- 1Institute of Biotechnology, Federal University of Uberlandia, Uberlandia, Brazil
- 2School of Veterinary Medicine, Federal University of Uberlandia, Uberlandia, Brazil
- 3Institute of Biomedical Sciences, Federal University of Uberlandia, Uberlandia, Brazil
Lately, the bacterial multidrug resistance has been a reason to public health concerning around world. The development of new pharmacology therapies against infections caused by multidrug-resistant bacteria is urgent. In this work, we developed 10 NLC formulations composed of essential oils (EO), vegetable butter and surfactant. The formulations were evaluated for long-term and thermal cycling stability studies in terms of (particle size, polydispersion index and Zeta potential). In vitro antimicrobial assays were performed using disk diffusion test and by the determination of the minimum inhibitory concentration (MIC) performed with fresh and a year-old NLC. The most promising system and its excipients were structurally characterized through experimental methodologies (FTIR-ATR, DSC and FE-SEM). Finally, this same formulation was studied through nanotoxicity assays on the chicken embryo model, analyzing different parameters, as viability and weight changes of embryos and annexes. All the developed formulations presented long-term physicochemical and thermal stability. The formulation based on cinnamon EO presented in vitro activity against strains of Acinetobacter baumannii, Klebsiella pneumoniae and Pseudomonas aeruginosa isolated from humans and in vivo biocompatibility. Considering these promising results, such system is able to be further tested on in vivo efficacy assays.
1 Introduction
The emergence of multi-resistant bacteria (MDR) to antibiotics represents one of the principal global threats to public health (Murray et al., 2022). The clinicians have been considered the use of the last therapeutics options, such as vancomycin, polymyxin B, carbapenems and colistin, with low rates of success (Wang et al., 2022; Yao et al., 2023). The infections by MDR are currently associated with considerable increase in morbidity and mortality of intensive care units (Braga et al., 2018), increasing hospital costs (Osme et al., 2020) and resistance against the strategies used to combating them, as antibiotics association (Batchelder et al., 2023). There is an estimative that by 2050 will there will be 10 million deaths from MDR, annually (O’Neill, 2016).
In addition, in the COVID-19 pandemic, there was an indiscriminate use of broad-spectrum antibiotics (Freire et al., 2023), increasing the antimicrobial resistance in worldwide (Kariyawasam et al., 2022), as reported for bacterial resistance to carbapenems (Pan American Health Organization, 2021).
In this sense, the use of compounds of natural origin, such as essential oils (EO) can represent an interesting alternative to combat infections by MDR pathogens. EO are promising drug candidates, once they have several intrinsic biological properties, such as antibacterial, antifungal, antiviral, antioxidant, anticancer, analgesic, anti-inflammatory, biopesticide, repellent, among others (Elshafie and Camele, 2017; Cimino et al., 2021). These compounds come from the secondary metabolism of plants, being extracted from bark, leaves, flowers, buds, seeds, roots and fruits. Its chemical constitution is complex, being mainly composed of monoterpenes, sesquiterpenes and terpenoids (El Asbahani et al., 2015). However, the application of EO in the clinical approaches is limited, due to its low solubility in water, low physicochemical stability, high volatility and hydrolysis (Carbone et al., 2018; Guidotti-Takeuchi et al., 2022). Moreover, the toxicity of the esophageal and gastrointestinal mucosa limits its use by enteral administration route, as well as allergic reactions can be observed in the skin if they are insufficiently diluted (Lammari et al., 2021).
Thus, the EO nanoencapsulation can be an interesting alternative to overcome such limitations. The lipid nanoparticles, including the nanostructured lipid carriers (NLC), which is composed by solid and liquid lipids at room temperature (Ribeiro et al., 2021), seems to be an excellent strategy. NLC are biodegradable, biocompatible, scalable and ecofriendly (Dupuis et al., 2022). Such systems allow the encapsulation of hydrophobic molecules as EO, improving its physicochemical stability (Cimino et al., 2021), protecting against degradation and evaporation. This process still increases the EO solubility in water, optimizing its bioavailability, allowing its sustained release (Katopodi and Detsi, 2021) and, consequently, decrease the EO intrinsic toxicity (Dajic Stevanovic et al., 2020).
This work reports the development of NLC formulations based on natural matrices composed of vegetable butter and EO with activity against strains of Acinetobacter baumannii, Klebsiella pneumoniae and Pseudomonas aeruginosa isolated from humans. These bacterial species were selected because they are drug multi-resistant, including the resistance to antibiotics considered the last therapeutic choice. Consequently, they are the most prevalent species of the most of infections described in clinics, increasing hospital costs and mortality rates (Tacconelli et al., 2018; Osme et al., 2020; World Health Organization, 2021; Murray et al., 2022; Wang et al., 2022; Micheli et al., 2023; Yao et al., 2023). Firstly, a screening of 14 natural oils was carried out to select those with the most promising in vitro antibacterial activity as the structural and bioactive excipient in the preparation of different NLC formulations. The resultant formulations were monitored by a long-term physicochemical stability study (1 year, 25°C), followed by thermal cycling stability test. Then, the in vitro antibacterial activity was evaluated through the determination of the minimum inhibitory concentration (MIC). Finally, the structural characterization and the in vivo nanotoxicity test on chicken embryo model (CE) were performed to determinate compatibility between the excipients, thermal stability and the safety of the most promising nanosystems.
2 Materials and methods
2.1 Materials
Ucuuba butter, cinnamon bark EO (Cynnamomum zeylanicum), oregano EO (Origanum vulgare), tea tree EO (Melaleuca artenifolia), peppermint EO (Mentha pipperita), rosemary EO (Rosmarinus officinalis), clove EO (Eugenia caryophyllus), ginger EO (Zingiber officinale), salvia EO (Salvia sclarea), basil EO (Ocimum basilicum), citronella EO (Cymbopogon nardus), star anise EO (Illicium verum), frankincense EO (Boswellia carterii), candeia (Eremanthus erythropappus) and copaiba (Copaifera officinalis) vegetable oils were commercially obtained from the company Engetec/Engenharia das Essências®, Brazil. Poloxamer (Kolliphor® P188), used as a surfactant, was purchased from Sigma-Aldrich, USA. Deionized water (18 MΩ) was obtained from a Milli-Q® Direct 8 ultra-pure water purifier. Bacterial isolates of A. baumannii (65JF, OXA-23 and POA4-28), K. pneumoniae (ATCC700603, Responsive and Bactray) and P. aeruginosa (IMP-1 and PAO-1) were obtained from the collection of the Molecular Microbiology Laboratory of the Federal University of Uberlandia, Brazil. Klebsiella pneumoniae ATCC700603 is a standard strain. Responsive K. pneumoniae was isolated from a hospitalized patient in Uberlandia – MG that we used as a secondary control for sensitivity to antibiotics. All the other strains were isolated from hospitalized patients in Brazil and characterized as MDR, once they came from an acquired resistant infection not susceptible to at least 1 agent in 3 or more antimicrobial categories (Magiorakos et al., 2012). The medium culture used were: Soy Triptone Agar (Isofar, Brazil), MacConkey Agar (Ion, India), Mueller Hinton Agar (Laboratorios Conda S.A., Spain), Mueller Hinton Broth (Biolog®). Disks impregnated with Polymyxin B were purchased from Cefar Diagnóstica Ltda, São Paulo, Brazil.
2.2 Screening of vegetable and essential oils
The screening of different vegetable and EO was carried out through the disk diffusion test against A. baumannii, K. pneumoniae and P. aeruginosa strains. Commercial polymyxin B disks were used as a positive control. The strains of interest were inoculated in Soy Triptone agar (TSA) or MacConkey agar medium culture, separately, and incubated at 37°C for 24 h. After this period, isolated colonies of 3 to 5 species of the same morphological type were collected and diluted in 10 mL of 0.9% sterile saline solution, with turbidity adjusted according 0.5 MacFarland scale, up to a final concentration of colony forming units 1.5 x 108 (CFU) mL-1 (Ribeiro et al., 2021). The resulting bacterial suspension was used in the disk diffusion test (pure EO, screening stage) and in the MIC determination assay. Plates containing Mueller Hinton agar were previously prepared. With a sterile swab, each bacterial suspension analyzed (A. baumannii, P. aeruginosa and K. pneumoniae) was equally distributed over the agar surface and stored by 5 min (25°C). After this period, disks impregnated with polymyxin B (positive control) and EO were positioned over the agar, in duplicate. Approximately 20 μL of each pure oil was added to sterile filter disks (6 mm in diameter) immediately before being positioned on the plates. The plates were incubated at 37 ± 1 °C for 24 h (Ribeiro et al., 2020). Finally, the diameters growth inhibition zones (mm) were registered using a millimeter scale. One-way ANOVA/Tukey post hoc statistical tests were used to evaluate intergroup statistical differences (p< 0.05), determined by Microsoft Excel Professional Plus 2019 and Action version 2.9 software.
2.3 Preparation of nanostructured lipid carriers
Different NLC formulations were prepared by the hot ultrasonication-emulsification method. The lipid phase of NLC was composed of ucuuba butter (solid lipid) and EO (liquid lipid) with a total lipid concentration of 10% (w/v) at a ratio of liquid lipids to total lipids of 0.1:1. So, the final EO concentration in NLC was 10 mg/mL, corresponding to 10% of the total lipid matrix of NLC. Briefly, in a water bath, the lipid phase was heated 10°C above the melting point of the solid lipid (ucuuba butter). Simultaneously, the aqueous phase containing 5% poloxamer was heated to the same temperature as the lipid phase. Then, the aqueous phase was added dropwise to the lipid phase, under stirring at 10,000 rpm for 2 min, in an Ultra-Turrax high pressure homogenizer (Ultra-Turrax® T18). The obtained microemulsion was immediately ultrasonicated for 20 min in a Vibracell tip sonicator (Sonics & Mat. Inc., Danbury, USA) operated at 500 W and 20 kHz, with alternating cycles (on/off) at 30 s. After this step, the formed nanoemulsion was immediately cooled to room temperature (25° C) in an ice bath to solidify the nanoparticles (Ribeiro et al., 2021). For control experiments, solid lipid nanoparticles (without EO addition) were prepared following the same procedure. For didactic purposes, this system was called NLC control in this work (Table 1).
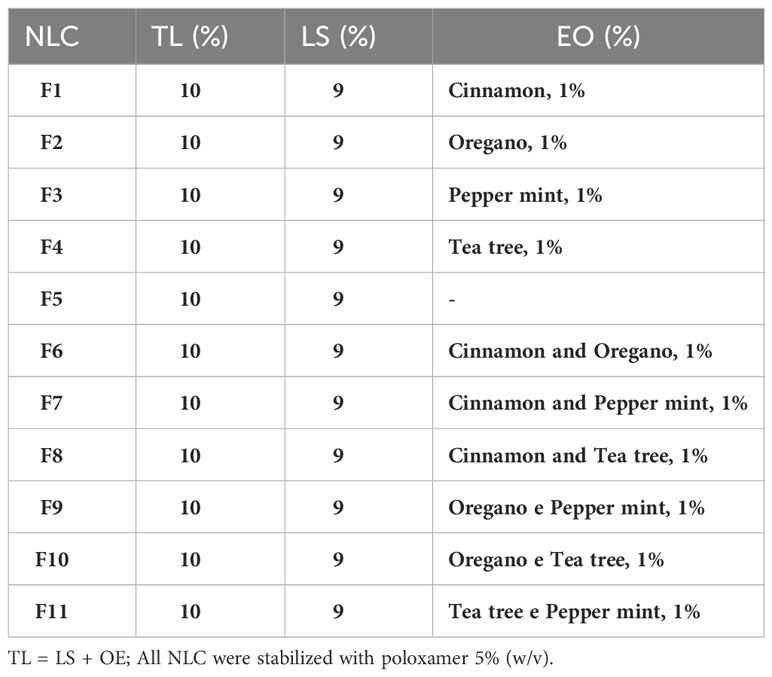
Table 1 Composition and concentration of total lipids (TL), ucuuba butter (LS), essential oils (EO) and surfactant used in each NLC formulation.
2.4 Long-term physicochemical stability study
The nanoparticle size (nm), polydispersion index (PDI) and Zeta potential (mV) were analyzed by diluting the NLC formulations (1:1000 v/v) in deionized water through the dynamic light scattering (DLS) technique by Litesizer equipment (Anton Paar Instruments, Anton Paar, Berlin, Germany). The same parameters were monitored for 12 months at 25°C, in triplicate (Ribeiro et al., 2017). One-way ANOVA/Tukey post hoc statistical tests were used to evaluate intragroup statistical differences over time (p< 0.05), calculated by GraphPad Prism 8 software.
2.5 Evaluation of in vitro antibacterial activity
All prepared NLC were evaluated by microdilution method to determine the MIC. This experiment was performed in 96-well plates, in triplicate. Different strains of A. baumannii, P. aeruginosa and K. pneumoniae isolated from humans were analyzed. Bacterial suspensions were prepared as previously detailed in the screening step. They were diluted in 96-well plate to a final concentration per well of 1 × 106 CFU·mL-1. Dilutions of all formulations were added into 96-well plates to reach 0.1 mL final volume. The negative control was Mueller Hinton Broth without bacteria. The 96-well plate of each bacterial strain was incubated at 37°C for 24 h. After this period, the MIC of A. baumannii, P. aeruginosa and K. pneumoniae was determined for each NLC. One-way ANOVA/Tukey post hoc statistical tests were adopted to evaluate inter-group statistical differences (p< 0.05), determined by Microsoft Excel Professional Plus 2019 and Action version 2.9 software.
2.6 Structural characterization
The structural characterization was performed by infrared spectroscopy measurement (FTIR-ATR), differential scanning calorimetry (DSC) and field emission scanning electron analyses (FE-SEM). In the FTIR-ATR technique, the spectral range was 650 to 4000 cm-1, with resolution of 2 cm-1. The DSC analyses were performed using a TA equipment, model Q20. Samples were added in sealed aluminum pans. Analyzes were carried out in a nitrogen atmosphere, at a flow rate of 50 mL/min-1, in the temperature range from 0 to 100°C, at a heating rate of 10°C/min. In order to elucidate the morphology of NLC, FE-SEM analyses was performed using Tescan brand, model VEGA 3 microscope, operating in high vacuum under a voltage of 20 kV. Briefly, a drop of each sample was adhered to a cover glass previously fixed in a stub. After, the stubs were subjected to sputtering procedure for 120 s at 30 kV. Then, the samples were visualized in different magnifications.
2.7 Thermal cycling stability study
The thermal stability was evaluated by 3 consecutive temperature cycles totaling 21 days of monitoring in a Climatic Chamber Stability (Solab, Piracicaba, Brazil). Each cycle corresponded to 7 days of NLC storage under different critical conditions, such as: 4 days at 40°C followed by 3 days at 15°C. At the end each cycle, nanoparticle size (nm), polydispersity index (PDI) and Zeta potential (mV) were measured, by DLS through Litesizer equipment (Anton Paar Instruments, Anton Paar, Berlin, Germany), in triplicate. One-way ANOVA/Tukey post hoc statistical tests were used to evaluate intragroup statistical differences over time (p < 0.05), calculated by GraphPad Prism 8 software.
2.8 In vivo nanotoxicity assay on chicken embryo model
The nanotoxicity of NLC formulation was evaluated through in vivo chicken embryo model (CE), according to the following parameters: viability (%) and weight (g) (Ribeiro et al., 2020). 80 eggs of Gallus gallus, lineage W-36, were used. Before analyses, the eggs were submitted to ovoscopy to ensure the viability of the embryos at 7 days of development. Then, they were weighted and divided into 10 CE groups (n = 8) as follow: negative control (NC), eggs treated with 0.85% saline; NLC control (without EO), cinnamon EO emulsified with poloxamer (both at the same concentration as the NLC formulation) and NLC/CAN. All treatments were administered on CAM of CE at different concentrations: 0.5, 0.3 and 0.1% (w/v). Then, the eggs were incubated in automatic incubator (Premium Ecológica®) at 37.5°C and 55% air humidity for 7 days. The CE mortality was daily observed to determine the viability (%). At 14 days of embryonic development, the eggs were weighted.
The weight changes of CE were calculated through the difference between the weight of the eggs before and after the treatments, according to the following equation:
where aW = egg weight adjusted to 50 g; ce.ysW = embryo or annexes weight on 14 day of embryonic develop; ieW = initial egg weight on 7 day of embryonic develop.
The One-way ANOVA/Tukey tests were used to elucidate the intergroup statistical differences, in terms of CE weight (p< 0.05). For the CE viability test, chi-square test was used followed by the difference between two proportions test (p< 0.05). GraphPad Prism 8 software was used for these analyses.
3 Results
3.1 Screening of vegetable and essential oils
A preliminary screening of 14 natural oils was carried out in order to select those with the best activity against those microbial strains, in comparison with polymyxin B (Table 2). It was observed that cinnamon and oregano EO have inhibited all bacterial species and strains tested. Cinnamon EO exhibited inhibition zones ranging from 21.0 to 37.5 mm while oregano EO was responsible for inhibition zones ranging from 9.5 to 39.5 mm. Polymyxin B exhibited an average inhibition zone of 14.1 mm, considering all strains of the bacterial species evaluated. The assay also showed that peppermint EO and tea tree EO were capable of inhibiting the bacterial growth, producing inhibition zones ranging from 10.0 to 33.0 mm and 10.5 to 20.0 mm, respectively. Then, these EO were nanoencapsulated as active and structural compound of NLC.
3.2 Long-term physicochemical stability study
Figure 1 showed the results of the long-term physicochemical stability monitoring of the investigated formulations. The control formulation (F5) exhibited an average particle size of 303.5 ± 46.3 nm statistically higher (p< 0.05) than the others NLC based-EO, with the highest fluctuation in particle size over time.
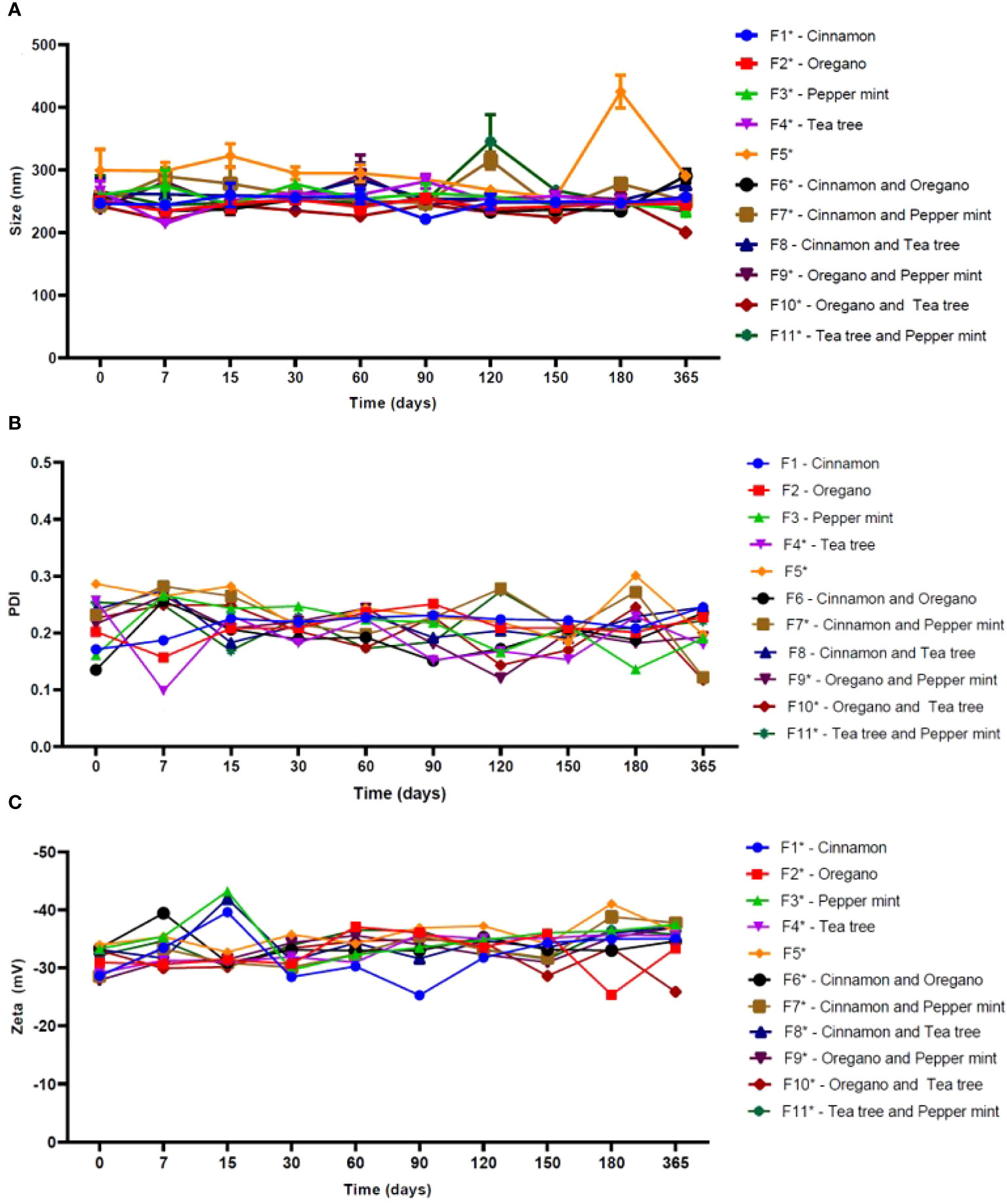
Figure 1 Physicochemical stability of NLC formulations in terms of size (A), PDI (B) and Zeta potential (C), over 365 days at 25°C (n = 3). NOTE: One-way ANOVA/Tukey were used to analyze intragroup statistics; *p< 0.05. PDI: polydispersion index.
Among the samples, only F8 formulation showed no statistically significant changes (p = 0.09) over time. Mean values of PDI ranged from 0.11 to 0.23 among the systems containing EO in its structure, while NLC control had a mean value of 0.24. In this case, F1 (p = 0.36), F2 (p = 0.45), F3 (p = 0.08), F6 (p = 0.05) and F8 (p = 0.22) did not show significant changes over time. The average values of Zeta potential of F5 were -35.7 mV. Among the systems containing EO, these values ranged from -32.2 to -35.2 mV. In this parameter, all NLC showed statistically significant variations (p< 0.05) over time.
3.3 Evaluation of the in vitro antibacterial activity
All prepared NLC were submitted to the in vitro antimicrobial activity test against the different bacterial strains through MIC test. This assay determined that the formulations F1, F6, F7 and F8 showed antimicrobial activity against all bacterial species and strains evaluated. However, F1 formulation, composed of cinnamon EO, ucuuba butter and stabilized by poloxamer, was the most efficient system against A. baumannii, P. aeruginosa and K. pneumoniae inhibiting their growth, in the lowest possible concentration (Table 3). Thus, F1 after 12 months of storage was submitted to MIC assay again, in order to elucidate if the antimicrobial activity was maintained over time. It was observed that this nanosystem maintained the activity against all bacterial species investigated. Moreover, MIC values after a year was higher than that observed at the beginning of the study (Table 3) with the exception of Bactray K. pneumoniae, Responsive K. pneumoniae and IMP-1 P. aeruginosa species.
3.4 Structural characterization
Structural characterization was performed for NLC/CAN (F1), NLC control and its excipients. In FTIR-ATR analysis (Figure 2), the cinnamon EO spectrum revealed intense absorption bands at 1672 cm-1 (aldehyde C=O stretch), 1628 cm-1 (alkene C=C stretch) and 1450 cm-1 (C-C in the aromatic ring). In the vibrational spectrum of ucuuba butter, there are characteristic bands centered at 2915 cm-1 and a group of bands between 1393 and 1177 cm-1, corresponding to the –CH2 vibration. Strong bands centered at 1732 and 2848 cm-1 related to the vibration C=O and O-CH2, respectively, were also observed. The surfactant poloxamer showed typical bands to aliphatic chain ethers centered at 2880 and 1348 cm-1, associated with vibrations υO-CH2 and υO-C-O, respectively. In this same spectrum, it was evidenced characteristic bands of the symmetric deformation δCH2 at 1470 cm-1 and the axial stretching of CO (υCO) at 1098 cm-1.
FTIR-ATR spectra of NLC/CAN and NLC (control) revealed characteristic bands for these systems, resulting from the overlap of most of ucuuba butter and poloxamer bands, as expected. However, the NLC/CAN spectrum showed that the absorption band in the regions at 2852 - 2883 cm-1 became less intense when compared to the NLC spectrum in the region of 2850-2916 cm-1. Also, in the NLC/CAN spectrum, the absorption bands at 1672, 1628 and 1450 cm-1 observed in cinnamon EO were not detected.
Calorimetric analyses are necessary to demonstrate thermodynamic changes of formulations in relation to their excipients. Figure 3 shows the thermograms of NLC control, NLC/CAN and all their excipients. DSC analysis showed that NLC/CAN presented a thermal profile compatible with its solid lipid matrix (ucuuba butter, 45.4°C), with the melting point of 47.1°C. Endothermic peaks corresponding to the melting point of poloxamer and NLC control was observed at 54.5 and 47.6°C, respectively.
FE-SEM images of NLC/CAN and NLC control showed that both formulations presented nanoparticles with spherical morphology and with well-defined contours, as expected for this type of system (Figure 4). In addition, the images revealed spherical monodisperse distributed nanoparticles, compatible with the morphology of NLC composed of synthetic and traditional lipids excipients (Ribeiro et al., 2016; Calixto et al., 2021; Castro et al., 2021; Moura et al., 2021).
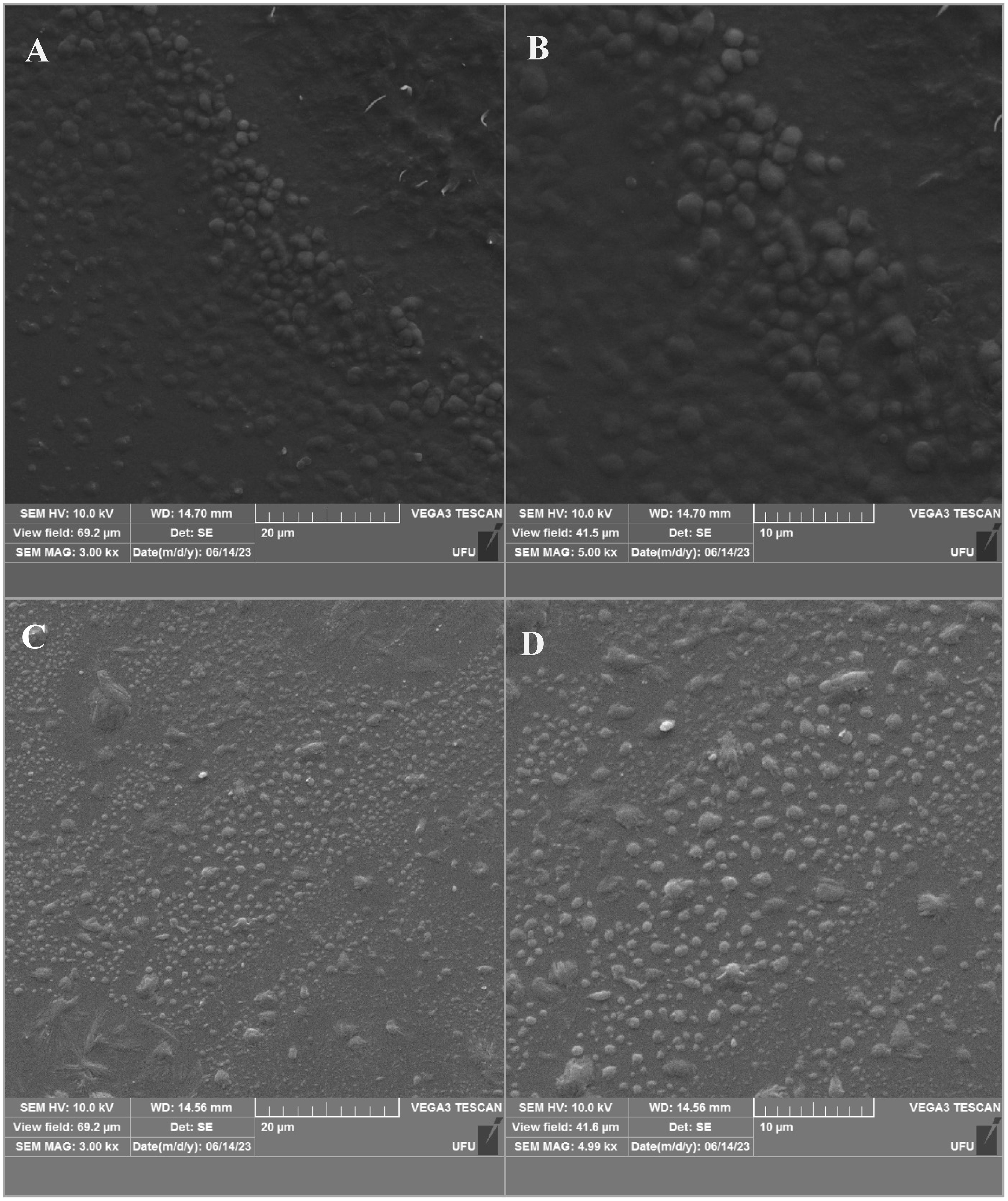
Figure 4 Scanning Electron Microscopy images of NLC/CAN (A, B) and NLC control (C, D). The images are available in two different magnifications: 3000x (left) and 5000x (right).
3.5 Thermal cycling stability study
Thermal cycling stability study is useful to evaluate possible biophysical changes of nanoparticles under critical conditions of storage. Figure 5 showed a thermal cycling stability study of the NLC/CAN and NLC (control) formulations.
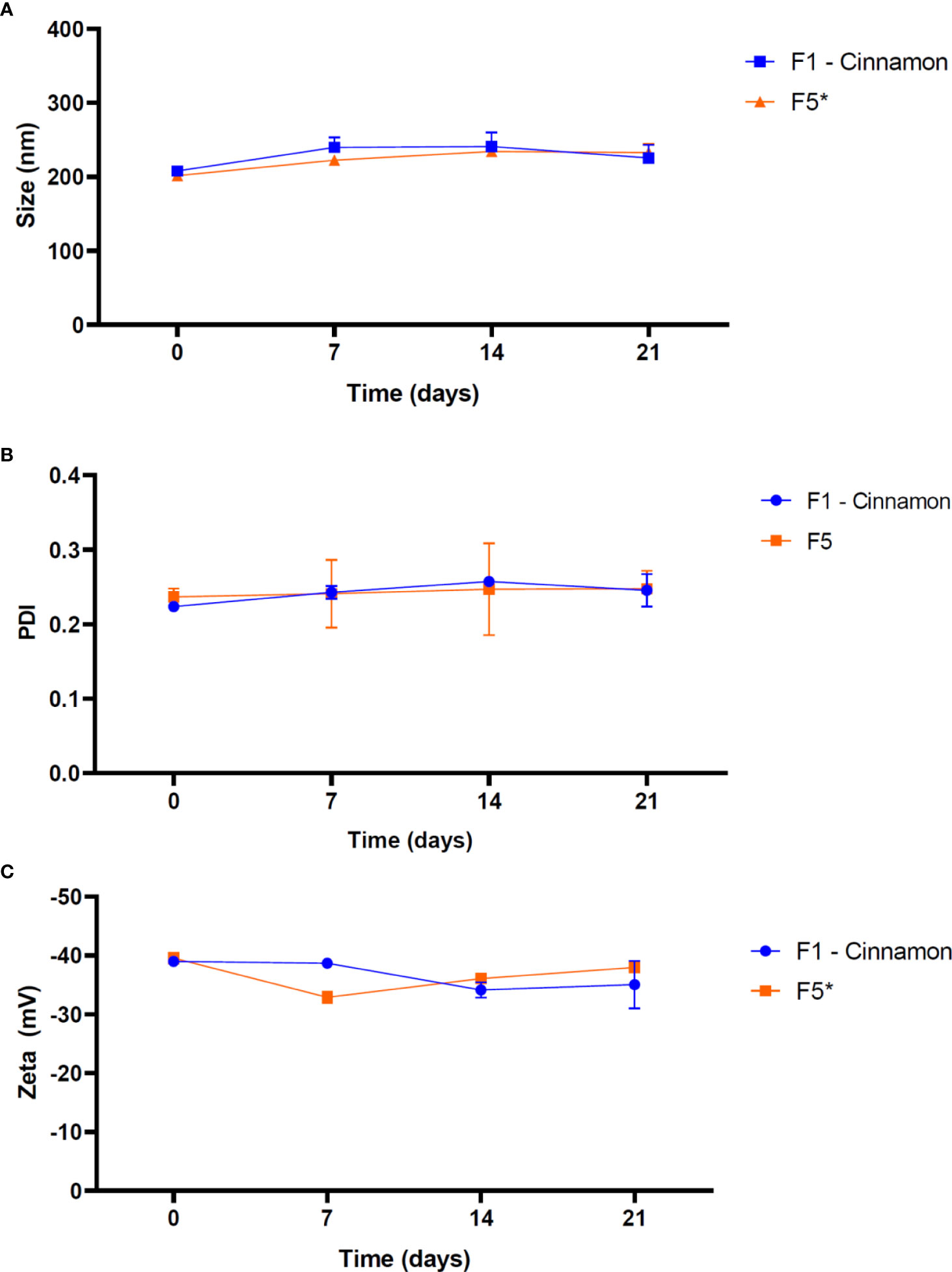
Figure 5 Thermal stability of NLC formulations in terms of size (A), PDI (B) and Zeta potential (C), at the end each temperature cycle. 1 cycle = 7 days of storage (4 days at 40°C followed by 3 days at 15°C). NOTE: One-way ANOVA/Tukey were used to analyze intragroup statistics; *p< 0.05. PDI: polydispersion index.
Particle size of NLC/CAN exhibited no statistically significant changes over time, ranging from 208.00 nm to 241.20 nm at the end of 21 days. The PDI values obtained guaranteed that NLC/CAN presented a monodisperse size distribution even after storage under critical temperature conditions, which did not show statistically significant changes. ZP values ranged from - 34.13 to - 39.00 mV for NLC/CAN.
3.6 In vivo nanotoxicity test through chicken embryo model
Figure 6 shows the mortality of embryos treated with negative control (NC = saline solution), NLC/CAN, NLC and cinnamon EO emulsified with poloxamer. There was no statistical difference between the treatments in comparison to the NC. Additionally, among alive embryos, there were no statistically significant macroscopic alterations compared to NC. However, regarding to the weight changes, the Figure 7 demonstrates that there was a statistically significant difference (p< 0.05) in the weight of embryos treated with EO emulsion in comparison to the NC.
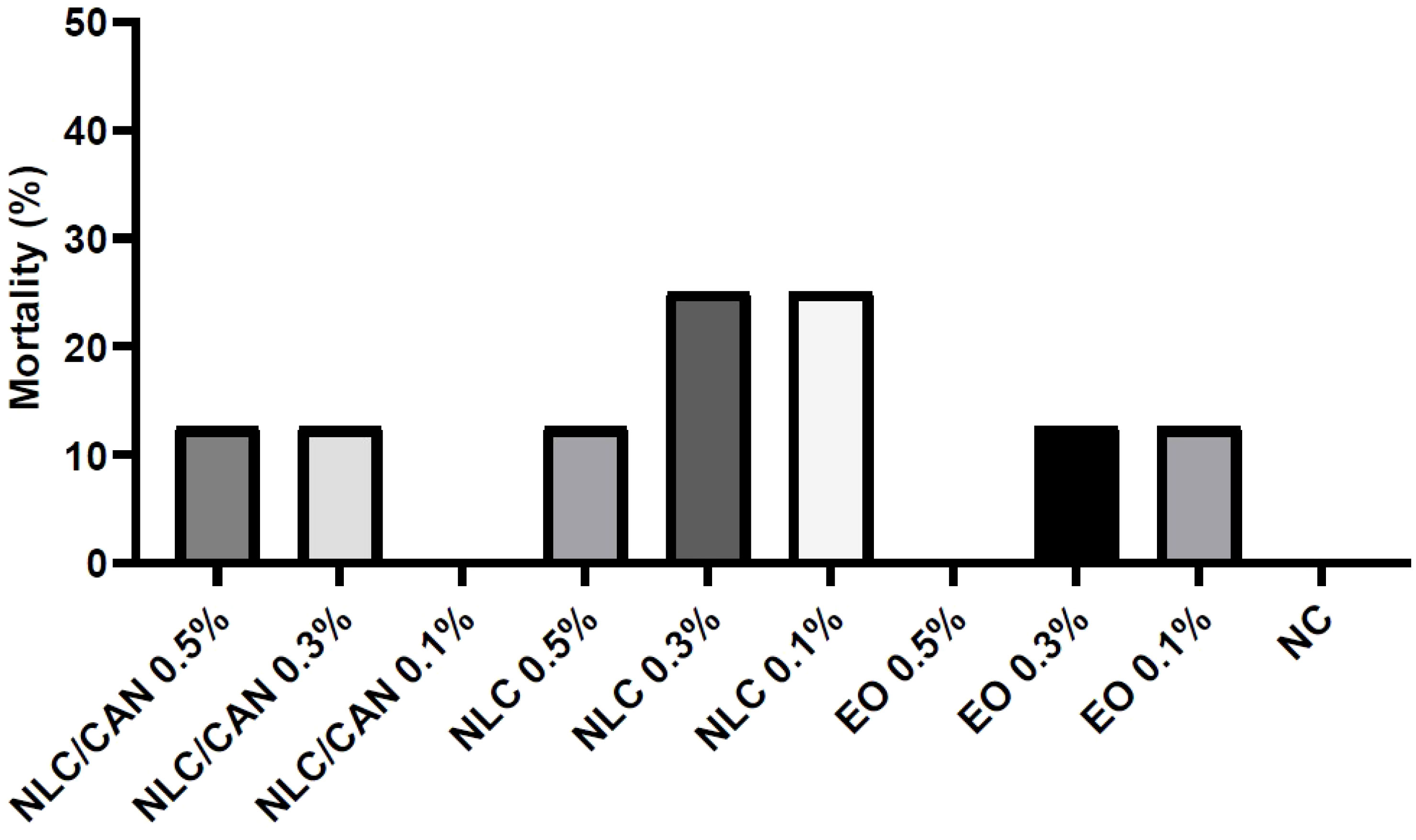
Figure 6 Mortality of chicken embryos treated with NLC/CAN, NLC and cinnamon EO emulsion (EO) at different concentrations.
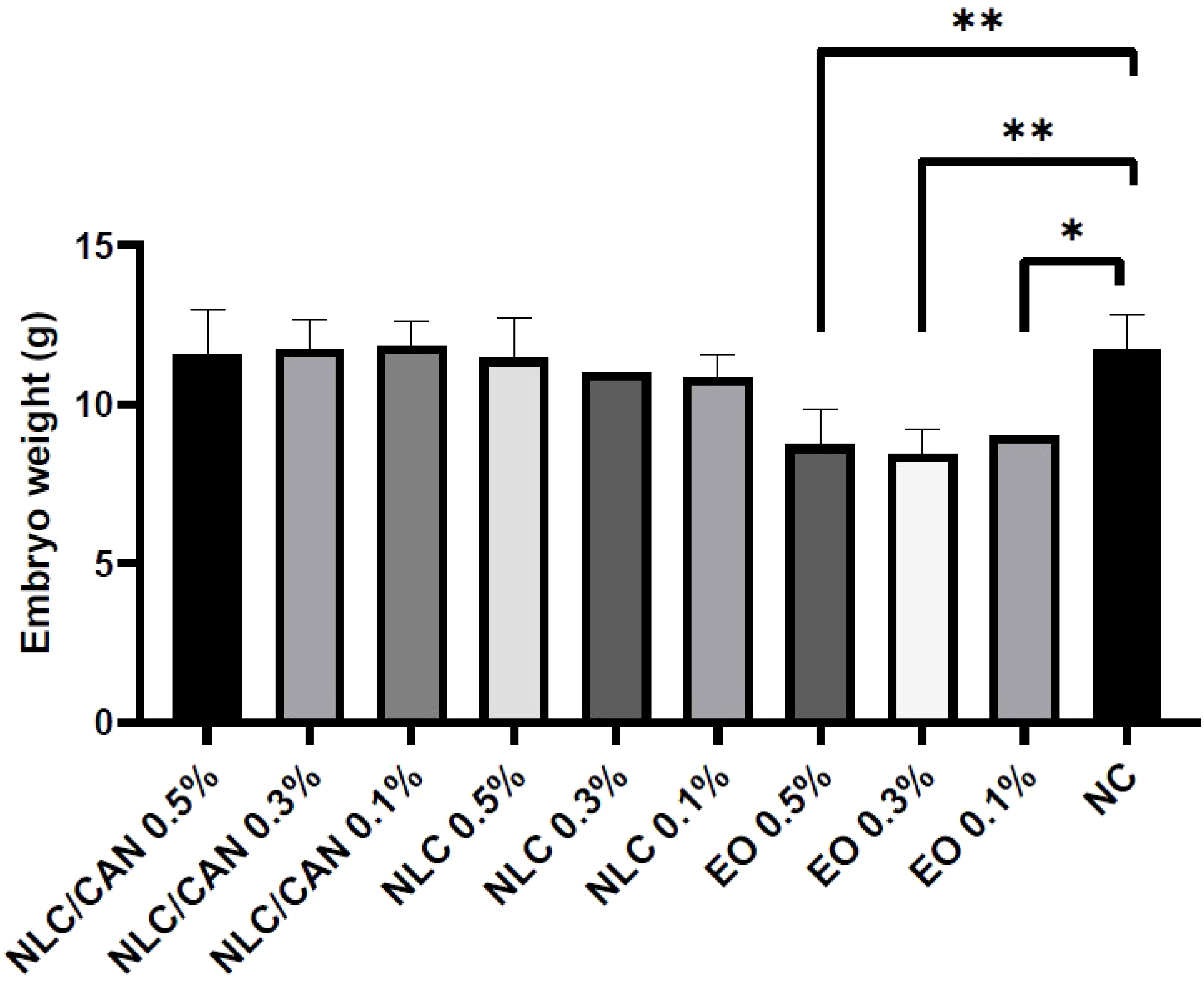
Figure 7 In vivo toxicity study in terms of chicken embryo weight under treatment with NLC/CAN, NLC and cinnamon EO emulsion (EO) at different concentrations. ** p< 0.01 and * p< 0.03.
4 Discussion
Currently, there is a huge interest in the elucidation of the therapeutic properties of EO. There are several works that have described exciting results of EO uses in the cosmetic, agriculture, food and pharmaceutical fields (Castro et al., 2019; Guidotti-Takeuchi et al., 2022; Chukwuma et al., 2023).
The identification of bioactive compounds that represent an effective alternative to antibiotics is an important step in drug development in an attempt to minimize the bacterial resistance (Kaskatepe et al., 2016). In here, it was identified that cinnamon, oregano, peppermint and tea tree EO showed activity against the bacterial strains studied. However, the performance of oregano EO was strain-dependent in relation to P. aeruginosa, once a single strain showed resistance to this EO. Similar result was found for strains of P. aeruginosa, tested under the same conditions, showed different responses to the same EO (Coseriu et al., 2023), corroborating the difficulty to treat this type of infection. Other studies have also showed significant antibacterial properties for cinnamon EO, which inhibited the growth of A. baumannii, P. aeruginosa and K. pneumoniae (El-Kattan and Allam, 2021; Ganić et al., 2022; Coseriu et al., 2023). Furthermore, this EO exhibited higher antibiofilm activity against K. pneumoniae, followed by peppermint EO (Sarwar et al., 2022). Man et al. (2019) investigated the antibacterial activity of some EO against some Gram-positive and Gram-negative bacteria, including K. pneumoniae strains. It was also observed that oregano EO is a bioactive compound with promising antibiotic activity.
NLC formulations were monitored is terms of particle size (nm), polydispersion index (PDI) and Zeta potential (mV) in the long-term stability study in order to ensure the shelf time of such systems. This study is mandatory to ensure the quality control of the formulations. Regarding the particle size, there was not observed any tendency to increase in size as a function of time, which would be indicative of some physicochemical instability process, such as agglutination of nanoparticles. Moreover, regarding the size distribution profiles, a successful lipid nanocarriers should present homogeneous size distribution (monodisperse), with values as low as possible, to ensure long-term stability. Values ≤ 0.2 are usually accepted to describe a monodisperse distribution. Additionally, nanocarrier formulations with constant and narrow size distribution are correlated with better clinical results (Danaei et al., 2018). According to observed here, EO was responsible for reducing and making more uniform the particle size distribution of NLC when compared to the control formulation (without EO), elucidating its structural role in the system in addition to the already bioactivity claimed (Ribeiro et al., 2021). Furthermore, Zeta potential also brings information about the stability of colloidal aqueous dispersions (Souto et al., 2004). In general, good stability is achieved with Zeta potential values above ±25 mV ensuring electrokinetic stabilization of the colloidal dispersion (Thatipamula et al., 2011). For sterically stabilized systems, as those use non-ionic as surfactants, the Zeta values are more related to the sample composition, presenting slightly or moderately negative values, as observed here (Scioli Montoto et al., 2020), indicating a desirable colloidal electrokinetic stability.
In addition, the thermal cycling study was carried out to bring some evidence regarding the control quality of NLC submitted to critical storage conditions. Such test provides information of the temperature and humidity conditions for a successful transport and distribution of NLC. In agreement with the long-term stability study. Such results strongly suggested that a cheap process to scale up, storage and distribution of such nanoformulations will be possible.
The in vitro antimicrobial activity of such formulations was determined by MIC. It was observed that the formulations that had cinnamon EO in their composition (F1, F6, F7 and F8) have showed activity against all the analysed strains/species and that the F1 formulation maintained in vitro antibacterial activity even after 1 year of storage at room temperature. Other work also revealed that the loaded cinnamon EO exhibited promising antibacterial activity and prevented both biofilm formation from A. baumannii strains, also exhibiting antibiofilm capacity (Ganić et al., 2022). Another work described that the strains of P. aeruginosa also showed susceptibility to cinnamon EO at lowest concentrations, (0.125% v/v). Such bactericidal effect of EO from the bark was higher than cinnamon leaf, probably due to the presence of Σ-cinnamaldehyde as the main component (85.3%). Furthermore, it was already shown that this EO induced rapid death of P. aeruginosa PAO-1, possibly due to the bacterial cell membrane rupture (Elcocks et al., 2020) and the synergistic action among its bioactive components, affecting gene expression and the activity of bacterial efflux pumps (Coseriu et al., 2023).
Then, it was selected NLC/CAN (F1) as the most effective nanoformulation. However, it is still necessary to continue investigating the efficacy of this nanosystem in more complex biological models, in order to obtain more robust results.
The F1 structural characterization through FTIR-ATR, DSC, FE-SEM methods, evidenced that the excipients were compatible with each other. In general, the F1 spectrum was based on the overlapped bands of ucuuba butter and poloxamer, their major components. However, in the NLC/CAN spectrum, there is strong evidence of interaction with EO, due to the absence of absorption bands in the regions at 1672, 1628 and 1450 cm-1 of cinnamon EO, suggested an excellent miscibility between ucuuba butter and EO. Furthermore, NLC have showed thermal stability up to 100°C, without any thermal event related to the degradation of fatty acids, as required for this type of system. The structural and thermal analyses have also confirmed that the EO is part of the structure of the NLC, providing lower viscosity and crystallinity to the lipid matrix (Ribeiro et al., 2021). Such achieved corroborated the complex supramolecular arrangement of NLC in comparison with SLN (composed of only solid lipid in the structural matrix). The blend of lipids results in a more amorphous form with structural imperfections resulting in more stable and efficient formulations (Souto et al., 2004).
Finally, the safety of NLC/CAN formulation was ensured through the in vivo nanotoxicity test on CE model. All the nanoformulations and concentrations tested were safe, once they did not show any statistically significant differences in comparison to the NC, in all the parameters analysed. However, the CE weight changes treated with cinnamon EO emulsion was statistically significant different (p< 0.05) compared to the NC, confirming the protective effect of loading EO by NLC. It is worth mentioning that emulsion was the first colloidal delivery systems already reported, and it is widely known that system is unstable, being not applicable as treatment candidate or nanosanitizer (Araújo et al., 2019). This approach had also determined the higher safe dose on embryos for further efficacy tests. Moreover, it was observed that NLC/CAN, composed of cinnamon EO, ucuuba butter and poloxamer was safe up to 50 mg/mL of cinnamon EO loaded by NLC, much higher than the effective concentration value given by MIC, which was around 1.2 mg/mL.
The benefits of nanostructured drugs can be realized mainly for cancer treatments, with some nanocolloidal systems applied as gold standard treatments, as Doxil® (Ben Venue Laboratories, Inc Bedford, OH) and Myocet® (GP-Pharm, Barcelona, Spain). In the commercial antimicrobials nanoparticle-based drugs, 3 lipid-based formulations of amphotericin B are commonly used for the systemic treatment of fungal infections: Ambisome® (Gilead Sciences, Inc, San Dimas, CA), Amphotec® (Ben Venue Laboratories, Inc, Bedford, OH) and Abelcet® (Sigma-Tau PharmaSource, Inc, Indianapolis, IN) (Adler-Moore and Proffitt, 2002; Yeh et al., 2011). Despite the excellent biophysical and biological activity of nanoformulations, there are a lot of challenges to be overcome until they reach the market. This is still necessary considering the particularities on nanostructured system in the regulatory issues, as well as in the biological assays planning, in order to decrease the time of approval and provided standardized methods to specifically evaluated the nanotoxicity in the biological tissues and environment.
The data found in here strongly suggest that NLC/CAN formulation remained stable up to 12 months, exhibiting excellent properties in terms of particle size, homogeneity and surface charge at the end of experiment. So, the NLC/CAN is a promising system, being economically viable to be used as a surface disinfectant or antiseptic of hospitals. Besides, the same system has structural properties that allows its administration on parenteral and topical (skin and mucosa) routes, aiming to be a safe and more efficient antibiotic treatment against multidrug resistant species.
5 Conclusions
NLC formulations that encapsulated EO demonstrated excellent long-term stability (1 year, at 25°C). Such system was effective against Acinetobacter baumannii, Klebsiella pneumoniae and Pseudomonas aeruginosa isolated from humans. The structural characterization confirmed a supramolecular organization compatible with NLC formulations and thermal stability of the systems up to 100°C, making them suitable for use in biomedical applications. The evaluation of nanotoxicity in embryos showed that the NLC/CAN formulation was safe under all conditions tested. Furthermore, it was observed that the safe concentration was higher than the effective one. Based on these promising results, the NLC/CAN will be further submitted in vivo efficacy studies on chicken embryo and mammal model, in order to find an effective, safe and low-cost therapeutic option against infections by multidrug-resistant bacteria.
Data availability statement
The original contributions presented in the study are included in the article/Supplementary Material. Further inquiries can be directed to the corresponding author.
Ethics statement
The manuscript presents research on animals that do not require ethical approval for their study.
Author contributions
Ed: Conceptualization, Data curation, Formal Analysis, Investigation, Methodology, Visualization, Writing – original draft, Writing – review & editing. LB: Funding acquisition, Project administration, Resources, Supervision, Writing – review & editing. BF: Methodology, Formal analysis, Data curation, Funding acquisition, Project administration, Resources, Supervision, Writing – review & editing. RR: Writing – review & editing. SS: Investigation, Writing – review & editing. HP: Investigation, Writing – review & editing. Fd: Investigation, Writing – review & editing. LR: Conceptualization, Data curation, Formal Analysis, Funding acquisition, Investigation, Methodology, Project administration, Resources, Supervision, Visualization, Writing – review & editing.
Funding
The author(s) declare financial support was received for the research, authorship, and/or publication of this article. This research was funded by Coordenação de Aperfeiçoamento de Pessoal de Nível Superior – CAPES (88882.461704/2019-01), Project CNPq/MCTI/CT-Health N° 52/2022 - Actions in Science, Technology and Innovation to face Antimicrobial Resistance (408640/2022-7) and INCT em Teranóstica e Nanobiotecnologia (INCT-TeraNano, CNPq Processo n° 403193/2022-2 e FAPEMIG Processo n° CBB - APQ-03613-17).
Acknowledgments
The authors would like to thank the Brazilian funding agencies (CNPq and CAPES) for providing financial support. This work is dedicated to the memory of Professor Luiz Ricardo Goulart Filho.
Conflict of interest
The authors declare that the research was conducted in the absence of any commercial or financial relationships that could be construed as a potential conflict of interest.
Publisher’s note
All claims expressed in this article are solely those of the authors and do not necessarily represent those of their affiliated organizations, or those of the publisher, the editors and the reviewers. Any product that may be evaluated in this article, or claim that may be made by its manufacturer, is not guaranteed or endorsed by the publisher.
References
Adler-Moore, J., Proffitt, R. T. (2002). AmBisome: liposomal formulation, structure, mechanism of action and pre-clinical experience. J. Antimicrobial Chemother. 49, 21–30. doi: 10.1093/jac/49.suppl_1.21
Araújo, D. R., Ribeiro, L. N., de, M., de Paula, E. (2019). Lipid-based carriers for the delivery of local anesthetics. Expert Opin. Drug Delivery 16, 701–714. doi: 10.1080/17425247.2019.1629415
Batchelder, J. I., Hare, P. J., Mok, W. W. K. (2023). Resistance-resistant antibacterial treatment strategies. Front. Antibiotics 2. doi: 10.3389/frabi.2023.1093156
Braga, I. A., Campos, P. A., Gontijo-Filho, P. P., Ribas, R. M. (2018). Multi-hospital point prevalence study of healthcare-associated infections in 28 adult intensive care units in Brazil. J. Hosp. Infection 99, 318–324. doi: 10.1016/j.jhin.2018.03.003
Calixto, G. M. F., Muniz, B. V., Castro, S. R., de Araujo, J. S. M., de Souza Amorim, K., Ribeiro, L. N. M., et al. (2021). Mucoadhesive, thermoreversible hydrogel, containing tetracaine-loaded nanostructured lipid carriers for topical, intranasal needle-free anesthesia. Pharmaceutics 13, 1760. doi: 10.3390/pharmaceutics13111760
Carbone, C., Martins-Gomes, C., Caddeo, C., Silva, A. M., Musumeci, T., Pignatello, R., et al. (2018). Mediterranean essential oils as precious matrix components and active ingredients of lipid nanoparticles. Int. J. Pharm. 548, 217–226. doi: 10.1016/j.ijpharm.2018.06.064
Castro, S. R., Paula, E., Mendonça, T. C., Ribeiro, L. N., de, M., Lancelotti, M., et al. (2019). Carreadores Lipídicos Nanoestruturados Antimicrobianos. 1–31. BR1020190267305, filing date: 15/12/2019, patented by Instituto Nacional da Propriedade Industrial (INPI), Brazil. (Brazil: Instituto Nacional da Propriedade Industrial).
Castro, S. R., Ribeiro, L. N. M., Breitkreitz, M. C., Guilherme, V. A., Rodrigues da Silva, G. H., Mitsutake, H., et al. (2021). A pre-formulation study of tetracaine loaded in optimized nanostructured lipid carriers. Sci. Rep. 11, 21463. doi: 10.1038/s41598-021-99743-6
Chukwuma, I. F., Uchendu, N. O., Asomadu, R. O., Ezeorba, W. F. C., Ezeorba, T. P. C. (2023). African and Holy Basil - a review of ethnobotany, phytochemistry, and toxicity of their essential oil: Current trends and prospects for antimicrobial/anti-parasitic pharmacology. Arabian J. Chem. 16, 104870. doi: 10.1016/j.arabjc.2023.104870
Cimino, C., Maurel, O. M., Musumeci, T., Bonaccorso, A., Drago, F., Souto, E. M. B., et al. (2021). Essential oils: Pharmaceutical applications and encapsulation strategies into lipid-based delivery systems. Pharmaceutics 13, 1–35. doi: 10.3390/pharmaceutics13030327
Coseriu, R. L., Vintilă, C., Pribac, M., Mare, A. D., Ciurea, C. N., Togănel, R. O., et al. (2023). Antibacterial Effect of 16 Essential Oils and Modulation of mex Efflux Pumps Gene Expression on Multidrug-Resistant Pseudomonas aeruginosa Clinical Isolates: Is Cinnamon a Good Fighter? Antibiotics 12, 163. doi: 10.3390/antibiotics12010163
Dajic Stevanovic, Z., Sieniawska, E., Glowniak, K., Obradovic, N., Pajic-Lijakovic, I. (2020). Natural macromolecules as carriers for essential oils: from extraction to biomedical application. Front. Bioeng. Biotechnol. 8. doi: 10.3389/fbioe.2020.00563
Danaei, M., Dehghankhold, M., Ataei, S., Hasanzadeh Davarani, F., Javanmard, R., Dokhani, A., et al. (2018). Impact of particle size and polydispersity index on the clinical applications of lipidic nanocarrier systems. Pharmaceutics 10, 57. doi: 10.3390/pharmaceutics10020057
Dupuis, V., Cerbu, C., Witkowski, L., Potarniche, A.-V., Timar, M. C., Żychska, M., et al. (2022). Nanodelivery of essential oils as efficient tools against antimicrobial resistance: a review of the type and physical-chemical properties of the delivery systems and applications. Drug Delivery 29, 1007–1024. doi: 10.1080/10717544.2022.2056663
El Asbahani, A., Miladi, K., Badri, W., Sala, M., Addi, E. H. A., Casabianca, H., et al. (2015). Essential oils: From extraction to encapsulation. Int. J. Pharm. 483, 220–243. doi: 10.1016/j.ijpharm.2014.12.069
Elcocks, E. R., Spencer-Phillips, P. T. N., Adukwu, E. C. (2020). Rapid bactericidal effect of cinnamon bark essential oil against Pseudomonas aeruginosa. J. Appl. Microbiol. 128, 1025–1037. doi: 10.1111/jam.14538
El-Kattan, N., Allam, K. A. M. (2021). The antibacterial activity of nano-encapsulated basil and cinnamon essential oils against certain multidrug-resistant bacteria recovered from infected wounds. Novel Res. Microbiol. J. 5, 1447–1462. doi: 10.21608/nrmj.2021.207867
Elshafie, H. S., Camele, I. (2017). An overview of the biological effects of some mediterranean essential oils on human health. BioMed. Res. Int. 2017, 14. doi: 10.1155/2017/9268468
Freire, M. P., de Assis, D. B., Tavares, B., de, M., Brito, V. O. C., Marinho, I., et al. (2023). Impact of COVID-19 on healthcare-associated infections: Antimicrobial consumption does not follow antimicrobial resistance. Clinics 78, 100231. doi: 10.1016/j.clinsp.2023.100231
Ganić, T., Vuletić, S., Nikolić, B., Stevanović, M., Kuzmanović, M., Kekić, D., et al. (2022). Cinnamon essential oil and its emulsion as efficient antibiofilm agents to combat Acinetobacter baumannii. Front. Microbiol. 13. doi: 10.3389/fmicb.2022.989667
Guidotti-Takeuchi, M., Ribeiro, L. N., de, M., dos Santos, F. A. L., Rossi, D. A., Della, F., et al. (2022). Essential oil-based nanoparticles as antimicrobial agents in the food industry. Microorganisms 10, 1504–1526. doi: 10.3390/microorganisms10081504
Kariyawasam, R. M., Julien, D. A., Jelinski, D. C., Larose, S. L., Rennert-May, E., Conly, J. M., et al. (2022). Antimicrobial resistance (AMR) in COVID-19 patients: a systematic review and meta-analysis (November 2019–June 2021). Antimicrob. Resist. Infect. Control 11, 45. doi: 10.1186/s13756-022-01085-z
Kaskatepe, B., Kiymaci, M. E., Suzuk, S., Erdem, S. A., Cesur, S., Yildiz, S. (2016). Antibacterial effects of cinnamon oil against carbapenem resistant nosocomial Acinetobacter baumannii and Pseudomonas aeruginosa isolates. Ind. Crops Prod. 81, 191–194. doi: 10.1016/j.indcrop.2015.11.058
Katopodi, A., Detsi, A. (2021). Solid Lipid Nanoparticles and Nanostructured Lipid Carriers of natural products as promising systems for their bioactivity enhancement: The case of essential oils and flavonoids. Colloids Surf. A. Physicochem. Eng. Asp. 630, 127529. doi: 10.1016/j.colsurfa.2021.127529
Lammari, N., Louaer, O., Meniai, A. H., Fessi, H., Elaissari, A. (2021). Plant oils: From chemical composition to encapsulated form use. Int. J. Pharm. 601, 120538. doi: 10.1016/j.ijpharm.2021.120538
Magiorakos, A.-P., Srinivasan, A., Carey, R. B., Carmeli, Y., Falagas, M. E., Giske, C. G., et al. (2012). Multidrug-resistant, extensively drug-resistant and pandrug-resistant bacteria: an international expert proposal for interim standard definitions for acquired resistance. Clin. Microbiol. Infection 18, 268–281. doi: 10.1111/j.1469-0691.2011.03570.x
Man, A., Santacroce, L., Iacob, R., Mare, A., Man, L. (2019). Antimicrobial activity of six essential oils against a group of human pathogens: A comparative study. Pathogens 8, 15. doi: 10.3390/pathogens8010015
Micheli, G., Sangiorgi, F., Catania, F., Chiuchiarelli, M., Frondizi, F., Taddei, E., et al. (2023). The hidden cost of COVID-19: focus on antimicrobial resistance in bloodstream infections. Microorganisms 11, 1299. doi: 10.3390/microorganisms11051299
Moura, L. D., Ribeiro, L. N. M., de Carvalho, F. V., Rodrigues da Silva, G. H., Lima Fernandes, P. C., Brunetto, S. Q., et al. (2021). Docetaxel and lidocaine co-loaded (NLC-in-hydrogel) hybrid system designed for the treatment of melanoma. Pharmaceutics 13, 1552. doi: 10.3390/pharmaceutics13101552
Murray, C. J. L., Ikuta, K. S., Sharara, F., Swetschinski, L., Robles Aguilar, G., Gray, A., et al. (2022). Global burden of bacterial antimicrobial resistance in 2019: a systematic analysis. Lancet 399, 629–655. doi: 10.1016/S0140-6736(21)02724-0
O’Neill, J. (2016) The Review on Antimicrobial Resistance. Tackling Drug-Resistant Infections Globally: final report and recommendations. Available at: https://amr-review.org/sites/default/files/160518_Final%20paper_with%20cover.pdf (Accessed September 21, 2023).
Osme, S. F., Almeida, A. P. S., Lemes, M. F., Barbosa, W. O., Arantes, A., Mendes-Rodrigues, C., et al. (2020). Costs of healthcare-associated infections to the Brazilian public Unified Health System in a tertiary-care teaching hospital: a matched case–control study. J. Hosp. Infection 106, 303–310. doi: 10.1016/j.jhin.2020.07.015
Pan American Health Organization (2021) Epidemiological Alert Emergence and increase of new combinations of carbapenemases in Enterobacterales in Latin America and the Caribbean. Available at: https://www.paho.org/en/documents/epidemiological-alert-emergence-and-increase-new-combinations-carbapenemases (Accessed September 21, 2023).
Ribeiro, L. N. M., Breitkreitz, M. C., Guilherme, V. A., da Silva, G. H. R., Couto, V. M., Castro, S. R., et al. (2017). Natural lipids-based NLC containing lidocaine: from pre-formulation to in vivo studies. Eur. J. Pharm. Sci. 106, 102–112. doi: 10.1016/j.ejps.2017.05.060
Ribeiro, L. N. M., de Paula, E., Rossi, D. A., Martins, F. A., de Melo, R. T., Monteiro, G. P., et al. (2021). Nanocarriers from natural lipids with in vitro activity against campylobacter jejuni. Front. Cell Infect. Microbiol. 10. doi: 10.3389/fcimb.2020.571040
Ribeiro, L. N. M., de Paula, E., Rossi, D. A., Monteiro, G. P., Júnior, E. C. V., Silva, R. R., et al. (2020). Hybrid pectin-liposome formulation against multi-resistant bacterial strains. Pharmaceutics 12, 769. doi: 10.3390/pharmaceutics12080769
Ribeiro, L. N. M., Franz-Montan, M., Breitkreitz, M. C., Alcântara, A. C. S., Castro, S. R., Guilherme, V. A., et al. (2016). Nanostructured lipid carriers as robust systems for topical lidocaine-prilocaine release in dentistry. Eur. J. Pharm. Sci. 93, 192–202. doi: 10.1016/J.EJPS.2016.08.030
Sarwar, W., Ali, Q., Ahmed, S. (2022). Microscopic visualization of the antibiofilm potential of essential oils against Staphylococcus aureus and Klebsiella pneumoniae. Microsc. Res. Tech. 85, 3921–3931. doi: 10.1002/jemt.24243
Scioli Montoto, S., Muraca, G., Ruiz, M. E. (2020). Solid lipid nanoparticles for drug delivery: pharmacological and biopharmaceutical aspects. Front. Mol. Biosci. 7. doi: 10.3389/fmolb.2020.587997
Souto, E. B., Wissing, S. A., Barbosa, C. M., Müller, R. H. (2004). Evaluation of the physical stability of SLN and NLC before and after incorporation into hydrogel formulations. Eur. J. Pharmaceut. Biopharmaceut. 58, 83–90. doi: 10.1016/j.ejpb.2004.02.015
Tacconelli, E., Carrara, E., Savoldi, A., Harbarth, S., Mendelson, M., Monnet, D. L., et al. (2018). Discovery, research, and development of new antibiotics: the WHO priority list of antibiotic-resistant bacteria and tuberculosis. Lancet Infect. Dis. 18, 318–327. doi: 10.1016/S1473-3099(17)30753-3
Thatipamula, R. P., Palem, C. R., Gannu, R., Mudragada, S., Yamsani, S. (2011). Formulation and in vitro characterization of domperidone loaded solid lipid nanoparticles and nanostructured lipid carriers. Daru 19, 23–32.
Wang, Z., Koirala, B., Hernandez, Y., Zimmerman, M., Park, S., Perlin, D. S., et al. (2022). A naturally inspired antibiotic to target multidrug-resistant pathogens. Nature 601, 606–611. doi: 10.1038/s41586-021-04264-x
World Health Organization (2021) Global Antimicrobial Resistance and Use Surveillance System (GLASS) Report (Geneva). Available at: https://www.who.int/publications/i/item/9789240027336 (Accessed June 23, 2023).
Yao, J., Zou, P., Cui, Y., Quan, L., Gao, C., Li, Z., et al. (2023). Recent advances in strategies to combat bacterial drug resistance: antimicrobial materials and drug delivery systems. Pharmaceutics 15, 1188. doi: 10.3390/pharmaceutics15041188
Keywords: multi-resistant bacteria, nanostructured lipid carriers, vegetable butter, essential oil, natural antibiotics
Citation: da Silva EF, Bastos LM, Fonseca BB, Ribas RM, Sommerfeld S, Pires HM, dos Santos FAL and Ribeiro LNM (2024) Lipid nanoparticles based on natural matrices with activity against multidrug resistant bacterial species. Front. Cell. Infect. Microbiol. 13:1328519. doi: 10.3389/fcimb.2023.1328519
Received: 26 October 2023; Accepted: 19 December 2023;
Published: 09 January 2024.
Edited by:
Ritesh Sevalkar, University of Alabama at Birmingham, United StatesReviewed by:
Sónia Silva, National Institute for Agrarian and Veterinarian Research, PortugalSudeepa Rajan, University of California, Los Angeles, United States
Joana Rolo, University of Beira Interior, Portugal
Copyright © 2024 da Silva, Bastos, Fonseca, Ribas, Sommerfeld, Pires, dos Santos and Ribeiro. This is an open-access article distributed under the terms of the Creative Commons Attribution License (CC BY). The use, distribution or reproduction in other forums is permitted, provided the original author(s) and the copyright owner(s) are credited and that the original publication in this journal is cited, in accordance with accepted academic practice. No use, distribution or reproduction is permitted which does not comply with these terms.
*Correspondence: Lígia Nunes de Morais Ribeiro, bnVuZXNsaWNhQGdtYWlsLmNvbQ==