., Sun, Z., Cheng, Y., et al. (2022a). Protective effects and mechanisms of yinchen linggui zhugan decoction in HFD-induced nonalcoholic fatty liver disease rats based on network pharmacology and experimental verification. Front. Pharmacol. 13, 908128. doi: 10.3389/fphar.2022.908128
Jiang, H., Mao, T., Sun, Z., Shi, L., Han, X., Zhang, Y., et al. (2022b). Yinchen Linggui Zhugan decoction ameliorates high fat diet-induced nonalcoholic fatty liver disease by modulation of SIRT1/Nrf2 signaling pathway and gut microbiota. Front. Microbiol. 13, 1001778. doi: 10.3389/fmicb.2022.1001778
Jiao, Y., Zhang, M., Wang, S., Yan, C. (2017). Consumption of guava may have beneficial effects in type 2 diabetes: A bioactive perspective. Int. J. Biol. Macromolecules 101, 543–552. doi: 10.1016/j.ijbiomac.2017.03.130
Jin, C. J., Sellmann, C., Engstler, A. J., Ziegenhardt, D., Bergheim, I. (2015). Supplementation of sodium butyrate protects mice from the development of non-alcoholic steatohepatitis (NASH). Br. J. Nutr. 114, 1745–1755. doi: 10.1017/S0007114515003621
Ju, M., Liu, Y., Li, M., Cheng, M., Zhang, Y., Deng, G., et al. (2019). Baicalin improves intestinal microecology and abnormal metabolism induced by high-fat diet. Eur. J. Pharmacol. 857, 172457. doi: 10.1016/j.ejphar.2019.172457
Kalhan, S. C., Guo, L., Edmison, J., Dasarathy, S., McCullough, A. J., Hanson, R. W., et al. (2010). Plasma metabolomic profile in nonalcoholic fatty liver disease. Metabolism 60 (3), 404–413. doi: 10.1016/j.metabol.2010.03.006
Kim, Y.-J., Yoon, D. S., Jung, U. J. (2021). Efficacy of nobiletin in improving hypercholesterolemia and nonalcoholic fatty liver disease in high-cholesterol diet-fed mice. Nutr. Res. Pract 15 (4), 431–443. doi: 10.4162/nrp.2021.15.4.431
Kuo, C. F., Yu, K. H., Luo, S. F., Chiu, C. T., Ko, Y. S., Hwang, J. S., et al. (2010). Gout and risk of non-alcoholic fatty liver disease. Scand. J. Rheumatol 39, 466–471. doi: 10.3109/03009741003742797
Kuziel, G. A., Rakoff-Nahoum, S. (2022). The gut microbiome. Curr. Biol 32 (6), R257–R264. doi: 10.1016/j.cub.2022.02.023
Lan, T., Wang, W., Huang, D. L., Zeng, X. X., Wang, X. X., Wang, J., et al. (2023). Essential oil extracted from Quzhou Aurantii Fructus prevents acute liver failure through inhibiting lipopolysaccharide-mediated inflammatory response. Natural Products Bioprospecting 13 (1), 36. doi: 10.1007/s13659-023-00398-9
Lebeaupin, C., Vallée, D., Hazari, Y., Hetz, C., Chevet, E., Bailly-Maitre, B. (2018). Endoplasmic Reticulum stress signaling and the pathogenesis of Non-Alcoholic Fatty Liver Disease. J. Hepatol 69 (4), 927–947. doi: 10.1016/j.jhep.2018.06.008
Lee, Y.-S., Cha, B. Y., Saito, K., Yamakawa, H., Choi, S. S., Yamaguchi, K., et al. (2010). Nobiletin improves hyperglycemia and insulin resistance in obese diabetic ob/ob mice. Biochem. Pharmacol. 79 (11), 1674–1683. doi: 10.1016/j.bcp.2010.01.034
Lee, Y.-S., Cha, B. Y., Choi, S. S., Choi, B. K., Yonezawa, T., Teruya, T., et al. (2012). Nobiletin improves obesity and insulin resistance in high-fat diet-induced obese mice. J. Nutr. Biochem. 24 (1), 156–162. doi: 10.1016/j.jnutbio.2012.03.014
Lee, S.-W., Lee, Y. J., Baek, S. M., Kang, K. K., Kim, T. U., Yim, J. H., et al. (2022). Mega-dose vitamin C ameliorates nonalcoholic fatty liver disease in a mouse fast-food diet model. Nutrients 14 (11), 2195. doi: 10.3390/nu14112195
Leng, J., Huang, F., Hai, Y., Tian, H., Liu, W., Fang, Y., et al. (2020). Amelioration of non-alcoholic steatohepatitis by Qushi Huayu decoction is associated with inhibition of the intestinal mitogen-activated protein kinase pathway. Phytomedicine 66, 153135. doi: 10.1016/j.phymed.2019.153135
León-Mimila, P., Villamil-Ramírez, H., Li, X. S., Shih, D. M., Hui, S. T., Ocampo-Medina, E., et al. (2020). Trimethylamine N-Oxide levels are associated with NASH in obese subjects with type 2 diabetes. Diabetes Metab. 47 (2), 101183. doi: 10.1016/j.diabet.2020.07.010
Le Roy, T., Llopis, M., Lepage, P., Bruneau, A., Rabot, S., Bevilacqua, C., et al. (2012). Intestinal microbiota determines development of non-alcoholic fatty liver disease in mice. Gut 62 (12), 1787–1794. doi: 10.1136/gutjnl-2012-303816
Li, N., Wang, B., Wu, Y., Luo, X., Chen, Z., Sang, C., et al. (2019). Modification effects of SanWei GanJiang Powder on liver and intestinal damage through reversing bile acid homeostasis. BioMed. Pharmacother. 116, 109044. doi: 10.1016/j.biopha.2019.109044
Li, X., Wang, Y., Xing, Y., Xing, R., Liu, Y., Xu, Y. (2020). Changes of gut microbiota during silybin-mediated treatment of high-fat diet-induced non-alcoholic fatty liver disease in mice. Hepatol. Res. 50, 5–14. doi: 10.1111/hepr.13444
Li, L., Chen, J., Lin, L., Pan, G., Zhang, S., Chen, H., et al. (2020). Quzhou Fructus Aurantii Extract suppresses inflammation via regulation of MAPK, NF-κB, and AMPK signaling pathway. Sci. Rep 10 (1), 1593. doi: 10.1038/s41598-020-58566-7
Li, Q., Li, M., Li, F., Zhou, W., Dang, Y., Zhang, L., et al. (2020). Qiang-Gan formula extract improves non-alcoholic steatohepatitis via regulating bile acid metabolism and gut microbiota in mice. J. EthnoPharmacol. 258, 112896. doi: 10.1016/j.jep.2020.112896
Li, J., Hu, Q., Xiao-Yu, D., Zhu, L., Miao, Y. F., Kang, H. X., et al. (2020). Effect of sheng-jiang powder on gut microbiota in high-fat diet-induced NAFLD. Evidence-based complementary and alternative medicine : eCAM. 2020, 6697638. doi: 10.1155/2020/6697638
Li, X., Hong, J., Wang, Y., Pei, M., Wang, L., Gong, Z. (2021). Trimethylamine-N-oxide pathway: A potential target for the treatment of MAFLD. Front. Mol. BioSci. 8, 733507. doi: 10.3389/fmolb.2021.733507
Li, S., You, J., Wang, Z., Liu, Y., Wang, B., Du, M., et al. (2021). Curcumin alleviates high-fat diet-induced hepatic steatosis and obesity in association with modulation of gut microbiota in mice. Food Res. Int. 143, 110270. doi: 10.1016/j.foodres.2021.110270
Li, C., Zhou, W., Li, M., Shu, X., Zhang, L., Ji, G. (2021). Salvia-Nelumbinis naturalis extract protects mice against MCD diet-induced steatohepatitis via activation of colonic FXR-FGF15 pathway. Biomedicine Pharmacotherapy 139, 111587. doi: 10.1016/j.biopha.2021.111587
Li, L., Huang, Q., Yang, L., Zhang, R., Gao, L., Han, X., et al. (2022). The association between non-alcoholic fatty liver disease (NAFLD) and advanced fibrosis with serological vitamin B12 markers: results from the NHANES 1999–2004. Nutrients 14 (6), 1224. doi: 10.3390/nu14061224
Li, Q., Liu, W., Zhang, H., Chen, C., Liu, R., Hou, H., et al. (2022). α-D-1,3-glucan from Radix Puerariae thomsonii improves NAFLD by regulating the intestinal flora and metabolites. Carbohydr. Polymers 299, 120197. doi: 10.1016/j.carbpol.2022.120197
Li, X., Zhao, W., Xiao, M., Yu, L., Chen, Q., Hu, X., et al. (2022). Penthorum chinense Pursh. extract attenuates non-alcholic fatty liver disease by regulating gut microbiota and bile acid metabolism in mice. J. EthnoPharmacol. 294, 115333. doi: 10.1016/j.jep.2022.115333
Li, Y., Bai, D., Lu, Y., Chen, J., Yang, H., Mu, Y., et al. (2022). The crude guava polysaccharides ameliorate high-fat diet-induced obesity in mice via reshaping gut microbiota. Int. J. Biol. Macromolecules 213, 234–246. doi: 10.1016/j.ijbiomac.2022.05.130
Li, C., Yu, S., Li, X., Cao, Y., Li, M., Ji, G., et al. (2022). Medicinal formula huazhi-rougan attenuates non-alcoholic steatohepatitis through enhancing fecal bile acid excretion in mice. Front. Pharmacol. 13, 833414. doi: 10.3389/fphar.2022.833414
Li, S.-Z., Zhang, N. N., Yang, X., Huang, T. Q., Lin, Y., Jiang, Z. M., et al. (2023). Nobiletin ameliorates nonalcoholic fatty liver disease by regulating gut microbiota and myristoleic acid metabolism. J. Agric. Food Chem 71 (19), 7312–7323. doi: 10.1021/acs.jafc.2c08637
Li, L., Hou, X., Xu, R., Liu, C., Tu, M. (2016). Research review on the pharmacological effects of astragaloside IV. Fundam. Clin. Pharmacol. 31 (1), 17–36. doi: 10.1111/fcp.12232
Li, Y., Li, J., Su, Q., Liu, Y. (2019). Sinapine reduces non-alcoholic fatty liver disease in mice by modulating the composition of the gut microbiota. Food Funct. 10, 3637–3649. doi: 10.1039/c9fo00195f
Li, H., Xi, Y., Xin, X., Tian, H., Hu, Y. (2020). Gypenosides regulate farnesoid X receptor-mediated bile acid and lipid metabolism in a mouse model of non-alcoholic steatohepatitis. Nutr. Metab. (Lond) 17, 34. doi: 10.1186/s12986-020-00454-y
Li, R., Yao, Y., Gao, P., Bu, S. (2020). The therapeutic efficacy of curcumin vs. Metformin in modulating the gut microbiota in NAFLD rats: A comparative study. Front. Microbiol. 11, 555293. doi: 10.3389/fmicb.2020.555293
Liang, S., Zhang, Y., Deng, Y., He, Y., Liang, Y., Liang, Z., et al. (2018). The potential effect of Chinese herbal formula hongqijiangzhi fang in improving NAFLD: focusing on NLRP3 inflammasome and gut microbiota. Evid Based Complement Alternat Med. 2018, 5378961. doi: 10.1155/2018/5378961
Liang, W., Zhou, K., Jian, P., Chang, Z., Zhang, Q., Liu, Y., et al. (2021). Ginsenosides improve nonalcoholic fatty liver disease via integrated regulation of gut microbiota, inflammation and energy homeostasis. Front. Pharmacol. 12, 622841. doi: 10.3389/fphar.2021.622841
Liao, J., Cao, Y., Zhao, J., Yu, B., Wang, Y., Li, W., et al. (2023). Aqueous extract of Polygala japonica Houtt. ameliorated nonalcoholic steatohepatitis in mice through restoring the gut microbiota disorders and affecting the metabolites in feces and liver. Phytomedicine 118, 154937. doi: 10.1016/j.phymed.2023.154937
Lin, H., Zhang, X., Li, G., Wong, G. L.-H., Wong, V. W.-S. (2021). Epidemiology and clinical outcomes of metabolic (Dysfunction)-associated fatty liver disease. J. Clin. Trans. Hepatol 9(6), 972–982. doi: 10.14218/jcth.2021.00201
Liu, W., Luo, X., Tang, J., Mo, Q., Zhong, H., Zhang, H., et al. (2020). A bridge for short-chain fatty acids to affect inflammatory bowel disease, type 1 diabetes, and non-alcoholic fatty liver disease positively: by changing gut barrier. Eur. J. Nutr 60 (5), 2317–2330. doi: 10.1007/s00394-020-02431-w
Liu, X., Sun, R., Li, Z., Xiao, R., Lv, P., Sun, X., et al. (2021). Luteolin alleviates non-alcoholic fatty liver disease in rats via restoration of intestinal mucosal barrier damage and microbiota imbalance involving in gut-liver axis. Arch. Biochem. Biophysics 711, 109019. doi: 10.1016/j.abb.2021.109019
Liu, S., Chen, P., Mohammed, S. A.D., Li, Z., Jiang, X., Wu, J., et al. (2022). Exploration of the potential mechanism of Baicalin for hepatic fibrosis based on network pharmacology, gut microbiota, and experimental validation. Front. Microbiol. 13, 1051100. doi: 10.3389/fmicb.2022.1051100
Liu, H., Xu, J., Li, H., Zhang, L., Xu, P. (2021). Network pharmacology-based investigation to explore the effect and mechanism of Erchen decoction against the nonalcoholic fatty liver disease. Anatomical Record: Adv. Integr. Anat. Evolutionary Biol 304 (11), 2605–2619. doi: 10.1002/ar.24770
Long, S. L., Gahan, C. G. M., Joyce, S. A. (2017). Interactions between gut bacteria and bile in health and disease. Mol. Aspects Med. 56, 54–65. doi: 10.1016/j.mam.2017.06.002
Luo, X., Zhang, B., Pan, Y., Gu, J., Tan, R., Gong, P. (2022). Phyllanthus emblica aqueous extract retards hepatic steatosis and fibrosis in NAFLD mice in association with the reshaping of intestinal microecology. Front. Pharmacol. 13, 893561. doi: 10.3389/fphar.2022.893561
Ma, Q., Li, Y., Li, P., Wang, M., Wang, J., Tang, Z., et al. (2019). Research progress in the relationship between type 2 diabetes mellitus and intestinal flora. Biomedicine Pharmacotherapy 117, 109138. doi: 10.1016/j.biopha.2019.109138
Ma, C., Yan, K., Wang, Z., Zhang, Q., Gao, L., Xu, T., et al. (2021). The association between hypertension and nonalcoholic fatty liver disease (NAFLD): literature evidence and systems biology analysis. Bioengineered 12 (1), 2187–2202. doi: 10.1080/21655979.2021.1933302
Maddie, N., Perez, A., Yuen, A., Kiernan, R., Carrillo-Sepulveda, M. A. (2022). Hyperacetylation of Toll-like receptor 4 (TLR4): a potential factor contributing to non-alcoholic liver disease (NAFLD), a silent killer in obesity. FASEB J. 36 (S1). doi: 10.1096/fasebj.2022.36.s1.r6275
Maslowski, K. M., Vieira, A. T., Ng, A., Kranich, J., Sierro, F., Yu, D., et al. (2009). Regulation of inflammatory responses by gut microbiota and chemoattractant receptor GPR43. Nature 461 (7268), 1282–1286. doi: 10.1038/nature08530
McMahan, R. H., Wang, X. X., Cheng, L. L., Krisko, T., Smith, M., El Kasmi, K., et al. (2013). Bile acid receptor activation modulates hepatic monocyte activity and improves nonalcoholic fatty liver disease. J. Biol. Chem 288 (17), 11761–11770. doi: 10.1074/jbc.m112.446575
Miao, J., Guo, L., Cui, H., Wang, L., Zhu, B., Lei, J., et al. (2022). Er-chen decoction alleviates high-fat diet-induced nonalcoholic fatty liver disease in rats through remodeling gut microbiota and regulating the serum metabolism. Evidence-Based Complementary Altern. Med. 2022, 6221340. doi: 10.1155/2022/6221340
Mitrovic, B., Gluvic, Z., Macut, D., Obradovic, M., Sudar-Milovanovic, E., Soskic, S., et al. (2022). Effects of metformin-single therapy on the level of inflammatory markers in serum of non-obese T2DM patients with NAFLD. Endocrine Metab. Immune Disord. - Drug Targets 22 (1), 117–124. doi: 10.2174/1871530321666210225110140
Mo, W., Zou, J., Wu, M.T., Peng, Z., He, W., Li, W., et al. (2023). Noni (Morinda citrifolia L.) fruit polysaccharide ameliorated high-fat diet-induced obesity by modulating gut microbiota and improving bile acid metabolism. J. Funct. Foods 101 (11), 105408. doi: 10.1016/j.jff.2023.105408
Mu, H., Zhou, Q., Yang, R., Zeng, J., Li, X., Zhang, R., et al. (2020). Naringin attenuates high fat diet induced non-alcoholic fatty liver disease and gut bacterial dysbiosis in mice. Front. Microbiol. 11, 585066. doi: 10.3389/fmicb.2020.585066
Nilsson, N. E., Kotarsky, K., Owman, C., Olde, B. (2003). Identification of a free fatty acid receptor, FFA2R, expressed on leukocytes and activated by short-chain fatty acids. Biochem. Biophys. Res. Commun 303 (4), 1047–1052. doi: 10.1016/s0006-291x(03)00488-1
Orivuori, L., Mustonen, K., de Goffau, M. C., Hakala, S., Paasela, M., Roduit, C., et al. (2015). High level of fecal calprotectin at age 2 months as a marker of intestinal inflammation predicts atopic dermatitis and asthma by age 6. Clin. Exp. Allergy 45 (5), 928–939. doi: 10.1111/cea.12522
Pan, M.-X., Zheng, C. Y., Deng, Y. J., Tang, K. R., Nie, H., Xie, J. Q., et al. (2021). Hepatic protective effects of Shenling Baizhu powder, a herbal compound, against inflammatory damage via TLR4/NLRP3 signalling pathway in rats with nonalcoholic fatty liver disease. J. Integr. Med. 19 (5), 428–438. doi: 10.1016/j.joim.2021.07.004
Paola, B., Luigi De, M., Daniela, R. (2023). Functional properties of natural products and human health. Nutrients 15 (13), 2961. doi: 10.3390/nu15132961
Perino, A., Velázquez-Villegas, L. A., Bresciani, N., Sun, Y., Huang, Q., Fénelon, V. S., et al. (2021). Central anorexigenic actions of bile acids are mediated by TGR5. Nat. Metab. 3 (5), 595–603. doi: 10.1038/s42255-021-00398-4
Perino, A., Schoonjans, K. (2022). Metabolic Messengers: bile acids. Nat. Metab. 4 (4), 416–423. doi: 10.1038/s42255-022-00559-z
Perumpail, B., Li, A. A., John, N., Sallam, S., Shah, N. D., Kwong, W., et al. (2018). The role of vitamin E in the treatment of NAFLD. Diseases 6 (4), 86. doi: 10.3390/diseases6040086
Pineda Torra, I., Claudel, T., Duval, C., Kosykh, V., Fruchart, J. C., Staels, B., et al. (2003). Bile acids induce the expression of the human peroxisome proliferator-activated receptor alpha gene via activation of the farnesoid X receptor. Mol. Endocrinol. 17 (2), 259–272. doi: 10.1210/me.2002-0120
Poli, M. C., Orange, J. (2017). Variation in microbiome LPS immunogenicity contributes to autoimmunity in humans. Pediatrics 140 (S3), S184–S185. doi: 10.1542/peds.2017-2475w
Pop, T. L., Sîrbe, C., Benţa, G., Mititelu, A., Grama, A. (2022). The role of vitamin D and vitamin D binding protein in chronic liver diseases. Int. J. Mol. Sci. 23 (18), 10705. doi: 10.3390/ijms231810705
Porras, D., Nistal, E., Martínez-Flórez, S., Pisonero-Vaquero, S., Olcoz, J. L., Jover, R., et al. (2016). Protective effect of quercetin on high-fat diet-induced non-alcoholic fatty liver disease in mice is mediated by modulating intestinal microbiota imbalance and related gut-liver axis activation. Free Radical Biol. Med. 102, 188–202. doi: 10.1016/j.freeradbiomed.2016.11.037
Qian, Y., Yang, X., Xu, S., Wu, C., Song, Y., Qin, N., et al. (2018). Alteration of the fecal microbiota in Chinese patients with Parkinson’s disease. Brain Behavior Immun 70, 194–202. doi: 10.1016/j.bbi.2018.02.016
Quek, J., Chan, K. E., Wong, Z. Y., Tan, C., Tan, B., Lim, W. H., et al. (2022). Global prevalence of non-alcoholic fatty liver disease and non-alcoholic steatohepatitis in the overweight and obese population: a systematic review and meta-analysis. Lancet Gastroenterol. Hepatol 8 (1), 20–30. doi: 10.1016/s2468-1253(22)00317-x
Raman, M., Ahmed, I., Gillevet, P. M., Probert, C. S., Ratcliffe, N. M., Smith, S., et al. (2013). Fecal microbiome and volatile organic compound metabolome in obese humans with nonalcoholic fatty liver disease. Clin. Gastroenterol. Hepatol 11 (7), 868–75.e753. doi: 10.1016/j.cgh.2013.02.015
Safari, Z., Gérard, P. (2019). The links between the gut microbiome and non-alcoholic fatty liver disease (NAFLD). Cell. Mol. Life Sci. 76 (8), 1541–1558. doi: 10.1007/s00018-019-03011-w
Sang, T., Guo, C., Guo, D., Wu, J., Wang, Y., Wang, Y., et al. (2021). Suppression of obesity and inflammation by polysaccharide from sporoderm-broken spore of Ganoderma lucidum via gut microbiota regulation. Carbohydr Polym 256, 117594. doi: 10.1016/j.carbpol.2020.117594
Schaap, F. G., Trauner, M., Jansen, P. L. M. (2013). Bile acid receptors as targets for drug development. Nat. Rev. Gastroenterol. Hepatol 11 (1), 55–67. doi: 10.1038/nrgastro.2013.151
Sekiguchi, H., Kasubuchi, M., Hasegawa, S., Pelisch, N., Kimura, I., Ichimura, A. (2015). A novel antidiabetic therapy: free fatty acid receptors as potential drug target. Curr. Diabetes Rev 11 (2), 107–115. doi: 10.2174/1573399811666150302112421
Sharifnia, T., Antoun, J., Verriere, T. G., Suarez, G., Wattacheril, J., Wilson, K. T., et al. (2015). Hepatic TLR4 signaling in obese NAFLD. Am. J. Physiology-Gastrointestinal Liver Physiol. 309 (4), G270–G278. doi: 10.1152/ajpgi.00304.2014
Shen, F., Zheng, R. D., Sun, X. Q., Ding, W. J., Wang, X. Y., Fan, J. G. (2017). Gut microbiota dysbiosis in patients with non-alcoholic fatty liver disease. Hepatobiliary Pancreatic Dis. Int 16 (4), 375–381. doi: 10.1016/s1499-3872(17)60019-5
Shi, C., Pei, M., Wang, Y., Chen, Q., Cao, P., Zhang, L., et al. (2022). Changes of flavin-containing monooxygenases and trimethylamine-N-oxide may be involved in the promotion of non-alcoholic fatty liver disease by intestinal microbiota metabolite trimethylamine. Biochem. Biophys. Res. Commun 594, 1–7. doi: 10.1016/j.bbrc.2022.01.060
Shojaee-Moradie, F., Cuthbertson, D. J., Barrett, M., Jackson, N. C., Herring, R., Thomas, E. L., et al. (2016). Exercise training reduces liver fat and increases rates of VLDL clearance but not VLDL production in NAFLD. J. Clin. Endocrinol. Metab. 101 (11), 4219–4228. doi: 10.1210/jc.2016-2353
Shu, X., Li, M., Cao, Y., Li, C., Zhou, W., Ji, G., et al. (2021). Berberine alleviates non-alcoholic steatohepatitis through modulating gut microbiota mediated intestinal FXR activation. Front. Pharmacol. 12, 750826. doi: 10.3389/fphar.2021.750826
Singh, N., Gurav, A., Sivaprakasam, S., Brady, E., Padia, R., Shi, H., et al. (2014). Activation of gpr109a, receptor for niacin and the commensal metabolite butyrate, suppresses colonic inflammation and carcinogenesis. Immunity 40 (1), 128–139. doi: 10.1016/j.immuni.2013.12.007
Song, X., Tan, L., Wang, M., Ren, C., Guo, C., Yang, B., et al. (2020). Myricetin: A review of the most recent research. Biomedicine Pharmacotherapy 134, 111017. doi: 10.1016/j.biopha.2020.111017
Song, J., Liu, Q., Hao, M., Zhai, X., Chen, J. (2023). Effects of neutral polysaccharide from Platycodon grandiflorum on high-fat diet-induced obesity via the regulation of gut microbiota and metabolites. Front. Endocrinol. (Lausanne) 14, 1078593. doi: 10.3389/fendo.2023.1078593
Song, M. J., Malhi, H. (2019). The unfolded protein response and hepatic lipid metabolism in non alcoholic fatty liver disease. Pharmacol. Ther. 203, 107401. doi: 10.1016/j.pharmthera.2019.107401
Suga, T., Yamaguchi, H., Ogura, J., Shoji, S., Maekawa, M., Mano, N. (2019). Altered bile acid composition and disposition in a mouse model of non-alcoholic steatohepatitis. Toxicol. Appl. Pharmacol. 379, 114664. doi: 10.1016/j.taap.2019.114664
Sun, R., Yang, N., Kong, B., Cao, B., Feng, D., Yu, X., et al. (2016). Orally administered berberine modulates hepatic lipid metabolism by altering microbial bile acid. Metab. Intestinal FXR Signaling Pathway. Mol. Pharmacol. 91 (2), 110–122. doi: 10.1124/mol.116.106617
Sun, W. L., Li, X. Y., Dou, H. Y., Wang, X. D., Li, J. D., Shen, L., et al. (2021). Myricetin supplementation decreases hepatic lipid synthesis and inflammation by modulating gut microbiota. Cell Rep. 36 (9), 109641. doi: 10.1016/j.celrep.2021.109641
Sun, J., Fan, J., Li, T., Yan, X., Jiang, Y. (2022). Nuciferine protects against high-fat diet-induced hepatic steatosis via modulation of gut microbiota and bile acid metabolism in rats. J. Agric. Food Chem. 70 (38), 12014–12028. doi: 10.1021/acs.jafc.2c04817
Tan, J., McKenzie, C., Potamitis, M., Thorburn, A. N., Mackay, C. R., Macia, L. (2014). The role of short-chain fatty acids in health and disease. Adv. Immunol. 121, 91–119. doi: 10.1016/b978-0-12-800100-4.00003-9
Tan, X., Liu, Y., Long, J., Chen, S., Liao, G., Wu, S., et al. (2019). Trimethylamine N-oxide aggravates liver steatosis through modulation of bile acid metabolism and inhibition of farnesoid X receptor signaling in nonalcoholic fatty liver disease. Mol. Nutr. Food Res. 63 (17), e1900257. doi: 10.1002/mnfr.201900257
Tanase, D. M., Gosav, E. M., Costea, C. F., Ciocoiu, M., Lacatusu, C. M., Maranduca, M. A., et al. (2020). The intricate relationship between type 2 diabetes mellitus (T2DM), insulin resistance (IR), and nonalcoholic fatty liver disease (NAFLD). J. Diabetes Res. 2020, 3920196. doi: 10.1155/2020/3920196
Tang, K., Deng, Y., Zheng, C., Nie, H., Pan, M., Chen, R., et al. (2020). Prevention of nonalcoholic hepatic steatosis by Shenling Baizhu powder: involvement of adiponectin-induced inhibition of hepatic SREBP-1c. Oxid. Med. Cell. Longevity 2020, 9701285. doi: 10.1155/2020/9701285
Targher, G., Byrne, C. D., Lonardo, A., Zoppini, G., Barbui, C. (2016). Non-alcoholic fatty liver disease and risk of incident cardiovascular disease: A meta-analysis. J. Hepatol 65 (3), 589–600. doi: 10.1016/j.jhep.2016.05.013
Theofilis, P., Vordoni, A., Kalaitzidis, R. G. (2022). Trimethylamine N-oxide levels in non-alcoholic fatty liver disease: A systematic review and meta-analysis. Metabolites 12 (12), 1243. doi: 10.3390/metabo12121243
Thomas, C., Gioiello, A., Noriega, L., Strehle, A., Oury, J., Rizzo, G., et al. (2009). TGR5-mediated bile acid sensing controls glucose homeostasis. Cell Metab. 10 (3), 167–177. doi: 10.1016/j.cmet.2009.08.001
Thorburn, A. N., Macia, L., Mackay, Charles, R. (2014). Diet, metabolites, and “Western-lifestyle” Inflammatory diseases. Immunity 40 (6), 833–842. doi: 10.1016/j.immuni.2014.05.014
Thuy, S., Ladurner, R., Volynets, V., Wagner, S., Strahl, S., Königsrainer, A., et al. (2008). Nonalcoholic fatty liver disease in humans is associated with increased plasma endotoxin and plasminogen activator inhibitor 1 concentrations and with fructose intake. J. Nutr 138 (8), 1452–1455. doi: 10.1093/jn/138.8.1452
Tian, L., Lei, X., Fu-Hua, C., Yi-Bo, Z. (2020). Association of serum vitamin D level and nonalcoholic fatty liver disease: a meta-analysis. Eur. J. Gastroenterol. Hepatol 32 (2), 140–147. doi: 10.1097/meg.0000000000001486
Tong, Q. (2018). Dihydromyricetin improves obesity and insulin resistance by modulating the composition of gut microbiota. [dissertation/doctoral dissertation]. (Wuhan (Hubei, People’s Republic of China): Huazhong University of Science and Technology). Available at: https://kns.cnki.net/KCMS/detail/detail.aspx?dbname=CDFDLAST2019&filename=1018210528.nh.
Wang, L., Wu, Y., Zhuang, L., Chen, X., Min, H., Song, S., et al. (2019). Puerarin prevents high-fat diet-induced obesity by enriching Akkermansia muciniphila in the gut microbiota of mice. PloS One 14 (6), e0218490. doi: 10.1371/journal.pone.0218490
Wang, P., Li, D., Ke, W., Liang, D., Hu, X., Chen, F. (2019). Resveratrol-induced gut microbiota reduces obesity in high-fat diet-fed mice. Int. J. Obes. 44 (1), 213–225. doi: 10.1038/s41366-019-0332-1
Wang, P., Gao, J., Ke, W., Wang, J., Li, D., Liu, R., et al. (2020a). Resveratrol reduces obesity in high-fat diet-fed mice via modulating the composition and metabolic function of the gut microbiota. Free Radical Biol. Medicine3 156, 83–98. doi: 10.1016/j.freeradbiomed.2020.04.013
Wang, P., Wang, J., Li, D., Ke, W., Chen, F., Hu, X. (2020b). Targeting the gut microbiota with resveratrol: a demonstration of novel evidence for the management of hepatic steatosis. J. Nutr. Biochem. 81, 108363. doi: 10.1016/j.jnutbio.2020.108363
Wang, Y., Yao, W., Li, B., Qian, S., Wei, B., Gong, S., et al. (2020). Nuciferine modulates the gut microbiota and prevents obesity in high-fat diet-fed rats. Exp. Mol. Med. 52 (12), 1959–1975. doi: 10.1038/s12276-020-00534-2
Wang, F., Park, J. S., Ma, Y., Ma, H., Lee, Y. J., Lee, G. R., et al. (2021). Ginseng saponin enriched in rh1 and rg2 ameliorates nonalcoholic fatty liver disease by inhibiting inflammasome activation. Nutrients 13 (3), 856. doi: 10.3390/nu13030856
Wang, T., Liu, L., Deng, J., Jiang, Y., Yan, X., and Liu, W. (2023). Analysis of the mechanism of action of quercetin in the treatment of hyperlipidemia based on metabolomics and intestinal flora. Food Funct. 14 (4), 2112–2127. doi: 10.1039/d2fo03509j
Wang, Z., Sun, Y., Han, Y., Chen, X., Gong, P., Zhai, P., et al. (2023a). Eucommia bark/leaf extract improves HFD-induced lipid metabolism disorders via targeting gut microbiota to activate the Fiaf-LPL gut-liver axis and SCFAs-GPR43 gut-fat axis. Phytomedicine 110, 154652. doi: 10.1016/j.phymed.2023.154652
Wang, Z., Yao, W., Sun, Y., Han, Y., Chen, X., Gong, P., et al. (2023b). Eucommia bark/leaf extract improves lipid metabolism disorders by affecting intestinal microbiota and microbiome–host interaction in HFD mice. J. Agric. Food Chem. 71 (7), 3297–3314 doi: 10.1021/acs.jafc.2c07239
Wang, L., Gou, X., Ding, Y., Liu, J., Wang, Y., Wang, Y., et al. (2023). The interplay between herbal medicines and gut microbiota in metabolic diseases. Front. Pharmacol. 14, 1105405. doi: 10.3389/fphar.2023.1105405
Wang, H., Gao, J., Kou, J., Zhu, D., Yu, B. (2007). Anti-inflammatory activities of triterpenoid saponins from Polygala japonica. Phytomedicine 15 (5), 321–326. doi: 10.1016/j.phymed.2007.09.014
Wang, S., Sheng, F., Zou, L., Xiao, J., Li, P. (2021). Hyperoside attenuates non-alcoholic fatty liver disease in rats via cholesterol metabolism and bile acid metabolism. J. Adv. Res. 34, 109–122. doi: 10.1016/j.jare.2021.06.001
Wang, X., Shi, L., Wang, X., Feng, Y., Wang, Y. (2019). MDG-1, an Ophiopogon polysaccharide, restrains process of non-alcoholic fatty liver disease via modulating the gut-liver axis. Int. J. Biol. Macromolecules 141, 1013–1021. doi: 10.1016/j.ijbiomac.2019.09.007
Wang, F., Xu, J., Jakovlic, I., Wang, W. M., Zhao, Y. H. (2019). Dietary betaine reduces liver lipid accumulation via improvement of bile acid and trimethylamine-N-oxide metabolism in blunt-snout bream. Food Funct. 10, 6675–6689. doi: 10.1039/c9fo01853k
Watanabe, M., Houten, S. M., Wang, L., Moschetta, A., Mangelsdorf, D. J., Heyman, R. A., et al. (2004). Bile acids lower triglyceride levels via a pathway involving FXR, SHP, and SREBP-1c. J. Clin. Invest 113 (10), 1408–1418. doi: 10.1172/jci21025
Whiting, S. J., Calvo, M. S. (2021). Vitamin D: nutrition information brief. Adv. Nutr. 12 (5), 2037–2039. doi: 10.1093/advances/nmab051
Xiao, Z., Xiao, W., Li, G. (2022). Research progress on the pharmacological action of schisantherin A. Evidence-Based Complementary Altern. Med. 2022, 6420865. doi: 10.1155/2022/6420865
Xiong, Y., Miyamoto, N., Shibata, K., Valasek, M. A., Motoike, T., Kedzierski, R. M., et al. (2004). Short-chain fatty acids stimulate leptin production in adipocytes through the G protein-coupled receptor GPR41. Proc. Natl. Acad. Sci. U. S. A. 101 (4), 1045–1050. doi: 10.1073/pnas.2637002100
Xiong, F., Zheng, Z., Xiao, L., Su, C., Chen, J., Gu, X., et al. (2021). Soyasaponin A(2) alleviates steatohepatitis possibly through regulating bile acids and gut microbiota in the methionine and choline-deficient (MCD) diet-induced nonalcoholic steatohepatitis (NASH) mice. Mol. Nutr. Food Res. 65, e2100067. doi: 10.1002/mnfr.202100067
Xu, J., Liu, T., Li, Y., Liu, W., Ding, Z., Ma, H., et al. (2019). amun (Eugenia jambolana lam.) fruit extract prevents obesity by modulating the gut microbiome in high-fat-diet-fed mice. Mol. Nutr. Food Res. 63 (9), e1801307. doi: 10.1002/mnfr.201801307
Xu, H., Wang, X., Feng, W., Liu, Q., Zhou, S., Liu, Q., et al. (2020). The gut microbiota and its interactions with cardiovascular disease. Microbial Biotechnol 13 (3), 637–656. doi: 10.1111/1751-7915.13524
Xu, Y., Wang, N., Tan, H. Y., Li, S., Zhang, C., Zhang, Z., et al. (2020). Panax notoginseng saponins modulate the gut microbiota to promote thermogenesis and beige adipocyte reconstruction via leptin-mediated AMPKα/STAT3 signaling in diet-induced obesity. Theranostics 10 (24), 11302–11323. doi: 10.7150/thno.47746
Xu, Y., Wang, N., Tan, H. Y., Li, S., Zhang, C., Feng, Y. (2021). Gut-liver axis modulation of Panax notoginseng saponins in nonalcoholic fatty liver disease. Hepatol. Int. 15, 350–365. doi: 10.1007/s12072-021-10138-1
Xue, L., He, J., Gao, N., Lu, X., Li, M., Wu, X., et al. (2017). Probiotics may delay the progression of nonalcoholic fatty liver disease by restoring the gut microbiota structure and improving intestinal endotoxemia. Sci. Rep. 7, 45176. doi: 10.1038/srep45176
Yan, C., Zhang, Y., Zhang, X., Aa, J., Wang, G., Xie, Y. (2018). Curcumin regulates endogenous and exogenous metabolism via Nrf2-FXR-LXR pathway in NAFLD mice. BioMed. Pharmacother. 105, 274–281. doi: 10.1016/j.biopha.2018.05.135
Yang, H., Yang, T., Heng, C., Zhou, Y., Jiang, Z., Qian, X., et al. (2019). Quercetin improves nonalcoholic fatty liver by ameliorating inflammation, oxidative stress, and lipid metabolism in db/db mice. Phytother. Res. 33, 3140–3152. doi: 10.1002/ptr.6486
Yang, Z., Yu, Y., Sun, N., Zhou, L., Zhang, D., Chen, H., et al. (2020). Ginsenosides Rc, as a novel SIRT6 activator, protects mice against high fat diet induced NAFLD. J. Ginseng Res. 47 (3), 376–384. doi: 10.1016/j.jgr.2020.07.005
Yang, X., Mo, W., Zheng, C., Li, W., Tang, J., Wu, X. (2020). Alleviating effects of noni fruit polysaccharide on hepatic oxidative stress and inflammation in rats under a high-fat diet and its possible mechanisms. Food Funct. 11 (4), 2953–2968. doi: 10.1039/d0fo00178c
Yang, M., Yin, Y., Wang, F., Zhang, H., Ma, X., Yin, Y., et al. (2021). Supplementation with Lycium barbarum polysaccharides reduce obesity in high-fat diet-fed mice by modulation of gut microbiota. Front. Microbiol. 12, 719967. doi: 10.3389/fmicb.2021.719967
Yang, Y., Chang, Y., Wu, Y., Liu, H., Liu, Q., Kang, Z., et al. (2021). A homogeneous polysaccharide from Lycium barbarum: Structural characterizations, anti-obesity effects and impacts on gut microbiota. Int. J. Biol. Macromolecules 183, 2074–2087. doi: 10.1016/j.ijbiomac.2021.05.209
Yang, Y., Wu, C. (2022). Targeting gut microbial bile salt hydrolase (BSH) by diet supplements: new insights into dietary modulation of human health. Food Funct. 13 (14), 7409–7422. doi: 10.1039/d2fo01252a
Ye, J., Lv, L., Wu, W., Li, Y., Shi, D., Fang, D., et al. (2018). Butyrate protects mice against methionine-choline-deficient diet-induced non-alcoholic steatohepatitis by improving gut barrier function, attenuating inflammation and reducing endotoxin levels. Front. Microbiol. 9, 1967. doi: 10.3389/fmicb.2018.01967
Yin, X., Liao, W., Li, Q., Zhang, H., Liu, Z., Zheng, X., et al. (2020). Interactions between resveratrol and gut microbiota affect the development of hepatic steatosis: A fecal microbiota transplantation study in high-fat diet mice. J. Funct. Foods 67, 103883. doi: 10.1016/j.jff.2020.103883
Younossi, Z. M., Koenig, A. B., Abdelatif, D., Fazel, Y., Henry, L., Wymer, M. (2016). Global epidemiology of nonalcoholic fatty liver disease-Meta-analytic assessment of prevalence, incidence, and outcomes. Hepatology 64 (1), 73–84. doi: 10.1002/hep.28431
Yu, S., Jiang, J., Li, Q., Liu, X., Wang, Z., Yang, L., et al. (2022). Schisantherin A alleviates non-alcoholic fatty liver disease by restoring intestinal barrier function. Front. Cell Infect. Microbiol. 12, 855008. doi: 10.3389/fcimb.2022.855008
Yu, X., Meng, X., Yan, Y., Wang, H., Zhang, L. (2022). Extraction of naringin from pomelo and its therapeutic potentials against hyperlipidemia. Molecules 27 (24), 9033. doi: 10.3390/molecules27249033
Zaibi, M. S., Stocker, C. J., O'Dowd, J., Davies, A., Bellahcene, M., Cawthorne, M. A., et al. (2010). Roles of GPR41 and GPR43 in leptin secretory responses of murine adipocytes to short chain fatty acids. FEBS Lett 584 (11), 2381–2386. doi: 10.1016/j.febslet.2010.04.027
Zhai, Y., Zhou, W., Yan, X., Qiao, Y., Guan, L., Zhang, Z., et al. (2022). Astragaloside IV ameliorates diet-induced hepatic steatosis in obese mice by inhibiting intestinal FXR via intestinal flora remodeling. Phytomedicine 107, 154444. doi: 10.1016/j.phymed.2022.154444
Zhang, Y.-J., Li, S., Gan, R. Y., Zhou, T., Xu, D. P., Li, H. B. (2015). Impacts of gut bacteria on human health and diseases. Int. J. Mol. Sci. 16 (4), 7493–7519. doi: 10.3390/ijms16047493
Zhang, Y., Tang, K., Deng, Y., Chen, R., Liang, S., Xie, H., et al. (2018). Effects of shenling baizhu powder herbal formula on intestinal microbiota in high-fat diet-induced NAFLD rats. Biomedicine Pharmacotherapy 102, 1025–1036. doi: 10.1016/j.biopha.2018.03.158
Zhang, L., Wang, Y., Wu, F., Wang, X., Feng, Y., Wang, Y. (2021). MDG, an Ophiopogon japonicus polysaccharide, inhibits non-alcoholic fatty liver disease by regulating the abundance of Akkermansia muciniphila. Int. J. Biol. Macromolecules 196, 23–34. doi: 10.1016/j.ijbiomac.2021.12.036
Zhang, X.-L., Chen, L., Yang, J., Zhao, S. S., Jin, S., Ao, N., et al. (2023). Vitamin D alleviates non-alcoholic fatty liver disease via restoring gut microbiota and metabolism. Front. Microbiol. 14, 1117644. doi: 10.3389/fmicb.2023.1117644
Zhang, N.-N., Jiang, Z.-M., Li, S.-Z., Yang, X., Liu, E. H. (2023). Evolving interplay between natural products and gut microbiota. Eur. J. Pharmacol. 949, 175557. doi: 10.1016/j.ejphar.2023.175557
Zhang, J., Ma, X., Fan, D. (2021). Ginsenoside CK ameliorates hepatic lipid accumulation via activating the LKB1/AMPK pathway in vitro and in vivo. Food Funct. 13 (3), 1153–1167. doi: 10.1039/d1fo03026d
Zhao, W., Xiao, M., Yang, J., Zhang, L., Ba, Y., Xu, R., et al. (2019). The combination of Ilexhainanoside D and ilexsaponin A1 reduces liver inflammation and improves intestinal barrier function in mice with high-fat diet-induced non-alcoholic fatty liver disease. Phytomedicine 63, 153039. doi: 10.1016/j.phymed.2019.153039
Zhao, W. W., Xiao, M., Wu, X., Li, X. W., Li, X. X., Zhao, T., et al. (2021). Ilexsaponin A(1) ameliorates diet-induced nonalcoholic fatty liver disease by regulating bile acid metabolism in mice. Front. Pharmacol. 12, 771976. doi: 10.3389/fphar.2021.771976
Zhong, M., Yan, Y., Yuan, H., Rong, A., Xu, G., Cai, F., et al. (2022). Astragalus mongholicus polysaccharides ameliorate hepatic lipid accumulation and inflammation as well as modulate gut microbiota in NAFLD rats. Food Funct. 13 (13), 7287–7301. doi: 10.1039/d2fo01009g
Zhong, F.-W., Li, G.-X., Zeng, L. (2022). [Gynostemma pentaphyllum saponins alleviate non-alcoholic fatty liver disease in rats by regulating intestinal flora and short-chain fatty acid metabolism]. Zhongguo Zhong yao za zhi = Zhongguo zhongyao zazhi = China J. Chin. materia Med. 47 (9), 2500–2508. doi: 10.19540/j.cnki.cjcmm.20211208.401
Zhou, D., Pan, Q., Xin, F. Z., Zhang, R. N., He, C. X., Chen, G. Y., et al. (2017). Sodium butyrate attenuates high-fat diet-induced steatohepatitis in mice by improving gut microbiota and gastrointestinal barrier. World J. Gastroenterol 23 (1), 60–75. doi: 10.3748/wjg.v23.i1.60
Zhou, D., Fan, J.-G. (2019). Microbial metabolites in non-alcoholic fatty liver disease. World J. Gastroenterol 25 (17), 2019–2028. doi: 10.3748/wjg.v25.i17.2019
Zhou, D., Zhang, J., Xiao, C., Mo, C., Ding, B.-S. (2022). Trimethylamine-N-oxide (TMAO) mediates the crosstalk between the gut microbiota and hepatic vascular niche to alleviate liver fibrosis in nonalcoholic steatohepatitis. Front. Immunol. 13, 964477. doi: 10.3389/fimmu.2022.964477
Zhu, L., Baker, R. D., Baker, S. S. (2014). Gut microbiome and nonalcoholic fatty liver diseases. Pediatr. Res. 77 (1-2), 245–251. doi: 10.1038/pr.2014.157
Zou, J., Xiang, Q., Tan, D., Shi, L., Liu, X., Wu, Y., et al. (2022). Zuogui-Jiangtang-Qinggan-Fang alleviates high-fat diet-induced type 2 diabetes mellitus with non-alcoholic fatty liver disease by modulating gut microbiome-metabolites-short chain fatty acid composition. Biomedicine Pharmacotherapy 157, 114002. doi: 10.1016/j.biopha.2022.114002
Zou, J. J., Zhou, M., Huang, Q. Q., Wu, Y. J., Yu, R. (2023). [Zuogui Jiangtang Qinggan Prescription promotes recovery of intestinal mucosal barrier in mice with type 2 diabetes mellitus and nonalcoholic fatty liver disease by improving intestinal flora homeostasis]. Zhongguo Zhong Yao Za Zhi 48, 525–533. doi: 10.19540/j.cnki.cjcmm.20220913.703
Keywords: MAFLD, gut microbiota, plant natural products, metabolite, gut-liver axis
Citation: Cai T, Song X, Xu X, Dong L, Liang S, Xin M, Huang Y, Zhu L, Li T, Wang X, Fang Y, Xu Z, Wang C, Wang M, Li J, Zheng Y, Sun W and Li L (2024) Effects of plant natural products on metabolic-associated fatty liver disease and the underlying mechanisms: a narrative review with a focus on the modulation of the gut microbiota. Front. Cell. Infect. Microbiol. 14:1323261. doi: 10.3389/fcimb.2024.1323261
Received: 17 October 2023; Accepted: 30 January 2024;
Published: 20 February 2024.
Edited by:
Suhana Chattopadhyay, University of Maryland, College Park, United StatesReviewed by:
Raksha Rao, University of Texas Health Science Center at Houston, United StatesLeena Malayil, University of Maryland, College Park, United States
Copyright © 2024 Cai, Song, Xu, Dong, Liang, Xin, Huang, Zhu, Li, Wang, Fang, Xu, Wang, Wang, Li, Zheng, Sun and Li. This is an open-access article distributed under the terms of the Creative Commons Attribution License (CC BY). The use, distribution or reproduction in other forums is permitted, provided the original author(s) and the copyright owner(s) are credited and that the original publication in this journal is cited, in accordance with accepted academic practice. No use, distribution or reproduction is permitted which does not comply with these terms.
*Correspondence: Lingru Li, lilingru912@163.com; Wenlong Sun, 512649113@qq.com; Yanfei Zheng, yanfei_z@163.com
†These authors have contributed equally to this work