- Department of Pharmacology, School of Medicine, University of California, Davis, Davis, CA, United States
Cryptococcus neoformans can invade the central nervous system by crossing the blood-brain barrier via a transcellular mechanism that relies on multiple host factors. In this narrative, we review the evidence that a direct interplay between C. neoformans and brain endothelial cells forms the basis for invasion and transmigration across the brain endothelium. Adherence and internalization of C. neoformans is dependent on transmembrane proteins, including a hyaluronic acid receptor and an ephrin receptor tyrosine kinase. We consider the role of EphA2 in facilitating the invasion of the central nervous system by C. neoformans and highlight experimental evidence supporting macropinocytosis as a potential mechanism of internalization and transcytosis. How macropinocytosis might be conclusively demonstrated in the context of C. neoformans is also discussed.
The pathophysiology of Cryptococcal neuro-infection
Diseases caused by fungal pathogens affect over a billion people and kill approximately 1.7 million annually (Bongomin et al., 2017). The severity of fungal disease varies from asymptomatic in healthy hosts to disseminated, life-threatening infections in immunosuppressed individuals. When compared to other infections of the central nervous system (CNS), infections caused by fungi have the highest morbidity and mortality. The primary reasons are that it is nearly impossible to completely eradicate the fungus from the brain and these infections tend to occur in individuals that are highly immunosuppressed, including HIV positive patients, organ transplant recipients and patients undergoing cancer treatments (Williamson et al., 2017).
Among fungal pathogens that can breach the CNS, Cryptococcus neoformans (Cn) is the most prevalent cause of adult brain fungal infection (Bongomin et al., 2017; Rajasingham et al., 2017; Rajasingham et al., 2022). Cryptococcal meningitis (CM) is the typical clinical presentation and cause of death during cryptococcosis (Mitchell and Perfect, 1995; Gottfredsson and Perfect, 2000). Without rapid intervention, CM is uniformly fatal regardless of the immune status of the host. Once inhaled, spores/desiccated yeast of Cn initially proliferate in lung tissue and eventually disseminate to the blood and then the CNS (Ellis and Pfeiffer, 1990; Velagapudi et al., 2009; Walsh et al., 2019), reviewed in (Chen et al., 2022). While dissemination can occur in any tissue, the CNS is the major site of invasion and growth of cryptococci. After breaching the blood-brain barrier (BBB), Cn forms self-contained cystic lesions referred to as cryptococcomas, where fungal cells can proliferate and damage the brain (Kwon-Chung et al., 2000; Park et al., 2009). Brain pathology consists of early cerebral vessel damage followed by fungal proliferation, first in the perivascular spaces and then in the deeper layer of the brain parenchyma with secondary seeding of the leptomeninges. Neurologic sequelae including visual loss, cranial palsies, neurologic deficit and mental impairment occurs in 40-50% of treated patients and 20-25% experience a relapsing course (Traino et al., 2019). Treatment guidelines for HIV-associated CM recommend amphotericin B with flucytosine for greater than 2 weeks as induction therapy followed by a triazole for a minimum of 10 weeks (Perfect et al., 2010). Fifty percent of AIDS patients treated for CM will experience a relapse of the disease unless they receive maintenance therapy. Unfortunately, in Africa and Asia, where disease burden is the highest, flucytosine treatment is prohibitively expensive (Loyse et al., 2013a; Loyse et al., 2013b). Despite the recent increase in antiretroviral therapy (ART) reducing the immunocompromised population in resource-deprived regions, the incidence of CM remains high (Pyrgos et al., 2013; Muzazu et al., 2022).
Neuroinvasion of Cn and the role of the blood-brain barrier
Recent studies have focused on the underlying molecular mechanisms facilitating the translocation of fungi from blood-to-brain along with the structural and molecular changes in the neurovascular unit (NVU) and how that informs neurological changes in the brain. Diffusion of bloodborne molecules across the BBB is highly restricted due to specialized endothelial cells that line the lumen of the brain microvasculature and the tight junction proteins in between endothelial cells that restrict paracellular flow (Keaney and Campbell, 2015). The BBB is part of the larger NVU, including pericytes and perivascular astrocytes that further support BBB function (Zheng, 2001; Weiss et al., 2009). Real-time imaging of mice after inoculation with Cn revealed that translocation of Cn from blood-to-brain begins with capillary sequestration, followed by a direct association between fungal cells and the endothelial surface of the capillary (Shi et al., 2010). This internalization and migration of Cn across the BBB was further confirmed in vivo with a flow cytometry approach that quantified Cn migration into the brain (Chen et al., 2021). This entire process can occur without involvement of macrophages or the breakdown of junctional proteins, suggesting that Cn can interact directly with proteins on the surface of brain endothelial cells (Kozel and Gotschlich, 1982; Ibrahim et al., 1995; Chang et al., 2004; Vu et al., 2009; Huang et al., 2011). However, other studies have clearly demonstrated the presence of the Trojan horse pathway, where Cn resides within host phagocytes on their way to the CNS during the course of infections (Charlier et al., 2009; Sorrell et al., 2016; Santiago-Tirado et al., 2017; Gaylord et al., 2020). The relative contribution to CNS invasion between transcellular crossing, where Cn is first internalized and then eventually exocytosed on the abluminal surface, and the Trojan horse pathway still remains to be resolved (Figure 1).
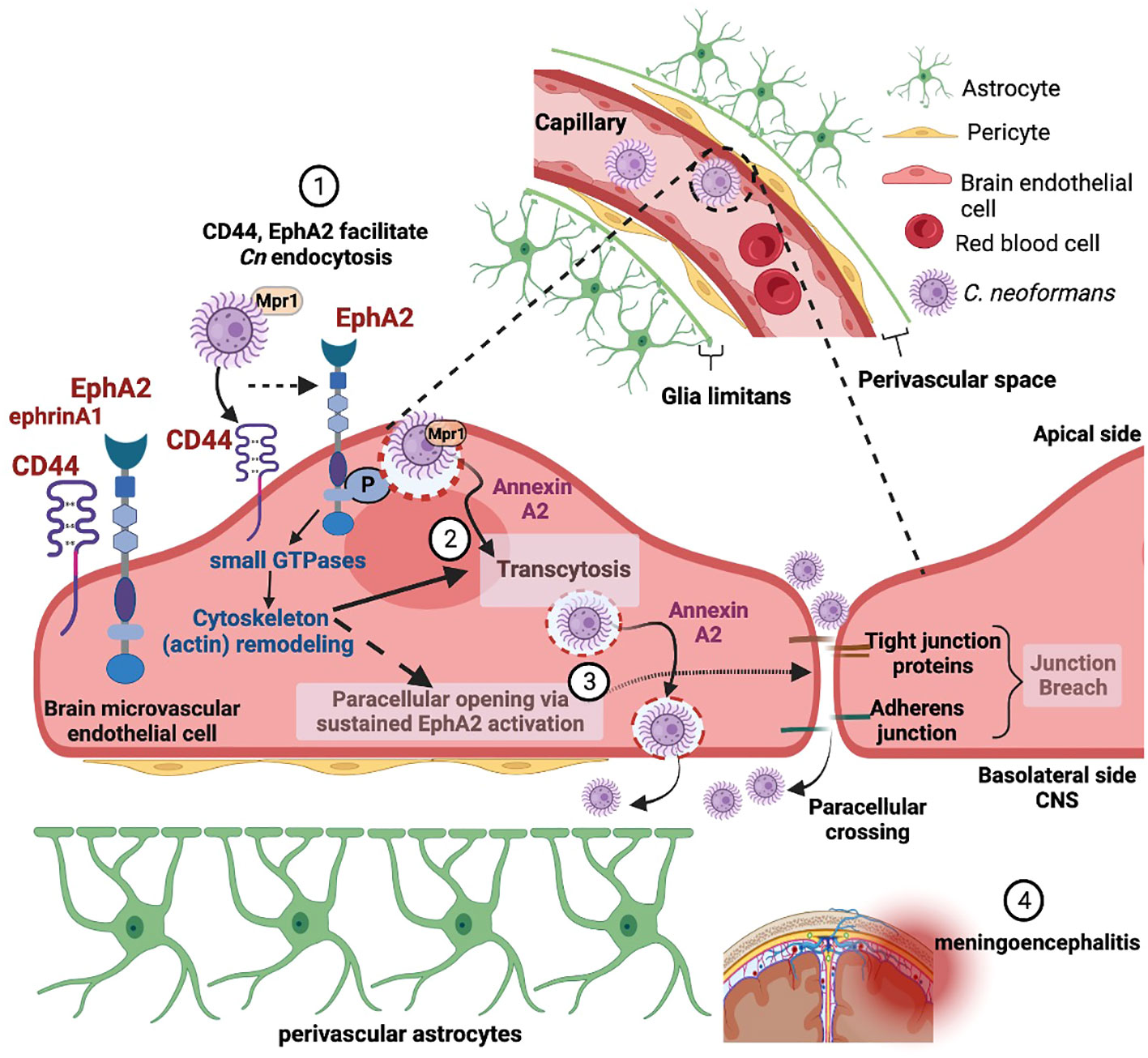
Figure 1 Adherence and internalization of C. neoformans depends on the (1) hyaluronic acid receptor (CD44) and an ephrin receptor tyrosine kinase (EphA2) - triggering membrane and cytoskeleton remodeling (2). EphA2-mediated signaling promotes macropinocytic transcytosis and opens a paracellular path via the re-modeling of tight junctions (3), thus facilitating invasion of the central nervous system by C. neoformans (4). Created with Biorender.com.
Mechanisms facilitating Cn across the blood-brain barrier
Although observations of fungal cells in the CNS have been reported in real-time and at autopsy, the molecular interactions at the brain endothelium or the pathways that mediate the movement of fungal cells into the CNS do not yet form a coherent picture. Early studies using an in vitro BBB model system have shown that when Cn contacts the brain endothelium, the endothelial cells form membrane ruffles and cup-like F-actin structures (Chen et al., 2003; Chang et al., 2004). The surface changes are indicative of membrane and cytoskeleton re-modeling – alterations required for Cn to breach the BBB (Chen et al., 2003). Cytoskeletal rearrangements may eventually lead to altered permeability of the BBB via tight junction disruption, and Cn internalization itself may cause an upregulation in endocytic vesicle formation. Several studies have confirmed that the translocation of Cn from blood to the brain begins with brain microcapillary sequestration and involves a coordination of several virulence factors including phospholipase B1 (PLB1) (Maruvada et al., 2012), a metalloprotease (Mpr1) (Vu et al., 2014), urease (Olszewski et al., 2004), laccase (Qiu et al., 2012), a serine protease (Xu et al., 2014) and the host receptor, CD44 (Jong et al., 2008b; Jong et al., 2012),
CD44
CD44 is critical for Cn adherence and invasion through an actin-dependent process of brain endothelial cells (Chang et al., 2006; Jong et al., 2007b; Jong et al., 2008a; Jong et al., 2008b; Jong et al., 2012) (Figure 1). CD44 functions as a receptor and anchor for hyaluronic acid (HA), a macromolecule that serves many roles, including as a component of the extracellular matrix of blood vessels and the capsule of Cn (Chen et al., 2021). Cn adheres to CD44 via hyaluronic acid on lipid rafts immediately prior to internalization (Huang et al., 2011). Perhaps analogously, lipid rafts form on brain endothelial cells prior to endocytosis of neuroinvasive Escherichia coli (Loh et al., 2017). The variant of CD44 expressed on brain endothelial cells appears to be the standard variant (Jong et al., 2008b), and therefore, Cn neurotropism cannot be a consequence of selective binding to a particular CD44 isoform expressed in brain microvasculature. CD44 is also not upregulated upon Cn exposure (Jong et al., 2008b). Nevertheless, acquisition of inositol, an abundant sugar in the brain, by Cn increases the expression of hyaluronic acid synthase (CPS1) and leads to greater production of HA which would promote adherence and stimulate internalization (Liu et al., 2013). Moreover, without hyaluronic acid synthase (CPS1), Cn neither produces a capsule nor invades the CNS (Chang et al., 2006; Jong et al., 2007).
The Ephrin receptor tyrosine kinase, EphA2
Although hyaluronic-acid-CD44 interaction plays an early role in Cn invasion, there are other factors and/or processes necessary for Cn endocytosis (Maruvada et al., 2012; Vu et al., 2014; Na Pombejra et al., 2017). Transcriptomic analysis of brain endothelial cells exposed to Cn revealed increased expression of ephrinA1 (EFNA1), the ligand for the receptor tyrosine kinase EphA2 (Aaron et al., 2018). EphA2 belongs to the Eph A class of the Ephrin family of receptor tyrosine kinases (RTK) and along with their ligands (Ephrins) they make up the largest RTK subfamily (Himanen and Nikolov, 2003). Upregulation of ephrinA1 expression in endothelial cells has also been reported in response to inflammation (Funk et al., 2012), serum depletion (Wiedemann et al., 2017) ischemia (Chen et al., 2018a) and increased cell density (Wiedemann et al., 2017). The EphrinA1 ligand is membrane-tethered by a GPI linkage that subsequently binds to and activates the Eph A class of receptors (i.e., EphA2). Once activated, EphA2 receptors oligomerize and become autophosphorylated at juxtamembrane tyrosine residues.
In the case of Cn, silencing the EphA2 transcript in brain endothelial cells or inhibiting EphA2 activity with an antibody or an inhibitor (dasatinib) prevents Cn from crossing the brain endothelium in an in vitro model of the BBB, whereas treatment with recombinant ephrin A1 or an agonist enhances Cn crossing (Aaron et al., 2018). The inability of the CPS1 deletion strain to induce the phosphorylation of EphA2 or to cross the BBB upon stimulating EphA2 with ephrinA1 supports the involvement of CD44 in the internalization Cn and suggests a connection between these host-expressed transmembrane proteins in the context of Cn invasion (Aaron et al., 2018). Interestingly, Kaposi’s sarcoma-associated herpesvirus (Hahn et al., 2012), Chlamydia trachomatis (Subbarayal et al., 2015), Plasmodium falciparum (Kaushansky et al., 2015; Darling et al., 2020), Helicobacter pylori (Leite et al., 2020), Epstein-Barr virus (Chen et al., 2018b) and uropathogens (Prakash et al., 2022) also enter host cells via EphA2.
Why or how Cn engages EphA2 is still under investigation, however the role of EphA2 in cytoskeleton remodeling (Pitulescu and Adams, 2010), in triggering signaling cascades of macropinocytosis (Hahn et al., 2012; Bandyopadhyay et al., 2014), and its influence on the integrity of the brain endothelium (Tanaka et al., 2005; Fang et al., 2008; Zhou et al., 2011) would suggest that Cn may be exploiting EphA2 for invasion of the CNS (Figure 1). Consistent with this notion, host factors (PI3K, MAPK, Src kinases, Rac1 and Rho- GTPases) activated by Cn to induce transcytosis across the BBB are known to be triggered by EphA2 for endocytosis (Jong et al., 2008a; Kim et al., 2012; Maruvada et al., 2012).
Macropinocytosis
Macropinocytosis is a form of endocytosis defined by large endocytic vesicles and non-specificity (Salloum et al., 2023). Macropinosomes form from membrane protrusions supported by polymerizing actin; these protrusions extend then collapse inward, entrapping an extracellular volume within macropinosomes of 0.5-10 µm in diameter (Lin et al., 2020). Macropinocytosis is “macro” in the sense that the macropinosomes can be easily observed with traditional light microscopy, and exploited for cellular uptake by bacteria, larger pathogens, and protein aggregates, in contrast to other forms of pinocytosis (Mercer and Helenius, 2009; Bloomfield and Kay, 2016; Yerbury, 2016). Although the triggers leading to macropinocytosis are extremely diverse, there is some commonality in their dependence on Rac1 (Grimmer et al., 2002; Salloum et al., 2023). Macropinocytosis is common throughout Eukarya (Lin et al., 2020), including many human cell types. This form of endocytosis is particularly pronounced and well-studied in immune cells, with an extreme example being macrophages, which constantly sample the extracellular environment via this process (Lin et al., 2020). In endothelial cells, macropinocytosis occurs at a low background level but can be elevated in the presence of external stimuli, such as growth factors (Lin et al., 2020). By this process, endothelial cells take up materials from blood, including extracellular vesicles (Ajikumar et al., 2019) and platelets (Faille et al., 2012).
Evidence for macropinocytosis of Cn by brain endothelial cells
Huang et al., (Huang et al., 2011) concluded that “C. neoformans may utilize the endocytic signaling pathway in [brain endothelial cells] to traverse the blood-brain barrier,” proposing that macropinocytosis was one of the potential types of endocytosis utilized by Cn but did not come to a final conclusion. Brain endothelia has been shown to engage macropinocytosis when exposed to viruses, bacteria, platelets and microvesicles (Liu et al., 2002; Faille et al., 2012; Loh et al., 2017; Ajikumar et al., 2019; Espinal et al., 2022). Evidence for Cn transcellular invasion of human brain endothelial cells beginning with internalization by macropinocytosis includes the dramatic plasma membrane rearrangement observed upon contact with Cn, including the formation of membrane projections that engulf fungal cells (Vu et al., 2013; Aaron et al., 2018). These rearrangements result from Cn inducing actin polymerization (profilin upregulation, cofilin downregulation) upon contact (Chen et al., 2003; Maruvada et al., 2012). Although this observation alone could also point to phagocytosis, the lack of identification of a specific receptor after decades of research is consistent with a nonspecific process. In other words, macropinocytosis represents a null hypothesis for Cn entry – if increasingly exhaustive screens fail to identify a ligand indicative of phagocytosis, the conclusion ought to default to Cn entry into brain endothelial cells relying on macropinocytosis.
There are other positive indications of macropinocytosis for Cn entry. Rac 1, commonly implicated in macropinocytosis of extremely diverse extracellular entities, is targeted by Cryptococcus (Maruvada et al., 2012). Other pathogens such as E. coli K1 (Loh et al., 2017) have also been shown to invade human brain endothelial cells in a Rac1-dependent macropinocytic pathway. Engulfment of Cn by brain endothelial cells is not a dead end for the pathogen as Cn can survive intracellularly in vivo (Davis et al., 2016; Chen et al., 2021). Heat killed Cn can adhere to the brain endothelium, but must be viable in order to be internalized and cross, implying that the process leading to adherence and internalization are separate, unlike what would be expected from an entirely receptor-mediated process (Shi et al., 2010; Chen et al., 2021). Disruption of actin filaments by cytochalasin D prevents Cn invasion of brain endothelial cells, demonstrating that entry is facilitated in an actin-dependent manner (Jong et al., 2008b).
How macropinocytosis might be conclusively demonstrated for Cn in brain endothelial cells
Because macropinocytosis combines several features associated with both phagocytosis and pinocytosis, macropinocytosis can be difficult to establish experimentally. Endocytic vesicle size and membrane ruffling distinguish macropinocytosis from other forms of pinocytosis (Mercer and Helenius, 2009; Lin et al., 2020). High-resolution microscopy, preferably with actin staining to visualize the support structures beneath potential macropinosomes, is necessary to make this determination. However, protruding actin-supported membrane ruffles can superficially resemble phagocytosis of particles, but macropinocytosis and phagocytosis differ in that macropinocytosis also entraps a large extracellular fluid volume (Mercer and Helenius, 2009; Bloomfield and Kay, 2016). This can also be identified by microscopy, with staining for extracellular solutes or the introduction of large molecular weight tracer molecules (Espinal et al., 2022), or via flowcytometry (Loh et al., 2017). Fluorescently labelled dextran uptake can even be used quantitatively to determine the rate of endocytosis (Wang et al., 2014). Because both macropinocytosis, with its characteristic non-specific internalization of extracellular solutes, and BBB disruption would be expected to lead to increased blood solutes in the brain parenchyma, the presence or absence of these solutes cannot alone be used to differentiate between the pathways. Therefore, the presence and subcellular localization of tight-junction proteins must also be determined. If tight junctions are intact in vivo as is suggested in vitro (Chen et al., 2003), then macropinocytosis is indicated over paracellular leakage.
Macropinocytosis is also dependent on a number of processes that can be chemically inhibited; inhibition of actin polymerization, Rac1/Cdc42; and Na/H+ exchange should all also lead to a decrease of internalization (Mercer and Helenius, 2009). The most critical set of experiments for determining involvement of macropinocytosis in Cn CNS invasion is chemical inhibition of BBB crossing by amiloride (or one of its derivatives), accompanied by a lack of inhibition when alternate pathways are specifically blocked. Amiloride and its derivatives block macropinocytosis by preventing the dissipation of low submembranous pH, which inhibits the activity of GTPase activity crucial for actin polymerization (Koivusalo et al., 2010). Cholesterol is also critical for Rac1 membrane localization leading to macropinocytosis in at least some cells (Grimmer et al., 2002). By contrast, specific inhibitors of other forms of endocytosis, such as clathrin- and caveolin-dependent processes, should have little effect on Cn internalization (Medrano-Gonzalez et al., 2021). However, the outcome of each of these tests may not be completely binary, as macropinocytosis is by definition non-specific, and many pathogens are known to invade host cells through other endocytic pathways in parallel with macropinocytosis (Bauherr et al., 2020).
Conclusions and future directions
As a pathogen, Cn is unusual in that its neurotropism drives its translocation from blood to brain despite the restricted passage imposed by the BBB; however, the reasons underlying the neurotropism are speculative in the absence of a BBB specific receptor/molecules. While EphA2 (and CD44) is not specific to brain endothelial cells, by engaging EphA2 Cn may be hedging its bets to avoid destruction in the bloodstream and instead exploit an EphA2-mediated binary pathway across the BBB into the CNS, where Cn can continue to thrive. This binary path involves both an EphA2-mediated macropinocytic transcellular pathway and a paracellular path via the re-modeling of the tight junctions by EphA2 (Figure 1). Additional work to investigate the molecular and cellular interplay between Cn, EphA2, its downstream signaling components and the NVU in mediating the translocation of Cn from blood-to-brain will be necessary to test this idea. The commonality among diverse pathogens to co-opt EphA2 in order to access the host is an emerging area of study that may yield a therapeutic intervention with broad spectrum activity.
Author contributions
DL: Conceptualization, Writing – original draft, Writing – review & editing. AB: Conceptualization, Writing – original draft, Writing – review & editing. KV: Conceptualization, Writing – original draft, Writing – review & editing. AG: Conceptualization, Funding acquisition, Supervision, Writing – original draft, Writing – review & editing.
Funding
The author(s) declare financial support was received for the research, authorship, and/or publication of this article. This work was supported with funding provided by the National Institutes of Health (NINDS) to AG; NIAID T32 training grant to DL; NINDS diversity supplement to AB.
Acknowledgments
The authors would like to acknowledge S. Menon for her contributions to Figure 1.
Conflict of interest
The authors declare that the research was conducted in the absence of any commercial or financial relationships that could be construed as a potential conflict of interest.
The author(s) declared that they were an editorial board member of Frontiers, at the time of submission. This had no impact on the peer review process and the final decision.
Publisher’s note
All claims expressed in this article are solely those of the authors and do not necessarily represent those of their affiliated organizations, or those of the publisher, the editors and the reviewers. Any product that may be evaluated in this article, or claim that may be made by its manufacturer, is not guaranteed or endorsed by the publisher.
References
Aaron, P. A., Jamklang, M., Uhrig, J. P., Gelli, A. (2018). The blood-brain barrier internalises Cryptococcus neoformans via the EphA2-tyrosine kinase receptor. Cell Microbiol. 20 (3), 138–149. doi: 10.1111/cmi.12811
Ajikumar, A., Long, M. B., Heath, P. R., Wharton, S. B., Ince, P. G., Ridger, V. C., et al. (2019). Neutrophil-derived microvesicle induced dysfunction of brain microvascular endothelial cells in vitro. Int. J. Mol. Sci. 20 (20). doi: 10.3390/ijms20205227
Bandyopadhyay, C., Valiya-Veettil, M., Dutta, D., Chakraborty, S., Chandran, B. (2014). CIB1 synergizes with EphrinA2 to regulate Kaposi’s sarcoma-associated herpesvirus macropinocytic entry in human microvascular dermal endothelial cells. PloS Pathog. 10 (2), e1003941. doi: 10.1371/journal.ppat.1003941
Bauherr, S., Larsberg, F., Petrich, A., Sperber, H. S., Klose-Grzelka, V., Luckner, M., et al. (2020). Macropinocytosis and clathrin-dependent endocytosis play pivotal roles for the infectious entry of puumala virus. J. Virol. 94 (14). doi: 10.1128/JVI.00184-20
Bloomfield, G., Kay, R. R. (2016). Uses and abuses of macropinocytosis. J. Cell Sci. 129 (14), 2697–2705. doi: 10.1242/jcs.176149
Bongomin, F., Gago, S., Oladele, R. O., Denning, D. W. (2017). Global and multi-national prevalence of fungal diseases-estimate precision. J. Fungi (Basel) 3 (4). doi: 10.3390/jof3040057
Chang, Y. C., Stins, M. F., McCaffery, M. J., Miller, G. F., Pare, D. R., Dam, T., et al. (2004). Cryptococcal yeast cells invade the central nervous system via transcellular penetration of the blood-brain barrier. Infect. Immun. 72 (9), 4985–4995. doi: 10.1128/iai.72.9.4985-4995.2004
Chang, Z. L., Netski, D., Thorkildson, P., Kozel, T. R. (2006). Binding and internalization of glucuronoxylomannan, the major capsular polysaccharide of by murine peritoneal macrophages. Infection Immun. 74 (1), 144–151. doi: 10.1128/Iai.74.1.144-151.2006
Charlier, C., Nielsen, K., Daou, S., Brigitte, M., Chretien, F., Dromer, F. (2009). Evidence of a role for monocytes in dissemination and brain invasion by Cryptococcus neoformans. Infect. Immun. 77 (1), 120–127. doi: 10.1128/iai.01065-08
Chen, Y., Li, C., Sun, D., Strickland, A. B., Liu, G., Shi, M. (2021). Quantitative analysis reveals internalisation of Cryptococcus neoformans by brain endothelial cells in vivo. Cell Microbiol. 23 (6), e13330. doi: 10.1111/cmi.13330
Chen, F., Liu, Z., Peng, W., Gao, Z., Ouyang, H., Yan, T., et al. (2018a). Activation of EphA4 induced by EphrinA1 exacerbates disruption of the blood-brain barrier following cerebral ischemia-reperfusion via the Rho/ROCK signaling pathway. Exp. Ther. Med. 16 (3), 2651–2658. doi: 10.3892/etm.2018.6460
Chen, J., Sathiyamoorthy, K., Zhang, X., Schaller, S., Perez White, B. E., Jardetzky, T. S., et al. (2018b). Ephrin receptor A2 is a functional entry receptor for Epstein-Barr virus. Nat. Microbiol. 3 (2), 172–180. doi: 10.1038/s41564-017-0081-7
Chen, Y., Shi, Z. W., Strickland, A. B., Shi, M. (2022). Cryptococcus neoformans infection in the central nervous system: the battle between host and pathogen. J. Fungi (Basel) 8 (10), 1–21. doi: 10.3390/jof8101069
Chen, S. H., Stins, M. F., Huang, S. H., Chen, Y. H., Kwon-Chung, K. J., Chang, Y., et al. (2003). Cryptococcus neoformans induces alterations in the cytoskeleton of human brain microvascular endothelial cells. J. Med. Microbiol. 52 (Pt 11), 961–970. doi: 10.1099/jmm.0.05230-0
Darling, T. K., Mimche, P. N., Bray, C., Umaru, B., Brady, L. M., Stone, C., et al. (2020). EphA2 contributes to disruption of the blood-brain barrier in cerebral malaria. PloS Pathog. 16 (1), e1008261. doi: 10.1371/journal.ppat.1008261
Davis, J. M., Huang, M. W., Botts, M. R., Hull, C. M., Huttenlocher, A. (2016). A zebrafish model of cryptococcal infection reveals roles for macrophages, endothelial cells, and neutrophils in the establishment and control of sustained fungemia. Infection Immun. 84 (10), 3047–3062. doi: 10.1128/Iai.00506-16
Ellis, D. H., Pfeiffer, T. J. (1990). Ecology, life cycle, and infectious propagule of Cryptococcus neoformans. Lancet 336 (8720), 923–925.
Espinal, E. R., Matthews, T., Holder, B. M., Bee, O. B., Humber, G. M., Brook, C. E., et al. (2022). Group B-induced macropinocytosis contributes to bacterial invasion of brain endothelial cells. Pathogens 11 (4). doi: 10.3390/pathogens11040474
Faille, D., El-Assaad, F., Mitchell, A. J., Alessi, M. C., Chimini, G., Fusai, T., et al. (2012). Endocytosis and intracellular processing of platelet microparticles by brain endothelial cells. J. Cell. Mol. Med. 16 (8), 1731–1738. doi: 10.1111/j.1582-4934.2011.01434.x
Fang, W. B., Ireton, R. C., Zhuang, G., Takahashi, T., Reynolds, A., Chen, J. (2008). Overexpression of EPHA2 receptor destabilizes adherens junctions via a RhoA-dependent mechanism. J. Cell Sci. 121 (Pt 3), 358–368. doi: 10.1242/jcs.017145
Funk, S. D., Yurdagul, A., Jr., Albert, P., Traylor, J. G., Jr., Jin, L., Chen, J., et al. (2012). EphA2 activation promotes the endothelial cell inflammatory response: a potential role in atherosclerosis. Arterioscler. Thromb. Vasc. Biol. 32 (3), 686–695. doi: 10.1161/ATVBAHA.111.242792
Gaylord, E. A., Choy, H. L., Doering, T. L. (2020). Dangerous liaisons: interactions of cryptococcus neoformans with host phagocytes. Pathogens 9 (11). doi: 10.3390/pathogens9110891
Gottfredsson, M., Perfect, J. R. (2000). Fungal meningitis. Semin. Neurol. 20 (3), 307–322. doi: 10.1055/s-2000-9394
Grimmer, S., van Deurs, B., Sandvig, K. (2002). Membrane ruffling and macropinocytosis in A431 cells require cholesterol. J. Cell Sci. 115 (14), 2953–2962. doi: 10.1242/jcs.115.14.2953
Hahn, A. S., Kaufmann, J. K., Wies, E., Naschberger, E., Panteleev-Ivlev, J., Schmidt, K., et al. (2012). The ephrin receptor tyrosine kinase A2 is a cellular receptor for Kaposi’s sarcoma-associated herpesvirus. Nat. Med. 18 (6), 961–966. doi: 10.1038/nm.2805
Himanen, J. P., Nikolov, D. B. (2003). Eph receptors and ephrins. Int. J. Biochem. Cell Biol. 35 (2), 130–134. doi: 10.1016/s1357-2725(02)00096-1
Huang, S. H., Long, M., Wu, C. H., Kwon-Chung, K. J., Chang, Y. C., Chi, F., et al. (2011). Invasion of into Human Brain Microvascular Endothelial Cells Is Mediated through the Lipid Rafts-Endocytic Pathway via the Dual Specificity Tyrosine Phosphorylation-regulated Kinase 3 (DYRK3). J. Biol. Chem. 286 (40), 34761–34769. doi: 10.1074/jbc.M111.219378
Ibrahim, A. S., Filler, S. G., Alcouloumre, M. S., Kozel, T. R., Edwards, J. E., Jr., Ghannoum, M. A. (1995). Adherence to and damage of endothelial cells by Cryptococcus neoformans in vitro: role of the capsule. Infect. Immun. 63 (11), 4368–4374. doi: 10.1128/iai.63.11.4368-4374.1995
Jong, A., Wu, C. H., Chen, H. M., Luo, F., Kwon-Chung, K. J., Chang, Y. C., et al. (2007). Identification and characterization of CPS1 as a hyaluronic acid synthase contributing to the pathogenesis of infection. Eukaryotic Cell 6 (8), 1486–1496. doi: 10.1128/Ec.00120-07
Jong, A., Wu, C. H., Gonzales-Gomez, I., Kwon-Chung, K. J., Chang, Y. C., Tseng, H. K., et al. (2012). Hyaluronic acid receptor CD44 deficiency is associated with decreased Cryptococcus neoformans brain infection. J. Biol. Chem. 287 (19), 15298–15306. doi: 10.1074/jbc.M112.353375
Jong, A., Wu, C. H., Prasadarao, N. V., Kwon-Chung, K. J., Chang, Y. C., Ouyang, Y., et al. (2008a). Invasion of Cryptococcus neoformans into human brain microvascular endothelial cells requires protein kinase C-alpha activation. Cell Microbiol. 10 (9), 1854–1865. doi: 10.1111/j.1462-5822.2008.01172.x
Jong, A., Wu, C. H., Shackleford, G. M., Kwon-Chung, K. J., Chang, Y. C., Chen, H. M., et al. (2008b). Involvement of human CD44 during Cryptococcus neoformans infection of brain microvascular endothelial cells. Cell Microbiol. 10 (6), 1313–1326. doi: 10.1111/j.1462-5822.2008.01128.x
Kaushansky, A., Douglass, A. N., Arang, N., Vigdorovich, V., Dambrauskas, N., Kain, H. S., et al. (2015). Malaria parasites target the hepatocyte receptor EphA2 for successful host infection. Science 350 (6264), 1089–1092. doi: 10.1126/science.aad3318
Keaney, J., Campbell, M. (2015). The dynamic blood-brain barrier. FEBS J. 282 (21), 4067–4079. doi: 10.1111/febs.13412
Kim, J. C., Crary, B., Chang, Y. C., Kwon-Chung, K. J., Kim, K. J. (2012). Cryptococcus neoformans activates RhoGTPase proteins followed by protein kinase C, focal adhesion kinase, and ezrin to promote traversal across the blood-brain barrier. J. Biol. Chem. 287 (43), 36147–36157. doi: 10.1074/jbc.M112.389676
Koivusalo, M., Welch, C., Hayashi, H., Scott, C. C., Kim, M., Alexander, T., et al. (2010). Amiloride inhibits macropinocytosis by lowering submembranous pH and preventing Rac1 and Cdc42 signaling. J. Cell Biol. 188 (4), 547–563. doi: 10.1083/jcb.200908086
Kozel, T. R., Gotschlich, E. C. (1982). The capsule of cryptococcus neoformans passively inhibits phagocytosis of the yeast by macrophages. J. Immunol. 129 (4), 1675–1680. doi: 10.4049/jimmunol.129.4.1675
Kwon-Chung, K. J., Sorrell, T. C., Dromer, F., Fung, E., Levitz, S. M. (2000). Cryptococcosis: clinical and biological aspects. Med. Mycol 38 Suppl 1, 205–213. doi: 10.1080/mmy.38.s1.205.213
Leite, M., Marques, M. S., Melo, J., Pinto, M. T., Cavadas, B., Aroso, M., et al. (2020). Helicobacter pylori targets the EPHA2 receptor tyrosine kinase in gastric cells modulating key cellular functions. Cells 9 (2). doi: 10.3390/cells9020513
Lin, X. P., Mintern, J. D., Gleeson, P. A. (2020). Macropinocytosis in different cell types: similarities and differences. Membranes 10 (8). doi: 10.3390/membranes10080177
Liu, T. B., Kim, J. C., Wang, Y., Toffaletti, D. L., Eugenin, E., Perfect, J. R., et al. (2013). Brain inositol is a novel stimulator for promoting Cryptococcus penetration of the blood-brain barrier. PloS Pathog. 9 (4), e1003247. doi: 10.1371/journal.ppat.1003247
Liu, N. Q., Lossinsky, A. S., Popik, W., Li, X., Gujuluva, C., Kriederman, B., et al. (2002). Human immunodeficiency virus type 1 enters brain microvascular endothelia by macropinocytosis dependent on lipid rafts and the mitogen-activated protein kinase signaling pathway. J. Virol. 76 (13), 6689–6700. doi: 10.1128/Jvi.76.13.6689-6700.2002
Loh, L. N., McCarthy, E. M. C., Narang, P., Khan, N. A., Ward, T. H. (2017). K1 utilizes host macropinocytic pathways for invasion of brain microvascular endothelial cells. Traffic 18 (11), 733–746. doi: 10.1111/tra.12508
Loyse, A., Dromer, F., Day, J., Lortholary, O., Harrison, T. S. (2013a). Flucytosine and cryptococcosis: time to urgently address the worldwide accessibility of a 50-year-old antifungal. J. Antimicrob. Chemother. 68 (11), 2435–2444. doi: 10.1093/jac/dkt221
Loyse, A., Thangaraj, H., Easterbrook, P., Ford, N., Roy, M., Chiller, T., et al. (2013b). Cryptococcal meningitis: improving access to essential antifungal medicines in resource-poor countries. Lancet Infect. Dis. 13 (7), 629–637. doi: 10.1016/S1473-3099(13)70078-1
Maruvada, R., Zhu, L., Pearce, D., Zheng, Y., Perfect, J., Kwon-Chung, K. J., et al. (2012). Cryptococcus neoformans phospholipase B1 activates host cell Rac1 for traversal across the blood-brain barrier. Cell Microbiol. 14 (10), 1544–1553. doi: 10.1111/j.1462-5822.2012.01819.x
Medrano-Gonzalez, P. A., Rivera-Ramirez, O., Montano, L. F., Rendon-Huerta, E. P. (2021). Proteolytic processing of CD44 and its implications in cancer. Stem Cells Int. 2021. doi: 10.1155/2021/6667735
Mercer, J., Helenius, A. (2009). Virus entry by macropinocytosis. Nat. Cell Biol. 11 (5), 510–520. doi: 10.1038/ncb0509-510
Mitchell, T. G., Perfect, J. R. (1995). Cryptococcosis in the era of AIDS–100 years after the discovery of Cryptococcus neoformans. Clin. Microbiol. Rev. 8 (4), 515–548. doi: 10.1128/CMR.8.4.515
Muzazu, S. G. Y., Assefa, D. G., Phiri, C., Getinet, T., Solomon, S., Yismaw, G., et al. (2022). Prevalence of cryptococcal meningitis among people living with human immuno-deficiency virus and predictors of mortality in adults on induction therapy in Africa: A systematic review and meta-analysis. Front. Med. (Lausanne) 9. doi: 10.3389/fmed.2022.989265
Na Pombejra, S., Salemi, M., Phinney, B. S., Gelli, A. (2017). The metalloprotease, mpr1, engages annexinA2 to promote the transcytosis of fungal cells across the blood-brain barrier. Front. Cell Infect. Microbiol. 7. doi: 10.3389/fcimb.2017.00296
Olszewski, M. A., Noverr, M. C., Chen, G. H., Toews, G. B., Cox, G. M., Perfect, J. R., et al. (2004). Urease expression by Cryptococcus neoformans promotes microvascular sequestration, thereby enhancing central nervous system invasion. Am. J. Pathol. 164 (5), 1761–1771. doi: 10.1016/s0002-9440(10)63734-0
Park, B. J., Wannemuehler, K. A., Marston, B. J., Govender, N., Pappas, P. G., Chiller, T. A. (2009). Estimation of the current global burden of cryptococcal meningitis among persons living with HIV/AIDS. Aids 23 (4), 525–530. doi: 10.1097/QAD.0b013e328322ffac
Perfect, J. R., Dismukes, W. E., Dromer, F., Goldman, D. L., Graybill, J. R., Hamill, R. J., et al. (2010). Clinical practice guidelines for the management of cryptococcal disease: 2010 update by the infectious diseases society of america. Clin. Infect. Dis. 50 (3), 291–322. doi: 10.1086/649858
Pitulescu, M. E., Adams, R. H. (2010). Eph/ephrin molecules–a hub for signaling and endocytosis. Genes Dev. 24 (22), 2480–2492. doi: 10.1101/gad.1973910
Prakash, P. S., Kruse, A., Vogel, C., Schagdarsurengin, U., Wagenlehner, F. (2022). Targeting host tyrosine kinase receptor EPHA2 signaling affects uropathogen infection in human bladder epithelial cells. Pathogens 11 (10). doi: 10.3390/pathogens11101176
Pyrgos, V., Seitz, A. E., Steiner, C. A., Prevots, D. R., Williamson, P. R. (2013). Epidemiology of cryptococcal meningitis in the US: 1997-2009. PloS One 8 (2), e56269. doi: 10.1371/journal.pone.0056269
Qiu, Y., Davis, M. J., Dayrit, J. K., Hadd, Z., Meister, D. L., Osterholzer, J. J., et al. (2012). Immune modulation mediated by cryptococcal laccase promotes pulmonary growth and brain dissemination of virulent Cryptococcus neoformans in mice. PloS One 7 (10), e47853. doi: 10.1371/journal.pone.0047853
Rajasingham, R., Govender, N. P., Jordan, A., Loyse, A., Shroufi, A., Denning, D. W., et al. (2022). The global burden of HIV-associated cryptococcal infection in adults in 2020: a modelling analysis. Lancet Infect. Dis. 22 (12), 1748–1755. doi: 10.1016/S1473-3099(22)00499-6
Rajasingham, R., Smith, R. M., Park, B. J., Jarvis, J. N., Govender, N. P., Chiller, T. M., et al. (2017). Global burden of disease of HIV-associated cryptococcal meningitis: an updated analysis. Lancet Infect. Dis. 17 (8), 873–881. doi: 10.1016/S1473-3099(17)30243-8
Salloum, G., Bresnick, A. R., Backer, J. M. (2023). Macropinocytosis: mechanisms and regulation. Biochem. J. 480 (5), 335–362. doi: 10.1042/BCJ20210584
Santiago-Tirado, F. H., Onken, M. D., Cooper, J. A., Klein, R. S., Doering, T. L. (2017). Trojan horse transit contributes to blood-brain barrier crossing of a eukaryotic pathogen. MBio 8 (1). doi: 10.1128/mBio.02183-16
Shi, M., Li, S. S., Zheng, C., Jones, G. J., Kim, K. S., Zhou, H., et al. (2010). Real-time imaging of trapping and urease-dependent transmigration of Cryptococcus neoformans in mouse brain. J. Clin. Invest. 120 (5), 1683–1693. doi: 10.1172/jci41963
Sorrell, T. C., Juillard, P. G., Djordjevic, J. T., Kaufman-Francis, K., Dietmann, A., Milonig, A., et al. (2016). Cryptococcal transmigration across a model brain blood-barrier: evidence of the Trojan horse mechanism and differences between Cryptococcus neoformans var. grubii strain H99 and Cryptococcus gattii strain R265. Microbes Infect. 18 (1), 57–67. doi: 10.1016/j.micinf.2015.08.017
Subbarayal, P., Karunakaran, K., Winkler, A. C., Rother, M., Gonzalez, E., Meyer, T. F., et al. (2015). EphrinA2 receptor (EphA2) is an invasion and intracellular signaling receptor for chlamydia trachomatis. PloS Pathog. 11 (4). doi: 10.1371/journal.ppat.1004846
Tanaka, M., Kamata, R., Sakai, R. (2005). EphA2 phosphorylates the cytoplasmic tail of Claudin-4 and mediates paracellular permeability. J. Biol. Chem. 280 (51), 42375–42382. doi: 10.1074/jbc.M503786200
Traino, K., Snow, J., Ham, L., Summers, A., Segala, L., Shirazi, T., et al. (2019). HIV-negative cryptococcal meningoencephalitis results in a persistent frontal-subcortical syndrome. Sci. Rep. 9 (1), 18442. doi: 10.1038/s41598-019-54876-7
Velagapudi, R., Hsueh, Y. P., Geunes-Boyer, S., Wright, J. R., Heitman, J. (2009). Spores as infectious propagules of Cryptococcus neoformans. Infect. Immun. 77 (10), 4345–4355. doi: 10.1128/iai.00542-09
Vu, K., Eigenheer, R. A., Phinney, B. S., Gelli, A. (2013). Cryptococcus neoformans Promotes Its Transmigration into the Central Nervous System by Inducing Molecular and Cellular Changes in Brain Endothelial Cells. Infection Immun. 81 (9), 3139–3147. doi: 10.1128/Iai.00554-13
Vu, K., Tham, R., Uhrig, J. P., Thompson, G. R., Pombejra, S. N., Jamklang, M., et al. (2014). Invasion of the central nervous system by cryptococcus neoformans requires a secreted fungal metalloprotease. Mbio 5 (3). doi: 10.1128/mBio.01101-14
Vu, K., Weksler, B., Romero, I., Couraud, P. O., Gelli, A. (2009). Immortalized human brain endothelial cell line HCMEC/D3 as a model of the blood-brain barrier facilitates in vitro studies of central nervous system infection by Cryptococcus neoformans. Eukaryot Cell 8 (11), 1803–1807. doi: 10.1128/ec.00240-09
Walsh, N. M., Botts, M. R., McDermott, A. J., Ortiz, S. C., Wuthrich, M., Klein, B., et al. (2019). Infectious particle identity determines dissemination and disease outcome for the inhaled human fungal pathogen Cryptococcus. PloS Pathog. 15 (6), e1007777. doi: 10.1371/journal.ppat.1007777
Wang, J. T. H., Teasdale, R. D., Liebl, D. (2014). Macropinosome quantitation assay. Methodsx 1, 36–41. doi: 10.1016/j.mex.2014.05.002
Weiss, N., Miller, F., Cazaubon, S., Couraud, P. O. (2009). The blood-brain barrier in brain homeostasis and neurological diseases. Biochim. Biophys. Acta 1788 (4), 842–857. doi: 10.1016/j.bbamem.2008.10.022
Wiedemann, E., Jellinghaus, S., Ende, G., Augstein, A., Sczech, R., Wielockx, B., et al. (2017). Regulation of endothelial migration and proliferation by ephrin-A1. Cell Signal 29, 84–95. doi: 10.1016/j.cellsig.2016.10.003
Williamson, P. R., Jarvis, J. N., Panackal, A. A., Fisher, M. C., Molloy, S. F., Loyse, A., et al. (2017). Cryptococcal meningitis: epidemiology, immunology, diagnosis and therapy. Nat. Rev. Neurol. 13 (1), 13–24. doi: 10.1038/nrneurol.2016.167
Xu, C. Y., Zhu, H. M., Wu, J. H., Wen, H., Liu, C. J. (2014). Increased permeability of blood-brain barrier is mediated by serine protease during Cryptococcus meningitis. J. Int. Med. Res. 42 (1), 85–92. doi: 10.1177/0300060513504365
Yerbury, J. J. (2016). Protein aggregates stimulate macropinocytosis facilitating their propagation. Prion 10 (2), 119–126. doi: 10.1080/19336896.2016.1141860
Zheng, W. (2001). Neurotoxicology of the brain barrier system: new implications. J. Toxicol. Clin. Toxicol. 39 (7), 711–719. doi: 10.1081/clt-100108512
Keywords: macropinocytosis, EphA2, Ephrin receptor, EphrinA1, CD44, transcytosis, blood-brain barrier, brain endothelial cells
Citation: Lanser DM, Bennett AB, Vu K and Gelli A (2023) Macropinocytosis as a potential mechanism driving neurotropism of Cryptococcus neoformans. Front. Cell. Infect. Microbiol. 13:1331429. doi: 10.3389/fcimb.2023.1331429
Received: 01 November 2023; Accepted: 27 November 2023;
Published: 11 December 2023.
Edited by:
Andrew Alspaugh, Duke University, United StatesReviewed by:
Felipe H. Santiago-Tirado, University of Notre Dame, United StatesMeiqing Shi, University of Maryland, College Park, United States
Copyright © 2023 Lanser, Bennett, Vu and Gelli. This is an open-access article distributed under the terms of the Creative Commons Attribution License (CC BY). The use, distribution or reproduction in other forums is permitted, provided the original author(s) and the copyright owner(s) are credited and that the original publication in this journal is cited, in accordance with accepted academic practice. No use, distribution or reproduction is permitted which does not comply with these terms.
*Correspondence: Angie Gelli, YWNnZWxsaUB1Y2RhdmlzLmVkdQ==
†These authors have contributed equally to this work