- 1INRAE, Université de Tours, UMR ISP, Nouzilly, France
- 2INRAE, UE PFIE, Nouzilly, France
- 3INRAE, Oniris, PAnTher, APEX, Nantes, France
- 4Laboratoire IHP VETO, Nantes, France
Introduction: Coccidiosis, a disease caused by intestinal apicomplexan parasites Eimeria, is a threat to poultry production. Eimeria tenella is one of the most pathogenic species, frequently causing a high prevalence of opportunistic infections.
Objective: The objective of this study is to investigate the role of the microbiota in the pathogenesis of severe Eimeria tenella infection.
Methods: We have previously shown that microbiota can promote parasite development. To study the effect of the microbiota on the pathogenesis of this infection, we used an experimental condition (inoculum of 10 000 oocysts E. tenella INRAE) in which the parasite load is similar between germ-free and conventional broilers at 7 days post-infection (pi). Thirteen conventional and 24 germ-free chickens were infected. Among this latter group, 12 remained germ-free and 12 received a microbiota from conventional healthy chickens at 4 days pi. Caeca and spleens were collected at 7 days pi.
Results: Our results demonstrated caecal lesions and epithelium damage in conventional chickens at 7 days pi but not in germ-free infected chickens. Administration of conventional microbiota to germ-free chickens partially restored these deleterious effects. At day 7 pi, both infected conventional and germ-free chickens exhibited increased gene expression of inflammatory mediators, including IL15, IFNγ, TNFα and the anti-inflammatory mediator SOCS1, whereas the inflammatory mediators CXCLi2, CCL20, IL18, CSF1, NOS2, PTGS2, IL1β, IL6, the receptor CCR2, and the anti-inflammatory mediators TGFβ1 and IL10 were upregulated only in infected conventional chickens. Notably, the IL18, PTGS2 gene expression was significantly higher in the infected conventional group. Overall, the inflammatory response enhanced by the microbiota might be in part responsible for higher lesion scores. Epithelial tight junction protein gene expression analysis revealed a significant upregulation of CLDN1 with the infection and microbiota, indicating a potential loss of the intestinal barrier integrity.
Conclusion: These observations imply that, during E. tenella infection, the caecal microbiota could trigger an acute inflammatory response, resulting in a loss of intestinal integrity. Increase in bacterial translocation can then lead to the likelihood of opportunistic infections. Hence, modulating the microbiota may offer a promising strategy for improving poultry gut health and limiting caecal coccidiosis.
Introduction
Coccidiosis is a disease caused by Eimeria, a type of apicomplexan parasite that colonizes the intestine of various animals. In poultry, Eimeria tenella (E. tenella) is considered one of the most pathogenic species, with high doses of infection leading to the loss of performances, including body weight, feed intake, feed conversion ratio, hemorrhagic diarrhea, and mortality in severe cases. Prophylaxis of Eimeria infection is costly and results in significant economic losses of approximately $13 billion per year worldwide in the poultry industry (Blake et al., 2020).
E. tenella proliferates in caecal epithelial cells and causes acute inflammation characterized by a local increase in several immune cells, such as T lymphocytes, natural killer cells, and macrophages (Lillehoj and Trout, 1996). Studies have found that various cytokines and chemokines, including interleukin (IL)1β, IL2, IL4, IL6, IL8, IL10, IL12, IL15, IL17, and IL18, interferon (IFN)γ, transforming growth factor beta (TGFβ)1–4, tumor necrosis factor alpha (TNFα), and TNF superfamily 15 (TNFSF15) and lipopolysaccharide-induced TNFα factor (LITAF) are increased in caecal tissues during coccidiosis infection (Lillehoj and Trout, 1996; Laurent et al., 2001; Hong et al., 2006; Kim et al., 2019).
The intestinal epithelium acts as a natural barrier to prevent pathogens from entering and spreading throughout the organism. However, Eimeria infection damages the epithelium (Witlock et al., 1975), potentially predisposing the animal to opportunistic entry of pathogens. Coccidiosis has been shown to be a factor for necrotic enteritis, which is associated with the presence of the pathogenic bacterium Clostridium perfringens (Helmboldt and Bryant, 1971; Van Immerseel et al., 2004). Studies focusing on intestinal integrity in coccidiosis have found an increase in intestinal permeability and an upregulation of the tight junction proteins, claudin-1 (CLDN1), and junctional adhesion molecule-2 (JAM2) in a mixed-species Eimeria infection (Teng et al., 2020). In E. tenella infection, an increase in intestinal permeability at day 5 post-infection (pi) associated with an increase in CLDN1 and claudin-2 (CLDN2) and a decrease in occludin (OCLN), E-cadherin and tight junction protein-1 (Pham et al., 2021), reflects an alteration in the integrity of the intestinal barrier, potentially favoring secondary infections by opportunistic pathogens.
E. tenella develops in the caeca which is part of the intestine the richest in bacterial abundance and diversity. As a result of E. tenella infection, alterations in the microbiota diversity and composition are observed (Wei et al., 2013; Oakley et al., 2014; Qi et al., 2019), with an increase in Enterobacteriaceae (Proteobacteria), while non-pathogenic bacteria Lactobacillus and Faecalibacterium (Firmicutes) are decreased (Macdonald et al., 2017; Huang et al., 2018a). Moreover, during E. tenella infection, Clostridium is found to be increased (Macdonald et al., 2017; Huang et al., 2018a; Huang et al., 2018b). These changes in the microbiota composition and damage to the epithelium may potentially contribute to the development of opportunistic infections.
In a previous study using a recently developed model of germ-free fast-growing chickens (Guitton et al., 2020), we showed that the microbiota plays a role in the development of the parasite E. tenella (Gaboriaud et al., 2020). In the present study, we aimed to study the influence of the microbiota on the physiopathology of E. tenella infection by increasing the doses of inoculum to the level where the parasite load was similar in both germ-free and conventional chickens. The results suggest a critical role of the microbiota in promoting the acute inflammatory response and loss of intestinal barrier integrity in E. tenella infection, predisposing the animal to opportunistic infections through potential bacterial translocation. Future strategies involving the modulation of the composition of the microbiota may improve coccidiosis prophylaxis.
Results
Microbiota plays a major role in the genesis of caecal lesions associated with severe E. tenella infection
To study the intricate interplay between the microbiota and E. tenella pathophysiology, we infected ROSS PM3 fast-growing chickens, both germ-free and conventional, with E. tenella. Our previous findings revealed that the microbiota significantly influences parasite development and is instrumental in generating caecal lesions. However, to evaluate the contribution of the microbiota on the formation of lesions, we needed to insure similar parasite development in both conventional and germ-free chickens. Following our previous studies (Gaboriaud et al., 2020), we opted for a high inoculum dose of 10 000 oocysts to achieve a similar parasite load at 7 days pi, as evidenced by oocyst counts in the caecal contents of conventional, germ-free, and germ-free chickens supplemented by conventional microbiota (Supplementary Figure 1). At day 7 pi, we observed lesions, as described by (Johnson and Reid, 1970). Notably, under these experimental conditions, germ-free chickens had significantly fewer macroscopic lesions than their conventional counterparts. Furthermore, the addition of microbiota from healthy conventional chickens to germ-free chickens at day 4 pi induced the appearance of macroscopic lesions by day 7 pi. These observations suggest that the microbiota contributes to lesion development (Figure 1A) regardless of parasite development. Additionally, increasing the inoculum dose to a very high 50 000 oocysts in germ-free chickens resulted in some lesions reaching score 3 in 25% of the animals. Still, the score levels did not match those of conventional chickens receiving 10 000 oocysts (Figure 1B). Our results conclusively indicate that microbiota play a critical role in the generation of lesions during E. tenella infection.
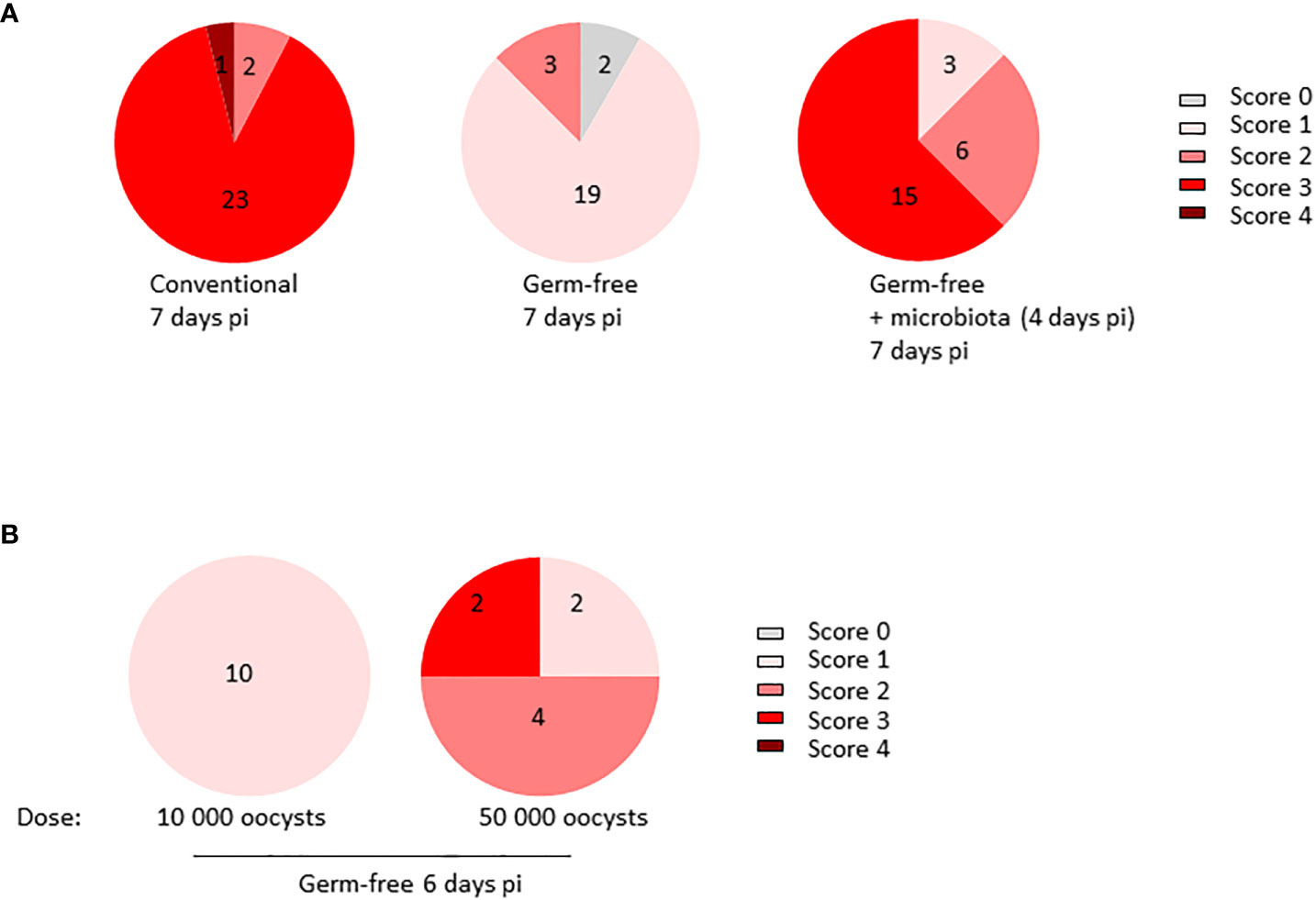
Figure 1 Microbiota plays a major role in the generation of caecal lesions generated by E. tenella infection. (A) Conventional and germ-free chickens (n ≥ 12/group) were orally infected with E. tenella with a fixed dose of inoculum (10 000 oocysts/animal) leading to similar parasite load in germ-free and conventional chickens. At day 4 pi, a part (12 chickens) of infected germ-free chickens received a microbiota from conventional and healthy three-week-old chickens. Lesions were assessed at day 7 pi. Statistical analysis was performed on lesion scores using a Kruskall-Wallis test with a Dunn’s multiple comparisons post-test. Conventional versus germ-free: ****P < 0.0001; germ-free versus germ-free + microbiota: ****P < 0.0001; conventional versus germ-free + microbiota: ns: non significant (B) Germ-free chickens were infected for 6 days with a high dose of inoculum (10 000 or 50 000 oocysts/animal; n ≥ 4). Lesion scores were evaluated by the method described by (Johnson and Reid, 1970) and assessed on each caecum (two per animal). Statistical analysis was performed on lesion scores using Mann and Whitney test. **P < 0.01.
Presence of microbiota leads to caecal lesions during E. tenella infection and favours mucosal damage
The presence of microbiota has been shown to induce caecal lesions in the course of E. tenella infection and therefore exacerbate mucosal damage. Histological analysis of caecal tissues from infected conventional chickens revealed extensive mucosal alterations ranging from epithelial erosions to ulcers. Conversely, germ-free chickens exhibited fewer and less severe epithelial alterations, primarily erosions. Moreover, introduction of microbiota at day 4 pi led to increased mucosal damage in germ-free chickens, as evidenced by the presence of erosions and/or ulcers, thus confirming its contributory role in the pathology (Figures 2A, B). Notably, infected conventional chickens displayed thickened mucosa compared to their non-infected counterparts, and at day 7 pi, mucosal thickness increased in both conventional and germ-free chickens to varying extents likely due to more or less severe inflammatory cell infiltration. The addition of microbiota at day 4 pi appeared to promote cellular infiltration (Figures 2C, 3) and/or local cellular proliferation most probably due to inflammation, ultimately resulting in a partial increase in mucosal thickness in germ-free chickens receiving microbiota comparable to that observed in conventional chickens.
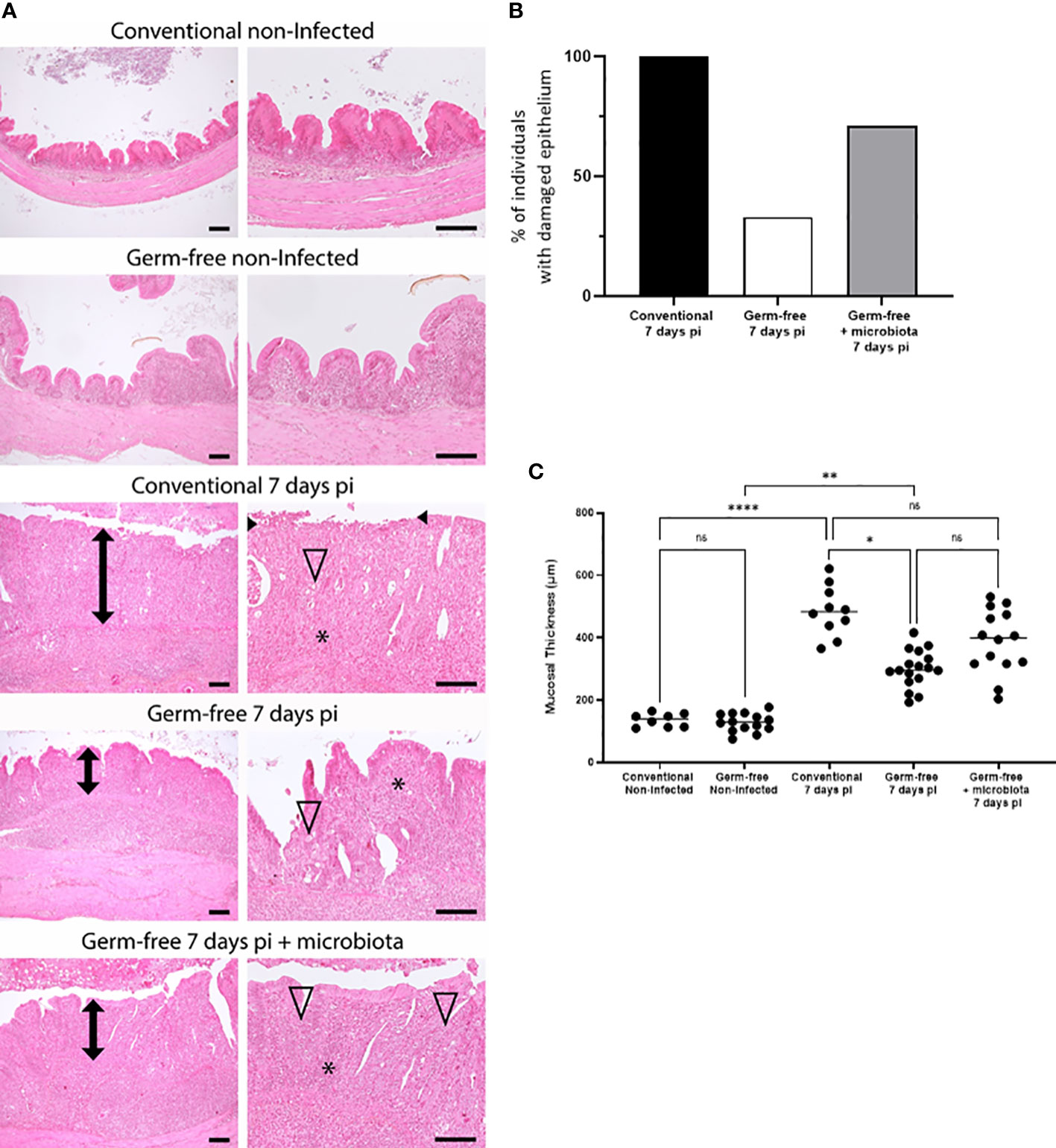
Figure 2 Microbiota favours mucosal damages and increased thickness associated with E. tenella infection. (A) Mucosal damage was less frequently observed in germ-free E. tenella infected chickens compared to conventional infected chickens. Representative histopathological pictures of caeca. Compared to control non-infected animals, infected conventional, germ-free, and germ-free receiving intestinal microbiota animals displayed respectively a severe (ulcerated epithelium indicated into black arrowhead), mild, and marked mucosa thickening (double black arrow). Thickening of the mucosa and submucosa was mainly composed of severe mixed inflammatory cell infiltration (*). E. tenella (open arrowhead) were mainly observed within epithelial cells of the mucosa. Hemalun-Eosin-Saffran. Bar=100µm. (B) Evaluation of the percentage of animals with epithelium damage (black arrow head) for each infected group. (C) Measure of the mucosal thickness (double black arrow) was performed for each animal and are expressed as the median (n ≥ 6/group). Statistical analysis was performed using a Kruskall-Wallis test with a Dunn’s multiple comparisons post-test. *P < 0.05; **P < 0.01; ****P < 0.0001; ns: non significant.
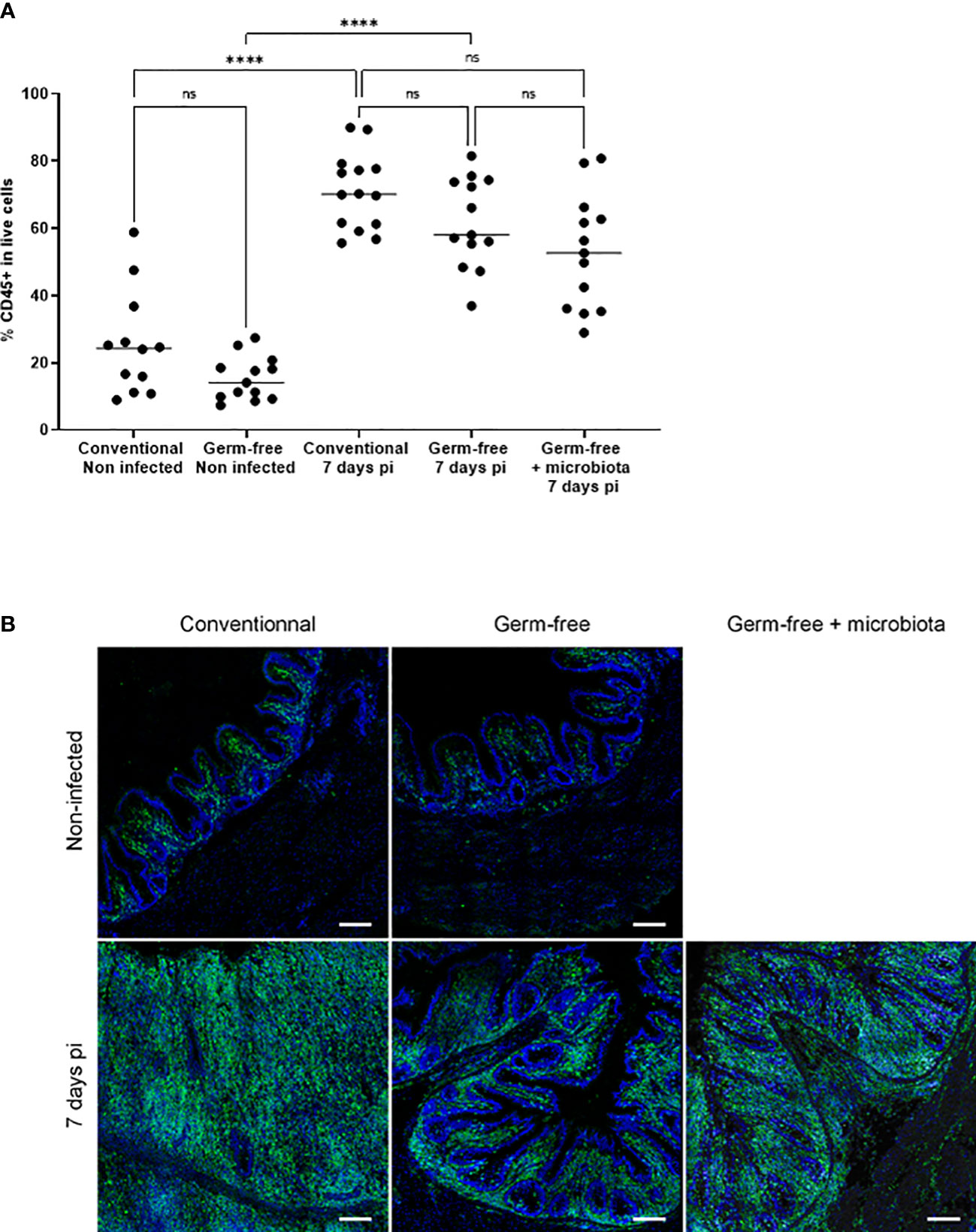
Figure 3 Increase in the percentage of CD45 positive cells in the caecal tissues of infected chickens both germ-free and conventional animals compared to non-infected chickens. Caeca were collected at 7 days pi. Cells were isolated, stained with anti-CD45 antibody, assessed for cell viability (ZombieAqua™) and analysed by flow cytometry (A) as described in the materials and methods section. Data are expressed as the median (n ≥ 5/group for at least 2 experiments). Statistical analysis was performed using a Kruskall-Wallis test with a Dunn’s multiple comparisons post-test. ****P < 0.0001; ns: non significant. (B) Representative image of CD45+ cells recruitment in E. tenella infected conventional and germ-free chickens compared to non-infected chickens (SP8 confocal laser-scanning Leica microscope; magnification 63X; image: 2048×2048 pixels, Z stacks and mosaic merge; Leica Application Suite X software).
Inflammatory response in the E. tenella infected caeca is strongly enhanced by the presence of microbiota
The cell infiltration observed in the caeca of E. tenella infected animals was significantly amplified by the presence of microbiota. Through flow cytometry, we identified CD45+ cells and found a similar increase in the percentage of total leukocytes in the mucosa of infected conventional and germ-free animals compared to non-infected animals (Figure 3A). However, CD45 staining of caecal tissues showed a higher number of total leukocytes in conventional chickens compared to germ-free chickens (Figure 3B) likely due to an increased thickness of the caecal mucosa. Gene expression analysis demonstrated that the response to infection differed between conventional and germ-free chickens, with the latter overlapping both groups when supplemented with microbiota (Figure 4A); this was confirmed by a PERMANOVA test. Some mediators, such as IL15, IFNγ, Suppressor of Cytokine Signaling protein 1 (SOCS1), and TNFα were increased in response to E. tenella in both germ-free and conventional infected chickens, while others, including IL18, Prostaglandin-Endoperoxide Synthase 2 (PTGS2), and in a lesser extent IL1β, were specifically increased in conventional infected chickens. Additionally, chemokines (CCL20, CXCLi2), colony stimulating factor 1 (CSF1), Nitric Oxide Synthase 2 (NOS2) and IL6 were highly expressed in conventional infected chickens compared to conventional non-infected chickens; however, probably due to variability between animals, the expression of these genes is not significantly different between conventional infected and germ-free infected chickens (Figures 4B, 5). Overall, our results suggest that the presence of microbiota modulates the nature of inflammatory mediators in response to E. tenella infection.
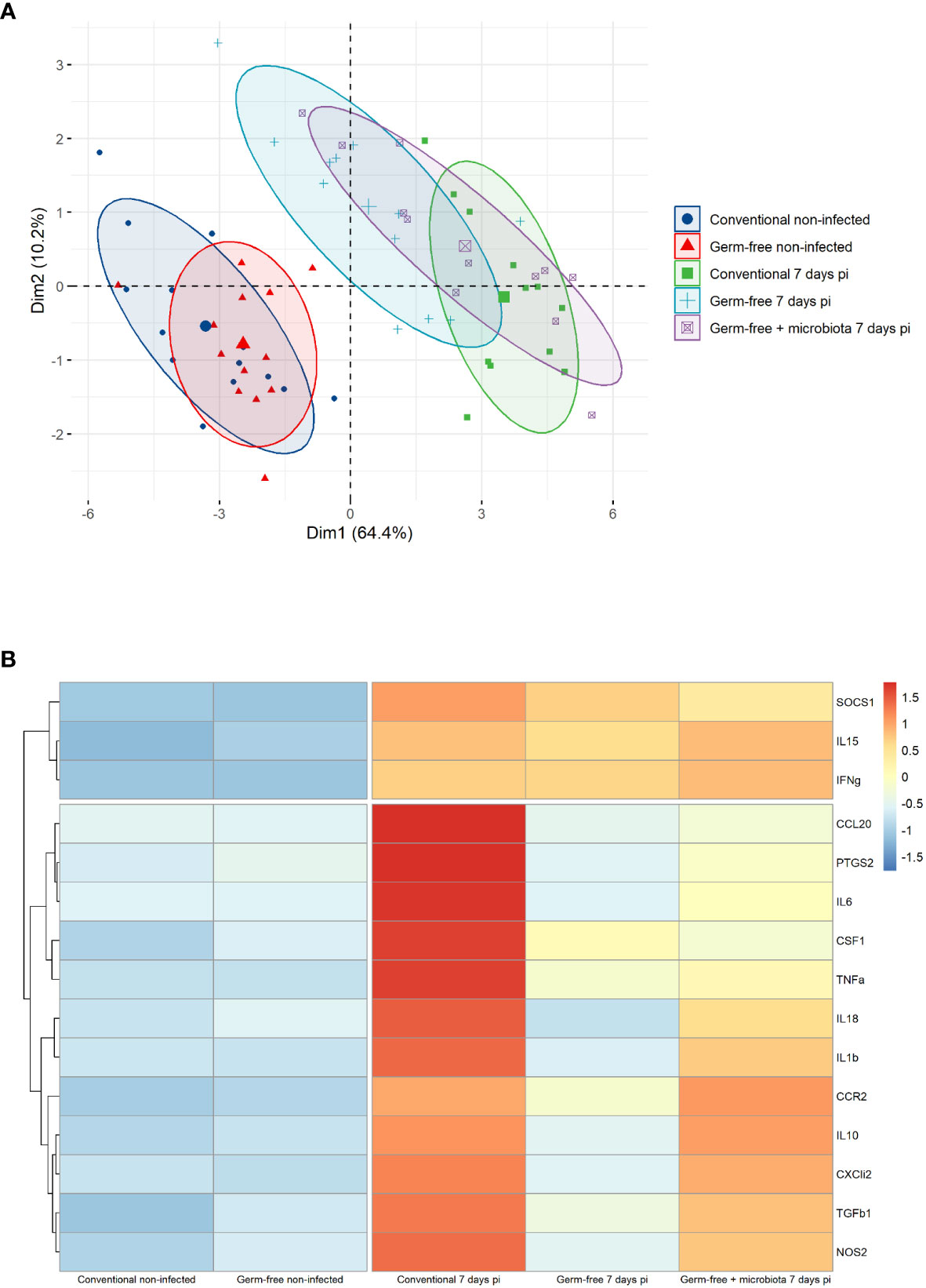
Figure 4 Genes associated to inflammation in germ-free infected chickens are different than the one in conventional infected chickens. (A) Principal component analysis (PCA) of RT-qPCR gene expression values from caeca of infected and non-infected chickens following presence or absence of microbiota. Each chicken represented as a dot in specific colour according to its group assignment. Dim 1 (dimension 1) explained 64.4 of the total data variation between animals and Dim 2 (dimension 2) a further 10.2 of the variation. Barycenters for each group are represented in large dots compared to individual values. PERMANOVA test confirmed a significant difference between non-infected and infected chicken clusters. No dissimilarity was observed in conventional and germ-free non-infected groups. At 7 days pi conventional and germ-free clusters were distinct. However germ-free + microbiota cluster was not different from conventional and germ-free infected chickens. (B) Z-score hierarchical clustering heatmap depending of median genes expression. Colours represent scaled median values of gene expression with blue for low and red for high values.
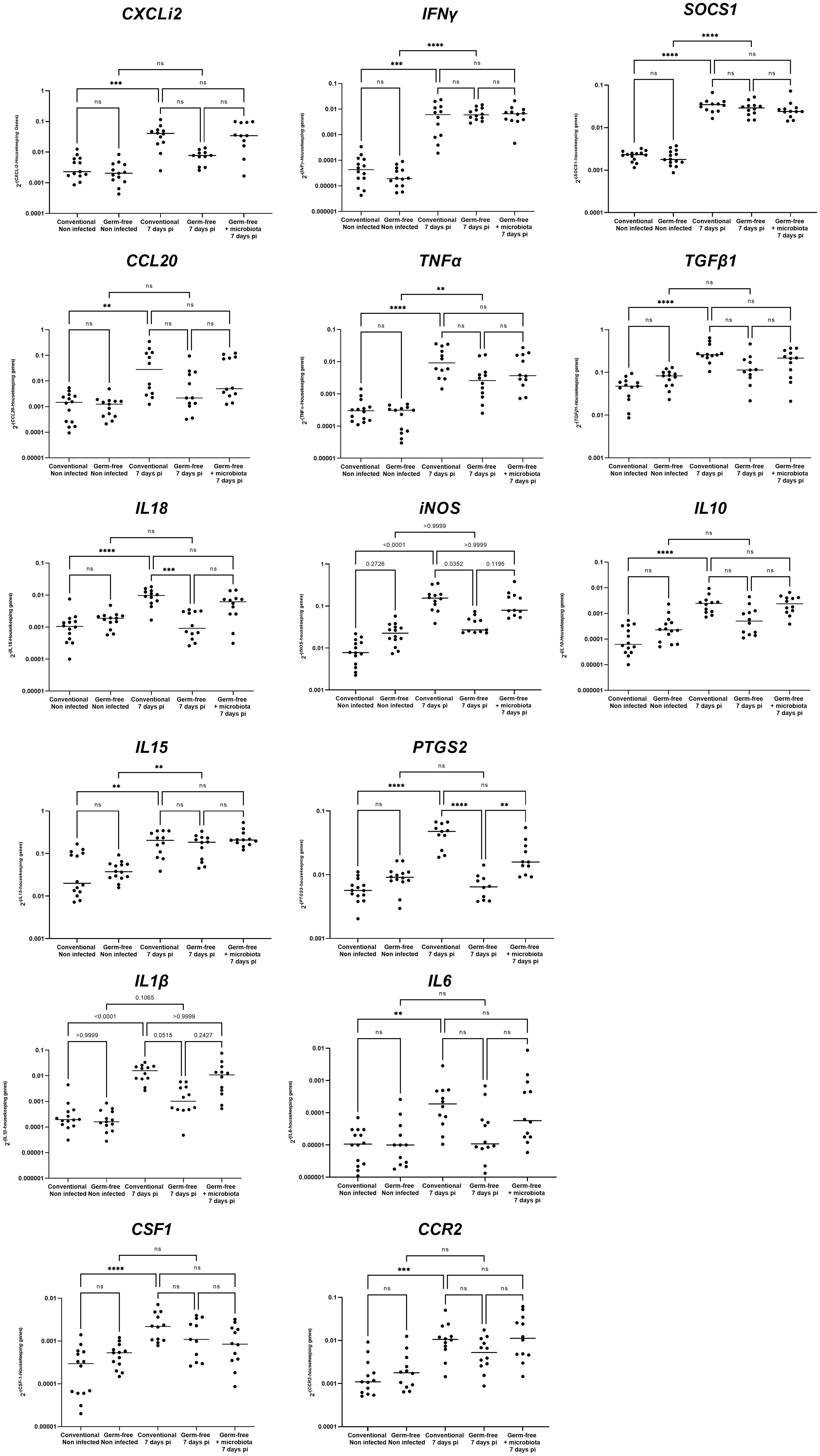
Figure 5 Detailed gene expression assessed by RT-qPCR. Data are expressed as the median. (n ≥ 10/group). Statistical analysis was performed using a Kruskall-Wallis test with a Dunn’s multiple comparisons post-test. **P < 0.01 *** P < 0.001 ****P < 0.0001; ns: non significant.
The expression of the tight junction protein CLDN1 is enhanced by the presence of microbiota in caeca of E. tenella infected chickens
The presence of microbiota in the caeca of E. tenella infected chickens has been shown to enhance the expression of CLDN1, a tight junction protein at 5- and 6-days pi (Pham et al., 2021). E. tenella infection disrupts the epithelial layer and can cause changes in the expression of tight junction proteins, which are typically regulated by inflammatory cytokines (Andrews et al., 2018). In this study, at day 7 pi, we found that conventional infected chickens had a significant increase in CLDN1 gene expression compared to conventional non-infected and germ-free infected chickens. Furthermore, the expression of other tight junction protein, such as CLDN2 and OCLN genes, were also significantly altered by the infection in conventional chickens compared to non-infected ones. This effect, however, was not observed in germ-free infected chickens when compared to germ-free non-infected chickens (Figure 6). Although there was no significant difference between conventional and germ-free infected chickens due to high data dispersion, these changes in tight junction protein gene expression suggest that the intestinal barrier integrity may be compromised.
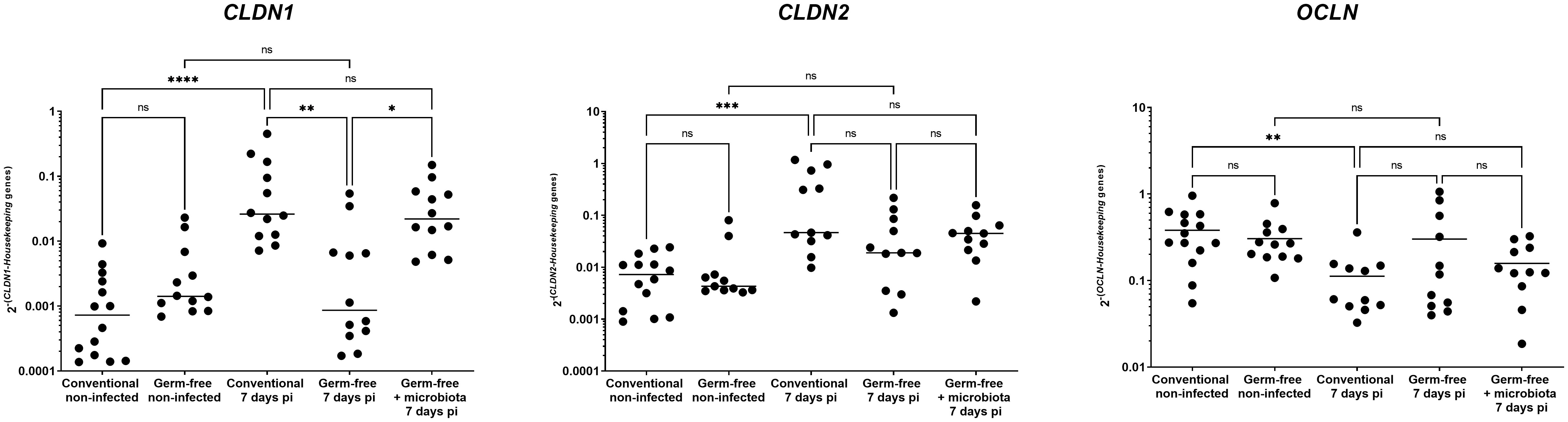
Figure 6 CLDN1 gene expression increase during infection by E. tenella is dependent on the microbiota. Gene expression was assessed by qPCR. CLDN1 gene expression was increased in conventional chickens at 7 days pi compared to germ-free infected chickens. CLDN2 gene expression was increased while OCLN gene expression was decreased in conventional chickens at 7 days pi compared to conventional non-infected chickens. However, these gene expressions were unchanged in conventional infected chickens compared to germ-free infected chickens due to high variance. Data are expressed as the median (n ≥ 10/group). Statistical analysis was performed using a Kruskall-Wallis test with a Dunn’s multiple comparisons post-test. *P < 0.05 **P < 0.01 *** P < 0.001 ****P < 0.0001; ns: non significant.
Severe E. tenella infection leads to bacteria translocation from the caeca to an extraintestinal site
As previously stated, infection with E. tenella results in conspicuous damage to the mucosal lining and substantial alterations in tight junction protein gene expression in conventionally infected chickens, as opposed to germ-free ones. This led to the hypothesis that such mucosal damage may facilitate the translocation from the caeca of commensal bacteria in conventional animals. To investigate this hypothesis, the presence of enterobacteria in the spleen was examined in different models. Interestingly, basal levels of bacterial translocation were observed in non-infected ROSS PM3 chickens, which were undetected in layers (unpublished data), hinting at leaky gut in fast-growing chickens.
Furthermore, results indicated that bacterial translocation was increased more frequently in conventionally infected chickens (4 out of 7) compared to non-infected ones (1 out of 11). Intriguingly, introducing microbiota to germ-free infected chickens four days pi partially resulted in bacterial translocation (Figure 7A) in 1 out of 7 chickens. This correlated well with intermediate epithelial damage, as depicted in histological analysis and with the change in the CLDN1 gene expression with the infection.
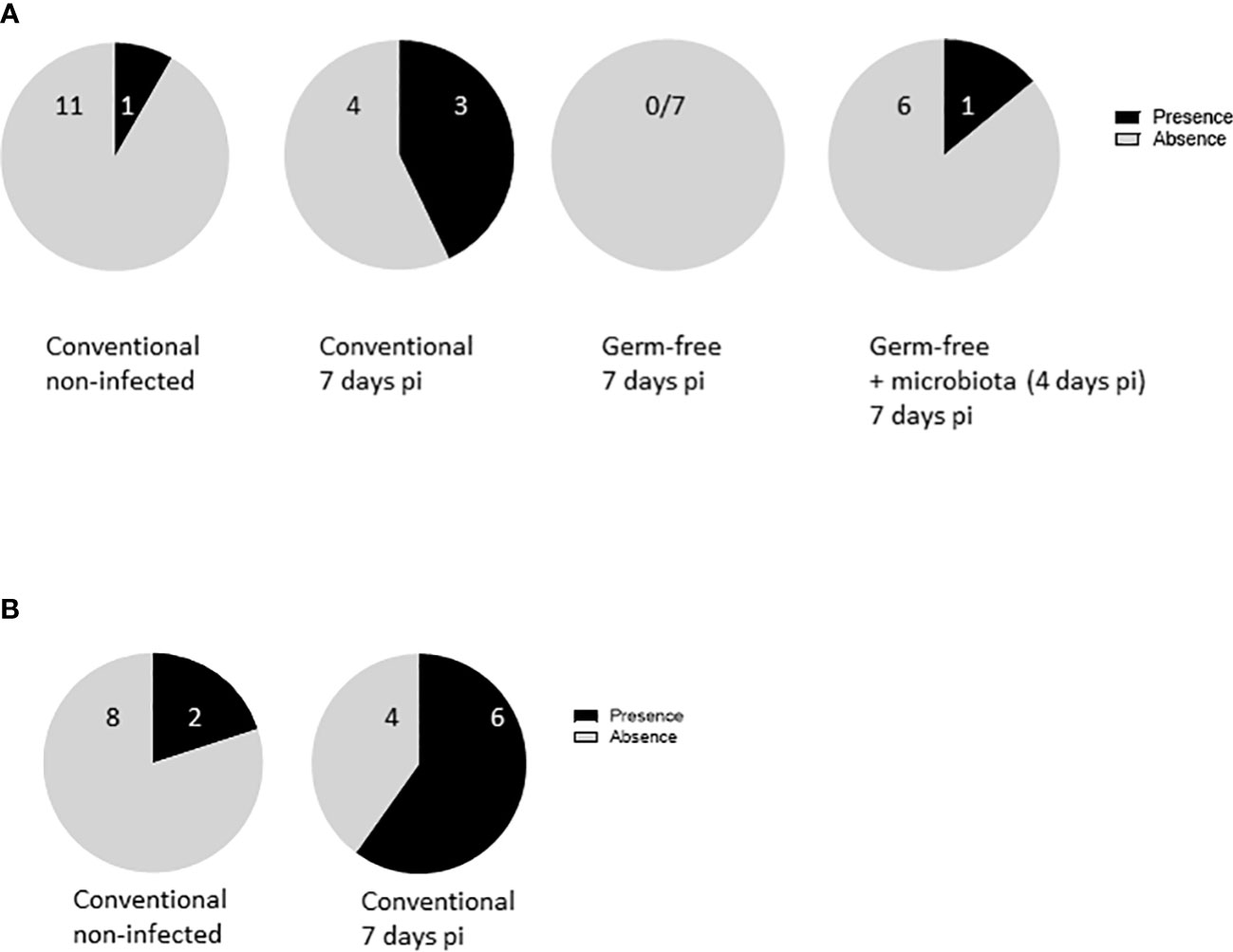
Figure 7 E. tenella infection leads to bacterial translocation. (A) Commensal enterobacteria were followed in the spleen of infected and non-infected chickens at 7 days pi (n ≥ 7/group). (B) APEC were administered to the animals at 1 day old. Chickens were infected with E. tenella at 14 days old. Translocation of bacteria in the spleen was performed at day 7 pi (n = 10). Data are expressed as the number of spleens positive or negative for bacteria.
To validate these findings, APEC was administered to chicks at 7 day old, followed by E. tenella infection at 14 day old. Spleens were collected from both infected and non-infected chickens, and bacteriological analysis was performed for the presence of APEC. After E. tenella infection, there was a conspicuous increase in APEC presence in the spleens of conventionally infected chickens (4 out of 6) compared to conventionally non-infected ones (2 out of 8), pointing to a potential bacterial translocation resulting from the infection (Figure 7B). These data support the notion that epithelial damage in conventionally infected chickens presents an opportunity for bacterial translocation, ultimately leading to an exaggerated inflammatory response, as opposed to germ-free ones (Figure 8).
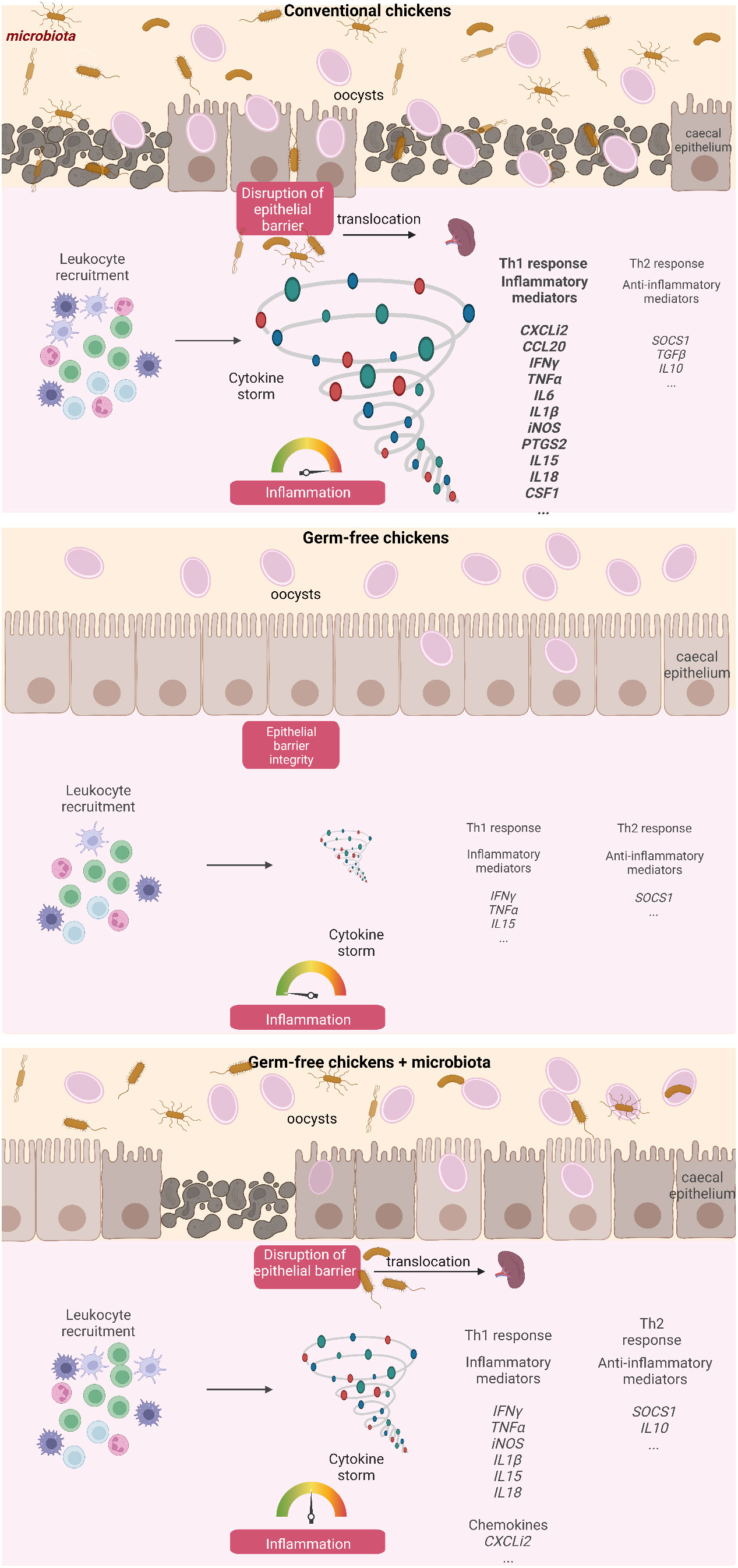
Figure 8 Summary diagram describing the influence of the caecal microbiota on the development of inflammation and the loss of intestinal integrity at day 7 pi with E. tenella. Created with BioRender.com (JV25NHUMTD, FE257PUXU1, RV257PUXXN).
Discussion
The present study has shed light on the pivotal role that the microbiota plays in the physiopathology of E. tenella infection, as evidenced by higher levels of caecal lesions and inflammation in conventional chickens compared to their germ-free counterparts. Moreover, the presence of microbiota during infection led to disruption of the epithelial layer, causing bacterial translocation and potentially resulting in opportunistic infections.
At homeostasis, the microbiota confers manifold benefits to the immune system by providing metabolites such as short chain fatty acids, which are derived from alimentary fibers. These metabolites promote the maturation of the innate immune system, especially at an early age, and foster the development of the intestinal barrier structure and function, thus shielding the host from potential pathogen entry (Hayes et al., 2018). However, when this balance is perturbed, as in the case of a severe infection, the microbiota’s role can turn deleterious for the host (Belkaid and Hand, 2014). In this study, we investigated the impact of the microbiota on the physiopathology of E. tenella infection. Conventional and germ-free chickens were then infected with E. tenella for 7 days and microbiota from conventional chickens was administered to germ-free chickens at 4 days pi. To compare the physiopathology of infected with non-infected conditions, non-infected germ-free and conventional chickens were used. In the present study, conventional microbiota was not administered to non-infected germ-free chickens for 3 days. Even tough an effect of the microbiota in non-infected germ-free animals cannot be rule out, as we observed no differences in the parameters measured in non-infected germ-free and conventional chickens, it is unlikely to observe a different response in non-infected germ-free chickens receiving a microbiota. Previous research revealed that the microbiota plays a role in parasite development, as evidenced by lower parasite loads in germ-free chickens compared to conventional chickens, despite similar invasion rates in host epithelial cells (Gaboriaud et al., 2020). In this latter study, we showed that a high dose of inoculum of 10 000 oocysts with the INRAE E tenella strain led to a similar parasite load in conventional and germ-free chickens at day 7 post-infection. In the present work, we chose this high dose of inoculum and confirmed a similar parasite load between groups. This allowed us to investigate the role of microbiota on the physiopathology of the infection independently of the parasite load, which also contributes to the pathology by inducing mechanical disruption of epithelial cells. We first assessed lesion scores based on several parameters, such as the presence of petechiae, the thickening of the caecal wall, the aspect of the caecal content (presence of blood or caseous core), distension of the caecal wall with its content, or death of the animal (Johnson and Reid, 1970). Under our experimental conditions, we observed markedly lower lesion scores in germ-free chickens, which did not reach the levels seen in conventional chickens even at a very high inoculum dose (50 000 oocysts). Additionally, the microbiota transplantation from healthy conventional chickens into germ-free chickens at 4 days pi led to a significant increase in lesions. Our findings underscore the critical role of microbiota in the formation of lesions during E. tenella infection. Undoubtedly, an elevated inoculation dose of the parasite corresponds to a greater degree of lesion scores even in germ-free chickens, indicating that not only the microbiota, but also the replication of the parasite and the ensuing inflammation associated with the infection are involved in the genesis of lesions. Interestingly, the physiopathology of the infection E. acervulina appears to be unchanged by microbiota, as germ-free chickens infected with E. acervulina demonstrate comparable clinical and biochemical parameters (namely, weight loss, serum protein and lipid changes) to the conventional ones (Lafont JP et al, 1975). Although the parasite load and the lesion scores were not determined in that study, the differences between the physiopathology of the two species could potentially be explained by the considerably higher bacterial load and diversity present in the caeca as compared to the duodenum. Furthermore, the nature of bacteria residing in these two compartments varies, with the caecal microbiota composition and complexity being greater than that of the duodenum, which mostly contains Lactobacilli. In the caeca, nearly 1000 diverse species belong to two major phyla, namely, Bacillota (former name Firmicutes) and Bacteroidota (former name Bacteroidetes), along with two minor phyla, namely, Actinomycetota) and Pseudomonadota (former name Proteobacteria) (Rychlik, 2020). Consequently, we contend that the discrepancies in microbiota composition may account for differences in the clinical symptoms between Eimeria species.
A prior histological investigation has indicated that in conventional chickens, the mucosa thickens as a result of an infiltration of inflammatory cells, including macrophages, heterophils, and lymphocytes (Lillehoj and Trout, 1996). The present study demonstrates that a thickening of the mucosa and recruitment of leukocytes occurs in infected conventional chickens, as well as to a lesser extent in germ-free infected chickens, potentially due to a lower or different inflammatory response. Supporting this observation, the gene expression profile of inflammatory and anti-inflammatory mediators shows a distinct pattern between germ-free and conventional infected chickens. In both types of infected chickens, transcription of only certain cytokines, including IL15, IFNγ, TNFα, and the anti-inflammatory mediator SOCS1, increase, indicating that inflammation also occurs in germ-free infected chickens. Similar to previous findings in conventional animals (Laurent et al., 2001; Hong et al., 2006), a significant increase in the expression of genes related to inflammation (CXCLi2, CCL20, IL18, CSF1, iNOS, PTGS2, IL1β, IL6, CCR2) and the anti-inflammatory (TGFβ1 and IL10) response is observed with E. tenella infection. However, the expression of these genes is not significantly increased in germ-free infected chickens compared to non-infected germ-free chickens. Notably, IL18, PTGS2, and, to a lesser extent, IL1β have significantly higher expressions in infected conventional compared to infected germ-free chickens. IL18 and IL1β are potent pro-inflammatory cytokines (Hua et al., 2015; Venuprasad and Theiss, 2021) while PTGS2 is induced by inflammatory stimuli such as IL1β and TNFα but also by bacterial components and is involved in the regulation of NLRP3 inflammasome (Hua et al., 2015). The activation of the inflammasome pathway can lead to the activation and release of pro-inflammatory cytokines such as IL18 and IL1β, which, in a murine model of infection by Cryptosporidium tizzeri, have been shown to promote the production of IFNγ, known to play a crucial role in parasite control (Sateriale et al., 2021). Similarly, in E. tenella infection, IFNγ has been shown to inhibit its replication (Heriveau et al., 2000). Given the known role of IFNγ in parasite control, the similarity in its expression between the two models implies that IFNγ may be similarly effective in controlling E. tenella replication in both conventional and germ-free animals. Overall, our data suggest that in E. tenella infection, the microbiota may induce the NLRP3 inflammasome, participating to the cleavage of procaspase 1 and subsequent leads to activation and release of pro-inflammatory cytokines IL1β and IL18. Thus, it is possible that in E. tenella infection in the presence of microbiota, bacterial moieties and/or metabolites may potentiate the epithelial cell response such as IL1β and IL18 production leading to an amplified inflammatory cascade in the caecal tissue with the involvement of different mediators by various type of leukocytes.
The intestinal epithelial layer protects the organism against the entry of potential pathogens and is preserved with the maintenance of junction proteins present between epithelial cells. Modification of the tight junction protein expression by inflammatory cytokines can then alter the intestinal permeability (Andrews et al., 2018). In the neonatal model of infection with the apicomplexan parasite, Cryptosporidium parvum, we showed that increased permeability was the result of both the parasite on the adherens junctions proteins E-cadherin and β-catenin and the release of inflammatory cytokines (TNFα and IL1β) by inflammatory monocytes (De Sablet et al., 2016). In the present study, in conventional chickens, severe E. tenella infection led to an acute inflammation, the disruption of the epithelial layer, an alteration of tight junction protein gene expression more particularly with an increase in CLDN1 gene expression and a higher frequency of bacterial translocation. Overall, in severe E. tenella infection and in the presence of microbiota, the acute inflammatory response may modify the expression of tight junction proteins resulting in an increase in intestinal permeability and to potential bacterial translocation.
Recent studies using mouse models have demonstrated the crucial role of commensal bacteria in host defense against various pathogenic infections caused by bacteria, fungi, and parasites. The absence of microbiota has been shown to increase susceptibility to infections caused by Listeria monocytogenes, Salmonella Typhimurium, Klebsiella pneumoniae, and Cryptococcus gatti, indicating a protective role of commensal bacteria in host defense (Nardi et al., 1989; Fagundes et al., 2012; Mittrucker et al., 2014; Costa et al., 2016). This protection may be attributed to the competition between pathogenic and commensal bacteria, limiting the growth and colonization of pathogenic bacteria. However, in some cases, the microbiota may exacerbate inflammation, tissue damage, and promote pathogen transmission. For instance, the interaction between the microbiota and Trichuris muris is necessary for parasite egg hatching and subsequent transmission (Hayes et al., 2010). In the case of Toxoplasma gondii infection, germ-free mice were found to have less inflammation and intestinal permeability than conventionally-raised mice, suggesting the contribution of the microbiota to an exaggerated inflammatory response (Nascimento et al., 2017). A similar deleterious role of Gram-positive bacteria was observed in E. falciformis, that developed also in the caecum of mice. Indeed, antibiotic administration depleting Gram-positive bacteria reduced histopathological scores and oocyst excretion (Gong et al., 2022). In addition, in a mouse model of dextran sodium sulfate-induced colitis, Gram-positive bacteria were responsible for the recruitment of phagocytes and enhanced inflammation, indicating a potential harmful role of commensal bacteria (Nakanishi et al., 2015). Further studies are needed to investigate the role of the microbiota in E. tenella infection in chickens.
The contribution of the microbiota to host defense against infection is complex and context-dependent, and further studies are needed to understand the underlying mechanisms. In the context of acute inflammation, it is not uncommon to observe a dysbiosis marked by an increase in bacterial populations that can potentially exacerbate inflammation and tissue damage. In Eimeria infection, an increase in Proteobacteria and a decrease in Firmicutes has been noted (Macdonald et al., 2017). This shift towards a dominance of Proteobacteria has been shown to be associated with more severe physiopathology due to the translocation of these bacteria, which can contribute to an exacerbation of inflammation by promoting differentiation and activation of cells towards an inflammatory phenotype (Belkaid and Hand, 2014). To address this issue, it is hypothesized that replenishing Firmicutes to levels and diversity comparable to non-infected animals or administering bacterial probiotics may help to attenuate inflammation and its adverse effects on the integrity of the intestinal barrier. Further research is needed to validate these hypotheses and identify potential molecular mechanisms underlying these effects.
In conclusion, microbiota has revealed its crucial impact in the physiopathology of E. tenella infection. The microbiota is responsible for inducing acute inflammation and extensive lesions in the caeca, leading to bacterial translocation and secondary opportunistic infections. The identification of key microbiota bacteria responsible for the pathology of coccidiosis can aid in the development of alternative strategies for managing and preventing this infection in the poultry industry. Early age administration, dietary modification, and prebiotic use could be potential methods for modulating the microbiota and improving the outcomes of coccidiosis in poultry. Therefore, further research in this field may pave the way for more effective management strategies and improved animal welfare in the poultry industry.
Materials and methods
Ethical statement
Animal experiments were approved by the French legislation (Décret: 2001‐464 29/05/01), the EEC regulation (86/609/CEE) and by the ethics committee of Centre Val de Loire (CEEA VdL n°19): 2018‐04‐26 (APAFIS N°13904).
Animals
Hatching and growth of conventional and germ-free Ross PM3 broilers were performed in the facility for Infection of Farm, Model and Wildlife Animals (PFIE, Centre INRAE Val De Loire: https://doi.org/10.15454/1.5572352821559333E12; member of the National Infrastructure EMERG’IN). Ross PM3 eggs were obtained from French farms, decontaminated with a 1.5% Divosan Plus (VT53, Johnson Diversey, France). Eggs were incubated, decontaminated a second time. Animals were hatched in an incubator for conventional chicks or an isolator for germ-free animals. The absence of bacteria was confirmed in germ-free chicks; and the development of a microbiota was present in conventional chickens (Guitton et al., 2020).
Infection
Oral infection was performed on two-week-old broilers with 10 000 sporulated oocysts PAPt36 strain (Et-INRAE). At day 7 pi, chickens were euthanized using electronarcosis. Caeca were collected and lesion scoring performed as described by (Johnson and Reid, 1970). After washing the caeca, the tissue was fixed in 4% formaldehyde (Laurypath, Chaponost, France) for histological analysis or kept on ice for flow cytometry or directly frozen in liquid nitrogen and stored at -80°C for transcriptomic analyses. Spleens were collected for further bacteriological analysis.
Bacterial translocation
For bacterial translocation study, the avian pathogenic Escherichia coli (E. coli) strain BEN2908, O2:K1:H5 ST95, a nalidixic acid-resistant derivative of strain MT78 isolated from the trachea of a chicken with a respiratory infection (Dho and Lafont, 1982) was used. This strain is able to colonize the intestine at high level even in the presence of others E. coli strains of the microbiota (Porcheron et al., 2012). An overnight culture in LB-Miller medium at 37°C with agitation was diluted in sterile apyrogenic DPBS (Sigma Aldrich) at a final concentration of 5×104 cfu/mL. Chickens were orally inoculated at one day old with 200 µl of the bacterial suspension. Intestinal colonization of BEN2908 was assessed by plating serial dilutions of feces onto Drigalski agar plates (Bio-Rad, Hercules, CA, USA) supplemented with nalidixic acid at 30 µg/ml. To assess the systemic dissemination of the APEC strain and enterobacteria at day 7 pi, animals were euthanized, spleens were collected, weighted and homogenized in 3.5 mL of sterile DPBS using gentleMACS™ Dissociator (Miltenyi Biotec, Bergisch Gladbach, Germany). Serial dilutions were plated onto Drigalski agar plates (Bio-Rad) supplemented with nalidixic acid at 30 µg/mL (selection of BEN2908) or without antibiotic (selection of enterobacteria and BEN2908) for bacterial quantification.
Cell isolation, staining and flow cytometry analysis
For analysing the percentage of leukocytes in the caeca, tissues were scraped and cells were dissociated in a DMEM-F12 medium supplemented with collagenase H (1 mg/ml, Roche Basel, Switzerland) for 30 min at 37°C under stirring. After washing and filtering, viable caecal cells were counted using Trypan blue and 2 × 106 live cells were harvested for staining. First, cells were incubated for 10 min at 4°C with PBS supplemented with 2% naïve chicken serum and 2 mM EDTA for blocking unspecific staining. Then, cells were stained with anti-CD45 antibody labelled with APC for leukocytes (SouthernBiotech, Birmingham, AL, USA) and ZombieAqua™ for assessing cell viability (BioLegend, San Diego, CA, USA) in PBS supplemented with 2% FBS and 2 mM EDTA for 30 min at 4°C. Cells were washed, fixed with fixation buffer (BD Biosciences, Franklin Lakes, NJ, USA) and filtered before flow cytometry analysis (LSR Fortessa X-20, BD Biosciences). Debris were excluded and only viable cells were selected. In this selected region, CD45-positive cells were assessed and represented as a percentage of live cells.
Histological analysis
Tissues fixed in formaldehyde (Laurypath) were embedded in paraffin wax, sliced and stained with Hemalun Eosin Saffron.
Immunofluorescence staining
Caecal tissues were directly frozen in OCT. Tissues were sliced and fixed in ice cold 50% ethanol, 50% acetone. Leukocytes were stained for 1 h at 37°C using anti-anti-CD45 Alexa Fluor 488 (SouthernBiotech). Cell nuclei were counterstained with DAPI (ThermoFisher Scientific, Waltham, MA, USA) for 5 min at room temperature and mounted using Permafluor™ (Epredia, Kalamazoo, MI, USA). Stack of tiled images were acquired with SP8 confocal laser-scanning microscope (HC PL APO CS2 63x/1.20 WATER; 2048 × 2048 pixels; Leica Application Suite X software (3.7.4.23463 version) and a mosaic merge was performed to obtain wide images of the caecal tissue slices.
Gene expression analysis
Gene expression analysis was performed as described previously (Gaboriaud et al., 2020). Briefly, caecal tissues total RNA was extracted using TRIzol (Life Technologies, Carlsbad, CA, USA) and cDNA was synthetized using M-MLV Reverse Transcriptase (Promega, Madison, WI, USA). qPCRs were realised on CFX96 Touch Real-Time PCR Detection System (Bio-Rad) using iQ™ SYBR® Green Supermix (Bio-Rad). Inflammatory, anti-inflammatory and protein junction gene expressions were analysed using Gallus gallus housekeeping genes G10 and GAPDH (Supplementary data: Table 1, Eurogentec, Seraing, Belgium). The protocol used for qPCR was: 95°C for 5 min and 40 cycles at 95°C for 10 s and 60°C for 15 s followed by 60°C for 5 s. Melting curves were performed at 60°C for 5 s followed by gradual heating (0.5°C/s) to 95°C. qPCRs were performed in duplicate for each experiment. Gene expression was normalised to Ct values obtained for Gallus gallus G10 and GAPDH using the formula: 2-(Ct Gallus gallus specific gene – Ct mean of Gallus gallus housekeeping genes). Gene expression values are expressed as the median. To obtain a global view of inflammatory gene expression, a heatmap analysis was performed using the R package Pheatmap (Kolde, 2019). Median of gene expression values were normalized with a Z-score approach and scaled by genes. Hierarchical clustering analysis was also performed according to Z-score values. To investigate if gene expression depends on the animal group, a Principal Component Analysis (PCA) was performed. 2-dCT values were transformed in log10, quality of variables based on cos2 was established and PCA was generated using the R package Factominer (Lê et al, 2008).
Statistical analysis
For all data except PCA, statistical analysis was performed using GraphPad Prism® 9 (GraphPad Software Inc., La Jolla, CA, USA). For two groups, a non-parametric Mann-Whitney test was used. For more than two groups, a Kruskall-Wallis test with a Dunn’s multiple comparisons post-test was performed. For PCA, statistical differences between groups were performed using a pairwise PERMANOVA (Permutational multivariate analysis of variance) test (Anderson, 2001) with 999 permutations via Vegan (Jari et al, 2022) and pairwiseAdonis R packages (Martinez Arbizu, 2017). All packages were performed through Rstudio software version 4.1.0 (RStudio: Integrated Development for R. RStudio, PBC, Boston).
Data availability statement
The original contributions presented in the study are included in the article/Supplementary Material. Further inquiries can be directed to the corresponding author.
Ethics statement
The animal study was approved by ethics committee of Centre Val de Loire (CEEA VdL n°19). The study was conducted in accordance with the local legislation and institutional requirements.
Author contributions
FB designed the experiments. PG, GS, FT, NL, LS, and FB performed the experiments. EG provided the conventional and germ-free chickens. TL and ML performed the histology staining on caecal tissues and the analysis. CR and GS performed the immunofluorescence staining and confocal microscopy acquisition. PG, GS, and FT performed the cell staining and flow cytometry analysis. PG, GS, FT, and LS performed the qPCR and analysed the data. FT performed the PCA and Heatmap analysis. CS and NL realised the bacteriological controls and bacteriological analysis in the spleens. FB, PG, GS, and FT analysed the data. FB, CS, FL, EG and RG obtained funding. FB, PG, GS, FT, SL-L, FL, RG, and CS discussed the data. FB, GS, FT, AS, SL-L, FL, RG, and CS, wrote and/or reviewed the manuscript. All authors approved the final version of the manuscript. All authors contributed to the article.
Funding
This project was granted by the Région Centre Val de Loire, France (APR-IA “INTEGRITY” 2017-2019).
Acknowledgments
We are grateful to all the employees of the facility for Infection of Farm, Model and Wildlife Animals (PFIE), for the animal care and skills with the animal experiments (T. Chaumeil, M. Renouard, S. Lavillatte, P. Cousin, A. Faurie, O. Dubes, M. Girault). The authors are thankful to the society Boyé accouvage (La Boissière en Gâtine, France) for providing fertilized eggs.
Conflict of interest
The authors declare that the research was conducted in the absence of any commercial or financial relationships that could be construed as a potential conflict of interest.
Publisher’s note
All claims expressed in this article are solely those of the authors and do not necessarily represent those of their affiliated organizations, or those of the publisher, the editors and the reviewers. Any product that may be evaluated in this article, or claim that may be made by its manufacturer, is not guaranteed or endorsed by the publisher.
Supplementary material
The Supplementary Material for this article can be found online at: https://www.frontiersin.org/articles/10.3389/fcimb.2023.1250080/full#supplementary-material
Supplementary Figure 1 | Oocysts load in caecal contents at 7 days pi. Conventional and germ-free chickens were orally infected with 10 000 oocysts of E. tenella. Oocyst load in caecal contents was evaluated at day 7 pi. Medians are represented (Kruskall-Wallis test with a Dunn’s multiple comparisons post-test; n ≥ 11).
References
Anderson, M. J. (2001). A new method for non-parametric multivariate analysis of variance. Austral Ecol. 26, 32–46. doi: 10.1111/j.1442-9993.2001.01070.pp.x
Andrews, C., Mclean, M. H., Durum, S. K. (2018). Cytokine tuning of intestinal epithelial function. Front. Immunol. 9. doi: 10.3389/fimmu.2018.01270
Belkaid, Y., Hand, T. W. (2014). Role of the microbiota in immunity and inflammation. Cell 157, 121–141. doi: 10.1016/j.cell.2014.03.011
Blake, D. P., Knox, J., Dehaeck, B., Huntington, B., Rathinam, T., Ravipati, V., et al. (2020). Re-calculating the cost of coccidiosis in chickens. Vet. Res. 51, 115. doi: 10.1186/s13567-020-00837-2
Costa, M. C., Santos, J. R., Ribeiro, M. J., Freitas, G. J., Bastos, R. W., Ferreira, G. F., et al. (2016). The absence of microbiota delays the inflammatory response to Cryptococcus gattii. Int. J. Med. Microbiol. 306, 187–195. doi: 10.1016/j.ijmm.2016.03.010
De Sablet, T., Potiron, L., Marquis, M., Bussiere, F. I., Lacroix-Lamande, S., Laurent, F. (2016). Cryptosporidium parvum increases intestinal permeability through interaction with epithelial cells and IL-1beta and TNFalpha released by inflammatory monocytes. Cell Microbiol. 18, 1871–1880. doi: 10.1111/cmi.12632
Dho, M., Lafont, J. P. (1982). Escherichia coli colonization of the trachea in poultry: comparison of virulent and avirulent strains in gnotoxenic chickens. Avian Dis. 26, 787–797. doi: 10.2307/1589865
Fagundes, C. T., Amaral, F. A., Vieira, A. T., Soares, A. C., Pinho, V., Nicoli, J. R., et al. (2012). Transient TLR activation restores inflammatory response and ability to control pulmonary bacterial infection in germfree mice. J. Immunol. 188, 1411–1420. doi: 10.4049/jimmunol.1101682
Gaboriaud, P., Sadrin, G., Guitton, E., Fort, G., Niepceron, A., Lallier, N., et al. (2020). The absence of gut microbiota alters the development of the apicomplexan parasite eimeria tenella. Front. Cell Infect. Microbiol. 10. doi: 10.3389/fcimb.2020.632556
Gloux, A., Le Roy, N., Brionne, A., Bonin, E., Juanchich, A., Benzoni, G., et al. (2019). Candidate genes of the transcellular and paracellular calcium absorption pathways in the small intestine of laying hens. Poult Sci. 98, 6005–6018. doi: 10.3382/ps/pez407
Gong, Y., Liu, X., Zhang, S., Tang, X., Zou, J., Suo, X. (2022). Antibiotic changes host susceptibility to eimeria falciformis infection associated with alteration of gut microbiota. Infect. Immun. 90, e0022922. doi: 10.1128/iai.00229-22
Guitton, E., Faurie, A., Lavillatte, S., Chaumeil, T., Gaboriaud, P., Bussiere, F., et al. (2020). Production of germ-free fast-growing broilers from a commercial line for microbiota studies. J. Vis. Exp. 10, 3791/61148. doi: 10.3791/61148
Hayes, K. S., Bancroft, A. J., Goldrick, M., Portsmouth, C., Roberts, I. S., Grencis, R. K. (2010). Exploitation of the intestinal microflora by the parasitic nematode Trichuris muris. Science 328, 1391–1394. doi: 10.1126/science.1187703
Hayes, C. L., Dong, J., Galipeau, H. J., Jury, J., Mccarville, J., Huang, X., et al. (2018). Commensal microbiota induces colonic barrier structure and functions that contribute to homeostasis. Sci. Rep. 8, 14184. doi: 10.1038/s41598-018-32366-6
Helmboldt, C. F., Bryant, E. S. (1971). The pathology of necrotic enteritis in domestic fowl. Avian Dis. 15, 775–780. doi: 10.2307/1588866
Heriveau, C., Dimier-Poisson, I., Lowenthal, J., Naciri, M., Quere, P. (2000). Inhibition of Eimeria tenella replication after recombinant IFN-gamma activation in chicken macrophages, fibroblasts and epithelial cells. Vet. Parasitol. 92, 37–49. doi: 10.1016/s0304-4017(00)00275-2
Hong, Y. H., Lillehoj, H. S., Lee, S. H., Dalloul, R. A., Lillehoj, E. P. (2006). Analysis of chicken cytokine and chemokine gene expression following Eimeria acervulina and Eimeria tenella infections. Vet. Immunol. Immunopathol. 114, 209–223. doi: 10.1016/j.vetimm.2006.07.007
Hua, K. F., Chou, J. C., Ka, S. M., Tasi, Y. L., Chen, A., Wu, S. H., et al. (2015). Cyclooxygenase-2 regulates NLRP3 inflammasome-derived IL-1beta production. J. Cell Physiol. 230, 863–874. doi: 10.1002/jcp.24815
Huang, G., Tang, X., Bi, F., Hao, Z., Han, Z., Suo, J., et al. (2018a). Eimeria tenella infection perturbs the chicken gut microbiota from the onset of oocyst shedding. Vet. Parasitol. 258, 30–37. doi: 10.1016/j.vetpar.2018.06.005
Huang, G., Zhang, S., Zhou, C., Tang, X., Li, C., Wang, C., et al. (2018b). Influence of eimeria falciformis infection on gut microbiota and metabolic pathways in mice. Infect. Immun. 86(5), e00073–18. doi: 10.1128/IAI.00073-18
Jari, O., Simpson, G. L., Blanchet, F. G., Kindt, R., Legendre, P., Minchin, P. R., et al. (2022) Vegan: community ecology package. Available at: https://cran.r-project.org/package=vegan.
Johnson, J., Reid, W. M. (1970). Anticoccidial drugs: lesion scoring techniques in battery and floor-pen experiments with chickens. Exp. Parasitol. 28, 30–36. doi: 10.1016/0014-4894(70)90063-9
Kim, W. H., Chaudhari, A. A., Lillehoj, H. S. (2019). Involvement of T cell immunity in avian coccidiosis. Front. Immunol. 10. doi: 10.3389/fimmu.2019.02732
Kolde, R. (2019) pheatmap: Pretty Heatmaps. R package version 1.0.12. Available at: https://CRAN.R-project.org/package=pheatmap.
Lafont JP, Y. P., Bree, A, Peloille, M. (1975). Pouvoir pathogène d’eimeria tenella et d’eimeria acervulina chez des poulets axéniques et monoxéniques. Annales recherches vétérinaires 6, 35–42.
Laurent, F., Mancassola, R., Lacroix, S., Menezes, R., Naciri, M. (2001). Analysis of chicken mucosal immune response to Eimeria tenella and Eimeria maxima infection by quantitative reverse transcription-PCR. Infect. Immun. 69, 2527–2534. doi: 10.1128/IAI.69.4.2527-2534.2001
Lê, S., Josse, J., Husson, F. (2008). FactoMineR: an R package for multivariate analysis. J. Stat. Software 25, 18. doi: 10.18637/JSS.V025.I01
Lillehoj, H. S., Trout, J. M. (1996). Avian gut-associated lymphoid tissues and intestinal immune responses to Eimeria parasites. Clin. Microbiol. Rev. 9, 349–360. doi: 10.1128/CMR.9.3.349
Macdonald, S. E., Nolan, M. J., Harman, K., Boulton, K., Hume, D. A., Tomley, F. M., et al. (2017). Effects of Eimeria tenella infection on chicken caecal microbiome diversity, exploring variation associated with severity of pathology. PloS One 12, e0184890. doi: 10.1371/journal.pone.0184890
Martinez Arbizu, P. (2017) PairwiseAdonis: pairwise multilevel comparison using adonis. Available at: https://github.com/pmartinezarbizu/pairwiseAdonis.
Mittrucker, H. W., Seidel, D., Bland, P. W., Zarzycka, A., Kaufmann, S. H., Visekruna, A., et al. (2014). Lack of microbiota reduces innate responses and enhances adaptive immunity against Listeria monocytogenes infection. Eur. J. Immunol. 44, 1710–1715. doi: 10.1002/eji.201343927
Nakanishi, Y., Sato, T., Ohteki, T. (2015). Commensal Gram-positive bacteria initiates colitis by inducing monocyte/macrophage mobilization. Mucosal Immunol. 8, 152–160. doi: 10.1038/mi.2014.53
Nardi, R. M., Silva, M. E., Vieira, E. C., Bambirra, E. A., Nicoli, J. R. (1989). Intragastric infection of germfree and conventional mice with Salmonella typhimurium. Braz. J. Med. Biol. Res. 22, 1389–1392.
Nascimento, B. B., Cartelle, C. T., Noviello, M. L., Pinheiro, B. V., De Almeida Vitor, R. W., Souza, D. D. G., et al. (2017). Influence of indigenous microbiota on experimental toxoplasmosis in conventional and germ-free mice. Int. J. Exp. Pathol. 98, 191–202. doi: 10.1111/iep.12236
Oakley, B. B., Lillehoj, H. S., Kogut, M. H., Kim, W. K., Maurer, J. J., Pedroso, A., et al. (2014). The chicken gastrointestinal microbiome. FEMS Microbiol. Lett. 360, 100–112. doi: 10.1111/1574-6968.12608
Pham, H. H. S., Matsubayashi, M., Tsuji, N., Hatabu, T. (2021). Relationship between Eimeria tenella associated-early clinical signs and molecular changes in the intestinal barrier function. Vet. Immunol. Immunopathol. 240, 110321. doi: 10.1016/j.vetimm.2021.110321
Porcheron, G., Chanteloup, N. K., Trotereau, A., Bree, A., Schouler, C. (2012). Effect of fructooligosaccharide metabolism on chicken colonization by an extra-intestinal pathogenic Escherichia coli strain. PloS One 7, e35475. doi: 10.1371/journal.pone.0035475
Qi, Z., Shi, S., Tu, J., Li, S. (2019). Comparative metagenomic sequencing analysis of cecum microbiotal diversity and function in broilers and layers. 3 Biotech. 9, 316. doi: 10.1007/s13205-019-1834-1
Rychlik, I. (2020). Composition and function of chicken gut microbiota. Anim. (Basel) 10(1), 103. doi: 10.3390/ani10010103
Sateriale, A., Gullicksrud, J. A., Engiles, J. B., Mcleod, B. I., Kugler, E. M., Henao-Mejia, J., et al. (2021). The intestinal parasite Cryptosporidium is controlled by an enterocyte intrinsic inflammasome that depends on NLRP6. Proc. Natl. Acad. Sci. U.S.A. 118(2), e2007807118. doi: 10.1073/pnas.2007807118
Teng, P. Y., Yadav, S., Castro, F. L. S., Tompkins, Y. H., Fuller, A. L., Kim, W. K. (2020). Graded Eimeria challenge linearly regulated growth performance, dynamic change of gastrointestinal permeability, apparent ileal digestibility, intestinal morphology, and tight junctions of broiler chickens. Poult Sci. 99, 4203–4216. doi: 10.1016/j.psj.2020.04.031
Van Immerseel, F., De Buck, J., Pasmans, F., Huyghebaert, G., Haesebrouck, F., Ducatelle, R. (2004). Clostridium perfringens in poultry: an emerging threat for animal and public health. Avian Pathol. 33, 537–549. doi: 10.1080/03079450400013162
Venuprasad, K., Theiss, A. L. (2021). NLRP6 in host defense and intestinal inflammation. Cell Rep. 35, 109043. doi: 10.1016/j.celrep.2021.109043
Wei, S., Morrison, M., Yu, Z. (2013). Bacterial census of poultry intestinal microbiome. Poult Sci. 92, 671–683. doi: 10.3382/ps.2012-02822
Keywords: Eimeria tenella, microbiota, germ-free, chicken, inflammation, bacterial translocation
Citation: Tomal F, Sadrin G, Gaboriaud P, Guitton E, Sedano L, Lallier N, Rossignol C, Larcher T, Rouille E, Ledevin M, Guabiraba R, Silvestre A, Lacroix-Lamandé S, Schouler C, Laurent F and Bussière FI (2023) The caecal microbiota promotes the acute inflammatory response and the loss of the intestinal barrier integrity during severe Eimeria tenella infection. Front. Cell. Infect. Microbiol. 13:1250080. doi: 10.3389/fcimb.2023.1250080
Received: 29 June 2023; Accepted: 28 July 2023;
Published: 23 August 2023.
Edited by:
Shahram Solaymani-Mohammadi, University of North Dakota, United StatesReviewed by:
Katarzyna B. Miska, Agricultural Research Service (USDA), United StatesEmanuel Heitlinger, Leibniz Institute for Zoo and Wildlife Research (LG), Germany
Copyright © 2023 Tomal, Sadrin, Gaboriaud, Guitton, Sedano, Lallier, Rossignol, Larcher, Rouille, Ledevin, Guabiraba, Silvestre, Lacroix-Lamandé, Schouler, Laurent and Bussière. This is an open-access article distributed under the terms of the Creative Commons Attribution License (CC BY). The use, distribution or reproduction in other forums is permitted, provided the original author(s) and the copyright owner(s) are credited and that the original publication in this journal is cited, in accordance with accepted academic practice. No use, distribution or reproduction is permitted which does not comply with these terms.
*Correspondence: Françoise I. Bussière, RnJhbmNvaXNlLkJ1c3NpZXJlQGlucmFlLmZy
†These authors contributed equally to this work and share first authorship