- Department of Veterinary Medicine, College of Animal Science, Guizhou University, Guiyang, China
Bovine viral diarrhea virus (BVDV) is a significant pathogen that causes great economic losses in the global livestock industry. During the long-term interactions between BVDV and its hosts, the virus has evolved multiple strategies to evade the host’s innate immunity and adaptive immunity, thereby promoting viral survival and replication. This review focuses on the most recent research on immune evasion strategies employed by BVDV, including evading type I IFN signaling pathway, evading host adaptive immunity, mediating NF-κB signaling pathway, mediating cell apoptosis and inducing autophagy. Unraveling BVDV’s immune evasion strategies will enhance our understanding of the pathogenesis of BVDV and contribute to the development of more effective therapies for the prevention, control and eradication of BVDV.
1 Introduction
Bovine viral diarrhea virus (BVDV) is a major pathogen responsible for bovine viral diarrhea (BVD), causing substantial economic losses in the global livestock industry (Lanyon et al., 2014; Oguejiofor et al., 2019; Al-Kubati et al., 2021). BVDV, border disease virus (BDV) and classical swine fever virus (CSFV) belong to Pestivirus genus of the Flaviviridae family which consists of 11 species named from Pestivirus A to Pestivirus K (Yesilbag et al., 2017; Gomez-Romero et al., 2021). BVDV exhibits great genetic diversity and is classified as three genotypes, BVDV-1 (Pestivirus A), BVDV-2 (Pestivirus B) and BVDV-3 (Pestivirus H or HoBi-like pestivirus). BVDV-1 can be classified into at least 21 sub-genotypes (BVDV1a-1u) while BVDV-2 and BVDV-3 can be classified into three (BVDV 2a-2c) and four (a-d) sub-genotypes, respectively (de Oliveira et al., 2020; Nugroho et al., 2022). According to the lytic activity of the virus in cell culture, BVDV strains of each genotype are divided into two distinct biotypes, cytopathic (cp) and non-cytopathic (ncp) (Peterhans et al., 2003). Most natural infection and persistent fetal infection are caused by ncp isolates, whereas cp isolates are uncommon and primarily associated with fatal BVD outbreaks referred to as mucosal disease(MD) (Brackenbury et al., 2003).
BVDV is a small, enveloped, single-stranded, positive-sense RNA virus. The BVDV particles are spherical in shape with a diameter of approximately 50 nm and consist of a core region of genomic RNA coated with the capsid or core protein (C). The core is surrounded by an outer lipid bilayer envelope in which three envelope proteins Erns, E1, and E2 are inserted into the membrane (Neill, 2013; Chi et al., 2022). The size of the BVDV genome ranges from 12.3 kilobases to 12.5 kilobases and consists of a single large open reading frame (ORF) flanked by short 5′- and 3′-untranslated regions (UTRs) (Chi et al., 2022; Hong et al., 2023). The ORF encodes a single large polyprotein that is post-translationally cleaved into four structural proteins (C, Erns, E1, and E2) and eight non-structural proteins (Npro, p7, NS2, NS3, NS4A, NS4B, NS5A, and NS5B) (Figure 1) (Mirosław and Polak, 2019; King et al., 2021). The cp BVDV is characterized by the constructive expression of NS3, while ncp BVDV strains produce a fusion of NS2 and NS3 proteins named NS2-3 (Darweesh et al., 2015). BVDV infection causes variable symptoms including growth retardation, hemorrhaging, respiratory and gastrointestinal disorders, reproductive failures, persistent infection, and lethal mucosal disease (MD) (Mirosław et al., 2022; Zhu et al., 2022).
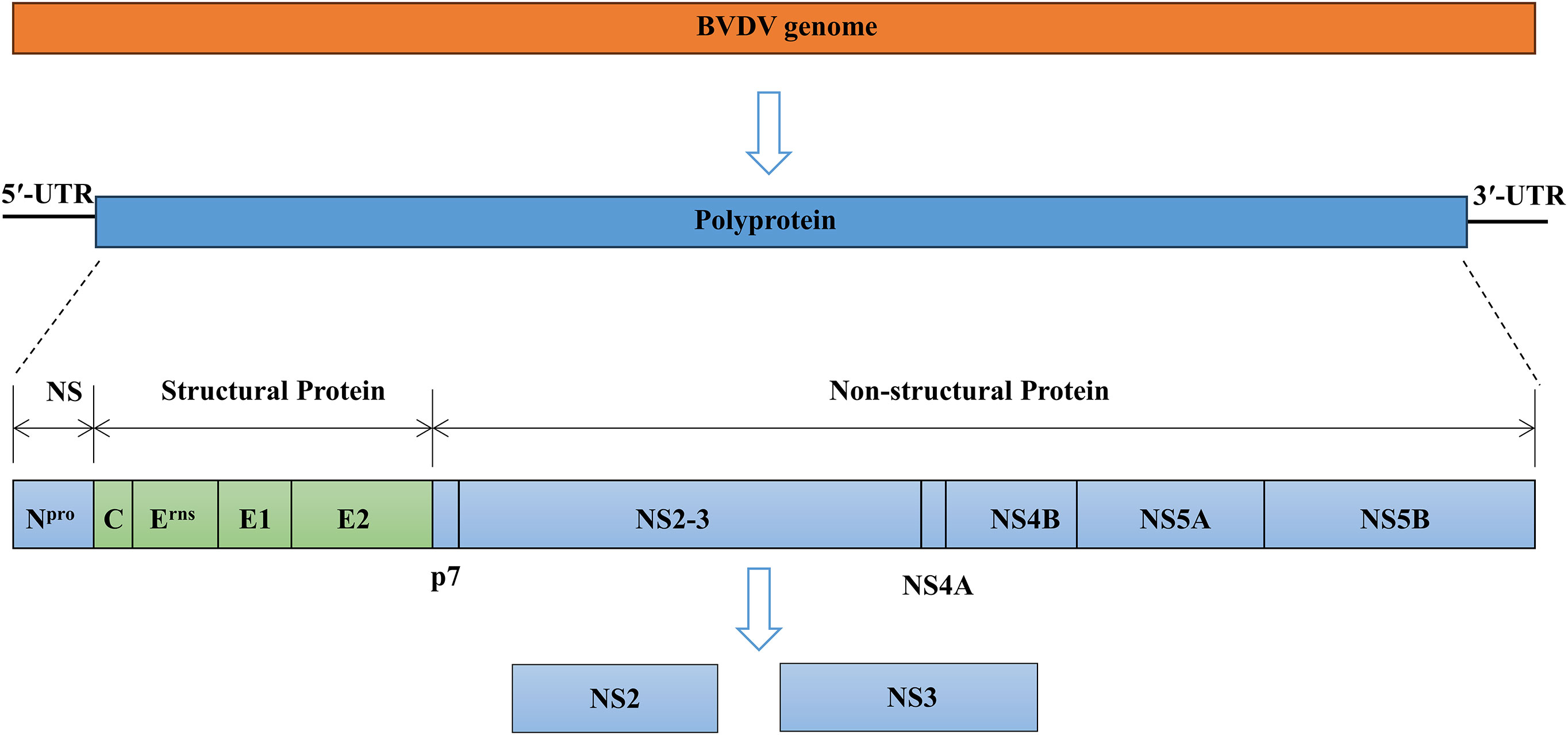
Figure 1 Overview of the genome organization of BVDV virion. BVDV encodes a single large polyprotein that is post-translationally cleaved into four structural proteins (C, Erns, E1, and E2) and eight non-structural (NS) proteins (Npro, p7, NS2, NS3, NS4A, NS4B, NS5A, and NS5B).
Throughout the co-evolutionary process between BVDV and its hosts, the virus has devised multiple tactics to evade host immune defense. This article summarizes the latest research on how BVDV evades host immune response, aimed to determine potential new targets and develop novel therapies for the prevention and control of BVDV infection.
2 BVDV evades host innate immunity
2.1 Innate immune sensing of BVDV
The innate immune system is the host’s first line of defense against invading pathogens. It responds quickly to infection by recognizing common pathogen characteristics, in contrast to the adaptive immune system, which takes days to generate adequate activated antigen-specific lymphocytes. Upon infection, the host′s innate immune response is initiated by various pattern recognition receptors (PRRs). PRRs recognize and bind to pathogen-associated molecular patterns (PAMPs), triggering a signaling cascade that ultimately leads to the production of type I interferon and other inflammatory cytokines (Zhu and Zheng, 2020; Roetman et al., 2022; Chen et al., 2023). The major PRRs for sensing RNA viruses are Toll-like Receptors (TLRs), RIG-I-like receptors (RLRs), C-type lectin receptors (CLRs) and NOD-like receptors (NLRs)(Chow et al., 2018; Wang et al., 2021b).
2.1.1 TLR-mediated recognition of BVDV
TLRs are important innate receptors expressed in a wide variety of mammalian immune and non-immune cells. TLR3, TLR7, TLR8 and TLR9 are expressed in intracellular vesicles such as the endosome and the endoplasmic reticulum (ER) while other TLRs are expressed on the cell surface. TLR3 recognizes double-stranded RNA (dsRNA) while TLR7 and TLR8 recognize single-stranded RNA (ssRNA) produced by viruses during viral infection (Bayraktar et al., 2019; de Marcken et al., 2019). When monocytes were infected with ncp BVDV, the mRNA levels of TLR3, type I IFN and IL-12 were significantly increased at 1 h post infection, whereas the TLR7 mRNA was significantly upregulated in monocytes infected with both biotypes at 24 h post infection (Lee et al., 2008). Moreover, the mRNA levels of TLR3, TLR7 and CD86 were significantly increased in spleen and tracheobronchial lymph node on day 5 post inoculation with high virulence ncp BVDV, but not the low virulence strains (Palomares et al., 2014).
2.1.2 RLR-mediated recognition of BVDV
RLRs include three members, retinoic acid-inducible gene I (RIG-I), melanoma differentiation-associated protein 5 (MDA5) and laboratory of genetics and physiology 2 (LGP2). RIG-I recognizes 5’-di/triphosphate-containing, short, panhandle double-stranded RNA and activates downstream signaling pathway in an ATP-dependent manner. RIG-I has a preference for short viral dsRNA, whereas MDA-5 binds selectively to long dsRNA. Both RIG-I and MDA5 interact with the adapter molecule mitochondrial antiviral signaling protein (MAVS), activating RLR signaling pathway, inducing the production of proinflammatory cytokines and type I IFN. The RLRs play a critical role in sensing of RNA virus infection to initiate and modulate antiviral immunity (Jensen and Thomsen, 2012; Onomoto et al., 2021; Zhang et al., 2022). Based on lncRNA sequencing results and lncRNA-mRNA co-expression network, researchers found that differentially expressed lncRNAs in cp BVDV-infected MDBK cells were mainly enriched in apoptosis, NOD-like receptor (NLR) and RIG-I-like receptor (RLR) signaling pathways (Gao et al., 2021; Gao et al., 2022).
2.2 Host innate immune response to BVDV infection
Interferons (IFNs) are generally classified into three types: type I, type II, and type III IFNs (Hoffmann et al., 2015). They show distinct expression patterns and are involved in innate and adaptive immunity in a variety of ways. Type I and type III IFNs are primary antiviral IFNs. Type I IFNs are composed of IFN-α (13 subtypes in humans and 14 subtypes in mice), IFN-β, IFN-ϵ, IFN-κ, IFN-ω (humans) and IFN-ξ (mice). In mammals, the type I IFN response is essential for innate antiviral responses. Binding of IFN-α/β to its receptor (IFNAR) activates downstream Janus kinase (JAK)-signal transducers and activators (STAT) signaling pathway, ultimately leading to the induction of large numbers of IFN-stimulated genes (ISGs) to trigger a global antiviral state (Lazear et al., 2019; Song et al., 2022). Type II IFNs include only one member, IFN-γ, which is secreted by activated T cells, natural killer (NK) cells, NKT cells, and dendritic cells (Rojas et al., 2021). Generally, type II IFNs act as a bridge between the innate and adaptive immune responses. Type III IFNs (IFN-λ1, IFN-λ2, IFN-λ3 and IFN-λ4) are genetically different from type I IFNs, but they activate antiviral pathways similar to type I IFNs. The type III IFN receptor is mainly restricted to epithelial cells, while the type I IFN receptor is present on all nucleated cell types (Mesev et al., 2019).
It has been reported that acute infection with ncp BVDV resulted in a significant increase in bovine serum IFN-γ and IFN-α levels. Specifically, in the peripheral blood mononuclear cells (PBMCs) of BVDV-infected cows, classical IFN-activated signaling pathways, such as JAK-STAT were activated and ISGs were significantly induced (Van Wyk et al., 2016). Moreover, cp BVDV infection induced IFN-β production in MDBK cells by activating interferon regulatory factor 1 (IRF1), IRF7, and NF-κB signaling pathways in a way similar to poly I:C stimulation (Maldonado et al., 2020). Infection with cp BVDV was found to induce the activation of dsRNA-dependent protein kinase (PKR) that phosphorylated alpha subunit of the translational initiation factor (eIF2α), leading to translation inhibition while ncp BVDV did not induce PKR activity and eIF2α phosphorylation, aiming to establish a persistent infection (Gil et al., 2006b). However, the impact of BVDV on the JAK-STAT signaling pathway remained largely unknown, which was required for further studies.
2.3 BVDV evades type I IFN signaling pathway
For successful infection, most pestiviruses have evolved two proteins, the non-structural protein Npro and the envelope glycoprotein Erns to prevent the activation of innate immunity (De Martin and Schweizer, 2022). Erns has been reported to inhibit dsRNA-mediated signaling pathways, depending on its dsRNA binding properties and RNase activity (Iqbal et al., 2004). Erns had the ability to inhibit type I IFN production induced by viral dsRNA or ssRNA, due to its RNase activity to degrade viral RNA(Mätzener et al., 2009; Zürcher et al., 2014). NPro was sufficient to suppress IFN-β induction by targeting IRF3 for polyubiquitination and proteasomal degradation, without relying on its autoprotease activity (Hilton et al., 2006). Others showed that cp BVDV infection did not induce detectable levels of IFN-α/β and that the N-terminal domain of Npro protein was required for type I IFN antagonism, independent of its catalytic endoproteinase activity (Gil et al., 2006a). Furthermore, the ncp BVDV Npro protein was determined to interact with the cellular S100A9 protein and reduce its activity in infected cells, leading to reduced type I IFN production, thereby promoting viral replication (Darweesh et al., 2018).
The cp BVDV NS4B protein significantly inhibited RLR-mediated IFN-β promoter activity as well as MDA5 mRNA levels (Shan et al., 2021). Furthermore, NS4B protein was shown to inhibit the MDA5-mediated signal transduction by acting as an IFN-β antagonist through direct interaction with two N-terminal caspase-recruitment domains (CARDs) of MDA5. In addition, BVDV infection induced higher mRNA and protein levels of DNA damage-inducible transcript 3 (DDIT3), which inhibited type I IFN production by regulating MAVS degradation during viral infection in MDBK cells and in mice (Wang et al., 2021a).
High-throughput sequencing, such as RNA-seq, is a powerful tool to study viral pathogenesis and virus-host interaction. A RNA-Seq-based transcriptome analysis of MDBK cells infected with BVDV suggested that ncp BVDV infection significantly inhibited the expression of several antiviral ISGs, such as ISG15, Mx1, and OSA1Y, which were involved in the inhibition of host′s innate immunity at the early stage during BVDV infection (Liu et al., 2019). Another similar study showed that cp BVDV infection significantly upregulated two negative NF-κB regulators, IER3 and TNFAIP3, thus possibly blocking this signaling pathway (Villalba et al., 2016). The evasion of type I IFN signaling pathway by BVDV is illustrated in Figure 2.
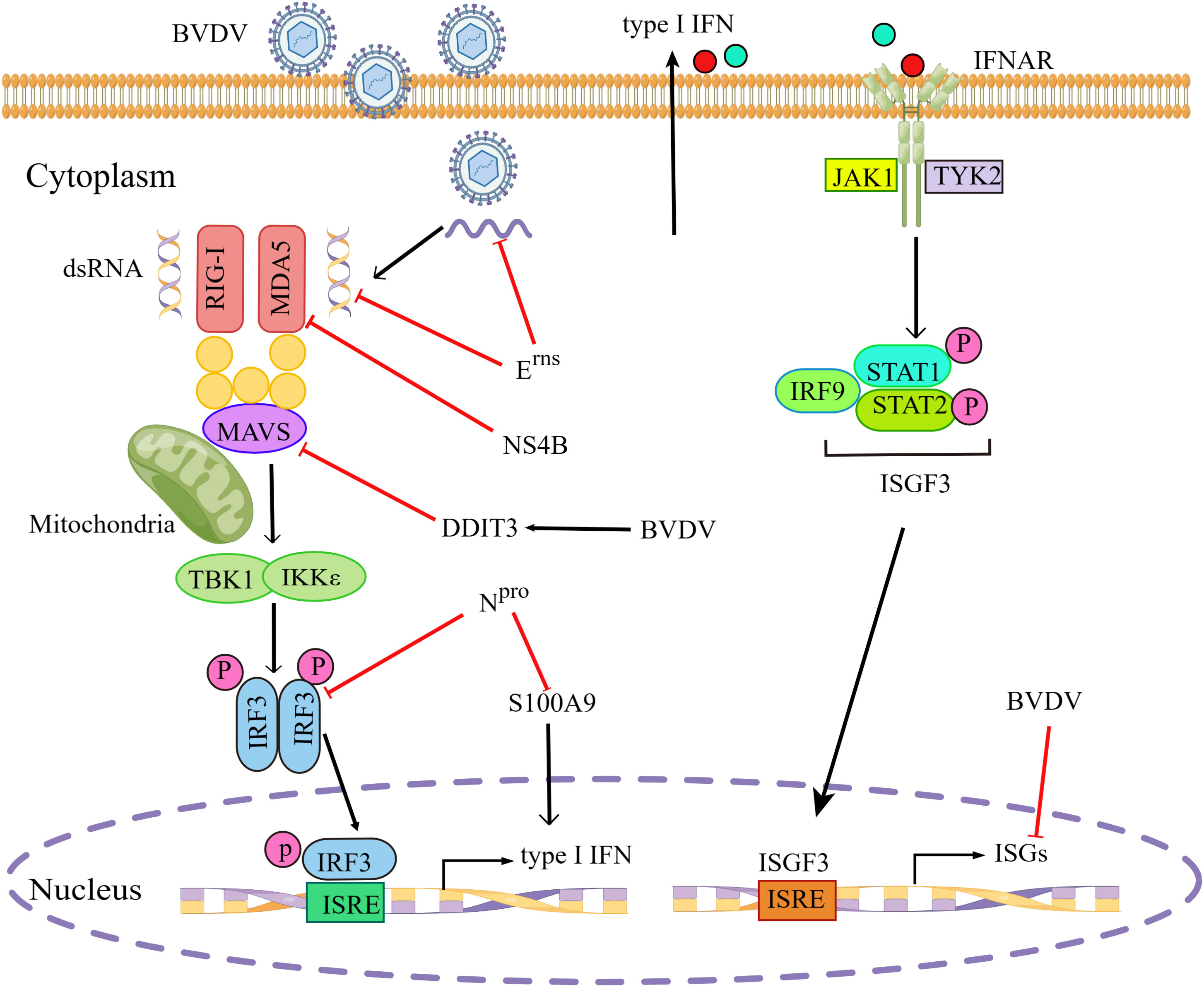
Figure 2 BVDV evades type I IFN signaling pathway. BVDV has evolved various strategies to evade type I IFN signaling pathway by inhibiting multiple steps in the signal transduction. BVDV, Bovine viral diarrhea virus; RIG-I, retinoic acid-inducible gene I; MDA5, melanoma-differentiation-associated gene 5; MAVS, mitochondrial antiviral-signaling protein; TBK1, TANK-binding kinase 1; IKKϵ, inhibitor of IκB kinase ϵ; IRF3/9, interferon regulatory transcription factor 3/9; IFNAR, interferon-α/β receptor; JAK1, janus kinase 1; TYK2, tyrosine kinases 2; STAT1/2, signal transducer and activation of transcription 1/2; ISGs, interferon-stimulated genes; P, phosphate; ISRE, interferon-stimulated response element; ISGF3, interferon-stimulated gene factor 3; DDIT3, DNA damage-inducible transcript 3; S100A9, S100 calcium binding protein A9. Red blunt end arrows indicated inhibition of the signaling pathway by BVDV or BVDV proteins. Black solid arrows indicated pathway connection. The figure was drawn by Figdraw.
3 BVDV evades host adaptive immunity
3.1 BVDV impairs antigen presentation and evades cellular immunity
The innate immune system is the first line of defense against various pathogens. Adaptive immune cells then mount antigen-specific responses and establish long-term memory immunity. Previous evidence indicated that cp BVDV infection resulted in a significant reduction in the expression of the Fc receptor (FcR) and the complement receptor (C3R) in alveolar macrophages, which are required for phagocytic activity, thereby decreasing antigen uptake and presentation (Welsh et al., 1995). The effect of BVDV infection on antigen presentation was dependent on viral strains and/or the maturity of antigen presenting cells (APCs). Both monocytes and dendritic cells (DCs) were susceptible to two biotypes BVDV infection, but monocytes were lysed by cp BVDV while DCs were not (Glew et al., 2003; Chase, 2013). Furthermore, monocytes infected with ncp BVDV failed to stimulate CD4+ T cell responses while DCs remained unaffected. When monocytes were differentiated into monocyte-derived dendritic cells (Mo-DCs), Mo-DCs lost the ability to produce infectious virions, but were able to produce viral RNA, as well as viral NS5A and E2 proteins, likely due to the interruption of virus packaging or release process (Rajput et al., 2014). In addition, ncp BVDV downregulated the expression of MHC-I, MHC-II, and CD86 on Mo-DC surface, which may account for the immunosuppression and persistent infection caused by ncp BVDV (Rajput et al., 2014). Proteomic profiling revealed that cp BVDV infection significantly decreased the expression of 9 MHC-I proteins and 6 MHC-II proteins in bovine monocytes compared to mock-infected controls, thereby inhibiting antigen presentation to immunocompetent T cells (Lee et al., 2009). Bovine CD4+ T cells played a critical role in controlling BVDV infection. Evidence suggested that depletion of CD4+ T cells in calves exposed to ncp BVDV resulted in an increase in blood viral load and prolonged duration of viremia, whereas depletion of either CD8+ T cells or γδ+ T cells had no observable effect(Howard et al., 1992). Both CD4+ and CD8+ T cell proliferation was elicited from virus-infected stimulatory cells whereas only CD4+ T cells responded to non-infectious BVDV antigens, including Erns, E2, NS2-3, capsid and/or Npro proteins (Collen and Morrison, 2000).
3.2 BVDV evades humoral immunity
The glycoprotein Erns of BVDV, a highly glycosylated envelope protein, induced significant antibody production in animals. However, the neutralizing activity of antibodies targeting Erns was limited (Donis, 1995). E2 was the largest glycoprotein and antigenic target for the induction of neutralizing antibodies (Liang et al., 2005; Li et al., 2013; Cheng et al., 2023). After natural infection or inoculation with a modified live vaccine, the B cell response, as measured by serum antibody levels, was predominantly directed against viral E2 and NS2/3 proteins, with lesser responses directed against Erns and E1 proteins. Vaccination with killed vaccines led to the production of serum antibodies directed primarily against the E2 protein (Ridpath, 2013; Griebel, 2015; Al-Kubati et al., 2021).
When calves were infected with cp BVDV, total IgG and IgG1 concentrations were significantly decreased at 7 days post infection (DPI) and then recovered by 21 DPI (Rajput et al., 2020). Ncp BVDV infection did not affect total IgG concentration at 7, 14 and 21 DPI until an obvious rise occurred from 21 to 35 DPI. For both biotypes, the IgG1 concentration and the IgG1:IgG2 ratio were significantly reduced from 0 DPI to 7 DPI. Calves infected with ncp BVDV had significantly higher IgG1 levels at all time points and higher IgG2 concentrations at 7 and 35 DPI compared to cp TGAC strain. Ncp BVDV induced significantly higher levels of serum neutralizing antibodies at 14, 21 and 35 DPI than cp BVDV, suggesting that ncp BVDV evoked a stronger immune response than cp BVDV.
4 BVDV mediates NF-κB signaling pathway
NF-κB is a key transcriptional complex that regulates the expression of genes involved in inflammation and the immune response (Zhang et al., 2017; Reus et al., 2022). A previous study showed that cp BVDV infection induced the production of various inflammatory cytokines and antiviral molecules in MDBK cells. Interestingly, all BVDV activities were inhibited by pharmacological NF-κB pathway inhibitors. Infection with cp BVDV promoted NF-κB activation and p65 translocation to the nucleus within minutes of virus entry into cells (Fredericksen et al., 2015). More recently, scientists identified that cp BVDV infection resulted in the activation the NF-κB signaling pathway and enhanced the expression of NLRP3 inflammasome components and inflammatory cytokine pro-IL-1β post BVDV infection (Fan et al., 2022). As a result, pro-caspase-1 and pro-IL-1β were cleaved into their active forms, caspase-1 and IL-1β. Further experimental results indicated that viral NS5A and Erns proteins were involved in the BVDV-induced inflammatory response by activating the NF-κB signaling pathway.
5 BVDV mediates cell apoptosis
Ncp BVDV strains did not cause cell death, but were capable of establishing persistent infection, whereas cp BVDV strains induced cytopathic effects (CPE) via apoptosis in bovine cells. Cp BVDV infection led to the activation of the intrinsic apoptotic pathway, which was characterized by the translocation of cytochrome c from mitochondria to the cytosol, Apaf-1 overexpression, significantly increased caspase-9 activity and the formation of apoptosome (Grummer et al., 2002). It was found that the mRNA levels of apoptosis-associated genes such as Mx1, iNOS, TNF-α and OAS1 were upregulated following cp BVDV infection (Yamane et al., 2006). RNAi silencing of both PKR and OAS-1 resulted in almost complete inhibition of apoptosis induced by cp BVDV infection without affecting viral titers. In conclusion, dsRNA was a major inducer of apoptosis in cp BVDV-infected cells whereas ncp BVDV produced minimal levels of dsRNA without induction of apoptosis to establish a persistent infection. Furthermore, overexpression of cp BVDV NS3 protein alone or NS3Δ50 (deleting N- terminal 50 amino acids) truncated protein was able to induce apoptosis in vitro that correlated with initiator caspase-8 and caspase-9 activation (St-Louis et al., 2005). Cp BVDV NS3/4A induced an intrinsic apoptotic pathway characterized by increased activity of caspase-9 and caspase-3 in MDBK cells dependent on NS3 protease activity (Gamlen et al., 2010). Apart from an intrinsic pathway, cp BVDV can also induce apoptosis through an extrinsic pathway by upregulating TNF-α and iNOS mRNA levels and inducing the expression of both TNFR-1 and TNFR-2, which was required to convert an extrinsic factor signal into an intracellular death signal(Yamane et al., 2005).
Moreover, cp BVDV induced endoplasmic reticulum (ER) stress-induced apoptosis in MDBK cells (Jordan et al., 2002). Cp BVDV activated the ER transmembrane kinase PERK and caused phosphorylation of the translation initiation factor eIF2α, which induced CHOP/GADD153 expression and consequently led to the downregulation of anti-apoptotic Bcl-2 protein and a decrease in intracellular GSH levels. Finally, cp BVDV infection induced the expression of caspase-12 and initiated apoptosis. In a recent study, cp BVDV was demonstrated to trigger ER stress-related apoptosis in bovine placental trophoblast cells (BTCs), thereby promoting the spread of BVDV (Yuan et al., 2023). Inhibition of ER stress-induced apoptosis blocked BVDV replication and significantly reduced BVDV virulence. To sum up, cp BVDV induced apoptosis via the intrinsic pathway, the extrinsic pathway and the ER stress-mediated apoptosis signaling pathway.
6 BVDV induces autophagy in various cell types
Autophagy, a catabolic process of great significance, plays a critical role in the maintenance of cellular homeostasis (Klionsky et al., 2021). There are three types of autophagy: macro-, micro-, and chaperone-mediated autophagy (CMA). Macro-autophagy, referred to as “autophagy”, is the major pathway (Khandia et al., 2019). A previous study has demonstrated that infection with cp BVDV induced autophagy and significantly upregulated the mRNA and protein levels of autophagy-related genes, Beclin1 and ATG14 in MDBK cells at 12 h post infection (Fu et al., 2014a). Nevertheless, the knockdown of Beclin1 and ATG14 did not impact cp BVDV infection-related autophagy. The viral envelope proteins Erns and E2 were responsible for the induction of autophagy by increasing the formation of autophagosomes, the percentage of GFP-LC3 puncta-positive cells and the protein levels of Beclin1 and ATG14 (Fu et al., 2014b). Both biotypes had the ability to induce autophagy in MDBK and primary bovine turbinate (Bt) cells at similar levels and used autophagy to replicate themselves (Rajput et al., 2017). Similarly, ncp BVDV infection induced autophagy in MDBK cells by significantly increasing the conversion of LC3-I to LC3-II, upregulating the autophagy-related proteins ATG5 and Beclin 1 and degrading p62/SQSTM1, thereby promoting viral replication(Shin et al., 2023). The BVDV non-structural protein NS4B alone was able to induce autophagosomes in bovine kidney LB9.k cells, representing a novel and significant function of NS4B in BVDV replication (Suda et al., 2019). Two biotypes of BVDV utilized different strategies to regulate the unfolded protein response (UPR) mechanisms and ER stress-mediated autophagy for their own benefit (Wang et al., 2023). In an unconventional manner, the cp BVDV downregulated the expression level of the ER chaperone GRP78, thereby promoting self-replication through the UPR, while the ncp BVDV retained activating transcription factor 4 (ATF4) in the cytoplasm to provide an advantage for its persistent infection.
7 Conclusions and perspectives
BVDV has evolved multiple immune evasion strategies to establish persistent infection and facilitate its transmission. Understanding these mechanisms is critical for the development of effective vaccines and control measures against BVDV. Further research is needed to clarify the molecular mechanisms responsible for BVDV-induced immunosuppression and discover novel targets for antiviral intervention. In addition, it is crucial to develop advanced vaccines that offer comprehensive defense against various strains of BVDV in order to minimize the influence of this economically important pathogen on the livestock industry.
Author contributions
FP: Conceptualization, Funding acquisition, Visualization, Writing – original draft, Writing – review & editing. QL: Conceptualization, Writing – review & editing. MW: Writing – review & editing.
Funding
The author(s) declare financial support was received for the research, authorship, and/or publication of this article. This work was supported by Guizhou Provincial Science and Technology Project ([2022]093); Cultivation project of National Natural Science Foundation for young teachers of Guizhou University ([2020]42); Guizhou University Laboratory open projects (SYSKF2023-026).
Conflict of interest
The authors declare that the research was conducted in the absence of any commercial or financial relationships that could be construed as a potential conflict of interest.
Publisher’s note
All claims expressed in this article are solely those of the authors and do not necessarily represent those of their affiliated organizations, or those of the publisher, the editors and the reviewers. Any product that may be evaluated in this article, or claim that may be made by its manufacturer, is not guaranteed or endorsed by the publisher.
References
Al-Kubati, A. A., Hussen, J., Kandeel, M., Al-Mubarak, A. I., Hemida, M. G. (2021). Recent advances on the bovine viral diarrhea virus molecular pathogenesis, immune response, and vaccines development. Front. veterinary Sci. 8, 665128. doi: 10.3389/fvets.2021.665128
Bayraktar, R., Bertilaccio, M. T. S., Calin, G. A. (2019). The interaction between two worlds: microRNAs and Toll-like receptors. Front. Immunol. 10, 1053. doi: 10.3389/fimmu.2019.01053
Brackenbury, L., Carr, B., Charleston, B. (2003). Aspects of the innate and adaptive immune responses to acute infections with BVDV. Veterinary Microbiol. 96 (4), 337–344. doi: 10.1016/j.vetmic.2003.09.004
Chase, C. C. (2013). The impact of BVDV infection on adaptive immunity. Biologicals 41 (1), 52–60. doi: 10.1016/j.biologicals.2012.09.009
Chen, X.-x., Qiao, S., Li, R., Wang, J., Li, X., Zhang, G. (2023). Evasion strategies of porcine reproductive and respiratory syndrome virus. Front. Microbiol. 14, 1140449. doi: 10.3389/fmicb.2023.1140449
Cheng, Y., Tu, S., Chen, T., Zou, J., Wang, S., Jiang, M., et al. (2023). Evaluation of the mucosal immunity effect of bovine viral diarrhea virus subunit vaccine E2Fc and E2Ft. Int. J. Mol. Sci. 24 (4), 4172. doi: 10.3390/ijms24044172
Chi, S., Chen, S., Jia, W., He, Y., Ren, L., Wang, X. (2022). Non-structural proteins of bovine viral diarrhea virus. Virus Genes 58 (6), 491–500. doi: 10.1007/s11262-022-01914-8
Chow, K. T., Gale, M., Jr., Loo, Y.-M. (2018). RIG-I and other RNA sensors in antiviral immunity. Annu. Rev. Immunol. 36, 667–694. doi: 10.1146/annurev-immunol-042617-053309
Collen, T., Morrison, W. I. (2000). CD4+ T-cell responses to bovine viral diarrhoea virus in cattle. Virus Res. 67 (1), 67–80. doi: 10.1016/S0168-1702(00)00131-3
Darweesh, M. F., Rajput, M. K., Braun, L. J., Ridpath, J. F., Neill, J. D., Chase, C. C. (2015). Characterization of the cytopathic BVDV strains isolated from 13 mucosal disease cases arising in a cattle herd. Virus Res. 195, 141–147. doi: 10.1016/j.virusres.2014.09.015
Darweesh, M. F., Rajput, M. K., Braun, L. J., Rohila, J. S., Chase, C. C. (2018). BVDV Npro protein mediates the BVDV induced immunosuppression through interaction with cellular S100A9 protein. Microbial pathogenesis 121, 341–349. doi: 10.1016/j.micpath.2018.05.047
de Marcken, M., Dhaliwal, K., Danielsen, A. C., Gautron, A. S., Dominguez-Villar, M. (2019). TLR7 and TLR8 activate distinct pathways in monocytes during RNA virus infection. Sci. Signaling 12 (605), eaaw1347. doi: 10.1126/scisignal.aaw1347
De Martin, E., Schweizer, M. (2022). Fifty shades of erns: innate immune evasion by the viral endonucleases of all pestivirus species. Viruses 14 (2), 265. doi: 10.3390/v14020265
de Oliveira, L. G., Mechler-Dreibi, M. L., Almeida, H. M., Gatto, I. R. (2020). Bovine viral diarrhea virus: Recent findings about its occurrence in pigs. Viruses 12 (6), 600. doi: 10.3390/v12060600
Donis, R. O. (1995). Molecular biology of bovine viral diarrhea virus and its interactions with the host. Veterinary Clinics North America: Food Anim. Pract. 11 (3), 393–423. doi: 10.1016/S0749-0720(15)30459-X
Fan, W., Wang, Y., Jiang, S., Li, Y., Yao, X., Wang, M., et al. (2022). Identification of key proteins of cytopathic biotype bovine viral diarrhoea virus involved in activating NF-κB pathway in BVDV-induced inflammatory response. Virulence 13 (1), 1884–1899. doi: 10.1080/21505594.2022.2135724
Fredericksen, F., Carrasco, G., Villalba, M., Olavarría, V. H. (2015). Cytopathic BVDV-1 strain induces immune marker production in bovine cells through the NF-κB signaling pathway. Mol. Immunol. 68 (2), 213–222. doi: 10.1016/j.molimm.2015.08.010
Fu, Q., Shi, H., Ren, Y., Guo, F., Ni, W., Qiao, J., et al. (2014a). Bovine viral diarrhea virus infection induces autophagy in MDBK cells. J. Microbiol. 52, 619–625. doi: 10.1007/s12275-014-3479-4
Fu, Q., Shi, H., Shi, M., Meng, L., Bao, H., Zhang, G., et al. (2014b). Roles of bovine viral diarrhea virus envelope glycoproteins in inducing autophagy in MDBK cells. Microbial pathogenesis 76, 61–66. doi: 10.1016/j.micpath.2014.09.011
Gamlen, T., Richards, K. H., Mankouri, J., Hudson, L., McCauley, J., Harris, M., et al. (2010). Expression of the NS3 protease of cytopathogenic bovine viral diarrhea virus results in the induction of apoptosis but does not block activation of the beta interferon promoter. J. Gen. Virol. 91 (1), 133–144. doi: 10.1099/vir.0.016170-0
Gao, X., Niu, C., Wang, Z., Jia, S., Han, M., Ma, Y., et al. (2021). Comprehensive analysis of lncRNA expression profiles in cytopathic biotype BVDV-infected MDBK cells provides an insight into biological contexts of host–BVDV interactions. Virulence 12 (1), 20–34. doi: 10.1080/21505594.2020.1857572
Gao, X., Sun, X., Yao, X., Wang, Y., Li, Y., Jiang, X., et al. (2022). Downregulation of the long noncoding RNA IALNCR targeting MAPK8/JNK1 promotes apoptosis and antagonizes bovine viral diarrhea virus replication in host cells. J. Virol. 96 (17), e01113–e01122. doi: 10.1128/jvi.01113-22
Gil, L. H., Ansari, I. H., Vassilev, V., Liang, D., Lai, V. C., Zhong, W., et al. (2006a). The amino-terminal domain of bovine viral diarrhea virus Npro protein is necessary for alpha/beta interferon antagonism. J. Virol. 80 (2), 900–911. doi: 10.1128/JVI.80.2.900-911.2006
Gil, L. H., van Olphen, A. L., Mittal, S. K., Donis, R. O. (2006b). Modulation of PKR activity in cells infected by bovine viral diarrhea virus. Virus Res. 116 (1-2), 69–77. doi: 10.1016/j.virusres.2005.08.011
Glew, E., Carr, B., Brackenbury, L., Hope, J., Charleston, B., Howard, C. J. (2003). Differential effects of bovine viral diarrhoea virus on monocytes and dendritic cells. J. Gen. Virol. 84 (7), 1771–1780. doi: 10.1099/vir.0.18964-0
Gomez-Romero, N., Ridpath, J. F., Basurto-Alcantara, F. J., Verdugo-Rodriguez, A. (2021). Bovine viral diarrhea virus in cattle from Mexico: Current Status. Front. Veterinary Sci. 8, 673577. doi: 10.3389/fvets.2021.673577
Griebel, P. J. (2015). BVDV vaccination in North America: risks versus benefits. Anim. Health Res. Rev. 16 (1), 27–32. doi: 10.1017/S1466252315000080
Grummer, B., Bendfeldt, S., Wagner, B., Greiser-Wilke, I. (2002). Induction of the intrinsic apoptotic pathway in cells infected with cytopathic bovine virus diarrhoea virus. Virus Res. 90 (1-2), 143–153. doi: 10.1016/S0168-1702(02)00150-8
Hilton, L., Moganeradj, K., Zhang, G., Chen, Y.-H., Randall, R. E., McCauley, J. W., et al. (2006). The NPro product of bovine viral diarrhea virus inhibits DNA binding by interferon regulatory factor 3 and targets it for proteasomal degradation. J. Virol. 80 (23), 11723–11732. doi: 10.1128/JVI.01145-06
Hoffmann, H.-H., Schneider, W. M., Rice, C. M. (2015). Interferons and viruses: an evolutionary arms race of molecular interactions. Trends Immunol. 36 (3), 124–138. doi: 10.1016/j.it.2015.01.004
Hong, T., Yang, Y., Wang, P., Zhu, G., Zhu, C. (2023). Pestiviruses infection: Interferon-virus mutual regulation. Front. Cell. Infection Microbiol. 13, 210. doi: 10.3389/fcimb.2023.1146394
Howard, C., Clarke, M., Sopp, P., Brownlie, J. (1992). Immunity to bovine virus diarrhoea virus in calves: the role of different T-cell subpopulations analysed by specific depletion in vivo with monoclonal antibodies. Veterinary Immunol. Immunopathol. 32 (3-4), 303–314. doi: 10.1016/0165-2427(92)90052-R
Iqbal, M., Poole, E., Goodbourn, S., McCauley, J. W. (2004). Role for bovine viral diarrhea virus Erns glycoprotein in the control of activation of beta interferon by double-stranded RNA. J. Virol. 78 (1), 136–145. doi: 10.1128/jvi.78.1.136-145.2004
Jensen, S., Thomsen, A. R. (2012). Sensing of RNA viruses: a review of innate immune receptors involved in recognizing RNA virus invasion. J. Virol. 86 (6), 2900–2910. doi: 10.1128/JVI.05738-11
Jordan, R., Wang, L., Graczyk, T. M., Block, T. M., Romano, P. R. (2002). Replication of a cytopathic strain of bovine viral diarrhea virus activates PERK and induces endoplasmic reticulum stress-mediated apoptosis of MDBK cells. J. Virol. 76 (19), 9588–9599. doi: 10.1128/JVI.76.19.9588-9599.2002
Khandia, R., Dadar, M., Munjal, A., Dhama, K., Karthik, K., Tiwari, R., et al. (2019). A comprehensive review of autophagy and its various roles in infectious, non-infectious, and lifestyle diseases: current knowledge and prospects for disease prevention, novel drug design, and therapy. Cells 8 (7), 674. doi: 10.3390/cells8070674
King, J., Pohlmann, A., Dziadek, K., Beer, M., Wernike, K. (2021). Cattle connection: molecular epidemiology of BVDV outbreaks via rapid nanopore whole-genome sequencing of clinical samples. BMC Veterinary Res. 17 (1), 1–10. doi: 10.1186/s12917-021-02945-3
Klionsky, D. J., Petroni, G., Amaravadi, R. K., Baehrecke, E. H., Ballabio, A., Boya, P., et al. (2021). Autophagy in major human diseases. EMBO J. 40 (19), e108863. doi: 10.15252/embj.2021108863
Lanyon, S. R., Hill, F. I., Reichel, M. P., Brownlie, J. (2014). Bovine viral diarrhoea: pathogenesis and diagnosis. Vet. J. 199 (2), 201–209. doi: 10.1016/j.tvjl.2013.07.024
Lazear, H. M., Schoggins, J. W., Diamond, M. S. (2019). Shared and distinct functions of type I and type III interferons. Immunity 50 (4), 907–923. doi: 10.1016/j.immuni.2019.03.025
Lee, S.-R., Nanduri, B., Pharr, G. T., Stokes, J. V., Pinchuk, L. M. (2009). Bovine viral diarrhea virus infection affects the expression of proteins related to professional antigen presentation in bovine monocytes. Biochim. Biophys. Acta (BBA)-Proteins Proteomics 1794 (1), 14–22. doi: 10.1016/j.bbapap.2008.09.005
Lee, S.-R., Pharr, G., Boyd, B., Pinchuk, L. (2008). Bovine viral diarrhea viruses modulate toll-like receptors, cytokines and co-stimulatory molecules genes expression in bovine peripheral blood monocytes. Comp. immunology Microbiol. Infect. Dis. 31 (5), 403–418. doi: 10.1016/j.cimid.2007.06.006
Li, Y., Wang, J., Kanai, R., Modis, Y. (2013). Crystal structure of glycoprotein E2 from bovine viral diarrhea virus. Proc. Natl. Acad. Sci. 110 (17), 6805–6810. doi: 10.1073/pnas.1300524110
Liang, R., Van den Hurk, J. V., Zheng, C., Yu, H., Pontarollo, R. A., Babiuk, L. A. (2005). Immunization with plasmid DNA encoding a truncated, secreted form of the bovine viral diarrhea virus E2 protein elicits strong humoral and cellular immune responses. Vaccine 23 (45), 5252–5262. doi: 10.1016/j.vaccine.2005.06.025
Liu, C., Liu, Y., Liang, L., Cui, S., Zhang, Y. (2019). RNA-Seq based transcriptome analysis during bovine viral diarrhoea virus (BVDV) infection. BMC Genomics 20 (1), 1–18. doi: 10.1186/s12864-019-6120-4
Maldonado, N., Fredericksen, F., Espiñeira, C., Toledo, C., Oltra, J., de la Barra, V., et al. (2020). BVDV-1 induces interferon-beta gene expression through a pathway involving IRF1, IRF7, and NF-κB activation. Mol. Immunol. 128, 33–40. doi: 10.1016/j.molimm.2020.09.018
Mätzener, P., Magkouras, I., Rümenapf, T., Peterhans, E., Schweizer, M. (2009). The viral RNase Erns prevents IFN type-I triggering by pestiviral single-and double-stranded RNAs. Virus Res. 140 (1-2), 15–23. doi: 10.1016/j.virusres.2008.10.015
Mesev, E. V., LeDesma, R. A., Ploss, A. (2019). Decoding type I and III interferon signalling during viral infection. Nat. Microbiol. 4 (6), 914–924. doi: 10.1038/s41564-019-0421-x
Mirosław, P., Polak, M. (2019). Increased genetic variation of bovine viral diarrhea virus in dairy cattle in Poland. BMC veterinary Res. 15 (1), 1–12. doi: 10.1186/s12917-019-2029-z
Mirosław, P., Rola-Łuszczak, M., Kuźmak, J., Polak, M. P. (2022). Transcriptomic analysis of MDBK cells infected with cytopathic and non-cytopathic strains of bovine viral diarrhea Virus (BVDV). Viruses 14 (6), 1276. doi: 10.3390/v14061276
Neill, J. D. (2013). Molecular biology of bovine viral diarrhea virus. Biologicals 41 (1), 2–7. doi: 10.1016/j.biologicals.2012.07.002
Nugroho, W., Silitonga, R. J. P., Reichel, M. P., Irianingsih, S. H., Wicaksono, M. S. (2022). The epidemiology and control of bovine viral diarrhoea virus in tropical Indonesian cattle. Pathogens 11 (2), 215. doi: 10.3390/pathogens11020215
Oguejiofor, C. F., Thomas, C., Cheng, Z., Wathes, D. C. (2019). Mechanisms linking bovine viral diarrhea virus (BVDV) infection with infertility in cattle. Anim. Health Res. Rev. 20 (1), 72–85. doi: 10.1017/S1466252319000057
Onomoto, K., Onoguchi, K., Yoneyama, M. (2021). Regulation of RIG-I-like receptor-mediated signaling: interaction between host and viral factors. Cell. Mol. Immunol. 18 (3), 539–555. doi: 10.1038/s41423-020-00602-7
Palomares, R. A., Parrish, J., Woolums, A. R., Brock, K. V., Hurley, D. J. (2014). Expression of toll-like receptors and co-stimulatory molecules in lymphoid tissue during experimental infection of beef calves with bovine viral diarrhea virus of low and high virulence. Veterinary Res. Commun. 38, 329–335. doi: 10.1007/s11259-014-9613-2
Peterhans, E., Jungi, T. W., Schweizer, M. (2003). BVDV and innate immunity. Biologicals 31 (2), 107–112. doi: 10.1016/S1045-1056(03)00024-1
Rajput, M. K., Abdelsalam, K., Darweesh, M. F., Braun, L. J., Kerkvliet, J., Hoppe, A. D., et al. (2017). Both cytopathic and non-cytopathic bovine viral diarrhea virus (BVDV) induced autophagy at a similar rate. Veterinary Immunol. Immunopathol. 193, 1–9. doi: 10.1016/j.vetimm.2017.09.006
Rajput, M. K., Darweesh, M. F., Braun, L. J., Mansour, S. M., Chase, C. C. (2020). Comparative humoral immune response against cytopathic or non-cytopathic bovine viral diarrhea virus infection. Res. veterinary Sci. 129, 109–116. doi: 10.1016/j.rvsc.2020.01.012
Rajput, M. K., Darweesh, M. F., Park, K., Braun, L. J., Mwangi, W., Young, A. J., et al. (2014). The effect of bovine viral diarrhea virus (BVDV) strains on bovine monocyte-derived dendritic cells (Mo-DC) phenotype and capacity to produce BVDV. Virol. J. 11 (1), 1–15. doi: 10.1186/1743-422X-11-44
Reus, J. B., Rex, E. A., Gammon, D. B. (2022). How to inhibit nuclear factor-kappa B signaling: lessons from poxviruses. Pathogens 11 (9), 1061. doi: 10.3390/pathogens11091061
Ridpath, J. F. (2013). Immunology of BVDV vaccines. Biologicals 41 (1), 14–19. doi: 10.1016/j.biologicals.2012.07.003
Roetman, J. J., Apostolova, M. K., Philip, M. (2022). Viral and cellular oncogenes promote immune evasion. Oncogene 41 (7), 921–929. doi: 10.1038/s41388-021-02145-1
Rojas, J. M., Alejo, A., Martín, V., Sevilla, N. (2021). Viral pathogen-induced mechanisms to antagonize mammalian interferon (IFN) signaling pathway. Cell. Mol. Life Sci. 78, 1423–1444. doi: 10.1007/s00018-020-03671-z
Shan, Y., Tong, Z., Jinzhu, M., Yu, L., Zecai, Z., Chenhua, W., et al. (2021). Bovine viral diarrhea virus NS4B protein interacts with 2CARD of MDA5 domain and negatively regulates the RLR-mediated IFN-β production. Virus Res. 302, 198471. doi: 10.1016/j.virusres.2021.198471
Shin, S. U., Han, D. G., Cho, H. C., Kim, E. M., Choi, K. S. (2023). Non-cytopathic bovine viral diarrhoea virus 2 induces autophagy to enhance its replication. Veterinary Med. Sci. 9 (1), 405–416. doi: 10.1002/vms3.1052
Song, Q., Zhao, X., Cao, C., Duan, M., Shao, C., Jiang, S., et al. (2022). Research advances on interferon (IFN) response during BVDV infection. Res. Veterinary Sci. 149, 151–158. doi: 10.1016/j.rvsc.2022.04.011
St-Louis, M.-C., Massie, B., Archambault, D. (2005). The bovine viral diarrhea virus (BVDV) NS3 protein, when expressed alone in mammalian cells, induces apoptosis which correlates with caspase-8 and caspase-9 activation. Veterinary Res. 36 (2), 213–227. doi: 10.1051/vetres:2004059
Suda, Y., Murakami, S., Horimoto, T. (2019). Bovine viral diarrhea virus non-structural protein NS4B induces autophagosomes in bovine kidney cells. Arch. Virol. 164, 255–260. doi: 10.1007/s00705-018-4045-x
Van Wyk, B., Snider, M., Scruten, E., Napper, S. (2016). Induction of functional interferon alpha and gamma responses during acute infection of cattle with non-cytopathic bovine viral diarrhea virus. Veterinary Microbiol. 195, 104–114. doi: 10.1016/j.vetmic.2016.09.015
Villalba, M., Fredericksen, F., Otth, C., Olavarría, V. (2016). Transcriptomic analysis of responses to cytopathic bovine viral diarrhea virus-1 (BVDV-1) infection in MDBK cells. Mol. Immunol. 71, 192–202. doi: 10.1016/j.molimm.2016.01.009
Wang, J., Chen, K.-Y., Wang, S.-H., Liu, Y., Zhao, Y.-Q., Yang, L., et al. (2023). Effects of spatial expression of activating transcription factor 4 on the pathogenicity of two phenotypes of bovine viral diarrhea virus by regulating the endoplasmic reticulum-mediated autophagy process. Microbiol. Spectr. 11 (2), e04225–e04222. doi: 10.1128/spectrum.04225-22
Wang, S., Hou, P., Pan, W., He, W., He, D. C., Wang, H., et al. (2021a). DDIT3 targets innate immunity via the DDIT3-OTUD1-MAVS pathway to promote bovine viral diarrhea virus replication. J. Virol. 95 (6), e02351-20. doi: 10.1128/jvi.02351-02320
Wang, T.-Y., Sun, M.-X., Zhang, H.-L., Wang, G., Zhan, G., Tian, Z.-J., et al. (2021b). Evasion of antiviral innate immunity by porcine reproductive and respiratory syndrome virus. Front. Microbiol. 12, 693799. doi: 10.3389/fmicb.2021.693799
Welsh, M., Adair, B., Foster, J. (1995). Effect of BVD virus infection on alveolar macrophage functions. Veterinary Immunol. Immunopathol. 46 (3-4), 195–210. doi: 10.1016/0165-2427(94)05366-Z
Yamane, D., Kato, K., Tohya, Y., Akashi, H. (2006). The double-stranded RNA-induced apoptosis pathway is involved in the cytopathogenicity of cytopathogenic Bovine viral diarrhea virus. J. Gen. Virol. 87 (10), 2961–2970. doi: 10.1099/vir.0.81820-0
Yamane, D., Nagai, M., Ogawa, Y., Tohya, Y., Akashi, H. (2005). Enhancement of apoptosis via an extrinsic factor, TNF-α, in cells infected with cytopathic bovine viral diarrhea virus. Microbes Infect. 7 (15), 1482–1491. doi: 10.1016/j.micinf.2005.05.006
Yesilbag, K., Alpay, G., Becher, P. (2017). Variability and global distribution of subgenotypes of bovine viral diarrhea virus. Viruses 9 (6), 128. doi: 10.3390/v9060128
Yuan, N., Song, Q., Jin, Y., Zhang, Z., Wu, Z., Sheng, X., et al. (2023). Replication of standard bovine viral diarrhea strain OregonC24Va induces endoplasmic reticulum stress–mediated apoptosis of bovine trophoblast cells. Cell Stress Chaperones 28 (1), 49–60. doi: 10.1007/s12192-022-01300-1
Zhang, Q., Lenardo, M. J., Baltimore, D. (2017). 30 years of NF-κB: a blossoming of relevance to human pathobiology. Cell 168 (1), 37–57. doi: 10.1016/j.cell.2016.12.012
Zhang, S., Wang, L., Cheng, G. (2022). The battle between host and SARS-CoV-2: Innate immunity and viral evasion strategies. Mol. Ther 30 (5), 1869–1884. doi: 10.1016/j.ymthe.2022.02.014
Zhu, J., Wang, C., Zhang, L., Zhu, T., Li, H., Wang, Y., et al. (2022). Isolation of BVDV-1a, 1m, and 1v strains from diarrheal calf in China and identification of its genome sequence and cattle virulence. Front. Veterinary Sci. 9, 1008107. doi: 10.3389/fvets.2022.1008107
Zhu, H., Zheng, C. (2020). The race between host antiviral innate immunity and the immune evasion strategies of herpes simplex virus 1. Microbiol. Mol. Biol. Rev. 84 (4), e00099-20. doi: 10.1128/mmbr.00099-00020
Keywords: bovine viral diarrhea virus (BVDV), immune evasion, innate immunity, adaptive immunity, therapies
Citation: Pang F, Long Q and Wei M (2023) Immune evasion strategies of bovine viral diarrhea virus. Front. Cell. Infect. Microbiol. 13:1282526. doi: 10.3389/fcimb.2023.1282526
Received: 24 August 2023; Accepted: 25 September 2023;
Published: 13 October 2023.
Edited by:
Ala E Tabor, The University of Queensland, AustraliaReviewed by:
Małgorzata Gieryńska, Warsaw University of Life Sciences, PolandCopyright © 2023 Pang, Long and Wei. This is an open-access article distributed under the terms of the Creative Commons Attribution License (CC BY). The use, distribution or reproduction in other forums is permitted, provided the original author(s) and the copyright owner(s) are credited and that the original publication in this journal is cited, in accordance with accepted academic practice. No use, distribution or reproduction is permitted which does not comply with these terms.
*Correspondence: Feng Pang, ZnBhbmdAZ3p1LmVkdS5jbg==