- 1Department of Laboratory Medicine, Affiliated Hospital of Zunyi Medical University, Zunyi, Guizhou, China
- 2School of Laboratory Medicine, Zunyi Medical University, Zunyi, Guizhou, China
Adaptation to oxidative stress is critical for survival of Vibrio cholerae in aquatic ecosystems and hosts. DegS activates the σE envelope stress response. We have previously revealed that DegS may be involved in regulating the oxidative stress response. In this study, we demonstrated that deletion of the degS gene attenuates the antioxidant capacity of V. cholerae. In addition, our results further revealed that the regulation of antioxidant capacity by DegS in V. cholerae could involve the cAMP-CRP complex, which regulates rpoS. XthA is an exonuclease that repairs oxidatively damaged cells and affects the bacterial antioxidant capacity. qRT-PCR showed that DegS, σE, cAMP, CRP, and RpoS positively regulate xthA gene transcription. XthA overexpression partially compensates for antioxidant deficiency in the degS mutant. These results suggest that DegS affects the antioxidant capacity of V.cholerae by regulating xthA expression via the cAMP-CRP-RpoS pathway. In a mouse intestinal colonization experiment, our data showed that V.cholerae degS, rpoE, and rpoS gene deletions were associated with significantly reduced resistance to oxidative stress and the ability to colonize the mouse intestine. In conclusion, these findings provide new insights into the regulation of antioxidant activity by V.cholerae DegS.
Introduction
Oxidative stress caused by excess reactive oxygen species (ROS) or a reduction in antioxidant capacity poses a significant threat to bacteria (Sudharsan et al., 2022). ROS, primarily superoxide anions, hydroxyl radicals, and hydrogen peroxide, are highly reactive substances produced as a result of the incomplete reduction of oxygen or redox production of chemicals (Si et al., 2015; Seixas et al., 2021). ROS can damage biomolecules such as DNA, RNA, proteins, and lipids (Chautrand et al., 2022). Hence, bacteria have developed antioxidant systems to cope with oxidative stress and ensure their survival and pathogenicity (Mishra and Imlay, 2012). For example, Escherichia coli utilizes OxyR and SoxRS to sense ROS signals and subsequently coordinate the expression of a set of genes encoding ROS-scavenging enzymes such as catalase and peroxidase (Honn et al., 2017).
Vibrio cholerae is an intestinal pathogen that lives in aquatic environments and is classified into more than 200 serogroups based on V.cholerae cell surface O antigens (Kaper et al., 1995; Clemens et al., 2017). Non-O1/non-O139 V.cholerae strains (NOVC) have been linked to sporadic localized cholera-like gastrointestinal diseases such as abdominal pain and diarrhea, as well as extra-intestinal infections like sepsis, infectious shock, pneumonia, and meningitis (Maraki et al., 2016; Chen et al., 2020). Despite the rise in NOVC infection cases, there has been little research on NOVC adaptability to oxidative stress. Antioxidant capability is critical for the survival of V.cholerae in vivo and in vitro. Therefore, it is of great significance to study the adaptability of non-O1/non-O139 V.cholerae under oxidative stress. V.cholerae can cause severe diarrhea and vomiting in humans when food contaminated with V. cholerae is ingested (Clemens et al., 2017; Qin et al., 2020; Wang et al., 2022). Diarrhea facilitates the passage of V.cholerae from the human body into the aquatic environment, where it can reinfect the human body. During this cycle, V.cholerae senses a variety of environmental signals and responds by adjusting gene expression to adapt effectively to its new ecological niche. Oxidative stress is one of the main stresses that must be overcome when V. cholerae infects the host and in the external environment (Qadri et al., 2002; Bhattacharyya et al., 2004; Lesser, 2006; Muller et al., 2017). ROS levels in the stools of patients with cholera are considerably higher than those in normal individuals (Wang et al., 2017). Duodenal samples from patients with cholera showed that oxidative stress-related proteases were activated (Ellis et al., 2015) and that V.cholerae isolated from the slop-like stools of cholera patients displayed greater tolerance to ROS (Qadri et al., 2002). The host produces large amounts of ROS to combat V.cholerae invasion and colonization, while V.cholerae regulates the expression of antioxidative stress genes to adapt to the host environment (Wang et al., 2017). For example, OxyR is critical for hydrogen peroxide resistance in V.cholerae, whereas OhrR, a regulator of ohrA, regulates the susceptibility of V.cholerae to organic hydrogen peroxide (Wang et al., 2012; Liu et al., 2016). Consequently, antioxidant activity is vital for the survival and pathogenicity of V.cholerae in aquatic environments and humans. From the perspective of cholera control, it would be beneficial to explore the details of antioxidant regulation in V.cholerae and establish methods to weaken this response.
DegS is a serine protease located in the bacterial periplasm that strictly controls the σE (rpoE) stress-response pathway (de Regt et al., 2015). In Salmonella, the rpoE mutant shows significantly increased sensitivity to H2O2 (Testerman et al., 2002; Hews et al., 2019). Our previous RNA sequencing results showed that degS knockout led to the downregulation of genes involved in the oxidative stress response. Gene-Act network analysis indicated that the cAMP-CRP-RpoS pathway is suppressed in degS knockout strains (Huang et al., 2019). The cAMP-CRP-RpoS pathway plays an essential role in oxidative stress in Salmonella (Cheng and Sun, 2009). Our previous results showed that DegS regulated V.cholerae motility and chemotaxis via cAMP–CRP–RpoS–FlhF pathway(Zou et al., 2023).In the present study, we investigated the effect of DegS on the antioxidant capacity of V.cholerae. Our results show that DegS regulates the expression of the nucleic acid exonuclease III gene xthA through the cAMP-CRP-RpoS pathway, thereby affecting the antioxidant capacity of V.cholerae.
Materials and methods
Strains and culture conditions
V.cholerae HN375 strain is a non-O1/non-O139 strain obtained from the China Type Culture Collection Center (CCTCC AB209168) (Luo et al., 2011) and was the wild type (WT) in this study. Escherichia coli DH5α was used for cloning and WM3064 was used as donor bacteria in the conjugation transformation experiment. All strains were cultured on Luria Bertani (LB) medium. In the killing assay and survival under starvation stress assay, using AKI medium contains 1.5% Bacto-peptone, 0.4% yeast extract, 0.5% NaCl, and 0.3% NaHCO3. 100μg/mL ampicillin or 50μg/mL chloramphenicol were added to LB or AKI medium if necessary. All bacterial strains and plasmids used in this study are listed in Supplementary Table 1.
DNA manipulation and gene technology
All deletion mutants were constructed using the suicide plasmid pWM91 in the wild-type HN375 strain (Wu et al., 2015). The primers used are listed in Supplementary Table 2. To construct complementary mutants, the full-length coding regions of cyaA, crp, rpoS, and xthA were cloned into the pBAD24 vector and transformed by electroporation into ΔdegS strains named ΔdegS+pBAD24-cyaA, ΔdegS+pBAD24-crp, ΔdegS+pBAD24-rpoS, and ΔdegS+pBAD24-xthA. To obtain double overexpression strains, the full-length coding region of crp was cloned into the pBAD33 vector and transformed by electroporation into a ΔdegS+pBAD24-cyaA high expression strain named ΔdegS+pBAD24/33-cyaA/crp (Sun et al., 2014). All complementary and overexpression strains were cultured in LB medium containing 0.1% arabinose to induce gene expression.
Quantitative real-time quantitative polymerase chain reaction (qRT-PCR)
All strains were grown in LB liquid medium until the middle of the exponential period, reaching an OD600 of 0.6. Total RNA was extracted using the TRIzol reagent. qRT-PCR assays were performed as previously described using 16S RNA as an internal reference gene (Huang et al., 2019). 16sRNA expression was also assessed in each group (Supplementary Figure 1). All experiments were repeated three times.
Hydrogen peroxide (H2O2) and cumene hydroperoxide (CHP) disk diffusion assay
The bacterial concentration was adjusted to to 1×108 CFU/mL at the middle logarithm and 100μL of bacterial solution was taken for plate coating. Six sterile circular filter paper plates were placed flat on the surface of LB medium. Using a pipette to 10μL containing 0 (control), 30, 50, 100, 200, and 300 mmol/L H2O2 and 2μL containing 0 (control), 30, 50, 100, 200, and 300 mmol/L CHP were added dropwise onto sterile circular filter paper plates and incubated overnight at 37°C, and the diameter of the inhibition circle was measured by vernier calipers (Wang et al., 2012). All experiments were repeated three times.
Hydrogen peroxide (H2O2) and cumene hydroperoxide (CHP) killing assay
The strains were cultured on LB plates at 37°C overnight. They were normalized to an OD600 of 1.0 in liquid medium. A 1:1,000 dilution of normalized culture was used to inoculate fresh AKI medium, incubated at 37°C for 2 hours with standing and 2h with shaking, and treated with final concentrations of 1, 5, 10, 20, and 30 mmol/L H2O2 and CHP for 2 hours. Gradient dilutions were performed and the cells were counted on LB plates. The survival rate of each bacterium was calculated by comparing the number of colonies with those of samples not treated with H2O2 and CHP (Wang et al., 2017). All experiments were repeated three times.
Survival under starvation stress assay
These strains were cultured overnight on LB plates at 37°C and normalized to 109 in fresh liquid medium with an OD600 of 1.0. A 1:1000 dilution of the normalized culture was used to inoculate the fresh AKI medium, which was then placed in an anaerobic bag for incubation. At the start of the anaerobic incubation, we used an anaerobic indicator to test the anaerobic incubation conditions with no oxygen, and the anaerobic incubation was done at 37°C for 4 hours and incubated overnight at 22°C in artificial seawater. To create artificial seawater, 40.0g of sea salt is dissolved in 1 liter of sterilized ddH2O. Dilution spot plates were sampled every 24 hours on LB plates. The survival rate of each bacterium was calculated by comparing the number of colonies with that of the non-anaerobically cultured colonies (Wang et al., 2017). All experiments were repeated three times.
Mouse intestinal colonization assay
An intestinal colonization model was established using 5- to 6-week-old CD1 mice. All animal experiments conducted in this study were approved by the Ethics Committee of the Affiliated Hospital of Zunyi Medical University. One hundred forty-four mice were randomly divided into two groups according to sex, with 72 mice in each group. One group was treated with 1% N-acetylcysteine (Amrouche-Mekkioui and Djerdjouri, 2012) and 0.4% aspartame (NAC+) in drinking water, while the other group was treated with 0.4% aspartame (NAC-) in drinking water for 1 week. Subsequently, the two groups of mice were randomly divided into nine groups (n=8). The mice were fed sterile water containing streptomycin (5 mg/mL) from the day before the experiment until the end of the experiment. Streptomycin pretreatment of adult mice allows for some intestinal ROS production and inhibits the colonization ability of normal intestinal host bacteria in the mice intestinal tract, allowing for better colonization of the mice gut by V.cholerae (Spees et al., 2013; Liu et al., 2016). After the strains were cultured to the exponential growth phase, the cells were collected by centrifugation at 1200rpm for 3 min. The bacterial precipitates were washed twice with 1×phosphate-buffered saline, and the bacterial suspension was adjusted to an OD600 of 0.5, approximately 1.0×108 CFU/mL. Each mouse was gavaged with 100μL (1×107CFU) of the cell suspension, and 100μL of 1×phosphate-buffered saline was used as a negative control. The inoculum dose was determined by pre-experimental exploration, and it was found that a high concentration (1×108 CFU per 100μL of bacterial solution) resulted in massive mortality in mice within 18 hours, whereas 1×107CFU per 100μL of bacterial solution had no significant effect. The mice were euthanized 18hours after gavage, and small intestinal tissues were dissected, weighed, and homogenized. The 100μL bacterial solution was diluted and coated onto LB plates containing streptomycin 0.01 mg/mL. The number of colonies was counted after overnight incubation and expressed as the logarithm of the number of colonies formed per gram of intestine (CFU/g).
Statistical analysis
Differences between two groups were analyzed using unpaired two-tailed t-tests, differences between multiple groups using one-way ANOVA, and multiple comparison tests using Bonferroni analysis, with P<0.05 considered statistically different for comparisons between groups. All experiments were performed on at least three independent replicates, and all values are expressed as mean ± SD.
Results
DegS enhances the antioxidant capacity of V. cholerae
Our previous GO analysis of differential genes suggested that the genes downregulated after degS knockout were mainly involved in processes such as oxidative stress (Huang et al., 2019), implying that DegS may affect the oxidative stress response in V. cholerae. To verify this hypothesis, we performed a disk diffusion assay to determine the resistance of the WT and degS mutants to different concentrations of hydrogen peroxide (H2O2) and cumene hydroperoxide (CHP). These results showed that the diameter of the inhibition zone of the degS mutant was significantly larger than that of the WT after treatment with both oxidants and that the inhibition zone of the revertant strain ΔdegS::degS was restored to a diameter close to that of the WT strain (Figures 1A, B). The pBAD24 empty plasmid did not restore the antioxidant capacity of ΔdegS. Additionally, we used different concentrations of H2O2 and the CHP killing assay, and the results showed that a significantly reduced survival rate of ΔdegS compared with that of the WT strain; the survival rate of ΔdegS::degS was partially restored, and the pBAD24 empty plasmid had no restorative effect (Figure 1C). These results indicate that the degS mutation leads to a reduction in the resistance of the non-O1/non-O139 V. cholerae strain HN375 to H2O2 and CHP.
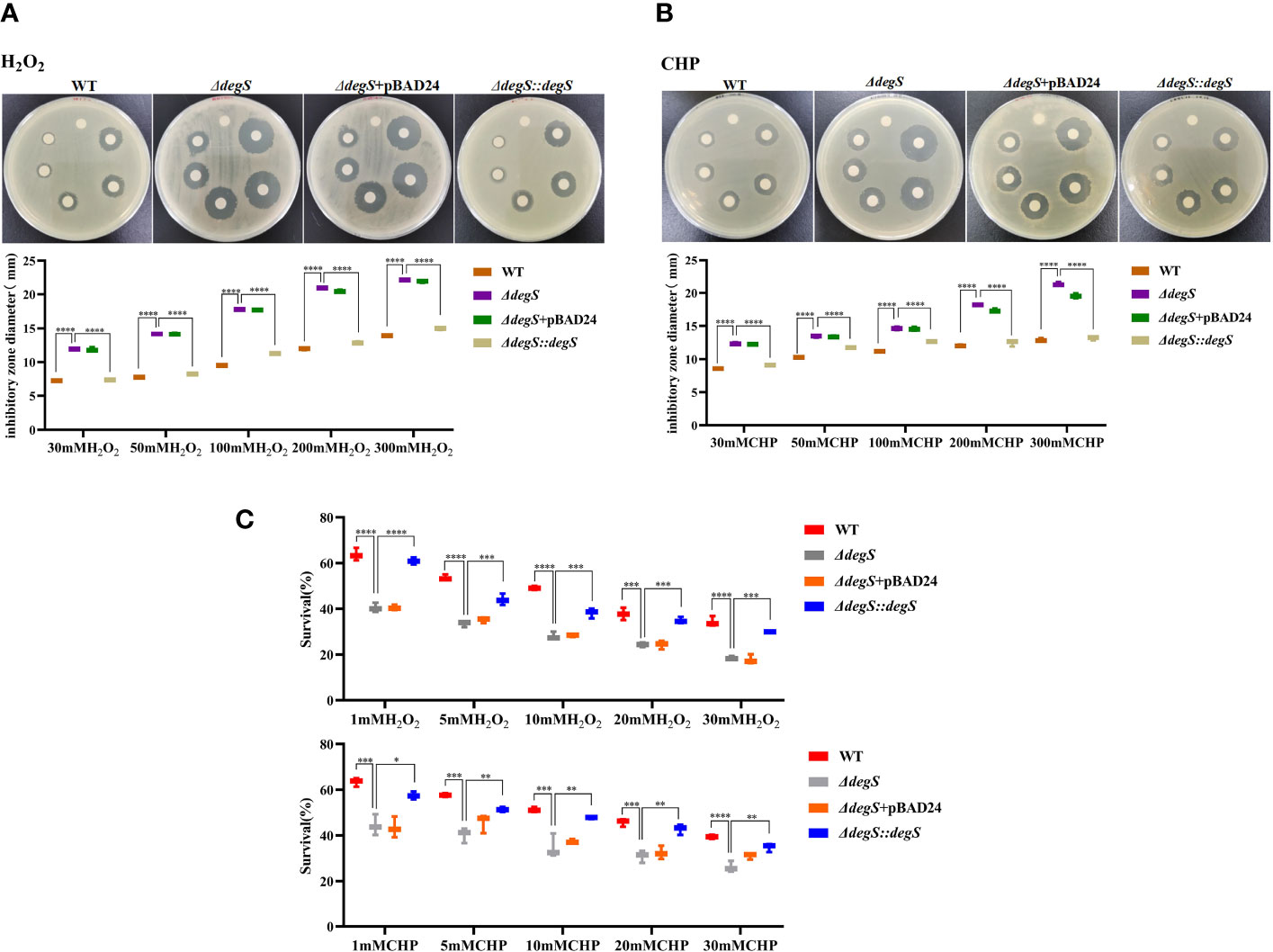
Figure 1 Effects of DegS on the antioxidant capacity of V. cholerae. (A, B) Sterile paper disk with different concentrations of H2O2 and CHP were placed on the corresponding strain plates for overnight incubation, with the oxidant concentrations ranging from small to large (0, 30, 50, 100, 200, and 300 mM) in a counterclockwise direction, and the diameter of the zone of inhibition was measured for each strain. (C) The killing assays were performed with 1, 5, 10, 20, and 30 mM H2O2 and CHP on the corresponding strains, and the survival rate of each strain was calculated by comparing the CFU of the treated and untreated samples. Data are means and standard deviations of three replicate experiments and were analyzed using one-way analysis of variance (ANOVA). *, P<0.05; **, P<0.01; ***, P<0.001; ****, P<0.0001.
The positive regulation of V. cholerae antioxidant capacity by DegS is partially through σE
DegS is the initiator of the σE stress response pathway and plays an important role in the regulation of stress response in E. coli (Bongard et al., 2019). RseA binds to σE as an anti-σ factor, preventing its release, and when the DegS protease is activated, the periplasmic structural domain of RseA is cleaved by DegS, thereby releasing σE to regulate the transcription of related stress-responsive genes (Kim, 2015). To investigate whether DegS influences antioxidant capacity through σE, we constructed a V. cholerae rpoE deletion mutant (ΔrpoE), and a double knockout strain ΔdegSΔrseA to revert to σE activity. qRT-PCR showed significantly reduced rpoE gene transcript levels in ΔdegS than in the WT strain, and the ΔdegSΔrseA strain could revert to rpoE levels close to those of WT (Figure 2A). The diameter of the inhibition zone of the ΔrpoE strain was significantly greater than that of the WT strain under both oxidant treatments, and the diameter of the inhibition zone of the ΔdegSΔrseA strain was able to revert to close to that of the WT strain (Figure 2B). The survival rate of the ΔrpoE strain in the H2O2 and CHP killing assay was significantly lower than that of the WT strain, and ΔdegSΔrseA strain restored close to the level of the WT strain (Figure 2C). These results suggest that DegS may positively regulates the antioxidant capacity of V. cholerae partially through σE.
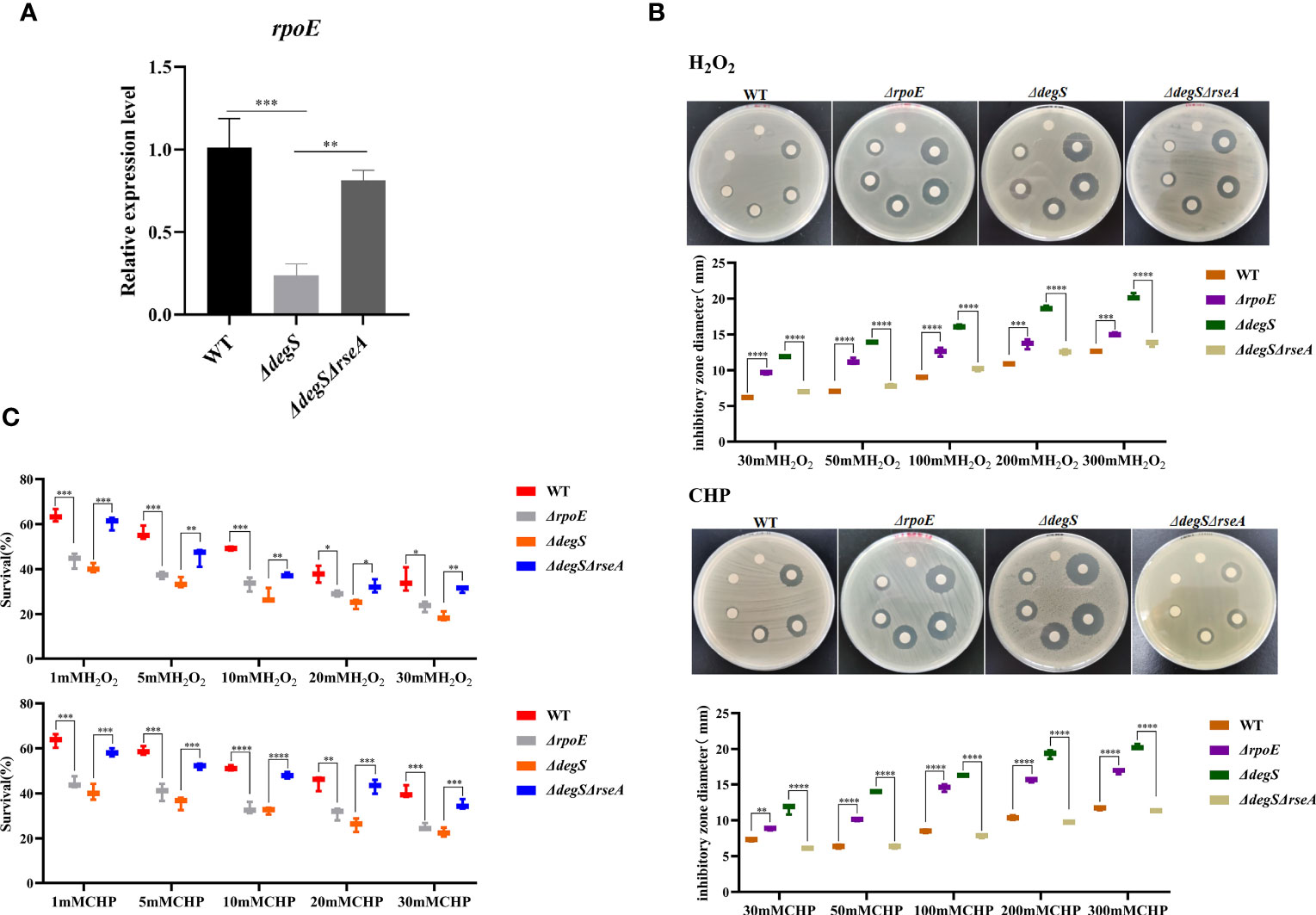
Figure 2 DegS regulates the antioxidant capacity of V. cholerae through σE. (A) Comparison of the relative expression levels of rpoE genes in different strains with 16sRNA as an internal reference gene (unpaired t-test). (B) Sterile paper disk with different concentrations of H2O2 and CHP were placed on the corresponding strain plates for overnight incubation, with the oxidant concentrations ranging from small to large (0, 30, 50, 100, 200, and 300 mM) in a counterclockwise direction, and the diameter of the zone of inhibition was measured for each strain. (C) The killing assays were performed with 1, 5, 10, 20, and 30 mM H2O2 and CHP on the corresponding strains, and the survival rate of each strain was calculated by comparing the CFU of the treated and untreated samples. Data are means and standard deviations of three replicate experiments and were analyzed using one-way analysis of variance (ANOVA). *, P<0.05; **, P<0.01; ***, P<0.001; ****, P<0.0001.
Regulation of antioxidant capacity of V. cholerae by DegS may involve the regulation of RpoS by the cAMP-CRP complex
Previous studies have shown that the cAMP-CRP-RpoS pathway plays a vital role in bacterial oxidative stress (Cheng and Sun, 2009). Gene-Act network analysis showed that the cAMP-CRP-RpoS pathway was significantly inhibited in degS mutants (Huang et al., 2019). CyaA encodes adenylate cyclase, which uses ATP as a substrate to synthesize cAMP, and crp encodes the cAMP receptor protein (CRP) (Emmer et al., 1970). qRT-PCR data showed that DegS and σE knockouts suppressed cyaA and crp expression at the transcriptional level (Figure 3A). To explore whether DegS regulates the antioxidant capacity of V. cholerae via cAMP and CRP, ΔdegS+pBAD24-cyaA, ΔdegS+pBAD24-crp and ΔdegS+pBAD24/33-cyaA/crp strains were constructed for H2O2 and CHP disk diffusion and killing assays. The results showed that ΔdegS+pBAD24-cyaA and ΔdegS+pBAD24-crp strains only partially recovered their inhibition zone diameter compared with ΔdegS strains, and, importantly, ΔdegS+pBAD24/33-cyaA/crp mostly recovered their inhibition zone diameter compared with ΔdegS strains (Figure 3B). In the killing assays, ΔdegS+pBAD24-cyaA and ΔdegS+pBAD24-crp were unable to revert survival compared with the ΔdegS strain, whereas the ΔdegS+pBAD24/33-cyaA/crp strain mostly reverted survival (Figure 3C). These results suggest that the regulation of antioxidant capacity by DegS in V. cholerae involves the synergistic action with cAMP-CRP complex.
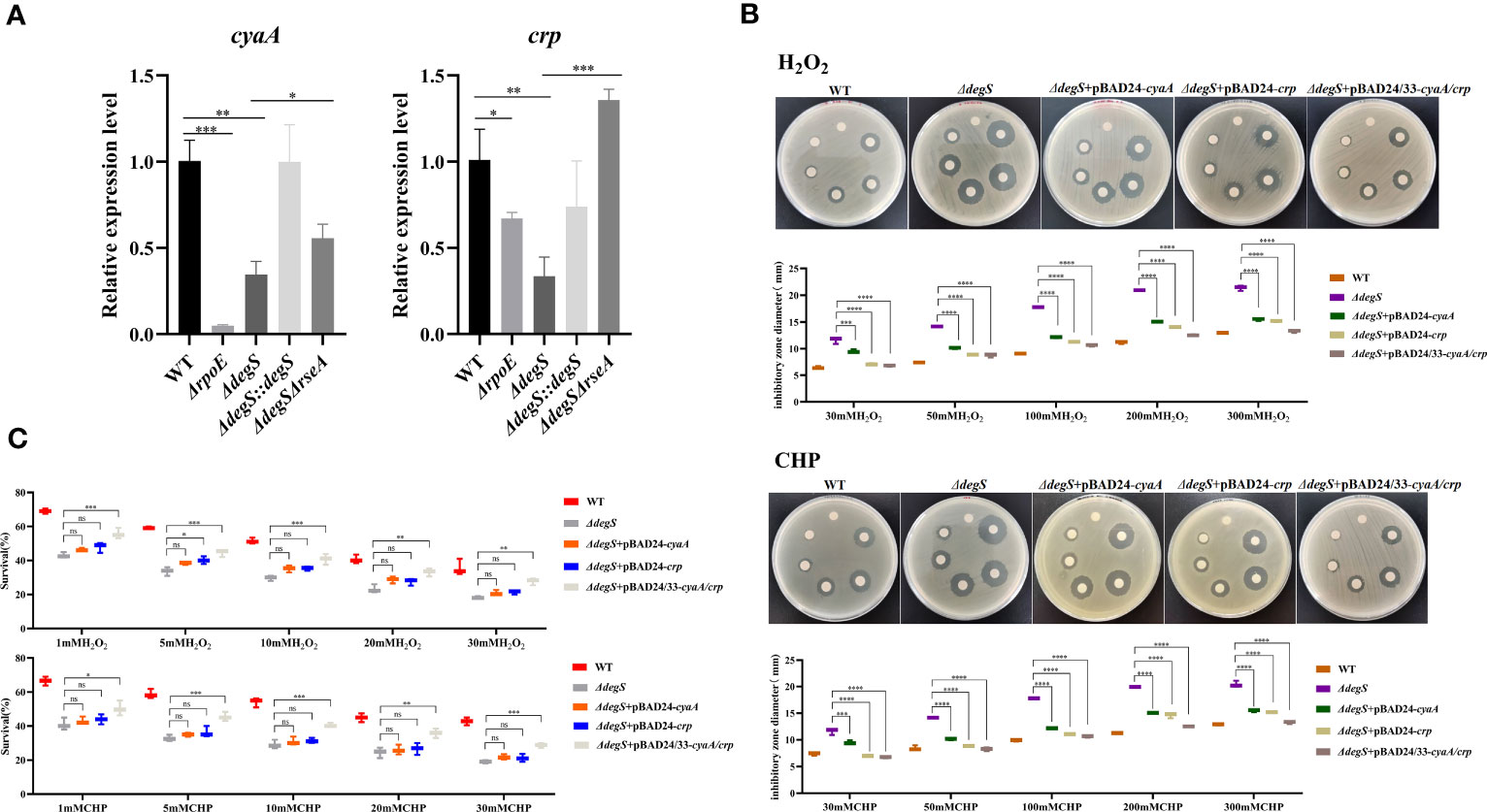
Figure 3 DegS regulation of antioxidant capacity in V. cholerae may depend on the cAMP-CRP complex. (A) The relative expression levels of cyaA and crp genes of different strains were compared, and 16sRNA was used for the internal reference gene (unpaired t-tests). (B) Sterile paper disk with different concentrations of H2O2 and CHP were placed on the corresponding strain plates for overnight incubation, with the oxidant concentrations ranging from small to large (0, 30, 50, 100, 200, and 300 mM) in a counterclockwise direction, and the diameter of the zone of inhibition was measured for each strain. (C) The killing assays were performed with 1, 5, 10, 20, and 30 mM H2O2 and CHP on the corresponding strains, and the survival rate of each strain was calculated by comparing the CFU of the treated and untreated samples. Data are means and standard deviations of three replicate experiments and were analyzed using one-way analysis of variance (ANOVA). *, P<0.05; **, P<0.01; ***, P<0.001; ****, P<0.0001, ns indicates no statistical significance.
V. cholerae with rpoS knockout is more sensitive to oxidative stress (Wurm et al., 2017). The cAMP-CRP complex is a transcriptional activator of rpoS (Guo et al., 2015). Our qRT-PCR experiments showed that DegS, σE, cAMP and CRP affected rpoS transcription (Figure 4A). To explore whether DegS antioxidation is dependent on RpoS, we constructed a rpoS gene deletion strain (ΔrpoS) and restored rpoS overexpression in ΔdegS strain (ΔdegS+pBAD24-rpoS). Compared with the WT, ΔrpoS showed an enlarged inhibition zone diameter in the disk diffusion assay and a significantly reduced survival rate in the killing assay (Figures 4B, C). Compared with the ΔdegS strain, ΔdegS+pBAD24-rpoS strain showed a reduced inhibition zone diameter in the disk diffusion assay and a significantly increased survival rate in the killing assay (Figures 4B, C). These results suggest that DegS enhances the antioxidant capacity of V. cholerae through positive regulation of RpoS transcription by cAMP-CRP.
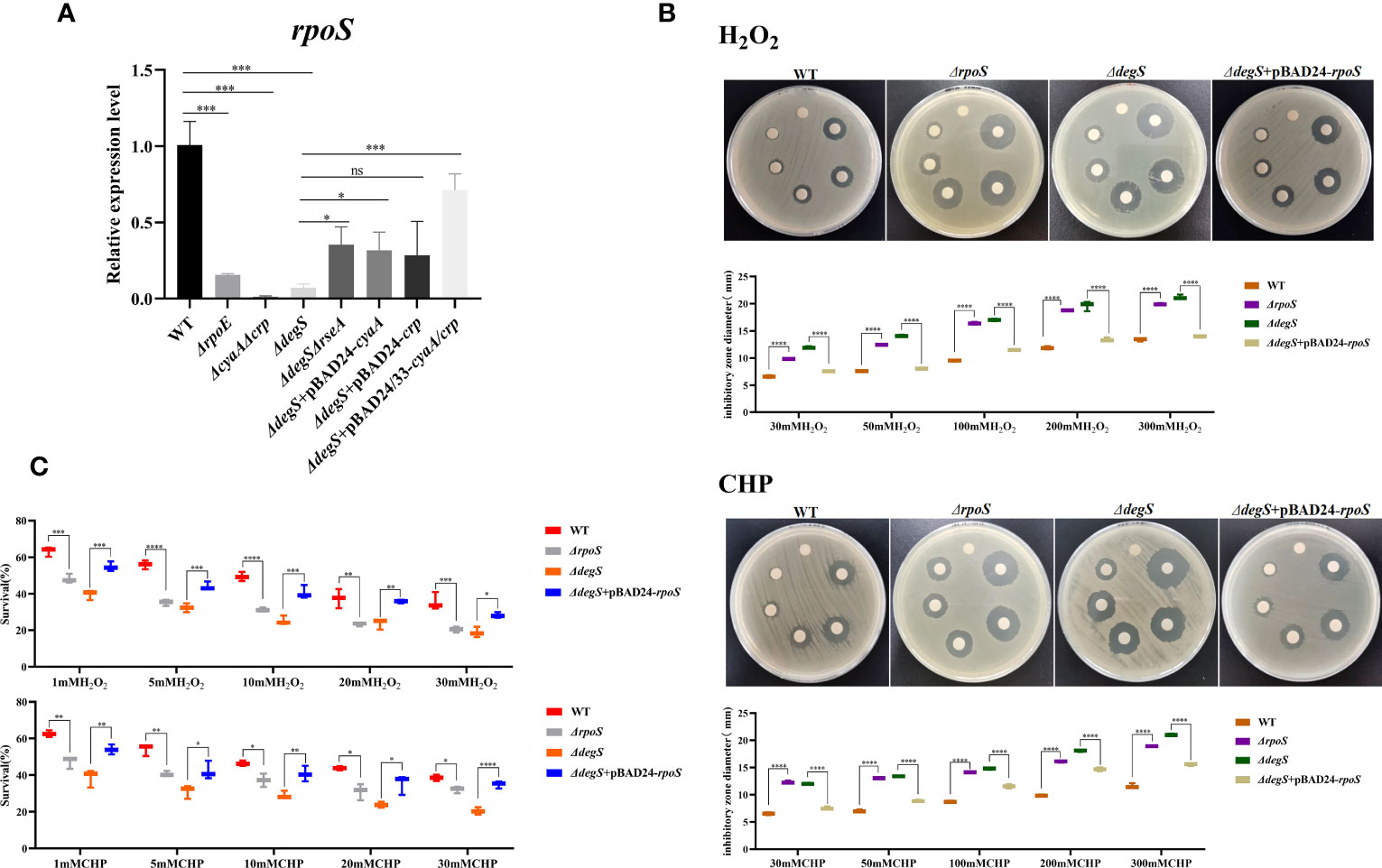
Figure 4 DegS regulation of antioxidant capacity in V. cholerae may depend on the regulation of RpoS by the cAMP-CRP complex. (A) The relative expression levels of rpoS gene of different strains were compared, and 16sRNA was used for the internal reference gene (unpaired t-tests). (B) Sterile paper disk with different concentrations of H2O2 and CHP were placed on the corresponding strain plates for overnight incubation, with the oxidant concentrations ranging from small to large (0, 30, 50, 100, 200, 300) in a counterclockwise direction, and the diameter of the zone of inhibition was measured for each strain. (C) The killing assay were performed with 1, 5, 10, 20, 30 mM H2O2 and CHP on the corresponding strains, and the survival rate of each strain was calculated by comparing the CFU of the treated and untreated samples. Data are means and standard deviations of three replicate experiments and were analyzed using one-way analysis of variance (ANOVA). *, P<0.05; **, P<0.01; ***, P<0.001; ****, P<0.0001, ns indicates no statistical significance.
Regulation of antioxidant capacity of V. cholerae by DegS involves the expression of XthA
We used qRT-PCR to screen genes with antioxidant capacity regulated by RpoS, including the nucleic acid exonuclease III xthA and catalase katG genes (Barth et al., 2009; Meireles et al., 2022). Moreover, the organic hydroperoxide stress resistance regulator OhrR was also examined (Barth et al., 2009; Meireles et al., 2022). These results showed that xthA gene transcript levels were downregulated in ΔdegS, ΔrpoE, ΔcyaAΔcrp, and ΔrpoS strains compared with WT, while xthA gene transcript levels were mostly reverted in ΔdegS::degS, ΔdegSΔrseA, ΔdegS+pBAD24/33-cyaA/crp, and ΔdegS+pBAD24-rpoS strains compared with WT (Figure 5A). The katG and ohrR gene transcript levels were downregulated in the ΔdegS, ΔrpoE, ΔcyaAΔcrp, and ΔrpoS strains compared with the WT, and partially reverted in ΔdegS+pBAD24/33-cyaA/crp, but not in the ΔdegSΔrseA and ΔdegS+pBAD24-rpoS strains (Figure 5A). Therefore, we speculated that rpoS exerts its antioxidant function in DegS by regulating xthA at the transcriptional level. Therefore, we overexpressed xthA in the ΔdegS mutant (ΔdegS+pBAD24-xthA). Compared with the ΔdegS strain, the inhibition zone diameter of the ΔdegS+pBAD24-xthA strain decreased (Figure 5B), and the killing survival rate of the ΔdegS+pBAD24-xthA strain significantly increased (Figure 5C). Taken together, these results suggest that regulation of the antioxidant capacity of V. cholerae by DegS involves XthA expression.
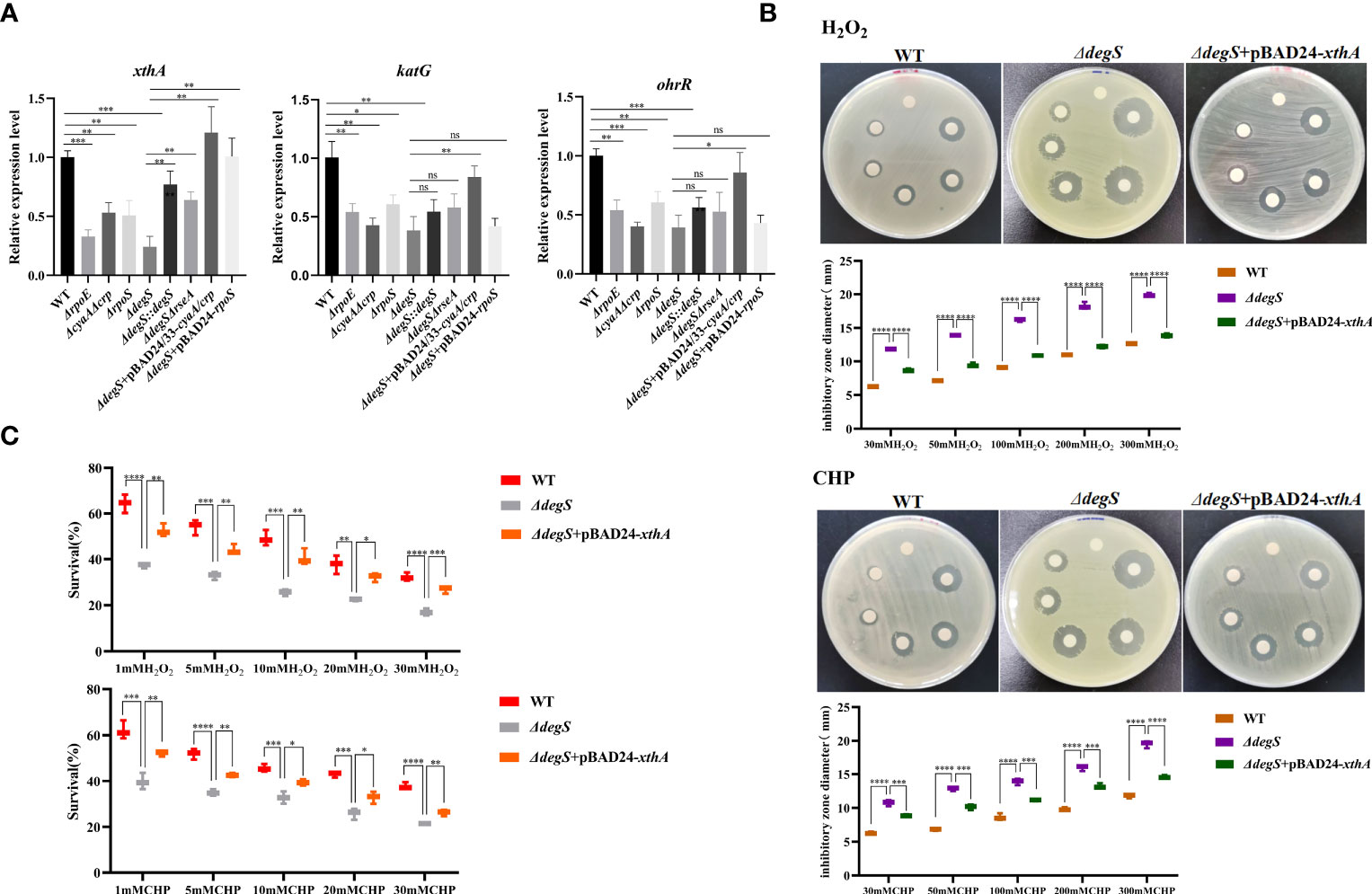
Figure 5 Regulation of antioxidant capacity of V. cholerae by DegS is related to XthA. (A) The relative expression levels of xthA, katG, and ohrR genes in different strains were compared, using 16sRNA as an internal reference gene (unpaired t-test). (B) Sterile paper disk with different concentrations of H2O2 and CHP were placed on the corresponding strain plates for overnight incubation, with the oxidant concentrations ranging from small to large (0, 30, 50, 100, 200, and 300 mM) in a counterclockwise direction, and the diameter of the zone of inhibition was measured for each strain. (C) The killing assays were performed with 1, 5, 10, 20, and 30 mM H2O2 and CHP on the corresponding strains, and the survival rate of each strain was calculated by comparing the CFU of the treated and untreated samples. Data are means and standard deviations of three replicate experiments and were analyzed using one-way analysis of variance (ANOVA). *, P<0.05; **, P<0.01; ***, P<0.001; ****, P<0.000; ns, no statistical significance.
DegS contributes to V. cholerae adaptation to starvation stress
Encountering a lack of nutrients in aquatic environments is an environmental stress that V.cholerae regularly encounters in natural ecosystems. Therefore, it is very important for V.cholerae adapt to the starvation stress. To explore whether DegS helps V.cholerae cope with starvation stress, we tested whether DegS is important for V.cholerae survival in artificial seawater. To imitate the process of V.cholerae starvation stress, we cultured the WT strain and mutants under oxygen-limited conditions at 37°C, and then transferred the bacterial cells to artificial seawater without a carbon source at 22°C. The ΔdegS strain showed a significantly lower survival rate than the WT strain (Figure 6A). The ΔdegS::degS strain showed a significantly higher survival rate than that of the ΔdegS strain. The ΔdegS+pBAD24 strain exhibited phenotypic characteristics similar to those of the ΔdegS strain. The ΔrpoE strain showed a significantly lower survival rate compared to the WT, and ΔdegSΔrseA showed a survival rate close to that of the WT (Figure 6B). Compared with ΔdegS, ΔdegS+pBAD24-cyaA and ΔdegS+pBAD24-crp showed no significant increase in survival rate. However, the ΔdegS+pBAD24/33-cyaA/crp strain showed a significant increase in survival compared with the ΔdegS strain (Figure 6C). The survival rate of the ΔrpoS strain was significantly lower than that of the WT. The survival rate of ΔdegS+pBAD24-rpoS and ΔdegS+pBAD24-xthA were both significantly higher than that of ΔdegS (Figure 6D). These findings indicate that DegS is important in alleviating low levels of starvation stress in the environment, and that this role correlates with σE, cAMP-CRP, and RpoS.
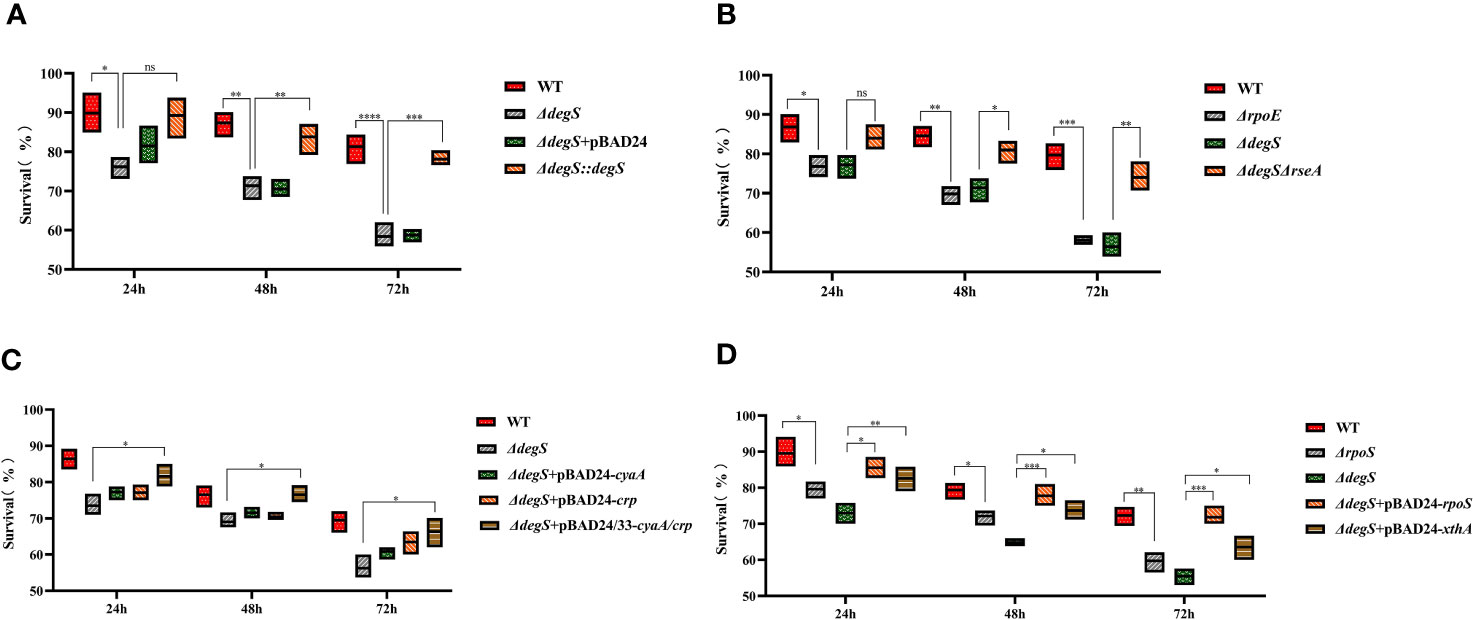
Figure 6 Effect of DegS on V. cholerae adaptation to starvation stress. (A-D) The bacteria were collected after anaerobic culture of the different strains and suspended in artificial seawater for 24, 48, and 72 hours by serial dilution and the number of viable cells was counted. Data are means and standard deviations of three replicate experiments and were analyzed using one-way analysis of variance (ANOVA). *, P<0.05; **, P<0.01; ***, P<0.001; ****, P<0.0001; ns, no statistical significance.
DegS affects intestinal colonization ability of V.cholerae with or without the antioxidant
To verify the effect of DegS on the intestinal colonization ability of V. cholerae under different oxidative stresses, the use of streptomycin allows for some oxidative stress in the mice intestine and promotes better colonization of the mice gut by V. cholerae (Spees et al., 2013; Liu et al., 2016), and employed N-acetylcysteine (NAC) as an antioxidant to reduce intestinal oxidative stress levels (Amrouche-Mekkioui and Djerdjouri, 2012). The mice were divided into two groups: a non-antioxidant group (NAC-) and an antioxidant group (NAC+). The results showed that in the NAC+ group, the ΔdegS, ΔrpoE, and ΔrpoS strains showed a significant decrease in colonization capacity compared with the WT strain (Figure 7). Interestingly, the colonization ability of ΔdegS, ΔrpoE, and ΔrpoS strains in NAC-treated mice was significantly higher than that of the untreated group. Compared with the ΔdegS strain, the colonization ability of the ΔdegS::degS, ΔdegSΔrseA, ΔdegS+pBAD24-rpoS, and ΔdegS+pBAD24-xthA strains was partially restored. In addition, we found no significant difference in the colonization ability of WT, ΔdegS::degS, ΔdegSΔrseA, ΔdegS+pBAD24-rpoS, and ΔdegS+pBAD24-xthA strains between the NAC- and NAC+ groups of mice. These results suggest that σE, RpoS, and XthA are involved in DegS-mediated V. cholerae colonization under different oxidative stress conditions.
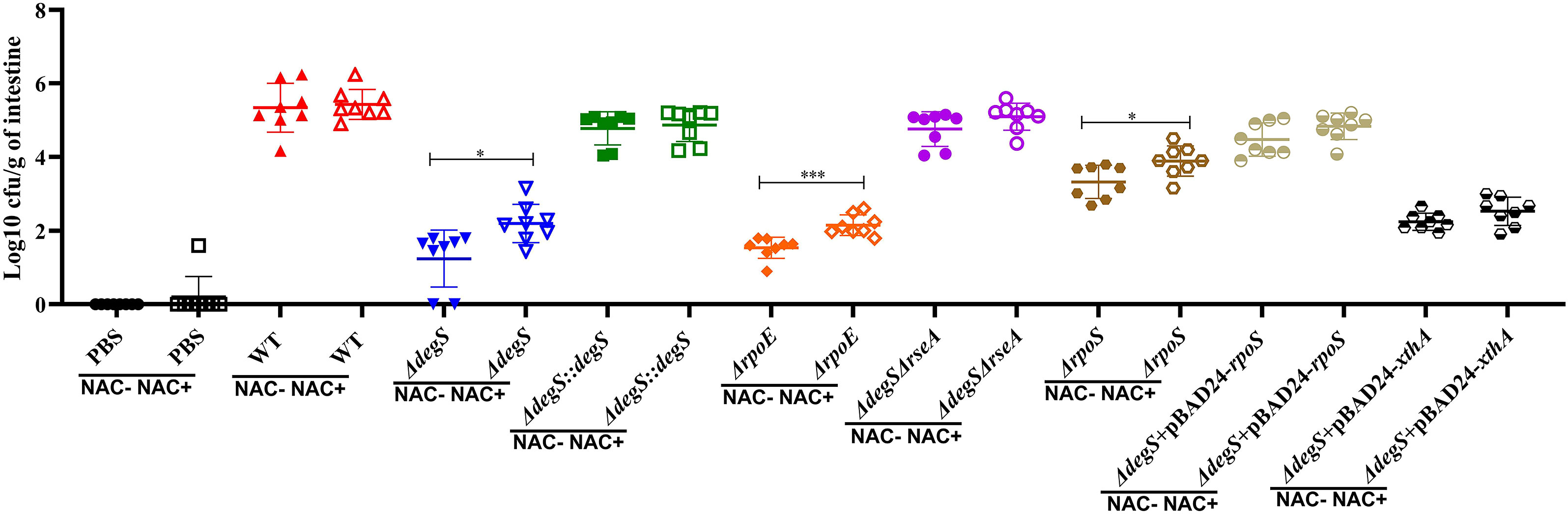
Figure 7 DegS affects the ability of V. cholerae to colonize the intestine under oxidative stress. Approximately 107 cells of the different strains were inoculated by gavage in 5-6-week-old CD1 mice and incubated for 18 hours. The logarithm of colony-forming units per gram of intestine was detected by serial dilution and LB agar plates (CFU/g; mean± SD, n = 8). The data were analyzed by unpaired t test. *, P<0.05; ***, P<0.001.
Discussion
Adapting to oxidative stress is crucial for the survival of V. cholerae, both in the aquatic ecosystem and within the host. When a pathogen infects a host, it can generate large amounts of ROS to protect itself from and eliminate the invading pathogen. Pathogens have developed antioxidant mechanisms to resist the deleterious effects of ROS. DegS is an influential factor in the virulence of extraintestinal infections caused by E. coli (Redford et al., 2003). We performed GO enrichment analysis of differential genes after degS knockout and showed that some of the downregulated genes were involved in the oxidative stress response. In this study, we demonstrated that DegS plays a significant role in the antioxidant capacity of V. cholerae and proposed a model in which DegS protease influences the expression of the nucleic acid exonuclease III xthA gene through the cAMP-CRP-rpoS signaling pathway, and thus regulating the antioxidant capacity and adaptation to the oxidative stress environment of V.cholerae (Figure 8).
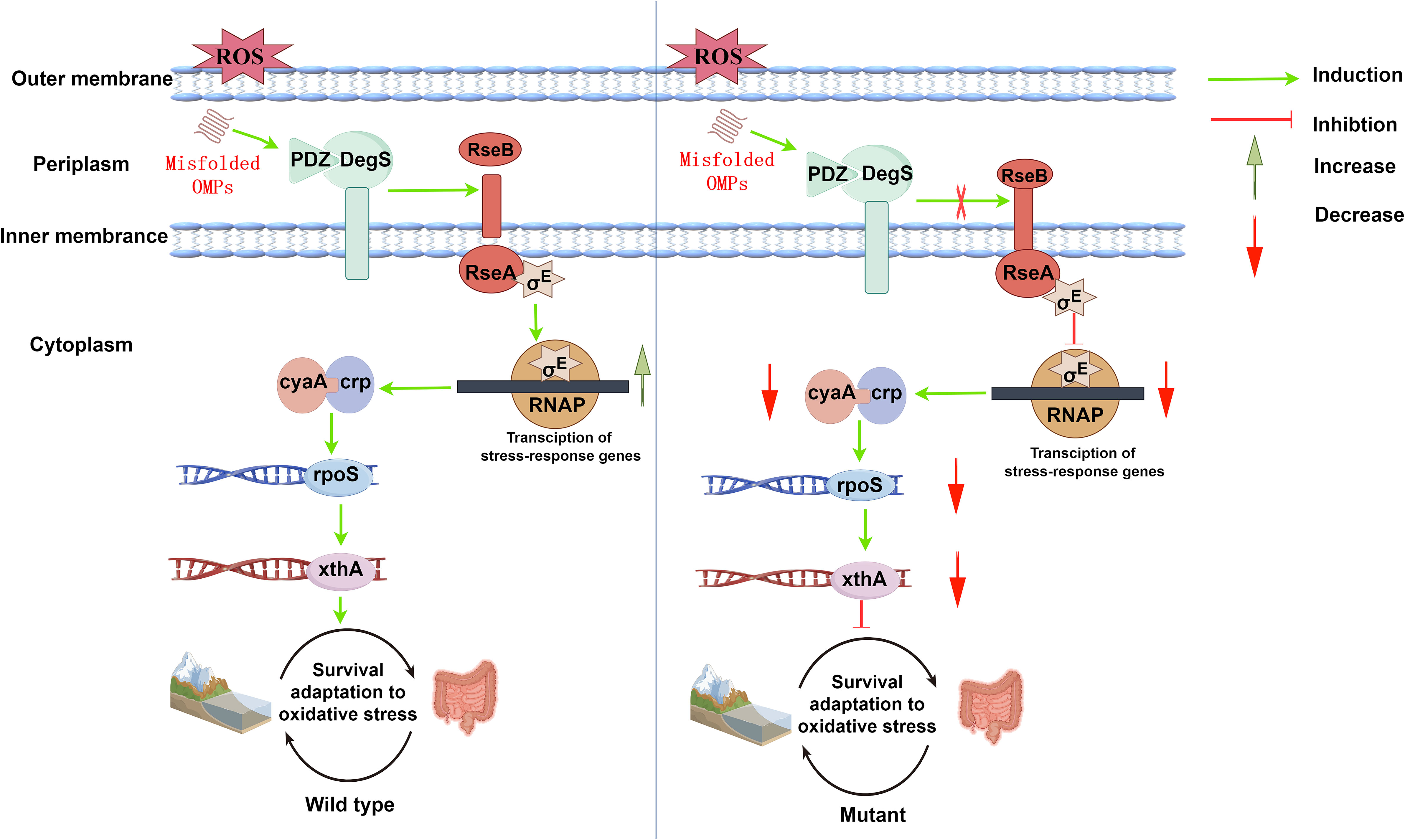
Figure 8 A proposed model showing the process by which DegS positively regulates V.cholerae antioxidant capacity and adaptation to oxidative stress environments. Under oxidative stress (wild type), the PDZ domain of DegS binds to a large number of misfolded outer membrane proteins and promotes RseA cleavage to release active σE. Subsequently, xthA expression is induced via the cAMP-CRP-RpoS signaling pathway, initiating bacterial oxidative damage repair. Under oxidative stress (degS gene deletion mutation), RseA cleavage is inhibited, the number of σE and cAMP-CRP-RpoS responsive protein complexes is reduced, and rpoS and xthA transcription is down-regulated, resulting in the blockage of initiating oxidative damage repair, and a decrease in antioxidant capacity and adaptation to the oxidative stress environment. Red arrows indicate inhibition, and downward-pointing red arrows indicate reduction. Green arrows indicate induction, and upward-pointing green arrows indicate an increase.
DegS, a serine protease present in bacterial periplasm, plays an important role in the σE stress response system (Kim, 2015). Bacterial sensing of external stresses leads to the production of unfolded or misfolded proteins in the outer membrane, which bind to the PDZ structural domain of DegS protease and activate DegS protease activity, hydrolyzing the anti-σ factor RseA protein, thereby releasing σE (Hasselblatt et al., 2007). σE, encoded by the rpoE gene, is a crucial factor for the envelope stress response in E. coli (Barth et al., 2009). In addition, σE also involves the regulation of bacterial antioxidant capacity. Salmonella rpoE mutants exhibit markedly elevated vulnerability to H2O2 (Testerman et al., 2002; Nuss et al., 2013). Our study demonstrates that the antioxidant capacity of V. cholerae is reduced when degS and rpoE are mutated, whereas the ΔdegSΔrseA strain can partially restore antioxidant activity by compensating σE activity.
To further investigate the regulatory pathways through which DegS affects the antioxidant capacity of V. cholerae, our RNA-seq analysis suggested that the cAMP-CRP-RpoS pathway was significantly inhibited after degS mutation, and related studies have shown that this pathway plays an antioxidant role in Salmonella (Cheng and Sun, 2009). Once CRP binds to cAMP to form a cAMP-CRP complex, the altered conformation of CRP can recognize and bind to specific sequences, thereby activating or inhibiting the expression of downstream genes (Manneh-Roussel et al., 2018). In E. coli, more than 7% of genes are regulated by the cAMP-CRP complex (Zheng et al., 2004). For instance, cAMP-CRP controls the supply of NADH to respiratory chain redox enzymes in central carbon catabolism (glycolysis and TCA cycles) and further controls the production of hydrogen peroxide (Kashyap et al., 2017). Our data revealed that cAMP and CRP affect the transcription of antioxidant-related genes in V. cholerae. Impaired antioxidant activity in degS mutants was partially reversed by overexpression of cyaA and crp. These results suggest that DegS affects the antioxidant capacity of V. cholerae possibly by regulating the cAMP-CRP complex. The cAMP-CRP complex is a transcriptional activator of rpoS (Guo et al., 2015). RpoS is a global transcriptional regulator involved in the regulation of oxidative stress (Chiang and Schellhorn, 2012). Our results indicate that the regulation of antioxidant capacity by DegS in V. cholerae may involve the cAMP-CRP complex, which regulates rpoS.
XthA is a nucleic acid exonuclease whose gene product enables the repair of oxidatively damaged cells (Nguyen et al., 1993). XthA transcription is dependent on rpoS in E. coli (Chiang and Schellhorn, 2012). RpoS exerts its antioxidant effects through the xthA gene (Charoenwong et al., 2011), which plays a dominant role in oxidative stress to hypochlorous acid (HOCl) and H2O2 stress in rpoS high expression strains (Barth et al., 2009). Here, qRT-PCR showed that DegS, σE, cAMP-CRP, and RpoS positively regulate xthA gene transcription. XthA overexpression partially compensates the sensitivity of degS mutants to H2O2 and CHP oxidants. XthA cleaves the phosphodiester backbone of DNA upstream and removes the phosphoglycolic acid residues produced by the oxidation of the ribose portion of DNA, thus helping repair oxidized bases (Demple et al., 1986; Gupta and Imlay, 2022). Based on these findings, we propose that the effect of DegS on the antioxidant capacity of V. cholerae through cAMP-CRP-RpoS may be related to XthA.
In the mouse intestinal colonization experiment, we divided the mice into two groups, non-antioxidant (NAC-) and antioxidant (NAC+), and used antioxidant treatment to reduce oxidative stress in the mouse intestine. The rpoS mutation significantly affects the ability of V. cholerae to colonize the intestinal tract (Merrell et al., 2000). Decreased V. cholerae intestinal colonization in suckling mice is caused by mutations in the rpoE gene (Kovacikova and Skorupski, 2002). Our findings suggest that V. cholerae degS, rpoE, and rpoS deletions are associated with significantly reduced resistance to oxidative stress and the ability to colonize mice intestines. In addition, all subgroups treated with NAC showed an increase in the number of colonies in the intestine compared with the untreated group, particularly in ΔdegS, ΔrpoE, and ΔrpoS. The above results suggest that DegS-mediated antioxidant and mouse intestinal colonization capacity of V. cholerae correlates possible with σE and RpoS. Compared with the NAC- group, the ability of the ΔdegS, ΔrpoE, and ΔrpoS strains to colonize the mouse intestine could be partially restored after the reduction of oxidative stress in the NAC+ group, but not to a level close to that of the wild-type, suggesting that adaptation to the oxidative stress environment is only a part of the V. cholerae’s ability to colonize the intestine. The main reason for this is that in NAC untreated, the ΔdegS, ΔrpoE, and ΔrpoS mutant strains significantly reduced V. cholerae’s ability to colonize the mice intestine, which is in agreement with our previous findings (Zou et al., 2023). However, in the present study, we also found that with and without NAC treatment, WT, ΔdegS::degS, ΔdegSΔrseA, ΔdegS+ pBAD24-rpoS, and ΔdegS+ pBAD24-xthA strains did not differ significantly in their ability to colonize the gut. Importantly, the colonization ability of the mutant strains was partially increased by NAC treatment, suggesting that the adaptation and colonization ability of ΔdegS, ΔrpoE, and ΔrpoS to the intestinal environment were improved to a certain extent after the reduction of the oxidative stress in the intestinal tract of the mice by NAC treatment.
The report by Davies et al. suggests that the xthA mutant does not affect V. cholerae colonization, which is inconsistent with our study. Our study showed that replenishment of the xthA gene in the ΔdegS strain by the pBAD24-xthA plasmid partially restored its ability to colonize the mouse intestine in the absence of NAC treatment. Compared with the NAC untreated group, the colonization ability of the ΔdegS strain increased after NAC treatment, while there was no significant difference in the colonization ability of the ΔdegS +pBAD24-xthA strain, suggesting that the diminished colonization ability of the ΔdegS strain was only partially affected by the oxidative stress environment. XthA overexpression could eliminate the influence of oxidative stress, and could not revert to the influence of other factors, including motility, chemotaxis on intestinal colonization (Zou et al., 2023).The differences with the Davies study may be due to the different strains we used and the different mice models.
Conclusion
In this study, we demonstrated that the deletion of degS may attenuate the antioxidant capacity of V. cholerae through rpoE, which in turn affects V. cholerae survival in aquatic environments and colonization in the mice gut. In addition, our results revealed a potential mechanism that may involve the affect of XthA expression through the cAMP-CRP-RpoS pathway. In conclusion, these findings provide novel evidence for our understanding of environmental adaptation and DegS biological function in V. cholerae and provide novel insights into the regulation of antioxidant activity in V. cholerae. However, the regulation of cAMP-CRP-RpoS and XthA by DegS-σE requires further investigation.
Data availability statement
The datasets presented in this study can be found in online repositories. The names of the repository/repositories and accession number(s) can be found in the article/Supplementary Material.
Ethics statement
The animal study was approved by Ethics Committee of the affiliated Hospital of Zunyi Medical University. The study was conducted in accordance with the local legislation and institutional requirements.
Author contributions
KW: Writing – original draft, Writing – review & editing, Data curation, Formal Analysis, Methodology. HL: Writing – review & editing, Data curation, Formal Analysis, Methodology. MZ: Data curation, Writing – review & editing, Formal Analysis, Methodology. GW: Writing – review & editing, Methodology, Formal Analysis. JZ: Writing – review & editing, Methodology. XH: Writing – review & editing, Methodology. FR: Methodology, Writing – review & editing. HH: Writing – review & editing, Methodology. JH: Writing – original draft, Writing – review & editing, Conceptualization, Funding acquisition, Project administration, Resources, Supervision. XM: Writing – review & editing, Conceptualization, Funding acquisition, Project administration, Resources, Supervision.
Funding
The author(s) declare financial support was received for the research, authorship, and/or publication of this article. This work was supported by grants from the National Natural Science Foundation of China [No. 32060035], the Science and Technology Project of Guizhou [Qiankehejichu-ZK[2021]470] and Research and Talent Training Project of Guizhou Moutai Hospital (MTyk 2022-08).
Conflict of interest
The authors declare that the research was conducted in the absence of any commercial or financial relationships that could be construed as a potential conflict of interest.
Publisher’s note
All claims expressed in this article are solely those of the authors and do not necessarily represent those of their affiliated organizations, or those of the publisher, the editors and the reviewers. Any product that may be evaluated in this article, or claim that may be made by its manufacturer, is not guaranteed or endorsed by the publisher.
Supplementary material
The Supplementary Material for this article can be found online at: https://www.frontiersin.org/articles/10.3389/fcimb.2023.1290508/full#supplementary-material
References
Amrouche-Mekkioui, I., Djerdjouri, B. (2012). N-acetylcysteine improves redox status, mitochondrial dysfunction, mucin-depleted crypts and epithelial hyperplasia in dextran sulfate sodium-induced oxidative colitis in mice. Eur. J. Pharmacol. 691, 209–217. doi: 10.1016/j.ejphar.2012.06.014
Barth, E., Gora, K. V., Gebendorfer, K. M., Settele, F., Jakob, U., Winter, J. (2009). Interplay of cellular cAMP levels, {sigma}S activity and oxidative stress resistance in Escherichia coli. Microbiol. (Reading England) 155, 1680–1689. doi: 10.1099/mic.0.026021-0
Bhattacharyya, S., Ghosh, S., Shant, J., Ganguly, N. K., Majumdar, S. (2004). Role of the W07-toxin on Vibrio cholerae-induced diarrhoea. Biochim. Biophys. Acta 1670, 69–80. doi: 10.1016/j.bbagen.2003.10.016
Bongard, J., Schmitz, A. L., Wolf, A., Zischinsky, G., Pieren, M., Schellhorn, B., et al. (2019). Chemical validation of degS as a target for the development of antibiotics with a novel mode of action. ChemMedChem 14, 1074–1078. doi: 10.1002/cmdc.201900193
Charoenwong, D., Andrews, S., Mackey, B. (2011). Role of rpoS in the development of cell envelope resilience and pressure resistance in stationary-phase Escherichia coli. Appl. Environ. Microbiol. 77, 5220–5229. doi: 10.1128/aem.00648-11
Chautrand, T., Souak, D., Chevalier, S., Duclairoir-Poc, C. (2022). Gram-negative bacterial envelope homeostasis under oxidative and nitrosative stress. Microorganisms 10 (5), 924. doi: 10.3390/microorganisms10050924
Chen, J., Huang, J., Huang, M., Chen, Z., Chen, A., Yang, J., et al. (2020). Two cases of septic shock with different outcomes caused by non-O1/non-O139 Vibrio cholerae isolates. J. Int. Med. Res. 48, 300060520933459. doi: 10.1177/0300060520933459
Cheng, Y., Sun, B. (2009). Polyphosphate kinase affects oxidative stress response by modulating cAMP receptor protein and rpoS expression in Salmonella typhimurium. J. Microbiol. Biotechnol. 19, 1527–1535. doi: 10.4014/jmb.0903.03030
Chiang, S. M., Schellhorn, H. E. (2012). Regulators of oxidative stress response genes in Escherichia coli and their functional conservation in bacteria. Arch. Biochem. Biophys. 525, 161–169. doi: 10.1016/j.abb.2012.02.007
Clemens, J. D., Nair, G. B., Ahmed, T., Qadri, F., Holmgren, J. (2017). Cholera. Lancet (London England) 390, 1539–1549. doi: 10.1016/s0140-6736(17)30559-7
Demple, B., Johnson, A., Fung, D. (1986). Exonuclease III and endonuclease IV remove 3’ blocks from DNA synthesis primers in H2O2-damaged Escherichia coli. Proc. Natl. Acad. Sci. United States America 83, 7731–7735. doi: 10.1073/pnas.83.20.7731
de Regt, A. K., Baker, T. A., Sauer, R. T. (2015). Steric clashes with bound OMP peptides activate the DegS stress-response protease. Proc. Natl. Acad. Sci. United States America 112, 3326–3331. doi: 10.1073/pnas.1502372112
Ellis, C. N., LaRocque, R. C., Uddin, T., Krastins, B., Mayo-Smith, L. M., Sarracino, D., et al. (2015). Comparative proteomic analysis reveals activation of mucosal innate immune signaling pathways during cholera. Infect Immun. 83, 1089–1103. doi: 10.1128/iai.02765-14
Emmer, M., deCrombrugghe, B., Pastan, I., Perlman, R. (1970). Cyclic AMP receptor protein of E. coli: its role in the synthesis of inducible enzymes. Proc. Natl. Acad. Sci. United States America 66, 480–487. doi: 10.1073/pnas.66.2.480
Guo, M., Wang, H., Xie, N., Xie, Z. (2015). Positive Effect of Carbon Sources on Natural Transformation in Escherichia coli: Role of Low-Level Cyclic AMP (cAMP)-cAMP Receptor Protein in the Derepression of rpoS. J. Bacteriol 197, 3317–3328. doi: 10.1128/jb.00291-15
Gupta, A., Imlay, J. A. (2022). Escherichia coli induces DNA repair enzymes to protect itself from low-grade hydrogen peroxide stress. Mol. Microbiol. 117, 754–769. doi: 10.1111/mmi.14870
Hasselblatt, H., Kurzbauer, R., Wilken, C., Krojer, T., Sawa, J., Kurt, J., et al. (2007). Regulation of the sigmaE stress response by DegS: how the PDZ domain keeps the protease inactive in the resting state and allows integration of different OMP-derived stress signals upon folding stress. Genes Dev. 21, 2659–2670. doi: 10.1101/gad.445307
Hews, C. L., Pritchard, E. J., Rowley, G. (2019). The salmonella specific, σ(E)-regulated, STM1250 and agsA, function with the sHsps ibpA and IbpB, to counter oxidative stress and survive macrophage killing. Front. Cell Infect. Microbiol. 9. doi: 10.3389/fcimb.2019.00263
Honn, M., Lindgren, H., Bharath, G. K., Sjöstedt, A. (2017). Lack of OxyR and KatG Results in Extreme Susceptibility of Francisella tularensis LVS to Oxidative Stress and Marked Attenuation In vivo. Front. Cell Infect. Microbiol. 7. doi: 10.3389/fcimb.2017.00014
Huang, J., Chen, Y., Chen, J., Liu, C., Zhang, T., Luo, S., et al. (2019). Exploration of the effects of a degS mutant on the growth of Vibrio cholerae and the global regulatory function of degS by RNA sequencing. PeerJ 7, e7959. doi: 10.7717/peerj.7959
Kaper, J. B., Morris, J. G., Jr., Levine, M. M. (1995). Cholera. Clin. Microbiol. Rev. 8, 48–86. doi: 10.1128/cmr.8.1.48
Kashyap, D. R., Kuzma, M., Kowalczyk, D. A., Gupta, D., Dziarski, R. (2017). Bactericidal peptidoglycan recognition protein induces oxidative stress in Escherichia coli through a block in respiratory chain and increase in central carbon catabolism. Mol. Microbiol. 105, 755–776. doi: 10.1111/mmi.13733
Kim, D. Y. (2015). Two stress sensor proteins for the expression of sigmaE regulon: DegS and RseB. J. Microbiol. 53, 306–310. doi: 10.1007/s12275-015-5112-6
Kovacikova, G., Skorupski, K. (2002). The alternative sigma factor sigma(E) plays an important role in intestinal survival and virulence in Vibrio cholerae. Infect Immun. 70, 5355–5362. doi: 10.1128/iai.70.10.5355-5362.2002
Lesser, M. P. (2006). Oxidative stress in marine environments: biochemistry and physiological ecology. Annu. Rev. Physiol. 68, 253–278. doi: 10.1146/annurev.physiol.68.040104.110001
Liu, Z., Wang, H., Zhou, Z., Sheng, Y., Naseer, N., Kan, B., et al. (2016). Thiol-based switch mechanism of virulence regulator AphB modulates oxidative stress response in Vibrio cholerae. Mol. Microbiol. 102, 939–949. doi: 10.1111/mmi.13524
Luo, P., Su, T., Hu, C., Ren, C. (2011). A novel and simple PCR walking method for rapid acquisition of long DNA sequence flanking a known site in microbial genome. Mol. Biotechnol. 47, 220–228. doi: 10.1007/s12033-010-9332-z
Manneh-Roussel, J., Haycocks, J. R. J., Magán, A., Perez-Soto, N., Voelz, K., Camilli, A., et al. (2018). cAMP receptor protein controls vibrio cholerae gene expression in response to host colonization. mBio 9 (4), e00966-18. doi: 10.1128/mBio.00966-18
Maraki, S., Christidou, A., Anastasaki, M., Scoulica, E. (2016). Non-O1, non-O139 Vibrio cholerae bacteremic skin and soft tissue infections. Infect. Dis. (London England) 48, 171–176. doi: 10.3109/23744235.2015.1104720
Meireles, D. A., da Silva Neto, J. F., Domingos, R. M., Alegria, T. G. P., Santos, L. C. M., Netto, L. E. S. (2022). Ohr - OhrR, a neglected and highly efficient antioxidant system: Structure, catalysis, phylogeny, regulation, and physiological roles. Free Radic. Biol. Med. 185, 6–24. doi: 10.1016/j.freeradbiomed.2022.04.001
Merrell, D. S., Tischler, A. D., Lee, S. H., Camilli, A. (2000). Vibrio cholerae requires rpoS for efficient intestinal colonization. Infect Immun. 68, 6691–6696. doi: 10.1128/iai.68.12.6691-6696.2000
Mishra, S., Imlay, J. (2012). Why do bacteria use so many enzymes to scavenge hydrogen peroxide? Arch. Biochem. Biophys. 525, 145–160. doi: 10.1016/j.abb.2012.04.014
Muller, D. A., Depelsenaire, A. C., Young, P. R. (2017). Clinical and laboratory diagnosis of dengue virus infection. J. Infect. Dis. 215, S89–s95. doi: 10.1093/infdis/jiw649
Nguyen, L. H., Jensen, D. B., Thompson, N. E., Gentry, D. R., Burgess, R. R. (1993). In vitro functional characterization of overproduced Escherichia coli katF/rpoS gene product. Biochemistry 32, 11112–11117. doi: 10.1021/bi00092a021
Nuss, A. M., Adnan, F., Weber, L., Berghoff, B. A., Glaeser, J., Klug, G. (2013). DegS and RseP homologous proteases are involved in singlet oxygen dependent activation of RpoE in Rhodobacter sphaeroides. PloS One 8, e79520. doi: 10.1371/journal.pone.0079520
Qadri, F., Raqib, R., Ahmed, F., Rahman, T., Wenneras, C., Das, S. K., et al. (2002). Increased levels of inflammatory mediators in children and adults infected with Vibrio cholerae O1 and O139. Clin. Diagn. Lab. Immunol. 9, 221–229. doi: 10.1128/cdli.9.2.221-229.2002
Qin, Z., Yang, X., Chen, G., Park, C., Liu, Z. (2020). Crosstalks between gut microbiota and vibrio cholerae. Front. Cell Infect. Microbiol. 10. doi: 10.3389/fcimb.2020.582554
Redford, P., Roesch, P. L., Welch, R. A. (2003). DegS is necessary for virulence and is among extraintestinal Escherichia coli genes induced in murine peritonitis. Infect Immun. 71, 3088–3096. doi: 10.1128/iai.71.6.3088-3096.2003
Seixas, A. F., Quendera, A. P., Sousa, J. P., Silva, A. F. Q., Arraiano, C. M., Andrade, J. M. (2021). Bacterial response to oxidative stress and RNA oxidation. Front. Genet. 12. doi: 10.3389/fgene.2021.821535
Si, M., Wang, J., Xiao, X., Guan, J., Zhang, Y., Ding, W., et al. (2015). Ohr Protects Corynebacterium glutamicum against Organic Hydroperoxide Induced Oxidative Stress. PloS One 10, e0131634. doi: 10.1371/journal.pone.0131634
Spees, A. M., Wangdi, T., Lopez, C. A., Kingsbury, D. D., Xavier, M. N., Winter, S. E., et al. (2013). Streptomycin-induced inflammation enhances Escherichia coli gut colonization through nitrate respiration. mBio 4 (4), e00430-13. doi: 10.1128/mBio.00430-13
Sudharsan, M., Prasad, N. R., Saravanan, R. (2022). Bacterial redox response factors in the management of environmental oxidative stress. World J. Microbiol. Biotechnol. 39, 11. doi: 10.1007/s11274-022-03456-5
Sun, F., Zhang, Y., Qiu, Y., Yang, H., Yang, W., Yin, Z., et al. (2014). H-NS is a repressor of major virulence gene loci in Vibrio parahaemolyticus. Front. Microbiol. 5. doi: 10.3389/fmicb.2014.00675
Testerman, T. L., Vazquez-Torres, A., Xu, Y., Jones-Carson, J., Libby, S. J., Fang, F. C. (2002). The alternative sigma factor sigmaE controls antioxidant defences required for Salmonella virulence and stationary-phase survival. Mol. Microbiol. 43, 771–782. doi: 10.1046/j.1365-2958.2002.02787.x
Wang, H., Chen, S., Zhang, J., Rothenbacher, F. P., Jiang, T., Kan, B., et al. (2012). Catalases promote resistance of oxidative stress in Vibrio cholerae. PloS One 7, e53383. doi: 10.1371/journal.pone.0053383
Wang, G., Fan, C., Wang, H., Jia, C., Li, X., Yang, J., et al. (2022). Type VI secretion system-associated FHA domain protein TagH regulates the hemolytic activity and virulence of Vibrio cholerae. Gut Microbes 14, 2055440. doi: 10.1080/19490976.2022.2055440
Wang, H., Naseer, N., Chen, Y., Zhu, A. Y., Kuai, X., Galagedera, N., et al. (2017). OxyR2 modulates oxyR1 activity and vibrio cholerae oxidative stress response. Infect Immun. 85 (4), e00929-16. doi: 10.1128/iai.00929-16
Wu, R., Zhao, M., Li, J., Gao, H., Kan, B., Liang, W. (2015). Direct regulation of the natural competence regulator gene tfoX by cyclic AMP (cAMP) and cAMP receptor protein (CRP) in Vibrios. Sci. Rep. 5, 14921. doi: 10.1038/srep14921
Wurm, P., Tutz, S., Mutsam, B., Vorkapic, D., Heyne, B., Grabner, C., et al. (2017). Stringent factor and proteolysis control of sigma factor RpoS expression in Vibrio cholerae. Int. J. Med. Microbiol. 307, 154–165. doi: 10.1016/j.ijmm.2017.01.006
Zheng, D., Constantinidou, C., Hobman, J. L., Minchin, S. D. (2004). Identification of the CRP regulon using in vitro and in vivo transcriptional profiling. Nucleic Acids Res. 32, 5874–5893. doi: 10.1093/nar/gkh908
Keywords: Vibrio cholerae, DegS protease, antioxidation, cAMP-CRP, XthA
Citation: Wang K, Lu H, Zou M, Wang G, Zhao J, Huang X, Ren F, Hu H, Huang J and Min X (2023) DegS protease regulates antioxidant capacity and adaptability to oxidative stress environment in Vibrio cholerae. Front. Cell. Infect. Microbiol. 13:1290508. doi: 10.3389/fcimb.2023.1290508
Received: 07 September 2023; Accepted: 06 November 2023;
Published: 20 November 2023.
Edited by:
George P. Munson, University of Miami, United StatesReviewed by:
Travis Bourret, Creighton University, United StatesJeremy C. Henderson, New England Biolabs, United States
Copyright © 2023 Wang, Lu, Zou, Wang, Zhao, Huang, Ren, Hu, Huang and Min. This is an open-access article distributed under the terms of the Creative Commons Attribution License (CC BY). The use, distribution or reproduction in other forums is permitted, provided the original author(s) and the copyright owner(s) are credited and that the original publication in this journal is cited, in accordance with accepted academic practice. No use, distribution or reproduction is permitted which does not comply with these terms.
*Correspondence: Jian Huang, 81537648@qq.com; Xun Min, minxunzmu@163.com
†These authors have contributed equally to this work