- 1State Key Laboratory of Medical Genomics, Shanghai Institute of Hematology, National Research Center for Translational Medicine, Shanghai Rui Jin Hospital, Shanghai Jiao Tong University School of Medicine, Shanghai, China
- 2Collaborative Innovation Center of Hematology, Shanghai Jiao Tong University School of Medicine, Shanghai, China
- 3Bone Marrow Transplantation Center, The First Affiliated Hospital, School of Medicine, Zhejiang University, Hangzhou, China
- 4Centre of Clinical Laboratory, The First Affiliated Hospital, School of Medicine, Zhejiang University, Hangzhou, China
- 5Department of Pulmonary and Critical Care Medicine, Ruijin Hospital, Shanghai Jiao Tong University School of Medicine, Shanghai, China
- 6Institute of Respiratory Diseases, Shanghai Jiao Tong University School of Medicine, Shanghai, China
- 7GoBroad Medical Institute of Hematology (Shanghai Center), Liquan Hospital, Shanghai, China
Introduction: Immunosuppression predisposes allogeneic hematopoietic stem cell transplantation (allo-HSCT) recipients to infection. Prompt and accurate identification of pathogens is crucial to optimize treatment strategies. This multi-center retrospective study aimed to assess the ability of metagenomic next-generation sequencing (mNGS) to detect causative pathogens in febrile allo-HSCT recipients and examined its concordance with conventional microbiological tests (CMT).
Methods: We performed mNGS and CMT on samples obtained from 153 patients with suspected infection during allo-HSCT. Patients were grouped based on their neutropenic status at the time of sampling.
Results: The mNGS test was more sensitive than CMT (81.1% vs. 53.6%, P<0.001) for diagnosing clinically suspected infection, especially in the non-neutropenia cohort. mNGS could detect fungi and viruses better than bacteria, with a higher sensitivity than CMT. Immune events were diagnosed in 57.4% (35/61) of the febrile events with negative mNGS results, and 33.5% (48/143) with negative CMT results (P=0.002). The treatment success rate of the targeted anti-infection strategy was significantly higher when based on mNGS than on empirical antibiotics (85% vs. 56.5%, P=0.004).
Conclusion: The mNGS test is superior to CMT for identifying clinically relevant pathogens, and provides valuable information for anti-infection strategies in allo-HSCT recipients. Additionally, attention should be paid to immune events in patients with negative mNGS results.
1 Introduction
Allogeneic hematopoietic stem cell transplantation (allo-HSCT) is a potentially curative therapy for various hematological disorders (Copelan et al., 2019). However, this life-saving procedure poses considerable challenges to patients. Post-transplant immunodeficiency, including severe neutropenia, increases the risk of life-threatening infection, one of the main causes of non-relapse mortality (Neofytos, 2019). The intensive conditioning regimens and immunosuppressive agents used in graft-versus-host disease (GVHD) prophylaxis and treatment further contribute to prolonged immune reconstitution (Zeiser and Blazar, 2017; Gagelmann and Kroger, 2021; Martinez-Cibrian et al., 2021). However, infection signs during transplantation are usually covert and difficult to differentiate from other non-infectious events, such as GVHD. On the other hand, infection, particularly virus infection, play an important role in the occurrence of immune events (Khosla et al., 2018; Ghobadi et al., 2019). Therefore, infection after allo-HSCT is a markedly intricate and multifactorial, distinguishable from infection following chemotherapy, and is the leading risk factor in different phases resulting from different responsible pathogens infection (Sahin et al., 2016). Hence, rapid and accurate identification of causative pathogens is urgently needed to facilitate timely therapy.
Smear microscopy, polymerase chain reaction (PCR) and culture are the conventional microbiological tests (CMT) most commonly used to identify pathogens. However, these methods are relatively insensitive, and pathogen culture is time-consuming. In last 5 years, metagenomic next-generation sequencing (mNGS) has emerged as a powerful alternative method to overcome suchchallenges (Lu et al., 2020), as it can efficiently detect pathogenic microorganisms which are difficult to detect via CMT and distinguish etiologic microorganisms from background commensals with high efficiency and short turnaround time(Huang et al., 2020). However, the diagnostic value of mNGS has not been well evaluated for infection in the context of allo-HSCT, in which more than 80% of febrile events with negative CMT results. In this study, we evaluated the ability of mNGS to detect pathogens in febrile allo-HSCT recipients and examined its concordance with CMT.
2 Materials and methods
2.1 Study design
We retrospectively analyzed the medical records of patients who received allo-HSCT at Ruijin Hospital, the First Affiliated Hospital of Zhejiang University, and Liquan Hospital, GoBroad Medical Institute of Hematology (Shanghai Center) from April 2021 to January 2023. Institutional databases were retrospectively reviewed to extract demographic, clinical and genetic data. All procedures complied with the tenets of the Helsinki Declaration. The requirement for written informed consent was waived, owing to the non-interventional and retrospective nature of the study.
2.2 Infection prophylaxis after allo-HSCT
For herpes simplex virus (HSV) prophylaxis, acyclovir was given at the dose of 400 mg twice a day for 12 months after transplantation (Henze et al., 2022). For pneumocystis jirovecii pneumonia (PJP) prophylaxis, sulfamethoxazole was administrated at a dose of 480mg thrice a week from 2 months to 12 months (Maschmeyer et al., 2016). Between April 2021 and August 2022 (before the introduction of letermovir in China), 111 allo-HSCT recipients were treated with ganciclovir with 5mg/kg twice a day for 7 days prior to transplantation as cytomegalovirus (CMV) prophylaxis (Ljungman et al., 2019). After August 2022, 42 allo-HSCT recipients were treated with letermovir at a dose adjusted to calcineurin inhibitors for at least 3 months. For primary fungal prophylaxis, all patients received posaconazole oral suspension at an initial dose of 200mg thrice a day at the start of the conditioning regimens (Maertens et al., 2018). The efficacy of posaconazole treatment was determined based on therapeutic drug monitoring. The optimal serum trough concentration is>0.5mg/ml for prophylaxis (Chen et al., 2018). In case the trough concentration did not reach the prophylactic concentration, we prefer to increment the dosing frequency to 200mg every 4-6 hours, or switching to tablets or intravenous formulation. The duration of fungal prevention is up to 6 months for allo-HSCT with haploidentical donors (HID) or matched/mismatched unrelated donors (MUD/MMUD) and 3 months for allo-HSCT with matched sibling donors (MSD).
2.3 Standards for mNGS and CMT detection
The criteria for determining positive mNGS detection varied depending on the type of microbes being detected (Xu et al., 2023). A microbe was considered a positive result for bacteria and fungi when the stringently mapped read number (SMRN) at the species level was ≥3 and relative abundance at the genus level was >30%. A microbe was considered a positive result for viruses when the SMRN was ≥3. A microbe was considered positive for parasites when the SMRN was ≥100.
All patients underwent CMT as indicated by the treating physicians. Different specimens were collected for testing based on the type of suspected infection (i.e., blood samples, puncture fluids, BALF, and CSF). Positive CMT results was verified when bacteria, fungi were detected via smear microscopy, culture, and (1,3)-β-D-glucan test (G test), or galactomannan test (GM test). Viruses including CMV and Epstein-Barr virus (EBV), were verified with plasma twice a week via the quantitative real-time PCR. HSV1 was verified by PCR with oropharyngeal swab samples.”
2.4 Definition for mNGS and CMT results assessment
The diagnosis was made by an independent clinical committee comprising two hematologists, one pulmonologist and one radiologist. We comprehensively evaluated the clinical symptoms, radiological manifestations, CMT, and responses to anti-infection therapy to classify the final diagnosis into four categories: clinically significant infection, infection without clinical significance, immune events, mixed events of clinically significant infection and immune events. Also, the identification of final causative pathogens was made based on comprehensive consideration. The mNGS results was classified as definite, probable, possible, unlikely, or false-negative causes of infection. (1) Definite: microbes detected were consistent with those detected by CMT; (2) Probable: microbes detected were probably causative pathogens; (3) Possible: microbes detected showed potential to cause infection, but not as a common cause based on the consideration of clinical medical records; (4) Unlikely: microbes detected by mNGS were non-causative pathogens; (5) False negative: mNGS result was negative, but the case was diagnosed with infection. On the condition of “Definite”, “Probable” and “Possible”, mNGS results were judged as true positive, while on the condition of “Unlikely” they tended to be false positive. The positive predictive value (PPV) and negative predictive value (NPV) of mNGS were calculated as the ratio of true positive or negative mNGS detection to all practical mNGS detection. Initial empirical antibiotic therapy was performed within 24-48 h of clinical signs (Wang et al., 2020). Treatment success was defined as the resolution or reduction of clinical symptoms. CMV detection is defined as the detection and quantification of CMV DNA by either mNGS or PCR in plasma (Ljungman et al., 2017). The definition of clinically significant CMV infection requires clinical symptoms and CMV DNA viremia, necessitating the initiation of anti-CMV therapy by a treating physician (Chemaly et al., 2020).
2.5 Statistical analysis
Data analysis and visual representation of the results were performed using IBM SPSS Statistics for Windows, version 25.0 (IBM Corp., Armonk, N.Y., USA) and R 4.2.0 software (R Foundation for Statistical Computing, Vienna, Austria). Continuous and categorical variables were presented as medians and rang variables, as well as counts and percentages, respectively. Comparative analysis was conducted by Pearson χ2 test and Fisher exact test for discrete variables where appropriate. P-value <0.05 was considered to be statistically significant.
3 Results
3.1 Patient demographics and sample collection
Based on the inclusion or exclusion criteria (Figure 1), we included 271 samples with parallel results for mNGS and CMT from 153 participants. General characteristics of 153 patients were summarized in Table 1. The median age was 47 (range, 15-69) years. We divided these samples into two cohorts based on neutropenic status at the time of sampling: cohort A (neutropenia [n=92]) and cohort B (non-neutropenia [n=179]) (Table 2). The most frequent mNGS sampling specimen was peripheral blood (78.2%), followed by BALF (10.7%), CSF (8.5%), and others (2.6%). Peripheral blood specimen was more common in cohort A than cohort B due to the non-invasive and easily accessible nature.
3.2 Performance of mNGS and CMT for pathogen detection
We excluded 13 paired mNGS samples collected from different tissues in the same febrile event, and a comparison of the diagnostic results of mNGS with those of CMT methods was made for all 153 participants with 258 samples. The positive detection rate of mNGS was 76.4%, significantly higher than 43.8% of CMT (P<0.001). The positive detection rate was much higher with mNGS when compared with CMT in both cohort A (70.7% vs. 39.1% P<0.001) and cohort B (79.5% vs. 46.4% P<0.001). mNGS and CMT were concordant for 50 of 92 (54.3%) patients (kappa=0.128) in cohort A, and 91 of 166 (54.8%) patients (kappa=0.130) in cohort B (Table 3).
The detection rate of mNGS in peripheral blood specimens was 75.0%, higher than 41.5% with CMT methods (P<0.001). In non-blood specimens, the detection rates with mNGS and CMT were 79.7% and 54.2%, respectively (P=0.003).
3.3 Microbes landscape detected with mNGS and CMT
Regarding the clinical causative pathogens, bacteria accounted for 57.4% of infection in cohort A, followed by viruses (29.4%) and fungi (13.2%). In cohort B, viruses accounted for 61.1%, followed by bacteria (24.8%) and fungi (14.1%).
For bacteria, mNGS reached a 22.9% positivity rate compared with CMT (16.7%, P=0.077). There was no significant difference in the detection rates of bacteria using mNGS and CMT in cohorts A and B (Figure 2A). Similar results were observed when peripheral blood specimens were analyzed (Figure 2B). Among the 31 suspected bacterial infection cases in cohort A, mNGS results for 16 cases were consistent with CMT methods, with a concordance rate of 51.6% (16/31), and the concordance rate was 35.7% (10/28) in cohort B (Figure 3). Distribution of microbes detected by mNGS and CMT is shown in Supplemental Figure S1.
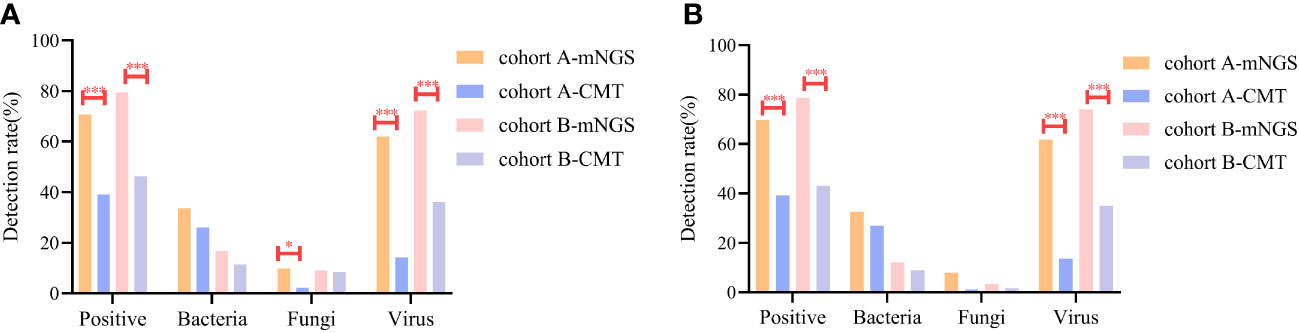
Figure 2 Comparison of detection sensitivities of the specific pathogens with mNGS and CMT in two cohorts. (A) Total specimens (B) Blood specimen. * P<0.05 *** P<0.001.
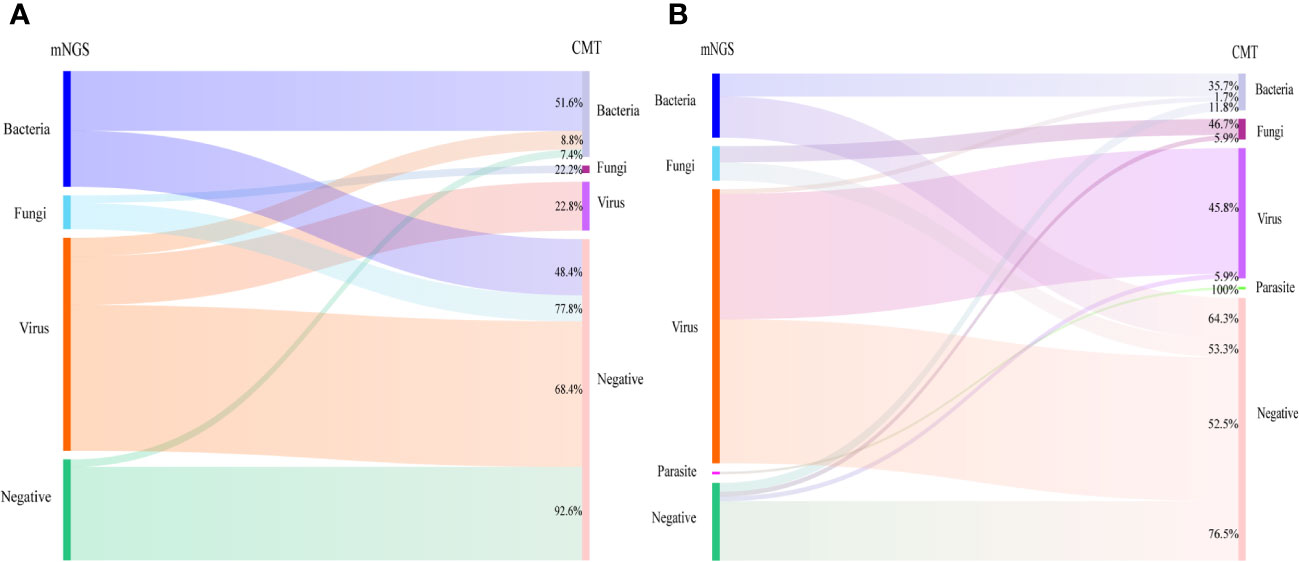
Figure 3 Summary of the relationship between mNGS and CMT results in cohort A (A) and cohort B (B). Cohort A: neutropenia; Cohort B: non-neutropenia.
For suspected fungi, the detection rate with mNGS was similar to that of CMT (9.3% vs. 6.2% P=0.188) in all cases, but was higher than that of CMT in cohort A (9.8% vs. 2.2% P=0.03, Figure 2A). In cohort A and cohort B, 77.8% and 53.3% of the fungal infection verified by mNGS were not confirmed by CMT, respectively (Figure 3). Of the 14 cases of Aspergillus infection, thirteen cases (92.9%) were detected via mNGS, and only one case (A7, Supplemental Table S1) was detected via CMT method. A1 patient had respiratory symptoms and radiologic manifestation one month after mNGS-positive detection with peripheral blood in the neutropenic stage (Supplemental Figure S2).
More viruses had been identified via mNGS than via CMT because of its high sensitivity (cohort A: 62.0% vs. 14.1% P<0.001; cohort B: 72.3% vs. 36.1% P<0.001) (Figure 2). The concordance rates between mNGS and CMT for viral detection were 22.8% (13/57) in cohort A and 45.8% (55/120) in cohort B (Figure 3). CMV was the most frequently detected virus in both cohorts (cohort A: 33.7%; cohort B: 45.2%). As shown in Figure 4, the peak CMV detection rate using mNGS and the clinically significant CMV infection rate were concentrated between 30 and 60 days after allo-HSCT.
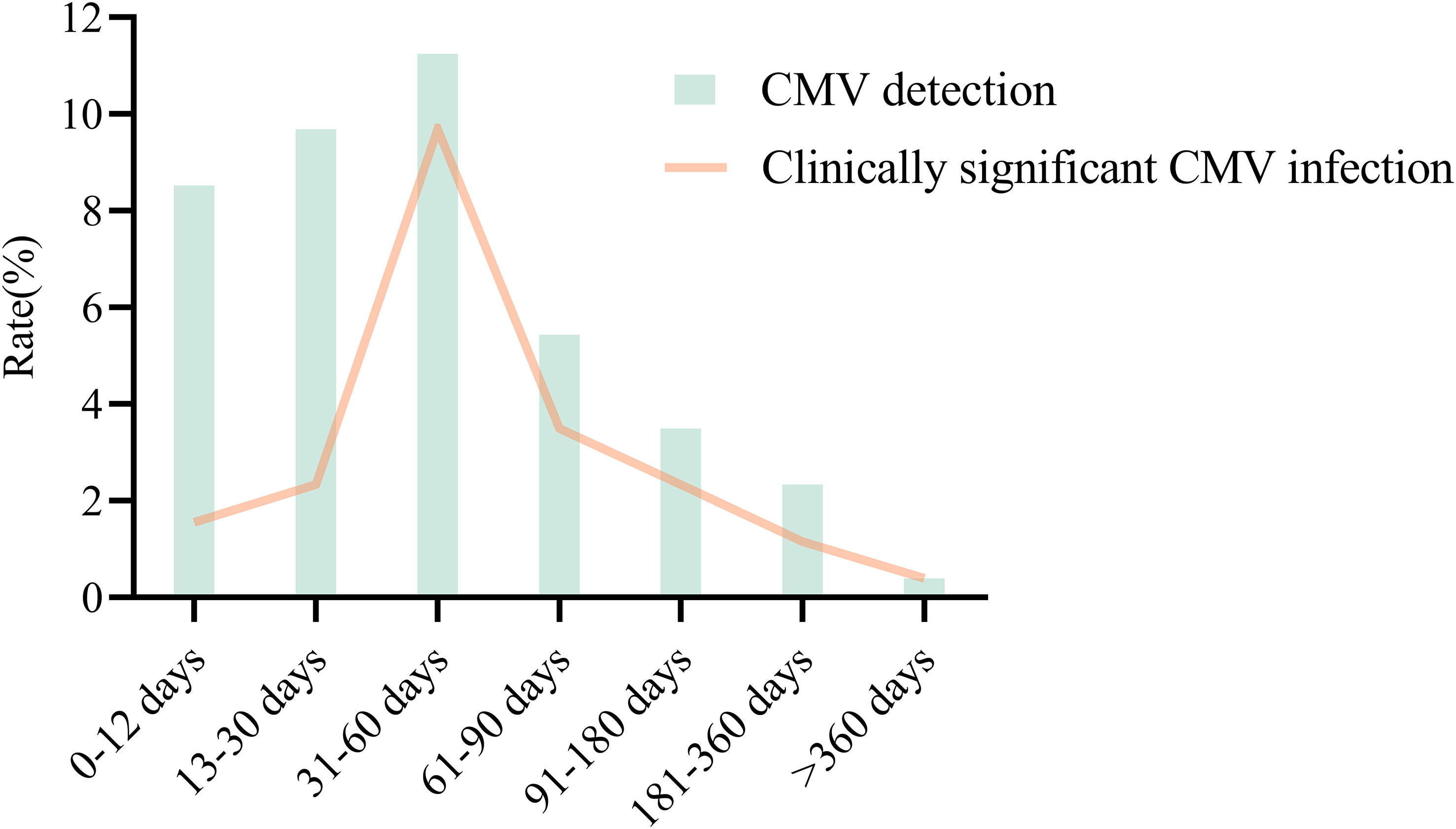
Figure 4 CMV detection and clinically significant CMV infection in different time periods after allo-HSCT.
3.4 Microbes in non-blood specimens
In BALF specimens (29 cases, Supplemental Table S2), Pneumocystis jirovecii (P. jirovecii) and viruses constituted 79.3% of the microbes detected (Supplemental Figure S3A). Notably, P. jirovecii was detected only by mNGS. In patients with confirmed infectious pneumonia, the mNGS-positive detection rates were 89.3% (25/28 cases) and 64.2% for CMT (18/28 cases). In cases with PJP, the positive rate of the G test in the BALF was 83.3% (5/6 cases), and 12.5% in the peripheral blood (1/8). In 11 cases with positive BALF G/GM results, fungi were detected by mNGS in 6 cases (54.5%), including Candida in 16.7% (1 case) and P. jirovecii in 83.3% (5 cases). In 13 cases with Aspergillus infection verified by mNGS, five of which (38.5%, 5/13) was detected in BALF with mNGS test.
Viruses accounted for 69.6% of causative pathogens in suspected encephalitis (Supplemental Table S3 and Figure S3B). Four patients (27.7%, C2, C5, C6, C18, C23) had simultaneous viremia when diagnosed with viral encephalitis, indicating that CSF testing is pivotal when encephalitis is suspected.
3.5 Diagnostic efficacies of mNGS and CMT on clinically suspected infection and immune events
A total of 168 patients (65.1%) were eventually diagnosed with clinically significant infection, 12 (4.7%) with infection but without clinical significance, 28 (10.9%) with mixed events, and 50 (19.4%) with immune events. The diagnostic capabilities of mNGS, CMT, and the combined protocols for different samples are shown in Table 4. The mNGS test yielded a higher sensitivity (77.4% vs. 52.9%, P<0.001) than the CMT for diagnosing clinically significant infection in peripheral blood samples, and the effect was more prominent in cohort B. In non-peripheral blood specimens, mNGS tended towards higher sensitivity than in peripheral blood specimens. (88.7% vs. 77.4%, P=0.075).
Among the febrile events with negative mNGS results (61 samples), 35 patients (57.4%) were eventually diagnosed with immune events. Among the patients with negative CMT results (143 samples), 48 (33.5%) were diagnosed with immune events (P=0.002). The detailed immune events types are displayed in Supplemental Table S4.
3.6 mNGS in patients with negative CMT results
The spectrum of microorganisms in CMT negative cases were displayed in Supplemental Figure S5. In cohort A, 30 cases with negative CMT results were clinically diagnosed with an infection. Among the 30 cases, mNGS detected pathogens matched the final causative pathogens in 20 cases, including 10 cases of bacterial infection, 2 of fungal infection, 5 of viral infection and 3 of polymicrobial infection. In cohort B, mNGS verified 7 cases of bacterial infection, 3 of fungal infection, 26 of viral infection, and 5 of polymicrobial infection in infected participants with negative CMT results.
Lastly, we retrospectively reviewed the antibiotic regimen and clinical outcomes of the 86 patients. The overall treatment success rate (TSR) was 85% (34/40) for patients treated based on the mNGS results, and 56.5% for those receiving empirical therapy (26/46, P=0.004, Supplemental Figure S4).
4 Discussion
Developing targeted and precise antibiotic therapies without pathogenic evidence remains a substantial challenge in allo-HSCT recipients with underlying infection. In this retrospective study, we evaluated two approaches for microbial detection in allo-HSCT recipients: mNGS and CMT. Few studies have explored the clinical application of mNGS in allo-HSCT recipients (Liu et al., 2021; Zanella et al., 2021; Qu et al., 2022). Our study revealed the diagnostic efficacy superiority of mNGS in immunocompromised patients regardless of their neutropenic status.
The positivity rate of mNGS (76.4%) in our study was approximately 30% higher than that of CMT, consistent with a previous report (Xu et al., 2023). CMT is typically limited by its low coverage rate or restricted detection of a number of suspected microbes (Duan et al., 2021). Real-time metagenomics methods can identify pathogens faster than traditional culture-based techniques and potentially identify pathogens that cannot grow in cultures (Pendleton et al., 2017). Based on the final clinical diagnosis, our results showed that the sensitivity of mNGS (81.1%) was significantly higher than that of CMT (53.6%) for clinical infection requiring intervention. Other groups have reported similar results, highlighting the potential of mNGS to provide etiological evidence in CMT-negative patients (Miao et al., 2018; Wang et al., 2020). However, the specificity was lower than that reported previously (Miao et al., 2018), possibly because of false-positive results of low-quality virus readings. Nevertheless, the combination of mNGS and CMT increased diagnostic accuracy to 88.3%. We compared different antibiotic regimens with or without reference to mNGS results and demonstrated that mNGS had a positive impact on antibiotic adjustment decisions, especially in cases with negative CMT results. Additionally,immune events accounted for a higher proportion of mNGS-negative cases (52.6%) after allo-HSCT, indicating that negative mNGS might hint the presence of immune events, spanning acute GVHD, haplo-fever, engraftment syndrome (ES) and cryptogenic organizing pneumonia (COP, Supplemental Table S4). When physicians make a diagnosis of immune events and initiate steroid therapy, mNGS is recommended to rule out infection events, particularly for non-blood specimens.
Risk factors in the pre-engraftment phase include the presence of neutropenia, lasting for approximately 15–30 days (Sahin et al., 2016). Compared with patients with neutropenia after chemotherapy (Zhu et al., 2018), allo-HSCT recipients with neutropenia presented with a similar pathogen spectrum, with bacteria accounting for 57.4%. Viral infection was observed in all phases after allo-HSCT, but was the dominant causative pathogen in the non-neutropenia phase. In our study, mNGS could detect more virus than PCR. This may be explained by the technical limitations of PCR. The use of PCR in viral detection relies on genetic sequences of known pathogens and high pathogens loads. There are difficulties in detecting viruses with a copy number of less than 103 copies. However, due to the high sensitivity of mNGS, it is easy to identify non-causative viruses that did not require clinical intervention. CMV infection is a serious complication after allo-HSCT. In our study, CMV reactivation was observed throughout the course of allo-HSCT. Previous studies have reported that CMV reactivation incidence ranges from 18% to 85%, with a median onset time of 32 to 41 days after allo-HSCT (Chang et al., 2020; Shen et al., 2022). It was documented that CMV reactivation after allo-HSCT was initiated approximately two weeks after engraftment (Talaya et al., 2020). Our results showed that clinically significant CMV infection was initiated before engraftment (< 12 days) in four cases. However, the incidence of clinically significant CMV infection peaked at 30 to 60 days.
Patients with neutropenia or those undergoing allo-HSCT are at high risk of fungi infection (Chien et al., 2019; Busca et al., 2021). In the present study, the fungi detection rate was 11.6%, which was lower than the 14.9% reported in another study (Qu et al., 2022). This difference may be due to the general prophylactic and pre-emptive therapy during allo-HSCT. It is worth noting that the mNGS test had higher sensibility than CMT on fungi-detection. Histopathological analysis is rarely performed in allo-HSCT recipients because of its invasive nature. In our study, nearly all patients (92.8%) were diagnosed with Aspergillosis using mNGS (including peripheral blood and BALF). Traditionally, Aspergillus cannot grow in peripheral blood, but its hyphae penetrate the air and blood barrier, thus eroding capillary endothelial cells and invading small arteries (Duan et al., 2021). Seven patients (7/14, 50%) were diagnosed with aspergillosis via mNGS with peripheral blood, six in cohort A (the neutropenic stage) and one in cohort B (non-neutropenic stage). P. jirovecii in BALF could only be detected with mNGS. The serum G test has been reported to be used as a reference index for PJP (Tasaka et al., 2007). In our study, of the six patients with PJP who received bronchial lavage for diagnosis, five patients (83.3%) were positive with the G test in the BALF sample, and only one patient (16.7% [1/6]) had a positive G test in peripheral blood.
This study has certain limitations. First, the sample size was small, and further studies with a larger cohort of allo-HSCT recipients are warranted. Second, the mNGS results could be influenced by many factors. In allo-HSCT procedures, fungi prophylaxis is generally performed for up to 3-6 months after transplantation. Prophylaxis could alter the atlas of causative pathogens detected by mNGS. Last, as we mentioned, in the non-neutropenia group, we were more predisposed to use non-blood samples for mNGS, which conferred a higher specificity than blood samples. Immune events were unique to allo-HSCT recipients. Whether mNGS would be used as a negative reference for immune events require further evaluation in future studies.
In summary, our study demonstrated that mNGS holds great potential for detecting causative pathogens of suspected infection and optimizing antibiotic treatment, through comparing the clinical efficacy of mNGS and CMT in allo-HSCT recipients. Further, we analyzed the differences on causative pathogen spectrum in different phases. Similar to the pathogen spectrum in patients with neutropenia after intensive chemotherapy, bacteria were most frequently detected in the neutropenic stage, while viruses were the dominant pathogens in the non-neutropenic stage. Immune events were unique for allo-HSCT recipients, with haplo-fever being commonly observed in the pre-engraftment phase, and GVHD in the post-engraftment phase. Therefore, immune events should be considered in patients with negative mNGS results.
Data availability statement
The raw data supporting the conclusions of this article will be made available by the authors, without undue reservation.
Ethics statement
Ethical approval and written informed consent for participation was not provided for this study on human participants because this study is a non-interventional and retrospective study and all procedures complied with the tenets of the Helsinki Declaration.
Author contributions
WC, SL, YMZ, and XH designed the study, wrote and revised the manuscript. JH, YQZ, and CJ were involved in collecting, analyzing or interpreting research data and writing the manuscript. DH, ZP, ZZ, and LW analyzed research data. All authors contributed to the article and approved the submitted version.
Funding
This work was supported by the National Natural Science Foundation (82170206 to XH, 82170210 to YMZ), Project of Disciplines of Excellence (20234Z0002 to XH), Shanghai Municipal Health Commission, the National Key Research and Development Program of China (2022YFA1103500 to YMZ), Shanghai Key Laboratory of Emergency Prevention, Diagnosis and Treatment of Respiratory Infectious Diseases (20dz2261100 to WC).
Conflict of interest
The authors declare that the research was conducted in the absence of any commercial or financial relationships that could be construed as a potential conflict of interest.
Publisher’s note
All claims expressed in this article are solely those of the authors and do not necessarily represent those of their affiliated organizations, or those of the publisher, the editors and the reviewers. Any product that may be evaluated in this article, or claim that may be made by its manufacturer, is not guaranteed or endorsed by the publisher.
Supplementary material
The Supplementary Material for this article can be found online at: https://www.frontiersin.org/articles/10.3389/fcimb.2023.1251509/full#supplementary-material
References
Busca, A., Cinatti, N., Gill, J., Passera, R., Dellacasa, C. M., Giaccone, L., et al. (2021). Management of invasive fungal infections in patients undergoing allogeneic hematopoietic stem cell transplantation: the Turin experience. Front. Cell Infect. Microbiol. 11. doi: 10.3389/fcimb.2021.805514
Chang, Y. J., Wang, Y., Xu, L. P., Zhang, X. H., Chen, H., Chen, Y. H., et al. (2020). Haploidentical donor is preferred over matched sibling donor for pre-transplantation MRD positive ALL: a phase 3 genetically randomized study. J. Hematol. Oncol. 13 (1), 27. doi: 10.1186/s13045-020-00860-y
Chemaly, R. F., El Haddad, L., Winston, D. J., Rowley, S. D., Mulane, K. M., Chandrasekar, P., et al. (2020). Cytomegalovirus (CMV) cell-mediated immunity and CMV infection after allogeneic hematopoietic cell transplantation: the REACT study. Clin. Infect. Dis. 71 (9), 2365–2374. doi: 10.1093/cid/ciz1210
Chen, L., Wang, Y., Zhang, T., Li, Y., Meng, T., Liu, L., et al. (2018). Utility of posaconazole therapeutic drug monitoring and assessment of plasma concentration threshold for effective prophylaxis of invasive fungal infections: a meta-analysis with trial sequential analysis. BMC Infect. Dis. 18 (1), 155. doi: 10.1186/s12879-018-3055-3
Chien, S. H., Liu, Y. C., Liu, C. J., Ko, P. S., Wang, H. Y., Hsiao, L. T., et al. (2019). Invasive mold infections in acute leukemia patients undergoing allogeneic hematopoietic stem cell transplantation. J. Microbiol. Immunol. Infect. 52 (6), 973–982. doi: 10.1016/j.jmii.2018.09.006
Copelan, E. A., Chojecki, A., Lazarus, H. M., Avalos, B. R. (2019). Allogeneic hematopoietic cell transplantation; the current renaissance. Blood Rev. 34, 34–44. doi: 10.1016/j.blre.2018.11.001
Duan, H., Li, X., Mei, A., Li, P., Liu, Y., Li, X., et al. (2021). The diagnostic value of metagenomic next rectanglegeneration sequencing in infectious diseases. BMC Infect. Dis. 21 (1), 62. doi: 10.1186/s12879-020-05746-5
Gagelmann, N., Kroger, N. (2021). Dose intensity for conditioning in allogeneic hematopoietic cell transplantation: can we recommend “when and for whom” in 2021? Haematologica 106 (7), 1794–1804. doi: 10.3324/haematol.2020.268839
Ghobadi, A., Milton, D. R., Gowda, L., Rondon, G., Chemaly, R. F., Hamdi, A., et al. (2019). HLA-DP mismatch and CMV reactivation increase risk of aGVHD independently in recipients of allogeneic stem cell transplant. Curr. Res. Transl. Med. 67 (2), 51–55. doi: 10.1016/j.retram.2019.01.001
Henze, L., Buhl, C., Sandherr, M., Cornely, O. A., Heinz, W. J., Khodamoradi, Y., et al. (2022). Management of herpesvirus reactivations in patients with solid tumours and hematologic Malignancies: update of the Guidelines of the Infectious Diseases Working Party (AGIHO) of the German Society for Hematology and Medical Oncology (DGHO) on herpes simplex virus type 1, herpes simplex virus type 2, and varicella zoster virus. Ann. Hematol. 101 (3), 491–511. doi: 10.1007/s00277-021-04746-y
Huang, J., Jiang, E., Yang, D., Wei, J., Zhao, M., Feng, J., et al. (2020). Metagenomic next-generation sequencing versus traditional pathogen detection in the diagnosis of peripheral pulmonary infectious lesions. Infect. Drug Resist. 13, 567–576. doi: 10.2147/IDR.S235182
Khosla, J., Yeh, A. C., Spitzer, T. R., Dey, B. R. (2018). Hematopoietic stem cell transplant-associated thrombotic microangiopathy: current paradigm and novel therapies. Bone Marrow Transpl. 53 (2), 129–137. doi: 10.1038/bmt.2017.207
Liu, W., Fan, Z., Zhang, Y., Huang, F., Xu, N., Xuan, L., et al. (2021). Metagenomic next-generation sequencing for identifying pathogens in central nervous system complications after allogeneic hematopoietic stem cell transplantation. Bone Marrow Transpl. 56 (8), 1978–1983. doi: 10.1038/s41409-021-01243-8
Ljungman, P., Boeckh, M., Hirsch, H. H., Josephson, F., Lundgren, J., Nichols, G., et al. (2017). Definitions of cytomegalovirus infection and disease in transplant patients for use in clinical trials. Clin. Infect. Dis. 64 (1), 87–91. doi: 10.1093/cid/ciw668
Ljungman, P., de la Camara, R., Robin, C., Crocchiolo, R., Einsele, H., Hill, J. A., et al. (2019). Guidelines for the management of cytomegalovirus infection in patients with haematological Malignancies and after stem cell transplantation from the 2017 European Conference on Infections in Leukaemia (ECIL 7). Lancet Infect. Dis. 19 (8), e260–e272. doi: 10.1016/s1473-3099(19)30107-0
Lu, R., Zhao, X., Li, J., Niu, P., Yang, B., Wu, H., et al. (2020). Genomic characterisation and epidemiology of 2019 novel coronavirus: implications for virus origins and receptor binding. Lancet 395 (10224), 565–574. doi: 10.1016/S0140-6736(20)30251-8
Maertens, J. A., Girmenia, C., Brüggemann, R. J., Duarte, R. F., Kibbler, C. C., Ljungman, P., et al (2018). European guidelines for primary antifungal prophylaxis in adult haematology patients: summary of the updated recommendations from the European Conference on Infections in Leukaemia. J. Antimicrob. Chemother. 73 (12), 3221–3230. doi: 10.1093/jac/dky286
Martinez-Cibrian, N., Zeiser, R., Perez-Simon, J. A. (2021). Graft-versus-host disease prophylaxis: Pathophysiology-based review on current approaches and future directions. Blood Rev. 48, 100792. doi: 10.1016/j.blre.2020.100792
Maschmeyer, G., Helweg-Larsen, J., Pagano, L., Robin, C., Cordonnier, C., Schellongowski, P. (2016). ECIL guidelines for treatment of Pneumocystis jirovecii pneumonia in non-HIV-infected haematology patients. J. Antimicrob. Chemother. 71 (9), 2405–2413. doi: 10.1093/jac/dkw158
Miao, Q., Ma, Y., Wang, Q., Pan, J., Zhang, Y., Jin, W., et al. (2018). Microbiological diagnostic performance of metagenomic next-generation sequencing when applied to clinical practice. Clin. Infect. Dis. 67 (suppl_2), S231–S240. doi: 10.1093/cid/ciy693
Neofytos, D. (2019). Antimicrobial prophylaxis and preemptive approaches for the prevention of infections in the stem cell transplant recipient, with analogies to the hematologic malignancy patient. Infect. Dis. Clin. North Am. 33 (2), 361–380. doi: 10.1016/j.idc.2019.02.002
Pendleton, K. M., Erb-Downward, J. R., Bao, Y., Branton, W. R., Falkowski, N. R., Newton, D. W., et al. (2017). Rapid pathogen identification in bacterial pneumonia using real-time metagenomics. Am. J. Respir. Crit. Care Med. 196 (12), 1610–1612. doi: 10.1164/rccm.201703-0537LE
Qu, Y., Ding, W., Liu, S., Wang, X., Wang, P., Liu, H., et al. (2022). Metagenomic next-generation sequencing vs. Traditional pathogen detection in the diagnosis of infection after allogeneic hematopoietic stem cell transplantation in children. Front. Microbiol. 13. doi: 10.3389/fmicb.2022.868160
Sahin, U., Toprak, S. K., Atilla, P. A., Atilla, E., Demirer, T. (2016). An overview of infectious complications after allogeneic hematopoietic stem cell transplantation. J. Infect. Chemother. 22 (8), 505–514. doi: 10.1016/j.jiac.2016.05.006
Shen, M. Z., Hong, S. D., Wang, J., Zhang, X. H., Xu, L. P., Wang, Y., et al. (2022). A predicted model for refractory/recurrent cytomegalovirus infection in acute leukemia patients after haploidentical hematopoietic stem cell transplantation. Front. Cell Infect. Microbiol. 12. doi: 10.3389/fcimb.2022.862526
Talaya, A., Gimenez, E., Pascual, M. J., Gago, B., Pinana, J. L., Hernandez-Boluda, J. C., et al. (2020). An investigation of the utility of plasma Cytomegalovirus (CMV) microRNA detection to predict CMV DNAemia in allogeneic hematopoietic stem cell transplant recipients. Med. Microbiol. Immunol. 209 (1), 15–21. doi: 10.1007/s00430-019-00632-7
Tasaka, S., Hasegawa, N., Kobayashi, S., Yamada, W., Nishimura, T., Takeuchi, T., et al. (2007). Serum indicators for the diagnosis of pneumocystis pneumonia. Chest 131 (4), 1173–1180. doi: 10.1378/chest.06-1467
Wang, S., Ai, J., Cui, P., Zhu, Y., Wu, H., Zhang, W. (2020). Diagnostic value and clinical application of next-generation sequencing for infections in immunosuppressed patients with corticosteroid therapy. Ann. Transl. Med. 8 (5), 227. doi: 10.21037/atm.2020.01.30
Xu, C., Chen, X., Zhu, G., Yi, H., Chen, S., Yu, Y., et al. (2023). Utility of plasma cell-free DNA next-generation sequencing for diagnosis of infectious diseases in patients with hematological disorders. J. Infect. 86 (1), 14–23. doi: 10.1016/j.jinf.2022.11.020
Zanella, M. C., Cordey, S., Laubscher, F., Docquier, M., Vieille, G., Van Delden, C., et al. (2021). Unmasking viral sequences by metagenomic next-generation sequencing in adult human blood samples during steroid-refractory/dependent graft-versus-host disease. Microbiome 9 (1), 28. doi: 10.1186/s40168-020-00953-3
Zeiser, R., Blazar, B. R. (2017). Acute graft-versus-host disease - biologic process, prevention, and therapy. N Engl. J. Med. 377 (22), 2167–2179. doi: 10.1056/NEJMra1609337
Keywords: metagenomic next-generation sequencing, conventional microbiological tests, diagnostic efficiency, clinical infection, immune events
Citation: Huang J, Zhao Y, Jiang C, Han D, Pan Z, Zhang Z, Wang L, Chen W, Li S, Zhao Y and Hu X (2023) Diagnostic efficiency of metagenomic next-generation sequencing for suspected infection in allogeneic hematopoietic stem cell transplantation recipients. Front. Cell. Infect. Microbiol. 13:1251509. doi: 10.3389/fcimb.2023.1251509
Received: 01 July 2023; Accepted: 28 August 2023;
Published: 13 September 2023.
Edited by:
Xin Zhou, Stanford University, United StatesReviewed by:
Donglin Yang, Chinese Academy of Medical Sciences and Peking Union Medical College, ChinaRen Lin, Southern Medical University, China
Copyright © 2023 Huang, Zhao, Jiang, Han, Pan, Zhang, Wang, Chen, Li, Zhao and Hu. This is an open-access article distributed under the terms of the Creative Commons Attribution License (CC BY). The use, distribution or reproduction in other forums is permitted, provided the original author(s) and the copyright owner(s) are credited and that the original publication in this journal is cited, in accordance with accepted academic practice. No use, distribution or reproduction is permitted which does not comply with these terms.
*Correspondence: Wei Chen, Y3cxMTI0MkByamguY29tLmNu; Su Li, TGlzdWFiY2RAMTI2LmNvbQ==; Yanmin Zhao, eWFubWluemhhb0B6anUuZWR1LmNu; Xiaoxia Hu, aHVfeGlhb3hpYUAxMjYuY29t
†These authors have contributed equally to this work and share first authorship