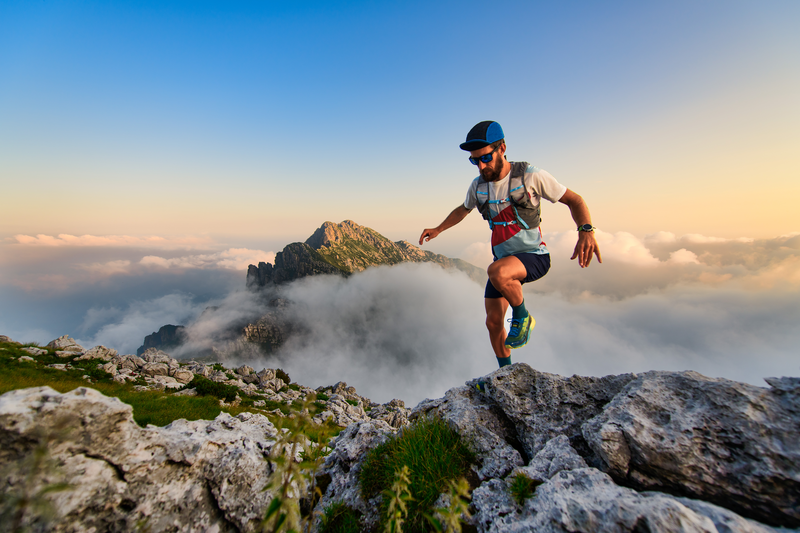
95% of researchers rate our articles as excellent or good
Learn more about the work of our research integrity team to safeguard the quality of each article we publish.
Find out more
ORIGINAL RESEARCH article
Front. Cell. Infect. Microbiol. , 11 October 2023
Sec. Bacteria and Host
Volume 13 - 2023 | https://doi.org/10.3389/fcimb.2023.1271912
This article is part of the Research Topic Effects of Dietary Nutrients on Intestinal Microbiome: Insights into Gastrointestinal Diseases in Animals View all 9 articles
The Chinese soft-shelled turtle (Pelodiscus sinensis) has become increasingly susceptible to frequent diseases with the intensification of farming, which severely impacts the development of the aquaculture industry. Sodium butyrate (SB) is widely used as a feed additive due to its promotion of growth, enhancement of immune function, and antioxidative properties. This study aimed to investigate the effects of dietary SB on the growth performance, immune function, and intestinal microflora of Chinese soft-shelled turtles. A total of 300 Chinese soft-shelled turtles (mean weight: 11.36 ± 0.21g) were randomly divided into four groups with three parallel sets in each group. Each group was fed a diet supplemented with 0%, 0.005%, 0.01%, or 0.02% SB for 60 days. The results demonstrated an upward trend in weight gain rate (WGR) and specific growth rate (SGR) with increasing SB supplementation, and the experimental group fed with 0.02% SB showed a significant increase in WGR and SGR compared to other groups (P< 0.05). These levels of SB also decreased the levels of feed conversion ratio (FCR) and the total cholesterol (TC) content of Chinese soft-shelled turtles, and the 0.02% SB was significantly lower than that of other groups (P< 0.05). The activity of complement protein in vivo increased with increases in SB content, and the activities of complement C3 and C4 reached the highest level with 0.02% SB. The species abundance of the experimental group D fed with 0.02% SB was significantly higher than that of other groups (P< 0.05). Furthermore, the relative abundance of Clostridium sensu stricto 1 was significantly increased with 0.02% SB (P< 0.05). In conclusion, adding 0.02% SB to the diet improves the growth performance, feed digestion ability, and intestinal microbiota of Chinese soft-shelled turtles.
The Chinese soft-shelled turtle (Pelodiscus sinensis) is a benthic aquatic animal that inhabits freshwater environments. Due to its highly sought-after nutritional value, it occupies a prominent position in traditional aquaculture in China. Renowned for its richness in protein and low-fat content, it remains a popular choice in the market. Among its various components, the skirt edge stands out with the highest crude protein content. Furthermore, the muscle of the Chinese soft-shelled turtle boasts an essential amino acid index exceeding 90%, making it a highly nutritious option (Huang and Bao, 2023). Many ponds in China cultivate Chinese soft-shelled turtles to meet market demands. However, with the intensification and refinement of cultivation, high breeding densities and insufficient carrying capacity in the ponds disrupt the aquatic animals’ mechanisms and bacterial balance (Yarahmadi et al., 2015), resulting in the proliferation of bacterial pathogens and subsequent deaths of Chinese soft-shelled turtles due to bacterial diseases (Chung et al., 2017). Previous studies have demonstrated that too many fish raised in a pond beyond its environmental capacity could trigger diseases and cause environmental pollution (Tang et al., 2021). In such intensive environments, aquatic animals reduce their feed intake and intestinal digestive capacity. To enhance the growth and immune ability of aquatic animals, numerous studies have been conducted on feed additives.
At present, SB is widely used as a feed additive due to its ability to promote growth and improve immune function and antioxidant properties (Abdel-Latif et al., 2020). Studies have demonstrated that SB can provide a fast and easily absorbed energy source for animals and exhibit a diverse array of biological effects (Cummings and Macfarlane, 1997). Furthermore, some researchers found that SB can repair the intestinal epidermal mucosa, which has protective effects on the intestinal tract of aquatic animals (Du et al., 2023). The profound impact of SB on the health of aquatic animals has led to its widespread adoption as a feed additive. (Tran et al., 2020). Although there are reports on the effects of SB supplementation in fish diets, studies on its supplementation in turtle diets are required. Hence, this study aimed to investigate the effects of SB on the growth performance, immune function, and gut microbiota of Chinese soft-shelled turtles.
Table 1 shows the nutritional composition of the basic feed. The protein source of the experimental feed was imported fish meal, soybean meal, and other raw materials, and the fat source was fish oil, peanut oil, and other raw materials. Four treatment diets were prepared by adding 0%, 0.005%, 0.01%, or 0.02% SB to the basic diet. The raw materials were crushed using an 80-mesh screen to remove large particles, and then precisely weighed according to the proportion of the feed formula. The mixed feed was put into a batch mixer, and a fixed amount of distilled water was added for full mixing. Then, the mixed feed was made into particles with a 1.5-mm diameter using a ring mold granulating machine and dried in an oven at 60 °C. Finally, the feed was air-dried and collected in sealed bags, which were stored in a freezer at −25 °C.
The 300 healthy Chinese soft-shelled turtles (mean weight: 11.36 ± 0.21 g) were obtained from Changde Hezhou Aquatic Products Co., Ltd, Changde, China. All animal studies were performed according to protocols approved by the Animal Care Advisory Committee of Animal Science and Technology, Hunan Agricultural University. They were randomly divided into four groups: the control group (group A) was fed experimental diets without SB, while the experimental groups B, C, and D were fed experimental diets supplemented with 0.005%, 0.01%, and 0.02% SB (Hangzhou Kantian Biotechnology Co., Ltd, Hangzhou, China), respectively. The Chinese soft-shelled turtles were raised in an indoor glass tank (1 m × 0.5 m × 0.5 m, length × width × depth) with water exchanged once every 2 weeks to ensure optimal growth conditions. To control the variables, the source of water change during feeding was purchased pure water, and the water temperature in the glass tank was kept at 25 °C~32 °C. Before the trial, Chinese soft-shelled turtles were starved for 2 days. At the beginning of the experiment, the body weight of the Chinese soft-shelled turtle was recorded, and they were fed twice a day with a prescribed dosage of 2% of their body mass. The feeding quantity was adjusted by weighing the turtles every 2 weeks. The remaining feed was collected one hour after each feeding to avoid its influence on water quality. The growth performance of each group was tested at 30 d and 60 d. At 60 d, 7 Chinese soft-shelled turtles were randomly selected from each group, and serum immune indexes and intestinal microbial population data were collected. The control group (group A) consisted of Chinese soft-shelled turtles labeled A1–A7, while the experimental groups B, C, and D consisted of Chinese soft-shelled turtles labeled B1–B6, C1–C7, and D1–D7, respectively.
Each group of Chinese soft-shelled turtle individuals was weighed on day 30 and day 60 of the experiment, and the body weight gain, body length, and number of deaths of Chinese soft-shelled turtle were recorded. Their daily food intake was calculated, and their liver mass was weighed. The following formulas were used to calculate their growth performance:
The variables used in the calculations were as follows: Wt represents the body mass of Chinese soft-shelled turtles at 30 d and 60 d; W0 stands for their initial mass; t represents the number of days of testing; WTC stands for the total food intake during the trial phase; n represents the number of turtles in each group; Wh stands for their liver mass; Nt stands for the final number of turtles; and N0 represents their initial number.
After feeding, 7 Chinese soft-shelled turtles were randomly selected from each group for blood collection. The collected blood was placed in a refrigerator at 4 °C for 4 h, followed by centrifugation for 10 min at 4 °C and 5000 RPM. After centrifugation, the upper serum was collected and serum indexes were detected using the kit from Nanjing Jiancheng Bioengineering Research Institute. The serum biochemical indexes included TC, triglyceride (TG), total protein (TP), aspartate aminotransferase (AST), and alanine aminotransferase (ALT). Serum immune indices were superoxide dismutase (SOD), malondialdehyde (MDA), and complement C3 and C4.
To study the intestinal microbial population of Chinese soft-shelled turtles, we sampled the intestinal tract of experimental and control groups. Before sampling, we anesthetized the turtles by placing them in water with MS-222 (100 μg/L) for 2 min. We then wiped 75% ethanol on the surface of the turtles, quickly disassembled them, removed their intestine, and squeezed the intestinal contents into a 5-ml centrifuge tube. The samples were then frozen with liquid nitrogen and stored at −80 °Cfor analysis of intestinal flora changes.
The intestinal genome DNA of Chinese soft-shelled turtles was extracted and sequenced using the combined multiomics technology of Shanghai Oyi Biotics. Genomic DNA was extracted from the sample with a DNA extraction kit, and the DNA concentration was detected using agarose gel electrophoresis and a NanoDrop spectrophotometer. The extracted genomic DNA was used as a template to delimit the genomic sequencing area, and specific primers were added to the DNA template, followed by mixing. The mixture was then put into a PCR instrument (Takara) for testing, and the accuracy and validity of the detection value were guaranteed.
The raw data obtained were in FASTQ format, and Cutadapt software was used to process the raw sequence to cut off the primer sequence. After that, DADA2 was used to process the original sequence according to Qiime2 setting procedures to obtain high-quality sequences and amplicon sequence variants (ASV) abundance tables. The diversity of the obtained data was analyzed, and the intestinal microflora information of Chinese soft-shelled turtles was obtained through various algorithms. This information was used to analyze SB-driven changes in the intestinal microflora of Chinese soft-shelled turtles.
Statistical analysis was performed according to Excel to sort out the recorded data. The recorded data results were expressed as Avg ± SD. The normality of the data was tested by SPSSAU, and then Duncan was used to conduct one-way ANOVA on the obtained data to identify significant differences (P< 0.05).
According to Tables 2, 3, the WGR and SGR of Chinese soft-shelled turtles supplemented with SB showed an upward trend with increases in SB concentration. WGR and SGR values in group D (0.02% SB) were significantly higher than those in the other groups (P< 0.05). The FCR showed a decreasing trend with increases in SB concentration. FCR values in group D (0.02% SB) were significantly lower than those in the other groups (P< 0.05). Although the HSI of each group fluctuated, the overall difference was not significant (P > 0.05).
As shown in Table 4, the TC content of Chinese soft-shelled turtles showed a decreasing trend with increased SB concentration. The TC content in group D (0.02% SB) was significantly different from that of other groups (P< 0.05). There were no significant differences in other indexes (P > 0.05).
According to Table 5, changes in indicators in the serum of Chinese soft-shelled turtles can affect their immune ability. It was found that the activity of complement protein increased with increases in SB concentrations, and the activities of C3 and C4 peaked at 0.02% SB, being significantly different from those of other groups (P< 0.05). In the serum immune index data, SOD and malondialdehyde showed a dynamic equilibrium state, indicating that SB had no significant effects on them (P > 0.05).
The intestinal sample of Chinese soft-shelled turtles was used to obtain 2,247,884 original sequences from 27 samples by high-throughput sequencing. The primers in the original sequences were cut by Cutadapt, and qualified original sequences were input into Qiime2 after quality filtering, noise reduction, splicing, and dechimerism. A total of 1,844,376 effective high-quality sequences were obtained, with an average effective data ratio of 82%. In DADA2, noise reduction, chimera removal, and duplication removal were performed according to the set procedures, and the resulting sequences were called ASVs. The number of tags assigned to ASVs in each sample was counted separately to determine the abundance of each ASV in each sample. The ASVs of groups A, B, C, and D were 1186, 1208, 1114, and 1300, respectively. As shown in Table 6, intestinal sample sequencing data and ASV abundance statistics were used to analyze the intestinal flora diversity of Chinese soft-shelled turtles.
Based on Figure 1, 7 ASVs were shared by both experimental and control groups. The numbers displayed in the petal diagram indicated the total ASVs subtracted by the number of common ASVs in each sample, and the unique ASVs of groups A, B, C, and D were 1179, 1201, 1107, and 1293, respectively. Moreover, the species abundance of group D (0.02% SB) was found to be significantly higher than that of other groups (P< 0.05).
Figure 1 Intestinal flora of Chinese soft-shelled turtle. A1-A7: Control group; B1-B6: Experimental group (0.005% SB); C1-C7: Experimental group (0.01% SB); D1-D7: Experimental group (0.02% SB).
Based on the diversity analysis of the top 15 species abundance in the phylum classification level of intestinal bacteria, as illustrated in Figure 2, Bacteroidota, Firmicutes, and Proteobacteria were found to be the dominant bacteria present in the intestinal flora of the 4 groups of Chinese soft-shelled turtles. Among them, Bacteroidota accounted for 50.3% in the top 15 species abundance of phylum, while the other two Bacteroidota accounted for 28% and 17.6%, respectively.Furthermore, the relative abundances of Bacteroidota and Campilobacterota in experimental group D were significantly higher compared to those of the control group (P< 0.05), with the relative abundance of Campilobacterota showing a rising trend with increases in SB. However, the relative abundance of Bacteroidota in experimental group C was significantly higher than that of other groups (P< 0.05), with an SB efficiency rate of 41.34%. Meanwhile, the relative abundances of Proteobacteria, Actinobacteriota, and Desulfobacterota in the experimental groups notably decreased in contrast to those of the control group (P< 0.05), and they exhibited a declining trend with increases in SB.
Figure 2 The top 15 bacteria in the intestinal phylum classification level of species abundance. A1-A7: Control group; B1-B6: Experimental group (0.005% SB); C1-C7: Experimental group (0.01% SB); D1-D7: Experimental group (0.02% SB).
The diversity analysis of the top 15 species abundance in the genus classification level of intestinal bacteria is depicted in Figure 3. Bacteroides and Citrobacter were identified as the main dominant bacterial genera present in the intestinal flora of the four groups of Chinese soft-shelled turtles, respectively accounting for 11.9% and 7.19% of the top 15 species abundance at the classification level.
Figure 3 The top 15 bacteria in the intestinal genus classification level of species abundance. A1-A7: Control group; B1-B6: Experimental group (0.005% SB); C1-C7: Experimental group (0.01% SB); D1-D7: Experimental group (0.02% SB).
Moreover, the relative abundance of Bacteroides and Citrobacter in all experimental groups was significantly higher than that of the control group (P< 0.05). Interestingly, in contrast to the other conditions, the content of SB in the two bacterial groups exhibited an inverse proportion to the species abundance, with a downward trend observed. Additionally, the relative abundance of Ralstonia and Weissella in the experimental group significantly decreased compared to that in the control group (P< 0.05), showing a curve decline. Furthermore, the total species abundance in group C (0.01% SB) was the lowest.
LefSe analysis is a useful tool for identifying microbial communities that exhibit significant differences in sample distribution. LDA score is typically utilized to determine the impact of a certain microbiome on the abundance, with a judgment criterion of LDA≥2. As shown in Figure 4A, 9 significant bacterial genera were identified in the control group, with Cetobacterium holding the LDA score of 4.37. On the other hand, 18 bacterial genera played an important role in the experimental group, with Clostridium sensu stricto 1 exhibiting the highest LDA score of 4.65, which was the highest among those of all identified genera. In terms of relative abundance, Clostridium sensu stricto 1 and Fusobacteriia are the genera with the most pronounced variations. The cladogram revealed the most correlated branches among groups, which was consistent with the above-mentioned results (Figure 4B).
Figure 4 (A) LEfSe analysis of intestinal flora. (B) Evolutionary branches of phylogenetic relationships. A: Control group; B: Experimental group (0.005% SB); C: Experimental group (0.01% SB); D: Experimental group (0.02% SB).
Nowadays, organic acid products are widely used as feed additives to address issues in aquaculture and promote the high-quality development of aquatic products (Dawood et al., 2021; Sotoudeh and Esmaeili, 2022; Abdel-Latif et al., 2020). SB, as an organic acid, has been found to have a significant positive effect on the growth performance of aquatic animals and plays a crucial role in the production of aquatic products (Khalil et al., 2018; Abdel-Latif et al., 2020).
According to the experimental results, dietary SB significantly improved the growth performance of Chinese soft-shelled turtles and promoted feed conversion efficiency, with the strongest effect achieved at an SB concentration of 0.02%. Similar results were also obtained in other studies (da Silva et al., 2016; Abdel-Latif et al., 2021; Mehrgan et al., 2022), all of which showed that adding SB to the diet of aquatic animals could improve their growth efficiency and accelerate their flesh growth.
Studies have found that the long-term consumption of diets containing SB increases intestinal thickness, which in turn increases the contact area between food and the intestinal tract, improves the absorption efficiency of nutrient elements, and promotes the growth of Nile tilapia juveniles (Abdel-Mohsen et al., 2018; Abdel-Tawwab et al., 2021). Additionally, Yamamoto et al. found that disorders in golden drum fish were reversed after adding SB to their feed during the early stages of breeding (Yamamoto et al., 2021). Moreover, Jesus et al. found that SB had little effect on the growth performance of protected and non-protected tilapia (Jesus et al., 2019). This study indicated that there was a dose-benefit relationship between SB and growth performance, which was consistent with the conclusions of Ullah Sami et al. (Ullah et al., 2020) and Zhou et al. (Zhou et al., 2019). Therefore, the efficacy of SB on the growth performance of Chinese soft-shelled turtles depends on the concentration added. When used at an appropriate concentration, SB can greatly improve their growth and feeding efficiency.
Biochemical indexes are important parameters for assessing the welfare of aquatic animals (Mitranescu et al., 2013), and adjusting these indexes can better meet the living conditions of aquatic animals and promote their growth and development. In our study, TC levels in the blood of Chinese soft-shelled turtles fed with SB were lower than those of turtles fed without it, and this was closely related to the increase in SB concentration (Zong et al., 2022). Studies have shown that adding 1–2 mg of SB to the diet of Nile tilapia juveniles can significantly improve the activities of TP and transaminase (Abdel-Latif et al., 2021). Additionally, the TP content in the blood of Nile tilapia was significantly increased when fed a diet containing SB (El-Sayed Ali et al., 2018; Dawood et al., 2020). The concentration of transaminase reflects liver health and function (Kou et al., 2021). Based on the results obtained in this study, there were no significant differences in TP, transaminase, or other indicators between Chinese soft-shelled turtles fed with SB and those in the control group. Furthermore, SB supplementation led to no adverse effects on the original health of Chinese soft-shelled turtles.
Total antioxidant capacity can reflect the enzyme activity in the immune system and inhibit peroxide products in vivo (Yarahmadi et al., 2022). SOD can remove free radicals in the body and protect tissues and cells from oxidative interference. Studies have indicated that enzyme activity in yellow catfish was enhanced when fed with 500 or 1000 mg/kg of SB, reaching significantly higher levels than those of the control group (Zhao et al., 2021). However, data on SOD expression in our study differed from these findings. The reason may be that SB has varying effects on different aquatic animals, which requires further research.
Li Tian et al. found that SB could improve complement protein content and enhance immune ability in juvenile grass carp (Tian et al., 2017). As a product of the body’s liver, complement proteins can eliminate inflammation from the body, which has been found that made significant progress in the immunity of aquatic animals in previous studies (Uribe et al., 2011), leading to the rapid development of the aquatic industry. In this experiment, feed supplemented with SB resulted in a significant enhancement in the levels of complement proteins, which aligns with the findings mentioned in the literature. This indicated that the addition of SB to the feed can enhance the immune system of Chinese soft-shelled turtles.
The results of our experiment showed that feeding diets containing SB had a positive effect on the beneficial intestinal microflora of Chinese soft-shelled turtles, which is consistent with the findings of Wing‐Keong Ng et al. (Ng and Koh, 2017). It has been reported that SB can increase intestinal acidity and inhibit the abundance of harmful microbial populations (Ricke, 2003). However, the mechanism by which SB can promote changes in intestinal microbial flora is not fully understood (Dibner and Buttin, 2002). It is generally believed that SB can form a physical barrier in the intestine to protect the intestinal microbial flora from damage (Wu et al., 2018).
In this experiment, adding 0.02% SB to the diet significantly improved the intestinal tract of Chinese soft-shelled turtles, enhanced the abundance of beneficial microbial populations in the intestine, and promoted intestinal digestion and absorption, which is similar to the conclusion of Han et al. (Han et al., 2020). Briefly, at the phylum level, Proteobacteria, Firmicutes, and Bacteroidota were found to be the dominant phyla. At the genus level, Citrobacter and Bacteroides were found to be the main dominant bacteria. Polysaccharides in the gut require specific digestive enzymes to decompose them due to their different structure compared to other foods (Porter and Martens, 2017). When these digestive enzymes are lacking in the intestine, bacteria in this organ must decompose them for absorption (Ai et al., 2019). In Chinese soft-shelled turtles, Bacteroides has been found to be predominant in the intestinal tract, which can digest dietary fiber polysaccharides and main carbohydrates (Porter et al., 2018), promoting the digestion and absorption of carbohydrates in the intestine and overall growth. This study found that the abundance of Bacteroides species in the intestinal tract of Chinese soft-shelled turtles increased, which improved the efficiency of feed digestion and utilization and accelerated the growth of Chinese soft-shelled turtles supplemented with an SB diet. Kan et al. (Kan et al., 2015), found that when the external environment changed, the microbial flora in the gut of crucian carp would be adjusted in a beneficial direction.
This study showed that SB had a beneficial effect on the intestinal tract of Chinese soft-shelled turtles, improving their growth performance, blood indexes, and intestinal microbiota compared to those in the control group. When the indexes changed, other influencing factors also changed. However, our exploration of the mechanism of the influence of SB on intestinal microorganisms is limited, and further research is needed to provide a reference for the subsequent establishment of an SB dietary supplementation system for Chinese soft-shelled turtles.
In conclusion, 0.02% SB supplementation could improve the growth performance, feed digestibility, and gut microbiota of Chinese soft-shelled turtles, promoting their healthy growth. In addition, this study found that SB improved the serum biochemical indexes and immune ability of Chinese soft-shelled turtles, including the activity of blood complement proteins. Subsequent trials should continue to investigate SB as a diet supplement for Chinese soft-shelled turtles and explore the actual dosage range of SB.
The data presented in the study are deposited in the SequenceRead Archive (https://www.ncbi.nlm.nih.gov/sra), under accession number PRJNA1000466 (https://www.ncbi.nlm.nih.gov/bioproject/PRJNA1000466/).
The animal study was approved by College of Fisheries, Hunan Agriculture University. The study was conducted in accordance with the local legislation and institutional requirements.
XW, ZD, and YH conceived the study. LG, YY, and KL performed the experiments. LG, HX, and QL analyzed the data. LG, YY, and SL visualized the statistical results. LG wrote the draft. XW, and YH wrote and edited the final manuscript. ZL improved the language and grammar of the manuscript. All authors contributed to the article and approved the submitted version.
The authors declare financial support was received for the research, authorship, and/or publication of this article. This work was supported by the Natural Science Foundation of Hunan Province (2018JJ3265), the National Natural Science Foundation of China (82102428).
The authors declare that the research was conducted in the absence of any commercial or financial relationships that could be construed as a potential conflict of interest.
All claims expressed in this article are solely those of the authors and do not necessarily represent those of their affiliated organizations, or those of the publisher, the editors and the reviewers. Any product that may be evaluated in this article, or claim that may be made by its manufacturer, is not guaranteed or endorsed by the publisher.
Abdel-Latif, H. M., Abdel-Tawwab, M., Dawood, M. A., Menanteau-Ledouble, S., El-Matbouli, M. (2020). Benefits of dietary butyric acid, sodium butyrate, and their protected forms in aquafeeds: a review. Rev. Fish. Sci. Aquacult. 28 (4), 421–448. doi: 10.1080/23308249.2020.1758899
Abdel-Latif, H. M., Hendam, B. M., Shukry, M., El-Shafai, N. M., El-Mehasseb, I. M., Dawood, M. A., et al. (2021). Effects of sodium butyrate nanoparticles on the hemato-immunological indices, hepatic antioxidant capacity, and gene expression responses in Oreochromis niloticus. Fish Shellfish Immunol. 119, 516–523. doi: 10.1016/j.fsi.2021.10.039
Abdel-Mohsen, H. H., Wassef, E. A., El-Bermawy, N. M., Abdel-Meguid, N. E., Saleh, N. E., Barakat, K. M., et al. (2018). Advantageous effects of dietary butyrate on growth, immunity response, intestinal microbiota and histomorphology of European Seabass (Dicentrarchus labrax) fry. Egyptian J. Aquat. Biol. Fish. 22 (4), 93–110. doi: 10.21608/EJABF.2018.12055
Abdel-Tawwab, M., Shukry, M., Farrag, F. A., El-Shafai, N. M., Dawood, M. A., Abdel-Latif, H. (2021). Dietary sodium butyrate nanoparticles enhanced growth, digestive enzyme activities, intestinal histomorphometry, and transcription of growth-related genes in Nile tilapia juveniles. Aquaculture 536, 736467. doi: 10.1016/j.aquaculture.2021.736467
Ai, C., Jiang, P., Liu, Y., Duan, M., Sun, X., Luo, T., et al. (2019). The specific use of alginate from Laminaria japonica by Bacteroides species determined its modulation of the Bacteroides community. Food Funct. 10 (7), 4304–4314. doi: 10.1039/C9FO00289H
Chung, T. H., Yi, S. W., Kim, B. S., Kim, W. I., Shin, G. W. (2017). Identification and antibiotic resistance profiling of bacterial isolates from septicaemic soft-shelled turtles (Pelodiscus sinensis). Veterinární medicína 62 (3), 169–177. doi: 10.17221/65/2016-VETMED
Cummings, J. H., Macfarlane, G. T. (1997). Role of intestinal bacteria in nutrient metabolism. J. Parenteral Enteral Nutr. 21 (6), 357–365. doi: 10.1177/0148607197021006357
da Silva, B. C., Jatobá, A., Schleder, D. D., Vieira, F. D. N., Mouriño, J. L. P., Seiffert, W. Q. (2016). Dietary supplementation with butyrate and polyhydroxybutyrate on the performance of pacific white shrimp in biofloc systems. J. World Aquacult. Soc. 47 (4), 508–518. doi: 10.1111/jwas.12284
Dawood, M. A., Eweedah, N. M., Elbialy, Z. I., Abdelhamid, A. I. (2020). Dietary sodium butyrate ameliorated the blood stress biomarkers, heat shock proteins, and immune response of Nile tilapia (Oreochromis niloticus) exposed to heat stress. J. thermal Biol. 88, 102500. doi: 10.1016/j.jtherbio.2019.102500
Dawood, M. A., Noreldin, A. E., Ali, M. A., Sewilam, H. (2021). Menthol essential oil is a practical choice for intensifying the production of Nile tilapia (Oreochromis niloticus): Effects on the growth and health performances. Aquaculture 543, 737027. doi: 10.1016/j.aquaculture.2021.737027
Dibner, J. J., Buttin, P. (2002). Use of organic acids as a model to study the impact of gut microflora on nutrition and metabolism. J. Appl. poultry Res. 11 (4), 453–463. doi: 10.1093/japr/11.4.453
Du, Y., Cheng, L., Zhao, J., de Cruz, C. R., Xu, H., Wang, L., et al. (2023). Effects of clostridium butyricum and sodium butyrate on growth performance, immunity, and gut microbiota of mirror carp cyprinus carpio fed with soybean meal based diet. Aquacult. Rep. 29, 101501. doi: 10.1016/j.aqrep.2023.101501
El-Sayed Ali, T., El-Sayed, A. M., Eissa, M. A. R., Hanafi, H. M. (2018). Effects of dietary Biogen and sodium butyrate on hematological parameters, immune response, and histological characteristics of Nile tilapia (Oreochromis niloticus) fingerlings. Aquacult. Int. 26, 139–150. doi: 10.1007/s10499-017-0205-3
Han, F., Xu, C., Qi, C., Lin, Z., Li, E., Wang, C., et al. (2020). Sodium butyrate can improve intestinal integrity and immunity in juvenile Chinese mitten crab (Eriocheir sinensis) fed glycinin. Fish shellfish Immunol. 102, 400–411. doi: 10.1016/j.fsi.2020.04.058
Huang, K., Bao, J. (2023). Progress on nutritional composition and efficacy ofChinese soft-shelled turtle. Acta Agriculturae Shanghai 39 (1), 145–152. doi: 10.15955/j.issn1000-3924.2023.01.23
Jesus, G. F., Pereira, S. A., Owatari, M. S., Syracuse, N., Silva, B. C., Silva, A., et al. (2019). Protected forms of sodium butyrate improve the growth and health of Nile tilapia fingerlings during sexual reversion. Aquaculture 499, 119–127. doi: 10.1016/j.aquaculture.2018.09.027
Kan, H., Zhao, F., Zhang, X. X., Ren, H., Gao, S. (2015). Correlations of gut microbial community shift with hepatic damage and growth inhibition of Carassius auratus induced by pentachlorophenol exposure. Environ. Sci. Technol. 49 (19), 11894–11902. doi: 10.1021/acs.est.5b02990
Khalil, M., Azmat, H., Khan, N., Javid, A., Hussain, A., Hussain, S. M., et al. (2018). Growth responses of striped catfish pangasianodon hypophthalmus (Sauvage 1878) to exogenous enzyme added feed. Pakistan J. Zool. 50 (2), 685–693. doi: 10.17582/journal.pjz/2018.50.2.685.693
Kou, H., Hu, J., Wang, A. L., Pan, X., Miao, Y., Lin, L. (2021). Impacts of dietary zinc on growth performance, haematological indicators, transaminase activity and tissue trace mineral contents of soft-shelled turtle (Pelodiscus sinensis). Aquacult. Nutr. 27 (6), 2182–2194. doi: 10.1111/anu.13353
Mehrgan, M. S., Shekarabi, S. P. H., Azari, A., Yilmaz, S., Lückstädt, C., Rajabi Islami, H. (2022). Synergistic effects of sodium butyrate and sodium propionate on the growth performance, blood biochemistry, immunity, and immune-related gene expression of goldfish (Carassius auratus). Aquacult. Int. 30 (6), 3179–3193. doi: 10.1007/s10499-022-00954-z
Mitranescu, E., Alexandru, A., Galis, A. M., Lataretu, A., Mitranescu, D., Tudor, L. (2013). Research concerning the quality of life and the blood biochemical parameters as indicators for fish welfare evaluation. Curr. Opin. Biotechnol. 24, S90. doi: 10.1016/j.copbio.2013.05.268
Ng, W. K., Koh, C. B. (2017). The utilization and mode of action of organic acids in the feeds of cultured aquatic animals. Rev. Aquacult. 9 (4), 342–368. doi: 10.1111/raq.12141
Porter, N. T., Luis, A. S., Martens, E. C. (2018). Bacteroides thetaiotaomicron. Trends Microbiol. 26 (11), 966–967. doi: 10.1016/j.tim.2018.08.005
Porter, N. T., Martens, E. C. (2017). The critical roles of polysaccharides in gut microbial ecology and physiology. Annu. Rev. Microbiol. 71, 349–369. doi: 10.1146/annurev-micro-102215-095316
Ricke, S. C. (2003). Perspectives on the use of organic acids and short chain fatty acids as antimicrobials. Poultry Sci. 82 (4), 632–639. doi: 10.1093/ps/82.4.632
Sotoudeh, E., Esmaeili, N. (2022). Effects of Biotronic® Top3, a feed additive containing organic acids, cinnamaldehyde and a permeabilizing complex on growth, digestive enzyme activities, immunity, antioxidant system and gene expression of barramundi (Lates calcarifer). Aquacult. Rep. 24, 101152. doi: 10.1016/j.aqrep.2022.101152
Tang, Y., Zhao, L., Cheng, Y., Yang, Y., Sun, Y., Liu, Q. (2021). Control of cyanobacterial blooms in different polyculture patterns of filter feeders and effects of these patterns on water quality and microbial community in aquacultural ponds. Aquaculture 542, 736913. doi: 10.1016/j.aquaculture.2021.736913
Tian, L., Zhou, X. Q., Jiang, W. D., Liu, Y., Wu, P., Jiang, J., et al. (2017). Sodium butyrate improved intestinal immune function associated with NF-κB and p38MAPK signalling pathways in young grass carp (Ctenopharyngodon idella). Fish Shellfish Immunol. 66, 548–563. doi: 10.1016/j.fsi.2017.05.049
Tran, N. T., Li, Z., Wang, S., Zheng, H., Aweya, J. J., Wen, X., et al. (2020). Progress and perspectives of short-chain fatty acids in aquaculture. Rev. Aquacult. 12 (1), 283–298. doi: 10.1111/raq.12317
Ullah, S., Zhang, G., Zhang, J., Shengli, T., Wang, L., Kalhoro, H., et al. (2020). Effects of microencapsulated sodium butyrate supplementation on growth performance, intestinal development and antioxidative capacity of juvenile black sea bream (Acanthopagrus schlegelii). Aquacult. Res. 51 (12), 4893–4904. doi: 10.1111/are.14824
Uribe, C., Folch, H., Enríquez, R., Moran, G. (2011). Innate and adaptive immunity in teleost fish: a review. Veterinarni medicina 56 (10), 486–503. doi: 10.17221/3294-VETMED
Wu, P., Tian, L. I., Zhou, X. Q., Jiang, W. D., Liu, Y., Jiang, J., et al. (2018). Sodium butyrate enhanced physical barrier function referring to Nrf2, JNK and MLCK signaling pathways in the intestine of young grass carp (Ctenopharyngodon idella). Fish Shellfish Immunol. 73, 121–132. doi: 10.1016/j.fsi.2017.12.009
Yamamoto, F. Y., Older, C. E., Hume, M. E., Hoffmann, A. R., Gatlin, D. M. (2021). Effects of butyrate, propionate, and their combination in vitro, and the impacts of their supplementation in high-plant-protein diets to the production performance, innate immune responses, and intestinal microbiota of red drum (Sciaenops ocellatus). Aquaculture 545, 737225. doi: 10.1016/j.aquaculture.2021.737225
Yarahmadi, P., Miandare, H. K., Hoseinifar, S. H., Gheysvandi, N., Akbarzadeh, A. (2015). The effects of stocking density on hemato-immunological and serum biochemical parameters of rainbow trout (Oncorhynchus mykiss). Aquacult. Int. 23, 55–63. doi: 10.1007/s10499-014-9797-z
Yarahmadi, P., Mirghaed, A. T., Shekarabi, S. P. H. (2022). Zootechnical performance, immune response, and resistance to hypoxia stress and Vibrio harveyi infection in Pacific white shrimp (Litopeneaus vannamei) fed different fishmeal diets with and without addition of sodium butyrate. Aquacult. Rep. 26, 101319. doi: 10.1016/j.aqrep.2022.101319
Zhao, H., Peng, K., Wang, G., Mo, W., Huang, Y., Cao, J. (2021). Metabolic changes, antioxidant status, immune response and resistance to ammonia stress in juvenile yellow catfish (Pelteobagrus fulvidraco) fed diet supplemented with sodium butyrate. Aquaculture 536, 736441. doi: 10.1016/j.aquaculture.2021.736441
Zhou, C., Lin, H., Huang, Z., Wang, J., Wang, Y., Wei, Y. (2019). Effect of dietary sodium butyrate on growth performance, enzyme activities and intestinal proliferation-related gene expression of juvenile golden pompano Trachinotus ovatus. Aquacult. Nutr. 25 (6), 1261–1271. doi: 10.1111/anu.12940
Zong, Q., Qu, H., Zhao, Y., Liu, H., Wu, S., Wang, S., et al. (2022). Sodium butyrate alleviates deoxynivalenol-induced hepatic cholesterol metabolic dysfunction via RORγ-mediated histone acetylation modification in weaning piglets. J. Anim. Sci. Biotechnol. 13 (1), 1–14. doi: 10.1186/s40104-022-00793-1
Keywords: aquatic feed, sodium butyrate, Pelodiscus sinensis, immunity, intestinal microbe
Citation: Ge L, Yu Y, Wen X, Xiao H, Liu K, Liu Z, Liu S, Li Q, Wang X, Deng Z and Hu Y (2023) Effects of dietary sodium butyrate on growth performance, immune function, and intestinal microflora of Chinese soft-shelled turtle (Pelodiscus sinensis). Front. Cell. Infect. Microbiol. 13:1271912. doi: 10.3389/fcimb.2023.1271912
Received: 03 August 2023; Accepted: 20 September 2023;
Published: 11 October 2023.
Edited by:
Jing Gao, Chinese Academy of Sciences (CAS), ChinaReviewed by:
Fan Yu, Chinese Academy of Fishery Sciences (CAFS), ChinaCopyright © 2023 Ge, Yu, Wen, Xiao, Liu, Liu, Liu, Li, Wang, Deng and Hu. This is an open-access article distributed under the terms of the Creative Commons Attribution License (CC BY). The use, distribution or reproduction in other forums is permitted, provided the original author(s) and the copyright owner(s) are credited and that the original publication in this journal is cited, in accordance with accepted academic practice. No use, distribution or reproduction is permitted which does not comply with these terms.
*Correspondence: Yazhou Hu, aHV5YXpob3VAaHVuYXUuZWR1LmNu; Zaofu Deng, emFvZnVkZW5nQHllYWgubmV0; Xiaoqing Wang, d2FuZ3hpYW84MjU4QDEyNi5jb20=
†These authors share first authorship
Disclaimer: All claims expressed in this article are solely those of the authors and do not necessarily represent those of their affiliated organizations, or those of the publisher, the editors and the reviewers. Any product that may be evaluated in this article or claim that may be made by its manufacturer is not guaranteed or endorsed by the publisher.
Research integrity at Frontiers
Learn more about the work of our research integrity team to safeguard the quality of each article we publish.