- 1Antibiotics, Biocides, Residues and Resistance Unit, Fougères Laboratory, French Agency for Food, Environmental and Occupational Health & Safety (ANSES), Fougères, France
- 2Université Paris-Saclay, National Research Institute for Agriculture, Food and the Environment (INRAE), AgroParisTech, Micalis Institute, Jouy-en-Josas, France
Antimicrobial resistance is a critical public health issue that requires a thorough understanding of the factors that influence the selection and spread of antibiotic-resistant bacteria. Biocides, which are widely used in cleaning and disinfection procedures in a variety of settings, may contribute to this resistance by inducing similar defense mechanisms in bacteria against both biocides and antibiotics. However, the strategies used by bacteria to adapt and develop cross-resistance remain poorly understood, particularly within biofilms –a widespread bacterial habitat that significantly influences bacterial tolerance and adaptive strategies. Using a combination of adaptive laboratory evolution experiments, genomic and RT-qPCR analyses, and biofilm structural characterization using confocal microscopy, we investigated in this study how Escherichia coli biofilms adapted after 28 days of exposure to three biocidal active substances and the effects on cross-resistance to antibiotics. Interestingly, polyhexamethylene biguanide (PHMB) exposure led to an increase of gentamicin resistance (GenR) phenotypes in biofilms formed by most of the seven E. coli strains tested. Nevertheless, most variants that emerged under biocidal conditions did not retain the GenR phenotype after removal of antimicrobial stress, suggesting a transient adaptation (adaptive resistance). The whole genome sequencing of variants with stable GenR phenotypes revealed recurrent mutations in genes associated with cellular respiration, including cytochrome oxidase (cydA, cyoC) and ATP synthase (atpG). RT-qPCR analysis revealed an induction of gene expression associated with biofilm matrix production (especially curli synthesis), stress responses, active and passive transport and cell respiration during PHMB exposure, providing insight into potential physiological responses associated with adaptive crossresistance. In addition, confocal laser scanning microscopy (CLSM) observations demonstrated a global effect of PHMB on biofilm architectures and compositions formed by most E. coli strains, with the appearance of dense cellular clusters after a 24h-exposure. In conclusion, our results showed that the PHMB exposure stimulated the emergence of an adaptive cross-resistance to gentamicin in biofilms, likely induced through the activation of physiological responses and biofilm structural modulations altering gradients and microenvironmental conditions in the biological edifice.
1 Introduction
Antimicrobial resistance (AMR) of bacteria stands as a significant threat to human health in the coming decades. Although resistant strains have existed for millions of years prior to the development of clinical drugs, their (mis)use has accelerated the rapid selection and spread of multidrug resistant bacteria (Davies and Davies, 2010). Among the different bacterial families, concerns on multidrug resistance are particularly high in Enterobacteriaceae (Iovleva and Doi, 2017; Alkofide et al., 2020; Atamna-Mawassi et al., 2021). Treatment against Enterobacteriaceae include several families of antibiotics, notably fluoroquinolones and aminoglycosides and understanding the mechanisms of bacterial resistance to these antibiotics is of primary importance (Krause et al., 2016; World Health Organization, 2019; Bodendoerfer et al., 2020; Zhu et al., 2020). Additional drivers can also promote the emergence and propagation of AMR in interconnected environments (Aslam et al., 2021), highlighting the importance of identifying these factors and understanding their actual role in AMR. Among these factors, biocides are widely used in various environments, such as food-processing industries to mitigate the risk of pathogenic bacteria dissemination through the food chain. Unlike antibiotics, which often have specific targets in the bacterial cell, biocides typically employ a multi-targeted mode of action. However, mechanisms developed by bacteria to resist biocides can be close or imply common cellular components to those involved in antibiotic resistance and multiple possible cross-resistances have already been identified in different bacterial species (Maillard, 2018; Charron et al., 2023). Schematically, when exposing bacteria to a biocide, cross-adaptations could result from two major types of mechanisms. First, bacteria can become cross-resistant if stable mutations occur in a genetic target involved in both biocide and antibiotic resistance (mutational resistance). Second, physiological adaptations and gene expression modulations in response to biocidal stresses can confer bacteria a greater ability to transiently withstand both biocide or antibiotic challenge (adaptive resistance) (Fernández et al., 2011; Fernández and Hancock, 2012; Sindeldecker and Stoodley, 2021; D’Aquila et al., 2023). The potential of some biocidal active susbtances such as triclosan (Chuanchuen et al., 2001; Sanchez et al., 2005; Karatzas et al., 2007; Tkachenko et al., 2007; Fernández-Cuenca et al., 2015; Lu et al., 2018; Sonbol et al., 2019) or quaternary ammonium compounds (Bore et al., 2007; Fernández-Cuenca et al., 2015; Soumet et al., 2016; Kim et al., 2018; Guérin et al., 2021; Douarre et al., 2022) to select cross-resistance to antibiotics have already been recurrently reported for instance. However, data regarding the antimicrobial resistance effects of biocides are still lacking for the majority of widely used molecules and the underlying mechanisms are unclear. Given their widespread and extensive usage, coupled with the potential for cross-resistance with antibiotics, it is imperative to investigate and comprehend the role of biocides in AMR selection and dissemination.
Numerous studies have examined the impact of biocide on AMR using planktonic bacteria, the most common lifestyle studied in laboratories. However, it is important to recognize that bacteria predominantly live in surface-associated communities, commonly known as biofilms (Römling, 2023). Biofilms represent intricate three-dimensional structures formed by bacterial communities, embedded within a self-produced extracellular matrix. This matrix is composed of a wide variety of substances including exopolysaccharides, lipids, proteins, amyloids and nucleic acids (Karygianni et al., 2020; Flemming et al., 2023). The composition and structure of biofilms depend on the bacterial species and microenvironmental conditions, significantly influencing bacterial metabolism in a reciprocal manner. The dense tridimensional structure creates chemical gradients, resulting in distinct microenvironments for bacteria residing in the periphery, exposed to the external medium, as opposed to those in the inner layers, typically with low access to nutrients and oxygen (Jo et al., 2022). This chemical heterogeneity favors varied physiological responses, giving rise to different populations, including persister cells or viable but non-culturable phenotypes, often characterized by a substantial growth slowdown (Charron et al., 2023). These metabolic states can confer a heightened tolerance to antimicrobials, thus enhancing bacterial survival and potential acquisition of genetic resistance (Levin-Reisman et al., 2017; Nordholt et al., 2021). Furthermore, the external matrix often acts as a barrier, restricting the entry and the diffusion of biocidal substances inside of the biofilm, further increasing bacterial survival (Davison et al., 2010; Bridier et al., 2011).
The combination of these intricate factors is likely to influence significantly how bacteria adapt to their environment. Understanding the potential interplay between biocide exposure, biofilm adaptation and the emergence of AMR is therefore crucial. In this study, we aimed to investigate the effect of the exposure to three commonly used biocidal active substances (benzalkonium chloride (BAC), polyhexamethylene biguanide (PHMB), and N-(3-aminopropyl)-N-dodecylpropane-1,3-diamine (TMN) on the emergence of resistance against two critically important antibiotics for human medicine, the aminoglycoside gentamicin and the fluoroquinolone ciprofloxacin in Escherichia coli biofilms (World Health Organization, 2019).
2 Materials and methods
2.1 Bacterial strains and growth conditions
Seven E. coli strains were used in this study. Six strains originated from the collection of the national reference laboratory (NRL) for Antimicrobial Resistance hosted in Anses Fougères laboratory composed of E. coli isolated in pork (Ec694; Ec709; Ec723; Ec956) and poultry industries (Ec775; Ec478) between 2014 and 2018. The Ec223 strain originated from the CIRM collection from INRAe and was initially isolated on a chicken. These strains were conserved at -80°C in cryotubes (Mast Group, Bootle, UK). The strains were grown at 37°C on trypticase soy agar (TSA) or in ten times diluted trypticase soy broth (1/10 TSB).
2.2 Biocidal substances used and minimal inhibitory concentration assay
Strains susceptibility to three biocides has been tested: BAC (Stepan France); PHMB (Matrix Scientific France) and TMN (Quaron SAS France). The minimal inhibitory concentration (MIC) is here defined as the lowest concentration where cells are unable to grow and were determined as previously described with slight modifications (Guérin et al., 2021). Strains were grown overnight in 1/10 TSB medium and then adjusted to 0.2 (+/− 0.02) optical density at 620 nm (OD620). Microplates were filled with 200 µL of biocides in line A at the highest challenging concentration. 100µL of 1/10 TSB were added in line B to H. A cascade dilution with 100µL of previous line was done from A to H. Finally, 10µL of adjusted bacterial preculture were added in each well. Microplates were incubated at 20°C overnight and MIC were determined after 24 and 48 h of incubation. Experiments were performed in duplicate.
2.3 Experimental evolution with three biocides
Strains were grown overnight in 5ml of 1/10 TSB medium from a colony picked on TSA. On the next day, strains were diluted in fresh 1/10 TSB to reach an OD of 0.2 (+/- 0.02). For each strain, 4 columns of 8 wells on a 96-wells flat-bottom microtiter plate (Greiner Bio-One 655161) were filled with 190µL of 1/10 TSB and 10µL of the adjusted subcultures were added to reach a final OD of 0.01 (with an empty column between each). Plates were incubated at 20°C during 1h to enable adhesion of bacteria. Supernatants were then removed and replaced with 200 µl of fresh 1/10 TSB medium and plates were then incubated during 72h at 20°C to enable biofilm development.
After initial biofilm formation, a first cycle began. On the first cycle day, supernatants were removed and 40µL of fresh 1/10 TSB were added. Biofilms were sampled by scratching a quarter of the well bottom surface using tips. 5µL of the biofilm suspension were dropped on TSA plates supplemented with 10 mg/L of the aminoglycoside gentamicin (Gen). This Gen concentration corresponds to 5x the Ecoff value for E. coli (https://mic.eucast.org). 5µL of the biofilm suspension were also dropped on TSA supplemented with 1.2 mg/L of the fluoroquinolone ciprofloxacin (Cip). This Cip concentration corresponds to 20x the Ecoff value for E. coli. Preliminary tests were done to confirm these concentrations were able to discriminate between a sensitive and a resistant E. coli strain. Antibiotic -supplemented TSA plates were then incubated 48 h at 37°C to enable growth of Gen resistant (GenR) and Cip resistant (CipR) variants. Concurrently, 200µL of fresh 1/10 TSB containing biocides were added in wells of the biofilm microtiter plate with each column (8 wells) corresponding to one condition: H2O, BAC, TMN, PHMB. The exposure concentrations used were respectively 6.25 mg/L for BAC, 2.5 mg/L for PHMB, 6.6 mg/L for TMN and were chosen based on MIC values determined on planktonic cells of the 7 strains (Table S1). Then, used medium was daily renewed with 200 µL fresh 1/10 TSB medium supplemented with biocides for 4 days. On the last day, the used medium was renewed, and the biofilm microtiter plate was incubated 72h at 20°C. The full cycle (one week) was repeated 4 times to reach a total of 28 days of exposure.
2.4 Assessment of resistance phenotypic stability
The stability of resistance phenotype in variants collected on antibiotic-supplemented plates after biocide exposure was assessed by repeatedly subculturing resistant variants without selective pressure and then checking the persistence of antibiotic resistance. Concretely, GenR variants collected from biofilms were spread on TSA plates and incubated at 37°C during 24 h. This step was repeated 4 times. Finally, variants were spread a fifth time on Gen-supplemented TSA plates to confirm they still displayed GenR phenotypes.
2.5 Enterobacterial repetitive intergenic consensus – PCR
ERIC-PCR was used to compare the patterns observed for the variants and their parental strain to ensure that the different collected variants were not due to contamination as previously described (Cuzin et al., 2021). DNA was first extracted using an InstaGene kit (Bio-rad, Marnes-la-Coquette, France) and amplified using a LightCycler® 480 thermocycler (Roche Diagnostics, Meylan, France) with primers ERIC1-R (ATGTAAGCTCCTGGGGATTCAC) and ERIC2 (AAGTAAGTGACTGGGGTGAGCG) and GoTaq Flexi polymerase (Promega, Charbonnières-les-bains, France) as follows: 95 °CC for 2 min for initial melting; 30 cycles at 95 °CC for 1 min, 54 °CC for 1 min, 72 °CC for 4 min; final extension at 72 °CC for 8 min followed by incubation at 4 °CC. PCR products were then checked on 1% agarose gel and migrated over 90 min at 110 V before being revealed using a GelRED stain (Biotium, Brumath, France).
2.6 DNA extraction, sequencing and genomic analyses
E. coli genomes were sequenced by paired-end short-reads WGS. Bacteria were first grown on Thermo Scientific™ Tryptone Soya Agar with 5% Sheep Blood plates (Thermo Fischer Scientific, PB5012A), overnight at 37°C. One colony was then inoculated in 5mL of a 1/10 Tryptone Soja Broth (TSB) solution and incubated overnight at 37°C. Total DNA was extracted from 1 ml of bacterial suspension using the Macherey-Nagel Nucleospin Tissue kit according to the manufacturer’s instructions (Macherey-Nagel, Düren, Germany). The concentration and quality of DNA were checked using a BioSpec-nano spectrophotometer (Shimadzu, Marne la Vallée, France). Illumina sequencing was performed on the strains at Institut du Cerveau et de la Moelle épinière (Paris, France) using the Novaseq 6000 SP. Nextera XT kit was used to prepare Illumina libraries. Before analyzing the reads, their qualities were analyzed with FastQC software and filtered with Trimmomatic (v0.39, ILLUMINACLIP : NexteraPE-PE.fa:2:30:10:8:keepBothReads HEADCROP:15 SLIDINGWINDOW:4:25). De novo assembly of parental strains was performed with Unicycler (v0.4.8). Contigs smaller than 200nt were removed from the newly formed genomes and Prokka (v1.14.6) was used for annotation. Variant calling was carried out using Snippy (v4.6.0) using filter reads for each variant, using as reference the genome of variant’s parental strain. The analysis pipelines are available on the following GitHub repository: https://github.com/Arnaud-Bridier/BAoBAb
2.7 RNA extraction and RT-qPCR
The levels of expression of various genes of interest were also assessed during adaptation to PHMB (list of genes available in Table S2). In that aim, Ec223 biofilms were prepared and exposed to H2O or PHMB for 28 days as previously described in section 3. At 0 (before exposure), 7, 14, and 28 days, total RNA was isolated from 8 wells for each experimental condition using the NucleoSpin RNA kit according to the manufacturer’s instructions (Macherey-Nagel, Hoerd, France) with the following modifications. A first enzymatic lyse was performed with per well 25 µL mix composed of lysozyme 1 mg/mL, Tris 10 mM and EDTA 1 mM for 10 minutes at 37°C. RNA was quantified, and its purity was assessed with the Biospec-Nano (Shimadzu, Marne la Vallée, France). Reverse transcription, qPCR, primers design and analyses were performed as previously described (Reale et al., 2019) with the following modifications. Reverse transcription was performed with 1.3 µg of total RNA, and quantitative PCR reactions were carried out with 2.6 ng cDNA. All primers were purchased from Sigma-Aldrich (Saint Quentin Fallavier, France), and additional information on oligonucleotide primers are listed in Table S2. Using NormFinder software, the gene hcaT was chosen as a reference gene since it did not exhibit any significant variation of expression among the samples. Three independent experiments were performed.
2.8 CLSM analysis of biofilm architectural modulations
Biofilm architectures were characterized using CLSM observations in microtiter plates compatible with high resolution imaging (Bridier et al., 2010). For the 7 strains, 72h-biofilms were first grown in 1/10 TSB as previously described and then challenged for 24 h by renewing the used biofilm growth medium with 200µL of 1/10 TSB medium supplemented with 1.25 mg/L of PHMB (or H2O for controls). Plates were incubated during 24 h at 20°C. Plates were analyzed with a Leica HCS-SP8 CLSM at the INRAE MIMA2 Imaging Core Facility (Microscopie et Imagerie des Microorganismes, Animaux et Aliments, INRAE, Jouy-en-Josas, doi.org/10.15454/1.5572348210007727E12). Total bacterial cells were dyed using the SYTO™9 green fluorescent permeant nucleic acid stain (Invitrogen, USA) and matrix production was contrasted using 8 µg/ml Congo red staining (Sigma-Aldrich, France). Both markers were excited with an Argon Laser set at 488 nm for SYTO™9 and 514 nm for Congo red. Fluorescence emissions were collected using photomultiplier tubes (PMT) at wavelengths of 500-550nm for SYTO™9 and 600-750nm for Congo red. Biofilm three-dimensional structures were scanned with a 63x water objective lens (numerical aperture: 1.2), with a resolution of 512x512 and a 1 µm Z-step. For each strain, a total of 12 stacks per condition (PHMB-exposed or H2O controls) was captured corresponding to 2 Z-stacks per well, with 6 different wells per strain on 2 different plates. Biofilm 3D reconstructions were performed using the Imaris 9.3.1 software (Bitplane, AG-Zürich, Switzerland) via the maximum intensity projection (MIP) section view. Quantitative biofilm biovolumes were extracted from CLSM images with BiofilmQ 0.2.2 (Hartmann et al., 2021).
2.9 Statistical analysis
Frequencies of occurrence of GenR variants in H2O and in every biocide conditions were compared using the Kruskal-Wallis test (P value: * = [0.05-0.01]; ** = [0.01-0.001]; *** = <0.001).
RT-q-PCR data analysis was performed with the Graphpad Prism software. For each week, a Student t-test was performed between the PHMB and H2O condition (P value: * = [0.05-0.01]; ** = [0.01-0.001]; *** = <0.001).
3 Results
3.1 Quantification of GenR variants during biofilm exposure to biocides
E. coli biofilms were exposed to BAC, PHMB and TMN for 28 days and bacterial cells collected and enumerated on antibiotic-supplemented TSA plates along biocide exposure to investigate how this stress could influence the emergence of resistant variants against two antibiotics: the fluoroquinolone Cip and the aminoglycoside Gen. We did not detect any resistant variants on Cip-supplemented plates (CipR variants) in the biofilm of the seven E. coli strains after exposure to the three different biocides or H2O (data not shown). Conversely, Gen resistant (GenR) variants were detected in different strains and conditions with differences between biocides and the control group (H2O) as displayed in Figure 1A, where the frequency of GenR variant occurrence corresponds to the proportion of replicates for each strain in which at least one GenR variant was detected. After the first week of exposure (day 7), GenR variants tended to emerge preferentially in the control group rather than in presence of biocides (without significative difference). However, upon PHMB exposure, the proportions of GenR-positive replicates variants progressively increased over time in all seven strains when compared to other biocides or in H2O controls, revealing a similar effect of PHMB on different E. coli strains. After 28 days, the frequency of occurrence of GenR variants was significantly higher in the PHMB-exposed group than in the other conditions, including H2O (p=0.008704). Conversely, in the H2O control, the increase in GenR variants frequency after 28 days was mainly attributed to one strain (Ec709), where GenR variants were seen in every replicate.
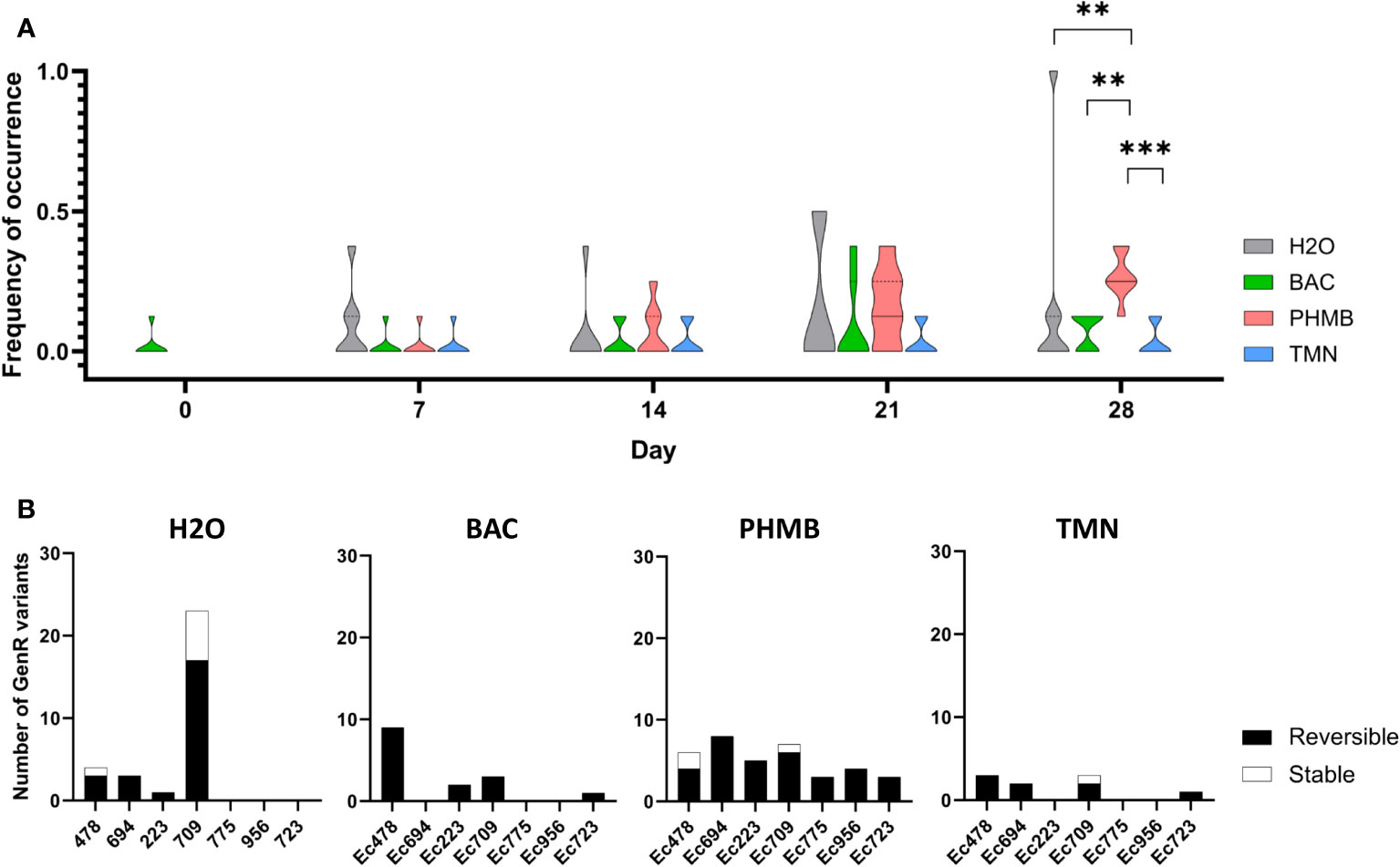
Figure 1 (A) Violin plots representing the dynamics of emergence of GenR variants (frequency of occurrence) in biofilms of the different strains in different conditions. Kruskal Wallis Tests were done for each week, comparing every condition, P value: *[0.05-0.01]; **[0.01-0.001]; ***<0.001 (B) Resistance phenotype stability of GenR variants collected for each of the seven (E) coli strains during experimental evolution (NB: Due to the high number of variants emerging in H2O for the Ec709 strain, a previous selection was made, this graph is thus not showing the real quantity of emerging variants for this specific condition).
Stability assays were performed for each GenR variant to differentiate the number of variants that acquired a stable GenR phenotype and those with a transitory response (Figure 1B). Proportions of unstable GenR variants (losing the GenR phenotype after 5 subcultures without Gen selective pressure and called hereafter transient GenR variants) were consistently higher than the stable ones in the different conditions with minor variations. Among the 31 variants isolated in H2O, 7 of them were stable (23%) and emerged in strain Ec478 and especially in strain Ec709. Notably, strain Ec709 was the only strain exhibiting a higher number of GenR variants in the H2O control than in the PHMB condition after the 28 days exposure. Concerning the biocides, only 4 of the 60 variants isolated in the different biocide conditions had a stable resistance phenotype, with 3 of them emerging in the PHMB condition (strains Ec478 and Ec709) and another in the TMN condition (strain Ec709).
3.2 Genomic analysis of stable GenR variants
The genomes of 11 GenR variants with stable resistance phenotype (8 from Ec709 and 3 from Ec478) that were collected during the experimental evolution phase were sequenced and analyzed in comparison with their respective parental strain. Genetic events responsible for the emergence of GenR phenotype were identified and are displayed in Table 1. Genomic analysis of these variants revealed several genetic targets, mainly involved in the electron transport chain. Variants that emerged under biocide conditions exhibited similar affected pathways to those in the H2O control group. Two genes were targeted in more than one variant: cydA and atpG. The most frequently targeted gene was cydA, which was mutated in 5 among the 11 variants and was found in both Ec478 and Ec709, as well as in both H2O and PHMB conditions. This gene encodes a subunit of cytochrome bd-I, involved in the production of H+, that can be converted into energy for the cell. The atpG gene, which codes for a subunit of the ATP synthase, was mutated in 3 out of the 11 variants and was exclusively found in Ec709 strain. Other mutations were identified in similar target genes, including cyoC, which codes for another cytochrome subunit, as well as genes associated with ferric transport systems (fdx + fre), transcription regulators (rpoS + arcB), tRNA processing (tsaB), glycolysis (aceE) and an elongation factor, fusA, which is implicated in the translocation of the ribosome during protein synthesis.
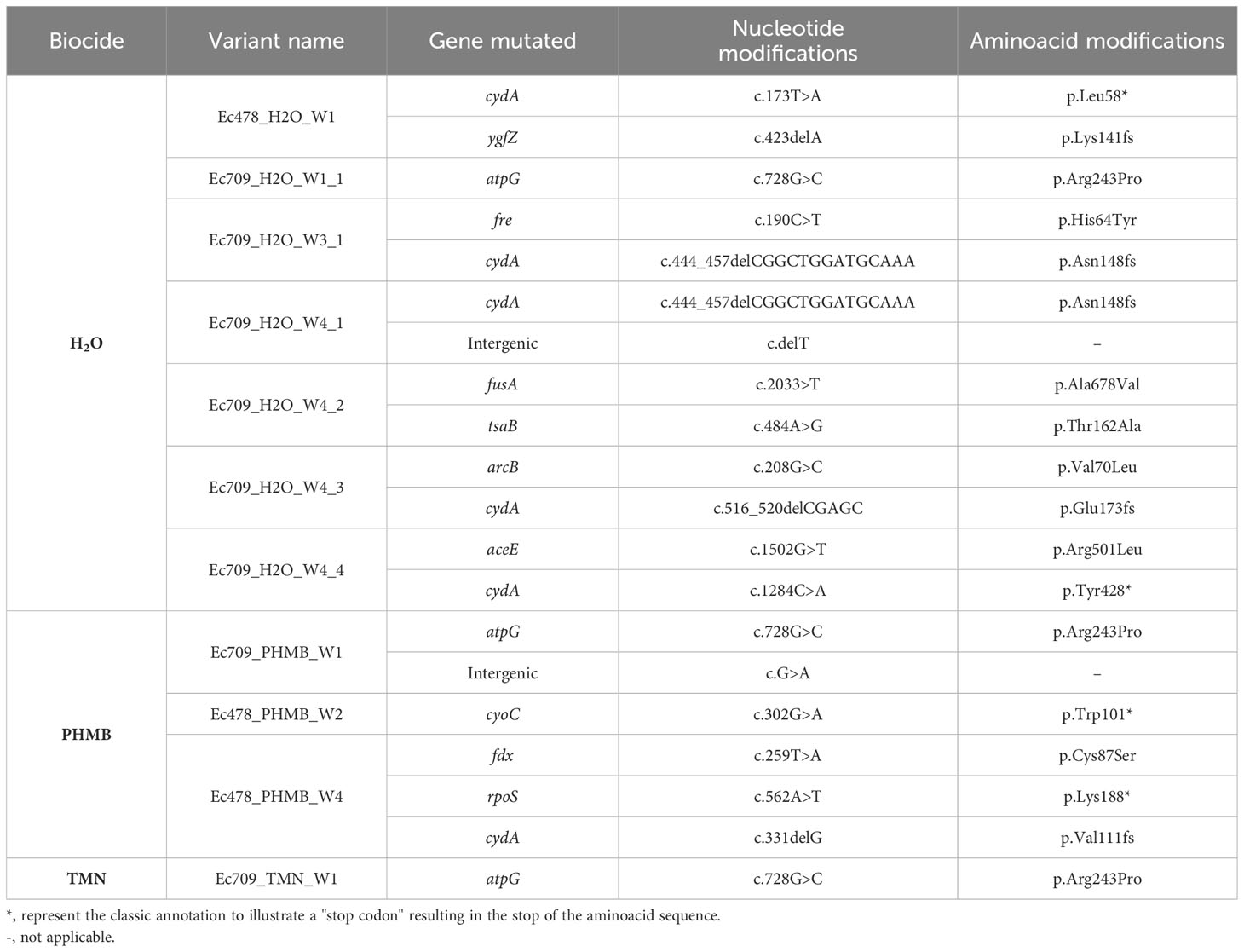
Table 1 GenR variants emerging in Ec478 and Ec709 in the control group, in PHMB and in TMN throughout the weeks and the associated mutations and aminoacid modifications.
3.3 Gene expression analysis in presence of PHMB
To gain better insights into biofilm adaptation and the bacterial response to PHMB, we examined changes in gene expression levels after 28 days of PHMB-exposure, comparing them to H2O condition using RT-qPCR in the Ec223 strain (Figure 2). Efflux pumps (acrAB) and porins (ompCF) were explored because of their known effect against antimicrobials. Biofilm formation genes and stress responses were also investigated because of their relevance in the adaptation in a biofilm model. Finally, cellular metabolism genes were chosen to see if transient resistance was linked to the same genetic targets as the variants with acquired resistance. Globally, most of the pronounced variations occurred after one week of exposure and decreased over subsequent weeks, suggesting an initial response to the PHMB stress followed by a possible adaptation. Various genes were thus significantly overexpressed in the presence of PHMB after 1 week of exposure. Notably, csgB (fold change: 7.23) which is involved in curli biosynthesis and biofilm formation, showed a substantial increase, along with genes involved in stress response, such as rpoS, the activator of global stress response (fold change: 3.64) and recA, which mediates the SOS response (fold change: 5.46). Levels of expression of csgB and recA in the presence of PHMB remained elevated with regards to fold changes values compared to H2O condition during the second part of experimental evolution at days 14 (3.40 and 3.78) and days 28 (7.58 and 3.56), although difference was not statistically significant. In a lesser extent, genes encoding proteins involved in passive transport ompC/F (fold changes: ompC: 2.77; ompF: 2.45) or active efflux acrA (fold change: 1.50) were also significantly up-regulated in PHMB compared to H2O after 1 week of exposure. Finally, some cell metabolism genes such as cydA (fold change: 2.75) and fur (fold change: 3.52), a transcription regulator regulating the expression of several respiratory chain genes and ferric transporters, were also differentially expressed in the first week of PHMB exposure. Other genes showed an overexpression tendency without statistically significant difference, including arcA, the global regulator of the cell respiration process, flu, the gene involved in the synthesis of the auto-aggregation factor Ag43, and other metabolism genes such as ackA, an acetate kinase, and aceE, a pyruvate dehydrogenase.
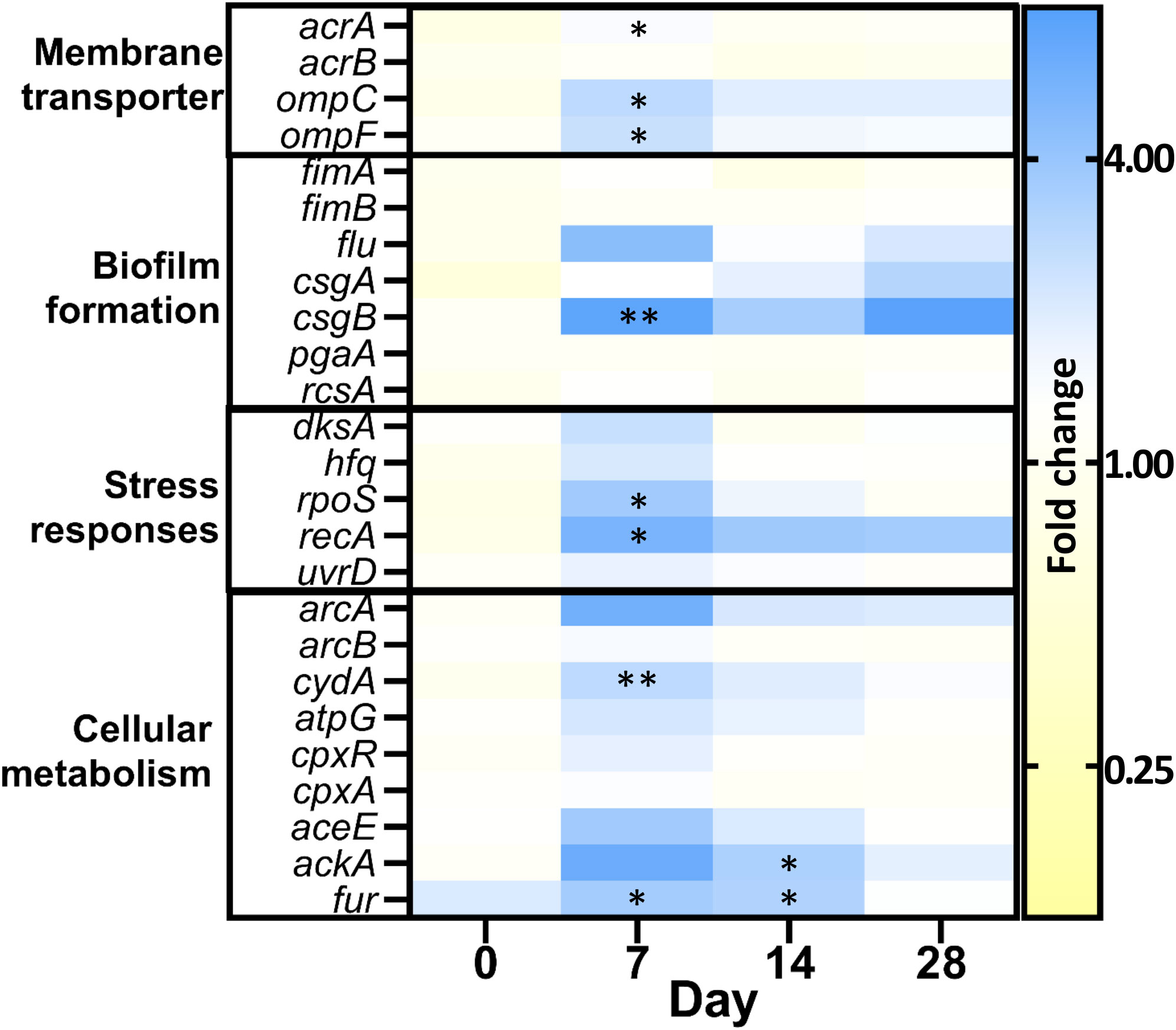
Figure 2 Gene expression differentiation in presence of PHMB, in the Ec223 strain. Fold changes were normalized on the hcaT gene and the H2O control. Values >1 indicate an increased expression in presence of PHMB and <1 a decreased expression in presence of PHMB. (P value: *[0.05-0.01]; **[0.01-0.001]).
3.4 Biofilm structural modulation after PHMB exposure
The impact of PHMB exposure on the architectural properties of biofilms formed by the seven E. coli strains was also investigated using CLSM to observe potential structural perturbations resulting from bacterial community adaptation. Bacterial cells were stained with SYTO™9 and part of the extracellular matrix was stained with Congo red, a dye known to stain amyloid fibers, including curli, which are essential components of the biofilm matrix. First, CLSM observations revealed that PHMB exposure provoked recurrent structural modifications among most E. coli strains, leading to the formation of dense clusters as shown on CLSM sections in Figure 3. The quantification of biofilm structures following both fluorescent staining (SYTO™9 and Congo red) provided complementary data showing that such architectural modulations in response to PHMB were related to slightly different processes depending on strains. One group composed of Ec223, Ec478, Ec709 and Ec775 had an induction of the matrix production and a reduction in cell biovolume after PHMB exposure. A second group comprising Ec694 and Ec723 also showed a reduction in cell biovolume but no induction of the biofilm matrix. Finally, Ec956 was the only strain with an increase in cell biovolume accompanied by an increase in biofilm matrix production after PHMB exposure.
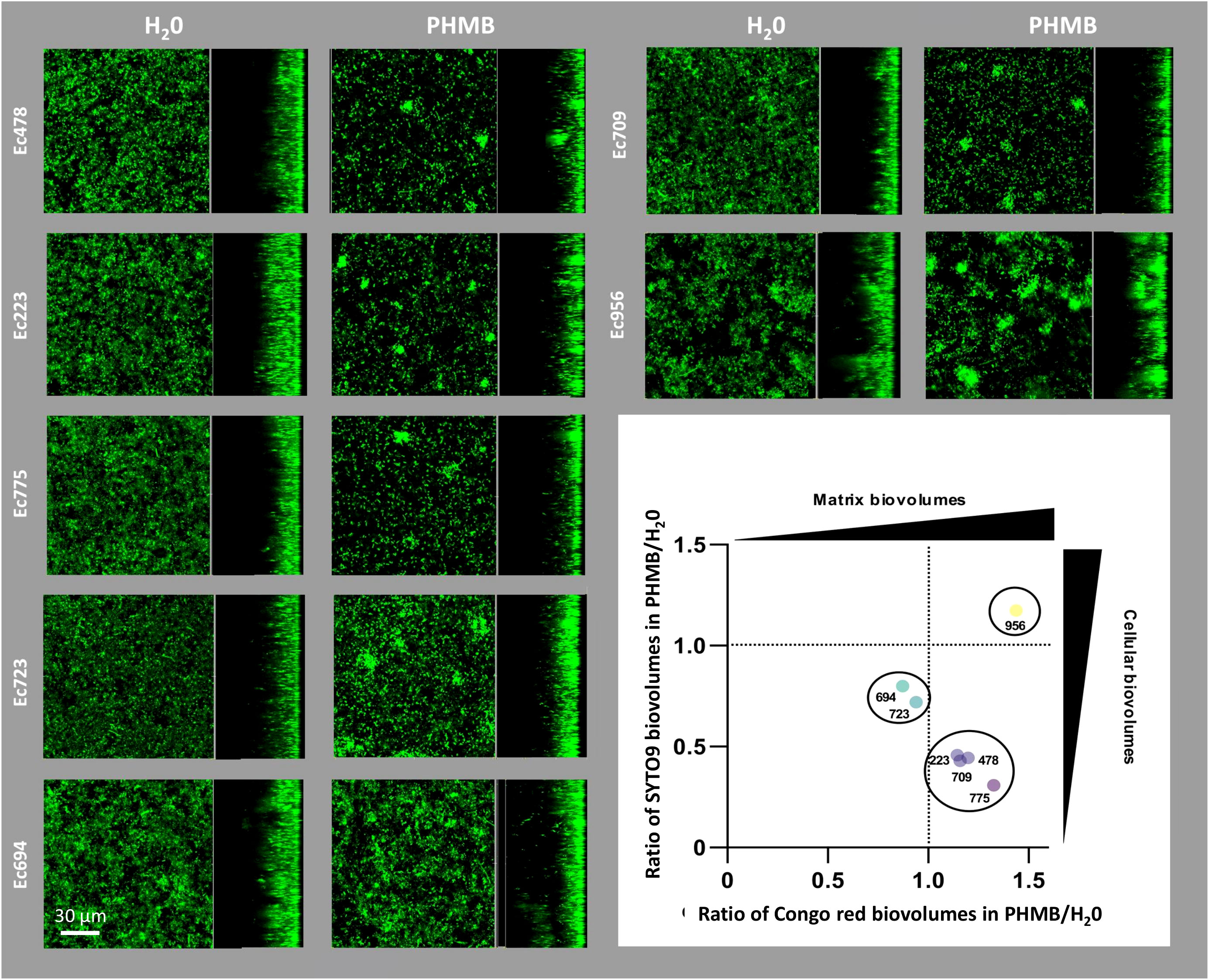
Figure 3 CLSM images of biofilms stained with SYTO™9 in presence or absence of sublethal concentrations of PHMB. On the bottom right, ratios between total cell biovolumes (SYTO™9, y-axis) and biofilm matrix (Congo red, x-axis) of biofilms exposed to PHMB or H2O.
4 Discussion
The escalating threat of antibiotic resistance challenges our understanding of bacterial adaptation mechanisms and the factors contributing to the emergence of resistant bacteria in our environments. Abundant evidence supported the central role of biofilm communities in bacterial adaptation to various antimicrobial substances (Uruén et al., 2020; Usui et al., 2023). However, the underlying mechanisms often remain ambiguous and are highly dependent on the molecules involved. In this contribution, we investigated the impacts of biocide exposures (BAC, TMN and PHMB) on aminoglycoside (Gen) and fluoroquinolone (Cip) resistance emergence in E. coli biofilms. Gen and Cip are indeed considered as crucial antibiotics used in the treatment of several gram-negative infections notably involving Enterobacteriaceae like E. coli (Krause et al., 2016; Zhu et al., 2020).
Experimental evolution experiments revealed here that PHMB exposure resulted in a recurrent increase of transient GenR emergence in the biofilms formed by most of the seven E. coli strains compared to H2O control. Only one strain, Ec709, showed a reduction in the quantity of variants in PHMB compared to H2O (especially due to a high number of GenR variants emerging in H2O). Such specific adaptive and mutability features of strain Ec709 would rely on its specific genetic background. Resistant variants appear naturally in bacterial populations and could be preferentially selected in Ec709 by the biofilm lifestyle itself rather than biocidal exposure. This can be related to particular chemical microenvironments in the three-dimensional structure of Ec709 biofilm that could participate in the induction of specific phenotypes and/or selection of genetic variants (Uruén et al., 2020; Charron et al., 2023). In addition, recent findings showed that hypermutator emergence rates are dependent of stress type in E. coli populations (Callens et al., 2023). It is therefore possible that in the case of Ec709, the presence of PHMB did not favor variant emergence and/or survival compared to H20 condition.
Conversely, the other biocides BAC and TMN led to a slight decrease of GenR emergence in most E. coli strains emphasizing the specificity of exposure effects depending on biocide molecules and their mode of action. Importantly, most variants that emerged under biocidal conditions did not retain the GenR phenotype after removal of antimicrobial stress, suggesting a transient adaptation rather than stable mutation-based resistance. Contrarily to Gen, no Cip variant was detected in any condition during the time of experiment. One assumption is that CipR variants potentially emerging in biofilms were outcompeted here due to a decrease of their ability to survive under biofilm conditions and biocidal exposure. Fluoroquinolones act by inhibiting DNA Gyrase and Topoisomerase IV, enzymes involved in chromosome replication and RNA transcription, and high-level resistance to fluoroquinolones in E. coli indeed often requires multiple mutations which are associated with a high fitness cost (Marcusson et al., 2009).
Genomic analysis of the 11 stable GenR variants revealed mutations predominantly affecting the cell respiration pathway and indistinctly selected in H2O or upon biocidal stress, showing unspecific evolutionary trajectories. Alteration of genes encoding components of the respiratory and electron transport chains was previously described to be associated with aminoglycoside resistance through the decrease of antibiotic uptake in E. coli and other gram-negative species including Klebsiella pneumoniae or Salmonella enterica for instance (Ibacache-Quiroga et al., 2018; Andersson et al., 2019). The cydA gene mutation was especially highly prevalent at the end of the experiment. Interestingly, most of the cydA variants had a second mutation, notably in aceE, arcB and fre. These genes are also linked to the cell respiration metabolism, ArcB being known as the main regulator of the cell anoxic respiration process and regulating several of the other mutated genes, like cydA (Brown et al., 2022) and atpG (Salmon et al., 2005). Moreover, aceE has already been associated with a Gen-PHMB cross-resistance mutation (Cuzin et al., 2021). Hence, associations of different mutations in this pathway could strengthen the resistance phenotype and compensate for potential fitness defects caused by the cydA mutation. The last cydA variant had no other mutated gene, but a mutation in an intergenic region, which could possibly play a role in the fitness of the bacteria. The only variant found in the last two weeks without a cydA mutation exhibited two mutations, one in fusA, which is also an already-known GenR mutation (Ibacache-Quiroga et al., 2018; Usui et al., 2023) and the other one in tsaB. The selection of similar mutations in the H2O control and upon biocidal exposure could result from the biofilm lifestyle itself, known to shape specifically adaptive trajectories due to the heterogeneity of microenvironments and associated stress in the three-dimensional structure (Bridier et al., 2017). In line with this, it was recently reported that E. coli biofilms upon aminoglycoside treatment favored specific resistance mutations otherwise counter-selected in planktonic cultures, illustrating such a specific biofilm-associated adaptive strategy (Usui et al., 2023).
Interestingly, we observed that PHMB promoted the emergence of transient GenR variants over subsequent weeks of exposure in most of the seven E. coli strains. This induction of transient variants upon PHMB exposure could rely on the activation of specific defensive responses to face biocide stress. Coherently, RT-qPCR gene expression analysis revealed the up-regulation of multiple genes linked to metabolic activity or membrane transport in response to PHMB in the first week of exposure, that could illustrate an attempt of bacteria to actively keep the biocide outside the cell. Previous researches reported the upregulation of efflux pumps in response to chlorhexidine digluconate (CHX), a biocide belonging, like PHMB, to the biguanide family (Morita et al., 2003; Fernández-Cuenca et al., 2015; Zhang et al., 2019; Tag ElDein et al., 2021). Interestingly, in another study performed on Acinetobacter baumannii, exposure to CHX reduced the resistance of one strain to Gen, while inducing several efflux pumps (Fernández-Cuenca et al., 2015). Here, efflux pumps (AcrA) were indeed slightly impacted by the PHMB pressure in the beginning of exposure. Additionally, general stress responses, like rpoS, and the SOS response through recA were globally overexpressed, affecting the expression of numerous genes playing a central role in the adaptation to suboptimal conditions and nucleic acid repair (Schellhorn, 2020). It has already been shown that PHMB can bind nucleic acids, which could explain why DNA repair systems are activated (Sowlati-Hashjin et al., 2020). Furthermore, the csgB gene involved in curli synthesis, a major amyloid proteinaceous component of extracellular matrix produced by many Enterobacteriaceae (Barnhart and Chapman, 2006), showed a prolonged overexpression in the presence of PHMB. Consequently, the denser biofilm matrix due to the overproduction of curli could thereby reinforce the biofilm structure and resistance by limiting PHMB diffusion. The role of curli in biofilm resistance to diverse molecules including biocides and antibiotics was indeed already shown in E. coli and other species (Ryu and Beuchat, 2005; Uhlich et al., 2006; Akbey and Andreasen, 2022). CLSM observations of biofilm here confirmed the overproduction of curli fibers after PHMB exposure illustrated by a modulation of the three-dimensional structure and the formation of dense clusters of bacteria associated with an increase in matrix biovolumes compared to H20 controls in most E. coli strains tested (Figure 3). Interestingly, previous studies have also linked specific subpopulations of cells, also organized into clusters, with increased expression of csgD, regulating curli production, and with the production of other matrix components, like cellulose (Grantcharova et al., 2010). The densification of biofilm matrix and reshaping of three-dimensional structure resulting from PHMB-induced curli overproduction could therefore potentially participate to the creation of specific microenvironments (here the clusters) promoting a higher tolerance to Gen notably through slow growth phenotypes (Uhlich et al., 2006; Yan and Bassler, 2019). This could be part of the origin of the increased frequencies of transient GenR variants upon PHMB exposure observed here for the various E. coli strains tested.
In this study, Gen variants emerging in biocide conditions mainly exhibited reversible resistance phenotypes. Nevertheless, recent studies suggest a potential link between bacterial tolerance and the facilitated emergence of stable genetic resistances toward antibiotics (Levin-Reisman et al., 2017; Levin-Reisman et al., 2019; Nordholt et al., 2021). An explanation if that tolerant bacteria, capable of surviving longer treatment periods, increase the likelihood of acquiring genetic resistance and often have elevated mutation rates due to the stress they undergo (Windels et al., 2019; Crane et al., 2021). Exposure to PHMB induced stress responses here, such as the SOS response mediated by recA and the general stress response involving rpoS. The SOS response, typically utilized by bacteria to repair damaged DNA, can lead to the emergence of antibiotic resistant variants due to the use of error-prone polymerases for instance (Crane et al., 2021). As PHMB globally induces physiological responses in biofilms that enhance survival against Gen in most of the E. coli strains tested here, it may thereby potentialize the emergence of mutants carrying stables genetic resistance during extended exposures, heightening the risk of antibiotic resistances dissemination and raising public health concerns. Such hypothesis should nevertheless be mitigated regarding the behavior of specific strain as Ec709 here, for which PHMB exposure rather results in a decrease of frequency of GenR emergence, underlining the strain-dependent nature of adaptive strategies.
5 Conclusion
We showed in this work that PHMB could indirectly stimulate the emergence of transient Gen resistance in E. coli biofilms. Bacterial response to PHMB in biofilms is characterized by the induction of stress responses and architectural modulations, notably through the production of extracellular curlis. These structural rearrangements resulted in the formation of clusters that may constitute specific microenvironments favoring the emergence of slow-growing bacteria with Gen tolerant phenotypes. Overall, this study provided valuable insights into the adaptive trajectories of biofilms exposed to biocidal stress and shed light on potential side effects on bacterial resistance to antibiotics. Further studies should be dedicated to deeply understanding collective biofilm adaptation to biocides and antimicrobial cross-resistance emergence.
Data availability statement
The datasets presented in this study can be found in online repositories. The names of the repository/repositories and accession number(s) can be found in the article/Supplementary Material.
Author contributions
RC: Conceptualization, Formal analysis, Investigation, Visualization, Writing – original draft, Writing – review & editing. PL: Data curation, Formal analysis, Investigation, Methodology, Software, Writing – original draft. AH: Investigation, Writing – review & editing. OM: Investigation, Writing – original draft. MB: Investigation, Writing – original draft. PH: Investigation, Writing – original draft. CS: Project administration, Resources, Writing – original draft. RB: Conceptualization, Funding acquisition, Supervision, Writing – review & editing, Writing – original draft. AB: Conceptualization, Funding acquisition, Project administration, Resources, Supervision, Visualization, Writing – review & editing, Writing – original draft.
Funding
The author(s) declare financial support was received for the research, authorship, and/or publication of this article. ANR JCJC BAoBAb (ANR-21-CE35-0001) financially supported this work. RC was also a recipient of an Anses-INRAE doctoral fellowship.
Acknowledgments
The authors express their gratitude to the French AntibioDEAL network of the Promise PPR antibioresistance ANR program for fostering valuable scientific exchanges.
Conflict of interest
The authors declare that the research was conducted in the absence of any commercial or financial relationships that could be construed as a potential conflict of interest.
The author(s) declared that they were an editorial board member of Frontiers, at the time of submission. This had no impact on the peer review process and the final decision.
Publisher’s note
All claims expressed in this article are solely those of the authors and do not necessarily represent those of their affiliated organizations, or those of the publisher, the editors and the reviewers. Any product that may be evaluated in this article, or claim that may be made by its manufacturer, is not guaranteed or endorsed by the publisher.
Supplementary material
The Supplementary Material for this article can be found online at: https://www.frontiersin.org/articles/10.3389/fcimb.2023.1324991/full#supplementary-material
References
Akbey, Ü., Andreasen, M. (2022). Functional amyloids from bacterial biofilms - structural properties and interaction partners. Chem. Sci. 13, 6457–6477. doi: 10.1039/d2sc00645f
Alkofide, H., Alhammad, A. M., Alruwaili, A., Aldemerdash, A., Almangour, T. A., Alsuwayegh, A., et al. (2020). Multidrug-resistant and extensively drug-resistant enterobacteriaceae: prevalence, treatments, and outcomes - A retrospective cohort study. Infect. Drug Resist. 13, 4653–4662. doi: 10.2147/IDR.S283488
Andersson, D. I., Nicoloff, H., Hjort, K. (2019). Mechanisms and clinical relevance of bacterial heteroresistance. Nat. Rev. Microbiol. 17, 479–496. doi: 10.1038/s41579-019-0218-1
Aslam, B., Khurshid, M., Arshad, M. I., Muzammil, S., Rasool, M., Yasmeen, N., et al. (2021). Antibiotic resistance: one health one world outlook. Front. Cell Infect. Microbiol. 11. doi: 10.3389/fcimb.2021.771510
Atamna-Mawassi, H., Huberman-Samuel, M., Hershcovitz, S., Karny-Epstein, N., Kola, A., Cortés, L. E. L., et al. (2021). Interventions to reduce infections caused by multidrug resistant Enterobacteriaceae (MDR-E): A systematic review and meta-analysis. J. Infect. 83, 156–166. doi: 10.1016/j.jinf.2021.05.005
Barnhart, M. M., Chapman, M. R. (2006). Curli biogenesis and function. Annu. Rev. Microbiol. 60, 131–147. doi: 10.1146/annurev.micro.60.080805.142106
Bodendoerfer, E., Marchesi, M., Imkamp, F., Courvalin, P., Böttger, E. C., Mancini, S. (2020). Co-occurrence of aminoglycoside and β-lactam resistance mechanisms in aminoglycoside- non-susceptible Escherichia coli isolated in the Zurich area, Switzerland. Int. J. Antimicrob. Agents 56, 106019. doi: 10.1016/j.ijantimicag.2020.106019
Bore, E., Hébraud, M., Chafsey, I., Chambon, C., Skjæret, C., Moen, B., et al. (2007). Adapted tolerance to benzalkonium chloride in Escherichia coli K-12 studied by transcriptome and proteome analyses. Microbiol. (Reading) 153, 935–946. doi: 10.1099/mic.0.29288-0
Bridier, A., Dubois-Brissonnet, F., Boubetra, A., Thomas, V., Briandet, R. (2010). The biofilm architecture of sixty opportunistic pathogens deciphered by a high-throughput CLSM method. J. Microbiol. Methods 82, 64–70. doi: 10.1016/j.mimet.2010.04.006
Bridier, A., Dubois-Brissonnet, F., Greub, G., Thomas, V., Briandet, R. (2011). Dynamics of the action of biocides in Pseudomonas aeruginosa biofilms. Antimicrob. Agents Chemother. 55, 2648–2654. doi: 10.1128/AAC.01760-10
Bridier, A., Piard, J.-C., Pandin, C., Labarthe, S., Dubois-Brissonnet, F., Briandet, R. (2017). Spatial organization plasticity as an adaptive driver of surface microbial communities. Front. Microbiol. 8. doi: 10.3389/fmicb.2017.01364
Brown, A. N., Anderson, M. T., Bachman, M. A., Mobley, H. L. T. (2022). The arcAB two-component system: function in metabolism, redox control, and infection. Microbiol. Mol. Biol. Rev. 86, e0011021. doi: 10.1128/mmbr.00110-21
Callens, M., Rose, C. J., Finnegan, M., Gatchitch, F., Simon, L., Hamet, J., et al. (2023). Hypermutator emergence in experimental Escherichia coli populations is stress-type dependent. Evol. Lett. 7, 252–261. doi: 10.1093/evlett/qrad019
Charron, R., Boulanger, M., Briandet, R., Bridier, A. (2023). Biofilms as protective cocoons against biocides: from bacterial adaptation to One Health issues. Microbiol. (Reading) 169. doi: 10.1099/mic.0.001340
Chuanchuen, R., Beinlich, K., Hoang, T. T., Becher, A., Karkhoff-Schweizer, R. R. (2001). and schweizer, H Cross-resistance between triclosan and antibiotics in Pseudomonas aeruginosa is mediated by multidrug efflux pumps: exposure of a susceptible mutant strain to triclosan selects nfxB mutants overexpressing MexCD-OprJ. P. Antimicrob. Agents Chemother. 45, 428–432. doi: 10.1128/AAC.45.2.428-432.2001
Crane, J. K., Alvarado, C. L., Sutton, M. D. (2021). Role of the SOS response in the generation of antibiotic resistance in vivo. Antimicrob. Agents Chemother. 65, e0001321. doi: 10.1128/AAC.00013-21
Cuzin, C., Houée, P., Lucas, P., Blanchard, Y., Soumet, C., Bridier, A. (2021). Selection of a gentamicin-resistant variant following polyhexamethylene biguanide (PHMB) exposure in escherichia coli biofilms. Antibiotics (Basel) 10, 553. doi: 10.3390/antibiotics10050553
D’Aquila, P., De Rango, F., Paparazzo, E., Passarino, G., Bellizzi, D. (2023). Epigenetic-based regulation of transcriptome in escherichia coli adaptive antibiotic resistance. Microbiol. Spectr. 11, e0458322. doi: 10.1128/spectrum.04583-22
Davies, J., Davies, D. (2010). Origins and evolution of antibiotic resistance. Microbiol. Mol. Biol. Rev. 74, 417–433. doi: 10.1128/MMBR.00016-10
Davison, W. M., Pitts, B., Stewart, P. S. (2010). Spatial and temporal patterns of biocide action against Staphylococcus epidermidis biofilms. Antimicrob. Agents Chemother. 54, 2920–2927. doi: 10.1128/AAC.01734-09
Douarre, P.-E., Sévellec, Y., Le Grandois, P., Soumet, C., Bridier, A., Roussel, S. (2022). FepR as a central genetic target in the adaptation to quaternary ammonium compounds and cross-resistance to ciprofloxacin in listeria monocytogenes. Front. Microbiol. 13. doi: 10.3389/fmicb.2022.864576
Fernández, L., Breidenstein, E. B. M., Hancock, R. E. W. (2011). Creeping baselines and adaptive resistance to antibiotics. Drug Resist. Update 14, 1–21. doi: 10.1016/j.drup.2011.01.001
Fernández, L., Hancock, R. E. W. (2012). Adaptive and mutational resistance: role of porins and efflux pumps in drug resistance. Clin. Microbiol. Rev. 25, 661–681. doi: 10.1128/CMR.00043-12
Fernández-Cuenca, F., Tomás, M., Caballero-Moyano, F.-J., Bou, G., Martínez-Martínez, L., Vila, J., et al. (2015). Reduced susceptibility to biocides in Acinetobacter baumannii: association with resistance to antimicrobials, epidemiological behaviour, biological cost and effect on the expression of genes encoding porins and efflux pumps. J. Antimicrob. Chemother. 70, 3222–3229. doi: 10.1093/jac/dkv262
Flemming, H.-C., van Hullebusch, E. D., Neu, T. R., Nielsen, P. H., Seviour, T., Stoodley, P., et al. (2023). The biofilm matrix: multitasking in a shared space. Nat. Rev. Microbiol. 21, 70–86. doi: 10.1038/s41579-022-00791-0
Grantcharova, N., Peters, V., Monteiro, C., Zakikhany, K., Römling, U. (2010). Bistable expression of CsgD in biofilm development of Salmonella enterica serovar typhimurium. J. Bacteriol 192, 456–466. doi: 10.1128/JB.01826-08
Guérin, A., Bridier, A., Le Grandois, P., Sévellec, Y., Palma, F., Félix, B., et al. (2021). Exposure to quaternary ammonium compounds selects resistance to ciprofloxacin in listeria monocytogenes. Pathogens 10, 220. doi: 10.3390/pathogens10020220
Hartmann, R., Jeckel, H., Jelli, E., Singh, P. K., Vaidya, S., Bayer, M., et al. (2021). Quantitative image analysis of microbial communities with BiofilmQ. Nat. Microbiol. 6, 151–156. doi: 10.1038/s41564-020-00817-4
Ibacache-Quiroga, C., Oliveros, J. C., Couce, A., Blázquez, J. (2018). Parallel evolution of high-level aminoglycoside resistance in escherichia coli under low and high mutation supply rates. Front. Microbiol. 9. doi: 10.3389/fmicb.2018.00427
Iovleva, A., Doi, Y. (2017). Carbapenem-resistant enterobacteriaceae. Clin. Lab. Med. 37, 303–315. doi: 10.1016/j.cll.2017.01.005
Jo, J., Price-Whelan, A., Dietrich, L. E. P. (2022). Gradients and consequences of heterogeneity in biofilms. Nat. Rev. Microbiol. 20, 593–607. doi: 10.1038/s41579-022-00692-2
Karatzas, K. A. G., Webber, M. A., Jorgensen, F., Woodward, M. J., Piddock, L. J. V., Humphrey, T. J. (2007). Prolonged treatment of Salmonella enterica serovar Typhimurium with commercial disinfectants selects for multiple antibiotic resistance, increased efflux and reduced invasiveness. J. Antimicrob. Chemother. 60, 947–955. doi: 10.1093/jac/dkm314
Karygianni, L., Ren, Z., Koo, H., Thurnheer, T. (2020). Biofilm matrixome: extracellular components in structured microbial communities. Trends Microbiol. 28, 668–681. doi: 10.1016/j.tim.2020.03.016
Kim, M., Weigand, M. R., Oh, S., Hatt, J. K., Krishnan, R., Tezel, U., et al. (2018). Widely used benzalkonium chloride disinfectants can promote antibiotic resistance. Appl. Environ. Microbiol. 84, e01201–e01218. doi: 10.1128/AEM.01201-18
Krause, K. M., Serio, A. W., Kane, T. R., Connolly, L. E. (2016). Aminoglycosides: an overview. Cold Spring Harb. Perspect. Med. 6, a027029. doi: 10.1101/cshperspect.a027029
Levin-Reisman, I., Brauner, A., Ronin, I., Balaban, N. Q. (2019). Epistasis between antibiotic tolerance, persistence, and resistance mutations. Proc. Natl. Acad. Sci. U.S.A. 116, 14734–14739. doi: 10.1073/pnas.1906169116
Levin-Reisman, I., Ronin, I., Gefen, O., Braniss, I., Shoresh, N., Balaban, N. Q. (2017). Antibiotic tolerance facilitates the evolution of resistance. Science 355, 826–830. doi: 10.1126/science.aaj2191
Lu, J., Jin, M., Nguyen, S. H., Mao, L., Li, J., Coin, L. J. M., et al. (2018). Non-antibiotic antimicrobial triclosan induces multiple antibiotic resistance through genetic mutation. Environ. Int. 118, 257–265. doi: 10.1016/j.envint.2018.06.004
Maillard, J. Y. (2018). Resistance of bacteria to biocides. Microbiol. Spectr. 6, 6.2.19. doi: 10.1128/microbiolspec.ARBA-0006-2017
Marcusson, L., Frimodt-Moller, N., Hughes, D. (2009). Interplay in the selection of fluoroquinolone resistance and bacterial fitness. PloS Pathog. 5,8, e1000541. doi: 10.1371/journal.ppat.1000541
Morita, Y., Murata, T., Mima, T., Shiota, S., Kuroda, T., Mizushima, T., et al. (2003). Induction of mexCD-oprJ operon for a multidrug efflux pump by disinfectants in wild-type Pseudomonas aeruginosa PAO1. J. Antimicrob. Chemother. 51, 991–994. doi: 10.1093/jac/dkg173
Nordholt, N., Kanaris, O., Schmidt, S. B. I., Schreiber, F. (2021). Persistence against benzalkonium chloride promotes rapid evolution of tolerance during periodic disinfection. Nat. Commun. 12. doi: 10.1038/s41467-021-27019-8
Reale, O., Huguet, A., Fessard, V. (2019). Novel insights on the toxicity of phycotoxins on the gut through the targeting of enteric glial cells. Mar. Drugs 17, 429. doi: 10.3390/md17070429
Römling, U. (2023). Is biofilm formation intrinsic to the origin of life? Environ. Microbiol. 25, 26–39. doi: 10.1111/1462-2920.16179
Ryu, J. H., Beuchat, L. R. (2005). Biofilm formation by Escherichia coli O157:H7 on stainless steel: effect of exopolysaccharide and Curli production on its resistance to chlorine. Appl. Environ. Microbiol. 71, 247–254. doi: 10.1128/AEM.71.1.247-254.2005
Salmon, K. A., Hung, S., Steffen, N. R., Krupp, R., Baldi, P., Hatfield, G. W., et al. (2005). Global gene expression profiling in Escherichia coli K12: effects of oxygen availability and ArcA. J. Biol. Chem. 280, 15084–15096. doi: 10.1074/jbc.M414030200
Sanchez, P., Moreno, E., Martinez, J. L. (2005). The biocide triclosan selects Stenotrophomonas maltophilia mutants that overproduce the SmeDEF multidrug efflux pump. Antimicrob. Agents Chemother. 49, 781–782. doi: 10.1128/AAC.49.2.781-782.2005
Schellhorn, H. E. (2020). Function, evolution, and composition of the rpoS regulon in escherichia coli. Front. Microbiol. 11. doi: 10.3389/fmicb.2020.560099
Sindeldecker, D., Stoodley, P. (2021). The many antibiotic resistance and tolerance strategies of Pseudomonas aeruginosa. Biofilm 3, 100056. doi: 10.1016/j.bioflm.2021.100056
Sonbol, F. I., El-Banna, T. E., Abd El-Aziz, A. A., El-Ekhnawy, E. (2019). Impact of triclosan adaptation on membrane properties, efflux and antimicrobial resistance of Escherichia coli clinical isolates. J. Appl. Microbiol. 126, 730–739. doi: 10.1111/jam.14158
Soumet, C., Méheust, D., Pissavin, C., Le Grandois, P., Frémaux, B., Feurer, C., et al. (2016). Reduced susceptibilities to biocides and resistance to antibiotics in food-associated bacteria following exposure to quaternary ammonium compounds. J. Appl. Microbiol. 121, 1275–1281. doi: 10.1111/jam.13247
Sowlati-Hashjin, S., Carbone, P., Karttunen, M. (2020). Insights into the polyhexamethylene biguanide (PHMB) mechanism of action on bacterial membrane and DNA: A molecular dynamics study. J. Phys. Chem. B 124, 4487–4497. doi: 10.1021/acs.jpcb.0c02609
Tag ElDein, M. A., Yassin, A. S., El-Tayeb, O., Kashef, M. T. (2021). Chlorhexidine leads to the evolution of antibiotic-resistant Pseudomonas aeruginosa. Eur. J. Clin. Microbiol. Infect. Dis. 40, 2349–2361. doi: 10.1007/s10096-021-04292-5
Tkachenko, O., Shepard, J., Aris, V. M., Joy, A., Bello, A., Londono, I., et al. (2007). A triclosan-ciprofloxacin cross-resistant mutant strain of Staphylococcus aureus displays an alteration in the expression of several cell membrane structural and functional genes. Res. Microbiol. 158, 651–658. doi: 10.1016/j.resmic.2007.09.003
Uhlich, G. A., Cooke, P. H., Solomon, E. B. (2006). Analyses of the red-dry-rough phenotype of an Escherichia coli O157:H7 strain and its role in biofilm formation and resistance to antibacterial agents. Appl. Environ. Microbiol. 72, 2564–2572. doi: 10.1128/AEM.72.4.2564-2572.2006
Uruén, C., Chopo-Escuin, G., Tommassen, J., Mainar-Jaime, R. C., Arenas, J. (2020). Biofilms as promoters of bacterial antibiotic resistance and tolerance. Antibiotics (Basel) 10, 3. doi: 10.3390/antibiotics10010003
Usui, M., Yoshii, Y., Thiriet-Rupert, S., Ghigo, J.-M., Beloin, C. (2023). Intermittent antibiotic treatment of bacterial biofilms favors the rapid evolution of resistance. Commun. Biol. 6, 275. doi: 10.1038/s42003-023-04601-y
Windels, E. M., Michiels, J. E., Fauvart, M., Wenseleers, T., Van den Bergh, B., Michiels, J. (2019). Bacterial persistence promotes the evolution of antibiotic resistance by increasing survival and mutation rates. ISME J. 13, 1239–1251. doi: 10.1038/s41396-019-0344-9
World Health Organization. (2019). Critically important antimicrobials for human medicine (Geneva: World Health Organization). Available at: https://iris.who.int/handle/10665/312266 (Accessed November 10, 2023).
Yan, J., Bassler, B. L. (2019). Surviving as a community: antibiotic tolerance and persistence in bacterial biofilms. Cell Host Microbe 26, 15–21. doi: 10.1016/j.chom.2019.06.002
Zhang, Y., Zhao, Y., Xu, C., Zhang, X., Li, J., Dong, G., et al. (2019). Chlorhexidine exposure of clinical Klebsiella pneumoniae strains leads to acquired resistance to this disinfectant and to colistin. Int. J. Antimicrob. Agents 53, 864–867. doi: 10.1016/j.ijantimicag.2019.02.012
Keywords: biofilm, biocide, antibiotic resistance, aminoglycoside, biguanide, bacterial adaptation, tolerance, experimental evolution
Citation: Charron R, Lemée P, Huguet A, Minlong O, Boulanger M, Houée P, Soumet C, Briandet R and Bridier A (2023) Polyhexamethylene biguanide promotes adaptive cross-resistance to gentamicin in Escherichia coli biofilms. Front. Cell. Infect. Microbiol. 13:1324991. doi: 10.3389/fcimb.2023.1324991
Received: 20 October 2023; Accepted: 16 November 2023;
Published: 11 December 2023.
Edited by:
Adline Princy Solomon, SASTRA University, IndiaReviewed by:
Tahereh Navidifar, Shoushtar Faculty of Medical Sciences, IranJordy Evan Sulaiman, University of Wisconsin-Madison, United States
Copyright © 2023 Charron, Lemée, Huguet, Minlong, Boulanger, Houée, Soumet, Briandet and Bridier. This is an open-access article distributed under the terms of the Creative Commons Attribution License (CC BY). The use, distribution or reproduction in other forums is permitted, provided the original author(s) and the copyright owner(s) are credited and that the original publication in this journal is cited, in accordance with accepted academic practice. No use, distribution or reproduction is permitted which does not comply with these terms.
*Correspondence: Arnaud Bridier, arnaud.bridier@anses.fr