- 1Department of Hematology, Tongji Hospital, Tongji Medical College, Huazhong University of Science and Technology, Wuhan, Hubei, China
- 2Key Laboratory of Organ Transplantation, Ministry of Education, NHC Key Laboratory of Organ Transplantation, Key Laboratory of Organ Transplantation, Chinese Academy of Medical Sciences, Wuhan, China
- 3Immunotherapy Research Center for Hematologic Diseases of Hubei Province, Wuhan, Hubei, China
Background: Timely diagnosis and appropriate antifungal therapy are critical for improving the prognosis of patients with invasive fungal disease (IFD) after hematopoietic stem cell transplantation (HSCT). We evaluated the performance of metagenomic next-generation sequencing (mNGS) and conventional microbiological testing (CMT), as well as the diagnosis, therapeutic management, and outcomes of IFD after HSCT.
Methods: We retrospectively studied 189 patients who underwent HSCT and were considered at risk for IFD. In total, 46 patients with IFD were enrolled in this study. The IFD consensus was followed for classifying IFD incidents.
Results: Forty-six patients were diagnosed with proven/probable (n = 12), possible (n = 27), and undefined (n = 7) IFD. Aspergillus was the most commonly detected fungal genus. Mucormycosis was found in 15 patients; two had Aspergillus, and one had Candida infections. Compared to CMT, mNGS significantly reduced the time required to identify pathogens (P = 0.0016). mNGS had a much higher sensitivity than CMT (84.78% vs. 36.96%; P < 0.0001). A total of 76.09% of patients received antifungal prophylaxis during fungal infections. All Pneumocystis infections occurred later than 100 days after transplantation. Among patients with Pneumocystis infection, 71.43% occurred following sulfonamide withdrawal, and subsequent treatment with sulfonamide alone or in combination with other drugs was effective. Based on the empirical antifungal treatment, the dosages, modes of administration, frequency of administration, or antifungal of 55.26% of the patients were changed according to the mNGS results. The 4-year overall survival rate of patients diagnosed with IFD after transplantation was 71.55% (95% CI, 55.18%–85.82%). Hypoproteinemia and corticosteroid use are independent risk factors for IFD.
Conclusion: mNGS, which has a high sensitivity and a short detection time, aids in the diagnosis and prognosis of pathogenic fungi. As a powerful technology, mNGS can influence treatment decisions in patients with IFD following HSCT.
1 Introduction
Invasive fungal disease (IFD) is a common complication after hematopoietic stem cell transplantation (HSCT) and a leading cause of transplant-related mortality (Pagano et al., 2007; Sun et al., 2015). Patients experience conditioning regimens, agranulocytosis, impaired mucosal barrier, use of central venous catheterization, graft-versus-host disease (GVHD), immunosuppression, and delayed immune reconstitution, which all significantly increase the risk of IFD (Kuster et al., 2018; Sun et al., 2021). Advances in microbiological techniques and antifungal drugs have resulted in improvements in IFD diagnosis and treatment (Akan et al., 2013; Tissot et al., 2017; Chinese Association, H and Chinese Invasive Fungal Infection Working, G, 2020; Ruhnke et al., 2020; Chinese Society Of Hematology and Chinese Medical Association, Antimicrobial Infection Branch, 2023). The specific symptoms and adequate diagnostic procedures for IFD remain lacking, resulting in delayed diagnosis and treatment of IFD and a poor prognosis of patients. Early detection of IFD and prompt initiation of appropriate treatment are critical factors in the survival of patients following HSCT (Puerta-Alcalde and Garcia-Vidal, 2021)
Standard diagnostic techniques are clinically challenging for IFD diagnosis because of their invasiveness, long detection period, and lack of sensitivity, specificity, and species identification (Ostrosky-Zeichner, 2012). The gold standard for diagnosing IFD is culture-based testing; unfortunately, sterile specimens frequently necessitate potentially invasive procedures (Donnelly et al., 2020). Moreover, serum biomarkers, including galactomannan (GM) and 1,3-β-D-glucan (BDG), are the adjunct to clinical diagnosis of IFD (Guo et al., 2010; Lu et al., 2011a; Li et al., 2015; Donnelly et al., 2020). However, GM and BDG cannot detect all fungal pathogens, and their diagnostic efficacy in HSCT varies (Ullmann et al., 2018; Warris et al., 2019). According to the European Conference on Infections in Leukemia guidelines, serum BDG can help diagnose Pneumocystis jirovecii pneumonia (Maertens et al., 2016). However, GM performance remains significantly lower in non-neutropenic patients and/or those receiving prophylactic therapy (Wu et al., 2021). Radiographic findings may aid in the diagnosis of fungal lung lesions in patients with fever and neutropenia (FN). Nonspecific observations, on the other hand, often lead to overdiagnosis and incorrect treatment. Although PCR-based technologies have been shown to be effective in the identification of some fungal pathogens, false-positive and false-negative results limit their widespread application (Lu et al., 2011b; Trubiano et al., 2016; Ala-Houhala et al., 2018).
Metagenomic next-generation sequencing (mNGS) is a promising culture-independent technique that has been extensively used to diagnose infections (Gabaldón, 2019; Irinyi et al., 2020). mNGS may detect many types of microorganisms in a microbial sample both rapidly and concurrently (Barreda-García et al., 2018) while also recognizing non-culturable microbes (Yang et al., 2022). The application of mNGS to identify fungal infections has recently increased (Alonso et al., 2018; Shivaji et al., 2019; Chien et al., 2022). However, there have been relatively few investigations on the use of mNGS for diagnosis in patients with IFD following HSCT, and its clinical application has not been standardized. Owing to the severity and specificity of IFD after HSCT, efforts to optimize pathogen detection technologies are important for a favorable prognosis. This study examined the efficacy of standard mNGS technology in detecting infections in patients with IFD after HSCT by comparing the diagnostic performance of mNGS and conventional microbiological testing (CMT). In addition, we describe IFD diagnoses, survival, and risk factors for IFD following HSCT.
2 Material and methods
2.1 Study design and patients
We retrospectively studied 189 patients who underwent HSCT and were considered at risk of IFD at the Hematopoietic Stem Cell Transplantation Center, Tongji Hospital, affiliated with Huazhong University of Science and Technology, between June 2020 and October 2022. At least one of the following enrollment criteria was present: (1) prolonged fever with neutropenia (FN) after broad-spectrum antibiotics (≥ 96 h), (2) FN recurrence, or (3) anomalous conditions to consider fungal infections. Ultimately, 46 patients with IFD were enrolled in this study. Figure 1 depicts a flow diagram of the study participants based on STARD 2015 (Cohen et al., 2016). All patients received antifungal prophylaxis, according to revised recommendations from a consensus process led by the Gruppo Italiano Trapianto Midollo Osseo (Girmenia et al., 2014). Treatment should be continued for at least 100 days following transplantation, or 180 days in higher-risk patients, or until immunosuppressive therapy is discontinued. Different types of specimens were collected depending on the type of suspected infection. CMT included microscopy, culture, serological tests, RT-PCR, and radiological examinations. NGS tests were performed at Huada Laboratories (Shenzhen, China) or Genskey Laboratories (Tianjin, China). Patients’ antifungal treatment as well as clinical and laboratory data were collected retrospectively at the onset of infection symptoms. The follow-up ended on 31 January 2023.
2.2 Diagnosis of IFD
According to the revised definitions of IFD from European Organization for Research on Treatment of Cancer (EORTC) and the Chinese guidelines for the diagnosis and treatment of IFD in patients with hematological disorders and cancers (Chinese Association, H and Chinese Invasive Fungal Infection Working, G, 2020; Donnelly et al., 2020; Alexander et al., 2021), the 46 patients were classified as having proven, probable, possible, or undefined IFD. Patients were defined as having a proven IFD if fungi were discovered in a sterile specimen using cytology, microscopy, or culture. Patients were classified as having probable IFD if a host factor met both clinical criteria (i.e., radiographic findings, bronchoscopy, or sinus analysis) and mycological criteria (i.e., direct detection of fungi in a sterile specimen or detection of specific fungal antigens and cell wall components). Patients were classified as having possible IFD if a host factor met clinical criteria but not mycologic criteria and as having undefined IFD if a host factor did not match both clinical and mycological criteria yet the diagnostic-driven therapy was effective. Positive results for multiple microorganisms in the same specimen were treated as separate events. Overall survival (OS) was measured from the start of HSCT until death or the last follow-up for any reason.
2.3 mNGS procedure
2.3.1 Plasma sample processing and DNA extraction
Within 8 h of collection, 3 mL of blood was collected from patients, deposited in a blood collection tube, and held at room temperature for 3 min to 5 min before plasma separation and centrifugation at 4,000 rpm for 10 min at 4°C. Plasma samples were transferred to new sterile tubes. TIANamp Micro DNA Kit (DP316, TIANGEN BIOTECH, Beijing, China) was used to extract DNA from 300 µL of plasma according to the manufacturer’s instructions. The extracted DNA specimens were then utilized to build DNA libraries (Long et al., 2016).
2.3.2 Other samples processing and DNA extraction
BALF/CSF/hydrothorax sample (1.5 mL to 3mL) from patient was collected according to standard procedures. A 1.5-mL microcentrifuge tube containing 0.6 mL of the sample and 250 μL of 0.5-mm enriching beads were attached to a horizontal platform on a vortex mixer and agitated vigorously at 2800–3200 rpm for 30 min. Then, 7.2 μL of lysozyme was added for wall-breaking reaction. The 0.3-mL sample was separated into a new 1.5-mL microcentrifuge tube, and DNA was extracted using the TIANamp Micro DNA Kit (DP316, TIANGEN BIOTECH) according to the manufacturer’s recommendation.
2.3.3 Construction of DNA libraries and sequencing
DNA libraries were constructed through DNA fragmentation, end-repair, adapter-ligation, and PCR amplification. Agilent 2100 (Agilent Technologies, Santa Clara, California) was used for quality control of the DNA libraries. Quality-qualified libraries were pooled, and DNA Nanoball (DNB) was made and sequenced by MGISEQ-200/MGISEQ-2000 platform (Jeon et al., 2014).
2.3.4 Bioinformatic analysis
High-quality sequencing data were generated by removing low-quality reads, followed by computational subtraction of human host sequences mapped to the human reference genome (hg19) using Burrows–Wheeler Alignment (Li and Durbin, 2009). The remaining data by removal of low-complexity reads were classified by simultaneously aligning to Pathogens metagenomics Database, consisting of bacteria, fungi, viruses, and parasites. The classification reference databases were downloaded from National Center for Biotechnology Information (NCBI) (ftp://ftp.ncbi.nlm.nih.gov/genomes/). RefSeq contains 4,945 whole-genome sequence of viral taxa, 6,350 bacterial genomes or scaffolds, 1,064 fungi related to human infection, and 234 parasites associated with human diseases.
2.3.5 Criteria for a positive mNGS result
1. The total number of sample sequences is higher than or equal to 20 million reads.
2. The ratio of the reads per million sample divided by the reads per million of the no-template control from any given taxon (species, genus, or family) ≥10 (Mongkolrattanothai et al., 2017; Simner et al., 2018).
3. Bacteria (mycobacteria excluded), virus, and parasites: mNGS identified a microbe (species level) whose coverage rate scored 10-fold greater than that of any other microbes (Langelier et al., 2018; Miao et al., 2018).
4. Fungi: mNGS identified a microbe (species level) whose coverage rate scored five-fold higher than that of any other fungus because of its low biomass in DNA extraction (Bittinger et al., 2014; Schlaberg et al., 2017; Miao et al., 2018)
2.4 Statistical analysis
We used the Kaplan–Meier method for survival analysis, and groups were compared using the log-rank test. The kappa (κ) statistic was used to assess the consistency of different assays. Independent risk factors for IFD were examined using univariate and multivariate logistic regression models. SPSS (version 26.0; SPSS Inc., Chicago, IL, USA), GraphPad Prism (version 8.0; GraphPad Software, La Jolla, CA, USA), and R (version 3.6.3; the R Foundation, Indianapolis, IN, USA) were used to analyze and generate the graphs. P-values < 0.05 (two-tailed) were considered statistically significant.
3 Results
3.1 Clinical characteristics
Table 1 displays the baseline patient information and baseline characteristics. Forty-six patients with a median age of 43 years were enrolled in this study. The majority of the patients (41.30%) had an underlying diagnosis of AML. Most (89.13%, n = 41) underwent allogeneic HSCT, whereas five (10.87%) underwent autologous HSCT. Eleven (23.91%) had a history of fungal infection. When the symptoms first appeared, 25 (32.2%) of the patients had agranulocytosis, and 36 (78.26%) were given immunosuppressive medication. GVHD occurred in 16 allogeneic HSCT patients, and 35/46 (76.09%) received prophylactic antifungal therapy during IFD. Central venous catheters were placed in 35 patients during the IFD. The median values of LDH, CRP, PCT, and IL-6 in all patients were 339.00 U/L [interquartile range (IQR), 252.50–552.50], 100.20 mg/L (IQR, 42.75–207.88), 0.63 ng/ml (IQR, 0.24–2.37) and 101.20 pg/ml (IQR, 36.53–415.60), respectively.
3.2 IFD diagnoses
In total, 46 patients were diagnosed with IFD, and the prevalence of proven, probable, possible, and undefined IFD was 2.18% (1), 23.91% (11), 58.69% (27), and 15.22% (7), respectively. Candida tropicalis was cultured from the peripheral blood of a patient with proven IFD. Figure 2A depicts the distribution of pathogens detected using mNGS and CMT. The most common IFD was Aspergillosis, which accounted for 30.43% of the episodes (14); Candidiasis and Pneumocystis each accounted for 15.22% of the episodes (7). One of whom had Candidiasis and Aspergillosis, and another had Candidiasis and Pneumocystis. Fifteen patients were diagnosed with mucormycosis, three of whom had Aspergillus infection and one had Candida infection. Trichosporon, Meyerozyma, and Stachybotrys were detected in one case each. The remaining six cases of IFD were diagnosed without formal identification of fungal species, for example, by BDG. mNGS outperformed other methods in detecting fungi and accurately detected specific fungal pathogens. The majority (56.52%) of patients with IFD were co-infected with bacteria and/or viruses (Figure 2B). Figure 2C displays the pathogen distribution in 46 patients with IFD at different times following transplantation. A total of 57.14% of the Aspergillus infections in our study occurred during the peri-planting period. All Pneumocystis infections occurred later than 100 days after transplantation.
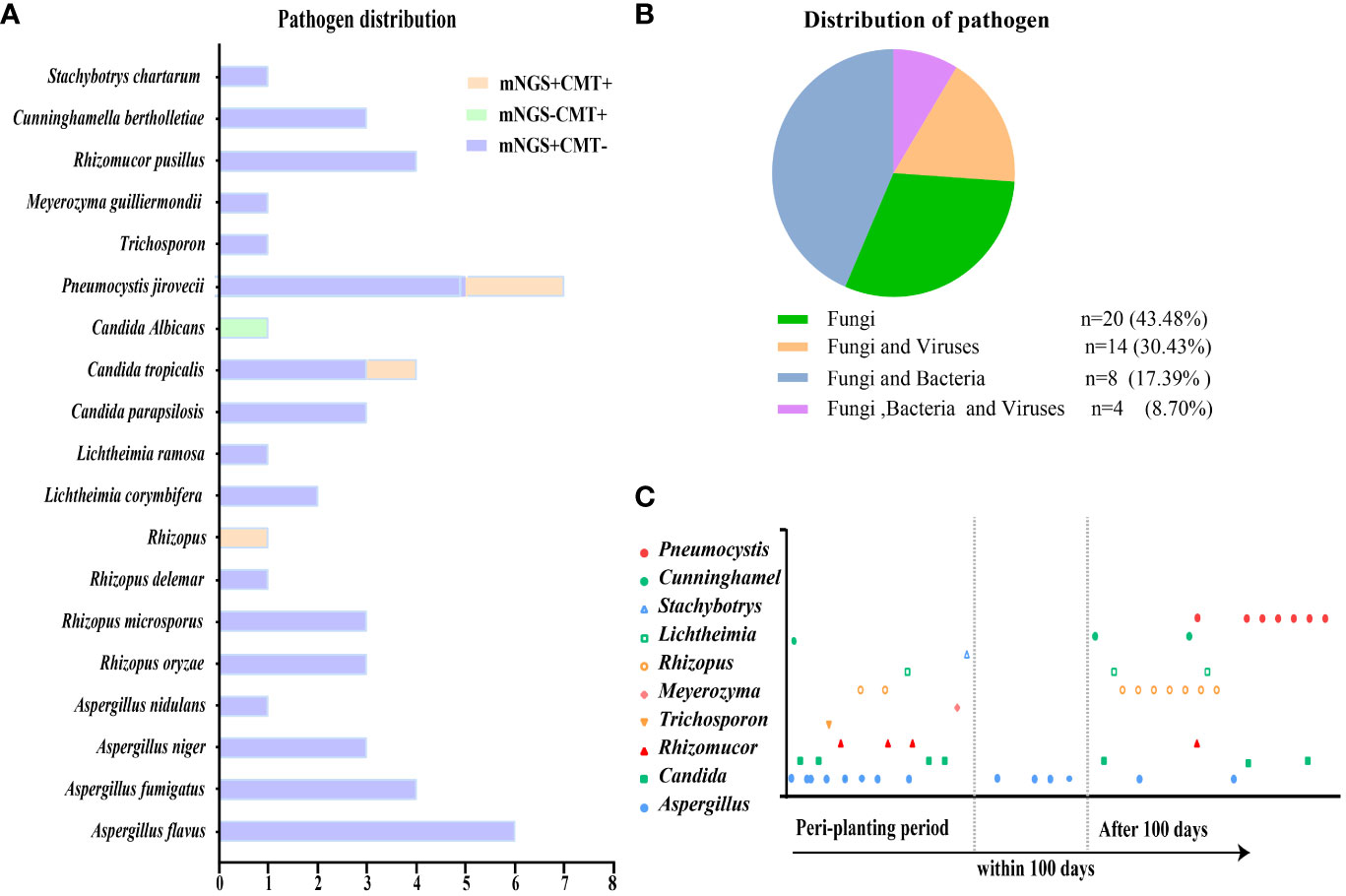
Figure 2 Distribution of pathogens identified in patients with IFD after HSCT. (A) The figure showed the number of subjects in whom each causative fungus was detected. Orange bars indicate fungi detected by both CMT and mNGS (mNGS+CMT+). Purple bars indicate fungi detected by mNGS only (mNGS+CMT−). Green bars indicate fungi detected only by CMT (mNGS−CMT+). (B) Distribution of pathogens was shown in patients. Fungal infections accounted for 43.48% among all the subjects. (C) Different fungal infections occurred at different periods after transplantation.
3.3 Diagnostic performance of mNGS compared to CMT
The sensitivity of both mNGS and CMT in all patients is shown in Table 2. Our results showed that mNGS and CMT had a sensitivity of 84.78% and 36.96%, respectively. mNGS significantly outperformed CMT (P < 0.0001). Fifteen (32.61%) of the 46 patients had congruent mNGS and CMT results. Additionally, sample selection is essential for testing performance. The sensitivity rates of mNGS and CMT for different specimens are shown in Figure 3A. The sensitivity of peripheral blood samples to mNGS was significantly higher (P < 0.0001), but, for specimens from potentially pathological tissues, such as sputum and bronchoalveolar lavage fluid (BALF) from patients with cough, the sensitivity rates of mNGS and CMT were comparable. When compared to CMT, mNGS significantly reduced the time needed to identify pathogens (P = 0.0016) (Figure 3B). mNGS had a higher positive testing % for the detection of various pathogenic fungi than CMT. The consistency for Pneumocystis was 85.72% (Figures 3C, D).
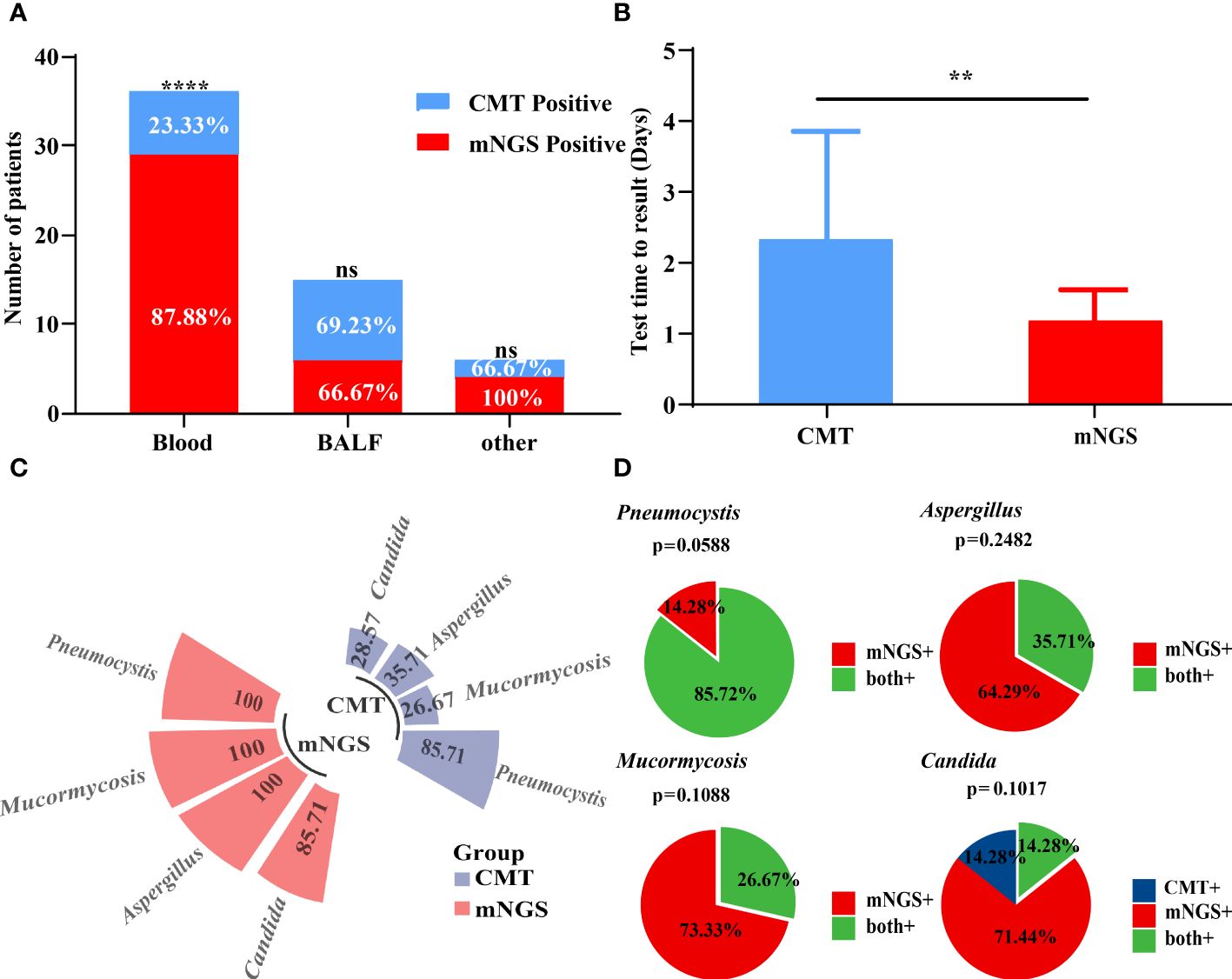
Figure 3 Performance of mNGS and CMT in pathogens identified. (A) The positive rates of mNGS and CMT in different samples were compared. The positive rate of mNGS in peripheral blood samples was significantly higher than that of CMT. (B) The diagnostic time required for mNGS and CMT were compared in patients with IFD. (C, D) The positive detection rate of mNGS and CMT and their consistency in different pathogens. **P < 0.01 and ****P < 0.0001 by Wilcoxon rank sum test.
3.4 Antifungal therapy
All patients received prophylactic antifungal therapy. When symptoms appeared, 35/46 (76.09%) patients received prophylactic antifungal therapy, including 29 patients who received voriconazole plus sulfamethoxazole and six patients who received posaconazole plus sulfamethoxazole. However, 71.43% of the patients with Aspergillus infection had breakthrough infection after starting voriconazole, and intravenous voriconazole was substituted in the context of routine drug monitoring. Of these, 78.75% received two or more antifungal drugs in subsequent treatment. In addition, 71.43% of pneumocystis infections occurred withrow the sulfamethoxazole, and all patients were still effectively treated in the subsequent therapy using sulfamethoxazole in combination with or without other antifungal drugs. At the time of infection, 12 of the 15 (80%) patients with mucormycosis received prophylactic antifungal therapy, with nine patients receiving voriconazole + sulfamethoxazole and the remaining three receiving posaconazole + sulfamethoxazole. Fourteen of the 15 patients (93.3%) with mucormycosis subsequently received posaconazole or amphotericin B in combination with other antifungal drugs. Two of them had undergone surgery, and one patient, according to mNGS results, was not treated with appropriate antifungal drugs in time, resulting in disease progression and death. All patients with Candida infection had the central venous line removed and received antifungal therapy. Posaconazole in combination with amphotericin B was administered to one patient with Trichosporon infection and another with Meyerozyma infection. The patient with Stachybotrys infection was treated with amphotericin B and pneumocystis. Based on the NGS results, dose adjustments or medication changes using empiric antifungal treatment occurred in 56.52% of patients with IFD. Details of the antifungal treatments are described in Table 3. Such high values may be attributed to the combination of antifungal therapies.
3.5 Outcomes and survival
The median follow-up time was 16.50 months (range, 0.57–59.79 months). The estimated 4-year OS of patients with IFD was 71.55% (95% CI, 55.18%–85.82%), and the median survival time (mOS) was 59.07 months (95% CI, 55.30–62.84 months). The median time from fungal infection to death or the end of follow-up was 11.79 months. The fungi-related mortality rate was 18.82% (95% CI, 9.08%–30.72%). The survival data of patients with IFD are presented in Figure 4. Multivariate logistic regression analyses were performed for all 189 patients to identify the independent risk factors for IFD following HSCT and to correct for potential confounding factors. Univariate analysis was used to select variables for the multivariate logistic regression studies. Independent risk factors for IFD (Table 4) included corticosteroid use (Hazard ratio (HR), 8.008; 95% CI, 3.550–18.063; P < 0.001) and hypoproteinemia (HR, 5.121; 95% CI, 2.245–11.681; P < 0.001).
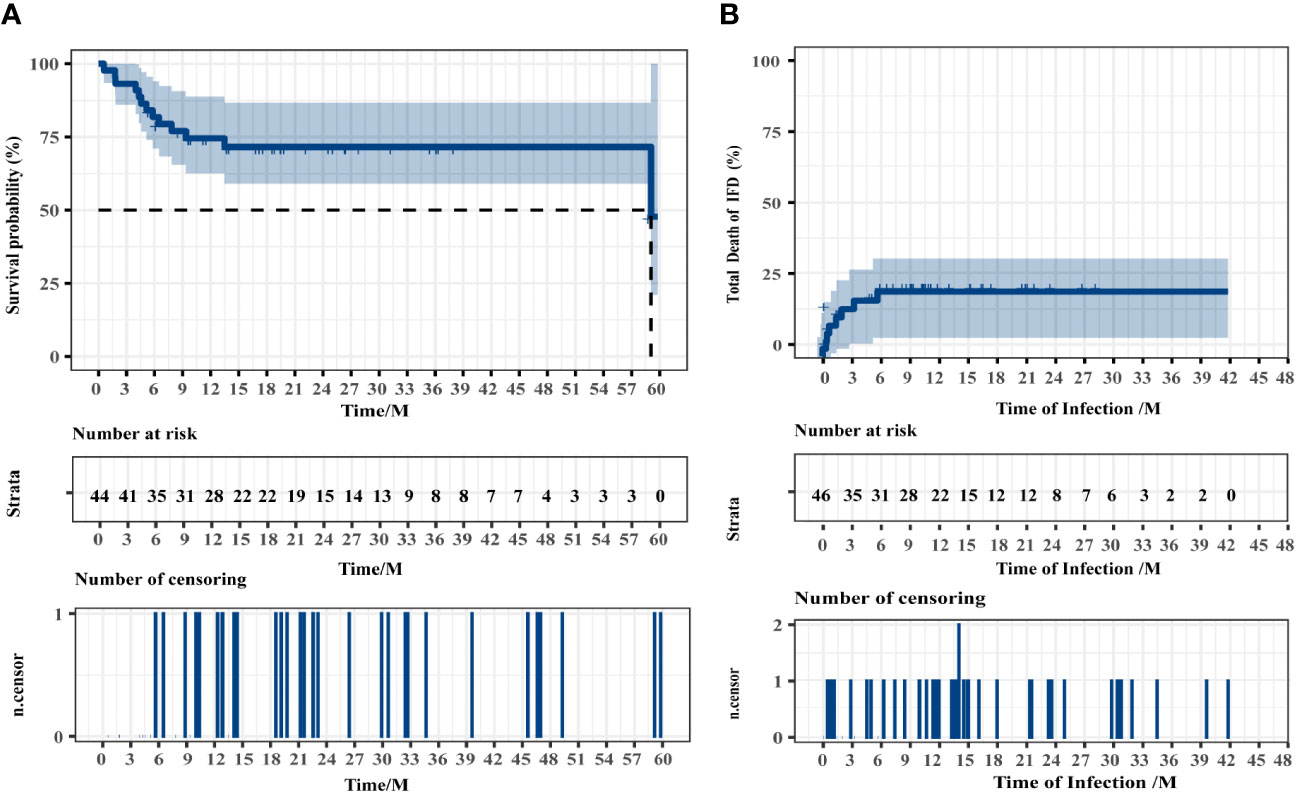
Figure 4 Overall survival of all patients (A) and the patients who died of IFD (B). Two patients were excluded from the overall survival analysis because of intracranial hemorrhage and disease recurrence during conditioning.
4 Discussion
IFD is a common complication of HSCT that is associated with high morbidity and mortality rate (Neofytos et al., 2009; Sun et al., 2015). Although IFD treatment has advanced significantly, delayed diagnosis causes significant morbidity and mortality (Ostrosky-Zeichner, 2012). Substantial advances in the diagnostic approaches for IFD include radiographic findings, biomarkers (e.g., GM and BDG), and PCR assays (Greene et al., 2007; Arvanitis et al., 2015). However, CMT may not be appropriate for rare fungi, particularly those that are difficult to culture, resulting in delayed detection, whereas mNGS can directly execute DNA or RNA sequencing (Tsang et al., 2021). Jiang et al. (2022) report that mNGS is increasingly being used to treat fungal infections.
The predominant fungal species in our study were Aspergillus (14 of 46, 30.43%) and Rhizopus (8 of 46, 17.39%). Candida and Pneumocystis accounted for 15.22% (7 of 46) of the infections. Owing to the different characteristics of immune function damage, the pathogens of IFD also differ at different stages after transplantation. Twenty (43.48%) of the 46 patients developed IFD during the conditioning period to the engraftment period, five (10.87%) within 100 days after transplantation, and 21 (45.65%) cases occurred more than 100 days later.
In our study, mNGS technology had distinct advantages over CMT in diagnosing different types of fungal species. The detectability increased significantly from 36.96% to 84.78%. The detection time was significantly reduced using mNGS. mNGS also provides accurate identification of specific fungal pathogens and is more specific than CMT. The mNGS results for 15 patients were consistent with those of CMT. The quick and accurate diagnosis of fungal species is critical for selecting appropriate antifungal agents, avoiding resistance, and managing patients (Omrani and Almaghrabi, 2017; Wang et al., 2020; Stemler et al., 2022). Pathogens are distributed in a variety of tissues and organs and have the ability to detect variations in different types of samples. The sensitivity of peripheral blood samples to CMT, particularly blood cultures, was significantly lower than that of mNGS; however, for specimens from possible pathological tissues, the sensitivity of mNGS and CMT was comparable.
In two incidents the CMT results in this study were positive, but the NGS produced a false negative in two patients. The specific analysis was as follows: mNGS detected human beta-herpesvirus 5 and torque teno virus in P1, whereas blood culture detected Candida albicans. Further analysis of the original mNGS data revealed that mNGS detected Candida albicans using an updated database. The second incident involved P6, where a positive GM test in the BALF was accompanied by an abnormal chest CT scan, which improved after receiving antifungal therapy.
Sometimes, the CMT results can indicate that the patient experienced suspected IFD following HSCT; however, the identification of fungal species is nonspecific. mNGS has low sensitivity when: 1) the pathogen load is below the detection limit or is filtered owing to low-ranking readings; 2) the pathogen’s nucleic acid is easily degraded; 3) the pathogen is suspected to be a background microorganism; and 4) the dataset or database has limitations. Further reasonable interpretations of the results are then required. The mcfDNA-Seq assay and serum GM can generally provide complementary results for the diagnosis of invasive pulmonary mold infections following HSCT (Hill et al., 2021). As proposed for P. jirovecii infection following HSCT (Liu et al., 2021), mNGS and BDG may aid in distinguishing colonization from infection. By using CMT in conjunction with mNGS technologies, the vision of a “one-stop” for IFD diagnosis seems promising in the foreseeable future.
Despite a decline in IFD-related deaths over the past decade, IFD remains a significant limiting factor for effective HSCT (Neofytos et al., 2009; Kontoyiannis et al., 2010). As a result, patients with suspected IFD after HSCT are frequently given prophylactic or empirical antifungal treatments. Most of the patients (76.09%) in our study received prophylactic antifungal therapy, with voriconazole being the most widely used drug. Although most patients received antifungal prophylaxis, many patients developed GVHD or required corticosteroids and immunosuppressants following HSCT. Among the patients with Pneumocystis infection, 71.43% occurred withrow the sulfamethoxazole, and all patients were still successfully treated in the subsequent therapy with sulfamethoxazole in combination with or without other antifungal drugs. This suggests that prophylactic antifungal therapy lowers the risk of IFD. According to the NGS results, dose adjustments or medication changes on the basis on empiric antifungal treatment, occurred in 56.52% of patients with IFD. The estimated 4-year OS was 71.55%, whereas the mOS of patients was 59.07 months. Eight patients died because of IFD. In this investigation, corticosteroid use (HR, 8.008; 95% CI, 3.550–18.063) and hypoproteinemia (HR, 5.121; 95% CI, 2.245–11.681) were identified as independent risk factors for IFD using multivariate logistic regression.
This study has several limitations. First, being a retrospective study, there were some inherent limitations, such as information bias and selection bias. The sample size of our study was relatively small. In addition, mNGS was usually performed only once owing to its high cost. Finally, there was no information on antifungal susceptibility or resistance. Further prospective randomized controlled studies with larger sample sizes are needed to be designed to elucidate the diagnostic value of mNGS for IFD following HSCT according to STARD 2015.
In conclusion, our study demonstrated the feasibility of mNGS in patients with IFD following HSCT. We recommend that clinicians use mNGS technology more actively when there is a clinical suspicion of IFD after HSCT. For clinical infection related samples, the traditional microbiological detection should be improved first. Pathology and sterile specimen culture are still the gold standard for infection diagnosis. Pathogenic mNGS is a powerful supplement and extension, which may be a helpful adjunct to the clinical diagnosis of IFD, allowing for more rapid and focused antifungal treatment.
Data availability statement
The datasets presented in this study can be found in online repositories. The name of the repository and accession number can be found below: NCBI: PRJNA1085906.
Author contributions
YZ and NW designed, supervised the clinical study, and revised the manuscript, who contributed equally to this work and should be considered corresponding authorship. XZ analyzed data and drafted the manuscript. LZ collected clinical data. YL was responsible for patient management. All authors contributed to the article and approved the submitted version.
Funding
The author(s) declare financial support was received for the research, authorship, and/or publication of this article. This study was supported by the National High Technology Research and Development Program of China (2021YFA1101500), the Key Research and Development Program of Hubei Province (No. 2022BCA017), and the National Natural Science Foundation of China (Grant No. 81873446 and 81600120).
Acknowledgments
The authors acknowledge the patients in the study, as well as the support from the Clinical and Laboratory Unit of the Department of Hematology, Tongji Hospital, Tongji Medical College, Huazhong University of Science and Technology staff and faculty.
Conflict of interest
The authors declare that the research was conducted in the absence of any commercial or financial relationships that could be construed as a potential conflict of interest.
Publisher’s note
All claims expressed in this article are solely those of the authors and do not necessarily represent those of their affiliated organizations, or those of the publisher, the editors and the reviewers. Any product that may be evaluated in this article, or claim that may be made by its manufacturer, is not guaranteed or endorsed by the publisher.
Supplementary material
The Supplementary Material for this article can be found online at: https://www.frontiersin.org/articles/10.3389/fcimb.2024.1210857/full#supplementary-material
References
Akan, H., Antia, V. P., Kouba, M., Sinko, J., Tanase, A. D., Vrhovac, R., et al. (2013). Preventing invasive fungal disease in patients with haematological Malignancies and the recipients of haematopoietic stem cell transplantation: Practical aspects. J. Antimicrob. Chemother. 68 Suppl 3, Iii5–Ii16. doi: 10.1093/jac/dkt389
Ala-Houhala, M., Koukila-Kähkölä, P., Antikainen, J., Valve, J., Kirveskari, J., Anttila, V. J. (2018). Clinical use of fungal pcr from deep tissue samples in the diagnosis of invasive fungal diseases: A retrospective observational study. Clin. Microbiol. Infect. 24, 301–305. doi: 10.1016/j.cmi.2017.08.017
Alexander, B. D., Lamoth, F., Heussel, C. P., Prokop, C. S., Desai, S. R., Morrissey, C. O., et al. (2021). Guidance on imaging for invasive pulmonary aspergillosis and mucormycosis: From the imaging working group for the revision and update of the consensus definitions of fungal disease from the eortc/msgerc. Clin. Infect. Dis. 72, S79–S88. doi: 10.1093/cid/ciaa1855
Alonso, R., Pisa, D., Fernández-Fernández, A. M., Carrasco, L. (2018). Infection of fungi and bacteria in brain tissue from elderly persons and patients with alzheimer's disease. Front. Aging Neurosci. 10. doi: 10.3389/fnagi.2018.00159
Arvanitis, M., Anagnostou, T., Mylonakis, E. (2015). Galactomannan and polymerase chain reaction-based screening for invasive aspergillosis among high-risk hematology patients: A diagnostic meta-analysis. Clin. Infect. Dis. 61, 1263–1272. doi: 10.1093/cid/civ555
Barreda-García, S., Miranda-Castro, R., De-Los-Santos-Álvarez, N., Miranda-Ordieres, A. J., Lobo-Castañón, M. J. (2018). Helicase-dependent isothermal amplification: A novel tool in the development of molecular-based analytical systems for rapid pathogen detection. Anal. Bioanal Chem. 410, 679–693. doi: 10.1007/s00216-017-0620-3
Bittinger, K., Charlson, E. S., Loy, E., Shirley, D. J., Haas, A. R., Laughlin, A., et al. (2014). Improved characterization of medically relevant fungi in the human respiratory tract using next-generation sequencing. Genome Biol. 15, 487. doi: 10.1186/s13059-014-0487-y
Chien, J. Y., Yu, C. J., Hsueh, P. R. (2022). Utility of metagenomic next-generation sequencing for etiological diagnosis of patients with sepsis in intensive care units. Microbiol. Spectr. 10, E0074622. doi: 10.1128/spectrum.00746-22
Chinese Association, H, Chinese Invasive Fungal Infection Working, G (2020). [The chinese guidelines for the diagnosis and treatment of invasive fungal disease in patients with hematological disorders and cancers (The 6th revision)]. Zhonghua Nei Ke Za Zhi 59, 754–763. doi: 10.3760/Cma.J.Cn112138-20200627-00624
Chinese Society Of Hematology, Chinese Medical Association, Antimicrobial Infection Branch (2023). [Chinese expert consensus for invasive fungal disease in patients after hematopoietic stem cell transplantation(2023)]. Zhonghua Xue Ye Xue Za Zhi 44, 92–97. doi: 10.3760/Cma.J.Issn.0253-2727.2023.02.002
Cohen, J. F., Korevaar, D. A., Altman, D. G., Bruns, D. E., Gatsonis, C. A., Hooft, L., et al. (2016). Stard 2015 guidelines for reporting diagnostic accuracy studies: Explanation and elaboration. BMJ Open 6, E012799. doi: 10.1136/bmjopen-2016-012799
Donnelly, J. P., Chen, S. C., Kauffman, C. A., Steinbach, W. J., Baddley, J. W., Verweij, P. E., et al. (2020). Revision and update of the consensus definitions of invasive fungal disease from the European organization for research and treatment of cancer and the mycoses study group education and research consortium. Clin. Infect. Dis. 71, 1367–1376. doi: 10.1093/cid/ciz1008
Gabaldón, T. (2019). Recent trends in molecular diagnostics of yeast infections: From pcr to ngs. FEMS Microbiol. Rev. 43, 517–547. doi: 10.1093/femsre/fuz015
Girmenia, C., Barosi, G., Piciocchi, A., Arcese, W., Aversa, F., Bacigalupo, A., et al. (2014). Primary prophylaxis of invasive fungal diseases in allogeneic stem cell transplantation: Revised recommendations from A consensus process by gruppo italiano trapianto midollo osseo (Gitmo). Biol. Blood Marrow Transplant. 20, 1080–1088. doi: 10.1016/j.bbmt.2014.02.018
Greene, R. E., Schlamm, H. T., Oestmann, J. W., Stark, P., Durand, C., Lortholary, O., et al. (2007). Imaging findings in acute invasive pulmonary aspergillosis: Clinical significance of the halo sign. Clin. Infect. Dis. 44, 373–379. doi: 10.1086/509917
Guo, Y. L., Chen, Y. Q., Wang, K., Qin, S. M., Wu, C., Kong, J. L. (2010). Accuracy of bal galactomannan in diagnosing invasive aspergillosis: A bivariate metaanalysis and systematic review. Chest 138, 817–824. doi: 10.1378/chest.10-0488
Hill, J. A., Dalai, S. C., Hong, D. K., Ahmed, A. A., Ho, C., Hollemon, D., et al. (2021). Liquid biopsy for invasive mold infections in hematopoietic cell transplant recipients with pneumonia through next-generation sequencing of microbial cell-free dna in plasma. Clin. Infect. Dis. 73, E3876–E3883. doi: 10.1093/cid/ciaa1639
Irinyi, L., Hu, Y., Hoang, M. T. V., Pasic, L., Halliday, C., Jayawardena, M., et al. (2020). Long-read sequencing based clinical metagenomics for the detection and confirmation of pneumocystis jirovecii directly from clinical specimens: A paradigm shift in mycological diagnostics. Med. Mycol 58, 650–660. doi: 10.1093/mmy/myz109
Jeon, Y. J., Zhou, Y., Li, Y., Guo, Q., Chen, J., Quan, S., et al. (2014). The feasibility study of non-invasive fetal trisomy 18 and 21 detection with semiconductor sequencing platform. PLoS One 9, E110240. doi: 10.1371/journal.pone.0110240
Jiang, S., Chen, Y., Han, S., Lv, L., Li, L. (2022). Next-generation sequencing applications for the study of fungal pathogens. Microorganisms 10(10), 1882. doi: 10.3390/microorganisms10101882
Kontoyiannis, D. P., Marr, K. A., Park, B. J., Alexander, B. D., Anaissie, E. J., Walsh, T. J., et al. (2010). Prospective surveillance for invasive fungal infections in hematopoietic stem cell transplant recipients 2001-2006: Overview of the transplant-associated infection surveillance network (Transnet) database. Clin. Infect. Dis. 50, 1091–1100. doi: 10.1086/651263
Kuster, S., Stampf, S., Gerber, B., Baettig, V., Weisser, M., Gerull, S., et al. (2018). Incidence and outcome of invasive fungal diseases after allogeneic hematopoietic stem cell transplantation: A swiss transplant cohort study. Transpl Infect. Dis. 20, E12981. doi: 10.1111/tid.12981
Langelier, C., Zinter, M. S., Kalantar, K., Yanik, G. A., Christenson, S., O'donovan, B., et al. (2018). Metagenomic sequencing detects respiratory pathogens in hematopoietic cellular transplant patients. Am. J. Respir. Crit. Care Med. 197, 524–528. doi: 10.1164/rccm.201706-1097LE
Li, H., Durbin, R. (2009). Fast and accurate short read alignment with burrows-wheeler transform. Bioinformatics 25, 1754–1760. doi: 10.1093/bioinformatics/btp324
Li, W. J., Guo, Y. L., Liu, T. J., Wang, K., Kong, J. L. (2015). Diagnosis of pneumocystis pneumonia using serum (1-3)-Β-D-glucan: A bivariate meta-analysis and systematic review. J. Thorac. Dis. 7, 2214–2225. doi: 10.3978/J.Issn.2072-1439.2015.12.27
Liu, L., Yuan, M., Shi, Y., Su, X. (2021). Clinical performance of bal metagenomic next-generation sequence and serum (1,3)-Β-D-glucan for differential diagnosis of pneumocystis jirovecii pneumonia and pneumocystis jirovecii colonisation. Front. Cell Infect. Microbiol. 11. doi: 10.3389/fcimb.2021.784236
Long, Y., Zhang, Y., Gong, Y., Sun, R., Su, L., Lin, X., et al. (2016). Diagnosis of sepsis with cell-free dna by next-generation sequencing technology in icu patients. Arch. Med. Res. 47, 365–371. doi: 10.1016/j.arcmed.2016.08.004
Lu, Y., Chen, Y. Q., Guo, Y. L., Qin, S. M., Wu, C., Wang, K. (2011a). Diagnosis of invasive fungal disease using serum (1→3)-Β-D-glucan: A bivariate meta-analysis. Intern. Med. 50, 2783–2791. doi: 10.2169/internalmedicine.50.6175
Lu, Y., Ling, G., Qiang, C., Ming, Q., Wu, C., Wang, K., et al. (2011b). Pcr diagnosis of pneumocystis pneumonia: A bivariate meta-analysis. J. Clin. Microbiol. 49, 4361–4363. doi: 10.1128/JCM.06066-11
Maertens, J., Cesaro, S., Maschmeyer, G., Einsele, H., Donnelly, J. P., Alanio, A., et al. (2016). Ecil guidelines for preventing pneumocystis jirovecii pneumonia in patients with haematological Malignancies and stem cell transplant recipients. J. Antimicrob. Chemother. 71, 2397–2404. doi: 10.1093/jac/dkw157
Miao, Q., Ma, Y., Wang, Q., Pan, J., Zhang, Y., Jin, W., et al. (2018). Microbiological diagnostic performance of metagenomic next-generation sequencing when applied to clinical practice. Clin. Infect. Dis. 67, S231–S240. doi: 10.1093/cid/ciy693
Mongkolrattanothai, K., Naccache, S. N., Bender, J. M., Samayoa, E., Pham, E., Yu, G., et al. (2017). Neurobrucellosis: unexpected answer from metagenomic next-generation sequencing. J. Pediatr. Infect. Dis. Soc. 6, 393–398. doi: 10.1093/jpids/piw066
Neofytos, D., Horn, D., Anaissie, E., Steinbach, W., Olyaei, A., Fishman, J., et al. (2009). Epidemiology and outcome of invasive fungal infection in adult hematopoietic stem cell transplant recipients: Analysis of multicenter prospective antifungal therapy (Path) alliance registry. Clin. Infect. Dis. 48, 265–273. doi: 10.1086/595846
Omrani, A. S., Almaghrabi, R. S. (2017). Complications of hematopoietic stem transplantation: fungal infections. Hematol. Oncol. Stem Cell Ther. 10, 239–244. doi: 10.1016/j.hemonc.2017.05.013
Ostrosky-Zeichner, L. (2012). Invasive mycoses: diagnostic challenges. Am. J. Med. 125, S14–S24. doi: 10.1016/j.amjmed.2011.10.008
Pagano, L., Caira, M., Nosari, A., Van Lint, M. T., Candoni, A., Offidani, M., et al. (2007). Fungal infections in recipients of hematopoietic stem cell transplants: Results of the seifem B-2004 study–sorveglianza epidemiologica infezioni fungine nelle emopatie Maligne. Clin. Infect. Dis. 45, 1161–1170. doi: 10.1086/522189
Puerta-Alcalde, P., Garcia-Vidal, C. (2021). Changing epidemiology of invasive fungal disease in allogeneic hematopoietic stem cell transplantation. J. Fungi (Basel) 7(10), 848. doi: 10.3390/jof7100848
Ruhnke, M., Cornely, O. A., Schmidt-Hieber, M., Alakel, N., Boell, B., Buchheidt, D., et al. (2020). Treatment of invasive fungal diseases in cancer patients-revised 2019 recommendations of the infectious diseases working party (Agiho) of the German society of hematology and oncology (Dgho). Mycoses 63, 653–682. doi: 10.1111/myc.13082
Schlaberg, R., Chiu, C. Y., Miller, S., Procop, G. W., Weinstock, G. (2017). Validation of metagenomic next-generation sequencing tests for universal pathogen detection. Arch. Pathol. Lab. Med. 141, 776–786. doi: 10.5858/arpa.2016-0539-RA
Shivaji, S., Jayasudha, R., Sai Prashanthi, G., Kalyana Chakravarthy, S., Sharma, S. (2019). The human ocular surface fungal microbiome. Invest. Ophthalmol. Vis. Sci. 60, 451–459. doi: 10.1167/iovs.18-26076
Simner, P. J., Miller, S., Carroll, K. C. (2018). Understanding the promises and hurdles of metagenomic next-generation sequencing as A diagnostic tool for infectious diseases. Clin. Infect. Dis. 66, 778–788. doi: 10.1093/cid/cix881
Stemler, J., De Jonge, N., Skoetz, N., Sinkó, J., Brüggemann, R. J., Busca, A., et al. (2022). Antifungal prophylaxis in adult patients with acute myeloid leukaemia treated with novel targeted therapies: A systematic review and expert consensus recommendation from the European hematology association. Lancet Haematol 9, E361–E373. doi: 10.1016/S2352-3026(22)00073-4
Sun, Y., Hu, J., Huang, H., Chen, J., Li, J., Ma, J., et al. (2021). Clinical risk score for predicting invasive fungal disease after allogeneic hematopoietic stem cell transplantation: Analysis of the China assessment of antifungal therapy in hematological diseases (Caesar) study. Transpl Infect. Dis. 23, E13611. doi: 10.1111/tid.13611
Sun, Y., Meng, F., Han, M., Zhang, X., Yu, L., Huang, H., et al. (2015). Epidemiology, management, and outcome of invasive fungal disease in patients undergoing hematopoietic stem cell transplantation in China: A multicenter prospective observational study. Biol. Blood Marrow Transplant. 21, 1117–1126. doi: 10.1016/j.bbmt.2015.03.018
Tissot, F., Agrawal, S., Pagano, L., Petrikkos, G., Groll, A. H., Skiada, A., et al. (2017). Ecil-6 guidelines for the treatment of invasive candidiasis, aspergillosis and mucormycosis in leukemia and hematopoietic stem cell transplant patients. Haematologica 102, 433–444. doi: 10.3324/haematol.2016.152900
Trubiano, J. A., Dennison, A. M., Morrissey, C. O., Chua, K. Y., Halliday, C. L., Chen, S. C., et al. (2016). Clinical utility of panfungal polymerase chain reaction for the diagnosis of invasive fungal disease: A single center experience. Med. Mycol 54, 138–146. doi: 10.1093/mmy/myv092
Tsang, C. C., Teng, J. L. L., Lau, S. K. P., Woo, P. C. Y. (2021). Rapid genomic diagnosis of fungal infections in the age of next-generation sequencing. J. Fungi (Basel) 7(8), 636. doi: 10.3390/jof7080636
Ullmann, A. J., Aguado, J. M., Arikan-Akdagli, S., Denning, D. W., Groll, A. H., Lagrou, K., et al. (2018). Diagnosis and management of aspergillus diseases: Executive summary of the 2017 escmid-ecmm-ers guideline. Clin. Microbiol. Infect. 24 Suppl 1, E1–E38. doi: 10.1016/j.cmi.2018.01.002
Wang, J., Zhou, M., Xu, J. Y., Zhou, R. F., Chen, B., Wan, Y. (2020). Comparison of antifungal prophylaxis drugs in patients with hematological disease or undergoing hematopoietic stem cell transplantation: A systematic review and network meta-analysis. JAMA Netw. Open 3, E2017652. doi: 10.1001/jamanetworkopen.2020.17652
Warris, A., Lehrnbecher, T., Roilides, E., Castagnola, E., Brüggemann, R. J. M., Groll, A. H. (2019). Escmid-ecmm guideline: Diagnosis and management of invasive aspergillosis in neonates and children. Clin. Microbiol. Infect. 25, 1096–1113. doi: 10.1016/j.cmi.2019.05.019
Wu, Z., Wang, L., Tan, L., Wu, J., Chen, Z., Hu, M. (2021). Diagnostic value of galactomannan in serum and bronchoalveolar lavage fluid for invasive pulmonary aspergillosis in non-neutropenic patients. Diagn. Microbiol. Infect. Dis. 99, 115274. doi: 10.1016/j.diagmicrobio.2020.115274
Yang, H., Smith, R. D., Sumner, K. P., Goodlett, D. R., Johnson, J. K., Ernst, R. K. (2022). A matrix-assisted laser desorption ionization-time of flight mass spectrometry direct-from-urine-specimen diagnostic for gram-negative pathogens. Microbiol. Spectr. 10, E0373022. doi: 10.1128/spectrum.03730-22
Keywords: metagenomic next-generation sequencing, invasive fungal disease, hematopoietic stem cell transplantation, immunosuppression, diagnosis
Citation: Zhang X, Zhang L, Li Y, Wang N and Zhang Y (2024) Clinical performance of metagenomic next-generation sequencing for diagnosis of invasive fungal disease after hematopoietic cell transplant. Front. Cell. Infect. Microbiol. 14:1210857. doi: 10.3389/fcimb.2024.1210857
Received: 23 April 2023; Accepted: 04 March 2024;
Published: 25 March 2024.
Edited by:
Ruijin Guo, Beijing Genomics Institute (BGI), ChinaReviewed by:
Wang Ke, Guangxi Medical University, ChinaAlfred Otoe Ankrah, Korle Bu Teaching Hospital, Ghana
Copyright © 2024 Zhang, Zhang, Li, Wang and Zhang. This is an open-access article distributed under the terms of the Creative Commons Attribution License (CC BY). The use, distribution or reproduction in other forums is permitted, provided the original author(s) and the copyright owner(s) are credited and that the original publication in this journal is cited, in accordance with accepted academic practice. No use, distribution or reproduction is permitted which does not comply with these terms.
*Correspondence: Yicheng Zhang, eWN6aGFuZ0B0amgudGptdS5lZHUuY24=; Na Wang, d2FuZ25hLjIwMDFAMTYzLmNvbQ==