- 1Department of Pathology, University of Texas Medical Branch, Galveston, TX, United States
- 2Department of Microbiology and Immunology, University of Texas Medical Branch, Galveston, TX, United States
- 3Center for Biodefense and Emerging Infectious Diseases, University of Texas Medical Branch, Galveston, TX, United States
- 4Sealy Institute for Vaccine Sciences, University of Texas Medical Branch, Galveston, TX, United States
- 5Institute for Human Infections and Immunity, University of Texas Medical Branch, Galveston, TX, United States
For decades, the defined antibody reactive proteins of Ehrlichia chaffeensis and E. canis were limited to a small group with linear antibody epitopes. Recently, our laboratory has utilized an immunomics-based approach to rapidly screen and identify undefined Ehrlichia chaffeensis and E. canis antigenic proteins and antibody epitopes. In this study, we analyzed the remaining portion (~50%) of the E. chaffeensis and E. canis proteomes (n = 444 and n = 405 proteins, respectively), that were not examined in previous studies, to define the complete immunomes of these important pathogens. Almost half of the E. chaffeensis proteins screened (196/444) reacted with antibodies in convalescent HME patient sera, while only 43 E. canis proteins reacted with CME dog sera. New major immunoreactive proteins were identified in E. chaffeensis (n = 7) and E. canis (n = 1), increasing the total number of E. chaffeensis (n = 14) and E. canis proteins (n = 18) that exhibited antibody reactivity comparable to well-defined major antigenic proteins (TRP120 and TRP19). All of the E. chaffeensis but only some E. canis major immunoreactive proteins contained major conformation-dependent antibody epitopes. The E. chaffeensis immunoreactive proteins were generally small (< 250 amino acids; ~27kDa) and the E. canis proteins were slightly larger (> 320 amino acids; ~35 kDa). The majority of these new Ehrlichia major immunoreactive proteins were predicted to be type I secreted effectors, some of which contained transmembrane domains. Characterization of the immunomes of E. chaffeensis and E. canis and understanding the host specific Ehrlichia immune responses will facilitate identification of protective antigens and define the biophysical epitope characteristics vital to effective vaccine development for the ehrlichioses.
Introduction
Ehrlichia spp. are tick-transmitted, obligate intracellular bacteria that cause disease in animals and humans, ranging from mild to severe and life-threatening (Paddock and Childs, 2003; McBride and Walker, 2011). E. chaffeensis is the etiologic agent of human monocytotropic ehrlichiosis (HME), an emerging zoonosis. E. canis is the etiologic agent of canine monocytic ehrlichiosis (CME), a highly prevalent globally distributed hemorrhagic disease in dogs (Paddock and Childs, 2003; Harrus and Waner, 2011).
Conventional immunoblotting approaches have helped identify major antibody reactive proteins from E. chaffeensis and E. canis, including major outer membrane proteins (OMPs) (Ohashi et al., 1998a; Ohashi et al., 1998b), tandem repeat proteins (TRPs) (Doyle et al., 2006; McBride et al., 2007; Luo et al., 2008; Luo et al., 2009; McBride et al., 2011) and ankyrin repeat proteins (Anks) (Nethery et al., 2007; Luo et al., 2010), that contain linear antibody epitopes. Many of these proteins elicit protective immune responses in Ehrlichia infection models (Li et al., 2001; Crocquet-Valdes et al., 2011; Kuriakose et al., 2012; Nambooppha et al., 2022). Moreover, experimental studies have demonstrated protection against infection using live-attenuated vaccines and subunit vaccines (Rudoler et al., 2012; McGill et al., 2016; Budachetri et al., 2022); however, there are no commercial human or veterinary vaccines available for HME or CME.
Until recently, the number of defined E. chaffeensis and E. canis antigenic proteins has been limited (McBride and Walker, 2010), compared to a large number of antigenic proteins (~7-20% of the proteome) identified in other pathogens, such as Chlamydia, Coxiella, Burkholderia and Bartonella (Barbour et al., 2008; Felgner et al., 2009; Vigil et al., 2010a; Vigil et al., 2010b; Cruz-Fisher et al., 2011; Vigil et al., 2011). Since the completion of whole-genome sequencing of the first bacterium Haemophilus influenzae in 1995, a variety of multiomics approaches are now available, including genomics, proteomics, transcriptomics, and immunomics which integrates these omics approaches to study the immunome (Sette et al., 2005; Loman and Pallen, 2015; De Sousa and Doolan, 2016; Babu and Snyder, 2023). The immunome is defined as the set of antigens or epitopes that interface with the host immune system. Genome-based in silico reverse vaccinology has also accelerated the identification of new vaccine candidates, but it largely relies on the accuracy of a serial of computational prediction tools, which are limited by the validated protective antigens in which the algorithm is based (Bidmos et al., 2018).
Intracellular bacteria E. chaffeensis and E. canis have relatively small genomes (1.2 Mbp and 1.3Mbp, respectively) that encode less than 1000 proteins (Dunning Hotopp et al., 2006; Mavromatis et al., 2006). In recent years, access to commercial gene synthesis and cloning has made experimental screening of antigenic proteins feasible. Therefore, we established a high-throughput immunomics-based antigen discovery approach to rapidly identify undiscovered antigenic proteins from E. chaffeensis and E. canis (Luo et al., 2020; Luo et al., 2021). Since previous investigations suggested that hypothetical proteins were potential antigens, our initial studies prioritized hypothetical proteins and a group of annotated proteins with high antigenicity scores predicted by ANTIGENpro, a sequence-based predictor of protein antigenicity (Magnan et al., 2010). About half of the proteins in the E. chaffeensis and E. canis proteome have been screened, and many new immunoreactive ehrlichial proteins were identified (Luo et al., 2020; Luo et al., 2021). Most of the recently discovered Ehrlichia immunoreactive proteins were predicted to be secreted effector proteins with antibody epitopes that exhibit complete or partial conformation dependence (Luo et al., 2020; Luo et al., 2021).
In this study, we used our established immunomics-based strategy to screen the remaining proteins (~50%) in the E. chaffeensis and E. canis proteomes. This comprehensive screening provides a detailed analysis of the antibody-reactive immunomes of E. chaffeensis and E. canis 30 years after E. chaffeensis GroEL was identified as the first antibody-reactive protein in 1993 (Sumner et al., 1993). The comprehensive identification and analysis of these Ehrlichia spp. immunomes reported herein will accelerate diagnostic, vaccine, and immunotherapeutic development for human and canine ehrlichiosis.
Materials and methods
Gene synthesis and cloning
E. chaffeensis (Arkansas strain) and E. canis (Jake strain) gene sequences are available in the Integrated Microbial Genomes (IMG) (https://img.jgi.doe.gov/) (Chen et al., 2019) and GenBank (https://www.ncbi.nlm.nih.gov/genbank). Ehrlichia genes were codon-optimized, chemically synthesized and cloned into pIVEX2.3d vector (containing a 6×His-tag sequence) by Twist Bioscience (San Francisco, CA) or GenScript (Piscataway, NJ). Plasmids were transformed into Escherichia coli to amplify, then extracted and lyophilized by the manufacturer.
In vitro transcription and translation (IVTT)
In vitro expression of Ehrlichia proteins was performed using the NEBExpress cell-free E. coli protein synthesis system (New England Biolabs, Ipswich, MA). Lyophilized plasmids were reconstituted in water and purified using the UltraClean 96 PCR cleanup kit (Qiagen, Germantown, MD). Plasmids were then added to E. coli extract and a reaction premix in a 96-well plate and incubated at 37°C for 3 h with orbital shaking (300 rpm) according to the manufacturer’s instructions.
HME and CME antisera
HME patient sera were kind gifts from the Centers for Disease Control and Prevention (Atlanta, GA), Vanderbilt University School of Medicine (Nashville, TN), Washington University and the St. Louis Children’s Hospital (St. Louis, MO). CME sera were obtained from naturally infected dogs from the United States and Colombia. All sera were confirmed to be positive against E. chaffeensis or E. canis by both indirect fluorescent-antibody assay (IFA) and enzyme-linked immunosorbent assay (ELISA). To avoid reactions with non-specific polyreactive IgM antibodies, which have been previously described in humans (Jones et al., 2012), assays were performed with convalescent sera and bound antibody detected with anti-IgG (H+L) secondary antibodies.
Dot immunoblot
The expression of Ehrlichia proteins by IVTT was confirmed by dot immunoblot with horseradish peroxidase (HRP)-labeled mouse anti-His tag monoclonal antibody (1:500; GenScript) as described previously (Luo et al., 2020). The immunoreactivity of native and denatured proteins was also examined by dot immunoblot using IVTT-expressed proteins purified by MagneHis protein purification system (Promega, Madison, WI) according to the manufacturer. Immunoblots were probed with either HME or CME serum (1:200) and developed with TMB 1-component substrate (Kirkegaard & Perry Laboratories, Gaithersburg, MD).
ELISA immunoscreening
The immunoreactivity of Ehrlichia IVTT-expressed proteins was performed by capturing His-tagged IVTT proteins on an ELISA plate coated with anti-His tag antibody as previously described with minor modifications (Luo et al., 2020). ELISA was performed with HME or CME sera (1:200) and bound antibody detected with alkaline phosphatase-labeled rabbit anti-human IgG (H+L) (1:7,000; Abcam, Cambridge, MA) or anti-dog IgG (H+L) secondary antibodies (1:5000) and BluePhos substrate (Kirkegaard & Perry Laboratories). Dilution buffer containing 4 M urea was used to denature IVTT-expressed proteins and the diluted protein was incubated for 10 min at 99°C before cooling on ice and coating the plate (or membrane for dot blot). Optical density was measured at 650 nm (OD650) on a SpectraMax iD5 plate reader (Molecular Devices, Sunnyvale, CA) and OD650 values represent the mean reading from 3 wells (± standard deviation) after negative control (IVTT negative protein control and a normal human or canine serum control) background subtraction. A sample ELISA OD650 value of ≥ 0.2 was considered positive and ≥ 0.5 a strong positive after subtracting the negative control OD650 reading (background). The proteins with mean ELISA OD650 of > 1.0 from multiple sera were considered immunodominant and proteins with mean ELISA OD650 of 0.5~1.0 subdominant.
Peptide ELISA
To identify linear antibody epitopes, ELISAs were performed using overlapping peptides (17-23 amino acids; 6 amino acid overlap) (Luo et al., 2009). All peptides were commercially synthesized and supplied as a lyophilized powder (GenScript) and resuspended in molecular biology grade water (1 mg/ml). A small amount of NH4OH, acetic acid or dimethyl sulfone was added to help dissolve some acidic, basic, or hydrophobic peptides, respectively, according to peptide solubility guidelines from the manufacturer.
IFA
The antibody titers in sera from HME patients and CME dogs were determined by IFA as previously described (Luo et al., 2020). Antigen slides were prepared from E. chaffeensis (Arkansas)-infected THP-1 cells or E. canis (Jake)-infected DH82 cells. Slides were examined with a BX61 epifluorescence microscope (Olympus, Japan).
Bioinformatic analysis
Online bioinformatic prediction tools used in this study include ANTIGENpro (http://scratch.proteomics.ics.uci.edu), TMHMM 2.0 (https://services.healthtech.dtu.dk/service.php?TMHMM-2.0), SignalP 6.0 (https://services.healthtech.dtu.dk/service.php?SignalP-6.0), SecretomeP 2.0 (https://services.healthtech.dtu.dk/service.php?SecretomeP-2.0), S4TE 2.0 (https://sate.cirad.fr), and PREFFECTOR (http://draco.cs.wpi.edu/preffector).
Results
E. chaffeensis and E. canis immunomics-based screening
Previously, we analyzed the predicted E. chaffeensis (Arkansas strain) and E. canis (Jake strain) ORFs in both databases of Integrated Microbial Genomes (IMG) and GenBank. After RNA genes, pseudogenes and short ORFs (coded proteins < 42 aa) were excluded, the total number of ORFs in E. chaffeensis and E. canis genome was determined to be 882 and 928, respectively (Luo et al., 2021). The predicted antigenicity of all proteins was determined using ANTIGENpro and respective antigenicity scores obtained (between 0 and 1). We previously investigated the immunoreactivity of all proteins distributed in the top 350 (with antigenicity scores > ~0.6), excluding previously characterized antigens (such as TRPs and OMPs). In addition, we also previously prioritized hypothetical proteins (including proteins with domain of unknown function [DUF]) regardless of ANTIGENpro rank (Luo et al., 2020; Luo et al., 2021) (Supplementary Figure 1).
To define the complete antigenic repertoire of E. chaffeensis and E. canis, in this study we further investigated the remaining proteins (n = 444 and n = 405, respectively) that were not examined in our previous studies. Proteins were expressed in the cell-free IVTT system, and the expression was confirmed by dot blot of randomly selected proteins (n = 22) from both E. chaffeensis and E. canis (Figure 1A). Since ELISA plate wells can be saturated by IVTT-expressed proteins, the differences in expression levels did not influence the relative immunoreactivity between different proteins as established in our previous investigations (Luo et al., 2020; Luo et al., 2021).
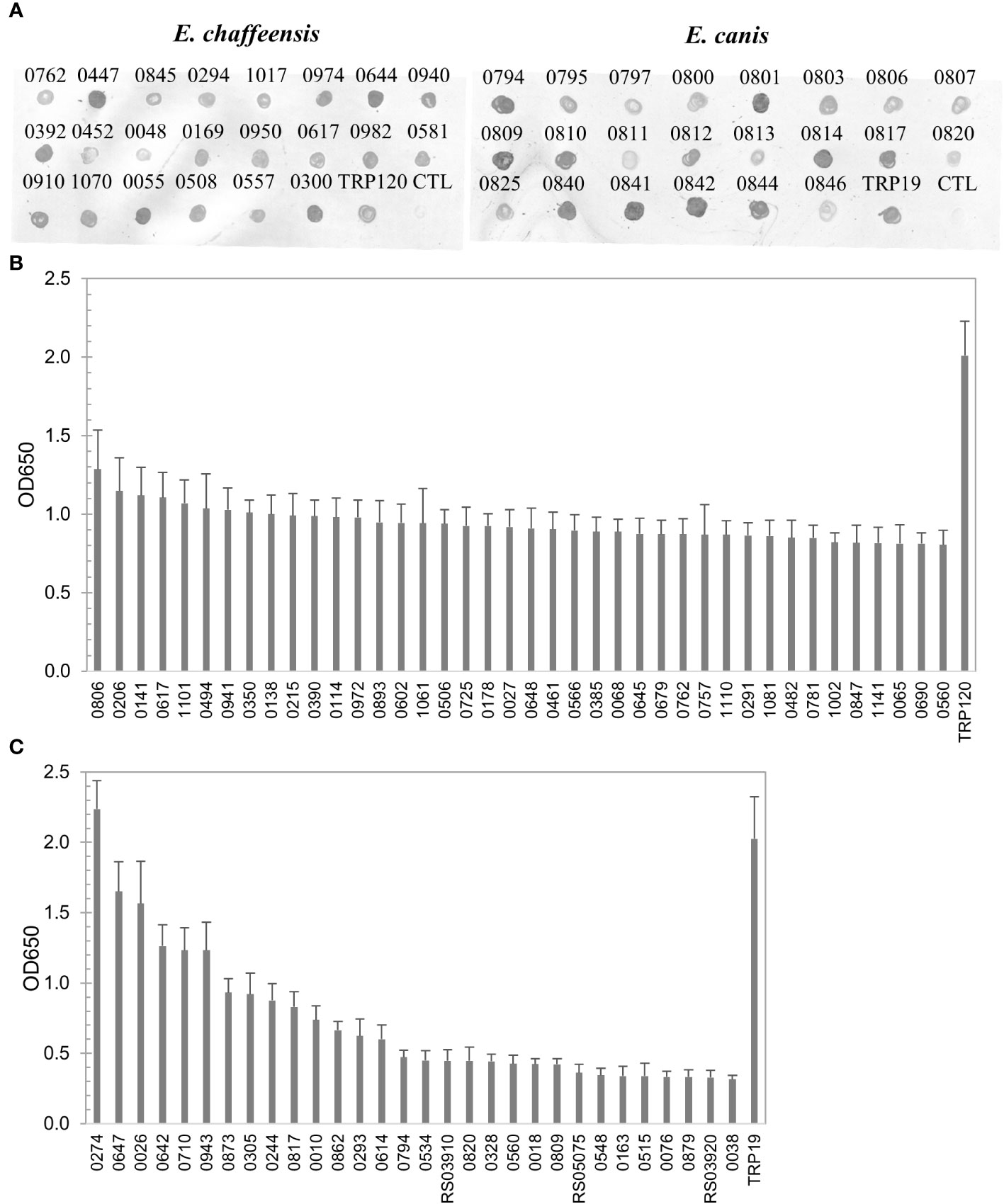
Figure 1 Expression of E. chaffeensis and E. canis proteins by IVTT and immunoreactivity screening by ELISA. (A) Detection of IVTT expression of selected proteins of E. chaffeensis and E. canis by dot immunoblot with anti-His-tag antibody. CTL, the negative control (IVTT reaction without plasmid template). Pooled sera of HME patients or CME dogs were used to screen E. chaffeensis (B) and E. canis (C) proteins, respectively. ELISA OD values represent the mean optical density reading from 3 wells (± standard deviation) after background subtraction. A sample OD of ≥0.2 was considered positive and ≥0.5 a strong positive after subtracting negative control (an IVTT reaction with empty plasmid template and a normal human or canine serum control) readings. TRP120 (B) and TRP19 (C) were used as positive controls.
The E. chaffeensis and E. canis proteins (n = 444 and n=405, respectively) were screened for immunoreactivity by ELISA using pooled convalescent HME or CME sera, respectively, which had indirect fluorescent-antibody assay (IFA) titers of 1600. A total of 196 (44%) E. chaffeensis and 43 (11%) E. canis proteins reacted with pooled sera (mean optical density at 650 nm [OD650] ≥ 0.2 with background subtracted). All E. chaffeensis and E. canis proteins were ranked according to ELISA OD value (from high to low) and are listed in Supplementary Tables 1, 2, respectively. The E. chaffeensis (n = 40; mean OD650 > 0.8) and E. canis proteins (n = 31; mean OD650 > 0.3) that exhibited the strongest immunoreactivity with pooled sera are shown in Figures 1B, C.
Identification of E. chaffeensis and E. canis immunodominant proteins
To further define and compare the antibody reactivity of these E. chaffeensis and E. canis proteins by ELISA, a panel of 8 HME and 8 CME sera were used. All patient and canine sera recognized E. chaffeensis or E. canis by IFA, respectively, with antibody titers ranging from 200 to 3200 (Figure 2). As previously described, well-defined immunodominant proteins (E. chaffeensis TRP120 or E. canis TRP19) were used as positive controls, respectively (Cardenas et al., 2007; Luo et al., 2010; Pritt and Dumler, 2019; Taques et al., 2020).
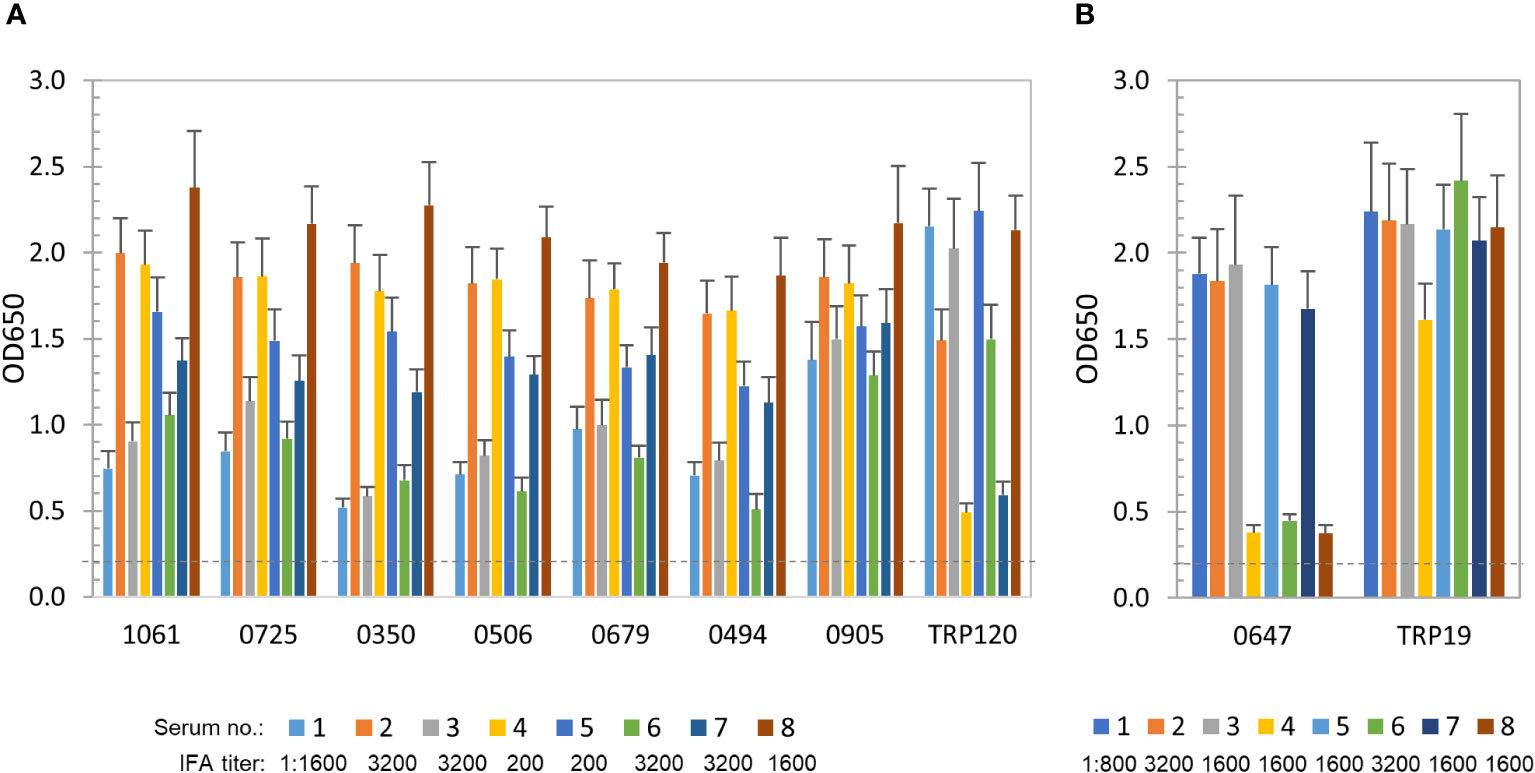
Figure 2 Immunoreactivity of new E. chaffeensis and E. canis immunodominant proteins. (A) Immunoreactivity comparison of 7 E. chaffeensis immunodominant proteins and TRP120 by ELISA. IVTT-expressed proteins were probed with a panel of convalescent sera from 8 HME patients. (B) Immunoreactivity comparison of E. canis immunodominant protein Ecaj_0647 and TRP19 by ELISA. IVTT-expressed proteins were probed with a panel of convalescent sera from 8 CME dogs. OD650 values represent the mean optical density reading from 3 wells (± standard deviation) after background subtraction. A sample OD650 of ≥ 0.2 was considered positive and ≥ 0.5 a strong positive after subtracting negative control (an IVTT reaction with empty plasmid template and a normal human or canine serum control) readings.
E. chaffeensis immunoreactive proteins (n = 56) were recognized by all or most of 8 HME sera. The top 7 proteins, including Ech_1061, 0725, 0350, 0506, 0679, 0494 and 0905, reacted strongly with all HME sera (similar to TRP120 positive control) and were considered immunodominant (mean OD650 values > 1.0) (Figure 2A). Additionally, some proteins (n = 49) reacted with all sera at lower levels (mean OD650 = 0.5~1.0), and only reacted strongly with some HME sera. Thus, these immunoreactive proteins were considered subdominant.
E. canis immunoreactive proteins (n = 15; mean OD650 values of > 0.5) were identified using 8 CME sera, but only 1 (Ecaj_0647) reacted strongly with most canine sera (mean OD650 value of > 1.0) and was considered immunodominant (Figure 2B). The positive control TRP19 reacted strongly with all CME sera. In addition, 3 E. canis proteins (Ecaj_0710, 0026 and 0274) with screening OD650 values of > 1.0 exhibited mean OD650 values of 0.5-1.0 with 8 CME sera, and thus were considered subdominant.
Antibody epitopes of immunodominant proteins
Recently, we have revealed that most immunoreactive proteins of E. chaffeensis and E. canis identified previously have conformation-dependent antibody epitopes (Luo et al., 2020; Luo et al., 2021). Thus, in this study we also investigated the conformation-dependence of 7 E. chaffeensis and 1 E. canis immunodominant proteins by denaturing ELISA. After denaturation using urea, only 2 E. chaffeensis immunodominant protein (Ech_1061 and 0905) among top 7 still reacted weakly with 2 HME sera (mean OD650 < 0.2 from 8 sera), compared to the native IVTT proteins (mean OD650 = 1.25 and 1.01, respectively). The remaining denatured E. chaffeensis proteins did not react with any HME patient sera, while the linear epitope-containing major immunoreactive protein control (TRP120) was not affected by denaturation (Figure 3A) (McBride and Walker, 2010). These results indicate that these E. chaffeensis immunoreactive proteins have conformation-dependent antibody epitopes.
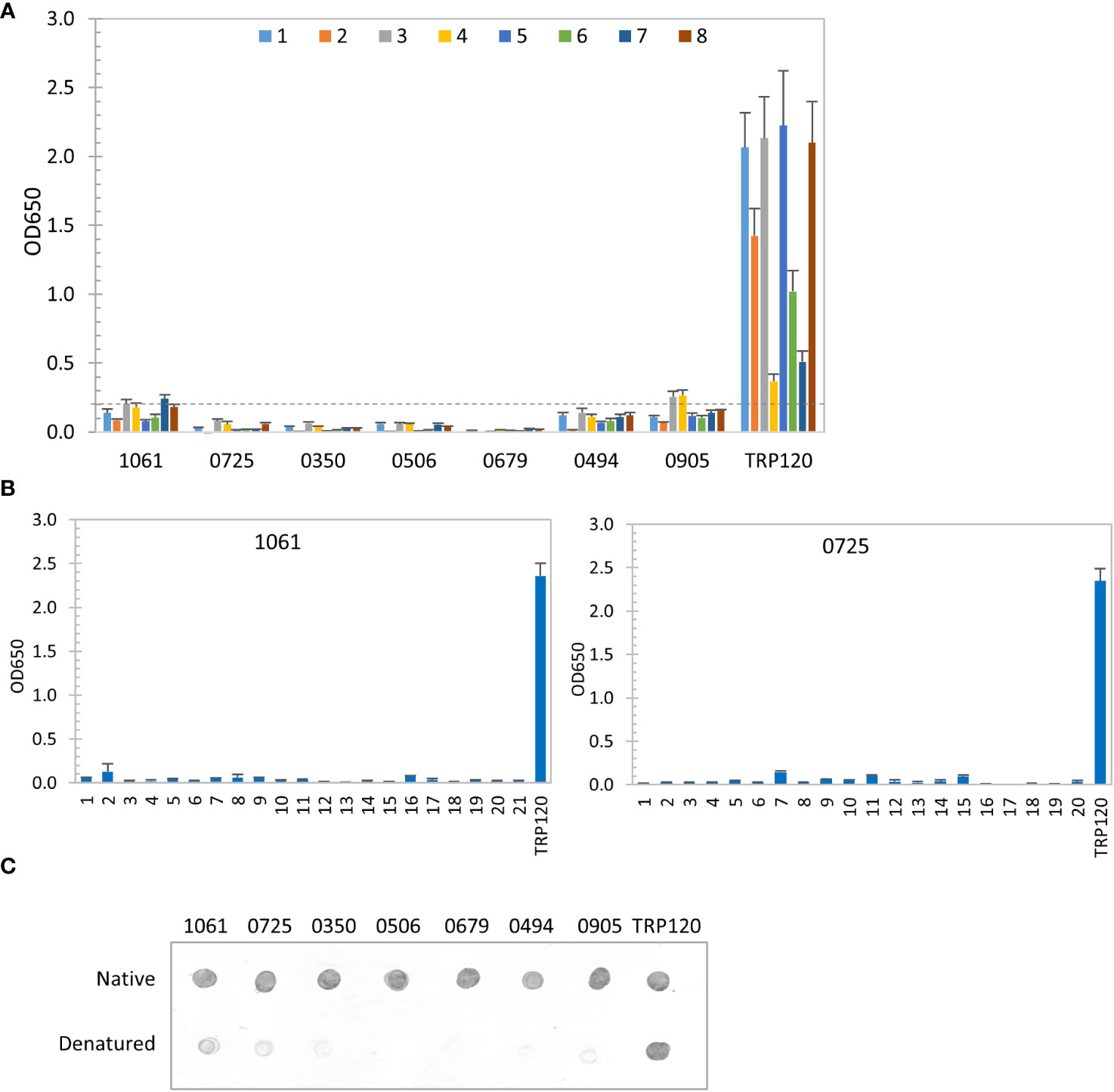
Figure 3 Conformation-dependent immunoreactivity of E. chaffeensis immunodominant proteins. (A) Immunoreactivity of the denatured IVTT-expressed E. chaffeensis proteins compared with TRP120 by ELISA using a panel of 8 HME sera. (B) Immunoreactivity of overlapping synthetic peptides spanning 2 E. chaffeensis immunoreactive proteins by ELISA with pooled HME sera. Positive control, a TRP120 epitope peptide. OD650 values represent the mean optical density reading from 3 wells (± standard deviation). A sample OD650 of ≥ 0.2 was considered positive and ≥ 0.5 a strong positive after subtracting negative control (A: an IVTT reaction with empty plasmid template and a normal human or canine serum control; B: a negative peptide) readings. (C) Conformation-dependent immunoreactivity of E. chaffeensis proteins by dot immunoblot. Immunoreactivity of the native and denatured proteins and TRP120 was detected with serum from an HME patient. All proteins were IVTT expressed and purified.
We also used a synthetic peptide ELISA to confirm the absence of major linear epitopes and presence of conformation-dependent epitopes in 2 selected E. chaffeensis immunodominant proteins (Ech_1061 and 0725) (Luo et al., 2008; Luo et al., 2009; McBride et al., 2011). Overlapping peptides (17-23 amino acids; 6 amino-acid overlap) covering each entire protein sequence were synthesized. The pooled HME sera used in our initial screening was used to test all peptides by ELISA (Figure 3B). None of these peptides reacted with HME sera, demonstrating that these E. chaffeensis immunodominant proteins do not contain major linear epitopes, consistent with ELISA results using native and denatured IVTT products (Figures 2A, 3A). We further examined the conformational dependence of epitopes in 7 new E. chaffeensis immunodominant proteins by dot immunoblot (Figure 3C). The immunoreactivity of native and denatured proteins was compared using an HME serum. After denaturation, these proteins did not react or reacted weakly with E. chaffeensis antibodies, consistent with our ELISA data in Figures 3A, B. These results support the conclusion that the many immunodominant proteins of E. chaffeensis are defined by conformation-dependent antibody epitopes.
The immunoreactivity of E. canis protein (Ecaj_0647) was only slightly reduced after denaturation by ELISA, indicating a major linear and minor conformation-dependent antibody epitopes were present. The well-defined E. canis major immunoreactive protein TRP19 containing a major linear antibody epitope was not affected by denaturation (Figure 4A). By dot immunoblot, denatured Ecaj_0647 protein reacted strongly with the E. canis antibodies, but at a lower level compared to native proteins, whereas TRP19 protein reacted at a level similar to the native proteins (Figure 4B). This result is consistent with our ELISA data in Figure 4A.
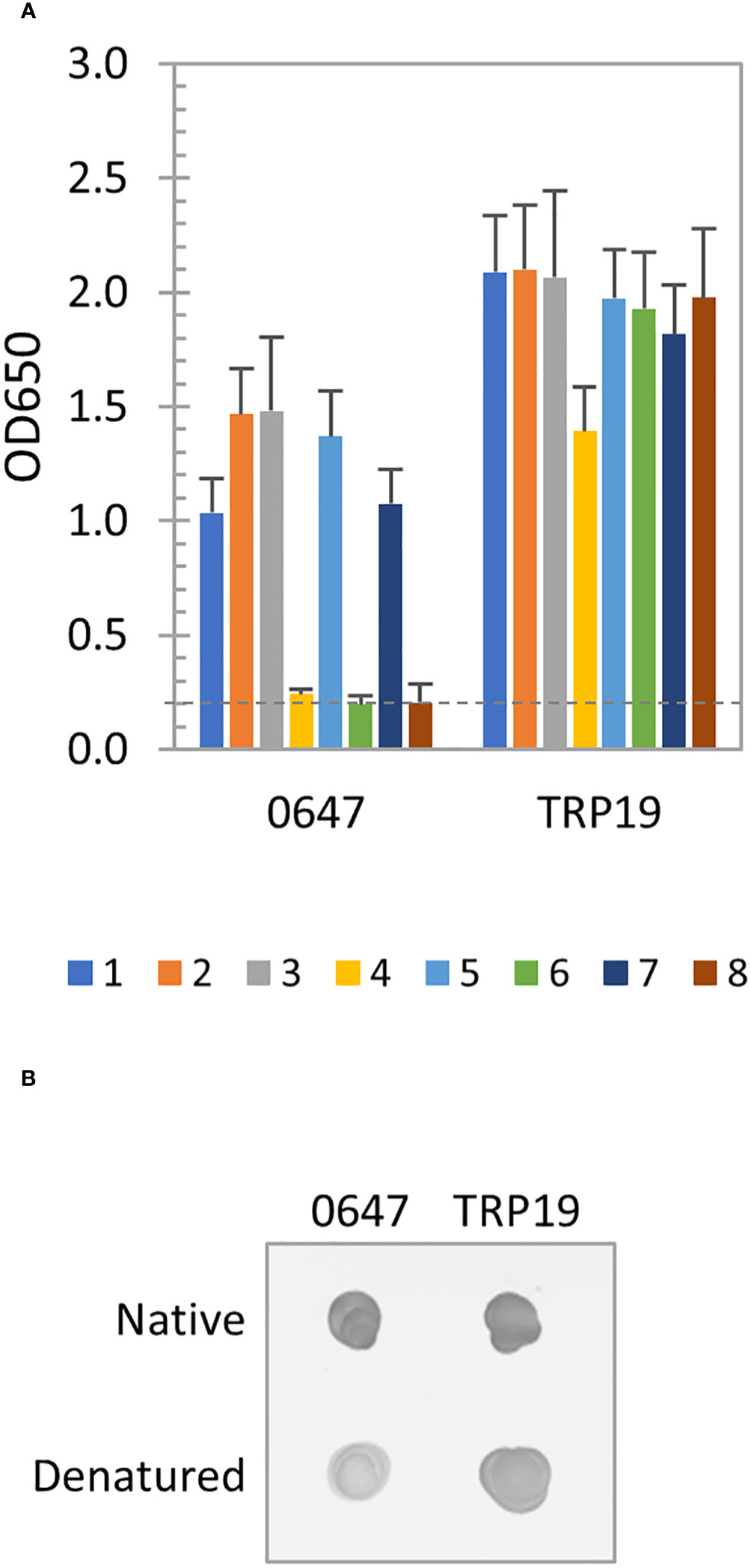
Figure 4 Immunoreactivity of new E. canis immunodominant protein. (A) Immunoreactivity of the denatured IVTT-expressed Ecaj_0647 protein compared with TRP19 by ELISA using a panel of 8 CME sera. OD650 values represent the mean optical density reading from 3 wells (± standard deviation). A sample OD650 of ≥ 0.2 was considered positive and ≥ 0.5 a strong positive after subtracting negative control (an IVTT reaction with empty plasmid template and a normal human or canine serum control) readings. (B) Immunoreactivity of native and denatured Ecaj_0647 protein compared with TRP19 by dot immunoblot with pooled CME sera. Both proteins were IVTT-expressed and purified.
Analysis of antigenic proteins
To compile the antigenic repertories of E. chaffeensis and E. canis, we combined data from this study with our recent studies and summarized the results (Supplementary Figure 1). In total, our studies investigated 857 E. chaffeensis proteins and 817 E. canis proteins, excluding known antigens (such as TRPs and OMPs) and ribosomal proteins, and found 272 (32% of 857) and 112 (14% of 817) immunoreactive proteins, respectively. More importantly, we identified a large number of previously undefined immunodominant proteins in E. chaffeensis (n = 14) and E. canis (n = 18). In addition, we identified numerous Ehrlichia subdominant proteins (n = 70 and n = 16, respectively) and other proteins exhibiting low immunoreactivity (n = 188 and n = 78, respectively) (Supplementary Figure 1). Figure 5 shows the quantity analysis of antigenic proteins in E. chaffeensis and E. canis immunomes, including previously known antigens. Both Ehrlichia immunomes contain ~3% immunodominant proteins; however, E. chaffeensis has more subdominant proteins and proteins exhibiting low immunoreactivity than E. canis (9% and 21% vs. 2% and 9%, respectively). Therefore, the proportion of the proteome that was not antigenic in E. canis and E. chaffeensis was 86% and 67%, respectively.
We performed a comprehensive analysis of all new immunodominant proteins of E. chaffeensis and E. canis. The immunoreactivity, E. canis orthologs and bioinformatic analysis of E. chaffeensis immunodominant proteins ranked by ELISA OD values are shown in Tables 1, 2. All these proteins contain major conformational epitopes, and a majority of these proteins (n = 11) were small (< 250 amino acids) (Table 1). Among E. chaffeensis immunodominant proteins (n = 14), there were 6 hypothetical protein and 8 annotated proteins, including an outer membrane beta-barrel protein, a type IV secretion system component VirB3 and 6 enzymes involved in important biological processes, such as dehydrogenase, transferase, kinase, and phosphatase (Table 2). A bioinformatic analysis using multiple online prediction tools found that most E. chaffeensis immunodominant proteins (n = 8) were predicted to contain at least 1 (up to 8) transmembrane domain by TMHMM 2.0; however, using SignalP 6.0 and SecretomeP 2.0, only 3 proteins (Ech_0745, 0678 and 0679) were predicted to be secreted by a standard secretory signal peptide or a nonclassical (not signal peptide-dependent) protein secretion. Notably, most E. chaffeensis immunodominant proteins (n = 8) were identified as effectors by PREFFECTOR (Dhroso et al., 2018). Therefore, these proteins were also further analyzed to identify the type of secretion system substrates. Type I and type IV secretion systems (T1SS and T4SS) have been identified in Ehrlichia; however, a consensus sequence of type IV secretory motif R-X(7)-R-X-R-X-R (Vergunst et al., 2005) was not identified in any E. chaffeensis protein and none of the immunodominant proteins identified in this study were predicted to be type IV substrates by the S4TE 2.0 tool (Noroy et al., 2019). In contrast, a putative type I secretion signal (LDAVTSIF-enriched and KHPMWC-poor) (Delepelaire, 2004; Wakeel et al., 2011) was identified in the last 50 C-terminal residues of these proteins, suggesting that these proteins are type I secreted substrates. Ech_0875 protein showed the greatest difference between the residue occurrences of LDAVTSIF (72%) and KHPMWC (8%) in the last 50 C-terminal amino acids, whereas Ech_0745 showed the least difference (32% versus 18%). These results are consistent with our previous reports and support the conclusion that many of these E. chaffeensis immunodominant proteins are type I secreted effectors, although additional experimental validation is required (Table 2).
The analysis of E. canis immunodominant protein, ranked ELISA OD values, and comparison with E. chaffeensis orthologs are shown in Tables 3, 4. The majority of these proteins (n = 14) contained major linear epitopes, including 5 proteins that also contained minor conformational epitopes. Four proteins (Ecaj_0128, 0348, 0857 and 0104) contained only major conformational epitopes (Table 3). A majority of E. canis immunodominant proteins (n = 11) were hypothetical, except for 7 annotated proteins including electron transport protein SCO1/SenC, an extracellular solute-binding protein, translation elongation factor 1A (EF-1A), 2-oxoglutarate dehydrogenase E2 component, peptidyl-prolyl cis-trans isomerase and 2 heat shock proteins (HSP60 and HSP70). Only 8 of these immunodominant proteins were smaller than 250 amino acids. Bioinformatic analysis identified 7 E. canis proteins predicted to contain transmembrane domains and 5 proteins that were predicted to be secreted (all by nonclassical mechanism). Importantly, many of E. canis immunodominant proteins (n = 9) were identified as effectors by PREFFECTOR (Dhroso et al., 2018). Although 3 proteins (Ecaj_0126, 0259 and 0334) were predicted to be type IV substrates by the S4TE 2.0 tool, no type IV secretory signal (R-X[7]-R-X-R-X-R) was identified in any of these E. canis proteins. Moreover, a putative type I secretion signal (LDAVTSIF-enriched and KHPMWC-poor) in the C-terminus of these proteins suggested that most of these proteins are type I secreted substrates, consistent with our previous conclusion (Luo et al., 2020; Luo et al., 2021) (Table 4). Ecaj_0104 protein had the largest difference between the residue occurrences of LDAVTSIF (70%) and KHPMWC (6%) in the last 50 C-terminal amino acids, whereas the predicted type IV substrate Ech_0259 had the least difference (36% versus 24%).
Discussion
The development of new and affordable biotechniques, such as next-generation genome sequencing, commercial gene synthesis and cloning, and in vitro protein expression, has made the analysis of entire bacterial immunomes feasible. Our recent studies have established a rapid high-throughput antigen discovery strategy and we have used this approach to successfully identify many previously undiscovered immunoreactive proteins from E. chaffeensis and E. canis (Luo et al., 2020; Luo et al., 2021). In this investigation, we identified many new immunodominant and subdominant ehrlichial proteins allowing us to reveal the antibody reactive antigenic repertoires of E. chaffeensis and E. canis. This information will ultimately expand and accelerate vaccine and diagnostic development for the ehrlichioses.
The application of IVTT in antigen discovery is the key to identification of conformation-dependent immunoreactive proteins, because IVTT generally expresses soluble proteins in native conformation, although posttranslational modifications may not exist (Shimizu et al., 2006; Carlson et al., 2012). The majority of bacterial B-cell epitopes are estimated to be conformational, and many pathogens are known to have immunoreactive proteins with conformational antibody epitopes (Portnyagina et al., 2018; Andrade et al., 2019; Liu et al., 2019; He et al., 2020). However, prior to our studies, the defined E. chaffeensis and E. canis immunoreactive proteins were limited to those with only major linear epitopes due to the limitations in the approaches used for screening (McBride and Walker, 2010; Lina et al., 2016). Our recent studies have revealed many immunoreactive proteins of E. chaffeensis and E. canis are predominated by conformation-dependent epitopes (Luo et al., 2020; Luo et al., 2021). Considering the antibody reactive proteins identified in our studies, including the present, all of the new E. chaffeensis immunodominant proteins contain major conformation-dependent epitopes; however, linear antibody epitopes are predominant in E. canis immunodominant proteins, although we have also identified many conformation-dependent epitopes in immunoreactive protein repertoires of E. canis.
Overall, E. canis immunodominant proteins appear to have higher ANTIGENpro score and rank than E. chaffeensis proteins, which may be related to the differences in the number of linear antibody epitopes found in E. canis antigenic proteins (Tables 1, 3). All previously characterized major immunoreactive proteins of E. chaffeensis and E. canis that contain major linear epitopes, including TRPs, Ank200, OMPs and MSP4, are represented in the top 250 list predicted by ANTIGENpro (Luo et al., 2020), suggesting that the machine learning model of ANTIGENpro may have a bias as it relates to known immunoprotective proteins used to train the algorithm that likely have a predominance of linear epitopes.
Many ehrlichial proteins that were previously considered to have unknown function (hypothetical), including TRPs and Anks, are now known to have defined functions during infection (Wakeel et al., 2009; Luo et al., 2011; Luo and McBride, 2012; Luo et al., 2018; Byerly et al., 2021). Of proteins that make up the E. chaffeensis and E. canis proteomes, ~25% are considered hypothetical or proteins with domain of unknown function (DUFs). Studies with other intracellular pathogens including our own empirical data in Ehrlichia have determined that a large number of these proteins are immunoreactive (Cruz-Fisher et al., 2011; Liu et al., 2019). As a result, we have recently reported that many immunoreactive proteins in the E. chaffeensis and E. canis proteomes are dominated by hypothetical proteins (Luo et al., 2020; Luo et al., 2021). An analysis of immunodominant proteins in Ehrlichia showed that while many hypothetical proteins are E. chaffeensis antigenic proteins, more immunoreactive hypothetical proteins exist in E. canis (Tables 2, 4).
Among new immunodominant proteins identified in E. chaffeensis and E. canis in these studies, there were notable proteins with known functions. Interestingly, most E. chaffeensis proteins were predicted to be enzymes involved in important biological processes, such as energy production and conversion, coenzyme transport and metabolism, glycerophospholipid metabolism and protein regulation, demonstrating that these metabolically functional Ehrlichia proteins are also antigenic. Other more antigenically established proteins included an outer membrane beta-barrel protein and a type IV secretion system protein VirB3 (Table 2). Multiple Ehrlichia/Anaplasma outer membrane proteins and type IV secretion system proteins, such as TRP, OMP, VirB and VirD, have also been previously identified as antigenic and protective (Sutten et al., 2010; Crocquet-Valdes et al., 2011; Kuriakose et al., 2012). Of new immunodominant proteins of E. canis identified in these studies, the antigenic annotated proteins are different from those identified in E. chaffeensis. For example, a peptidyl-prolyl cis-trans isomerase, an electron transport protein, an extracellular solute-binding protein, a translation elongation factor, and a heat shock protein HSP70 were identified in E. canis but not in E. chaffeensis (Table 4). Another heat shock protein GroEL (HSP60) has an ortholog in E. chaffeensis that was identified as the first immunodominant protein in 1993 (Sumner et al., 1993). Immunization with E. muris GroEL peptide is protective in a mouse model (Thomas et al., 2011). Notably, many immunodominant proteins of E. chaffeensis and E. canis were predicted to contain transmembrane domains, further highlighting this feature in many antigenic proteins.
Unlike the previously identified immunoreactive protein orthologs of Ehrlichia and E. canis that contain major linear epitopes, such as TRPs, Anks and OMPs, only 2 respective E. canis or E. chaffeensis orthologs (2-oxoglutarate dehydrogenase E2 component and GroEL) in these studies were also found to be immunodominant, and only a minority of E. chaffeensis or E. canis orthologs shared immunoreactivity, although the respective orthologs were identified for the majority of Ehrlichia immunodominant proteins (Tables 1, 3). These findings suggest that E. chaffeensis and E. canis do not have similar orthologous antigenic proteins as might be expected, and the antibody epitopes in majority of Ehrlichia immunodominant proteins are not conserved between E. chaffeensis and E. canis. Notably, E. chaffeensis immunodominant proteins reacted with HME sera more consistently than E. canis proteins with CME sera (Figure 2). Hence, it is possible that E. canis immunoreactive proteins are more antigenically variable among different E. canis strains. Antigenic diversity in E. canis is well defined, including TRP36, a major immunoreactive protein, and extensive phylogenetic analysis of TRP36 genes has identified several E. canis genogroups in North America, Central America, South America, Africa, Europe and Asia, which have antigenic variability (Zhang et al., 2008; Aguiar et al., 2013; Arroyave et al., 2020). Therefore, antigenic variability in some E. canis proteins may also contribute to the lower ratio of immunoreactive proteins we found in E. canis compared to E. chaffeensis in this study and the entire immunomes. In addition, the average size of new E. chaffeensis immunodominant proteins appear to be smaller than that of E. canis. A vast majority of 14 new E. chaffeensis immunodominant proteins (n = 11) are small (< 250 amino acids), while only 8 of 18 E. canis proteins are small (Tables 2, 4). Collectively, these results indicate the fundamental differences in antigenic protein profiles between E. chaffeensis and E. canis, which is potentially valuable information for development of specific diagnostics and vaccines for these Ehrlichia species.
Consistent with our recent reports (Luo et al., 2020; Luo et al., 2021), the majority of E. chaffeensis and E. canis immunodominant proteins were predicted to be type I secreted effectors, despite that fact that only 8 proteins were predicted to be secreted by SignalP or SecretomeP. This reinforces the conclusion that in addition to previously defined major immunoreactive proteins that have linear epitopes, such as TRPs and Ank200, there are also other proteins with conformational epitopes that appear to be T1SS substrates. These results further support an important role of the T1SS in Ehrlichia infection and potentially immunity (Wakeel et al., 2011). We have shown that several ehrlichial T1S substrates (TRPs) play important roles in pathobiology by regulating important cellular processes to promote ehrlichial survival (Dunphy et al., 2013; Lina et al., 2016; Bui et al., 2023).
Collectively, this investigation and combined with our other recent studies, has successfully defined the antigenic proteins contained in the E. chaffeensis and E. canis proteomes. We expect that this information will provide a defined set of antigens from which a rational vaccine and diagnostic development strategy can be implemented and tested for HME and CME. Further studies are also needed to determine the T-cell epitopes, secretion mechanism and roles of these proteins in ehrlichial pathobiology and immunity.
Data availability statement
The original contributions presented in the study are included in the article/Supplementary Material. Further inquiries can be directed to the corresponding author.
Ethics statement
Ethical approval was not required for the studies on humans in accordance with the local legislation and institutional requirements because only commercially available established cell lines were used.
Author contributions
TL: Data curation, Formal Analysis, Investigation, Methodology, Validation, Writing – original draft, Writing – review & editing. JP: Data curation, Formal Analysis, Investigation, Methodology, Writing – review & editing. XZ: Investigation, Methodology, Writing – review & editing. JM: Conceptualization, Data curation, Funding acquisition, Supervision, Writing – review & editing.
Funding
The author(s) declare financial support was received for the research, authorship, and/or publication of this article. This work was supported by the Clayton Foundation for Research.
Conflict of interest
The authors declare the existence of a financial/non-financial competing interest including a pending patent application.
Publisher’s note
All claims expressed in this article are solely those of the authors and do not necessarily represent those of their affiliated organizations, or those of the publisher, the editors and the reviewers. Any product that may be evaluated in this article, or claim that may be made by its manufacturer, is not guaranteed or endorsed by the publisher.
Supplementary material
The Supplementary Material for this article can be found online at: https://www.frontiersin.org/articles/10.3389/fcimb.2023.1321291/full#supplementary-material
References
Aguiar, D. M., Zhang, X., Melo, A. L., Pacheco, T. A., Meneses, A. M., Zanutto, M. S., et al. (2013). Genetic diversity of Ehrlichia canis in Brazil. Vet. Microbiol. 164 (3-4), 315–321. doi: 10.1016/j.vetmic.2013.02.015
Andrade, D. V., Warnes, C., Young, E., Katzelnick, L. C., Balmaseda, A., de Silva, A. M., et al. (2019). Tracking the polyclonal neutralizing antibody response to a dengue virus serotype 1 type-specific epitope across two populations in Asia and the Americas. Sci. Rep. 9 (1), 16258. doi: 10.1038/s41598-019-52511-z
Arroyave, E., Rodas-Gonzalez, J. D., Zhang, X., Labruna, M. B., Gonzalez, M. S., Fernandez-Silva, J. A., et al. (2020). Ehrlichia canis TRP36 diversity in naturally infected-dogs from an urban area of Colombia. Ticks Tick Borne Dis. 11 (3), 101367. doi: 10.1016/j.ttbdis.2019.101367
Babu, M., Snyder, M. (2023). Multi-omics profiling for health. Mol. Cell Proteomics 22 (6), 100561. doi: 10.1016/j.mcpro.2023.100561
Barbour, A. G., Jasinskas, A., Kayala, M. A., Davies, D. H., Steere, A. C., Baldi, P., et al. (2008). A genome-wide proteome array reveals a limited set of immunogens in natural infections of humans and white-footed mice with Borrelia burgdorferi. Infect. Immun. 76 (8), 3374–3389. doi: 10.1128/IAI.00048-08
Bidmos, F. A., Siris, S., Gladstone, C. A., Langford, P. R. (2018). Bacterial vaccine antigen discovery in the reverse vaccinology 2.0 era: progress and challenges. Front. Immunol. 9. doi: 10.3389/fimmu.2018.02315
Budachetri, K., Lin, M., Chien, R. C., Zhang, W., Brock, G. N., Rikihisa, Y. (2022). Efficacy and immune correlates of OMP-1B and virB2-4 vaccines for protection of dogs from tick transmission of ehrlichia chaffeensis. mBio 13 (6), e0214022. doi: 10.1128/mbio.02140-22
Bui, D.-C., Luo, T., McBride, J. W. (2023). Type 1 secretion system and effectors in Rickettsiales. Front. Cell. Infection Microbiol. 13. doi: 10.3389/fcimb.2023.1175688
Byerly, C. D., Patterson, L. L., McBride, J. W. (2021). Ehrlichia TRP effectors: moonlighting, mimicry and infection. Pathog. Dis. 79 (5). doi: 10.1093/femspd/ftab026
Cardenas, A. M., Doyle, C. K., Zhang, X., Nethery, K., Corstvet, R. E., Walker, D. H., et al. (2007). Enzyme-linked immunosorbent assay with conserved immunoreactive glycoproteins gp36 and gp19 has enhanced sensitivity and provides species-specific immunodiagnosis of Ehrlichia canis infection. Clin. Vaccine Immunol. 14 (2), 123–128. doi: 10.1128/CVI.00361-06
Carlson, E. D., Gan, R., Hodgman, C. E., Jewett, M. C. (2012). Cell-free protein synthesis: applications come of age. Biotechnol. Adv. 30 (5), 1185–1194. doi: 10.1016/j.bioteChadv.2011.09.016
Chen, I. A., Chu, K., Palaniappan, K., Pillay, M., Ratner, A., Huang, J., et al. (2019). IMG/M v.5.0: an integrated data management and comparative analysis system for microbial genomes and microbiomes. Nucleic Acids Res. 47 (D1), D666–D677. doi: 10.1093/nar/gky901
Crocquet-Valdes, P. A., Thirumalapura, N. R., Ismail, N., Yu, X., Saito, T. B., Stevenson, H. L., et al. (2011). Immunization with Ehrlichia P28 outer membrane proteins confers protection in a mouse model of ehrlichiosis. Clin. Vaccine Immunol. 18 (12), 2018–2025. doi: 10.1128/CVI.05292-11
Cruz-Fisher, M. I., Cheng, C., Sun, G., Pal, S., Teng, A., Molina, D. M., et al. (2011). Identification of immunodominant antigens by probing a whole Chlamydia trachomatis open reading frame proteome microarray using sera from immunized mice. Infect. Immun. 79 (1), 246–257. doi: 10.1128/IAI.00626-10
Delepelaire, P. (2004). Type I secretion in gram-negative bacteria. Biochim. Biophys. Acta 1694 (1-3), 149–161. doi: 10.1016/j.bbamcr.2004.05.001
De Sousa, K. P., Doolan, D. L. (2016). Immunomics: a 21st century approach to vaccine development for complex pathogens. Parasitology 143 (2), 236–244. doi: 10.1017/S0031182015001079
Dhroso, A., Eidson, S., Korkin, D. (2018). Genome-wide prediction of bacterial effector candidates across six secretion system types using a feature-based statistical framework. Sci. Rep. 8 (1), 17209. doi: 10.1038/s41598-018-33874-1
Doyle, C. K., Nethery, K. A., Popov, V. L., McBride, J. W. (2006). Differentially expressed and secreted major immunoreactive protein orthologs of Ehrlichia canis and E. chaffeensis elicit early antibody responses to epitopes on glycosylated tandem repeats. Infect. Immun. 74 (1), 711–720. doi: 10.1128/IAI.74.1.711-720.2006
Dunning Hotopp, J. C., Lin, M., Madupu, R., Crabtree, J., Angiuoli, S. V., Eisen, J. A., et al. (2006). Comparative genomics of emerging human ehrlichiosis agents. PloS Genet. 2 (2), e21. doi: 10.1371/journal.pgen.0020021
Dunphy, P. S., Luo, T., McBride, J. W. (2013). Ehrlichia moonlighting effectors and interkingdom interactions with the mononuclear phagocyte. Microbes Infect. 15 (14-15), 1005–1016. doi: 10.1016/j.micinf.2013.09.011
Felgner, P. L., Kayala, M. A., Vigil, A., Burk, C., Nakajima-Sasaki, R., Pablo, J., et al. (2009). A Burkholderia pseudomallei protein microarray reveals serodiagnostic and cross-reactive antigens. Proc. Natl. Acad. Sci. U.S.A. 106 (32), 13499–13504. doi: 10.1073/pnas.0812080106
Harrus, S., Waner, T. (2011). Diagnosis of canine monocytotropic ehrlichiosis (Ehrlichia canis): an overview. Vet. J. 187 (3), 292–296. doi: 10.1016/j.tvjl.2010.02.001
He, B., Zhang, Z., Zhang, X., Tang, Z., Liu, C., Zheng, Z., et al. (2020). Functional epitopes on hepatitis E virions and recombinant capsids are highly conformation-dependent. Hum. Vaccin Immunother. 16 (7), 1554–1564. doi: 10.1080/21645515.2019.1703454
Jones, D. D., DeIulio, G. A., Winslow, G. M. (2012). Antigen-driven induction of polyreactive IgM during intracellular bacterial infection. J. Immunol. 189 (3), 1440–1447. doi: 10.4049/jimmunol.1200878
Kuriakose, J. A., Zhang, X., Luo, T., McBride, J. W. (2012). Molecular basis of antibody mediated immunity against Ehrlichia chaffeensis involves species-specific linear epitopes in tandem repeat proteins. Microbes Infect. 14 (12), 1054–1063. doi: 10.1016/j.micinf.2012.05.012
Li, J. S., Yager, E., Reilly, M., Freeman, C., Reddy, G. R., Reilly, A. A., et al. (2001). Outer membrane protein-specific monoclonal antibodies protect SCID mice from fatal infection by the obligate intracellular bacterial pathogen Ehrlichia chaffeensis. J.Immunol. 166 (3), 1855–1862. doi: 10.4049/jimmunol.166.3.1855
Lina, T. T., Farris, T., Luo, T., Mitra, S., Zhu, B., McBride, J. W. (2016). Hacker within! Ehrlichia chaffeensis effector driven phagocyte reprogramming strategy. Front. Cell Infect. Microbiol. 6. doi: 10.3389/fcimb.2016.00058
Liu, J., Parrish, J. R., Hines, J., Mansfield, L., Finley, R. L., Jr. (2019). A proteome-wide screen of Campylobacter jejuni using protein microarrays identifies novel and conformational antigens. PloS One 14 (1), e0210351. doi: 10.1371/journal.pone.0210351
Loman, N. J., Pallen, M. J. (2015). Twenty years of bacterial genome sequencing. Nat. Rev. Microbiol. 13 (12), 787–794. doi: 10.1038/nrmicro3565
Luo, T., Kuriakose, J. A., Zhu, B., Wakeel, A., McBride, J. W. (2011). Ehrlichia chaffeensis TRP120 interacts with a diverse array of eukaryotic proteins involved in transcription, signaling, and cytoskeleton organization. Infect. Immun. 79 (11), 4382–4391. doi: 10.1128/IAI.05608-11
Luo, T., McBride, J. W. (2012). Ehrlichia chaffeensis TRP32 interacts with host cell targets that influence intracellular survival. Infect. Immun. 80 (7), 2297–2306. doi: 10.1128/IAI.00154-12
Luo, T., Mitra, S., McBride, J. W. (2018). Ehrlichia chaffeensis TRP75 Interacts with host cell targets involved in homeostasis, cytoskeleton organization, and apoptosis regulation to promote infection. mSphere 3 (2), e00147–e00118. doi: 10.1128/mSphere.00147-18
Luo, T., Patel, J. G., Zhang, X., Walker, D. H., McBride, J. W. (2020). Ehrlichia chaffeensis and E. canis hypothetical protein immunoanalysis reveals small secreted immunodominant proteins and conformation-dependent antibody epitopes. NPJ Vaccines 5, 85. doi: 10.1038/s41541-020-00231-1
Luo, T., Patel, J. G., Zhang, X., Walker, D. H., McBride, J. W. (2021). Immunoreactive Protein Repertoires of Ehrlichia chaffeensis and E. canis Reveal the Dominance of Hypothetical Proteins and Conformation-Dependent Antibody Epitopes. Infect. Immun. 89 (11), e0022421. doi: 10.1128/IAI.00224-21
Luo, T., Zhang, X., McBride, J. W. (2009). Major species-specific antibody epitopes of the Ehrlichia chaffeensis p120 and E. canis p140 orthologs in surface-exposed tandem repeat regions. Clin. Vaccine Immunol. 16 (7), 982–990. doi: 10.1128/CVI.00048-09
Luo, T., Zhang, X., Nicholson, W. L., Zhu, B., McBride, J. W. (2010). Molecular characterization of antibody epitopes of Ehrlichia chaffeensis ankyrin protein 200 and tandem repeat protein 47 and evaluation of synthetic immunodeterminants for serodiagnosis of human monocytotropic ehrlichiosis. Clin. Vaccine Immunol. 17 (1), 87–97. doi: 10.1128/CVI.00331-09
Luo, T., Zhang, X., Wakeel, A., Popov, V. L., McBride, J. W. (2008). A variable-length PCR target protein of Ehrlichia chaffeensis contains major species-specific antibody epitopes in acidic serine-rich tandem repeats. Infect. Immun. 76 (4), 1572–1580. doi: 10.1128/IAI.01466-07
Magnan, C. N., Zeller, M., Kayala, M. A., Vigil, A., Randall, A., Felgner, P. L., et al. (2010). High-throughput prediction of protein antigenicity using protein microarray data. Bioinformatics 26 (23), 2936–2943. doi: 10.1093/bioinformatics/btq551
Mavromatis, K., Doyle, C. K., Lykidis, A., Ivanova, N., Francino, M. P., Chain, P., et al. (2006). The genome of the obligately intracellular bacterium Ehrlichia canis reveals themes of complex membrane structure and immune evasion strategies. J. Bacteriol. 188 (11), 4015–4023. doi: 10.1128/JB.01837-05
McBride, J. W., Doyle, C. K., Zhang, X., Cardenas, A. M., Popov, V. L., Nethery, K. A., et al. (2007). Identification of a glycosylated Ehrlichia canis 19-kilodalton major immunoreactive protein with a species-specific serine-rich glycopeptide epitope. Infect. Immun. 75 (1), 74–82. doi: 10.1128/IAI.01494-06
McBride, J. W., Walker, D. H. (2010). Progress and obstacles in vaccine development for the ehrlichioses. Expert Rev. Vaccines 9 (9), 1071–1082. doi: 10.1586/erv.10.93
McBride, J. W., Walker, D. H. (2011). Molecular and cellular pathobiology of Ehrlichia infection: targets for new therapeutics and immunomodulation strategies. Expert Rev. Mol. Med. 13, e3. doi: 10.1017/S1462399410001730
McBride, J. W., Zhang, X., Wakeel, A., Kuriakose, J. A. (2011). Tyrosine-phosphorylated Ehrlichia chaffeensis and Ehrlichia canis tandem repeat orthologs contain a major continuous cross-reactive antibody epitope in lysine-rich repeats. Infect. Immun. 79 (8), 3178–3187. doi: 10.1128/IAI.01347-10
McGill, J. L., Nair, A. D., Cheng, C., Rusk, R. A., Jaworski, D. C., Ganta, R. R. (2016). Vaccination with an attenuated mutant of ehrlichia chaffeensis induces pathogen-specific CD4+ T cell immunity and protection from tick-transmitted wild-type challenge in the canine host. PloS One 11 (2), e0148229. doi: 10.1371/journal.pone.0148229
Nambooppha, B., Rittipornlertrak, A., Muenthaisong, A., Koonyosying, P., Chomjit, P., Sangkakam, K., et al. (2022). Recombinant ehrlichia canis GP19 protein as a promising vaccine prototype providing a protective immune response in a mouse model. Vet. Sci. 9 (8). doi: 10.3390/vetsci9080386
Nethery, K. A., Doyle, C. K., Zhang, X., McBride, J. W. (2007). Ehrlichia canis gp200 contains dominant species-specific antibody epitopes in terminal acidic domains. Infect. Immun. 75 (10), 4900–4908. doi: 10.1128/IAI.00041-07
Noroy, C., Lefrancois, T., Meyer, D. F. (2019). Searching algorithm for Type IV effector proteins (S4TE) 2.0: Improved tools for Type IV effector prediction, analysis and comparison in proteobacteria. PloS Comput. Biol. 15 (3), e1006847. doi: 10.1371/journal.pcbi.1006847
Ohashi, N., Unver, A., Zhi, N., Rikihisa, Y. (1998a). Cloning and characterization of multigenes encoding the immunodominant 30-kilodalton major outer membrane proteins of Ehrlichia canis and application of the recombinant protein for serodiagnosis. J. Clin. Microbiol. 36 (9), 2671–2680. doi: 10.1128/JCM.36.9.2671-2680.1998
Ohashi, N., Zhi, N., Zhang, Y., Rikihisa, Y. (1998b). Immunodominant major outer membrane proteins of Ehrlichia chaffeensis are encoded by a polymorphic multigene family. Infect. Immun. 66 (1), 132–139. doi: 10.1128/IAI.66.1.132-139.1998
Paddock, C. D., Childs, J. E. (2003). Ehrlichia chaffeensis: a prototypical emerging pathogen. Clin. Microbiol. Rev. 16 (1), 37–64. doi: 10.1128/cmr.16.1.37-64.2003
Portnyagina, O., Zelepuga, E., Khomenko, V., Solov'eva, E., Solov'eva, T., Novikova, O. (2018). In silico and in vitro analysis of cross-reactivity between Yersinia pseudotuberculosis OmpF porin and thyroid-stimulating hormone receptor. Int. J. Biol. Macromol. 107 (Pt B), 2484–2491. doi: 10.1016/j.ijbiomac.2017.10.133
Pritt, B., Dumler, J. S. (2019). “Ehrlichia, Anaplasma, and related intracellular bacteria,” in Manual of Clinical Microbiology, 12 (Washington, DC: ASM Press), 1173.
Rudoler, N., Baneth, G., Eyal, O., van Straten, M., Harrus, S. (2012). Evaluation of an attenuated strain of Ehrlichia canis as a vaccine for canine monocytic ehrlichiosis. Vaccine 31 (1), 226–233. doi: 10.1016/j.vaccine.2012.10.003
Sette, A., Fleri, W., Peters, B., Sathiamurthy, M., Bui, H. H., Wilson, S. (2005). A roadmap for the immunomics of category A-C pathogens. Immunity 22 (2), 155–161. doi: 10.1016/j.immuni.2005.01.009
Shimizu, Y., Kuruma, Y., Ying, B. W., Umekage, S., Ueda, T. (2006). Cell-free translation systems for protein engineering. FEBS J. 273 (18), 4133–4140. doi: 10.1111/j.1742-4658.2006.05431.x
Sumner, J. W., Sims, K. G., Jones, D. C., Anderson, B. E. (1993). Ehrlichia chaffeensis expresses an immunoreactive protein homologous to the Escherichia coli GroEL protein. Infect. Immun. 61 (8), 3536–3539. doi: 10.1128/iai.61.8.3536-3539.1993
Sutten, E. L., Norimine, J., Beare, P. A., Heinzen, R. A., Lopez, J. E., Morse, K., et al. (2010). Anaplasma marginale type IV secretion system proteins VirB2, VirB7, VirB11, and VirD4 are immunogenic components of a protective bacterial membrane vaccine. Infect. Immun. 78 (3), 1314–1325. doi: 10.1128/IAI.01207-09
Taques, I., Koiyama, M. F. G., Campos, A. N. S., Costa, J. S., Hongyu, K., Aguiar, D. M. (2020). Canonical correlative analyses among an enzyme-linked immunosorbent assay using synthetic peptides, an indirect fluorescent antibody test, and hematologic measurements in dogs infected with Ehrlichia canis. Vet. Clin. Pathol. 49 (4), 574–582. doi: 10.1111/vcp.12908
Thomas, S., Thirumalapura, N. R., Crocquet-Valdes, P. A., Luxon, B. A., Walker, D. H. (2011). Structure-based vaccines provide protection in a mouse model of ehrlichiosis. PloS One 6 (11), e27981. doi: 10.1371/journal.pone.0027981
Vergunst, A. C., van Lier, M. C., den Dulk-Ras, A., Stuve, T. A., Ouwehand, A., Hooykaas, P. J. (2005). Positive charge is an important feature of the C-terminal transport signal of the VirB/D4-translocated proteins of Agrobacterium. Proc. Natl. Acad. Sci. U.S.A. 102 (3), 832–837. doi: 10.1073/pnas.0406241102
Vigil, A., Chen, C., Jain, A., Nakajima-Sasaki, R., Jasinskas, A., Pablo, J., et al. (2011). Profiling the humoral immune response of acute and chronic Q fever by protein microarray. Mol. Cell Proteomics 10 (10), M110 006304. doi: 10.1074/mcp.M110.006304
Vigil, A., Davies, D. H., Felgner, P. L. (2010a). Defining the humoral immune response to infectious agents using high-density protein microarrays. Future Microbiol. 5 (2), 241–251. doi: 10.2217/fmb.09.127
Vigil, A., Ortega, R., Jain, A., Nakajima-Sasaki, R., Tan, X., Chomel, B. B., et al. (2010b). Identification of the feline humoral immune response to Bartonella henselae infection by protein microarray. PloS One 5 (7), e11447. doi: 10.1371/journal.pone.0011447
Wakeel, A., den Dulk-Ras, A., Hooykaas, P. J., McBride, J. W. (2011). Ehrlichia chaffeensis tandem repeat proteins and Ank200 are type 1 secretion system substrates related to the repeats-in-toxin exoprotein family. Front. Cell Infect. Microbiol. 1. doi: 10.3389/fcimb.2011.00022
Wakeel, A., Kuriakose, J. A., McBride, J. W. (2009). An Ehrlichia chaffeensis tandem repeat protein interacts with multiple host targets involved in cell signaling, transcriptional regulation, and vesicle trafficking. Infect. Immun. 77 (5), 1734–1745. doi: 10.1128/IAI.00027-09
Keywords: Ehrlichia, antibody epitope, conformation-dependent, immunome, immunomics
Citation: Luo T, Patel JG, Zhang X and McBride JW (2024) Antibody reactive immunomes of Ehrlichia chaffeensis and E. canis are diverse and defined by conformational antigenic determinants. Front. Cell. Infect. Microbiol. 13:1321291. doi: 10.3389/fcimb.2023.1321291
Received: 13 October 2023; Accepted: 19 December 2023;
Published: 09 January 2024.
Edited by:
Stacey Gilk, University of Nebraska Medical Center, United StatesReviewed by:
Isaura Simões, University of Coimbra, PortugalSusan Noh, United States Department of Agriculture, United States
Copyright © 2024 Luo, Patel, Zhang and McBride. This is an open-access article distributed under the terms of the Creative Commons Attribution License (CC BY). The use, distribution or reproduction in other forums is permitted, provided the original author(s) and the copyright owner(s) are credited and that the original publication in this journal is cited, in accordance with accepted academic practice. No use, distribution or reproduction is permitted which does not comply with these terms.
*Correspondence: Jere W. McBride, jemcbrid@utmb.edu