- 1Department of Pediatric Intensive Care Unit, West China Second University Hospital, Sichuan University, Chengdu, China
- 2Key Laboratory of Birth Defects and Related Diseases of Women and Children (Sichuan University), Ministry of Education, Chengdu, China
- 3Depertment of Bioinformation, Hugobiotech Co., Ltd., Beijing, China
- 4Department of Pediatrics, West China Second University Hospital, Sichuan University, Chengdu, China
Fusobacterium necrophorum (F. necrophorum) infection is rare in pediatrics. In addition, the detection time of F. necrophorum by blood culture is long, and the positive rate is low. Infection with F. necrophorum bacilli usually follows rapid disease progression, resulting in high mortality. In previous reports of F. necrophorum-related cases, the most dangerous moment of the disease occurred after the appearance of Lemierre’s syndrome. We report an atypical case of a 6-year-old female patient who developed septic shock within 24 h of admission due to F. necrophorum infection in the absence of Lemierre’s syndrome. F. necrophorum was identified in a blood sample by metagenomics next-generation sequencing (mNGS) but not by standard blood culture. The patient was finally cured and discharged after receiving timely and effective targeted anti-infection treatment. In the present case study, it was observed that the heightened virulence and invasiveness of F. necrophorum contribute significantly to its role as a primary pathogen in pediatric septic shock. This can precipitate hemodynamic instability and multiple organ failure, even in the absence of Lemierre’s syndrome. The use of mNGS can deeply and rapidly identify infectious pathogens, guide the use of targeted antibiotics, and greatly improve the survival rate of patients.
Introduction
Fusobacterium necrophorum (F. necrophorum) is an obligate anaerobic, gram-negative rod bacterium commonly located in the oropharyngeal, genitourinary, and intestinal tracts. F. necrophorum can colonize the human body for a long time as a benign, non-pathogenic bacteria. However, in recent years, there have been a few reports that F. necrophorum can transform into community-acquired invasive bacteria, leading to serious infections. It can cause either localized abscesses and throat infections or systemic, life-threatening disease (Almohaya et al., 2020). Lemierre’s syndrome is a rare but potentially fatal complication caused by infection with F. necrophorum, which can be defined as bacteremia, internal jugular vein (IJV) thrombophlebitis, and metastatic septic emboli secondary to acute pharyngeal infections (Lee et al., 2020; Carius et al., 2022). The annual incidence rate of Lemierre’s syndrome is relatively low, estimated at approximately one case per million individuals in the general population (Riordan, 2007; Sheehan et al., 2019; Sacco et al., 2019). Typically, previously healthy young adults suffer more from this disease. The incidence is disproportionately concentrated in adolescent populations aged 16–24 years (Almohaya et al., 2020; Carius et al., 2022; Sacco et al., 2019). The mortality rates of this disease reported in the pre-antibiotic era reached 90%, whereas currently, mortality rates range from 5% to 9% (Almohaya et al., 2020; Riordan, 2007; Nygren and Holm, 2020). Currently, advancements in imaging techniques facilitate the prompt identification of Lemierre’s syndrome in clinical practice, thereby enabling timely therapeutic intervention. However, we have found in clinical practice that the infection of F. necrophorum in young children is often fatal in a short period of time because of a rapidly progressing inflammatory storm and does not wait for Lemierre’s syndrome to develop. Conventional blood culture is time-consuming and has a very low positive rate, which delays the diagnosis of F. necrophorum and timely targeted drug use. Therefore, more rapid and accurate methods are needed to identify F. necrophorum, guide early medication, and prevent the spread of infection.
In this case report, we describe a critical incident involving a 6-year-old female patient who developed fatal septic shock, predominantly attributed to a severe infection dominated by F. necrophorum. The pathogens were swiftly identified in the patient’s blood through the application of metagenomic next-generation sequencing (mNGS). This patient was eventually cured by targeted anti-infective therapy.
Case presentation
A 6-year-old female child was admitted to the pediatric intensive care unit (PICU) of West China Second University in December 2022. One day before admission, the patient’s main clinical symptom was hyperpyrexia (>39°C), accompanied by headache, neck pain, sore throat, dysphagia, occasional non-projectile vomiting, and anorexia. Later, those symptoms worsened with new manifestations, including hoarse voice, shortness of breath, mouth breathing, purple lips, accelerated heart rate, and disturbance of consciousness. After an initial emergency visit, she was quickly admitted to the PICU. The girl’s physical development was normal. She had no history of recurrent respiratory infections or other major illnesses since birth. She was vaccinated against COVID-19 and the flu a year ago. Furthermore, she had no history of prior hospitalizations or surgeries. None of her family members had reported an immunodeficiency or an autoimmune disorder. There was no history of pet ownership in her family.
This patient’s vital signs at admission included an increased body temperature (38.8°C), heart rate of 174 beats per minute, respiratory rate of 68 breaths per minute, and blood pressure of 91/52 mmHg. She has severe cyanosis, dyspnea, and oxygen saturation of 75% without oxygen. The Glasgow Coma score was 9 (E3M5V1). The oropharynx showed a markedly swollen bilateral tonsil with small purulent exudates (Figure 1). Three concave signs were positive. Inspiratory laryngeal stridor was detected on auscultation. Coarse rales were heard in both lungs. Laboratory investigations yielded the following findings: blood gas analysis indicative of type I respiratory failure; white blood cell count (WBC) at 5.43 × 109/L (reference range: 4.0 × 109/L–12.0 × 109/L) with a predominance of neutrophils (87.3% neutrophils and 8.4% lymphocytes). The hemoglobin (Hb) level measured 101 g/L (reference range: 120 g/L–160 g/L), and the platelet count (PLT) was 123 × 109/L (reference range: 100–300 × 109/L). The C-reactive protein (CRP) concentration was elevated to 29.15 mg/L (0 mg/L–6 mg/L), and that of procalcitonin was 29.52 ng/mL (<0.05 ng/mL). The troponin level was 0.55 ng/mL (more than 10 times higher than the normal value). Coagulation function was significantly abnormal: prothrombin time (PT) was 17.1 s, activated partial thromboplastin time (APTT) was 37.7 s, the international normalized ratio (INR) was 1.57, the D-dimer level was 3.13 mg/L, and the fibrinogen degradation product (FDP) level was 20.74 µg/mL (<5 µg/mL). The antistreptolysin O test (ASO) showed negative results. We collected the patient’s cerebrospinal fluid (CSF) by lumbar puncture (5 mL–10 mL). The counting of nucleated cells, cell classification, protein qualitative test, glucose quantification, chloride quantification, protein quantification, lactate dehydrogenase quantification, and culture of the CSF were negative. Chest CT results showed multiple small patchy hyperdense shadows and nodular shadows in both lungs (Figure 2A), and the laryngeal pharyngeal area was slightly narrow (Figure 2B). An abdominal CT revealed no liver abscesses or intestinal abnormalities. A head CT showed no abnormalities. There were no abnormalities on cervical vascular ultrasound or abdominal ultrasound.
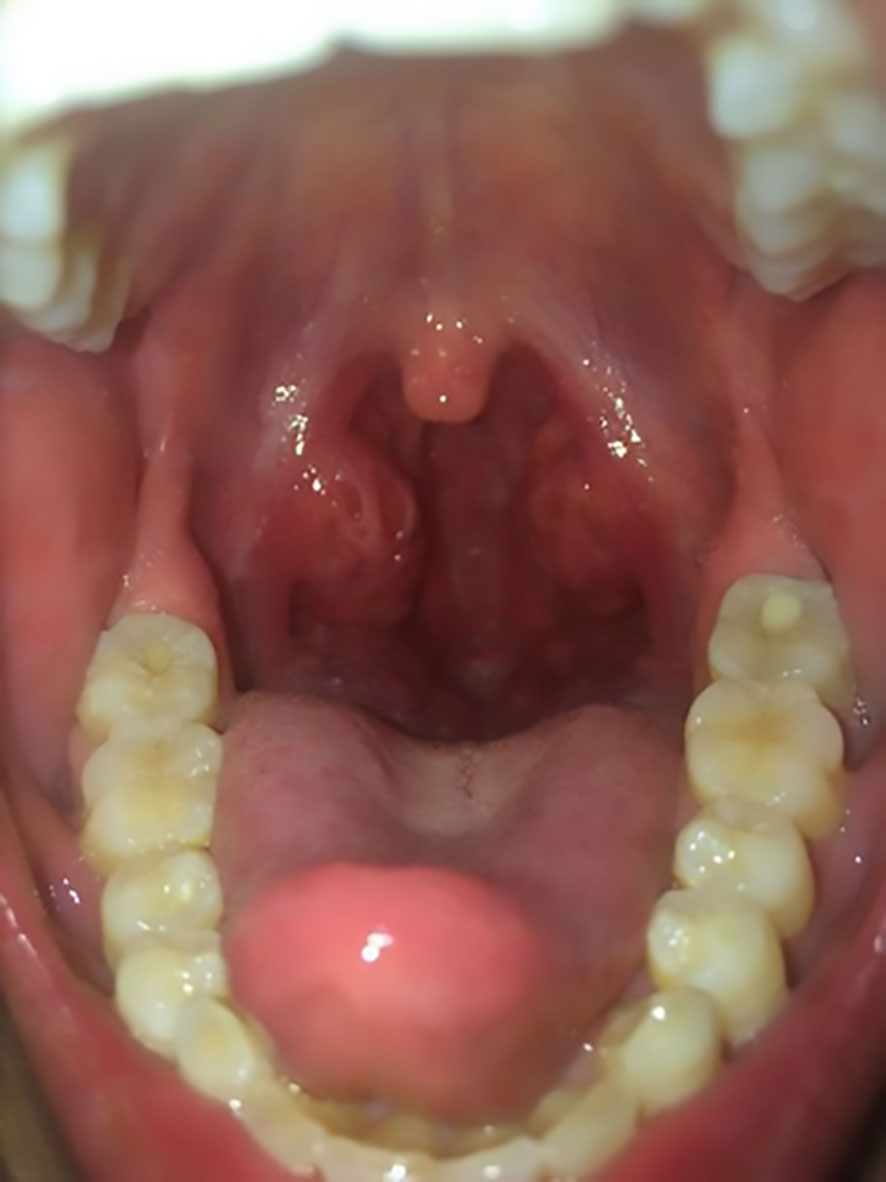
Figure 1 The patient’s oropharynx showed a markedly swollen bilateral tonsil with white, purulent exudates.
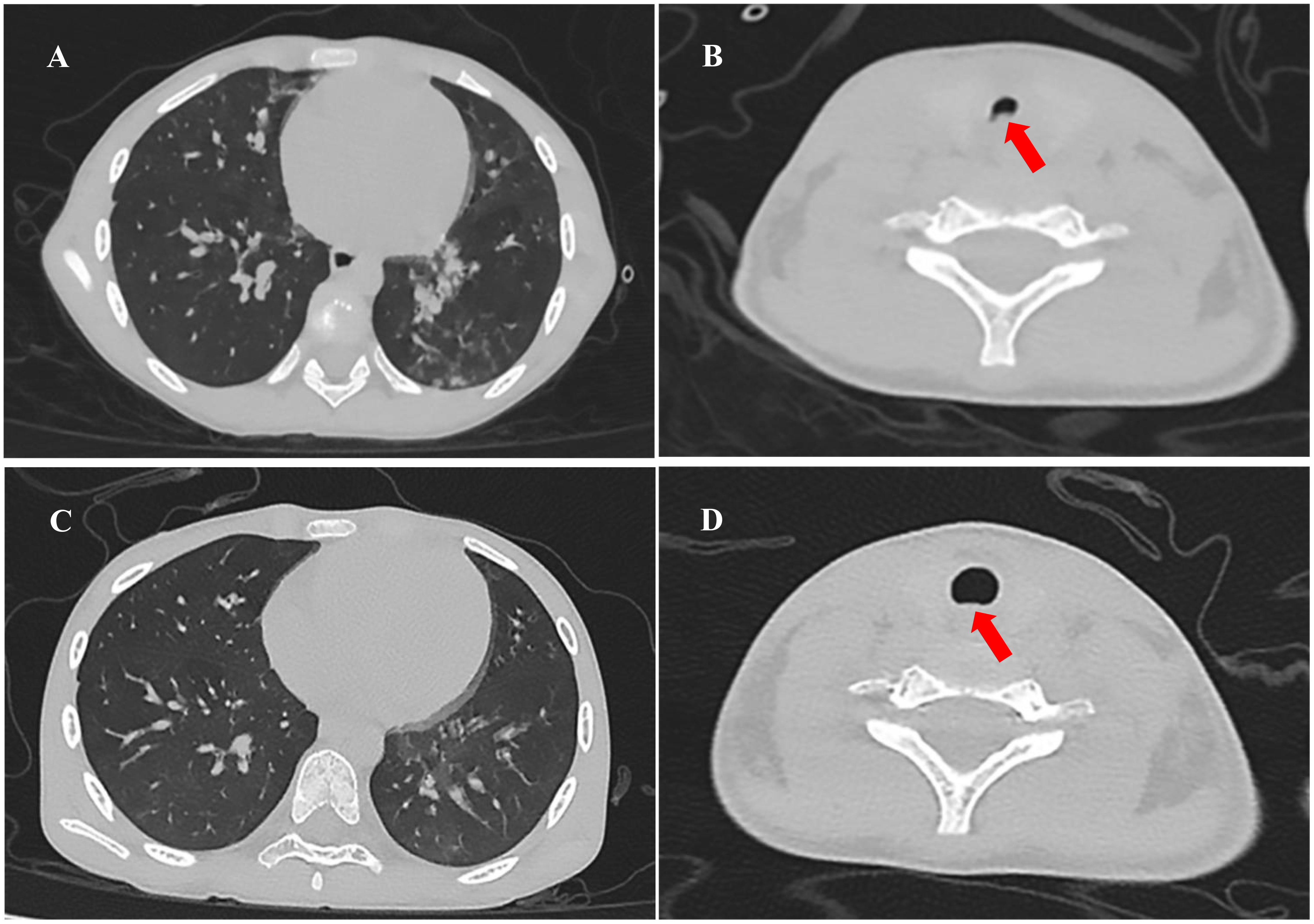
Figure 2 Chest CT in the clinical course of the patient: (A) Multiple small patchy hyperdense shadows and nodular shadows in both lungs (the day of admission). (B) The laryngeal–pharyngeal area was slightly narrow (red arrow, the day of admission). (C) Patchy hyperdense shadows were absorbed, and nodule shadows were significantly reduced (the 14th day of admission). (D) No evidence of internal jugular vein thrombophlebitis or abscess, the morphology of the laryngeal pharyngeal area returned to normal (red arrow, the 14th day of admission).
According to the above clinical symptoms and test parameters, the patient was preliminarily diagnosed with sepsis, severe pneumonia, acute laryngeal obstruction, myocardial damage, and coagulation disorders. She was immediately given a trachea cannula and subsequently treated with invasive mechanical ventilation. Meanwhile, she was empirically given ceftriaxone intravenous drip (50 mg/kg for 1 time, iv drip) combined with vancomycin (15 mg/kg q6h for 2 days, iv drip) for anti-infection, infusion of fresh frozen plasma, gamma globulin (1 g/kg qd for 2 days, iv drip) therapy, and other symptomatic treatments.
Within 24 h of admission to the PICU, the blood pressure decreased to 56/27 mmHg, and sinus tachycardia and cardiac auscultation showed low heart sounds with diastolic gallop rhythm. The capillary refill time (CRT) of the extremities was 4 s. Blood gas analysis suggested metabolic acidosis with hyperlactacidemia (6 mmol/L). Echocardiography indicated a decrease in left ventricular systolic function (EF 55%, FS 22%) with no evidence of endocarditis. The electrocardiogram showed sinus rhythm and incomplete right bundle branch block. Fluid resuscitation with 0.9% normal saline was performed, and vasoactive drugs were continuously pumped through the central vein to maintain hemodynamic stability: noradrenaline (0.05 μg/kg/min–0.1 μg/kg/min, continuous intravenous pumping) and dobutamine (5 μg/kg/min–10 μg/kg/min, continuous intravenous pumping). Based on the above performance and the relevant authoritative international clinical guidelines, the diagnosis was revised to septic shock and septic-associated cardiomyopathy (Singer et al., 2016). We switched from ceftriaxone to meropenem (40 mg/kg q8h for 14 days, iv drip) for anti-infective treatment and added phosphocreatine infusion for myocardial protection. On the second day of admission, reexamination of the laboratory blood report showed that CRP increased to 116.66 mg/L and procalcitonin increased to 56.30 ng/mL. As the coagulation function test suggested a hypercoagulable state, we gave the patient anticoagulation treatment with low-molecular-weight heparin (75 iu/kg q12h for 12 days, subcutaneous injection). The nucleic acid test by PCR of multiple respiratory pathogens, including the human influenza virus, rhinovirus, adenovirus, para influenza virus, metapneumovirus, SARS-CoV-2, Mycoplasma pneumoniae, and Chlamydia pneumoniae, showed negative results. On the third day of admission, Pathogen Capture Engine (PACE) seq mNGS (Hugo Biotech, Beijing, China) of blood detected F. necrophorum with 12 specific sequences and a relative abundance of 1.47%. Meanwhile, a variety of microbes were detected by mNGS in the blood sample (Figure 3). The sepsis and associated diseased condition in this case were likely attributable to a microbial consortium, composed of multiple microorganisms that cooperated with F. necrophorum. Among these, F. necrophorum was identified as the principal pathogenic microorganism responsible for the sepsis in the patient. The patient was referred with a diagnosis of sepsis with a bloodstream infection. We changed the antibiotic regimen to metronidazole (7.5 mg/kg q8h for 12 days, iv drip) and meropenem to suppress F. necrophorum and other pathogens.
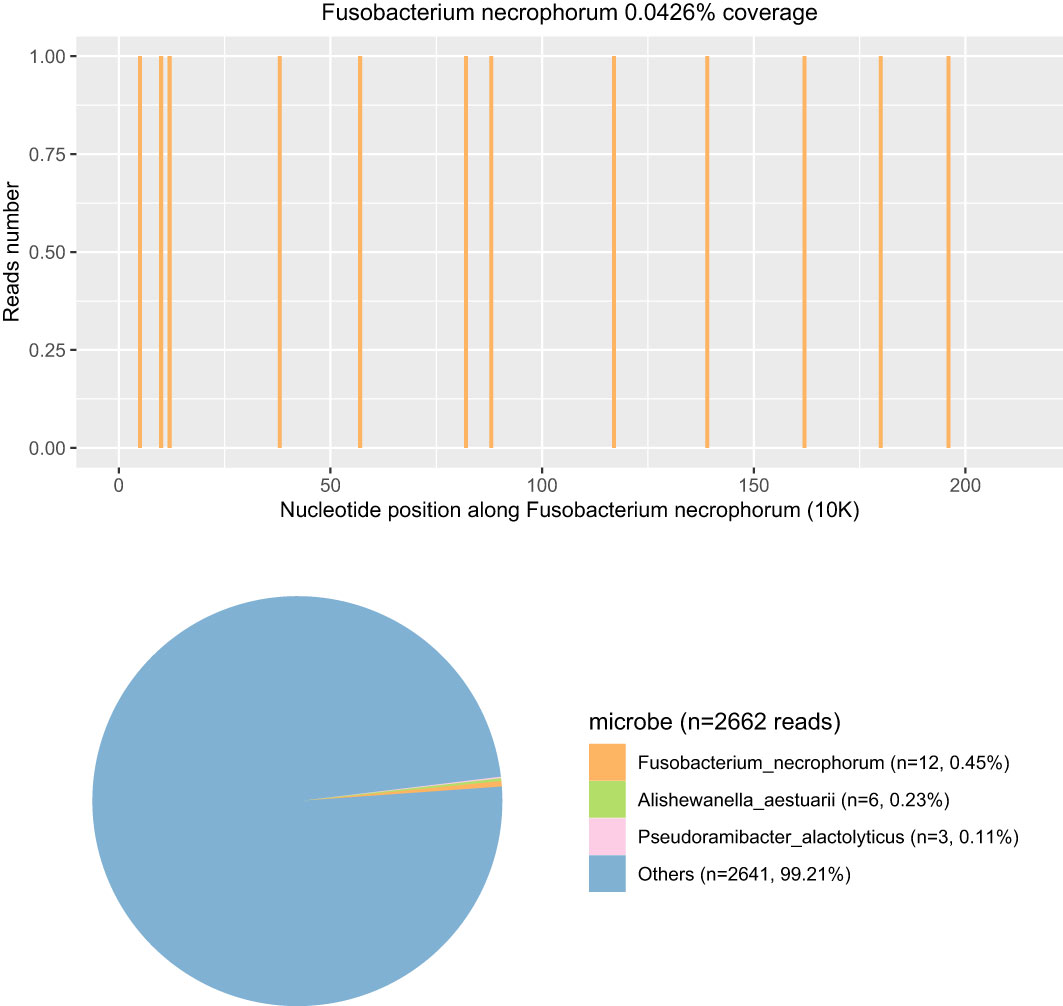
Figure 3 The coverage and abundance of F. necrophorum detected by mNGS using blood on the third day of admission. In the Others section, the total number of sequences was 2,642 reads and the percent was 99.21%, including Prevotella, Acinetobacter, Stenotrophomonas, and Neisseria (the human reads were removed).
On the fifth day of admission, throat swab culture revealed a positive result: a small amount of F. necrophorum and Streptococcus viridans. Throat swabs were collected on the first day of admission (required and anaerobic bacteria; the culture lasted for 5 days). Matrix-assisted laser desorption/ionization-time of flight mass spectrometry (MALDI-TOF MS; bioMérieux, France) was used to identify the cultured microbes. The patient switched to high-flow nasal cannula oxygen therapy as her spontaneous breathing had returned and laryngeal obstruction had resolved. Her heart function recovered (EF 63%, FS 33%), and vasoactive agents were no longer required to maintain blood pressure. Her temperature remained normal for 3 days, and blood tests showed that inflammatory indicators decreased (CRP 20.37 mg/L, PCT 3.48 ng/mL); coagulation function improved significantly. We performed two blood cultures on the first and third days of admission. In each blood culture collection, blood samples of the left peripheral vein and the right central vein (femoral vein) were collected for blood culture at the same time; that is, two vials of blood culture from different parts were collected at the same time. All blood samples were cultured for 7 days, and both aerobic and anaerobic cultures were performed. On the 10th day, the patient’s two blood cultures showed negative results, respectively. Following a 14-day stay in the PICU, there was a notable improvement in the patient’s condition: dependency on supplemental oxygen ceased, vital signs and infection markers normalized, and chest CT scan revealed absorption of previously observed patchy hyperdense shadows and significant reduction in nodule shadows (Figure 2C). Additionally, there was no evidence of internal jugular vein thrombophlebitis or abscess formation. The morphology of the laryngeal pharyngeal area returned to normal (Figure 2D). Then, she was discharged for outpatient follow-up for 3 months. Sequential oral metronidazole was continued at discharge (7.5 mg/kg tid for 14 days, po). During the follow-up, there were no signs of infection such as high fever, angina, and dyspnea. There were no neurological or respiratory complications. The inflammatory indicators of WBC, CRP, PCT, and echocardiography were normal. Bilateral cervical ultrasound showed no internal jugular vein thrombosis, and blood cultures were negative twice. The timeline of disease progression and treatment is shown in Figure 4A.
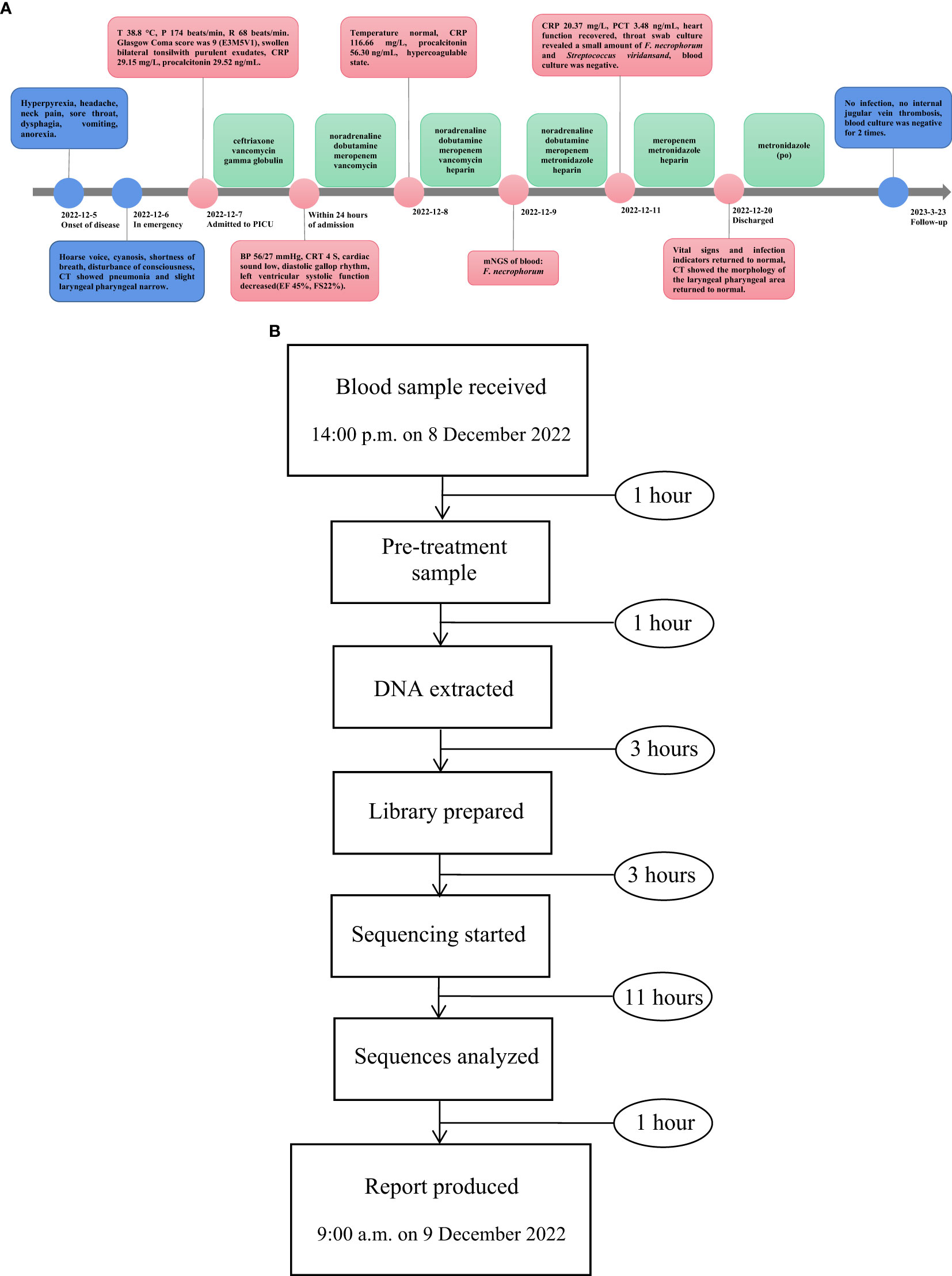
Figure 4 (A) The timeline of disease progression and treatment. (B) The flowchart of the mNGS process.
System review
As of 20/05/2022, we have searched the literature of the recent decade in PubMed, Web of Science, Springer Link, and Medline databases with the keywords “Fusobacterium necrophorum” and “sepsis”. Patients aged above 18 years were excluded from the study. Finally, a total of 12 articles were selected, and all the clinical data were collected and analyzed, including baseline data, clinical symptoms, means of detection, complications, treatment, and outcome. The characteristics of reported cases are shown in Table 1 (Sheehan et al., 2019; Gama et al., 2020; Tamura et al., 2020; El Chebib et al., 2020; Repper et al., 2020; Monroe and Amlie-Lefond, 2018; Dal Bo et al., 2019; Whittle et al., 2018; Rana et al., 2017; Sinatra and Alander, 2017; He et al., 2015; Creemers-Schild et al., 2014).
Sample collection and nucleic acid extraction
Enough whole blood (children, 2 mL–4 mL) was collected in Cell-Free DNA BCT Streck and then stored or shipped between 6 °C and 35 °C to perform mNGS detection immediately. The DNA was extracted and purified from 200 μL plasma according to the manufacturer’s instructions of QIAamp DNA Micro Kit (50) #56304. DNA concentration and quality were checked through Qubit and agarose gel electrophoresis.
Library generation and sequencing
The DNA was subjected to library construction through an end-repair method. The DNA libraries were constructed using QIAseq™ Ultralow Input Library Kit. The concentration and quality of libraries were checked using Qubit and agarose gel electrophoresis. Qualified libraries with different barcode labelings were pooled together and then sequenced on an Illumina NextSeq platform. Qualified DNA libraries with different barcode tags were pooled and then sequenced using the Illumina NextSeq 550 sequencing platform (Illumina, San Diego, USA) and a SE75bp sequencing strategy. Shotgun metagenomic sequencing was used in this case.
Bioinformation pipeline
After obtaining the sequencing data, high-quality data were generated after filtering out adapter, low-quality, low-complexity, and shorter reads. Next, human reads were removed by mapping reads to the human reference genome using SNAP software. The remaining data were aligned to the microbial genome database using Burrows–Wheeler alignment. The database collected microbial genomes from NCBI. It contains more than 20,000 microorganisms, including 11,910 bacteria, 7,103 viruses, 1,046 fungi, and 305 parasites. Finally, the microbial composition of the sample was obtained. In this case, 14 reads of F. necrophorum were detected by mNGS, of which 12 were mapped to F. necrophorum and 2 reads were mapped to Fusobacterium at the genus level. The identity of detected reads to the reference sequences ranged from 98% to 100%. The average read length was 75bp. The flow chart of the mNGS process is shown in Figure 4B.
Positivity criteria
Sterile deionized water was used as the no template control (NTC) and synthesized fragments with known quantities as a positive control (PC). NTC and PC were included in each wet lab procedure and bioinformatics analysis as quality control steps. The positivity criteria of mNGS were as follows: Viruses with non-overlapping reads mapping three distinct genomic regions were reported as positive. For bacteria and fungi (Mycobacteria and Cryptococcus were excluded), a positive mNGS result was given when its coverage ranked among the top 10 of the same kind of microbes and was absent in the NTC or when its ratio of reads per million (RPM) between sample and NTC (RPMsample/RPMNTC) > 10 if RPMNTC≠0. For M. tuberculosis and Cryptococcus, a positive mNGS result was considered when at least one unique read was mapped to species level and absent in NTC or RPMsample/RPMNTC > 5 when RPMNTC≠0.
Discussion
F. necrophorum constitutes a significant representative species within the Fusobacterium genus. It accounts for a minute fraction (less than 1%) of human infections caused by non-spore-forming anaerobic bacteria, and the literature only contains a few hundred case reports; there are even rarer cases in pediatrics (Carius et al., 2022). However, it is arguably unparalleled among non-spore-forming anaerobes due to its exceptionally strong association with clinically distinctive and severe septicemic infections that are referred to as Lemierre’s syndrome (Almohaya et al., 2020; Lee et al., 2020; Carius et al., 2022; Sheehan et al., 2019). In the pre-antibiotic era, this syndrome had a high mortality rate of up to 90%, with patients succumbing to a fatal course within 7–15 days. However, early and targeted use of antibiotics can greatly reduce the death rate from the disease (Almohaya et al., 2020; Lee et al., 2020; Carius et al., 2022; Riordan, 2007; Sacco et al., 2019; Nygren and Holm, 2020).
Leukocytotoxin, endotoxin, hemolysin, and hemagglutinin are the bacterial virulence factors of F. necrophorum, which have strong biological activity (Brennan and Garrett, 2019). In addition to its role in inducing platelet aggregation, which results in venous thrombosis and septic thrombophlebitis, this entity also plays a pivotal role in the development of metastatic septic embolization and the formation of characteristic abscesses (Riordan, 2007; Sheehan et al., 2019; Sacco et al., 2019). The primary infection site of F. necrophorum is commonly in the oropharynx, from which the infection spreads to surrounding tissues. Once F. necrophorum invades the internal jugular vein and enters the bloodstream, bacteremia can cause platelet aggregation and thrombosis, facilitating the further spread of infection and the development of metastatic septic embolism and metastatic abscess (Lee et al., 2020; Sheehan et al., 2019; Sacco et al., 2019; Tay and Vasoo, 2022). The phenomenon that distant bacterial transmission can directly impair prognosis is considered to be the main cause of severe infection in adult patients (Sacco et al., 2019). However, in addition to its propensity to cause abscesses or thrombosis, F. necrophorum carries strong adhesins and pili, resulting in its remarkable ability to adhere to gram-negative bacteria and gram-positive plaque microorganisms in biofilms, making it a highly invasive opportunistic infectious agent. For pediatric patients, as the immune function was not complete, F. necrophorum was more direct and rapid-invading (Brennan and Garrett, 2019). Due to the high virulence and invasiveness of the bacteria, the body would directly respond to severe sepsis, leading to hemodynamic failure and multiple-organ failure prior to internal jugular vein thrombosis and septic embolism (Sheehan et al., 2019; Tamura et al., 2020; El Chebib et al., 2020; Habib et al., 2019). In this case, in the absence of suppurative thrombophlebitis of the internal jugular vein, the F. necrophorum infection progressed very rapidly, leading to septic shock within 24 h and causing life-threatening failure of vital organs, including respiratory failure and heart failure. It is suggested that the pathophysiological changes and development of the disease in children infected with F. necrophorum may be different from those in adults. In the pathogenesis of sepsis, F. necrophorum exhibits synergistic interactions with other bacterial species, contributing to the disease’s progression. This synergism is primarily facilitated by the bacterium’s adhesin (FadA) and fimbriae, which enable adherence to host cells and soft tissues. Moreover, F. necrophorum can form extensive biofilms with various bacteria, such as Escherichia coli and Streptococcus hemolyticus, enhancing their proliferation. This co-aggregation promotes the invasion of typically non-invasive bacterial species, exacerbating sepsis and leading to multiple-organ dysfunction (Lee et al., 2020; Brennan and Garrett, 2019; Groeger et al., 2022). Additionally, F. necrophorum’s impact extends to disrupting the host’s normal microbiota, such as the intestinal and oral flora. This disruption results in an imbalance of the microbiota, further modulating the host immune response, thereby aggravating the infection and facilitating its spread (Hou et al., 2022). In this case, we considered that the septic shock of the child was due to a complex bacterial infection dominated by F. necrophorum.
The etiological identification of the disease often relies on bacterial culture. Blood culture is important diagnostic evidence. However, it is difficult to make a timely and accurate diagnosis of F. necrophorum infection by blood culture because of its obligate anaerobic condition, harsh culture conditions, long culture time (mean 6–8 days), and low positive rate (Riordan, 2007; Sacco et al., 2019; Sato et al., 2021). Through a review of the literature in Table 1, we found that only 12 cases of F. necrophorum infection have been successfully confirmed in children. Only one patient was under 12 years of age. The detection methods were all conventional bacterial culture methods, and the time from onset to diagnosis of the pathogen was 6–28 days. PCR technology is limited to the detection of anaerobic bacteria. At present, PCR is not routinely used to identify F. necrophorum. Sepsis in children caused by F. necrophorum may occur within 3 days after infection. Earlier recognition and treatment are imperative because of the possible extrapharyngeal sequalae and high mortality in the absence of antimicrobial treatment. Therefore, there is an urgent need for a more sensitive and accurate test to screen for the infection of F. necrophorum.
mNGS refers to high-throughput sequencing of the entire genome of organisms in a specimen, which is a new technology that can deeply and rapidly identify infectious pathogens (Bharucha et al., 2020). Studies have reported that the consistency between mNGS and blood culture results can reach 93.7%, which is more effective than other detection methods in the identification of pathogens causing sepsis (Timsit et al., 2020; Blauwkamp et al., 2019). In addition, the overall positive rate of mNGS sequencing is significantly higher than that of body fluid culture, which can identify potential bacterial pathogens missed by traditional culture methods (Hogan et al., 2021; Li et al., 2021). When interpreting mNGS results, we usually make a comprehensive judgment to distinguish true-positive and false-positive pathogens based on sequence number, coverage, abundance, and documentary evidence. The expert consensus recommended 20 million reads as average sequencing data for mNGS. In this sample, the amount of sequencing data was 45,185,658, which demonstrates that the data volume for mNGS sequencing was sufficient. However, the host abundance ratio achieved as high as 99.89%, leading to a low read number of microbes (<3k reads). Therefore, efficient host depletion is essential to mNGS. In this case, mNGS from the blood showed that the patient was infected with F. necrophorum, indicating moderate confidence. mNGS had further identified an array of additional pathogens. Significantly, F. necrophorum was recognized as the relative dominant species in the clearly defined species, followed by Alishewanella aestuarii and Pseudoramibacter alactolyticus. The entire microbial consortia promoted the development of sepsis. Throat swab culture results obtained 5 days after admission indicated F. necrophorum and Streptococcus viridans, which verified the accuracy of the mNGS results. Streptococcus vermicularis, as the most common colonizing bacteria in the human throat, was not considered a pathogenic bacterium here. In addition, the detection time of mNGS is shorter than that of culture, so infection can be confirmed as soon as possible and targeted medication can be directed. Currently, mNGS testing time can be cut down to 24 h. In addition to mNGS, 16S amplicon sequencing can quickly and efficiently identify bacteria as well, such as anaerobic bacteria. However, the species detected by 16S amplicon sequencing are mainly bacteria. The metabolic pathway, infection degree, virulence, and other properties of microorganisms cannot be detected by 16S amplicon sequencing. Therefore, in this case, since the pathogen type could not be determined, we could not only detect the bacteria by 16S amplicon sequencing and ignore other types of pathogens. Although reliable drug susceptibility information cannot be obtained, it should still be used as an important supplement to traditional pathogen detection, especially for such critical case as community-acquired infections (Timsit et al., 2020; Blauwkamp et al., 2019; Hogan et al., 2021; Li et al., 2021).
Early application of targeted antibiotics is a key factor affecting the prognosis of F. necrophorum infection. Antibiotic therapy with anaerobic coverage must be rapidly introduced (Nygren and Holm, 2020). As F. necrophorum contains β-lactamase, it is naturally resistant to quinolones and aminoglycosides, as well as having poor sensitivity to macrolides and tetracycline. Metronidazole is recommended in combination with β-lactam antibiotics (Sacco et al., 2019; Tay and Vasoo, 2022). In current research, the average duration of antibiotic use was as long as 2–4 weeks, and some severe patients were treated for 8–16 weeks (Sheehan et al., 2019; Gama et al., 2020; Tamura et al., 2020; El Chebib et al., 2020; Repper et al., 2020; Monroe and Amlie-Lefond, 2018; Dal Bo et al., 2019; Whittle et al., 2018; Rana et al., 2017; Sinatra and Alander, 2017; He et al., 2015; Creemers-Schild et al., 2014). In this case, to cover the anaerobic bacteria as well as some pathogenic or coinfected G-bacilli and streptococci, the broad-spectrum carbapenem antibiotics were initially used empirically. The use of mNGS to accurately detect pathogens at the early stage of the disease guided the transformation of the anti-infection program from empirical to target. Targeted use of metronidazole for anti-infection began before complications such as Lemierre’s syndrome appeared. This regimen, which was used early, shortened the course of anti-infective treatment and achieved good clinical efficacy, with no drug-related toxic reactions occurring. The hypercoagulable state of F. necrophorum infection leads to a high risk of thrombosis, and the timing of anticoagulant therapy is controversial (Lee et al., 2020; Carius et al., 2022). Recent studies suggested that early use of anticoagulants can help prevent the occurrence of disseminated intravascular coagulation (DIC) and Lemierre’s syndrome caused by sepsis (Tamura et al., 2020; Hayakawa et al., 2016). This patient was treated with low-molecular-weight heparin for anticoagulation in the early stage. There was no sign of jugular vein thrombosis or septic embolism in the long-term follow-up. More proactive anticoagulant therapy and individualized anticoagulant regimens may be considered for such infection, which can bring more prognostic benefits.
There are still some limitations to the case report. Rapid identification of the pathogen and its susceptibility to antibiotics are crucial steps in sepsis treatment. In this case, mNGS only detected F. necrophorum, but its drug resistance could not be determined. In addition, human infection with F. necrophorum usually involves F. necrophorum subsp. funduliforme, which we were unable to identify in this case. PCR has potential advantages in the identification of subspecies of F. necrophorum. In a study of adult patients with tonsillitis, Jensen et al. (2007) used a novel F. necrophorum subspecies-specific real-time PCR assay to compare the results and found that 51% of 105 patients with tonsillitis were positive for F. necrophorum subsp. funduliforme, compared with 21% of the control group. In future studies, we can pay more attention to the identification of resistance in mNGS to F. necrophorum and pathogenic subspecies. More similar cases can also be collected to explore the advantages and disadvantages of mNGS in the detection of F. necrophorum.
Conclusion
F. necrophorum infection is rare in children, which can lead to serious sepsis and high mortality. Early treatment with targeted antibiotics is generally effective. The use of mNGS can deeply and rapidly identify infectious pathogens, which is more sensitive than the traditional culture method. It also has good clinical significance for the treatment of antibacterial drugs and can remarkably improve the survival rate of patients.
Data availability statement
The datasets presented in this study can be found in online repositories. The names of the repository/repositories and accession number(s) can be found in the article/Supplementary Material.
Ethics statement
The studies involving humans were approved by West China Second University Hospital, Sichuan University. The studies were conducted in accordance with the local legislation and institutional requirements. Written informed consent for participation in this study was provided by the participants’ legal guardians/next of kin. Written informed consent was obtained from the individual(s), and minor(s)’ legal guardian/next of kin, for the publication of any potentially identifiable images or data included in this article.
Author contributions
LR summarized the case information and designed the manuscript. HZ drafted the manuscript. ZL analyzed the data. YG designed the bioinformatic analysis. DL and HL revised the manuscript. All authors contributed to the article and approved the submitted version.
Funding
This research was supported by the Sichuan Medical Youth Innovative Research Project (No. Q22028).
Acknowledgments
The authors thank all medical staff involved in the treatment of this patient and the implementation of this study.
Conflict of interest
Author YG is employed by Hugobiotech Co., Ltd.
The remaining authors declare that the research was conducted in the absence of any commercial or financial relationships that could be construed as a potential conflict of interest.
Publisher’s note
All claims expressed in this article are solely those of the authors and do not necessarily represent those of their affiliated organizations, or those of the publisher, the editors and the reviewers. Any product that may be evaluated in this article, or claim that may be made by its manufacturer, is not guaranteed or endorsed by the publisher.
Supplementary material
The Supplementary Material for this article can be found online at: https://www.frontiersin.org/articles/10.3389/fcimb.2024.1236630/full#supplementary-material
References
Almohaya, A. M., Almutairy, T. S., Alqahtani, A., Binkhamis, K., Almajid, F. M. (2020). Fusobacterium bloodstream infections: A literature review and hospital-based case series. Anaerobe 62, 102165. doi: 10.1016/j.anaerobe.2020.102165
Bharucha, T., Oeser, C., Balloux, F., Brown, J. R., Carbo, E. C., Charlett, A., et al. (2020). STROBE-metagenomics: a STROBE extension statement to guide the reporting of metagenomics studies. Lancet Infect. Dis. 20, e251–e260. doi: 10.1016/S1473-3099(20)30199-7
Blauwkamp, T. A., Thair, S., Rosen, M. J., Blair, L., Lindner, M. S., Vilfan, I. D., et al. (2019). Analytical and clinical validation of a microbial cell-free DNA sequencing test for infectious disease. Nat. Microbiol. 4, 663–674. doi: 10.1038/s41564-018-0349-6
Brennan, C. A., Garrett, W. S. (2019). Fusobacterium nucleatum-symbiont, opportunist and oncobacterium. Nat. Rev. Microbiol. 17, 156–166. doi: 10.1038/s41579-018-0129-6
Carius, B. M., Koyfman, A., Long, B. (2022). High risk and low prevalence diseases: Lemierre’s syndrome. Am. J. Emerg. Med. 61, 98–104. doi: 10.1016/j.ajem.2022.08.050
Creemers-Schild, D., Gronthoud, F., Spanjaard, L., Visser, L. G., Brouwer, C. N., Kuijper, E. J. (2014). Fusobacterium necrophorum, an emerging pathogen of otogenic and paranasal infections? New Microbes New Infect. 2, 52–57. doi: 10.1002/nmi2.39
Dal Bo, S., Calandriello, L., Biserna, L., Mantero, F., Minguzzi, T., Marchetti, F., et al. (2019). A sepsis that you don’t expect Lemierre’s syndrome. Description of a clinical case. Recenti Progressi Medicina 110, 543–547. doi: 10.1701/3265.32329
El Chebib, H., McArthur, K., Gorbonosov, M., Domachowske, J. B. (2020). Anaerobic necrotizing pneumonia: another potential life-threatening complication of vaping? Pediatrics 145, e20193204. doi: 10.1542/peds.2019-3204
Gama, R., Sousa, M., Castro, F. (2020). Lemierre’s syndrome: a forgotten life-threatening entity. BMJ Case Rep. 13, e236201. doi: 10.1136/bcr-2020-236201
Groeger, S., Zhou, Y., Ruf, S., Meyle, J. (2022). Pathogenic mechanisms of fusobacterium nucleatum on oral epithelial cells. Front. Oral. Health 3. doi: 10.3389/froh.2022.831607
Habib, S., Rajdev, K., Siddiqui, A. H., Azam, M., Memon, A., Chalhoub, M. (2019). Septic emboli of the lung due to Fusobacterium necrophorum, a case of Lemierre’s syndrome. Respir. Med. Case Rep. 28, 100867. doi: 10.1016/j.rmcr.2019.100867
Hayakawa, M., Saito, S., Uchino, S., Yamakawa, K., Kudo, D., Lizuka, Y., et al. (2016). Characteristics, treatments, and outcomes of severe sepsis of 3195 ICU-treated adult patients throughout Japan during 2011-2013. J. Intensive Care 4, 44. doi: 10.1186/s40560-016-0169-9
He, J., Lam, J. C., Adlan, T. (2015). Clival osteomyelitis and hypoglossal nerve palsy–rare complications of Lemierre’s syndrome. BMJ Case Rep. 2015, bcr–2015-209777. doi: 10.1136/bcr-2015-209777
Hogan, C., Yang, S. X., Garner, O. B., Green, D. A., Gomez, C. A., Bard, J. D., et al. (2021). Clinical impact of metagenomic next-generation sequencing of plasma cell-free DNA for the diagnosis of infectious diseases: A multicenter retrospective cohort study. Clin. Infect. Dis. 72, 239–245. doi: 10.1093/cid/ciaa035
Hou, K., Wu, Z. X., Chen, X. Y., Wang, J. Q., Zhang, D., Xiao, C., et al. (2022). Microbiota in health and diseases. Signal Transduct Target Ther. 7, 135. doi: 10.1038/s41392-022-00974-4
Jensen, A., Kristensen, L. H., Prag, J. (2007). Fusobacterium necrophorum in young patients with tonsillitis and healthy controls examined by real-time PCR. Clin. Microbiol. Infect. 13, 695–701. doi: 10.1111/j.1469-0691.2007.01719.x
Lee, W. S., Jean, S. S., Chen, F. L., Hsieh, S. M., Hsueh, P. R. (2020). Lemierre’s syndrome: A forgotten and re-emerging infection. J. Microbiol. Immunol. Infect. 53, 513–517. doi: 10.1016/j.jmii.2020.03.027
Li, N., Cai, Q. Q., Miao, Q., Song, Z. S., Fang, Y., Hu, B. J. (2021). High-throughput metagenomics for identification of pathogens in the clinical settings. Small Methods 5, 2000792. doi: 10.1002/smtd.202000792
Monroe, E. J., Amlie-Lefond, C. M. (2018). Cone beam computed tomography-guided transpterygoidal aspiration of a carotid space abscess in Lemierre’s syndrome. Radiol. Case Rep. 13, 618–621. doi: 10.1016/j.radcr.2018.03.007
Nygren, D., Holm, K. (2020). Invasive infections with Fusobacterium necrophorum including Lemierre’s syndrome: an 8-year Swedish nationwide retrospective study. Clin. Microbiol. Infect. 26, 1089.e7–1089.e12. doi: 10.1016/j.cmi.2019.12.002
Rana, M. A., Kumar, Y., Lashari, A. A., Mady, A. F. (2017). Human Infection with Fusobacterium necrophorum without Jugular Venous Thrombosis: A Varied Presentation of Lemierre’s Syndrome. Case Rep. Infect. Dis. 2017, 5358095. doi: 10.1155/2017/5358095
Repper, D. C., Arrieta, A. C., Cook, J. E., Renella, P. (2020). A case of lemierre syndrome in the era of COVID-19: all that glitters is not gold. Pediatr. Infect. Dis. J. 39, e445–e447. doi: 10.1097/INF.0000000000002939
Riordan, T. (2007). Human infection with Fusobacterium necrophorum (Necrobacillosis), with a focus on Lemierre’s syndrome. Clin. Microbiol. Rev. 20, 622–659. doi: 10.1128/CMR.00011-07
Sacco, C., Zane, F., Granziera, S., Holm, K., Creemers-Schild, D., Hotz, M. A., et al. (2019). Lemierre syndrome: clinical update and protocol for a systematic review and individual patient data meta-analysis. Hamostaseologie 39, 76–86. doi: 10.1055/s-0038-1654720
Sato, K., Matsubara, T., Imai, S., Hatada, K., Mitsuma, W., Kawasaki, S., et al. (2021). Fusobacterium necrophorum endocarditis with liver abscesses: A case report and review of the literature. Intern. Med. 60, 2445–2449. doi: 10.2169/internalmedicine.6348-20
Sheehan, M., McLoughlin, D., O’Sullivan, R. (2019). Fusobacterium necrophorum sepsis after tonsillitis/pharyngitis. BMJ Case Rep. 12, e222287. doi: 10.1136/bcr-2017-222287
Sinatra, P. M., Alander, D. H. (2017). Lemierre disease: A case with multilevel epidural abscess and aggressive neurological weakness: case report and literature review. J. Pediatr. Orthop. 37, e58–e61. doi: 10.1097/BPO.0000000000000652
Singer, M., Deutschman, C. S., Seymour, C. W., Shankar-Hari, M., Annane, D., Bauer, M., et al. (2016). The third international consensus definitions for sepsis and septic shock (Sepsis-3). JAMA 315, 801–810. doi: 10.1001/jama.2016.0287
Tamura, S., Jwa, S. C., Tarumoto, N. (2020). Septic Shock Caused by Fusobacterium Necrophorum after Sexual Intercourse during Recovery from Infectious Mononucleosis in an Adolescent: A Case Report. J. Pediatr. Adolesc. Gynecol. 33, 566–569. doi: 10.1016/j.jpag.2020.06.018
Tay, R. W. H., Vasoo, S. (2022). Fusobacterium necrophorum pharyngitis complicated by sepsis and extrapharyngeal dissemination in two young adults. Singapore Med. J. 64, 768–769. doi: 10.11622/smedj.2022084
Timsit, J. F., Ruppé, E., Barbier, F., Tabah, A., Bassetti, M. (2020). Bloodstream infections in critically ill patients: an expert statement. Intensive Care Med. 46, 266–284. doi: 10.1038/s41564-018-0349-6
Keywords: Fusobacterium necrophorum, sepsis, children, metagenomics next-generation sequencing, case report
Citation: Zhang H, Liu Z, Guan Y, Li D, Liu H and Ruan L (2024) Case report: Metagenomics next-generation sequencing in the diagnosis of septic shock due to Fusobacterium necrophorum in a 6-year-old child. Front. Cell. Infect. Microbiol. 14:1236630. doi: 10.3389/fcimb.2024.1236630
Received: 08 June 2023; Accepted: 26 January 2024;
Published: 16 February 2024.
Edited by:
Yair Dorsett, University of Connecticut Health Center, United StatesReviewed by:
Patrycja Leśnik, 4th Military Hospital of Wroclaw, PolandNazima Habibi, Kuwait Institute for Scientific Research, Kuwait
Copyright © 2024 Zhang, Liu, Guan, Li, Liu and Ruan. This is an open-access article distributed under the terms of the Creative Commons Attribution License (CC BY). The use, distribution or reproduction in other forums is permitted, provided the original author(s) and the copyright owner(s) are credited and that the original publication in this journal is cited, in accordance with accepted academic practice. No use, distribution or reproduction is permitted which does not comply with these terms.
*Correspondence: Lingying Ruan, MTUwMjM0MDE5ODhAMTYzLmNvbQ==