- 1Molecular Parasitology Unit, Institute of Tropical Medicine Antwerp, Antwerp, Belgium
- 2Instituto de Medicina Tropical “Alexander von Humboldt”, Universidad Peruana Cayetano Heredia, Lima, Peru
- 3Department of Microbiology, Immunology and Transplantation, Rega Institute for Medical Research, Katholieke Universiteit Leuven, Leuven, Belgium
Introduction: Resistance against anti-Leishmania drugs (DR) has been studied for years, giving important insights into long-term adaptations of these parasites to drugs, through genetic modifications. However, microorganisms can also survive lethal drug exposure by entering into temporary quiescence, a phenomenon called drug tolerance (DT), which is rather unexplored in Leishmania.
Methods: We studied a panel of nine Leishmania braziliensis strains highly susceptible to potassium antimonyl tartrate (PAT), exposed promastigotes to lethal PAT pressure, and compared several cellular and molecular parameters distinguishing DT from DR.
Results and discussion: We demonstrated in vitro that a variable proportion of cells remained viable, showing all the criteria of DT and not of DR: i) signatures of quiescence, under drug pressure: reduced proliferation and significant decrease of rDNA transcription; ii) reversibility of the phenotype: return to low IC50 after removal of drug pressure; and iii) absence of significant genetic differences between exposed and unexposed lineages of each strain and absence of reported markers of DR. We found different levels of quiescence and DT among the different L. braziliensis strains. We provide here a new in-vitro model of drug-induced quiescence and DT in Leishmania. Research should be extended in vivo, but the current model could be further exploited to support R&D, for instance, to guide the screening of compounds to overcome the quiescence resilience of the parasite, thereby improving the therapy of leishmaniasis.
1 Introduction
Chemotherapy is essential not only for the clinical management of infectious diseases but also for their control and elimination, especially if humans constitute the reservoir of respective pathogens (anthroponoses) and drugs can counter human- to-human transmission (WHO, n.d). Accordingly, treatment failure (TF) may have a major public health impact. TF is a clinical phenotype that can be expressed in different forms, like non-response, relapse, or recrudescence. It has a complex and multifactorial origin, essentially involving the drug (quality, compliance, dosage), the host (immune status, co-infections and concomitant morbidities, existence of sanctuary organs/cells), and the pathogen’s biology (Barrett et al., 2019). From the microbial point of view, drug resistance (DR) is the usual suspect and culprit. Hence, DR is often and wrongly confounded with TF, while —in contrast with TF— drug resistance is a long-term parasite adaptive phenotype, more specifically a decreased drug susceptibility which is acquired through genetic modification of the microbe. As such, DR is heritable and the phenotypic adaptation can persist even in the absence of drug exposure (Balaban et al., 2019) until a mutation reverting or compensating the phenotype emerges and is selected.
However, other mechanisms can cause a reduction of drug susceptibility in pathogens. DT is one of them and it is intrinsically totally different from DR. Indeed, it refers to a short-term adaptive phenotype, consisting of decreased susceptibility of the pathogen to the drug, which is not due to an acquired genetic modification of the parasite. It is often associated with quiescence (syn. dormancy), a physiological state of the cell triggered by environmental insults and involving a reversible cell division arrest driven by a dynamic and regulated cell and metabolic remodeling program (Balaban et al., 2019). In microbiology, there are several examples of pathogens that enter in a transient quiescent state, in which they are refractory to one or more drugs, allowing them to persist in the host for long periods: Mycobacterium tuberculosis/streptomycin, isoniazid, ciprofloxacin, and rifampin (Keren et al., 2011); Staphylococcus aureus/aminoglycoside (Michiels et al., 2016); yeasts (Bojsen et al., 2017); and Plasmodium falciparum/artemisinin (Teuscher et al., 2012), among many others.
Leishmania are parasitic protozoa causing a spectrum of clinical forms in (sub-)tropical regions but also in Southern Europe. Leishmaniasis belongs to the category of most neglected diseases, which is reflected among others by the small chemotherapeutic arsenal to combat them. Failure of the few available drugs jeopardizes elimination programs, and some studies associated the TF of leishmaniasis with DR (Lira et al., 1999). However, in two epidemiological settings (Peru/L. braziliensis and Nepal/L. donovani), we documented a poor correlation between resistance to antimonials (as measured by in-vitro susceptibility assays) and TF (Yardley et al., 2006; Rijal et al., 2007). This motivated us to look for alternative mechanisms leading to reduced drug susceptibility in the parasite, more specifically, quiescence. Research on quiescence in Leishmania is still in its infancy. Quiescence was reported to occur in vitro and in vivo, and it is characterized by a reduced level of translational machinery (ribosomal RNA and proteins), low rates of RNA synthesis, protein turnover, and membrane lipid synthesis (Kloehn et al., 2015). Last but not least, parasite populations are found to be heterogeneous in terms of activity, with deeply and semi-quiescent subpopulations (Mandell and Beverley, 2017).
There are only two studies on Leishmania quiescence in the context of drug exposure. First, in vivo, following treatment with miltefosine, quiescent cells of L. mexicana were encountered in mesothelium-like tissues surrounding granulomas, in which the drug did not accumulate (Kloehn et al., 2021). Secondly, in vitro, we showed that a strain of L. lainsoni entered quiescence under a stationary phase or antimony pressure. Several transcriptional signatures were shared by the parasites surviving both environmental insults: among these, a series of transcripts were present in higher abundance, in a general background of transcriptional shift (Jara et al., 2022). These molecular similarities validated the quiescent character of Leishmania under antimony pressure. The objectives of the present study were to further explore in vitro the link between quiescence and DT in Leishmania and to address the diversity of the DT phenotype in genetically different strains of the same species. Therefore, we exposed nine strains of L. braziliensis to high doses of trivalent antimonials and compared several parameters distinguishing DT from DR in pre- and post-exposure lineages (inhibitory concentration, proliferation, cell viability, metabolic activity, lethal dose, and genome).
2 Materials and methods
2.1 Parasites
Clinical isolates were collected in Peru at the Institute of Tropical Medicine Alexander von Humboldt between 2001 and 2004 within the framework of the multiregional LeishNatDrug project (Yardley et al., 2006). Nine of them were selected for the present study, according to the following inclusion criteria: i) first episode of leishmaniasis, ii) cutaneous form of the disease, iii) no previous treatment before attending the clinic, iv) complete therapy with antimonial post-diagnosis, v) no concomitant disease, and vi) infecting species typed as Leishmania braziliensis. One strain of Leishmania lainsoni was included in the study as control: this is a line highly susceptible to antimonials, for which the existence of quiescence after PAT exposure was demonstrated (Jara et al., 2022). For each isolate, enhanced green fluorescent protein (EGFP) was integrated within the 18S ribosomal DNA locus, further called rEGFP with the use of the pLEXSY-hyg system (Jena Bioscience, Jena, Germany) as previously reported elsewhere (Bolhassani et al., 2011; Jara et al., 2022). After the generation of the transgenic rEGFP parasites, clones were obtained with the “micro-drop” method, as described elsewhere (Van Meirvenne et al., 1975; Jara et al., 2022). All the work presented here was made with these clones, further called rEGFP strains.
2.2 Cell culture of promastigotes and generation of axenic amastigotes
Promastigotes were maintained in complete M199 (M199 medium at pH 7.2 supplemented with 20% fetal bovine serum, hemin 5 mg/L, 50 μg/mL of hygromycin Gold, 100 units/mL of penicillin, and 100 μg/mL of streptomycin) at 26°C with passages done twice per week. To obtain axenic amastigotes, 1 mL of stationary promastigotes was centrifuged (1, 500×g, 5 min), the pellet was resuspended in 5 mL of complete MAA (M199 at pH 5.5, supplemented with 20% fetal bovine serum, glucose 2.5 g/L, 5 g of tryptic soy broth, hemin 5 mg/L, 25 μg/mL of hygromycin Gold, 100 units/mL of penicillin, and 100 μg/mL of streptomycin), and parasites were incubated at 34°C. After observing morphological evidence of amastigogenesis on day 3, the lines were subcultured every 4 days, and growth was monitored microscopically over 3 weeks. If axenic amastigotes were not able to proliferate, three subsequent attempts for axenization were performed: the generation of axenic amastigotes was successful for three rEGFP strains (PER094, 122, and 362). Samples for further experimental procedures were prepared in complete M199 or MAA in the absence of hygromycin. The growth curves were monitored by daily counting of parasites, and the generation time was calculated with the following formula: G = t/(logb − logB)/log2), where t = time interval in hours, B = number of parasites at the beginning of a time interval, and b = number of parasites at the end of the time interval.
2.3 Cell recovery and monitoring of survival after drug pressure
Exponentially growing promastigotes were exposed to 9 µg/mL of potassium antimonyl tartrate (PAT) for 48 h after which an aliquot of 50 µL was sub cultured in a medium without drug pressure (post-PAT). For each strain, a control without exposure to drug pressure and instead treated with PBS was maintained in parallel (post-PBS). The cell cultures were monitored microscopically, and if positive cellular growth was observed, additional subcultures for the evaluation of drug susceptibility and pellets for sequencing were prepared. Throughout the paper, lineages that were exposed to PAT were referred to as “post-PAT,” while those that were not previously exposed to PAT were referred to as “post-PBS.”
2.4 Drug susceptibility and estimation of the IC50
The drug susceptibility was measured with the resazurin test. Briefly, exponentially growing parasites were plated into 96-well plates containing a serial dilution of PAT to reach final concentrations ranging from 83 µg/mL to 0.1 µg/mL. Each plate included controls without PAT and controls for monitoring proliferation and autofluorescence of the medium. Resazurin sodium salt (200 µg/mL, Sigma, Darmstadt, Germany) was added at two different time points: at the time of plating for the proliferation controls (T1) and 20 h post- drug pressure for the other wells (T2). After 4 h of incubation with resazurin, the fluorescence of its reduced form resorufin was recorded using the Victor X3 Multilabel Reader (Perkin Elmer, Waltham, USA) exciting at 560 nm and measuring emission at 590 nm. The test was considered valid for further analysis only if the fluorescence for the controls without drug pressure at T2 was at least 2- fold the fluorescence for the proliferation controls at T1. All experiments with the different lines were repeated three times with three technical replicates each. The blank-subtracted data expressed in relative fluorescence units (RFU) were exported to GraphPad Prism 8 to calculate the 50% inhibitory concentration (IC50), using a sigmoidal dose–response model with variable slope. Statistical analysis and data visualization were performed in R Studio with built-in functions and ggplot2, respectively.
2.5 Flow cytometry assay for monitoring single-cell viability and quiescence
Exponentially proliferating cells (promastigotes and amastigotes) were exposed to PAT at concentrations ranging from 1 µg/mL to 83 µg/mL, and both the cell viability and the rEGFP expression were measured by flow cytometry at three time points: before drug exposure and after drug pressure at 24 h and 48 h. The rEGFP expression is a negative marker of quiescence: highly expressed in proliferative cells and downregulated during quiescence (Jara et al., 2019; Jara et al., 2022). The cell viability was evaluated by preincubating cells with the NucRed Dead 647 (Thermo Fisher Scientific, Waltham, USA) for the staining of dead cells and Vybrant Dye Cycle violet (Thermo Fisher Scientific) for the staining of DNA in all cells. The Vybrant Dye Cycle violet was used to select the subpopulation of cells having a healthy pattern for their DNA. A wild-type (non-rEGFP) strain and a non-stained sample were included as negative controls, together with a sample exposed to thermal shock as a positive control for cellular death. The samples were analyzed with a calibrated flow cytometer BD FACS Verse ™ in the medium flow rate mode. In order to compare the rEGFP relative fluorescence units (RFU) among the samples, the acquisitions were made with the same settings during all the experiments. The FCS files were analyzed with the FCS 5 Express Plus Research edition. The subpopulation of cells with good cell viability was selected by sequential gating. Briefly, single cells were selected by pulse geometry gate; a first gate was selected by plotting the SSC-W vs. SSC-H, and a second gate was created by plotting the FCS-H and FCS-A. Among the single cells, a third gate was created by plotting the rEGFP vs. the NucRed and selecting the subpopulation NucRed-negative. Finally, a fourth gate was created by plotting the rEGFP vs. the Vybrant Dye Cycle violet and selecting the subpopulation having a healthy DNA pattern. The cell viability for each sample was estimated by multiplying the percentage of cells NucRed-negative and Vybrant DyeCycle-positive. For each developmental stage of Leishmania and for each time point post-exposure to PAT, the effect of the drug pressure over the rEGFP expression in the subpopulation of viable cells was evaluated with a two-way ANOVA considering the PAT drug pressure and strains as explanatory variables. Subsequently, a Tukey’s HSD test was used to evaluate the statistical significance of differences between pairwise comparisons.
2.6 Estimating the lethal doses 10, 20, and 50
The measures of cell viability obtained after the flow cytometry assay over increasing concentrations of PAT were used to estimate the lethal doses of PAT that kill 10%, 25%, and 50% of the population (LD10–LD50). The LDs were estimated using the drc R package (Ritz et al., 2015). Briefly, the data were fitted using a log-logistic model with a lower limit at 0 and having as a formula the % of cell viability as explained by the concentration of PAT and the line of the parasite as the grouping factor. Finally, the desired LDs were estimated having as a reference the upper limit and setting the confidence interval to 0.95.
2.7 Whole genome sequencing
For each lineage (post-PBS and post-PAT), DNA was isolated from a sample maintained without drug pressure, and a sample recovered after 48 h of PAT drug pressure, using the QIAamp DNA Micro Kit (Qiagen, Hilden, Germany). The DNA concentration was assessed with the Qubit DNA broad-range DNA quantification kit (Thermo Fisher). Library preparation and sequencing were performed at BGI with a DNBSEQ-WGS-PCR free library index and the DNBSEQ PE150 platform, respectively. The FASTQ files containing paired reads of 150 bp were subsequently analyzed for the assessment of sequence variants and ploidy. Briefly, the quality of the reads was evaluated with SAMtools and the multiqc command. Samples were aligned against the reference genome of L. braziliensis MHOM/BR/75/M2904 (Van den Broeck et al., 2020). The per position mean depth and the breadth of coverage were estimated with SAMtools. Variants (SNPs and INDELs) were called jointly for all 18 samples with BCFtools and the mpileup command (Danecek et al., 2021). Subsequently, the multisample vcf file was filtered according to the following criteria: overall variant quality (QUAL) higher than 200, the individual read depth per locus (FMT/DP) higher than 5, and the average mapping quality (MQ) and genotype quality (GQ) higher than 40. The annotation of the genomic location for the variants was done with SnpEff (Cingolani et al., 2012). Somy values were estimated by using the median read depth across each chromosome as reported elsewhere (Dumetz et al., 2017). A maximum likelihood phylogenetic tree was reconstructed with IQ-TREE v1.6.12 (Nguyen et al., 2015) reusing the HKY+G substitution model and 1,000 ultrafast bootstrap approximation (Hoang et al., 2018).
3 Results
3.1 Leishmania exposure to transient PAT drug pressure causes a reversible decrease of proliferation and does not select for parasites with lower drug susceptibility
For each of the nine rEGFP strains of L. braziliensis used, we measured the drug susceptibility of promastigotes of the corresponding post-PBS lineages with a resazurin test. Values ranged from 0.2 µg/mL to 7.48 µg/mL, with a median of 1.98 µg/mL (Figure 1A and Supplementary Figure 1). We then exposed all lines to the same concentration of PAT (9 µg/mL, representing ~ 4-fold the median IC50 of all nine strains) in order to evaluate the potential differences in their adaptation to survive. i) The potential cytostatic effect of PAT on Leishmania cells was monitored with the resazurin test by measuring the signal of its reduced form resorufin before the drug treatment and after 24 h of exposure to PAT or PBS. In the absence of the drug, the resorufin log2 FC signal ranged from 0.8 (PER206 EGFP Cl2) to ~ 3.2 relative fluorescence units (RFU, equivalent to 9- fold the initial signal, in PER122 EGFP Cl1), indicating that all strains were proliferating albeit at different rates. Under drug pressure, the resorufin log2 FC signal was significantly decreased compared with the one measured in the corresponding control without the drug in all strains suggesting that under our conditions of drug pressure, parasites have very diminished growth (Figure 1B). ii) After 48 h of exposure to 9 µg/mL of PAT, parasite death was observed in each lineage, but parasites were all able to resume their proliferation after subculturing in a fresh medium without a drug. This indicated that at least a proportion of the cells survived and maintained their capability to switch to a proliferative state once they were in optimal conditions. iii) We found that the susceptibility to the drug did not change in the post-PAT lineages (median IC50 = 2.35 µg/mL; interquartile range, IQR = 2.54) when compared with the corresponding post-PBS lineages (median IC50 = 2.00 µg/mL, IQR = 2.70, Mann–Whitney U test, P = 0.9, Figure 1A). Noteworthy, the capacity of Leishmania to survive concentrations of the drug beyond the IC50 of 9 µg/mL represents ~ 45 times the IC50 for the most susceptible line PER348 EGFP Cl1 (IC50 = 0.2 µg/mL).
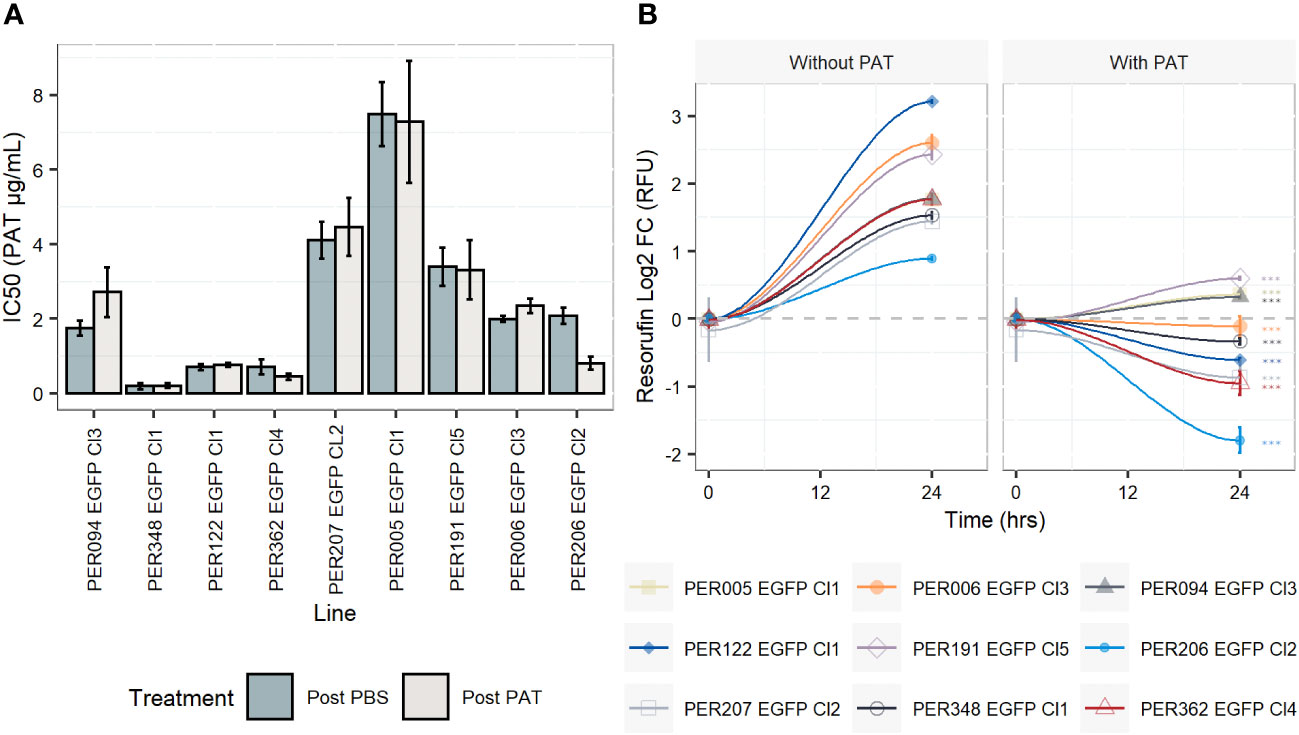
Figure 1 Drug susceptibility of Leishmania braziliensis promastigotes and their growth features under drug pressure. (A) Drug susceptibility to potassium antimonyl tartrate (PAT) in nine strains as estimated by the resazurin test after 24 h of drug pressure. For each strain, the IC50 was calculated in a lineage without prior exposure to the drug (post-PBS) and after the exposure to 9 µg/mL of PAT (~ 4 -fold the median IC50 considering all lineages, post-PAT). The results represent the mean ± SEM of three biological replicates. (B) Evaluation of the cytostatic effect of PAT at 9 µg/mL. The gray dashed line represents no change in the original resorufin signal compared with the day the cells were initially plated. A log2 FC above 1 indicates parasite duplication and a log2 FC below 0 indicates parasite arrest and/or cellular death. The asterisks represent statistically significant differences after Tukey’s post-hoc test (P < 0.001) between cells after 24 h of plating without drug compared with cells under drug pressure.
3.2 Leishmania exposure to transient PAT drug pressure is accompanied by a decrease in the expression of rEGFP among viable cells
The expression of rEGFP is a negative biomarker of quiescence: it is high in proliferative cells, while it decreases or disappears in quiescent cells (Jara et al., 2022). i) In promastigotes, the exposure of proliferating cells to PAT (9.0 µg/mL) led to a significant reduction in the levels of rEGFP expression (RFU) among viable cells of each line when compared with the control treated with PBS at both 24 h and 48 h (Figure 2A). Furthermore, rEGFP expression was also different among the evaluated strains (two-way ANOVA: P < 0.001 for PAT treatment and P < 0.001 for strain). The same significant effect of the drug was observed in amastigotes. ii) We then explored if the reduction in rEGFP expression changes in response to increasing concentrations of PAT. The results showed that higher doses of PAT induced a more profound reduction of the rEGFP expression compared with the control without PAT treatment at both 24 h and 48 h post-PAT treatment in both promastigotes and amastigotes (Figure 2B). The reduction in rEGFP expression follows a dose–response model where there is a linear response that reaches a plateau approximately 9.0 µg/mL of PAT with an average relative rEGFP reduction of 44% and 40% after 48 h of PAT in promastigotes and amastigotes, respectively. iii) The analysis of relative rEGFP reduction also shows differences among L. braziliensis strains (ranging between 23.7% and 56.2% in promastigotes and between 33.7% and 49.9% in amastigotes).
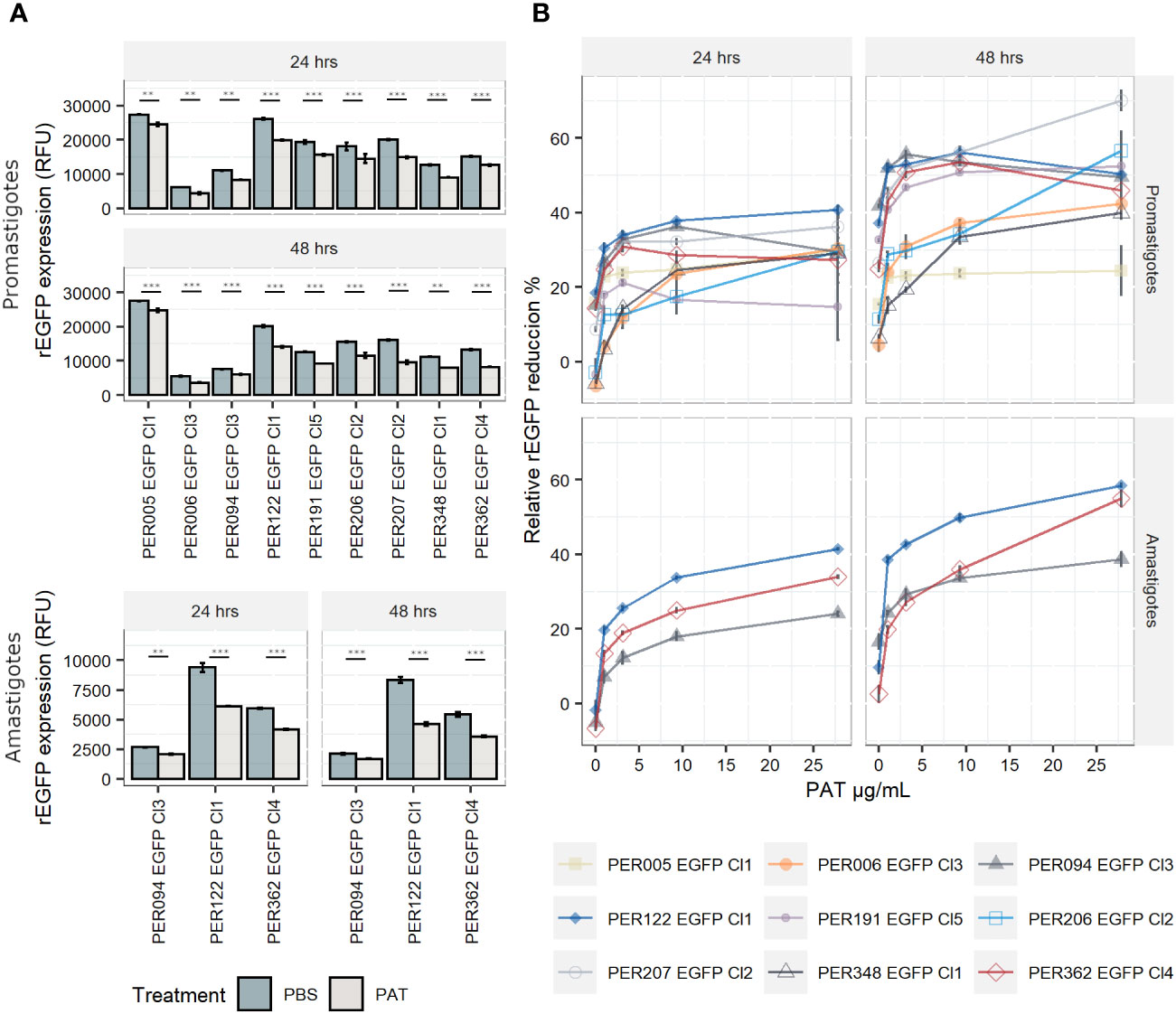
Figure 2 rEGFP expression in promastigotes (nine strains) and amastigotes (three strains) of Leishmania braziliensis under drug pressure; measures are made on viable cells. (A) rEGFP expression of L. braziliensis lineages after 24 and 48 h of exposure to 9 µg/mL of PAT. The results represent the mean ± SEM of three biological replicates. The asterisks represent statistically significant differences after Tukey’s post-hoc test; ** P < 0.01, *** P < 0.001. (B) Relative rEGFP reduction (in %) in relation to increasing concentrations of PAT after 24 and 48 h of PAT exposure.
3.3 Survival of Leishmania to high lethal doses of PAT is accompanied by a decrease in rEGFP expression
When measuring the drug susceptibility with standard fluorometric procedures such as the resazurin test at a single point in time, it is not possible to address with certainty if the drug has cytostatic or cytotoxic effects. The decreased signal of resorufin in samples with the drug pressure compared with the control could result from cellular death, arrested growth, or likely, a mixture of both events. Therefore, we evaluated the cell viability at the single-cell level by flow cytometry and calculated the lethal doses 10, 25, and 50 (LD10, LD25, and LD50), which are the concentrations of the drug that kill 10%, 25%, and 50% of the population, respectively. i) In promastigotes, the kinetics of the cell viability over different concentrations of PAT pressure suggests variable cytotoxic effects among the evaluated lines. The LD50 among all strains ranged from 5.98 µg/mL in PER206 EGFP Cl2 to 83.3 µg/mL in PER006 EGFP Cl3 (Figures 3A, B). The median LD50 among the nine strains was 28.2 µg/mL, which is approximately 10- fold the IC50 that was previously measured with the resazurin test. Noteworthy, four out of the nine lines had LD50 higher than 30 µg/mL of PAT, a non-physiological concentration. These strains may be considered highly tolerant to PAT compared with the other strains at the same parasite stage. ii) As promastigotes showed high variability in their rates of survival to increasing concentrations of PAT, we further evaluated the potential relationship between the capability to adopt a quiescent state as measured by the reduction in rEGFP expression and the success to overcome the drug pressure as measured by their cell viability. After 24 h of PAT treatment, there was a weak positive relationship at low concentrations of PAT (1 µg/mL to 3 µg/mL of PAT) that became strong at 9 µg/mL and 28 µg/mL of PAT (Figure 3C). iii) Amastigotes showed remarkably higher survival rates to PAT compared with the promastigotes of the respective strains. After twice the time of exposure to PAT (48 h), the highest concentration of the drug could not kill 50% of the population, and the estimation of the median LD25 for the three strains was 46.7 µg/mL (Figures 3D, E). Because these results with amastigotes of L. braziliensis were surprisingly high, we evaluated the LDs from a highly susceptible line of L. lainsoni. The same experimental setup estimated that in amastigotes, the LD25 and LD50 were 0.49 µg/mL and 0.97 µg/mL, respectively, ruling out that the L. braziliensis results would be an experimental artifact (Supplementary Figure 2). Accordingly, amastigotes of L. braziliensis are intrinsically more tolerant to PAT pressure compared with promastigotes. Further work is required to know if this could be due to a deeper quiescence state in amastigotes.
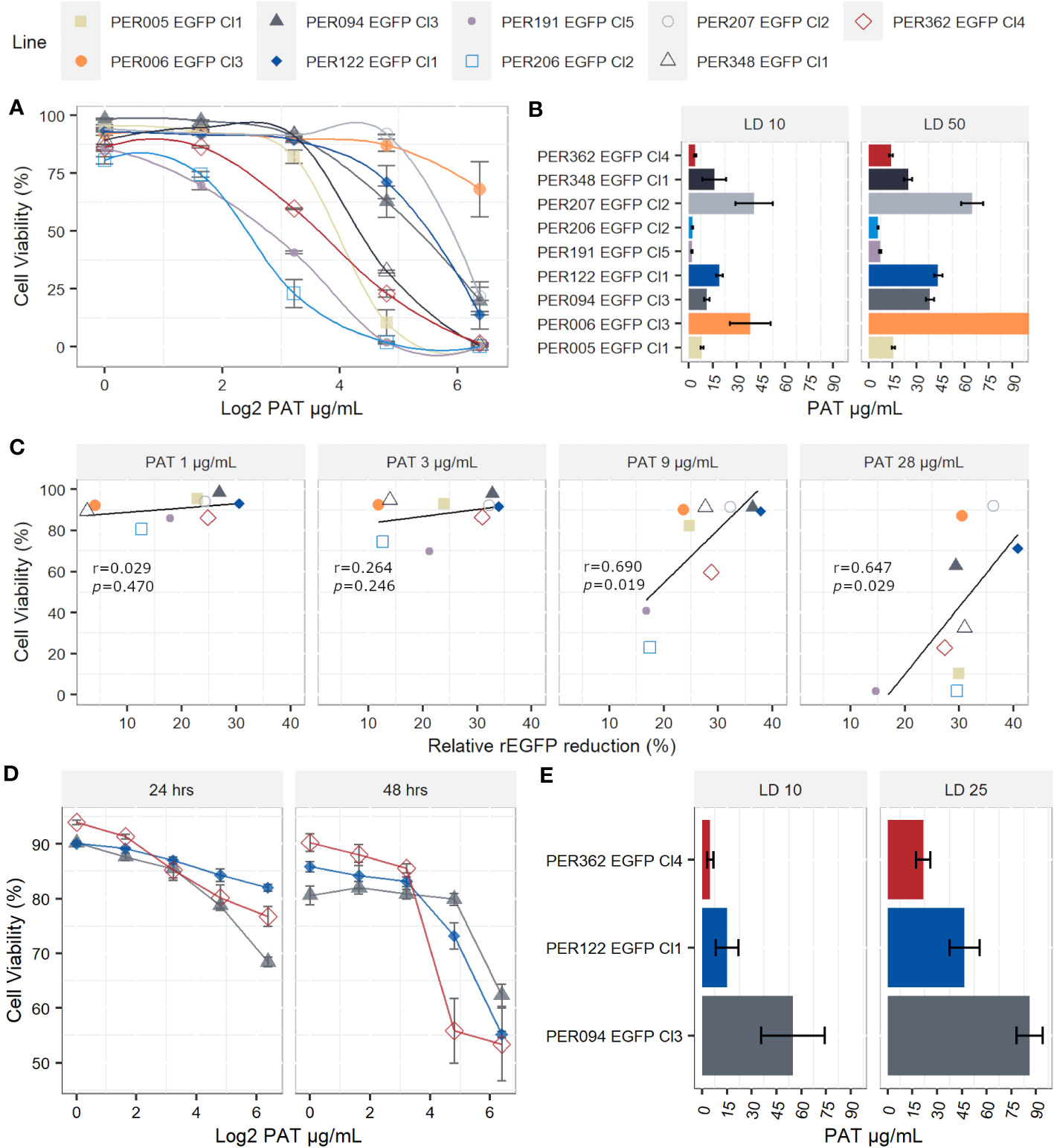
Figure 3 Lethal doses (LD) on Leishmania braziliensis promastigotes and amastigotes. (A) Viability of individual promastigotes after 24 h of exposure to increasing PAT concentration. (B) Lethal doses 10 and 50 on promastigotes. The results represent the mean ± SEM of three biological replicates. (C) The relationship between viability of promastigotes and reduction of rEGFP expression at different PAT concentrations. The Pearson’s correlation coefficients and P-values are shown. (D) Viability of individual amastigotes after 24 and 48 h of exposure to increasing PAT concentration. (E) Lethal doses 10 and 25 on amastigotes, calculated after 48 h of PAT exposure. The results represent the mean ± SEM of three biological replicates.
3.4 Leishmania lines exposed to PAT are genomically similar to their respective parental lines
We evaluated the genome stability between pre- and post-PAT lines by WGS, at the levels of somy, SNPs, and INDELs. i) At the somy level, we observed variability of karyotypes in a strain-specific manner. The number of aneuploid chromosomes/strain ranged from 1 to 10. Trisomy of chromosomes 5, 11, 25, and 29 and tetrasomy of chromosome 31 were among the most frequent occurrences being present in at least three out of the nine strains. For each strain, the original overall ploidy of the post-PAT lineage was identical to the corresponding post-PBS lineage (Figure 4A). ii) Compared with the L. braziliensis reference genome (MHOM/BR/75/M2904), a total of 163,823 high-quality SNPs and 17,055 high-quality INDELs were called across our panel of 18 lineages. A maximum likelihood phylogenetic tree based on SNPs shows that each post-PAT lineage clusters with its corresponding control, post-PBS lineage (Figure 4B), reflecting the high genomic similarity between post-PAT and post- PBS lineages. Indeed, each post-PAT lineage showed differences from its corresponding control lineage at only 11 to 208 SNP loci and 76 to 119 INDEL loci, the majority of which (73.4% SNPs and 93.1% INDELs) occurred in the non-coding region of the genome (Supplementary Data). None of these variants occurred in a homozygous state; in other words, all variants were either absent in the post-PBS lineage and heterozygous in the post-PAT lineage, or vice versa. For each strain, the genomic analysis indicates that PAT-exposed lines are genomically very similar to their parental line and that, although a limited number of mutations occur, they are not in genes known to be related to drug resistance in Leishmania.
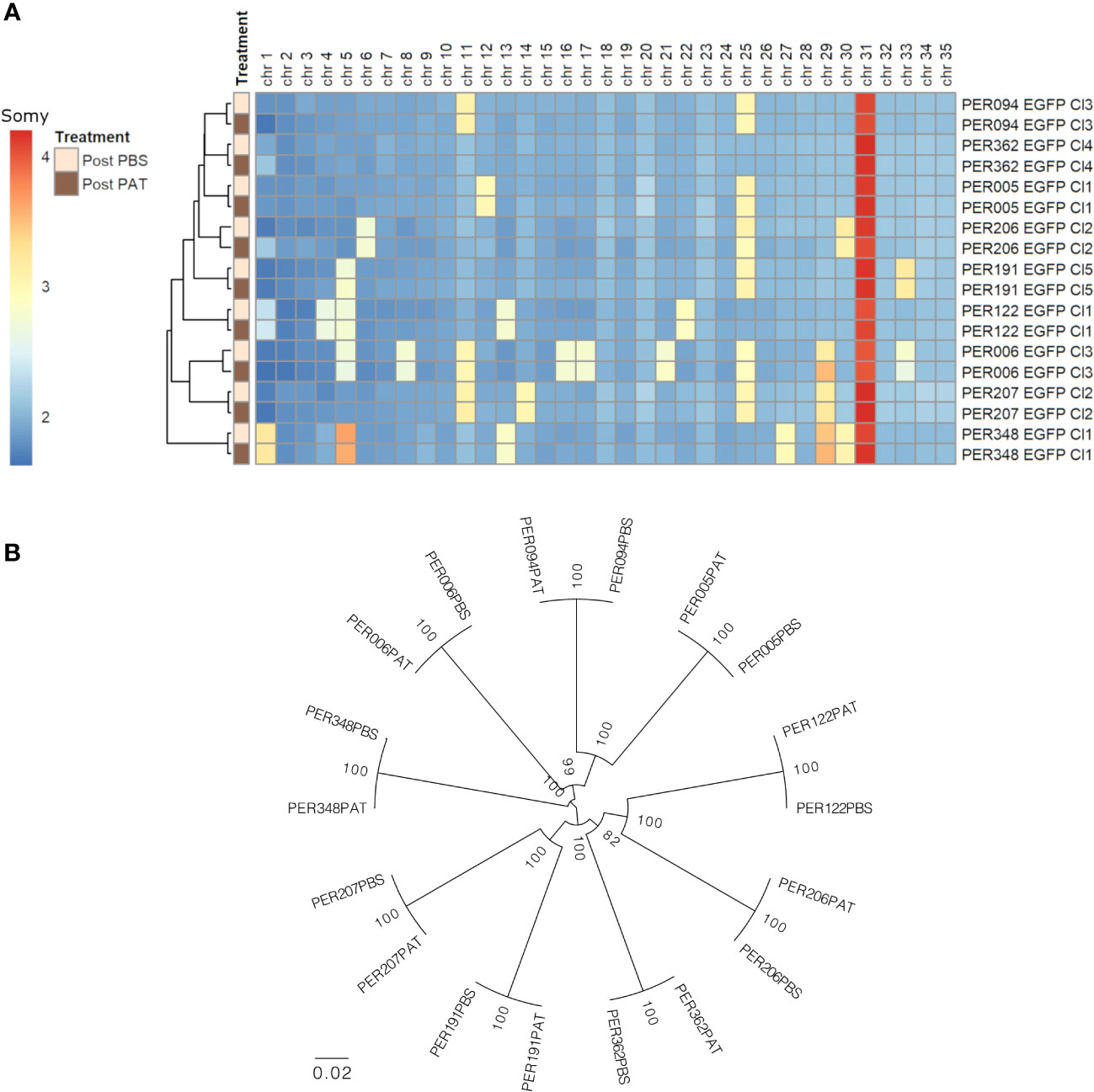
Figure 4 Genomic differences between the nine strains of Leishmania braziliensis and each lineage before (post-PBS) and after (post-PAT) drug pressure; bulk whole genome sequencing. (A) Ploidy. (B) Maximum likelihood phylogenetic tree based on 163,823 high-quality SNPs.
3.5 After prolonged exposure to environmental stress, a small fraction of viable cells survive and show reduced rEGFP expression
Persisters represent another manifestation of quiescence and DT; in bacteriology, these correspond to a subpopulation of DT cells that can survive antibiotic treatment much better than the majority of the population (Barrett et al., 2019). We explored the occurrence of persister-like cells in promastigotes of L. braziliensis PER191 EGFP Cl5 under two conditions of prolonged stress (see Figure 5A for a description of the experimental design): i) by long-term stationary culture, where cells were maintained without subculturing and without PAT over 14 days (Sta D14), and ii) by exposing exponentially growing cells to PAT and maintaining the drug pressure (exposure 1) over 14 days (Log PAT D14). We reasoned that in these cultures majority of the cells would be dead, and only the ones with persister-like properties would remain. In both stress conditions, we tested the resilience of the survivors on day 14, by treating them (exposure 2) with PAT or PBS as untreated control, over 24 h; we measured viability, rEGFP expression, and reversibility to proliferative state.
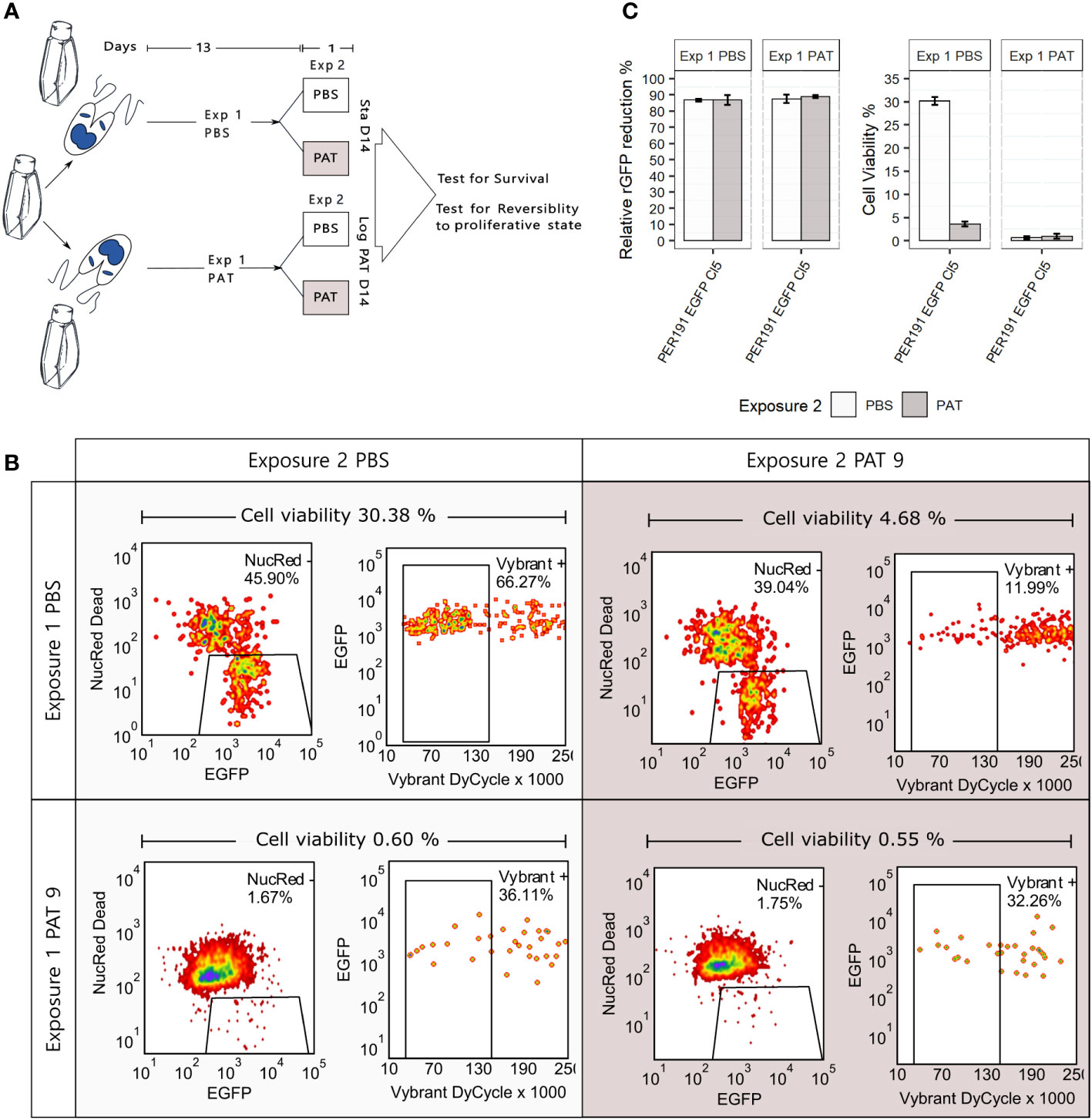
Figure 5 Promastigote (PER191 EGFP Cl5) viability under conditions of longer and stronger environmental stress. (A) Experimental outline. Cell viability as measured by flow cytometry in i) long-term stationary phase on day 14 (Sta D14) or ii) after exposing proliferating cells to 14 days of PAT pressure (log PAT D14); we tested the resilience of the survivors on day 14 after treating them with a second exposure to PAT or PBS as control over 24 h. The concentration of the first and second exposure to PAT was 9 µg/mL. See Materials and methods for details on sequential gating. (B) The density plots showing the percentage of survivors on day 14 in one representative biological replicate per experimental condition. (C) Cell viability and their levels of relative rEGFP reduction in long-term cultures as described in panel (A). The bars represent the mean ± SEM of three biological replicates.
3.5.1 Viability
First, the Sta D14 population receiving an exposure to PBS on day 14 (control) showed an average cell viability of 30.38% (three replicates, only one shown in Figure 5B), while Sta D14 cells treated with PAT on day 14 (exposure 2) showed a significant decrease of their average viability to 4.68% (t-test, P < 0.001). Secondly, the Log PAT D14 population showed a very small fraction of survivors (average of 0.60%) in the untreated control exposed to PBS on day 14, and after a second exposure to PAT, on day 14, there was no significant change in the proportion of survivors (average of 0.55%).
3.5.2 rEGFP expression
There was a significantly higher reduction of the levels of rEGFP expression compared with what was initially observed in cells exposed to 48 h of PAT (Figure 2): 87.5% vs. a maximum of 56.2%, respectively. There was no further rEGFP reduction when Sta D14 and Log PAT D14 samples received exposure 2 to PAT, suggesting that the minimum basal levels of expression required for survival were reached (Figure 5C). Aside from the analysis by flow cytometry, we also looked for the presence of survivors under a confocal microscope. It was possible to observe a very scarce number of cells that had very low levels of rEGFP expression and that, despite remaining at the same location, had a very clear motility of their flagellum.
3.5.3 Reversibility to proliferative state
Survivors from both conditions were able to reproliferate after passing them in a fresh medium. The new population reached 95% and 85% of cell viability by day 7 in Sta D14 and Log PAT D14, respectively.
4 Discussion
We studied here the resilience of L. braziliensis cells surviving high doses of PAT in vitro. Exposure to transient PAT pressure caused a reversible decrease in cell proliferation as well as a significant drop in rDNA transcription, measured here by a decrease in the rEGFP expression among viable cells. The coincidence of these two features is a hallmark of quiescence under stressful conditions (Jara et al., 2022), but this was also observed in an L. lainsoni strain exposed to PAT (Jara et al., 2022). We also found in the present study that PAT exposure did not select for parasites with lower drug susceptibility and that for each L. braziliensis strain, pre- and post-PAT lines were genomically similar, and no markers of DR were encountered. Altogether, these four observations suggest that surviving PAT exposure in our experimental conditions was not due to DR but to a form of quiescence-mediated DT.
Quiescence is a widespread adaptive strategy that allows cells to survive environmental insults. Pathogenic vector- borne parasites like Leishmania are “naturally” exposed to multiple stresses in the insect vector and the mammal host (Caljon et al., 2016), and quiescence could play a major adaptive role among other survival skills. Exposure to drugs is evolutionary recent and constitutes an “artificial” source of stress to which parasites can adapt by genetic variation (DR) or by the exploitation of pre-existing mechanisms of quiescence, through metabolic modulation (DT) as demonstrated here, with PAT. Under high concentrations of PAT (median LD50 of 28 µg/mL), a large proportion of cells remained viable, and this was significantly correlated with a decrease in metabolic activity as measured by the relative rEGFP reduction. As such, this is close to the definition of DT as given in bacteriology, i.e., the general ability of a population of cells to survive longer treatments, a.o. by having a lower killing rate (Balaban et al., 2019), here measured by the LD50 which was approximately 10 times the IC50. Pushing our experimental conditions further —by longer exposure to environmental stress: long-term stationary phase and/or PAT exposure— showed that there were still survivors, albeit a smaller proportion than under short exposure to the drug. This was accompanied by a higher reduction of the rEGFP expression, indicating a deeper quiescent stage in those survivors. These highly resilient parasites are very similar to persisters, a subpopulation of tolerant cells in bacteria, which can survive antibiotic treatment much better than the majority of the population (Balaban et al., 2019).
The study was done with axenic promastigotes (model for the developmental life stage of the sand fly), and the results were confirmed in axenized amastigotes (models for the developmental life stage living in the mammal). Further work is required to extend and confirm these results in an intracellular context and in vivo, where stress factors can be different. Our collection of L. braziliensis strains with high and low capacity to adopt a quiescent state in the promastigote model could be further explored to set up an in-vivo quiescence model. The only report currently available on in-vivo quiescence in the context of chemotherapy revealed, in miltefosine-treated L. mexicana-infected BALB/c mice, the presence of quiescent amastigotes in collagen-rich, dermal mesothelium surrounding granulomas (Kloehn et al., 2021): the authors concluded that quiescence, together with the lack of miltefosine accumulation in the mesothelium, may contribute to drug failure and non-sterile cure. The potential for DT and persistence shown here by L. braziliensis strains and by L. lainsoni (Jara et al., 2022) might explain several (sub)clinical features associated with the species of subgenus Viannia, like i) the presence of parasites in 80% of scars, years after treatment with pentavalent antimony therapy (Schubach et al., 1998); ii) the mucosal metastases from healed primary cutaneous lesions (Jha et al., 2022); and iii) the treatment failure in the absence of DR (Yardley et al., 2006). Longitudinal studies of infected individuals and monitoring of quiescence are needed to test these hypotheses. Such studies are not likely to be easy given the paucity —by definition— of persister cells, and they require positive markers (and not negative markers, like the rEGFP that can be used in experimental studies like here). A first step in that direction was made by a transcriptomic study which indicated that in an overall context of transcriptional shift, some transcripts were relatively more abundant in quiescent cells, like leishmanolysin (GP63), amastin and amastin-like proteins, and autophagy-related genes (Jara et al., 2022).
Noteworthy, the nine L. braziliensis strains here studied showed different levels of quiescence and DT, which was not associated with the large genetic distances between each of the nine strains: for instance, the four strains with the highest LD50 (PER006, 094, 122, and 207) were spread over the phylogenetic tree shown in Figure 4B. There was also no apparent link with the treatment outcome of the patients from which the parasites were originating: for instance, PER006 and PER206, which showed respectively the highest and lowest values of LD50, both came from unresponsive lesions. However, it is too premature to infer any impact of DT level measured in vitro on the treatment outcome in patients. Indeed, a huge selection bias is introduced when isolating and cultivating parasites. There is a strong bottleneck at isolation time, and during in-vitro maintenance, the parasites, which are the fittest in the culture medium, will be selected and will form a dominant population; this is not necessarily the same as the dominant population present in the patient, a phenomenon that we observed in L. donovani (Domagalska et al., 2019). Accordingly, in the next step, quiescence and DT should —as previously recommended for DR (Domagalska and Dujardin, 2020)— also be studied directly in the patient to know its clinical impact and discriminatory molecular markers, and sensitive detection methods should be developed for this.
It is very likely that the results here observed are drug-specific and cannot be generalized to all antileishmanial drugs. Indeed, quiescence is expected to protect against drugs i) interfering with the parasite metabolism and ii) which can be countered by decreasing this metabolism. This is the case for three of the anti-leishmania drugs: pentavalent antimonials (SbV), miltefosine (MIL), and paromomycin (PMM). The reduced form of SbV (PAT) has a direct effect on the parasite by disturbing its redox potential (Wyllie et al., 2004), and a similar effect is expected with SbV itself which has an indirect effect through interfering with the signaling of the macrophage and triggering ROI/RNI production in the host cell (Basu et al., 2006). MIL interferes with the biosynthesis of lipids including sterols and sphingolipids (Armitage et al., 2018) and the metabolism of alkyl-lipids, also inducing mitochondrial depolarization and a decrease of intracellular levels of ATP (Ponte-Sucre et al., 2017). PMM targets the decoding A-site of the ribosomes’ small subunit increasing misreading and translation inhibition (Chawla et al., 2011). In contrast, amphotericin B which binds to ergosterol-related sterols in the cell membrane, inducing the production of a pore and fatal exchange of ions (Ramos et al., 1996), is expected to induce less (if any) quiescence and DT.
Research on quiescence, DT, and persistence in Leishmania is still in its infancy. Aside from providing knowledge that might help better understand the pathophysiology of Leishmania infections, it might also support and guide further investigations on new chemotherapeutic interventions to counter the disease as well as the infection. Historically, research into new drugs screened replicative forms, creating a leishmaniasis intervention tool kit that neglects the impact of quiescent forms. We provide here a new model of quiescence and DT that could be further exploited for the in-vitro screening of compounds to overcome the quiescence resilience of the parasite, thereby improving the therapy of leishmaniasis.
Data availability statement
The datasets presented in this study can be found in online repositories. The names of the repository/repositories and accession number(s) can be found in the article. The raw data from the WGS for this study can be found in the Sequence Read Archive (SRA) submission: SUB12410472 (https://submit.ncbi.nlm.nih.gov/subs/sra/SUB12410472/overview).
Author contributions
All authors made substantial contributions to the conception or design of the work; the acquisition, analysis, or interpretation of data; drafted the work or revised it critically for important intellectual content; agreed to be accountable for all aspects of the work in ensuring that questions related to the accuracy or integrity of any part of the work are appropriately investigated and resolved. All authors contributed to the article and approved the submitted version.
Funding
This study was financially supported by the Flemish Fund for Scientific Research (grant G075121N, postdoctoral grant to MJ, FWO-1223420N, and postdoctoral grant to FVdB, FWO-1226120N), the European Commission (contracts ICA4-CT-2001-10076 and INCO-CT2005-015407), and the Flemish Ministry of Science and Innovation (Secondary Research Funding ITM – SOFI, Grant MADLEI).
Acknowledgments
We acknowledge Ilse Maes for her logistic support in the lab.
Conflict of interest
The authors declare that the research was conducted in the absence of any commercial or financial relationships that could be construed as a potential conflict of interest.
Publisher’s note
All claims expressed in this article are solely those of the authors and do not necessarily represent those of their affiliated organizations, or those of the publisher, the editors and the reviewers. Any product that may be evaluated in this article, or claim that may be made by its manufacturer, is not guaranteed or endorsed by the publisher.
Supplementary material
The Supplementary Material for this article can be found online at: https://www.frontiersin.org/articles/10.3389/fcimb.2023.1253033/full#supplementary-material
References
Armitage, E. G., Alqaisi, A. Q. I., Godzien, J., Peña, I., Mbekeani, A. J., Alonso-Herranz, V., et al. (2018). Complex interplay between sphingolipid and sterol metabolism revealed by perturbations to the leishmania metabolome caused by miltefosine. Antimicrob. Agents Chemother. 62, e02095-17. doi: 10.1128/AAC.02095-17
Balaban, N. Q., Helaine, S., Lewis, K., Ackermann, M., Aldridge, B., Andersson, D. I., et al. (2019). Definitions and guidelines for research on antibiotic persistence. Nat. Rev. Microbiol. 17, 441–448. doi: 10.1038/S41579-019-0196-3
Barrett, M. P., Kyle, D. E., Sibley, L. D., Radke, J. B., Tarleton, R. L. (2019). Protozoan persister-like cells and drug treatment failure. Nat. Rev. Microbiol. 17, 607–620. doi: 10.1038/s41579-019-0238-x
Basu, J. M., Mookerjee, A., Sen, P., Bhaumik, S., Sen, P., Banerjee, S., et al. (2006). Sodium antimony gluconate induces generation of reactive oxygen species and nitric oxide via phosphoinositide 3-kinase and mitogen-activated protein kinase activation in Leishmania donovani-infected macrophages. Antimicrob. Agents Chemother. 50, 1788–1797. doi: 10.1128/AAC.50.5.1788-1797.2006
Bojsen, R., Regenberg, B., Folkesson, A. (2017). Persistence and drug tolerance in pathogenic yeast. Curr. Genet. 63, 19–22. doi: 10.1007/S00294-016-0613-3
Bolhassani, A., Taheri, T., Taslimi, Y., Zamanilui, S., Zahedifard, F., Seyed, N., et al. (2011). Fluorescent Leishmania species: Development of stable GFP expression and its application for in vitro and in vivo studies. Exp. Parasitol. 127, 637–645. doi: 10.1016/j.exppara.2010.12.006
Caljon, G., De Muylder, G., Durnez, L., Jennes, W., Vanaerschot, M., Dujardin, J.C (2016). Alice in microbes’ land: Adaptations and counter-adaptations of vector-borne parasitic protozoa and their hostsa. FEMS Microbiol. Rev. 40, 664–685. doi: 10.1093/femsre/fuw018
Chawla, B., Jhingran, A., Panigrahi, A., Stuart, K. D., Madhubala, R. (2011). Paromomycin affects translation and vesicle-mediated trafficking as revealed by proteomics of paromomycin -susceptible -resistant Leishmania donovani. PloS One 6, e26660. doi: 10.1371/JOURNAL.PONE.0026660
Cingolani, P., Platts, A., Wang, L. L., Coon, M., Nguyen, T., Wang, L., et al. (2012). A program for annotating and predicting the effects of single nucleotide polymorphisms, SnpEff: SNPs in the genome of Drosophila melanogaster strain w1118; iso-2; iso-3. Fly (Austin) 6, 80–92. doi: 10.4161/fly.19695
Danecek, P., Bonfield, J. K., Liddle, J., Marshall, J., Ohan, V., Pollard, M. O., et al. (2021). Twelve years of SAMtools and BCFtools. Gigascience 10, giab008. doi: 10.1093/GIGASCIENCE/GIAB008
Domagalska, M. A., Dujardin, J.-C. (2020). Next-generation molecular surveillance of triTryp diseases. Trends Parasitol. 36, 356–367. doi: 10.1016/j.pt.2020.01.008
Domagalska, M. A., Imamura, H., Sanders, M., Van den Broeck, F., Bhattarai, N. R., Vanaerschot, M., et al. (2019). Genomes of Leishmania parasites directly sequenced from patients with visceral leishmaniasis in the Indian subcontinent. PloS Negl. Trop. Dis. 13, e0007900. doi: 10.1371/journal.pntd.0007900
Dumetz, F., Imamura, H., Sanders, M., Seblova, V., Myskova, J., Pescher, P. (2017). Modulation of aneuploidy in leishmania in vitro and in vivo environments and its. mBio 8, e00599–e00517. doi: 10.1128/mBio.00599-17
Hoang, D. T., Chernomor, O., Von Haeseler, A., Minh, B. Q., Vinh, L. S. (2018). UFBoot2: improving the ultrafast bootstrap approximation. Mol. Biol. Evol. 35, 518–522. doi: 10.1093/MOLBEV/MSX281
Jara, M., Barrett, M., Maes, I., Regnault, C., Imamura, H., Domagalska, M. A., et al. (2022). Transcriptional shift and metabolic adaptations during leishmania quiescence using stationary phase and drug pressure as models. Microorganisms 10, 97. doi: 10.3390/microorganisms10010097
Jara, M., Maes, I., Imamura, H., Domagalska, M. A., Dujardin, J. C., Arevalo, J. (2019). Tracking of quiescence in Leishmania by quantifying the expression of GFP in the ribosomal DNA locus. Sci. Rep. 9, 18951. doi: 10.1038/S41598-019-55486-Z
Jha, B., Reverte, M., Ronet, C., Prevel, F., Morgenthaler, F. D., Desponds, C., et al. (2022). In and out: Leishmania metastasis by hijacking lymphatic system and migrating immune cells. Front. Cell Infect. Microbiol. 12. doi: 10.3389/FCIMB.2022.941860
Keren, I., Minami, S., Rubin, E., Lewis, K. (2011). Characterization and transcriptome analysis of Mycobacterium tuberculosis persisters. mBio 2, e00100-11. doi: 10.1128/MBIO.00100-11
Kloehn, J., Boughton, B. A., Saunders, E. C., O’callaghan, S., Binger, K. J., McConville, M. J. (2021). Identification of metabolically quiescent leishmania mexicana parasites in peripheral and cured dermal granulomas using stable isotope tracing imaging mass spectrometry. mBio 12, e00129–e00121. doi: 10.1128/mBio.00129-21
Kloehn, J., Saunders, E. C., O’Callaghan, S., Dagley, M. J., McConville, M. J. (2015). Characterization of metabolically quiescent leishmania parasites in murine lesions using heavy water labeling. PloS Pathog. 11, e1004683. doi: 10.1371/journal.ppat.1004683
Lira, R., Sundar, S., Makharia, A., Kenney, R., Gam, A., Saraiva, E., et al. (1999). Evidence that the high incidence of treatment failures in Indian kala-azar is due to the emergence of antimony-resistant strains of Leishmania donovani. J. Infect. Dis. 180, 564–567. doi: 10.1086/314896
Mandell, M. A., Beverley, S. M. (2017). Continual renewal and replication of persistent Leishmania major parasites in concomitantly immune hosts. Proc. Natl. Acad. Sci. U.S.A. 114, E801–E810. doi: 10.1073/pnas.1619265114
Michiels, J. E., Van Den Bergh, B., Verstraeten, N., Fauvart, M., Michiels, J. (2016). In vitro emergence of high persistence upon periodic aminoglycoside challenge in the ESKAPE pathogens. Antimicrob. Agents Chemother. 60, 4630–4637. doi: 10.1128/AAC.00757-16
Nguyen, L. T., Schmidt, H. A., Von Haeseler, A., Minh, B. Q. (2015). IQ-TREE: a fast and effective stochastic algorithm for estimating maximum-likelihood phylogenies. Mol. Biol. Evol. 32, 268–274. doi: 10.1093/MOLBEV/MSU300
Ponte-Sucre, A., Gamarro, F., Dujardin, J. C., Barrett, M. P., López-Vélez, R., García-Hernández, R., et al. (2017). Drug resistance and treatment failure in leishmaniasis: A 21st century challenge. PloS Negl. Trop. Dis. 11, e0006052. doi: 10.1371/JOURNAL.PNTD.0006052
Ramos, H., Valdivieso, E., Gamargo, M., Dagger, F., Cohen, B. E. (1996). Amphotericin B kills unicellular leishmanias by forming aqueous pores permeable to small cations and anions. J. Membr. Biol. 152, 65–75. doi: 10.1007/S002329900086
Rijal, S., Yardley, V., Chappuis, F., Decuypere, S., Khanal, B., Singh, R., et al. (2007). Antimonial treatment of visceral leishmaniasis: are current in vitro susceptibility assays adequate for prognosis of in vivo therapy outcome? Microbes Infect. 9, 529–535. doi: 10.1016/J.MICINF.2007.01.009
Ritz, C., Baty, F., Streibig, J. C., Gerhard, D. (2015). Dose-response analysis using R. PloS One 10, e0146021. doi: 10.1371/journal.pone.0146021
Schubach, A., Haddad, F., Neto, M. P. O., Degrave, W., Pirmez, C., Grimaldi, G., et al. (1998). Detection of Leishmania DNA by polymerase chain reaction in scars of treated human patients. J. Infect. Dis. 178, 911–914. doi: 10.1086/515355
Teuscher, F., Chen, N., Kyle, D. E., Gatton, M. L., Cheng, Q. (2012). Phenotypic changes in artemisinin-resistant Plasmodium falciparum lines in vitro: evidence for decreased sensitivity to dormancy and growth inhibition. Antimicrob. Agents Chemother. 56, 428–431. doi: 10.1128/AAC.05456-11
Van den Broeck, F., Savill, N. J., Imamura, H., Sanders, M., Maes, I., Cooper, S., et al. (2020). Ecological divergence and hybridization of Neotropical Leishmania parasites. Proc. Natl. Acad. Sci. U.S.A. 117, 25159–25168. doi: 10.1073/pnas.1920136117
Van Meirvenne, N., Janssens, P. G., Magnus, E. (1975)Antigenic variation in syringe passaged populations of Trypanosoma (Trypanozoon) brucei. 1. Rationalization of the experimental approach (Accessed June 3, 2020).
WHO Ending the neglect to attain the Sustainable Development Goals: A road map for neglected tropical diseases 2021–2030. Available at: https://www.who.int/publications/i/item/9789240010352 (Accessed June 23, 2021).
Wyllie, S., Cunningham, M. L., Fairlamb, A. H. (2004). Dual action of antimonial drugs on thiol redox metabolism in the human pathogen Leishmania donovani. J. Biol. Chem. 279, 39925–39932. doi: 10.1074/JBC.M405635200
Keywords: Leishmania, antimonials, quiescence, drug tolerance, persisters
Citation: Jara M, Arevalo J, Llanos-Cuentas A, Broeck FVd, Domagalska MA and Dujardin J-C (2023) Unveiling drug-tolerant and persister-like cells in Leishmania braziliensis lines derived from patients with cutaneous leishmaniasis. Front. Cell. Infect. Microbiol. 13:1253033. doi: 10.3389/fcimb.2023.1253033
Received: 04 July 2023; Accepted: 21 August 2023;
Published: 18 September 2023.
Edited by:
Chaoqun Yao, Ross University, United StatesReviewed by:
Danilo Ciccone Miguel, State University of Campinas, BrazilBudhaditya Mukherjee, Indian Institute of Technology Kharagpur, India
Copyright © 2023 Jara, Arevalo, Llanos-Cuentas, Broeck, Domagalska and Dujardin. This is an open-access article distributed under the terms of the Creative Commons Attribution License (CC BY). The use, distribution or reproduction in other forums is permitted, provided the original author(s) and the copyright owner(s) are credited and that the original publication in this journal is cited, in accordance with accepted academic practice. No use, distribution or reproduction is permitted which does not comply with these terms.
*Correspondence: Marlene Jara, bWFybGVuZS5qYXJhLnBAZ21haWwuY29t; Jean-Claude Dujardin, amNkdWphcmRpbkBpdGcuYmU=