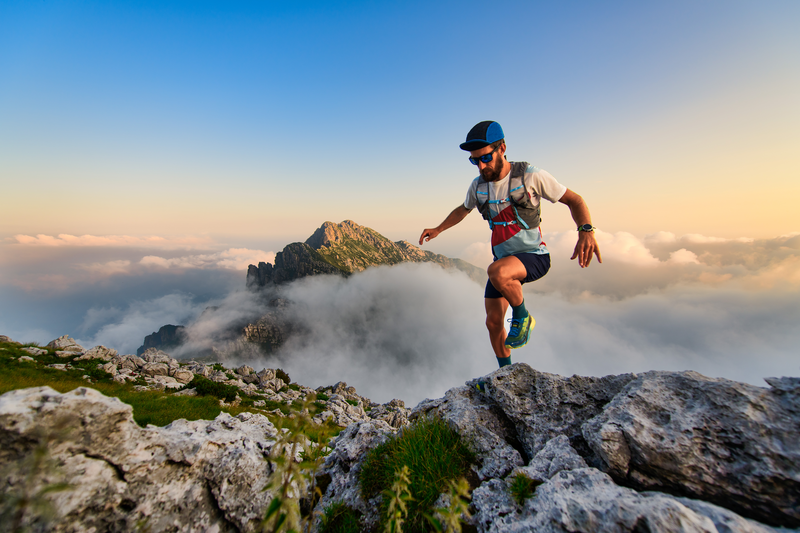
95% of researchers rate our articles as excellent or good
Learn more about the work of our research integrity team to safeguard the quality of each article we publish.
Find out more
EDITORIAL article
Front. Cell. Infect. Microbiol. , 30 August 2023
Sec. Microbes and Innate Immunity
Volume 13 - 2023 | https://doi.org/10.3389/fcimb.2023.1276271
This article is part of the Research Topic Understanding the Effects of Metabolites and Trace Minerals on Microbes During Infection View all 5 articles
Editorial on the Research Topic
Understanding the effects of metabolites and trace minerals on microbes during infection
Successful microbial pathogens establish infection and subvert the host immune response by utilizing their virulence determinants. Perhaps more importantly, the survival and replication of these pathogens within the microenvironments of infection sites depend on their ability to utilize alternative nutrient sources. This often involves the rewiring of metabolic pathways. Changes in microbial metabolic activities were historically used to identify microbial species by gold standard biochemical tests. However, over the last two decades, microbial metabolism has been neglected, particularly in favor of next generation sequencing.
The emerging field of immunometabolism, the intersection between host cellular metabolism and immune function, has reignited interest in microbial metabolism. This is because the metabolic reprogramming of host cells not only alters immune responses but also the nutritional environment that supports microbial growth. Conversely, microbial metabolism affects immune cell function by depleting immunoregulatory metabolites. Outcomes of host-pathogen interactions are further affected by the diet and microbiota-derived metabolites, not only in the gut but also in distal organs. This Frontiers Research Topic highlights the metabolic cross-talk between the host and pathogen during infection, the factors that affect these dynamics, and their consequences on the infection outcome.
Immunometabolism is an emerging concept that is central to both innate and adaptive immune regulation. The activation of specific metabolic pathways not only generates energy (ATP) but also dictates the function of immune cells (O'Neill et al., 2016). One prime example is the metabolic reprogramming which macrophages undergo following stimulation with the bacterial immunogen lipopolysaccharide (LPS) that fuels their pro-inflammatory signaling and microbicidal properties (O'Neill et al., 2016) (Figure 1 left panel). This involves switching from their basal metabolic state, mitochondrial oxidative phosphorylation (OXPHOS), to glycolysis. Several interruptions in the tricarboxylic acid (TCA) cycle are observed in LPS-stimulated macrophages, which result in the accumulation of the mitochondrial metabolites citrate, succinate, fumarate and itaconate and their export to the cytosol.
Figure 1 Metabolic activities govern the function of macrophages. (Left panel) Inflammatory (M1) macrophages accumulate the TCA metabolites citrate, succinate, fumarate and itaconate as a result of several breaks in the TCA cycle. Citrate, succinate and fumarate promote inflammation. Itaconate restores homeostasis via its anti-inflammatory and anti-oxidative properties. (Right panel) Anti-inflammatory (M2) macrophages downregulate glycolysis and upregulate metabolic pathways that fuel the TCA cycle, OXPHOS and the production of reactive oxygen species (ROS).
Citrate is converted to acetyl-coA that is directed towards the production of inflammatory lipid-mediators such as prostaglandins (PGs) (Infantino et al., 2011). Succinate stabilizes the transcription factor hypoxia-induced factor 1 alpha (HIF-1α), promoting glycolysis and the production of the pro-inflammatory cytokine IL-1β (Tannahill et al., 2013). Recently, fumarate was highlighted as a pro-inflammatory metabolite given its role in type I interferon (IFN) activation (Hooftman et al., 2023). Fumarate causes mitochondrial stress and damage, impairing respiration and releasing mitochondrial RNA (mtRNA), which induces the production of the cytokine IFNβ. Thus, the above-mentioned metabolites polarize macrophages to a pro-inflammatory M1-like phenotype. Following inflammation, itaconate is produced in the mitochondrial matrix by the enzyme aconitate decarboxylase 1, Acod1 (also called Irg1). Itaconate exerts anti-inflammatory and anti-oxidative properties to restore homeostatic balance (Peace and O'Neill, 2022).
In contrast, anti-inflammatory (M2-like) macrophages downregulate glycolysis and upregulate OXPHOS through catabolic pathways such as fatty acid oxidation (FAO) and glutaminolysis (Jha et al., 2015; Liu et al., 2017) (Figure 1 right panel), which can also play a critical role in resolving infection. For example, recruitment of M2-like macrophages facilitates the transition into a post-inflammatory resolution phase that is critical to resolving staphylococcal skin/soft tissue infections (Thurlow et al., 2018). Interestingly, it is increasingly being recognized that microbial pathogens can subvert immunometabolism to promote infection (Yamada et al., 2020; Wong Fok Lung et al., 2022; Tomlinson et al., 2023).
Live microbial pathogens impose a profound metabolic stress on the host that can override the macrophage reprogramming associated with LPS or other pathogen-associated molecular patterns (PAMPs). For example, during pulmonary infection, multidrug-resistant Klebsiella pneumoniae induces an airway metabolic response distinct from that stimulated by the heat-killed pathogen or its purified LPS (Wong Fok Lung et al., 2022). This response is characterized by an increase in metabolic pathways that fuel OXPHOS, promoting immunosuppression and tolerance to infection, rather than glycolysis that is associated with the highly pro-inflammatory signaling necessary for bacterial clearance. In addition, the host metabolite itaconate is particularly prominent during infection with metabolically-active K. pneumoniae. Absence of this immunomodulatory metabolite abrogates host tolerance to K. pneumoniae infection and is instead accompanied by excessive inflammation and immunopathology (Wong Fok Lung et al., 2022). The role of itaconate and other anti-inflammatory metabolites such as adenosine in promoting immunosuppression in myeloid cells is discussed by Urso and Prince.
The Gram-positive bacterial pathogen, Staphylococcus aureus, employs a similar metabolic strategy to cause persistent infection. The ability of staphylococcal biofilms to dampen inflammation via the induction of immunosuppressive myeloid cells and their production of the anti-inflammatory cytokine IL-10 is attributed to S. aureus production of lactate (Heim et al., 2020). This metabolite inhibits histone deacetylase 11 (HDAC11) to enhance Il-10 transcription. These highlight the crucial contribution of active bacterial metabolism in contrast to PAMPS in determining the outcome of the infection through metabolic cross-talks.
Whilst pathogens can manipulate immunometabolism to evade immune clearance, they must also adapt to the metabolic milieu to ensure their survival. Several metabolites and metabolic by-products that are produced by the host and microbial cells during infection are toxic, thereby imposing a strong selective pressure that drives microbial adaptation. Urso and Prince provide a detailed overview of how bacterial pathogens alter their own metabolism and transcriptional profile to optimize their fitness, particularly in response to the electrophile itaconate and to reactive oxygen species.
In addition, Mitchell and Ellermann focus on long chain fatty acids (LCFAs), derived from host and microbial cells as well as dietary sources, that act as signaling molecules and regulate virulence in enteric pathogens.
Host-pathogen dynamics are further complexed by commensal microbes and their metabolism. For example, short-chain fatty acids (SCFAs), the end products of the fermentation of dietary fibers by the anaerobic intestinal microbiome, have been associated with positive outcomes following microbial infections as well as other inflammatory diseases. In a mini review, Zhang et al. summarize the beneficial effects of SCFAs on the immune response to sepsis-associated encephalopathy (SAE). These are mediated by their anti-inflammatory and antioxidant properties. Lopez et al. discuss the immunomodulatory and therapeutic effects of SCFAs in inflammatory bowel disease (IBD) and close the cycle of host-pathogen-microbiota interactions by examining host factors that alter the gut microbiota and their production of microbiome-derived metabolites.
The articles in this Research Topic illustrate how the metabolic cross-talk between host, pathogen and microbiome affects the outcome of infection. The metabolic potential of microbes as a virulence strategy is being revisited in light of the more recent studies documenting the impact of microbial metabolic activity in shaping the immune response to in vivo infection (Heim et al., 2020; Tomlinson et al., 2021; Wong Fok Lung et al., 2022; Tomlinson et al., 2023). In addition, the ability of the bacteria to co-opt metabolites within the microenvironment enables their survival and adaptation.
TWFL: Writing – original draft, Writing – review & editing. TN, AMu, and Amo: Writing – review & editing
TN is supported by NHMRC (Australia) Ideas grant 1163556. AMu is supported by an EMBL-EBI and Wellcome Sanger Institute Research Fellowship. TWFL is supported by NIH (USA) grant K99 HL157550.
Figure 1 was created with BioRender.com.
The authors declare that the research was conducted in the absence of any commercial or financial relationships that could be construed as a potential conflict of interest.
All claims expressed in this article are solely those of the authors and do not necessarily represent those of their affiliated organizations, or those of the publisher, the editors and the reviewers. Any product that may be evaluated in this article, or claim that may be made by its manufacturer, is not guaranteed or endorsed by the publisher.
Heim, C. E., Bosch, M. E., Yamada, K. J., Aldrich, A. L., Chaudhari, S. S., Klinkebiel, D., et al. (2020). Lactate production by Staphylococcus aureus biofilm inhibits HDAC11 to reprogramme the host immune response during persistent infection. Nat. Microbiol. 5 (10), 1271–1284. doi: 10.1038/s41564-020-0756-3
Hooftman, A., Peace, C. G., Ryan, D. G., Day, E. A., Yang, M., McGettrick, A. F., et al. (2023). Macrophage fumarate hydratase restrains mtRNA-mediated interferon production. Nature 615 (7952), 490–498. doi: 10.1038/s41586-023-05720-6
Infantino, V., Convertini, P., Cucci, L., Panaro, M. A., Di Noia, M. A., Calvello, R., et al. (2011). The mitochondrial citrate carrier: a new player in inflammation. Biochem. J. 438 (3), 433–436. doi: 10.1042/BJ20111275
Jha, A. K., Huang, S. C., Sergushichev, A., Lampropoulou, V., Ivanova, Y., Loginicheva, E., et al. (2015). Network integration of parallel metabolic and transcriptional data reveals metabolic modules that regulate macrophage polarization. Immunity 42 (3), 419–430. doi: 10.1016/j.immuni.2015.02.005
Liu, P. S., Wang, H., Li, X., Chao, T., Teav, T., Christen, S., et al. (2017). alpha-ketoglutarate orchestrates macrophage activation through metabolic and epigenetic reprogramming. Nat. Immunol. 18 (9), 985–994. doi: 10.1038/ni.3796
O'Neill, L. A., Kishton, R. J., Rathmell, J. (2016). A guide to immunometabolism for immunologists. Nat. Rev. Immunol. 16 (9), 553–565. doi: 10.1038/nri.2016.70
Peace, C. G., O'Neill, L. A. (2022). The role of itaconate in host defense and inflammation. J. Clin. Invest. 132 (2), e148548. doi: 10.1172/JCI148548
Tannahill, G. M., Curtis, A. M., Adamik, J., Palsson-McDermott, E. M., McGettrick, A. F., Goel, G., et al. (2013). Succinate is an inflammatory signal that induces IL-1beta through HIF-1alpha. Nature 496 (7444), 238–242. doi: 10.1038/nature11986
Thurlow, L. R., Joshi, G. S., Richardson, A. R. (2018). Peroxisome Proliferator-Activated Receptor gamma Is Essential for the Resolution of Staphylococcus aureus Skin Infections. Cell Host Microbe 24 (2), 261–70 e4. doi: 10.1016/j.chom.2018.07.001
Tomlinson, K. L., Riquelme, S. A., Baskota, S. U., Drikic, M., Monk, I. R., Stinear, T. P., et al. (2023). Staphylococcus aureus stimulates neutrophil itaconate production that suppresses the oxidative burst. Cell Rep. 42 (2), 112064. doi: 10.1016/j.celrep.2023.112064
Tomlinson, K. L., Wong Fok Lung, T., Dach, F., Annavajhala, M. K., Gabryszewski, S. J., Groves, R. A., et al. (2021). Staphylococcus aureus induces an itaconate-dominated immunometabolic response that drives biofilm formation. Nat. Commun. 12 (1), 1399. doi: 10.1038/s41467-021-21718-y
Wong Fok Lung, T., Charytonowicz, D., Beaumont, K. G., Shah, S. S., Sridhar, S. H., Gorrie, C. L., et al. (2022). Klebsiella pneumoniae induces host metabolic stress that promotes tolerance to pulmonary infection. Cell Metab. 34 (5), 761–74.e9. doi: 10.1016/j.cmet.2022.03.009
Keywords: immunometabolism, host-pathogen interaction, bacterial metabolism, microbial metabolic activity, microbiota-derived metabolites, itaconate, adenosine, metabolic cross talk
Citation: Naderer T, Mu A, Monteith AJ and Wong Fok Lung T (2023) Editorial: Understanding the effects of metabolites and trace minerals on microbes during infection. Front. Cell. Infect. Microbiol. 13:1276271. doi: 10.3389/fcimb.2023.1276271
Received: 11 August 2023; Accepted: 16 August 2023;
Published: 30 August 2023.
Edited and Reviewed by:
Annemarie H. Meijer, Leiden University, NetherlandsCopyright © 2023 Naderer, Mu, Monteith and Wong Fok Lung. This is an open-access article distributed under the terms of the Creative Commons Attribution License (CC BY). The use, distribution or reproduction in other forums is permitted, provided the original author(s) and the copyright owner(s) are credited and that the original publication in this journal is cited, in accordance with accepted academic practice. No use, distribution or reproduction is permitted which does not comply with these terms.
*Correspondence: Tania Wong Fok Lung, dHcyNTk1QGN1bWMuY29sdW1iaWEuZWR1
Disclaimer: All claims expressed in this article are solely those of the authors and do not necessarily represent those of their affiliated organizations, or those of the publisher, the editors and the reviewers. Any product that may be evaluated in this article or claim that may be made by its manufacturer is not guaranteed or endorsed by the publisher.
Research integrity at Frontiers
Learn more about the work of our research integrity team to safeguard the quality of each article we publish.