- 1College of Life Sciences, Southwest Forestry University, Kunming, China
- 2State Key Laboratory of Pathogen and Biosecurity, Beijing Institute of Microbiology and Epidemiology, Beijing, China
Coxiella burnetii is an obligate intracellular bacterium that causes Q fever, a zoonotic disease typically manifests as a severe flu-illness. After invading into the host cells, C. burnetii delivers effectors to regulate the vesicle trafficking and fusion events to form a large and mature Coxiella-containing vacuole (CCV), providing sufficient space and nutrition for its intracellular growth and proliferation. Lysosomal trafficking regulator (LYST) is a member of the Beige and Chediak-Higashi syndrome (BEACH) family, which regulates the transport of vesicles to lysosomes and regulates TLR signaling pathway, but the effect of LYST on C. burnetii infection is unclear. In this study, a series of experiments has been conducted to investigate the influence of LYST on intracellular growth of C. burnetii. Our results showed that lyst transcription was up-regulated in the host cells after C. burnetii infection, but there is no significant change in lyst expression level after infection with the Dot/Icm type IV secretion system (T4SS) mutant strain, while CCVs expansion and significantly increasing load of C. burnetii appeared in the host cells with a silenced lyst gene, suggesting LYST inhibits the intracellular proliferation of C. burnetii by reducing CCVs size. Then, the size of CCVs and the load of C. burnetii in the HeLa cells pretreated with E-64d were significantly decreased. In addition, the level of iNOS was decreased significantly in LYST knockout THP-1 cells, which was conducive to the intracellular replication of C. burnetii. This data is consistent with the phenotype of L-NMMA-treated THP-1 cells infected with C. burnetii. Our results revealed that the upregulation of lyst transcription after infection is due to effector secretion of C. burnetii and LYST inhibit the intracellular replication of C. burnetii by reducing the size of CCVs and inducing nos2 expression.
Introduction
Coxiella burnetii, an obligate intracellular Gram-negative bacterium, is the etiological agent of Q fever (Reimer, 1993; Qin et al., 2022). In nature, arthropods (including ticks and mites) and domestic ruminants (including cattle, sheep and goats) are the main hosts of C. burnetii. The main routes of C. burnetii infection are tick bites or inhalation of contaminated aerosols (Maurin and Raoult, 1999; Raoult et al., 2005). People infected by C. burnetii cause acute Q fever clinically characterized by high fever, headache, fatigue even acute pneumonia (Vinetz et al., 2017). In rare cases, persistent infection of C. burnetii in local tissues or organs causes chronic Q fever, leading to serious complications such as pneumonia, endocarditis, myocarditis, and osteomyelitis, and will be accompanied by a higher mortality rate (Stein and Raoult, 1995; Kazar, 2005; Eldin et al., 2017; Straily et al., 2017; Ghanem-Zoubi et al., 2021). Chronic Q fever is more difficult to treat than acute Q fever, and patients often require prolonged antibiotic treatment (van Roeden et al., 2018). Serological and molecular biological studies of C. burnetii have demonstrated that it is almost distributed around the world (Georgiev et al., 2013), and many studies have reported that Q fever outbreaks and epidemics have occurred in dozens of provinces, municipalities, and autonomous regions in China, including Chongqing, Sichuan, Yunnan, Tibet, Gansu, and Guangzhou (El-Mahallawy et al., 2015a; El-Mahallawy et al., 2015b; Huang et al., 2021).
C. burnetii invades host cells through the endocytosis pathway and forms phagosomes in the cells, and C. burnetii deliver effectors into the cytosol of host cells through the Dot/Icm type IV secretion system (T4SS) to regulate various host cell functions, such as apoptosis (Lührmann et al., 2010; Klingenbeck et al., 2013; Schäfer et al., 2017), autophagy (Valdivia et al., 2014; Mansilla Pareja et al., 2017), ubiquitination (Voth et al., 2011), metabolism and vesicle transport system (Kohler and Roy, 2015), which delivers C. burnetii to lysosome, ultimately forming a specialized membrane-bound compartment called Coxiella-containing vacuole (CCV) (Beare et al., 2011; Valdivia et al., 2014; Roy et al., 2019). With the homologous fusion of CCVs and the heterologous fusion between CCVs and vesicles such as autophagosomes, lysosomes and late endocytic vesicles, a mature and single huge CCV will gradually form to provide sufficient space and nutrition for the intracellular growth of C. burnetii (Kohler and Roy, 2015).
Lysosomal transport regulatory factor (LYST) is a highly conserved homologous protein in mammals, which is classified as a member of the Beige and Chediak-Higashi syndrome (BEACH) family due to a special BEACH region (Burgess et al., 2009; Cullinane et al., 2013). LYST regulates the transport of vesicles to lysosomes in host cells (Holland et al., 2014) and specifically controls interferon regulatory factors 3 (IRF3)/TIR domain-containing adapter-inducing interferon β (TRIF-β), inducing endosomal TLR signaling pathways (Westphal et al., 2017). Inhibition of LYST function results in abnormal phagosome maturation, leading to impaired formation of activation-induced rab7+ endosomal/phagosomal compartment, which is the major milestones in the TRIF signaling pathway (Westphal et al., 2017). LYST single gene mutation will lead to abnormal autophagy and defective vesicles transport, thus giving rise to phagosome enlargement (Cullinane et al., 2013; Sepulveda et al., 2015; Lattao et al., 2021).
In this study, we found that the expression of lyst was up-regulated in host cells after infection with C. burnetii, and the up-regulation of lyst expression was closely related to the secretion of C. burnetii effector. Further investigation showed that LYST inhibited the intracellular replication of C. burnetii by restricting the enlargement of CCVs and up-regulating the expression of nos2. The findings provide a novel clue for elucidating the pathogenic mechanism of C. burnetii and identify a novel potential therapeutic target for Q fever.
Results
Promotion of C. burnetii growth in host cells with knockdown of lyst
The transcription level of lyst mRNA in C. burnetii infected Hela cells was detected at different time points. As a result, it showed that the mRNA transcription levels of lyst were approximately 6-fold increased at 24 hours after infection, compared with uninfected controls (Figure 1A), indicating that C. burnetii infection caused up-regulation of mRNA expression of lyst in HeLa cells, and this result was also obtained in THP-1 cells (Figure 2A). At 72 hours after infection with C. burnetii, a large CCV that contained the lysosomal marker Lamp1 was observed in the host cells in fluorescence confocal analysis (Figures 1B, 2B) (Roy et al., 2019).
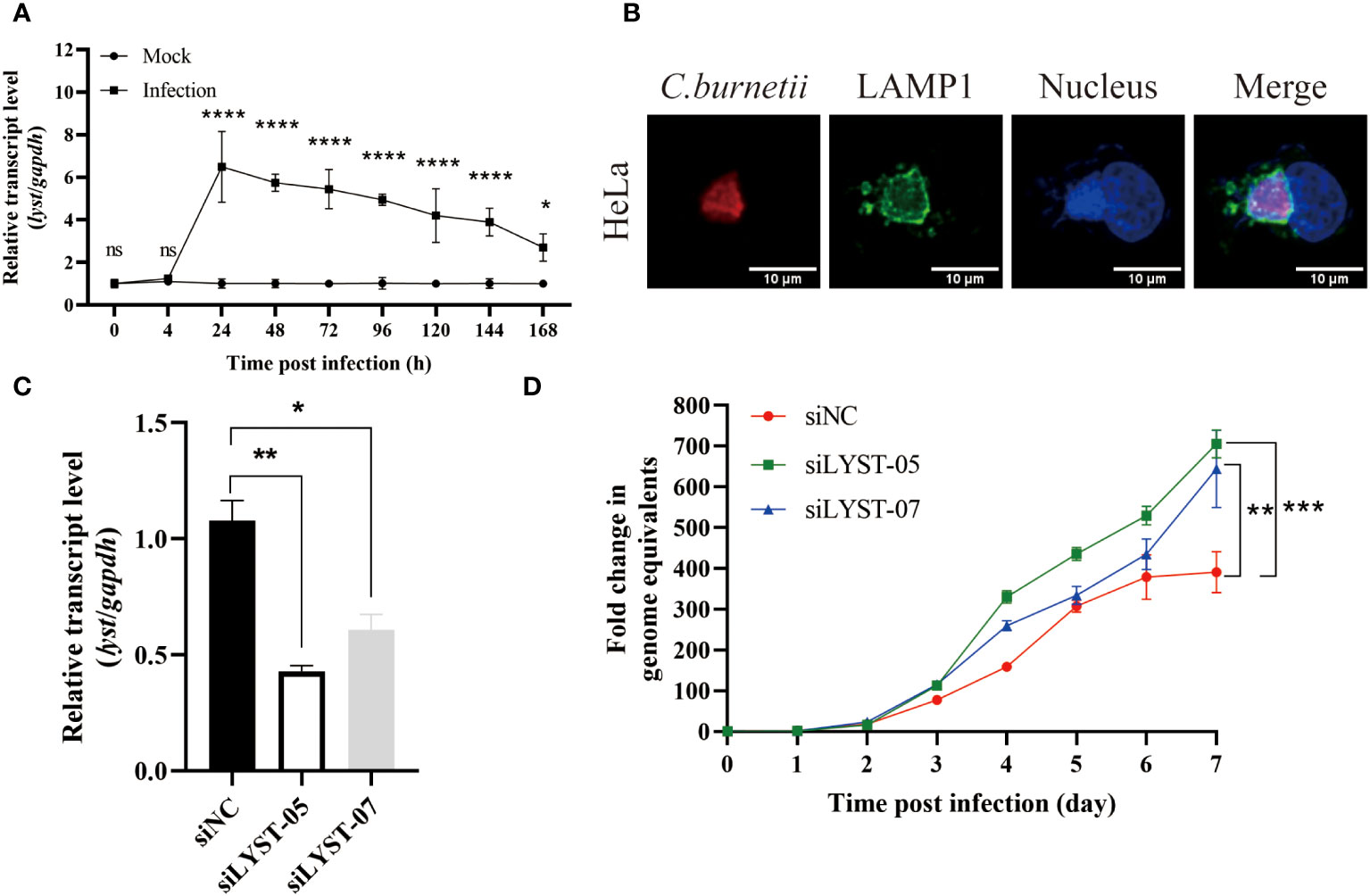
Figure 1 Phenotype of HeLa cells infected with C. burnetii. (A) The relative mRNA expression level of lyst in HeLa cells was analyzed after C. burnetii infection. Data are representative of three independent experiments and bars represent the mean ± SD. *, p<0.05, **, p<0.01, ***, p<0.001, ****, p<0.0001, ns, not significant; (B) CCVs (green) in HeLa cells were stained at 3 days post infection; (C) Inhibition efficiency of siRNA on lyst mRNA expression in HeLa cells. Data are representative of three independent experiments and bars represent the mean ± SD. *, p<0.05, **, p<0.01; (D) The growth curve of C. burnetii in HeLa cells. Data are representative of three independent experiments and bars represent the mean ± SD. **, p<0.01, ***, p<0.001 in comparison with control group at the day 7.
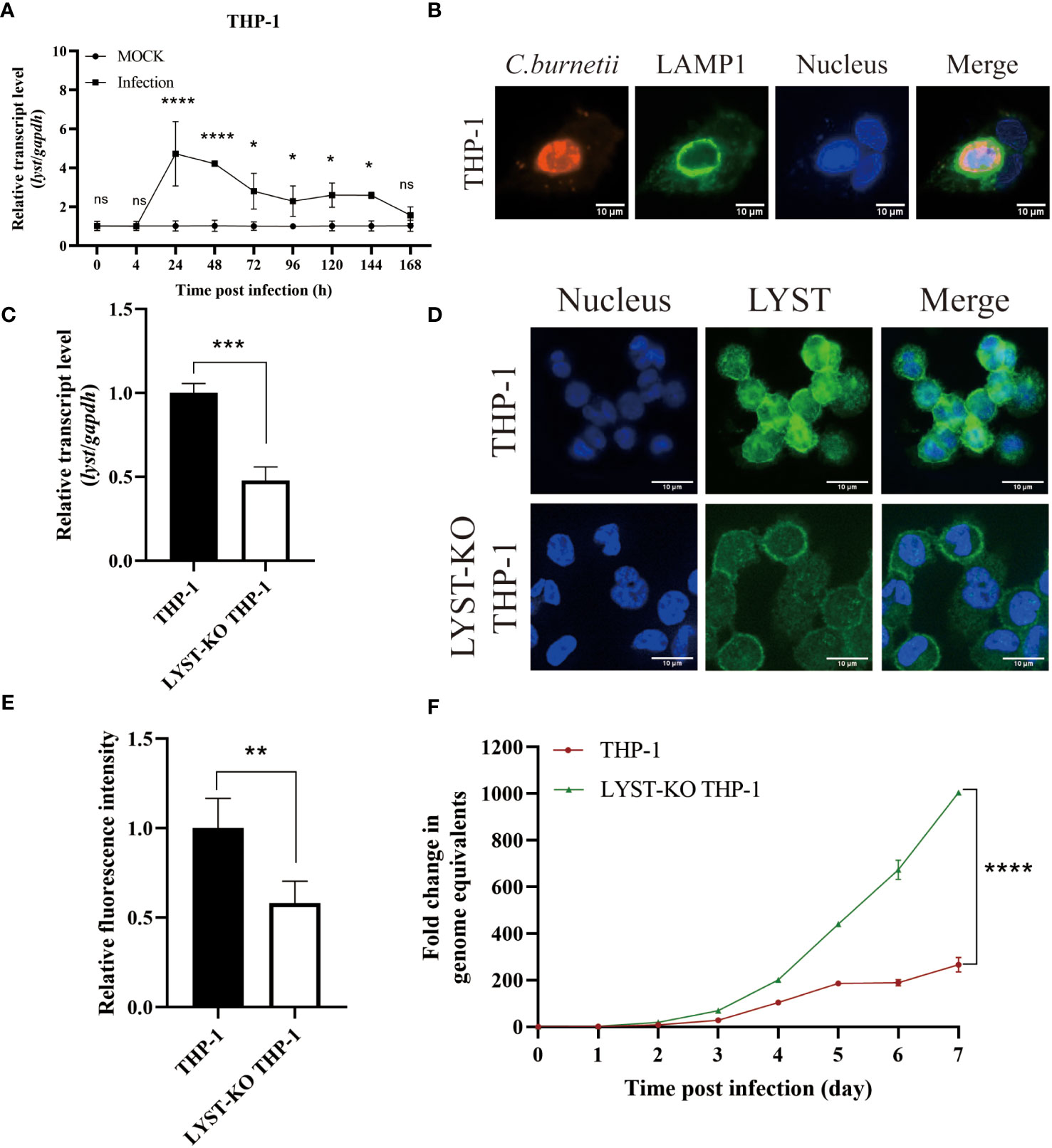
Figure 2 Phenotype of THP-1 cells in infected with C. burnetii. (A) The relative mRNA expression level of lyst in THP-1 cells was analyzed after C. burnetii infection. Data are representative of three independent experiments and bars represent the mean ± SD. *, p<0.05, ****, p<0.0001, ns, not significant; (B) CCVs (green) in THP-1 cells were stained at 3 days post infection; (C) Knockout efficiency of lyst mRNA expression in LYST-KO THP-1 cells. Data are representative of three independent experiments and bars represent the mean ± SD. ***, p<0.001; (D) LYST (green) was stained in THP-1 cells and LYST-KO THP-1 cells; (E) Relative fluorescence intensity of LYST in THP-1 cells and LYST-KO THP-1 cells. Data are representative of three independent experiments and bars represent the mean ± SD. **, p<0.01; (F) The growth curve of C. burnetii in THP-1 cells and LYST-KO THP-1 cells. Data are representative of three independent experiments and bars represent the mean ± SD. ****, p<0.0001 in comparison with control group at the day 7.
To assess the impact of LYST on intracellular replication of C. burnetii, HeLa cells were transfected with siRNAs (siLYST-05 or siLYST-07) to silence the expression of lyst, achieving an inhibition efficiency of approximately 50% (Figure 1C). Subsequently, the lyst-silenced HeLa cells were infected with C. burnetii, and then C. burnetii load was detected. As a result, the intracellular replication level of C. burnetii was enhanced significantly in the lyst-silenced cells (Figure 1D).
THP-1 cells were notoriously difficult to transfect as almost all well-established transfection approaches (Lorkowski et al., 2014; Tang et al., 2018), so we used LYST-knockout THP-1 (purchased from Cyagen, China) to investigate the effect of LYST on the intracellular reproduction of C. burnetii in THP-1 cells. The knockout efficiency of LYST in THP-1 was about 50% by qRT-PCR and cellular immunofluorescence assay (Figures 2C–E). At the same time, C. burnetii were infected with the LYST-knockout THP-1 cells and the bacterial load of C. burnetii was significantly increased (Figure 2F).
In conclusion, the expression level of lyst was significantly up-regulated in both HeLa cells and THP-1 cells after C. burnetii infection, and C. burnetii load were significantly increased in the lyst gene knockdown/konckout host cells, suggesting that LYST inhibits intracellular replication of C. burnetii.
The level of lyst expression correlates with the Dot/Icm type IV secretion system of C. burnetii
In the course of infection, a variety of effectors of C. burnetii with different functions were delivered through T4SS and interact with the functional regulatory molecules of host cells to promote the development of CCVs and enhance its intracellular replication.
In order to explore the relationship between the up-regulation of lyst expression after infection and the secretion of C. burnetii effectors, THP-1 cells were differentiated and infected with C. burnetii pJb-TEM1-CvpE [positive control, a T4SS effector (Lührmann et al., 2010; Larson et al., 2015)], C. burnetii pJb-TEM1-Cbu1754 [negative control, a structural protein (Valdivia et al., 2014; Steiner et al., 2021)], dotA::Tn pJb-TEM1-CvpE or icmD::Tn pJb-TEM1-CvpE and subjected to TEM translocation assays according to the LiveBLAzer™ FRET—B/G Loading Kit. The results showed that dotA::Tn and icmD::Tn did not transport 3×Flag-TEM1-CvpE to the host cytoplasm (Figures 3A–C).
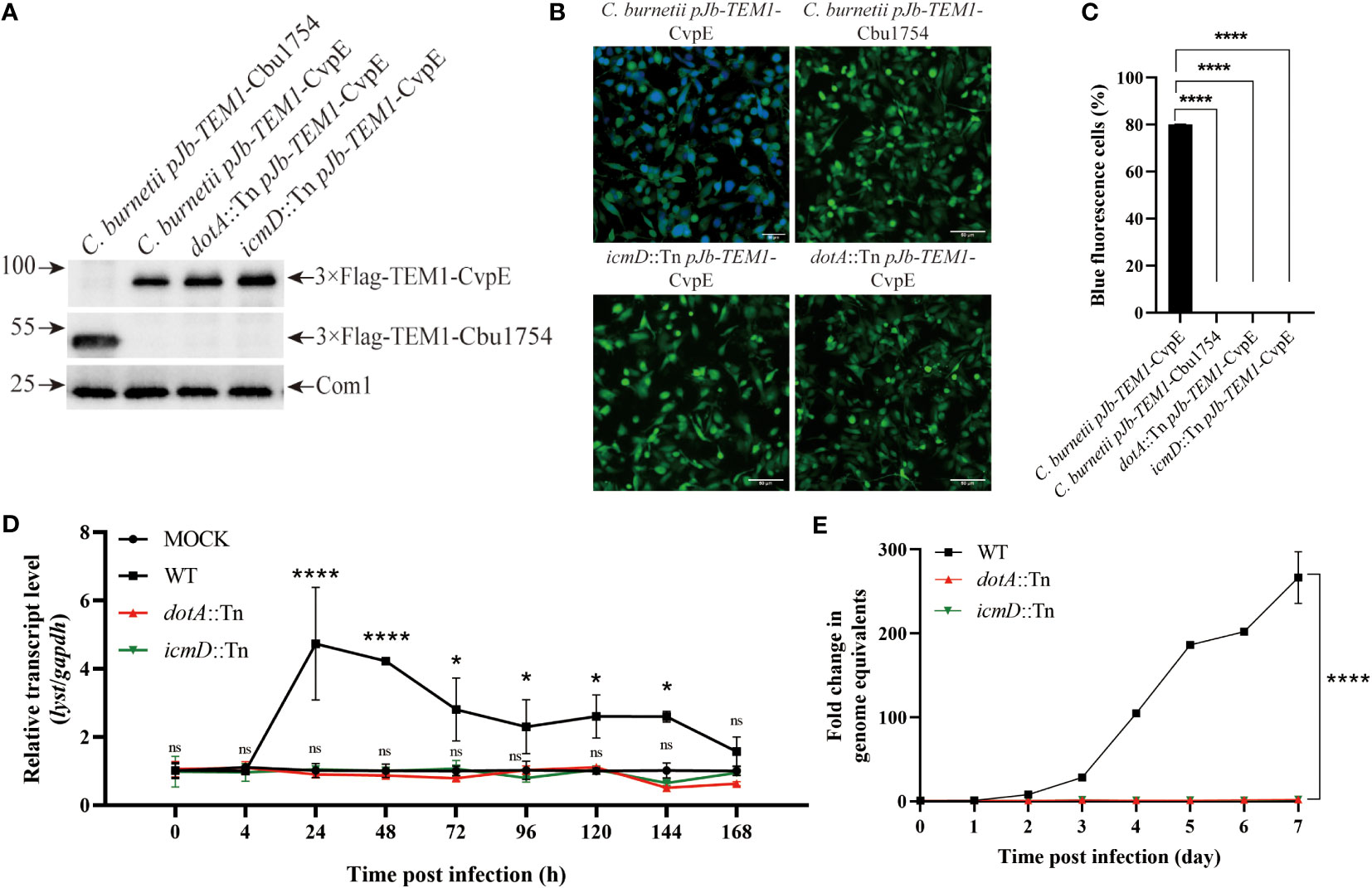
Figure 3 Identification of the up-regulation of lyst expression level in host cells was related to the secretion of C. burnetii effectors. (A) The fusion proteins expression of C. burnetii pJb-TEM1-CvpE, C. burnetii pJb-TEM1-Cbu1754, dotA::Tn pJb-TEM1-CvpE or icmD::Tn pJb-TEM1-CvpE cultured in ACCM-2 for 7 days were detected by Western blotting. (B) TEM translocation assays of C. burnetii pJb-TEM1-CvpE, C. burnetii pJb-TEM1-Cbu1754, dotA::Tn pJb-TEM1-CvpE or icmD::Tn pJb-TEM1-CvpE. (C) Proportion of blue fluorescence cells in each group. Data are representative of three independent experiments and bars represent the mean ± SD. ****, p<0.0001; (D) The relative mRNA expression level of lyst in THP-1 cells was analyzed after dotA::Tn, icmD::Tn or wile type C. burnetii infection. Data are representative of three independent experiments and bars represent the mean ± SD. *, p<0.05, ****, p<0.0001, ns, not significant; (E) The growth curve of dotA::Tn, icmD::Tn or wile type C. burnetii in THP-1 cells. Data are representative of three independent experiments and bars represent the mean ± SD. ****, p<0.0001 in comparison with control group at the day 7.
Then THP-1 cells were infected with wile type C. burnetii, dotA::Tn or icmD::Tn and the transcription level of lyst mRNA and intracellular replication level within 7 days were detected. The results showed that dotA::Tn and icmD::Tn infection had no effect on lyst expression (Figure 3D) and two strains hardly prolifed in host cells (Figure 3E), indicating that C. burnetii regulates lyst expression by effectors secretion, and affects CCVs development and intracellular replication of C. burnetii.
LYST inhibits the intracellular proliferation of C. burnetii by reducing CCVs size
With the infection of C. burnetii infection, a mature and huge CCV will gradually form in the host cell, which provides an acidic environment (pH~5.0) and adequate nutrients for the intracellular growth of C. burnetii (Kohler and Roy, 2015). After C. burnetii infection, both the CCVs size and the C. burnetii loads were significantly increased in the lyst-silenced cells compared with the unsilenced cells (Figures 4A, B), suggesting that LYST inhibits the intracellular proliferation of C. burnetii by reducing CCVs size in host cells.
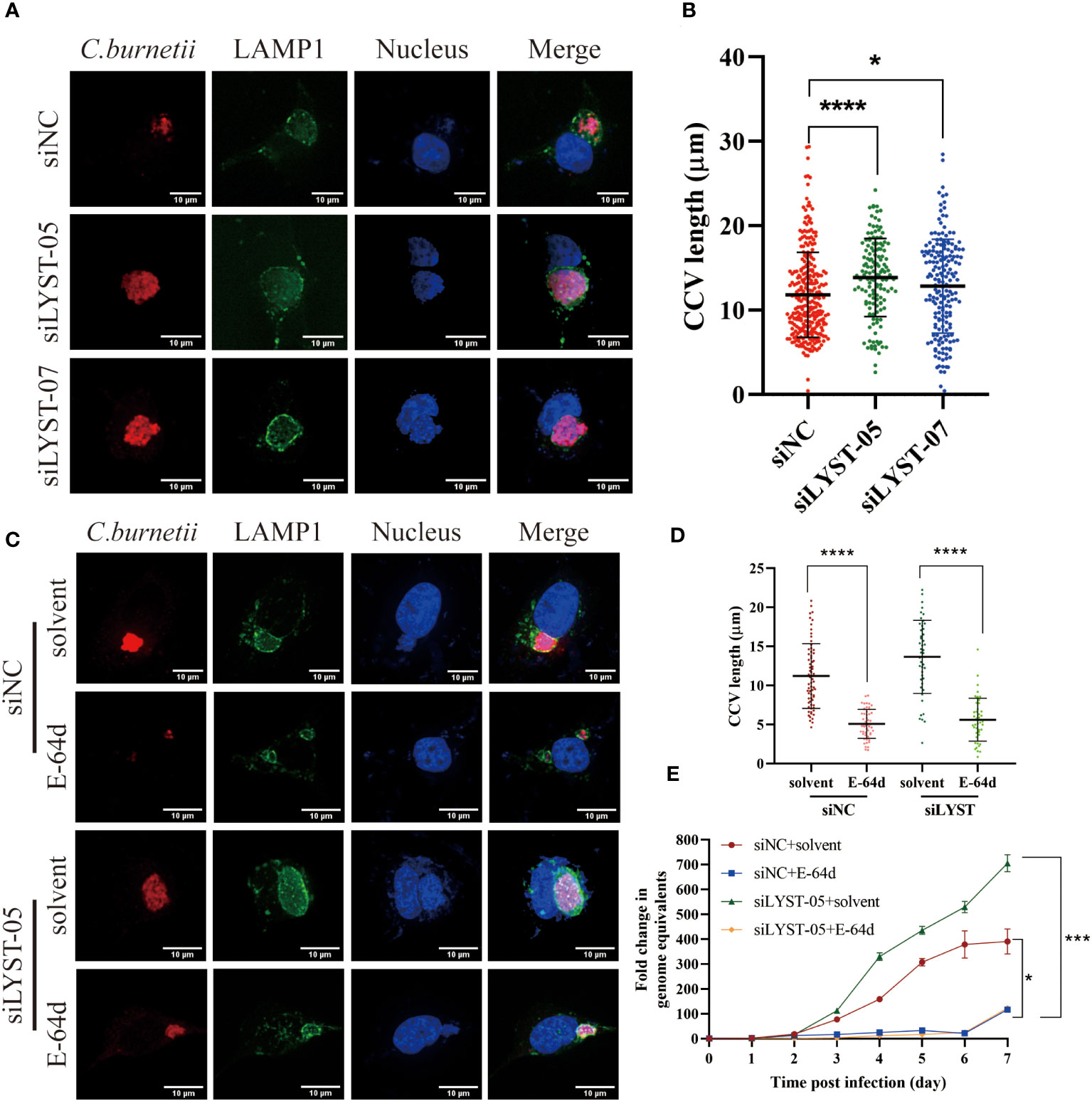
Figure 4 LYST inhibits the intracellular growth of C. burnetii by reducing CCVs expansion. (A) HeLa cells were transfected by different siRNAs, and CCVs (Green) were detected at 5 days post infection; (B) HeLa cells were transfected by different siRNAs, and CCV diameters were compared at 5 days post infection. Data are representative of two independent experiments and bars represent the mean ± SD. *, p<0.05, ****, p<0.0001.C. HeLa cells were transfected with siRNAs for 2 days and then pretreated with E-64d, following by infected with C. burnetii, CCVs (green) in HeLa cells were stained at 5 days post infection; (D) HeLa cells were transfected with siRNAs for 2 days and then pretreated with E-64d, CCV diameters were measured. Data are representative of two independent experiments and bars represent the mean ± SD. ****, p<0.0001; (E) HeLa cells were transfected with siRNAs for 2 days and then pretreated with E-64d, intracellular growth curves of C. burnetii were analyzed. Data are representative of three independent experiments and bars represent the mean ± SD. *, p<0.05, ***, p<0.001 in comparison with control group at the day 7.
Aloxistatin (E-64d), a cysteine proteases inhibitor, has been shown to inhibit the enlargement of cellular lysosomes (Tanabe et al., 2000; Huynh et al., 2004; Yamada et al., 2005) to restrict the intracellular proliferation of pathogenic microorganisms (Beverley et al., 2008). To investigate whether LYST hinders the intracellular replication of C. burnetii by suppressing CCVs expansion, HeLa cells were treated with E-64d and then infected with C. burnetii. The result showed that both the CCVs size and the intracellular C. burnetii load were significantly reduced in E-64d treated cells compared with the E-64d untreated cells (Figures 4C, D). These findings indicate that E-64d effectively inhibited the intracellular proliferation of C. burnetii by suppressing CCVs expansion. Furthermore, when lyst-silenced HeLa cells were pretreated with E-64d, both the CCVs size (Figures 4C, D) and the C. burnetii load (Figure 4E) were significantly reduced, also indicating that LYST plays a role in inhibiting intracellular proliferation of C. burnetii through reducing the size of CCVs.
Regulation of nos2 expression by LYST affects intracellular proliferation of C. burnetii
Inducible nitric oxide synthase (iNOS) is encoded by nitric oxide synthase 2 gene (nos2) in macrophages. It can be induced and activated by many compounds such as bacterial lipopolysaccharide (LPS), type I/II interferon, and cytokines to stimulate macrophages to release nitric oxide (NO), a powerful bactericide (Zamboni and Rabinovitch, 2004; Hill et al., 2011), which effectively inhibits the proliferation of intracellular bacteria (Howe et al., 2002; Matthiesen et al., 2023). This process is one of the important bactericidal mechanisms of macrophages (Kleinert et al., 2004; Tailleux et al., 2011; Schairer et al., 2014; Bogdan, 2015). The use of nitric oxide synthase inhibitor (NG-Monomethyl-L-arginine, L-NMMA) effectively inhibits the activity of iNOS (Chakravortty et al., 2002; Brennan et al., 2004).
To investigate the potential impact of LYST on the intracellular proliferation of C. burnetii by activating the expression of nos2, the LYST-knockout THP-1 cells were induced to adhere in advance and subsequently infected with C. burnetii. The total RNA samples extracted from the infected cells at different time points were used to detect the expression of nos2 mRNA in the host cells, and the supernatants of cell culture were quantitatively detected iNOS by ELISA. The results showed that a significant decrease both in nos2 mRNA level and iNOS content in LYST-knockdown THP-1 cells infected with C. burnetii compared with those of the infected normal THP-1 cells (Figures 5A, B).
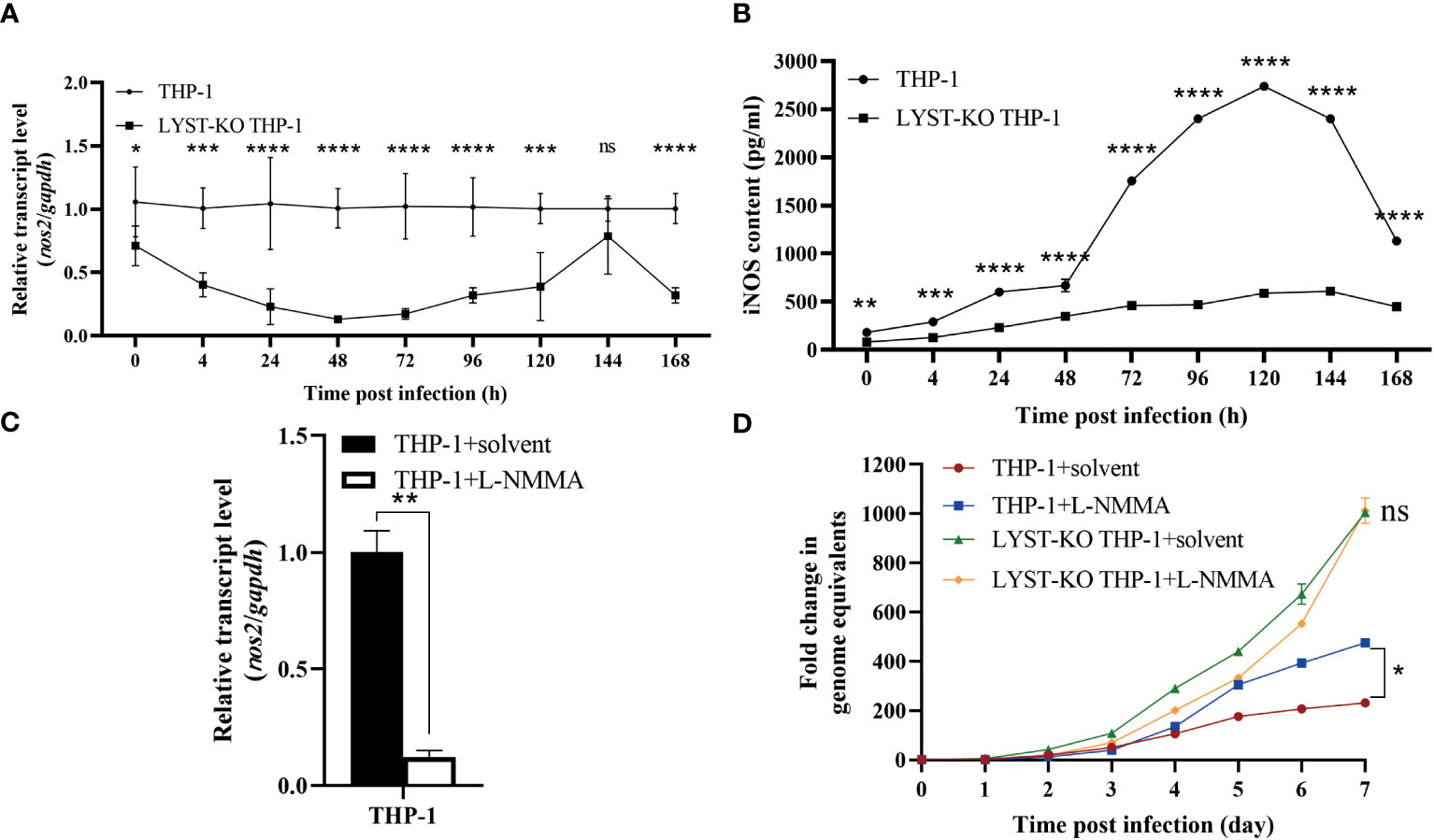
Figure 5 LYST inhibits the intracellular reproduction of C. burnetii by upregulating nos2 expression in host cells. (A) The expression level of nos2 in THP-1 cells after infection with C. burnetii. Data are representative of three independent experiments and bars represent the mean ± SD. *, p<0.05, **, p<0.01, ***, p<0.001, ****, p<0.0001, ns, not significant; (B) The iNOS content was measured in THP-1 cells after C. burnetii infection. Data are representative of three independent experiments and bars represent the mean ± SD. *, p<0.05, **, p<0.01, ***, p<0.001, ****, p<0.0001; (C) Relative expression level of nos2 in THP-1 cells pretreated with L-NMMA. Data are representative of three independent experiments and bars represent the mean ± SD. **, p<0.01; (D) Intracellular growth curves of C. burnetii in THP-1 cells pretreated with L-NMMA. Data are representative of three independent experiments and bars represent the mean ± SD. *, p<0.05, ***, p<0.001, ns, not significant in comparison with control group at the day 7.
Then the THP-1 cells were pretreated with L-NMMA to suppress nos2 expression with about 88% inhibition efficiency (Figure 5C). After infection with C. burnetii, the L-NMMA-treated THP-1 cells were significantly enhanced in intracellular C. burnetii load compared to that of controls (Figure 5D), suggesting that the expression of nos2 inhibits the intracellular proliferation of C. burnetii. However, there was no significant difference in intracellular C. burnetii load between LYST-knockdown THP-1 cells pretreated with L-NMMA and untreated LYST-knockdown THP-1 cells (Figure 5D), suggesting that LYST inhibits the reproduction of C. burnetii by inducing nos2 expression in host cells.
Discussion
C. burnetii is an obligate intracellular Gram-negative bacterium. After infecting host cells, it will be phagocytosed to form a phagosome which developed into CCV to provide a large and suitable environment and abundant nutrients for the growth and reproduction of C. burnetii in the cytosol of host cells (Christie et al., 2011; Kohler and Roy, 2015). Therefore, the development and maturation of CCV is a necessary for C. burnetii to multiply in host cells and sustain stable infection (Best and Abu Kwaik, 2019).
LYST, a member of the BEACH family (Cullinane et al., 2013), is a transporter protein that regulates the transport of small vesicles to lysosomes in host cells (Holland et al., 2014). Loss of functional LYST results in the abnormal maturation of phagosomes and enlargement of lysosomal associated organelles (Barbosa et al., 1996; Kaplan et al., 2008). Studies have shown that lyst gene-deficient mice exhibited increased susceptibility to bacteria (Westphal et al., 2017). Furthermore, study showed that host cells could inhibit intracellular growth of Leishmania amazonensis by suppressing the development of parasitic vesicles through LYST (Beverley et al., 2008). This process may be an innate immune response of host cells against intracellular pathogens. However, whether LYST affects the replication of C. burnetii in host cells remains unclear currently. This study aims to conduct an initial investigation into the influence of LYST on the intracellular reproduction of C. burnetii.
In our study, it was found that after infection with C. burnetii, the mRNA expression level of lyst in host cells was up-regulated, while both the CCVs size and C. burnetii load in the host cells silenced with lyst were increased significantly compared with those of the normal cell control. Therefore, it is extrapolated that LYST might inhibit the intracellular proliferation of C. burnetii by suppressing CCVs expansion after infection. This conjecture was confirmed by the experiment in the host cells pretreated with E-64d, a drug that inhibit the expansion of vesicles in cells, and the host cells pretreated with E-64d showed smaller CCVs and lower bacterial loads after infection with C. burnetii.
Since LYST can specifically affect the TLR3/TRIF-mediated endosomal TLR signal transduction pathway (Westphal et al., 2017), and the upregulation of nos2 is partly dependent on TLR3/TRIF (Zhang et al., 2016), we used RT-qPCR and ELISA to respectively detect the mRNA expression level of nos2 and iNOS content in LYST-knockout THP-1 cells infected with C. burnetii at different times. It was found that the mRNA expression level of nos2 and the content of iNOS in LYST-knockout THP-1 cells were significantly lower than those in normal THP-1 cells controls, while the loads of C. burnetii was significantly enhanced in LYST-knockout THP-1 cells and the THP-1 cells pretreated with a competitive inhibitor of nitric oxide synthase (L-NMMA).
Conclusion
In summary, our study found that the transcription of lyst, a gene previously shown to regulate lysosome size, was up-regulated in host cells after infection with C. burnetii, which were confirmed by experiments in the present study, demonstrating that the expression of lyst in host cells after infection is related to the effector secretion of C. burnetii and LYST not only inhibits the expansion of CCVs, thereby reducing the space for the growth and reproduction of C. burnetii, but also results in up-regulation of nos2 expression by activating the innate immune response of macrophages to catalyze the production of nitric oxide (NO), a key defense molecule. By the combination of the two pathways, the growth and reproduction of C. burnetii could be effectively inhibited in macrophages. Therefore, the results in the present study lay a theoretic basis for developing novel drugs against C. burnetii and providing novel therapeutic strategies for Q fever.
Materials and methods
Bacterial strains and cell culture
The C. burnetii Nine Mile RSA439, phase II strain, clone 4 (NMII) was preserved in our laboratory and propagated in freshly prepared modified acidified citrate cysteine medium (ACCM-2) at 37°C with 5% CO2 and 2.5% O2.
HeLa cells were purchased from ATCC and cultured in DMEM containing 10% fetal bovine serum (FBS) at 37°C with 5% CO2. THP-1 cells were purchased from ATCC and grown in RPMI 1640 medium with 10% FBS and 0.1% 2-mercaptoethanol at 37°C with 5% CO2.
Cell processing and immunofluorescence staining of the CCVs membrane and LYST
Before the experiment, Hela cells or THP-1 cells were seeded in a 12-well cell culture plate at the required cell density. Aloxistatin (E-64d, MCE, E8640) was added to monolayer cells at 1 μM for 4 hours prior to infection. NG-Monomethyl-L-arginine (L-NMMA, MCE, M7033) was added to monolayer cells at 50 μM for 4 hours prior to infection. Cells were washed three times with sterile PBS and then infected with C. burnetii at a multiplicity of infection (MOI) of 2.
For staining the CCVs, cells were treated as follow at 5 dpi: 1) cells were fixed with 4% paraformaldehyde for 15 minutes; 2) cells were permeabilized with 0.2% TritonX-100 for 15 minutes; 3) incubated with 1% BSA at room temperature for 30 minutes to block the cells; 4) anti-C. burnetii serum (prepared in our laboratory) and goat anti-mouse IgG Alexa Flour 594 antibody (Abcam, ab150116), anti-LAMP-1 antibody (CTS, 9091S) and goat anti-rabbit IgG Alexa Flour 488 antibody (Abcam, ab150113) were incubated successively, and each antibody was incubated at room temperature for 1 hour; 5) After washing with PBS, the cell nuclei were stained with DAPI at room temperature for 3 to 5 minutes. After each staining step, wash 3 times with sterile PBS for 5 minutes each time; 6) the coverslips were removed and mounted on slides with Prolong Gold antifade mounting solution (Thermo, p36935) to observe CCVs, and the results were photographed and saved.
For staining the LYST, THP-1 cells and LYST-KO THP-1 cells were differentiated with PMA (200 nM) for 48 h, 1) the cells were fixed, permeabilized and block (as show above); 2) anti-LYST antibody (Abcam, ab220481) and goat anti-rabbit IgG Alexa Flour 488 antibody (Abcam, ab150113) were incubated successively, and each antibody was incubated at room temperature for 1 hour; 3) follow-up operations are as described previously; 4) the relative fluorescence intensity of 488nm was calculated.
Electroporation of C. burnetii with plasmids
The pJb-TEM1-CvpE and pJb-TEM1-Cbu1754 were introduced into electrocompetent wild type C. burnetii respectively, and additionally, pJb-TEM1-CvpE was introduced into electrocompetent two T4SS mutant stains (dotA::Tn and icmD::Tn) at 1800 V, 500 Ω, 25 µF and duration between 9-13 msec. Immediately, 900 µl of RPMI 1640 medium was added into the mixture at room temperature (Bonazzi et al., 2015). Subsequently, the electroporated bacteria was inoculated into 5 ml of ACCM-2 containing 1% FBS and cultured at 37 °C, 2.5% O2, 5% CO2 for 7 days. Finally, 100 µl of the culture is coated with ACCM-2 plates containing kanamycin (375 µg/ml) and 0.2% agarose to isolate individual C. burnetii pJb-TEM1-CvpE, C. burnetii pJb-TEM1-Cbu1754, dotA::Tn pJb-TEM1-CvpE and icmD::Tn pJb-TEM1-CvpE.
TEM translocation assays
THP-1 cells were differentiated into adherent, macrophage- like cells and infected with C. burnetii strains carrying β-lactamase fusions at a MOI of 100. 6X CCF4-AM Substrate Loading Solution to cells to 1X final concentration at 1 day post infection and incubate the plate at room temperature for 60–120 minutes. Samples were observed under a fluorescence microscope and detection of the blue coumarin emission (~450 nm) and green fluorescein emission (~520 nm), and positive cells were marked blue.
Gene silencing and efficiency detection
HeLa cells were seeded in the cell culture plate at a density of 1×105 cells/ml in advance. After the cells adhere to the well, two lyst-specific siRNA (siLYST-05 and siLYST-04, Table 1) were transfected into HeLa cells with Lipofectamine RNAiMAX Reagent for 2 days. The total cellular RNA was extracted and reverse-transcribed into cDNA by TransScript one-step method. Subsequently, the cDNA was analyzed for lyst transcription levels by RT-qPCR, with the required primers listed in Table 2. The expression level was determined relative to the internal reference gene (gapdh).
Detection of intracellular growth of C. burnetii and cell nos2 expression level
The cells were infected with C. burnetii at a MOI of 2, and then the unabsorbed C. burnetii was washed away with sterile PBS 4 hours later. Cell samples were collected every 24 hours to extract DNA, and DNA samples were detected by qPCR to determine the bacterial loads of C. burnetii (primers such as Table 3).
According to the above infection method, THP-1 cells were infected with C. burnetii. Total RNA was extracted at different time points after infection, and the relative expression level of nos2 was detected by RT-qPCR (required primers such as Table 2). At the same time, the supernatant of cell culture was collected and the content of iNOS was detected by Human iNOS ELISA KIT.
Data availability statement
The original contributions presented in the study are included in the article/supplementary material. Further inquiries can be directed to the corresponding author.
Author contributions
WW: Investigation, Methodology, Writing – original draft. SZ: Investigation, Writing – original draft. MZ: Formal analysis, Writing – original draft. XO: Formal analysis, Writing – original draft. YY: Formal analysis, Writing – original draft. XX: Formal analysis, Writing – review & editing. NZ: Writing – review & editing. JJ: Funding acquisition, Project administration, Writing – review & editing.
Funding
The author(s) declare financial support was received for the research, authorship, and/or publication of this article. This work was supported by the National Natural Science Foundation of China [32000139] and the State Key Laboratory of Pathogen and Biosecurity (Academy of Military Medical Science) [SKLPBS2217].
Conflict of interest
The authors declare that the research was conducted in the absence of any commercial or financial relationships that could be construed as a potential conflict of interest.
Publisher’s note
All claims expressed in this article are solely those of the authors and do not necessarily represent those of their affiliated organizations, or those of the publisher, the editors and the reviewers. Any product that may be evaluated in this article, or claim that may be made by its manufacturer, is not guaranteed or endorsed by the publisher.
References
Barbosa, M. D. F. S., Nguyen, Q. A., Tchernev, V. T., Ashley, J. A., Detter, J. C., Blaydes, S. M., et al. (1996). Identification of the homologous beige and Chediak–Higashi syndrome genes. Nature 382, 262–265. doi: 10.1038/382262a0
Beare, P. A., Gilk, S. D., Larson, C. L., Hill, J., Stead, C. M., Omsland, A., et al. (2011). Dot/Icm type IVB secretion system requirements for coxiella burnetii growth in human macrophages. mBio 2, e00175-11. doi: 10.1128/mBio.00175-11
Best, A., Abu Kwaik, Y. (2019). Nutrition and bipartite metabolism of intracellular pathogens. Trends Microbiol. 27, 550–561. doi: 10.1016/j.tim.2018.12.012
Beverley, S. M., Wilson, J., Huynh, C., Kennedy, K. A., Ward, D. M., Kaplan, J., et al. (2008). Control of parasitophorous vacuole expansion by LYST/beige restricts the intracellular growth of leishmania amazonensis. PloS Pathog. 4 (10), e1000179. doi: 10.1371/journal.ppat.1000179
Bogdan, C. (2015). Nitric oxide synthase in innate and adaptive immunity: an update. Trends Immunol. 36, 161–178. doi: 10.1016/j.it.2015.01.003
Bonazzi, M., Cantet, F., Martinez, E. (2015). Generation and multi-phenotypic high-content screening of coxiella burnetii transposon mutants. J. Vis. Exp. (99), e52851. doi: 10.3791/52851
Brennan, R. E., Russell, K., Zhang, G., Samuel, J. E. (2004). Both inducible nitric oxide synthase and NADPH oxidase contribute to the control of virulent phase I Coxiella burnetii Infections. Infect. Immun. 72, 6666–6675. doi: 10.1128/IAI.72.11.6666-6675.2004
Burgess, A., Mornon, J. P., de Saint-Basile, G., Callebaut, I. (2009). A concanavalin A-like lectin domain in the CHS1/LYST protein, shared by members of the BEACH family. Bioinformatics 25, 1219–1222. doi: 10.1093/bioinformatics/btp151
Chakravortty, D., Hansen-Wester, I., Hensel, M. (2002). Salmonella pathogenicity island 2 mediates protection of intracellular salmonella from reactive nitrogen intermediates. J. Exp. Med. 195, 1155–1166. doi: 10.1084/jem.20011547
Christie, P., Carey, K. L., Newton, H. J., Lührmann, A., Roy, C. R. (2011). The Coxiella burnetii Dot/Icm System Delivers a Unique Repertoire of Type IV Effectors into Host Cells and Is Required for Intracellular Replication. PloS Pathog. 7 (5), e1002056. doi: 10.1371/journal.ppat.1002056
Cullinane, A. R., Schäffer, A. A., Huizing, M. (2013). The BEACH is hot: A LYST of emerging roles for BEACH-domain containing proteins in human disease. Traffic 14, 749–766. doi: 10.1111/tra.12069
Eldin, C., Mélenotte, C., Mediannikov, O., Ghigo, E., Million, M., Edouard, S., et al. (2017). From Q fever to coxiella burnetii infection: a paradigm change. Clin. Microbiol. Rev. 30, 115–190. doi: 10.1128/CMR.00045-16
El-Mahallawy, H. S., Kelly, P., Zhang, J., Yang, Y., Wei, L., Tian, L., et al. (2015a). Serological and molecular evidence of Coxiella burnetii in samples from humans and animals in China. Ann. Agric. Environ. Med. 23, 87–91. doi: 10.5604/12321966.1196859
El-Mahallawy, H. S., Lu, G., Kelly, P., Xu, D., Li, Y., Fan, W., et al. (2015b). Q fever in China: a systematic review, 1989-2013. Epidemiol. Infect. 143, 673–681. doi: 10.1017/S0950268814002593
Georgiev, M., Afonso, A., Neubauer, H., Needham, H., Thiéry, R., Rodolakis, A., et al. (2013). Q fever in humans and farm animals in four European countries, 1982 to 2010. Eurosurveillance 18 (8), pii=20407. doi: 10.2807/ese.18.08.20407-en
Ghanem-Zoubi, N., Karram, T., Kagna, O., Merhav, G., Keidar, Z., Paul, M. (2021). Q fever vertebral osteomyelitis among adults: a case series and literature review. Infect. Dis. 53, 231–240. doi: 10.1080/23744235.2020.1871508
Hill, J., Samuel, J. E., Fang, F. C. (2011). Coxiella burnetii Acid phosphatase inhibits the release of reactive oxygen intermediates in polymorphonuclear leukocytes. Infect. Immun. 79, 414–420. doi: 10.1128/IAI.01011-10
Holland, P., Torgersen, M. L., Sandvig, K., Simonsen, A. (2014). LYST affects lysosome size and quantity, but not trafficking or degradation through autophagy or endocytosis. Traffic 15, 1390–1405. doi: 10.1111/tra.12227
Howe, D., Barrows, L. F., Lindstrom, N. M., Heinzen, R. A. (2002). Nitric oxide inhibits Coxiella burnetii Replication and parasitophorous vacuole maturation. Infect. Immun. 70, 5140–5147. doi: 10.1128/IAI.70.9.5140-5147.2002
Huang, M., Ma, J., Jiao, J., Li, C., Chen, L., Zhu, Z., et al. (2021). The epidemic of Q fever in 2018 to 2019 in Zhuhai city of China determined by metagenomic next-generation sequencing. PloS Negl. Trop. Dis. 15 (7), e0009520. doi: 10.1371/journal.pntd.0009520
Huynh, C., Roth, D., Ward, D. M., Kaplan, J., Andrews, N. W. (2004). Defective lysosomal exocytosis and plasma membrane repair in Chediak–Higashi/beige cells. Proc. Natl. Acad. Sci. 101, 16795–16800. doi: 10.1073/pnas.0405905101
Kaplan, J., De Domenico, I., Ward, D. M. (2008). Chediak-higashi syndrome. Curr. Opin. Hematol. 15, 22–29. doi: 10.1097/MOH.0b013e3282f2bcce
Kazar, J. (2005). Coxiella burnetii infection. Ann. New York Acad. Sci. 1063, 105–114. doi: 10.1196/annals.1355.018
Kleinert, H., Pautz, A., Linker, K., Schwarz, P. M. (2004). Regulation of the expression of inducible nitric oxide synthase. Eur. J. Pharmacol. 500, 255–266. doi: 10.1016/j.ejphar.2004.07.030
Klingenbeck, L., Eckart, R. A., Berens, C., Lührmann, A. (2013). The Coxiella burnetii type IV secretion system substrate CaeB inhibits intrinsic apoptosis at the mitochondrial level. Cell. Microbiol. 15, 675–687. doi: 10.1111/cmi.12066
Kohler, L. J., Roy, C. R. (2015). Biogenesis of the lysosome-derived vacuole containing Coxiella burnetii. Microbes Infect. 17, 766–771. doi: 10.1016/j.micinf.2015.08.006
Larson, C. L., Beare, P. A., Voth, D. E., Howe, D., Cockrell, D. C., Bastidas, R. J., et al. (2015). Coxiella burnetii effector proteins that localize to the parasitophorous vacuole membrane promote intracellular replication. Infect. Immun. 83, 661–670. doi: 10.1128/IAI.02763-14
Lattao, R., Rangone, H., Llamazares, S., Glover, D. M. (2021). Mauve/LYST limits fusion of lysosome-related organelles and promotes centrosomal recruitment of microtubule nucleating proteins. Dev. Cell 56, 1000–1013.e6. doi: 10.1016/j.devcel.2021.02.019
Lorkowski, S., Wittig, B., Maeß, M. B. (2014). Highly efficient transfection of human THP-1 macrophages by nucleofection. J. Vis. Exp. (91), e51960. doi: 10.3791/51960
Lührmann, A., Nogueira, C. V., Carey, K. L., Roy, C. R. (2010). Inhibition of pathogen-induced apoptosis by a Coxiella burnetii type IV effector protein. Proc. Natl. Acad. Sci. 107, 18997–19001. doi: 10.1073/pnas.1004380107
Mansilla Pareja, M. E., Bongiovanni, A., Lafont, F., Colombo, M. I. (2017). Alterations of the coxiella burnetii replicative vacuole membrane integrity and interplay with the autophagy pathway. Front. Cell Infect. Microbiol. 7, 112. doi: 10.3389/fcimb.2017.00112
Matthiesen, S., Christiansen, B., Jahnke, R., Zaeck, L. M., Karger, A., Finke, S., et al. (2023). TGF-β/IFN-γ Antagonism in subversion and self-defense of phase II coxiella burnetii-infected dendritic cells. Infect. Immun. 91, e0032322. doi: 10.1128/iai.00323-22
Maurin, M., Raoult, D. (1999). Q fever. Clin. Microbiol. Rev. 12, 518–553. doi: 10.1128/CMR.12.4.518
Qin, T., Shi, M., Liu, Z., Feng, H., Sun, Y. (2022). Molecular Detection of Candidatus Coxiella mudorwiae in Haemaphysalis concinna in China. Zoonoses 2 (1). doi: 10.15212/ZOONOSES-2022-0041
Raoult, D., Marrie, T. J., Mege, J. L. (2005). Natural history and pathophysiology of Q fever. Lancet Infect. Dis. 5, 219–226. doi: 10.1016/S1473-3099(05)70052-9
Roy, C. R., Samanta, D., Clemente, T. M., Schuler, B. E., Gilk, S. D. (2019). Coxiella burnetii Type 4B Secretion System-dependent manipulation of endolysosomal maturation is required for bacterial growth. PloS Pathog. 15 (12), e1007855. doi: 10.1371/journal.ppat.1007855
Schäfer, W., Eckart, R. A., Schmid, B., Cagköylü, H., Hof, K., Muller, Y. A., et al. (2017). Nuclear trafficking of the anti-apoptotic Coxiella burnetii effector protein AnkG requires binding to p32 and Importin-α1. Cell. Microbiol. 19 (1). doi: 10.1111/cmi.12634
Schairer, D. O., Chouake, J. S., Nosanchuk, J. D., Friedman, A. J. (2014). The potential of nitric oxide releasing therapies as antimicrobial agents. Virulence 3, 271–279. doi: 10.4161/viru.20328
Sepulveda, F. E., Burgess, A., Heiligenstein, X., Goudin, N., Ménager, M. M., Romao, M., et al. (2015). LYST controls the biogenesis of the endosomal compartment required for secretory lysosome function. Traffic 16, 191–203. doi: 10.1111/tra.12244
Stein, A., Raoult, D. (1995). Q fever endocarditis. Eur. Heart J. 16, 19–23. doi: 10.1093/eurheartj/16.suppl_B.19
Steiner, S., Meir, A., Roy, C. R. (2021). Coxiella burnetii encodes an LvgA-related protein important for intracellular replication. Cell. Microbiol. 23 (6), e13331. doi: 10.1111/cmi.13331
Straily, A., Dahlgren, F. S., Peterson, A., Paddock, C. D. (2017). Surveillance for Q fever endocarditis in the United States, 1999–2015. Clin. Infect. Dis. 65, 1872–1877. doi: 10.1093/cid/cix702
Tailleux, L., Herbst, S., Schaible, U. E., Schneider, B. E. (2011). Interferon gamma activated macrophages kill mycobacteria by nitric oxide induced apoptosis. PloS One. 6 (5), e19105. doi: 10.1371/journal
Tanabe, F., Cui, S.-H., Ito, M. (2000). Abnormal down-regulation of PKC is responsible for giant granule formation in fibroblasts from CHS (beige) mice—a thiol proteinase inhibitor, E-64-d, prevents giant granule formation in beige fibroblasts. J. Leukocyte Biol. 67, 749–755. doi: 10.1002/jlb.67.5.749
Tang, X., Aljahdali, B., Alasiri, M., Bamashmous, A., Cao, F., Dibart, S., et al. (2018). A method for high transfection efficiency in THP-1 suspension cells without PMA treatment. Anal. Biochem. 544, 93–97. doi: 10.1016/j.ab.2017.12.032
Valdivia, R. H., Newton, H. J., Kohler, L. J., McDonough, J. A., Temoche-Diaz, M., Crabill, E., et al. (2014). A screen of coxiella burnetii mutants reveals important roles for Dot/Icm effectors and host autophagy in vacuole biogenesis. PloS Pathog. 10 (7), e1004286. doi: 10.1371/journal.ppat.1004286
van Roeden, S. E., Reukers, D. F. M., van Jaarsveld, C. H. M., Kampschreur, L. M., Hoepelman, I. M., Wever, P. C., et al. (2018). Chronic Q fever: patient and treatment-related factors influencing long-term quality of life. Qjm 111, 791–797. doi: 10.1093/qjmed/hcy171
Vinetz, J. M., Esmaeili, S., Golzar, F., Ayubi, E., Naghili, B., Mostafavi, E. (2017). Acute Q fever in febrile patients in northwestern of Iran. PloS Negl. Trop. Dis. 11 (4), e0005535. doi: 10.1371/journal.pntd.0005535
Voth, D. E., Beare, P. A., Howe, D., Sharma, U. M., Samoilis, G., Cockrell, D. C., et al. (2011). The coxiella burnetii cryptic plasmid is enriched in genes encoding type IV secretion system substrates. J. Bacteriol. 193, 1493–1503. doi: 10.1128/JB.01359-10
Westphal, A., Cheng, W., Yu, J., Grassl, G., Krautkrämer, M., Holst, O., et al. (2017). Lysosomal trafficking regulator Lyst links membrane trafficking to toll-like receptor–mediated inflammatory responses. J. Exp. Med. 214, 227–244. doi: 10.1084/jem.20141461
Yamada, K., Fuji, K., Shimada, T., Nishimura, M., Hara-Nishimura, I. (2005). Endosomal proteases facilitate the fusion of endosomes with vacuoles at the final step of the endocytotic pathway. Plant J. 41, 888–898. doi: 10.1111/j.1365-313X.2005.02349.x
Zamboni, D. S., Rabinovitch, M. (2004). Phagocytosis of apoptotic cells increases the susceptibility of macrophages to infection with Coxiella burnetii phase II through down-modulation of nitric oxide production. Infect. Immun. 72, 2075–2080. doi: 10.1128/IAI.72.4.2075-2080.2004
Zhang, L., Xiang, W., Wang, G., Yan, Z., Zhu, Z., Guo, Z., et al. (2016). Interferon β (IFN-β) Production during the Double-stranded RNA (dsRNA) Response in Hepatocytes Involves Coordinated and Feedforward Signaling through Toll-like Receptor 3 (TLR3), RNA-dependent Protein Kinase (PKR), Inducible Nitric Oxide Synthase (iNOS), and Src Protein. J. Biol. Chem. 291, 15093–15107. doi: 10.1074/jbc.M116.717942
Keywords: Coxiella burnetii, lysosomal trafficking regulator, Coxiella-containing vacuole, Dot/Icm type IV secretion system, inducible nitric oxide synthase
Citation: Wan W, Zhang S, Zhao M, OuYang X, Yu Y, Xiong X, Zhao N and Jiao J (2024) Lysosomal trafficking regulator restricts intracellular growth of Coxiella burnetii by inhibiting the expansion of Coxiella-containing vacuole and upregulating nos2 expression. Front. Cell. Infect. Microbiol. 13:1336600. doi: 10.3389/fcimb.2023.1336600
Received: 11 November 2023; Accepted: 26 December 2023;
Published: 11 January 2024.
Edited by:
Yong Zhang, Jilin University, ChinaReviewed by:
Wenping Gong, The 8th Medical Center of PLA General Hospital, ChinaChuang Li, Purdue University, United States
Copyright © 2024 Wan, Zhang, Zhao, OuYang, Yu, Xiong, Zhao and Jiao. This is an open-access article distributed under the terms of the Creative Commons Attribution License (CC BY). The use, distribution or reproduction in other forums is permitted, provided the original author(s) and the copyright owner(s) are credited and that the original publication in this journal is cited, in accordance with accepted academic practice. No use, distribution or reproduction is permitted which does not comply with these terms.
*Correspondence: Jun Jiao, amlhb2p1bjUxOTIwQHNpbmEuY29t
†These authors have contributed equally to this work