- Quorum Sensing Laboratory, Centre for Research in Infectious Diseases (CRID), School of Chemical and Biotechnology, SASTRA Deemed to be University, Thanjavur, India
Candida albicans-mediated vulvovaginal candidiasis (VVC) is a significant challenge in clinical settings, owing to the inefficacy of current antifungals in modulating virulence, development of resistance, and poor penetration into the biofilm matrix. Various predisposition factors are molecular drivers that lead to the dysbiosis of normal microflora of the vagina, upregulation of central metabolic pathways, morphogenesis, hyphal extension, adhesion, invasion, and biofilm formation leading to chronic infection and recurrence. Hence, it is crucial to understand the molecular mechanism behind the virulence pathways driven by those drivers to decode the drug targets. Finding innovative solutions targeting fungal virulence/biofilm may potentiate the antifungals at low concentrations without affecting the recurrence of resistance. With this background, the present review details the critical molecular drivers and associated network of virulence pathways, possible drug targets, target-specific inhibitors, and probable mode of drug delivery to cross the preclinical phase by appropriate in vivo models.
1 Introduction: VVC
Vulvovaginal candidiasis (VVC) is an inflammatory mucosal infection in the lower reproductive tracts of women. Among women of different ages, more reported cases of VVC are in the reproductive age. Also, 75% of women experience at least one episode of VVC in their lifetime, and 8% experience a recurrence of infection, at least four episodes per year. Global statistics show that 138 million women worldwide experience recurrent VVC each year, with an annual prevalence of 3,871/100,000. According to base case estimates considering the increase in female population worldwide, the expected number of individuals affected with recurrent VVC is predicted to rise to over 158 million by 2030. As a result, 20,240,664 more VVC-affected cases are added to the predicted increase in female population from 3.34 to 4.181 billion people (Żyrek et al., 2021).
The global statistics of VVC are hiked by Candida, a predominant fungal colonizer of the vaginal lumen (Ahangari et al., 2019). Candida albicans and several other related species, Candida glabrata, Candida tropicalis, Candida parapsilosis, and Candida krusei cause VVC (Guinea, 2014). Interestingly, C. albicans, the primary causative agent of VVC, is categorized as a critical fungal priority pathogen (CFPP) by the WHO (World Health Organization) (Fisher and Denning, 2023). C. albicans is also a benevolent partner of the microbiome of healthy individuals and is capable of switching from a commensal to a pathogenic state under adverse conditions driven by various predisposition factors (Mukaremera et al., 2017). Several predisposition factors are associated with VVC, which include diabetes mellitus (DM), elevated endogenous estrogens (from pregnancy or obesity), immunosuppression (i.e., transplant patients, antimetabolite medications or chemotherapy, or HIV infection), broad-spectrum antibiotic use, and other environmental factors (Gonçalves et al., 2016). Recent studies have shown that patients with DM are more prone to candidiasis, as glucose plays a significant role in the colonization and proliferation of these pathogens in the host (Gürsoy et al., 2018). Host-influenced (predisposition) factors are critical in driving C. albicans morphogenetic lifestyle changes from yeast to hyphal and enable it to cross its boundary from commensal to pathogenic form and vice versa (Mukaremera et al., 2017). C. albicans, in its lifestyle transition, express various virulence traits that increase its survival fitness in the host and lead to the rapid evolution of resistance to antifungals (Slutsky et al., 1985; Sudbery et al., 2004). The survival fitness of C. albicans enhances them to effectively colonize the host and leads to the spread of infection in an asymptomatic/symptomatic mode causing severe mucosal inflammation in affected individuals (Farhan et al., 2019). Mucosal inflammation is a symptomatic response in patients who may exhibit vaginal itching, vaginal burning, dyspareunia, and edema, and may form a thick or sticky discharge (Sheppard, 2020). It is quite alarming that no new class of antifungals has reached the recipients since the 2000s to treat VVC. The loss of productivity is mainly due to the eukaryotic nature of fungal cells, difficulties with compound permeability across the fungal cell wall and membrane, and a lack of interest from the pharmaceutical industry; the development of novel antifungal agents has generally been slow (Roemer and Krysan, 2014).
Infection and therapy must go hand in hand for a better cure. Once it fails, the global economic burden also increases with the emergence of antifungal resistance. Recent estimates show that the global VVC treatment market value will reach USD 986.5 million. In the next 10 years, the compound annual growth rate (CAGR) will be 4.1% according to a VVC treatment market report (Denning et al., 2018). In parallel, it would be a tremendous challenge for both high-/low-income countries, and there is a pressing need to address this global health-associated risk. Finding solutions through innovations relies on molecular information on the various drivers (predisposition factors), molecular pathways, and associated virulence that affect the pathogen colonization and cause infection in the host. Hence, in this review, we focus on the predisposition factors leading to the virulence of candidiasis, existing treatment strategies with their disadvantages, and how this can be tackled by deciphering possible drug targets and probable mode of drug delivery to cross the preclinical phase by appropriate in vivo models.
2 Molecular drivers of VVC
VVC recurrence relies on various intrinsic and extrinsic factors. The key host-specific factors, such as the pH of the vaginal environment, age and hormonal status, local defense mechanisms, pregnancy, allergies, psychosocial stress, metabolic issues, immunosuppression, and individual genetic susceptibility, are essential. Additionally, behavioral risk factors including oral contraceptives, overuse of antimicrobials, glucocorticoids, inhibitors of the sodium-glucose co-transporter-2 (SGLT2), intrauterine devices (IUDs), spermicides, and condoms, as well as sexual, hygienic, and dressing habits, demand intervention (Table 1) (Patel et al., 2004).
2.1 pH
C. albicans makes use of its remarkable capacity to respond to variations in nutrition availability, ion or serum concentrations, osmotic pressure, and ambient pH to thrive and spread within the host. Most strikingly, C. albicans could colonize and exhibit a normal growth rate on various host niches over a wide range of ambient pH (Brotman et al., 2014). However, in its transition from a commensal to a pathogenic state, the host environment pH changes to slightly alkaline and, to an extent, neutral, like a blood pH of 7.3. The change in the extracellular pH modulates several C. albicans genes, viz., Rim101-dependent or -independent pathways (Davis et al., 2000; Vylkova et al., 2011). Among various genes, PHR1 and PHR2 (pH-responsive genes 1 and 2) are the first reported Rim101-dependent pH-regulated genes in C. albicans. However, the expression of pH-responsive genes is finely regulated, where a pH ≤ 5.5 is required for PHR1 expression; in contrast, a pH ≤ 5.5 is required for PHR2. The pH-dependent control is essential for C. albicans to establish pathogenesis in the host niches. Highly comparable glycoproteins that are believed to be attached to the plasma membrane by a glycosylphosphatidylinositol (GPI) are encoded by PHR1 and PHR2, respectively (Davis et al., 2000). C. albicans deficient in expressing PHR1/PHR2 proteins invariably affects its morphogenesis, and cell wall synthesis is pH dependent (Lesage and Bussey, 2006). In addition, the weak organic (lactic) acid released from the host-epithelial or the co-colonized microbes dissociates directly into microbial cells because of their lipophilic characteristics. On internalization, the negatively charged counter-ion accumulates and leads to a rise in turgor pressure, oxidative stress, and the loss of vital cellular elements such as ribosomal RNA and cofactors, an effective antimicrobial against C. albicans (Zeise et al., 2021). The vaginal microbiota processes the glycogen released from the vaginal epithelial cells, especially by female hormones, to produce lactic acid. However, during the dysbiosis of the vaginal microbial consortium, even though glycogen levels are high, the pH remains alkaline, and the environment is prone to infections (d’Enfert et al., 2021).
2.2 Hormonal imbalance
Estrogen is a steroid hormone that regulates women’s immune systems and overall health (Brotman et al., 2014). Elevated estrogen levels retrench leukocyte infiltration and epithelial cell-mediated antifungal responses. Among the four isoforms of estrogen [estrone (E1), 17β-estradiol (E2), estriol (E3), and 17α-ethynylestradiol (EE2)], E2 is most potent and more associated with many gynecological disorders (Dubey et al., 2005).
Studies have shown that women of reproductive age are more susceptible to candidiasis. Postmenopausal and prepuberty girls are less prone to infection unless they undergo hormone replacement therapy or recurrent usage of oral contraceptives (Phillips et al., 2023). Furthermore, the effects of estrogen on the immune system and the vaginal microbiome can make it more conducive to the growth of C. albicans. C. albicans estrogen adaptation has been found to increase fungal virulence by suppressing phagocytosis, facilitating the yeast to circumvent the innate immune responses. The enhanced binding of Factor H on the fungal cell surface in the presence of estrogen is the cause of this emergence. A critical component of the innate immune response, the host’s complement system can recognize and assault fungal cells. The fungal cell surface protein encoded by GPD2 drives the estrogen-induced expression of Factor H. Factor H is a human complement regulatory protein that aids in inactivating the alternative complement system and, thus, preventing the assault on fungal cells (Kumwenda et al., 2022). However, preclinical studies on animal models have shown the role of exogenous estrogen in the fungal infection to persist in the vaginal area. The hormone estrogen promotes the process of keratinization and cornification of the upper layers of the vaginal epithelium, which provides an environment that is favorable for the growth of C. albicans (Dennerstein and Ellis, 2001; Willems et al., 2020). Furthermore, pregnancy, antibiotic misuse, and age might cause an imbalance in estrogen levels, boosting susceptibility to infection (Aguin and Sobel, 2015). In this context, estrogen appears to be crucial for C. albicans to cause hormone-associated vaginitis, whereas progesterone does not impact the same (Fidel et al., 2000).
2.3 Diabetes mellitus
Women with diabetes are more susceptible to VVC owing to increased serum glucose levels. Several case studies show the relationship between women affected with DM and recurrent VVC (Mohammed et al., 2021). DM can lead to systemic candidiasis through diabetic vasculopathy, hypoperfusion and hyperglycemia, and microvascular disease progression, thus damaging the host defense mechanism, leading to fungal growth and adhesion (Guimarães et al., 2012). The inter-relationship of DM and VVC is due to the expression of glucose-inducible surface proteins that resemble the complement system proteins. An increase in the level of such surface proteins weakens the immune system. Furthermore, the reduced neutrophil migration diminishes their functions, including phagocytosis, chemotaxis, and intercellular killing (Sustr et al., 2020).
Blood glucose levels and evasion of the immune system strengthen the major virulence factors in C. albicans, like secreted aspartyl protease (Sap), phospholipases, candidalysin, and biofilm formation. Sap proteins can break down various host-related components and aid in generating nitrogen, which is required for C. albicans penetration in the host cells (Czechowicz et al., 2022). Similarly, phospholipases target membrane phospholipids to initiate cell lysis and penetration (Ghannoum, 2000). Candidalysin encoded by the gene ECE1 promotes the influx of calcium ions and lactate dehydrogenase (LDH), impacting membrane and cell damage destabilization (Ho et al., 2019).
2.4 Overuse of antifungals
The repeated use of antifungals, especially azoles, polyenes, and echinocandins, as a first-line treatment strategy for VVC can develop resistomes in C. albicans, thereby fostering its adhesion and colonization in the epithelial cells to initiate infection (Bhattacharya et al., 2016; Bhattacharya et al., 2020). Overuse of antifungals can lead to a conformational change in the target, overexpression of efflux pumps, modulation of stress responses, and genomic modifications (Cowen, 2008). Also, antifungal overuse can disrupt the normal microflora of the vagina, leading to an increased recurrence of infection. Lack of proper diagnosis and use of antifungals after antibiotic treatment can also be prominent reasons for generating multidrug-resistant strains (Donders et al., 2011).
2.5 Other factors
Various other factors, including variations in vaginal temperature, nutrient stress, and immunocompromised state, can lead to the colonization of C. albicans in the host epithelial tissue (Willems et al., 2020). The equilibrium of the vaginal microbiota is critically dependent on vaginal temperature. The environment for the growth of C. albicans may be more favorable if the vaginal temperature rises (Antley and Hazen, 1988). Likewise, nutrient stress results from a vital nutrient deficiency that sustains a healthy vaginal environment. The immune system needs enough specific nutrients, such as vitamins, minerals, and antioxidants, to perform at its best and maintain a healthy vaginal microbiome. Some nutrient deficiencies might weaken the immune system and make it more challenging to regulate C. albicans overgrowth (Rutherford et al., 2019). Also, HIV/AIDS, a few autoimmune disorders, cancer treatments including chemotherapy or radiation, organ transplantation, and long-term immunosuppressive drug use are among the conditions that can lead to immunocompromise. Such immunocompromised women are more prone to C. albicans growth and proliferation, which can result in more frequent and severe vaginal candidiasis episodes (Willems et al., 2020).
3 Molecular driver-associated virulence determinants
The dysbiosis of the healthy microbiome in the vagina by various predisposition factors leads to the initiation of the virulence mechanism in C. albicans. The evolution of yeast-like fungi to an invasive pathogen depends on many fungal factors along with the host. Such host–pathogen interaction relies on many stages, including adhesion, invasion, biofilm formation, and spread of infection (Willems et al., 2020) (Figure 1).
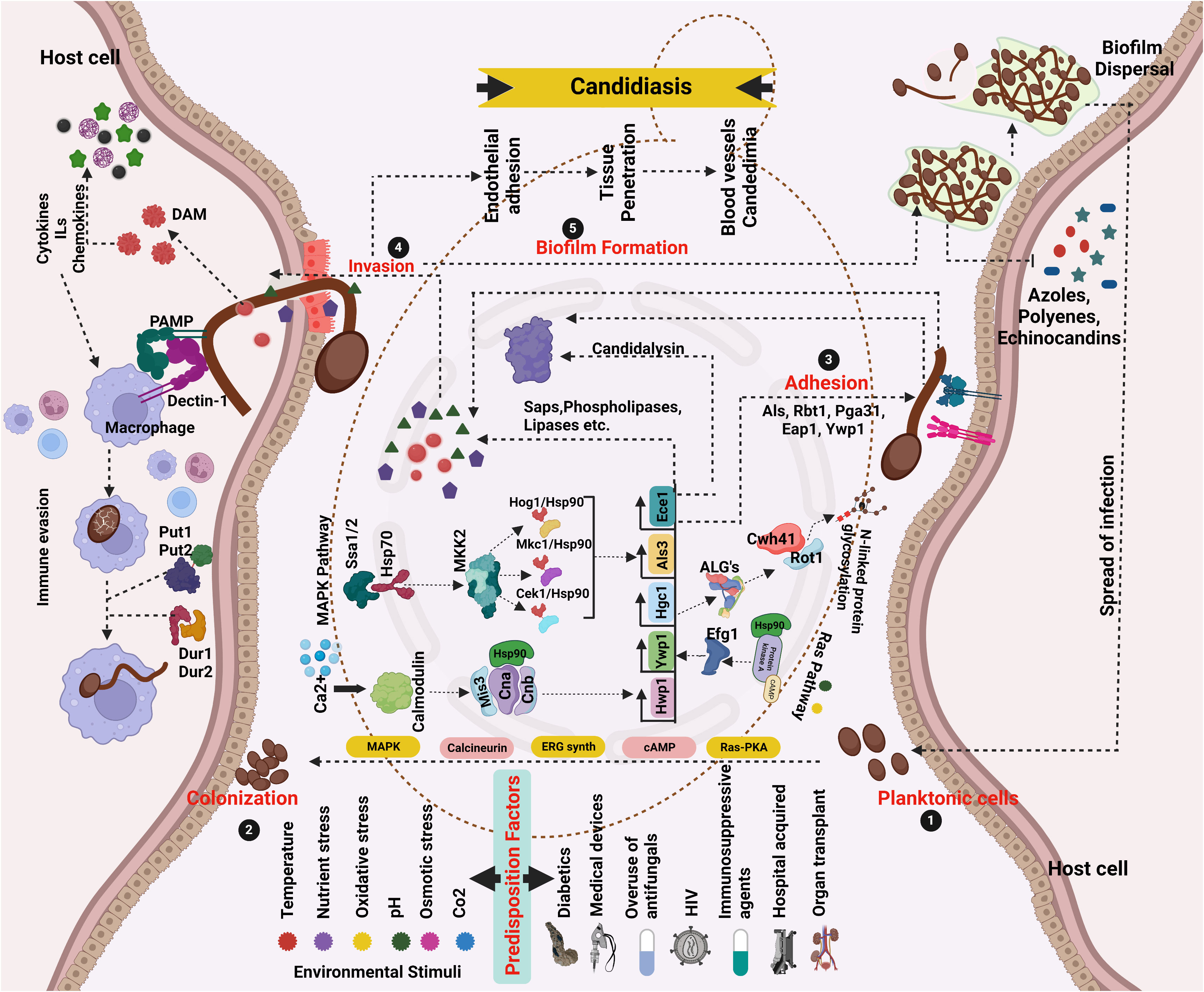
Figure 1 Network pathway analysis of Candida pathogenicity and virulence driven by various predisposition factors. (1 and 2) Yeast cells that are planktonic colonize surfaces. Favorable conditions promote overgrowth and adherence (3), where cells stick to host cells using adhesins, and hyphae formation/extension and environmental constraints activate HSPs, signaling, and adaptation pathways that activate genes related to morphology. The pathogenesis of C. albicans begins with hyphal development. (4 and 5) Epithelial/endothelial adhesion/invasion with the help of hydrolytic enzymes. Prior to the onset of infection, generate biofilms (6). After the maturation of the biofilm, the dispersed cells from the biofilm localize to other areas leading to the spread of infection. Different kinds of candidiasis are caused by cytolytic proteins and enzymes destroying epithelial and mucosal surfaces (created using BioRender.com).
3.1 Adhesion
Adhesion is a multifactorial complex process, and with the influence of various predisposition factors, C. albicans colonize and adhere to the epithelial cells. The colonization of the pathogen with the host epithelial cells is through non-specific interactions like hydrophobic and electrostatic interactions and effects changes in the central metabolic pathways like MAPKK, Ras-PKA, Calcineurin, and Ergosterol synthetic pathway (Biswas et al., 2007; Moyes et al., 2015).
Following successful colonization, various adhesive proteins will initiate adherence to the receptors on the host. These adhesive proteins are encoded by different families of genes, including ALS (Agglutinin Like Sequence), HWP1 (Hyphal Wall Protein), and EPA (Epithelial Adhesins). ALS is the largest among them and encodes eight proteins (ALS1–7 and ALS9) bound to glycosylphosphatidylinositol (GPI). All these proteins mainly have three regions, an N-terminal domain for specific substrates, the core region with tandem repeat sequences, and a C-terminal domain with a GPI anchor sequence (Nobile et al., 2006; Hoyer and Cota, 2016).
The other critical group of adhesins is the HWP1 protein, which has a characteristic of hyphal formation, with a unique mechanism of action. The N-terminal domain is rich in Gln-Pro, the binding site of host transglutaminase catalyzing the adhesion. A recent study reports that HWP1 and other adhesins are necessary for rat catheter-associated infections (Maras et al., 2021). Like HWP1, C. albicans adherence to host epithelial cells and biofilm formation includes Eap1p (Enhanced Adherence to Polystyrene) (Li and Palecek, 2003; Richardson et al., 2018; Samot and Rouabhia, 2021). Also, among the cell wall (GPI-anchored)-associated aspartyl proteinases, Sap10 enhances C. albicans adhesion compared to SAP9. Studies evidence the opposing effect of both the proteinases in that the deletion of the SAP9 gene in C. albicans increased its adhesion and vice versa in the other deleted (SAP10) mutants (Albrecht et al., 2006).
3.2 Invasion
The invasion of C. albicans follows the adhesion promoted by its hyphae, which causes epithelial cell damage, penetration, and host immune system inactivation by adhesion to the host cells. The process of invasions is enhanced by the secreted invasins, especially hydrolytic enzymes, including hemolysins, proteases, lipases, and phospholipases (Mayer et al., 2013). Among them, one crucial family of invasins is SAP 1–10 encoded by SAP genes. SAPs are classified into three classes based on their sequence homology by amino acid and specificity for the substrate (Schaller et al., 2003). The C. albicans SAP isoenzyme family displays sequence homology that indicates the presence of three distinct clusters: SAP1 to SAP3, exhibiting 67% sequence identity, and a closer association among members SAP4 to SAP6, which share a higher sequence homology of up to 90% (Staib et al., 1999; Naglik et al., 2003). SAP10 involved in the GPI-linked cell surface anchoring is classified as endopeptidases. SAP is involved in the hyphal formation, penetration, and degradation of defense molecules secreted by the host, like alpha-macroglobulin, collagen, mucin, complement proteins, immunoglobulins, and lactoperoxidase. Among different SAPs, SAP1–SAP3 are more crucial for invasion; literature has shown the importance of these proteins, especially SAP3, in the colonization and infection of vaginal epithelial cells (Correia et al., 2010; Ali et al., 2018). Recent research has shown that the gene ECE1, which codes for the protein candidalysin, is essential for destroying epithelial cells, the generation of cytokines, and neutrophil recruitment. This hyphae-specific protein has 271 amino acids spread over seven lysine-arginine (KR) repeats. Kex2 protease cleaves the protein into eight peptides. Peptide three (N-SIIGIIMGILGNIPQVIQIIMSIVKAFKGNK) produced can cause lysis and trigger inflammatory reactions, hence named candidalysin (Engku Nasrullah Satiman et al., 2020). During experimental VVC, deletion of ECE1 or candidalysin reduces immunopathologic indicators of infection (neutrophils, pro-inflammatory cytokines, and alarmins) and tissue damage. Notably, deletion mutants did not exhibit altered colonization or defects in filamentation in vivo, proving that candidalysin is necessary for promoting immunopathogenesis during VVC and that hyphae are insufficient to do so (Liu et al., 2021).
Another important class of invasins is phospholipases, a group of hydrolases known for the hydrolysis of cell membrane phospholipids inducing cell lysis and penetration to host tissue. Four classes of phospholipases are known to date, A, B, C, and D, classified based on the ester bond they cleave (Ghannoum, 2000; Bassyouni et al., 2015). Hemolysins and esterase are other virulence-causing enzymes in which hemolysins are necessary for iron acquisition and survival when esterase is known to exhibit cytotoxic effects (Tan et al., 2015).
3.3 Biofilm formation
C. albicans is well known for its multifaceted biofilm formation process, which begins with the successful adhesion of yeast cells to a surface, followed by developing a discrete colony (Sustr et al., 2020). Next, in the intermediate phase, cells organize and begin producing and secreting extracellular polymeric substances (EPS), which enable the maturation of a three-dimensional structure, forming the biofilm. The mature biofilms of C. albicans have a more heterogeneous structure, with blastophores and hyphae enclosed in an ECM (extracellular matrix) made of polysaccharide material. Not only cutaneous or mucosal but also biofilm formation associated with medical devices is also predominated by C. albicans. It is also critical to observe that the biofilm cells, after maturation, disperse by forming biofilms in new sites and the host tissue becomes vulnerable to the spread of infections (Cavalheiro and Teixeira, 2018). Several genes, including those involved in protein synthesis, the glycolytic cycle, glycolysis, and response to oxidative stress, are activated in C. albicans and persist and spread in the blood candidemia. The yeast spreads from the blood to the other sites of the body, where it causes systemic illnesses. Extracellular hydrolytic enzymes, adhesins, phenotypic flipping, and cytolytic proteins also aid in the spread of candidiasis (Atriwal et al., 2021).
3.4 Host immune response
C. albicans host interaction and the lifestyle transition from commensalism to pathogenic form activate specific virulence pathways and respond to the change in the host environment. Initially, innate immune cell populations recognize C. albicans in different ways. It involves the recognition of conserved pathogen-associated molecular patterns (PAMPs) by several families of PRRs, including the C-type lectin receptors (CLRs), Toll-like receptors (TLRs), RIG-I-like receptors (RLRs), and NOD-like receptors (NLRs) (Shibata et al., 2007). The cell wall of C. albicans has two distinct layers: the outer layer, primarily made up of O- and N-linked glycoproteins that contain 80%–90% mannose, and the inner cell wall, which contains the skeletal polysaccharides chitin, β-1,3-glucan, and β-1,6-glucan, which provide cell strength and shape. The β-1,3- and β-1,6-glucans are essential C. albicans cell wall elements recognized by CLRs (Munro et al., 1998). Dectin 1 (CLEC7A), a CLR expressed primarily on monocytes and macrophages that induce cytokine production as well as the internalization of the fungus through the establishment of a “phagocytic synapse,” is one of the most extensively investigated β-glucan receptors. Caspase activation and recruitment domain containing-9 (CARD9), spleen tyrosine kinase (SYK), protein kinase C12–16, and the RAF1 kinase signaling pathway are all involved in the process through which Dectin 1 produces intracellular signals (Whitney et al., 2014). The release of neutrophil extracellular traps (NETs) during fungal infection is said to be prevented by signaling via Dectin 1 (Branzk et al., 2014). The relevance of CARD9 is highlighted by the fact that CARD9-deficient mice are more susceptible to invasive candidiasis than wild-type mice and that humans with loss-of-function mutations in CARD9 likewise exhibit greater susceptibility to invasive candidiasis (Glocker et al., 2009). Complement receptor 3 (CR3) is primarily involved in detecting β-glucans by neutrophils, phagocytosis, and destroying unopsonized C. albicans (van Bruggen et al., 2009).
Various CLRs, including the mannose receptor, DC-SIGN (CD209), Dectin 2 (C-type lectin domain family 6 member E, CLEC6A), and MINCLE (C-type lectin domain family 4 member E, CLEC4E), are capable of recognizing mannans and mannoproteins (Cambi et al., 2008). Candida N-mannan is recognized by the mannose receptor, which is predominantly expressed in macrophages. Thus, signaling pathways are crucial for the generation of pro-inflammatory cytokines, including IL-17 (Netea et al., 2008). Candida α-mannan is recognized by Dectin 2, which is mainly expressed on dendritic cells (DCs), macrophages, and neutrophils. Dectin 2 has been linked to the generation of reactive oxygen species (ROS), in addition to its function in regulating T helper 17 (TH17) cell responses (Ifrim et al., 2014). During a C. albicans infection, dectin 2 and dectin 3 have been shown to heterodimerize, causing pro-inflammatory responses like the generation of tumor necrosis factor (TNF), IL-1, and IL-6. Both monocytes and neutrophils express the CLR MINCLE, which oversees activating protective responses against C. albicans, primarily by starting TNF production (Zhu et al., 2013). While DC-SIGN is found on both DCs and macrophages and recognizes Candida N-linked mannan, its activation promotes adaptive immune responses by increasing the expression of cytokines that drive TH cell activation and differentiation (Netea et al., 2008). The receptor, Galectin 3, on the surface of macrophages also detects mannoproteins and triggers the release of TNF, which, in turn, causes mouse macrophages to mount a protective antifungal defense (Linden et al., 2013). Likewise, the immunological response to C. albicans involves a complicated interaction between T regulatory cells (Tregs). Studies show that Tregs can stimulate and inhibit immunity while treating C. albicans infections. The result of the immune response to C. albicans depends critically on the interaction between Tregs and other immune cells, such as Th17 cells. Tregs can control inflammatory reactions and stop tissue damage from an overactive immune system. However, Tregs imbalance can obstruct the removal of C. Albicans and impair the protective immune response (Whibley and Gaffen, 2014). Similarly, a class of proteins called pentraxins is involved in inflammatory response by the immune system. C-reactive protein (CRP) and serum amyloid P component (SAP) are well-known pentraxins. The liver produces CRP, an acute-phase reactant, in reaction to inflammation. It attaches to several pathogens, including bacteria, viruses, and fungi, and then activates the complement system to aid in removing these pathogens. The other pentraxin, SAP, recognizes the glucans in the fungal cell wall, leading to opsonization and fungus clearing (Du Clos, 2013).
Although the human immune system is typically quite effective at preventing fungal infections, C. albicans adopt various strategies that include PAMPs for evading immune system clearance. The mannan-shielded glucans on the surface of C. albicans hyphae are less inflammatory than the yeast forms. Thus, the morphogenetic transition from yeast to a hyphal form of C. albicans may alter the immune response against the pathogen during the evasion process. However, the exact molecular mechanisms underlying these processes still need to be explored. C. albicans also suppress macrophage nitric oxide generation and phagolysosome maturation (van der Graaf et al., 2005). Furthermore, by causing macrophages to change from the more inflammatory M1 to the less inflammatory M2 phenotype, C. albicans can improve its ability to survive. It is interesting to note that C. albicans can hijack several PRR pathways. For instance, Candida-mediated activation of TLR2 can result in immunomodulatory signals that encourage the development of regulatory T cells and a tolerogenic DC phenotype (Reales-Calderón et al., 2014). Various virulent traits of C. albicans in causing the infection can be targeted and can be a better solution for the prevalence of RVVC. However, as a solution, the problem exists in the emergence of multidrug-resistant strains, showing resistance to existing treatment strategies.
4 Existing treatment strategies for VVC
Based on the severity of the infection, uncomplicated or complicated VVC, treatment strategies are classified into four different classes of antifungal agents. The various classes of antifungals clinically available include azoles, polyenes, echinocandins, and pyrimidine analogs (de Oliveira Santos et al., 2018) (Table 2). Azoles are heterocyclic compounds with ring structures containing at least one nitrogen and target the cytochrome P450 enzyme-lanosterol 14α-demethylase encoded by ERG11, producing ergosterol from lanosterol (de Oliveira Santos et al., 2018). Similarly, polyenes are polyunsaturated organic compounds containing at least three single and double carbon–carbon bonds, known to destroy the ergosterol content in the fungal cell membrane, leading to the loss of cell membrane permeability and causing antimycotic activity (Letscher-Bru, 2003).
On the other hand, echinocandins are amphiphilic lipopeptides that inhibit beta-1,3-glucan synthase encoded by FKS1 and FKS2 (Letscher-Bru, 2003). Pyrimidine analogues mimic the structure of natural pyrimidines, with a potential antimycotic ability by converting 5-fluorouracil to 5-fluoro deoxyuridine catalyzed by cytosine deaminase and thus interfere with the synthesis of DNA, RNA, and protein (Lee et al., 2021).
Like two sides of a coin, although antifungals are a solution, there also exists the problem of acquired resistance to such antifungals, which is mainly overseen (Table 2). The acquired resistance to antifungals refers to the capacity of C. albicans to evolve defense mechanisms that lessen their susceptibility to them or make them inactive. The molecular changes often involve mutations ranging from point mutations to chromosomal rearrangements. These mutations can have varied effects on drug resistance, from directly preventing the drug from binding to its target to causing gene expression alterations that encourage physiological conditions that increase drug resistance (Berman and Krysan, 2020). In recent years, there has been an increase in reports of the formation of drug-resistant strains, particularly those that become resistant to several different medications. Additionally, it has been shown that these resistant phenotypes can emerge during an illness and in response to therapy, posing even another risk to patients (Lee et al., 2021; Kalimuthu et al., 2022).
C. albicans adapt to various changes in the environment due to their high genetic flexibility. When exposed to antifungals, the yeast cell population undergoes a selection process that favors a group of cells with superior stress tolerance (Lee et al., 2021). The majority of acquired resistance mechanisms fall into two categories: (1) mutations that increase the target’s expression or change its affinity for the drug, and (2) mutations that decrease the amount of drug that accumulates intracellularly by either increasing the biological activity or overexpression of drug efflux pumps (Ksiezopolska and Gabaldón, 2018).
There are several ways C. albicans resist azoles, including (1) altering the biosynthesis of sterols to replace ergosterol, (2) overexpressing the target enzyme to increase activity in the presence of the antifungal medication, (3) overexpressing drug efflux pumps to lower the intracellular concentration of the drug, and (4) altering the target gene sequence to decrease the binding affinity. Point mutations in the ERG11 gene have been linked to the development of resistance in C. albicans. Interestingly, 21 of the 140 distinct point mutations for the ERG11 (lanosterol 14-alpha-demethylase) gene have been directly linked to fluconazole resistance. Also, suppression of the ERG3 gene expression confers azole resistance (Ksiezopolska and Gabaldón, 2018). Resistance to echinocandins is not as widespread as resistance to azoles, but it is far from uncommon and firmly correlated with prior medication exposure. By mutating specific spots in the FKS1 gene, C. albicans may circumvent the effects of echinocandins. Ergosterol levels in the cell membrane are typically associated with resistance to polyenes. It has been determined that mutations in the genes encoding the ergosterol synthesis-related enzymes ERG2, ERG3, ERG5, ERG6, and ERG11 are the cause of the lower abundance of the enzyme seen in polyene-resistant (Walker et al., 2013). Point mutations in the FCY1, FCY2, and FUR1 genes and C. albicans deficient to the genes FPS1 and FPS2 have all been linked to decreased susceptibility to flucytosine. Variations in FCY1 and FUR1 inactivate enzymes involved in the pyrimidine pathway and changes in FCY2 interfere with drug uptake. The absence of FPS1 and FPS2 lowered the drug accumulation in the cell (Chapeland-Leclerc et al., 2005). The emergence of resistance to these existing antifungals shows the need for next-generation antifungals with a novel mechanism of action.
5 Possible drug targets: finding a solution to VVC
In accordance with the clinically available treatment regimens, the exploration of medications in clinical trials for vaginal candidiasis encompasses various approaches. The investigation of potential treatments involves the study of Salvia officinalis, clotrimazole, and their combination (Ahangari et al., 2019). Similarly, the effectiveness of TOL-463 and ibrexafungerp in treating candidiasis is currently in the preliminary stages of research (Marrazzo et al., 2019; Azie et al., 2020). Additionally, researchers are specifically examining oteseconazole’s potential for managing recurrent VVC (Sobel et al., 2022). Furthermore, the exploration extends to boric acid as a potential therapeutic option for non-albicans yeast infections (Ray et al., 2007). However, with a restricted range of clinically accessible antifungals and ongoing challenges in developing novel antifungal classes, the emergence of antifungal resistance poses a persistent threat to the advancement of antifungal treatments. Multidrug-resistant fungus species have recently emerged, and their incidence has increased, spurring research into new treatments. The clinical limits of off-target effects and drug interactions make it an exciting option to optimize these pharmacological classes to increase fungal-specific, on-target effectiveness (Revie et al., 2018). Given the potential of spreading resistance, the scientific community and the pharmaceutical industry focus on developing antifungals with a novel mechanism of action against a fungal-specific pathway and potentiating the activity of the existing antifungals. Given that up to 80% of hits turn out to be false positives, discovering novel targets specific to fungus has proven difficult (Murphy and Bicanic, 2021).
Exploring possible therapeutic targets (Table 3) for candidiasis based on key pathways while considering biological functions and mechanisms is crucial (Spampinato and Leonardi, 2013). It is essential to identify therapeutic targets for candidiasis to treat candidiasis effectively. C. albicans central pathways that involve ergosterol biosynthesis, cell wall formation, mitochondrial function, signal transduction pathways, DNA replication and repair, and protein synthesis present promising drug targets for treatment. However, substantial experimental validation of their biological efficacy is required to ensure the effectiveness and safety of these pharmacological targets (Table 4) (Mazu et al., 2016).
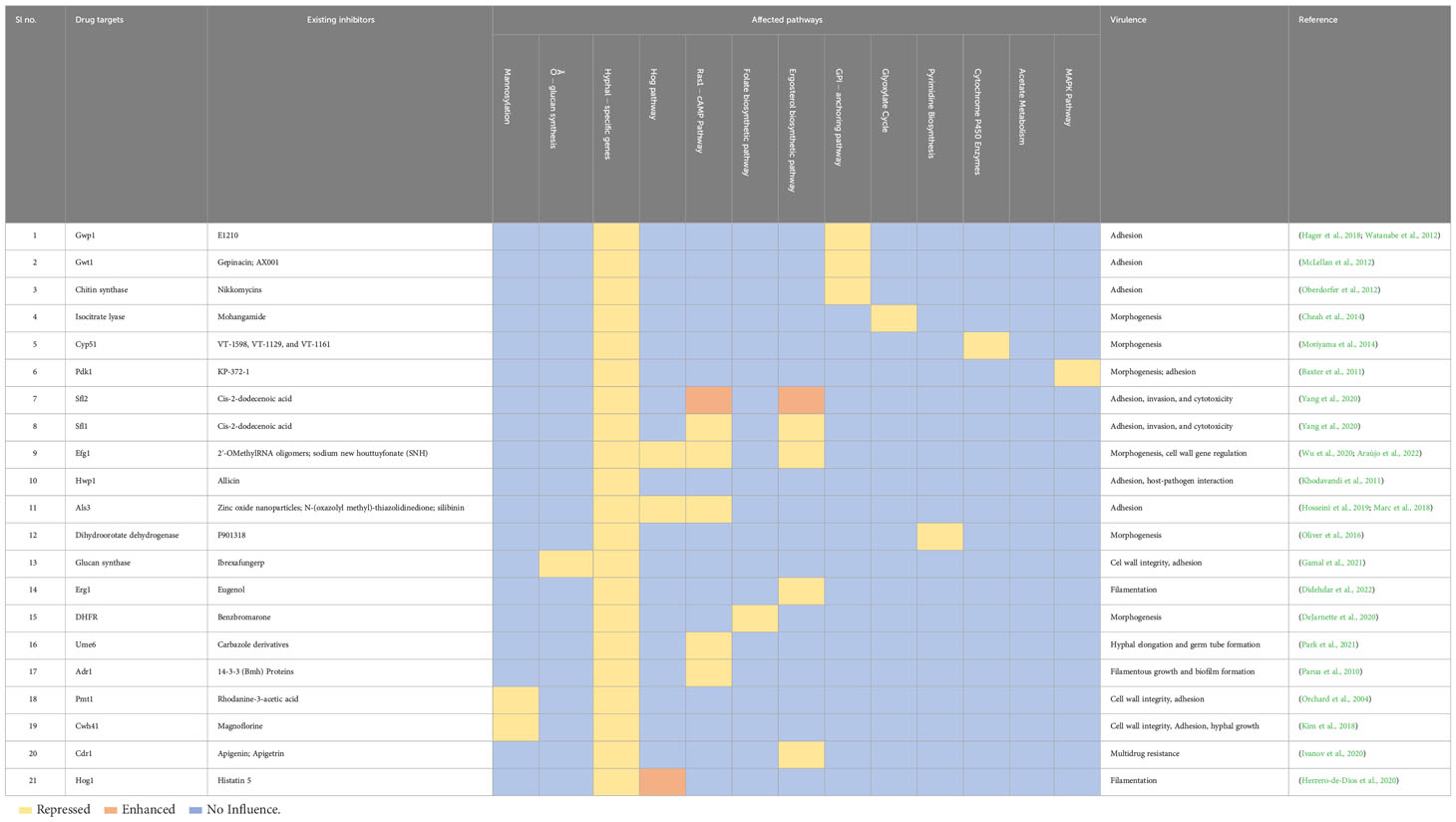
Table 4 Current inhibitors of the plausible pharmacological targets and biological pathways that are being directly and indirectly targeted. .
In the era of “antimicrobial resistance”, addressing candidiasis might entail a tactic of utilizing a combined treatment strategy involving specific anti-virulence/anti-infective agents alongside less effective antifungal drugs (Mota Fernandes et al., 2021). More specifically, the anti-infective/anti-virulence agents inhibit virulence traits without affecting the pathogen growth and, thus, do not exert selective pressure on the pathogen to develop resistance. Such anti-infective/anti-virulence drugs may potentiate the existing failed antifungals exhibiting a synergistic effect in fighting infections, an exciting backbone to develop next-generation antifungals. There are several strategies involved in the design and development of next-generation antifungal/anti-infective agents. Antifungal therapeutics from natural sources have drawn attention because they exhibit structural diversity and uniqueness in functional modes of action, making them desirable candidates to thwart the development of drug resistance (Seleem et al., 2017). Several such natural compounds, for example, piperine, cinnamaldehyde, berberine, and curcumin, are known to downregulate the virulence traits of C. albicans (Priya and Pandian, 2020; Yong et al., 2020; Rajasekar et al., 2021; Deng et al., 2022). Similarly, quorum-sensing molecules established to target biofilm formation and restore the pathogen’s susceptibility are also gaining attention. The sesquiterpene alcohol farnesol (C15H26O) was initially identified as a quorum-sensing molecule generated by C. albicans. Farnesol is produced by enzymatically phosphorylating farnesyl pyrophosphate, which inhibits the generation of hyphae in a concentration-dependent manner. The inhibition is achieved by negatively regulating the RAS1-cAMP-PKA pathway by targeting CYR1. Farnesol has recently become a prospective drug due to its antifungal efficacy (Nikoomanesh et al., 2023). Probiotic vaginal colonization, particularly with Lactobacillus sp., has also been widely studied and shown to have a significantly lower risk of VVC and to even treat VVC. Probiotics function by aiding in the vaginal microbiome’s restoration of balance and safeguarding against the overgrowth of C. albicans. They accomplish this by creating metabolic by-products that stop the growth of infections, such as organic acids, hydrogen peroxide, bacteriocins, and biosurfactants (Fuochi et al., 2019). In addition to probiotics, targeted antifungal therapy may be used as part of personalized medicine depending on the specific Candida strain infecting the patient and how they react to various antifungal medications. It deals with the problem of antimicrobial resistance and offers patients therapy options because of resistance to previous antifungal medications (Wu et al., 2022).
Another evolved strategy is the drug repurposing approach that is gaining importance in diseases like cancer and requires expansion in infectious diseases as well. It involves identifying new therapeutic applications for already approved, withdrawn, abandoned, and experimental drugs. It also has the added benefit of reducing the typical drug development period by up to 5–7 years (Ashburn and Thor, 2004). Notably, C. albicans are resistant to various classes of antifungal drugs available on the market, including the most recent ones (Pappas et al., 2016). Hence, combining the existing antifungals with newly developed anti-infectives can potentially overcome the problem of drug resistance, by acting through a different mechanism.
In summary, the next-generation antifungals are a novel class of antifungals with new targets with novel therapeutic indications, and old targets with a new therapeutic indication may be the probable first-line approaches (Bouz and Doležal, 2021). Furthermore, the development of a suitable drug delivery system is equally important in the process of drug development, which would increase the biological efficacy of the drugs, or else the nonspecific delivery leads to the accumulation of drugs in the body, causing increased toxicity.
6 Vaginal drug delivery systems
Systems for delivering organ (vagina)-specific medications offer a promising alternative for treating vaginal candidiasis (Pandey et al., 2020). Antifungal drugs, such as topical creams or oral tablets, are used in traditional treatment methods for vaginal candidiasis. These techniques might have limitations, like low patient compliance, systemic adverse effects, and the potential for antifungal resistance (Lírio et al., 2019). Advancements in drug delivery have an array of advantages over traditional approaches and the advancement is the direct delivery of antifungal drugs to the infection site, which enhances local drug concentrations while minimizing systemic exposure (Figure 2). Their focused and localized strategy and formulations with sustained release enhance therapy effectiveness, patient comfort, and acceptance (Garg et al., 2020). Drugs administered via vaginal routes are absorbed in three main ways: (1) transcellular, via concentration-dependent gradient; (2) paracellularly, through tight junctions present in between the cells; and (3) vesicular or receptor-mediated transport. The breakdown of drugs in the vaginal lumen and membrane penetration are the two primary phases in drug absorption from the vagina. Therefore, any factor affecting the physiology of the vagina and formulation elements like drug dissolution and membrane transport may change how a drug will be absorbed from vaginal drug delivery devices (Richardson and Illum, 1992). Different physiological factors influencing drug absorption in the vaginal cavity include physiological factors like epithelial thickness of the vagina, vaginal fluid, mucus, pH, and physiochemical factors like lipophilicity, molecular weight, solubility, and degree of ionization (Hussain and Ahsan, 2005).
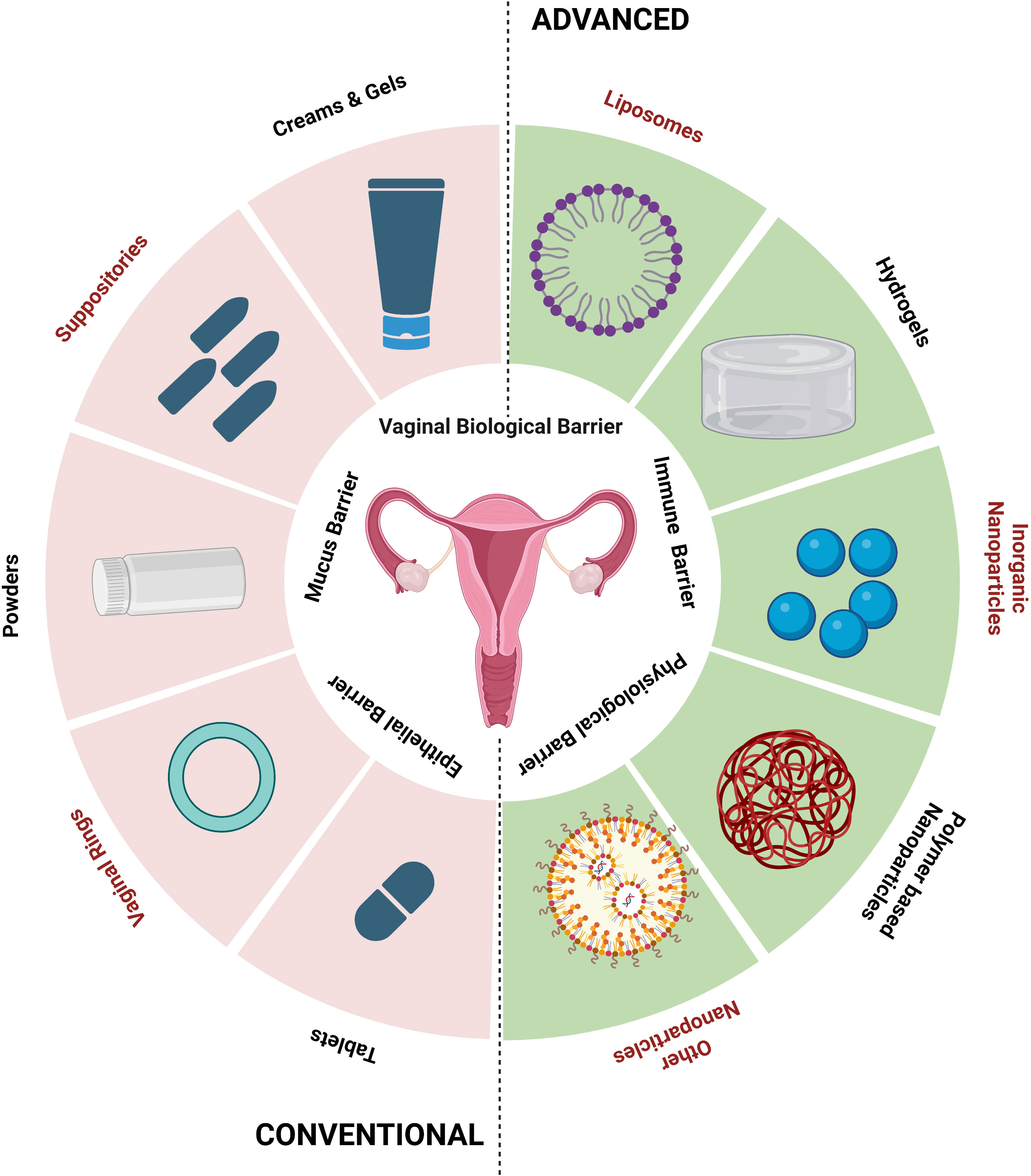
Figure 2 Conventional and advanced vaginal drug delivery systems (created using BioRender.com).
Using bio-adhesive, nanoparticle-based, and other new delivery systems for vaginal drug delivery is a novel concept (Table 5). Bio-adhesive formulations can speed up the healing of fungal infections by administering medications for a longer duration at a steady rate. Using time-release additives can achieve controlled-release medication delivery systems (Osmałek et al., 2021). Other new delivery techniques include phase change polymers, thermoplastic polymers, and mucoadhesive thermo-sensitive gels. Researchers have recently made advancements with hydrogels made with mucoadhesive polymers because of their capacity to interact with the mucus layer and epithelial cells, extending the duration of the drug’s residence time in the vaginal cavity and enhancing drug absorption, thereby achieving good patient compliance (Aka-Any-Grah et al., 2010; Fan et al., 2022).
Development of novel drugs with a suitable drug delivery system can only be successful from in vitro and, importantly, in vivo studies. Most of the drugs and treatment strategies fail in the preclinical validation. Thus, testing and proving these medications in appropriate animal models closely related to the human host can be a better way to reach the beneficiaries.
7 In vivo models for VVC
The evaluation of the efficacy and safety of a new drug candidate includes in vitro and in vivo studies that can be carried out throughout all stages of drug development. Studies in vitro concentrate on key factors that could affect medication release in vivo (Rayner et al., 2021). Basic knowledge of drug pharmacodynamics must be provided, and the selection and application of the right models, as well as accurate data interpretation, are crucial for decision-making and the successful advancement of drug candidates for clinical trials. Understanding a drug’s properties and effects on a living organism requires the use of in vivo investigations before the medicine is made available for purchase (Gallo, 2010).
The infectious mammalian models are used to understand the pathogenicity, pharmacokinetics, vaccination attempts involving immunization, and immune responses. Thus, mammalian species would seem most logical to simulate a human host; mice, rats, and rabbits are the earliest recognized animal models (Table 6) (Conti et al., 2014). Since the 19th century, these animal models have been explored for C. albicans-mediated vaginal candidiasis (Naglik et al., 2008; Conti et al., 2014; Cassone and Sobel, 2016). The mouse model exhibits various benefits, including its inexpensive cost, rapid reproduction, short generation time, and general acceptance in biological and genetic research. Although the mouse’s macroscopic anatomy differs from the humans, both have similar histological characteristics, cyclic estrus/menstrual cycles, and fundamental functions. Also, the murine vaginal microbiota is notably like humans, and although there may be differences between strains, Staphylococcus, Enterococcus, and Lactobacillus appear to be present consistently (Grasso et al., 1998; Vrbanac et al., 2018). Similarly, like the mouse model, rats have the potential to be an effective vaginal model since they are simple to procure, are inexpensive, and have a reproductive cycle that is similar to that of humans. Wistar and Sprague Dawley female rats are used in many current studies to study the vaginal environment. While the estrus cycle affects the vaginal flora in rats like that in humans, certain bacteria, such as Gram-negative rods, Streptococci, and members of the Bacteroidaceae family, may not be present. Generally, a less diversified vaginal microbiome is thought to be more stable and, therefore, healthier in people (Moalli et al., 2005; Levy et al., 2020).
For FDA-mandated preclinical assessments of vaginal irritation, the rabbit serves as the gold standard model; as a result, these investigations frequently use rabbit vaginal tissue models. European (O. cuniculus) and New Zealand White rabbits are frequent breeds used in these experiments. Compared to some other animal models, the rabbit’s reproductive system and vaginal environment are similar to those of humans, making it a valuable model for researching vaginal candidiasis. Also, the microbial composition of the rabbit’s vagina is like that of humans (Acartürk and Robinson, 1996; Shi et al., 2022). Other large animals like rhesus macaques (Macaca mulatta) are also being used for vaginal infection studies. However, these models’ cost, maintenance, and ethical clearance make it difficult (Steele et al., 1999).
8 Conclusion and future prospects
Establishing efficient therapies for VVC requires a thorough understanding of the risk factors, pathophysiology, and treatment that can limit the resistance generated. To overcome drug resistance and improve the results for people with VVC, it may be necessary to identify new therapeutic targets and investigate suitable drug delivery modalities. Furthermore, personalized medicine approaches hold promise in the management of VVC. Considering individual genetic susceptibility and host-specific factors, tailored treatment strategies can be developed to improve therapeutic outcomes. The therapeutic measures may involve probiotics, immunomodulatory agents, or combination therapies targeting multiple virulence factors and associated pathways without developing resistance.
Author contributions
HD: Drafted the article or revised it critically for important intellectual content, which is part of her doctoral studies. AS: Approved the version to be published and agreed to be accountable for all aspects of the work in ensuring that questions related to the accuracy or integrity of any part of the work are appropriately investigated and resolved. All authors contributed to the article and approved the submitted version.
Acknowledgments
The authors are grateful to SASTRA University for providing us with an excellent infrastructure and being a part of the Quorum Sensing Lab (QSL) and team AI at ABLEST for finding novel solutions to women-associated health risks.
Conflict of interest
The authors declare that the research was conducted in the absence of any commercial or financial relationships that could be construed as a potential conflict of interest.
Publisher’s note
All claims expressed in this article are solely those of the authors and do not necessarily represent those of their affiliated organizations, or those of the publisher, the editors and the reviewers. Any product that may be evaluated in this article, or claim that may be made by its manufacturer, is not guaranteed or endorsed by the publisher.
References
Abdellatif, M. M., Khalil, I. A., Elakkad, Y. E., Eliwa, H. A., Samir, T., Al-Mokaddem, A. K. (2020). Formulation and characterization of sertaconazole nitrate mucoadhesive liposomes for vaginal candidiasis. Int. J. Nanomed. Vol. 15, 4079–4090. doi: 10.2147/IJN.S250960
Acartürk, F., Robinson, J. R. (1996). Vaginal permeability and enzymatic activity studies in normal and ovariectomized rabbits. Pharm. Res. 13, 779–783. doi: 10.1023/A:1016016120392
Aguin, T. J., Sobel, J. D. (2015). Vulvovaginal candidiasis in pregnancy. Curr. Infect. Dis. Rep. 17, 30. doi: 10.1007/s11908-015-0462-0
Ahangari, F., Farshbaf-Khalili, A., Javadzadeh, Y., Adibpour, M., Sadeghzadeh Oskouei, B. (2019). Comparing the effectiveness of Salvia officinalis, clotrimazole and their combination on vulvovaginal candidiasis: A randomized, controlled clinical trial. J. Obstet. Gynaecol. Res. 45, 897–907. doi: 10.1111/jog.13918
Aka-Any-Grah, A., Bouchemal, K., Koffi, A., Agnely, F., Zhang, M., Djabourov, M., et al. (2010). Formulation of mucoadhesive vaginal hydrogels insensitive to dilution with vaginal fluids. Eur. J. Pharmaceut. Biopharmaceut. 76, 296–303. doi: 10.1016/j.ejpb.2010.07.004
Albash, R., Elmahboub, Y., Baraka, K., Abdellatif, M. M., Alaa-Eldin, A. A. (2020). Ultra-deformable liposomes containing terpenes (terpesomes) loaded fenticonazole nitrate for treatment of vaginal candidiasis: Box-Behnken design optimization, comparative ex vivo and in vivo studies. Drug Delivery 27, 1514–1523. doi: 10.1080/10717544.2020.1837295
Albrecht, A., Felk, A., Pichova, I., Naglik, J. R., Schaller, M., de Groot, P., et al. (2006). Glycosylphosphatidylinositol-anchored proteases of Candida albicans target proteins necessary for both cellular processes and host-pathogen interactions. J. Biol. Chem. 281, 688–694. doi: 10.1074/jbc.M509297200
Ali, A., Azab, M., Abdelrahman, A. (2018). Distribution of secreted aspartyl protease (SAP) virulence genes and antifungal resistance genes at vulvovaginal candidiasis isolates. GSC Biol. Pharm. Sci. 5, 086–094. doi: 10.30574/gscbps.2018.5.3.0149
Antley, P. P., Hazen, K. C. (1988). Role of yeast cell growth temperature on Candida albicans virulence in mice. Infect. Immun. 56, 2884–2890. doi: 10.1128/iai.56.11.2884-2890.1988
Araújo, D., Mil-Homens, D., Henriques, M., Silva, S. (2022). Anti-EFG1 2′-OMethylRNA oligomer inhibits Candida albicans filamentation and attenuates the candidiasis in Galleria mellonella. Mol. Ther. Nucleic Acids 27, 517–523. doi: 10.1016/j.omtn.2021.12.018
Ashburn, T. T., Thor, K. B. (2004). Drug repositioning: Identifying and developing new uses for existing drugs. Nat. Rev. Drug Discovery 3, 673–683. doi: 10.1038/nrd1468
Atriwal, T., Azeem, K., Husain, F. M., Hussain, A., Khan, M. N., Alajmi, M. F., et al. (2021). Mechanistic understanding of Candida albicans biofilm formation and approaches for its inhibition. Front. Microbiol. 12. doi: 10.3389/fmicb.2021.638609
Azie, N., Angulo, D., Dehn, B., Sobel, J. D. (2020). Oral Ibrexafungerp: an investigational agent for the treatment of vulvovaginal candidiasis. Expert Opin. Investig. Drugs 29, 893–900. doi: 10.1080/13543784.2020.1791820
Bassyouni, R. H., Wegdan, A. A., Abdelmoneim, A., Said, W., AboElnaga, F. (2015). Phospholipase and aspartyl proteinase activities of Candida Species causing vulvovaginal candidiasis in patients with Type 2 Diabetes Mellitus. J. Microbiol. Biotechnol. 25, 1734–1741. doi: 10.4014/jmb.1504.04009
Baxter, B. K., DiDone, L., Ogu, D., Schor, S., Krysan, D. J. (2011). Identification, in vitro activity and mode of action of phosphoinositide-dependent-1 kinase inhibitors as antifungal molecules. ACS Chem. Biol. 6, 502–510. doi: 10.1021/cb100399x
Bellotti, D., D’Accolti, M., Pula, W., Huang, N., Simeliere, F., Caselli, E., et al. (2023). Calcitermin-loaded smart gels activity against Candida albicans: A preliminary in vitro study. Gels 9, 165. doi: 10.3390/gels9020165
Bencova, A., Goffa, E., Morvova, M., Valachovic, M., Griač, P., Toth Hervay, N., et al. (2020). The absence of PDR16 gene restricts the overexpression of CaSNQ2 gene in the presence of fluconazole in Candida albicans. Mycopathologia 185, 455–465. doi: 10.1007/s11046-020-00459-4
Berman, J., Krysan, D. J. (2020). Drug resistance and tolerance in fungi. Nat. Rev. Microbiol. 18, 319–331. doi: 10.1038/s41579-019-0322-2
Bezerra, C. F., de Alencar Júnior, J. G., de Lima Honorato, R., dos Santos, A. T. L., Pereira da Silva, J. C., Gusmão da Silva, T., et al. (2020). Antifungal activity of farnesol incorporated in liposomes and associated with fluconazole. Chem. Phys. Lipids 233, 104987. doi: 10.1016/j.chemphyslip.2020.104987
Bhattacharya, S., Sae-Tia, S., Fries, B. C. (2020). Candidiasis and mechanisms of antifungal resistance. Antibiotics 9, 312. doi: 10.3390/antibiotics9060312
Bhattacharya, S., Sobel, J. D., White, T. C. (2016). A combination fluorescence assay demonstrates increased efflux pump activity as a resistance mechanism in azole-resistant vaginal Candida albicans Isolates. Antimicrob. Agents Chemother. 60, 5858–5866. doi: 10.1128/AAC.01252-16
Biswas, S., Van Dijck, P., Datta, A. (2007). Environmental sensing and signal transduction pathways regulating morpho pathogenic determinants of Candida albicans. Microbiol. Mol. Biol. Rev. 71, 348–376. doi: 10.1128/MMBR.00009-06
Bouz, G., Doležal, M. (2021). Advances in antifungal drug development: An up-to-date mini review. Pharmaceuticals 14, 1312. doi: 10.3390/ph14121312
Branzk, N., Lubojemska, A., Hardison, S. E., Wang, Q., Gutierrez, M. G., Brown, G. D., et al. (2014). Neutrophils sense microbe size and selectively release neutrophil extracellular traps in response to large pathogens. Nat. Immunol. 15, 1017–1025. doi: 10.1038/ni.2987
Brotman, R. M., Shardell, M. D., Gajer, P., Fadrosh, D., Chang, K., Silver, M. I., et al. (2014). Association between the vaginal microbiota, menopause status, and signs of vulvovaginal atrophy. Menopause 21, 450–458. doi: 10.1097/GME.0b013e3182a4690b
Bruno, V. M., Mitchell, A. P. (2005). Regulation of azole drug susceptibility by Candida albicans protein kinase CK2. Mol. Microbiol. 56, 559–573. doi: 10.1111/j.1365-2958.2005.04562.x
Calderon, J., Zavrel, M., Ragni, E., Fonzi, W. A., Rupp, S., Popolo, L. (2010). PHR1, a pH-regulated gene of Candida albicans encoding a glucan-remodeling enzyme, is required for adhesion and invasion. Microbiol. (N Y) 156, 2484–2494. doi: 10.1099/mic.0.038000-0
Calvo, N. L., Sreekumar, S., Svetaz, L. A., Lamas, M. C., Moerschbacher, B. M., Leonardi, D. (2019). Design and characterization of chitosan nanoformulations for the delivery of antifungal agents. Int. J. Mol. Sci. 20, 3686. doi: 10.3390/ijms20153686
Cambi, A., Netea, M. G., Mora-Montes, H. M., Gow, N. A. R., Hato, S. V., Lowman, D. W., et al. (2008). Dendritic cell interaction with Candida albicans critically depends on N-linked mannan. J. Biol. Chem. 283, 20590–20599. doi: 10.1074/jbc.M709334200
Cantero, P. D., Ernst, J. F. (2011). Damage to the glycoshield activates PMT-directed O-mannosylation via the Msb2-Cek1 pathway in Candida albicans. Mol. Microbiol. 80, 715–725. doi: 10.1111/j.1365-2958.2011.07604.x
Carbone, C., Fuochi, V., Zielińska, A., Musumeci, T., Souto, E. B., Bonaccorso, A., et al. (2020). Dual-drugs delivery in solid lipid nanoparticles for the treatment of Candida albicans mycosis. Colloids Surf B Biointerfaces 186, 110705. doi: 10.1016/j.colsurfb.2019.110705
Cassone, A., Sobel, J. D. (2016). Experimental models of vaginal candidiasis and their relevance to human candidiasis. Infect. Immun. 84, 1255–1261. doi: 10.1128/IAI.01544-15
Cavalheiro, M., Teixeira, M. C. (2018). Candida biofilms: threats, challenges, and promising strategies. Front. Med. (Lausanne) 5. doi: 10.3389/fmed.2018.00028
Cevher, E., Açma, A., Sinani, G., Aksu, B., Zloh, M., Mülazımoğlu, L. (2014). Bioadhesive tablets containing cyclodextrin complex of itraconazole for the treatment of vaginal candidiasis. Int. J. Biol. Macromol 69, 124–136. doi: 10.1016/j.ijbiomac.2014.05.033
Chapeland-Leclerc, F., Bouchoux, J., Goumar, A., Chastin, C., Villard, J., Noeül, T. (2005). Inactivation of the FCY2 gene encoding purine-cytosine permease promotes cross-resistance to flucytosine and fluconazole in Candida lusitaniae. Antimicrob. Agents Chemother. 49, 3101–3108. doi: 10.1128/AAC.49.8.3101-3108.2005
Cheah, H.-L., Lim, V., Sandai, D. (2014). Inhibitors of the glyoxylate cycle enzyme ICL1 in Candida albicans for potential use as antifungal agents. PloS One 9, e95951. doi: 10.1371/journal.pone.0095951
Cheng, S., Clancy, C. J., Xu, W., Schneider, F., Hao, B., Mitchell, A. P., et al. (2013). Profiling of Candida albicans gene expression during intra-abdominal candidiasis identifies biologic processes involved in pathogenesis. J. Infect. Dis. 208, 1529–1537. doi: 10.1093/infdis/jit335
Chien, C.-T., Chen, Y.-C., Liu, Y.-C., Liang, S.-H., Lin, H.-H., Lin, C.-H. (2019). The antimicrobial photodynamic inactivation resistance of Candida albicans is modulated by the Hog1 pathway and the Cap1 transcription factor. Med. Mycol 57, 618–627. doi: 10.1093/mmy/myy079
Conti, H. R., Huppler, A. R., Whibley, N., Gaffen, S. L. (2014). Animal models for candidiasis. Curr. Protoc. Immunol. 105, 19–6. doi: 10.1002/0471142735.im1906s105
Correia, A., Lermann, U., Teixeira, L., Cerca, F., Botelho, S., Gil da Costa, R. M., et al. (2010). Limited role of secreted aspartyl proteinases Sap1 to Sap6 in Candida albicans virulence and host immune response in murine hematogenously disseminated candidiasis. Infect. Immun. 78, 4839–4849. doi: 10.1128/IAI.00248-10
Cowen, L. E. (2008). The evolution of fungal drug resistance: modulating the trajectory from genotype to phenotype. Nat. Rev. Microbiol. 6, 187–198. doi: 10.1038/nrmicro1835
Czechowicz, P., Nowicka, J., Gościniak, G. (2022). Virulence factors of Candida spp. and host immune response important in the pathogenesis of vulvovaginal candidiasis. Int. J. Mol. Sci. 23, 5895. doi: 10.3390/ijms23115895
Davis, D., Wilson, R. B., Mitchell, A. P. (2000). RIM101 -dependent and -independent pathways govern pH responses in Candida albicans. Mol. Cell Biol. 20, 971–978. doi: 10.1128/MCB.20.3.971-978.2000
Dean, N., Jones, R., DaSilva, J., Chionchio, G., Ng, H. (2022). The Mnn10/Anp1-dependent N -linked outer chain glycan is dispensable for Candida albicans cell wall integrity. Genetics 221. doi: 10.1093/genetics/iyac048
de Araújo, P. R., Calixto, G. M. F., Araújo, V. H. S., Sato, M. R., Rodero, C. F., Oshiro-Junior, J. A., et al. (2021). In vivo study of hypericin-loaded poloxamer-based mucoadhesive in situ gelling liquid crystalline precursor system in a mice model of vulvovaginal candidiasis. Med. Mycol 59, 821–827. doi: 10.1093/mmy/myab006
de Bastiani, F. W. M., Spadari, C., de Matos, J. K. R., Salata, G. C., Lopes, L. B., Ishida, K. (2020). Nanocarriers provide sustained antifungal activity for amphotericin B and miltefosine in the topical treatment of murine vaginal candidiasis. Front. Microbiol. 10. doi: 10.3389/fmicb.2019.02976
DeJarnette, C., Luna-Tapia, A., Estredge, L. R., Palmer, G. E. (2020). Dihydrofolate reductase is a valid target for antifungal development in the human pathogen candida albicans. mSphere 5. doi: 10.1128/mSphere.00374-20
d’Enfert, C., Kaune, A.-K., Alaban, L.-R., Chakraborty, S., Cole, N., Delavy, M., et al. (2021). The impact of the fungus-host-microbiota interplay upon Candida albicans infections: current knowledge and new perspectives. FEMS Microbiol. Rev. 45. doi: 10.1093/femsre/fuaa060
Deng, K., Jiang, W., Jiang, Y., Deng, Q., Cao, J., Yang, W., et al. (2021). ALS3 expression as an indicator for Candida albicans biofilm formation and drug resistance. Front. Microbiol. 12. doi: 10.3389/fmicb.2021.655242
Deng, J.-H., Zhang, X.-G., Wang, G.-S., Luo, J.-N., Wang, J., Qi, X.-M., et al. (2022). Effect of cinnamaldehyde on C. albicans cell wall and (1,3)- β – D-glucans in vivo. BMC Complement Med. Ther. 22, 32. doi: 10.1186/s12906-021-03468-y
Dennerstein, G. J., Ellis, D. H. (2001). Oestrogen, glycogen and vaginal candidiasis. Aust. N Z J. Obstet Gynaecol 41, 326–328. doi: 10.1111/j.1479-828X.2001.tb01238.x
Denning, D. W., Kneale, M., Sobel, J. D., Rautemaa-Richardson, R. (2018). Global burden of recurrent vulvovaginal candidiasis: a systematic review. Lancet Infect. Dis. 18 (11), e339–e347. doi: 10.1016/S1473-3099(18)30103-8
de Oliveira Neto, A. S., Souza, I. L. A., Amorim, M. E. S., de Freitas Souza, T., Rocha, V. N., do Couto, R. O., et al. (2021). Antifungal efficacy of atorvastatin-containing emulgel in the treatment of oral and vulvovaginal candidiasis. Med. Mycol 59, 476–485. doi: 10.1093/mmy/myaa071
de Oliveira Santos, G. C., Vasconcelos, C. C., Lopes, A. J. O., de Sousa Cartágenes, M., do, S., Filho, A. K. D. B., et al. (2018). Candida infections and therapeutic strategies: mechanisms of action for traditional and alternative agents. Front. Microbiol. 9. doi: 10.3389/fmicb.2018.01351
Didehdar, M., Chegini, Z., Shariati, A. (2022). Eugenol: A novel therapeutic agent for the inhibition of Candida species infection. Front. Pharmacol. 13. doi: 10.3389/fphar.2022.872127
Donders, G., Bellen, G., Ausma, J., Verguts, L., Vaneldere, J., Hinoul, P., et al. (2011). The effect of antifungal treatment on the vaginal flora of women with vulvo-vaginal yeast infection with or without bacterial vaginosis. Eur. J. Clin. Microbiol. Infect. Dis. 30, 59–63. doi: 10.1007/s10096-010-1052-6
Dubey, R., Imthurn, B., Barton, M., Jackson, E. (2005). Vascular consequences of menopause and hormone therapy: Importance of timing of treatment and type of estrogen. Cardiovasc. Res. 66, 295–306. doi: 10.1016/j.cardiores.2004.12.012
Du Clos, T. W. (2013). Pentraxins: structure, function, and role in inflammation. ISRN Inflammation 2013, 1–22. doi: 10.1155/2013/379040
Dutton, L. C., Nobbs, A. H., Jepson, K., Jepson, M. A., Vickerman, M. M., Aqeel Alawfi, S., et al. (2014). O -Mannosylation in Candida albicans enables development of interkingdom biofilm communities. mBio 5, 10–1128. doi: 10.1128/mBio.00911-14
Engku Nasrullah Satiman, E. A. F., Ahmad, H., Ramzi, A. B., Abdul Wahab, R., Kaderi, M. A., Wan Harun, W. H. A., et al. (2020). The role of Candida albicans candidalysin ECE1 gene in oral carcinogenesis. J. Oral. Pathol. Med. 49, 835–841. doi: 10.1111/jop.13014
FacChinatto, W. M., Galante, J., Mesquita, L., Silva, D. S., Martins dos Santos, D., Moraes, T. B., et al. (2021). Clotrimazole-loaded N-(2-hydroxy)-propyl-3-trimethylammonium, O-palmitoyl chitosan nanoparticles for topical treatment of vulvovaginal candidiasis. Acta Biomater 125, 312–321. doi: 10.1016/j.actbio.2021.02.029
Fan, R., Cheng, Y., Wang, R., Zhang, T., Zhang, H., Li, J., et al. (2022). Thermosensitive hydrogels and advances in their application in disease therapy. Polymers (Basel) 14, 2379. doi: 10.3390/polym14122379
Farhan, M. A., Moharram, A. M., Salah, T., Shaaban, O. M. (2019). Types of yeasts that cause vulvovaginal candidiasis in chronic users of corticosteroids. Med. Mycol 57, 681–687. doi: 10.1093/mmy/myy117
Fidel, P. L., Cutright, J., Steele, C. (2000). Effects of reproductive hormones on experimental vaginal candidiasis. Infect. Immun. 68, 651–657. doi: 10.1128/IAI.68.2.651-657.2000
Fisher, M. C., Denning, D. W. (2023). The WHO fungal priority pathogens list as a game-changer. Nat. Rev. Microbiol. 21, 211–212. doi: 10.1038/s41579-023-00861-x
Fonzi, W. A. (1999). PHR1 and PHR2 of Candida albicans encode putative glycosidases required for proper cross-linking of β-1,3- and β-1,6-Glucans. J. Bacteriol 181, 7070–7079. doi: 10.1128/JB.181.22.7070-7079.1999
Fuochi, V., Cardile, V., Petronio, G., Furneri, P. M. (2019). Biological properties and production of bacteriocins-like-inhibitory substances by Lactobacillus sp. strains from human vagina. J. Appl. Microbiol. 126, 1541–1550. doi: 10.1111/jam.14164
Gallo, J. M. (2010). Pharmacokinetic/pharmacodynamic-driven drug development. Mount Sinai J. Medicine: A J. Trans. Personalized Med. 77, 381–388. doi: 10.1002/msj.20193
Gamal, A., Chu, S., McCormick, T. S., Borroto-Esoda, K., Angulo, D., Ghannoum, M. A. (2021). Ibrexafungerp, a Novel Oral Triterpenoid Antifungal in Development: Overview of Antifungal Activity Against Candida glabrata. Front Cell Infect Microbiol. 11. doi: 10.3389/fcimb.2021.642358
Garg, A., Sharma, G. S., Goyal, A. K., Ghosh, G., Si, S. C., Rath, G. (2020). Recent advances in topical carriers of anti-fungal agents. Heliyon 6, e04663. doi: 10.1016/j.heliyon.2020.e04663
Ghannoum, M. A. (2000). Potential role of phospholipases in virulence and fungal pathogenesis. Clin. Microbiol. Rev. 13, 122–143. doi: 10.1128/CMR.13.1.122-143.2000
Glazier, V. E. (2022). EFG1, everyone’s favorite gene in Candida albicans: A comprehensive literature review. Front. Cell Infect. Microbiol. 12. doi: 10.3389/fcimb.2022.855229
Glocker, E.-O., Hennigs, A., Nabavi, M., Schäffer, A. A., Woellner, C., Salzer, U., et al. (2009). A homozygous CARD9 mutation in a family with susceptibility to fungal infections. New Engl. J. Med. 361, 1727–1735. doi: 10.1056/NEJMoa0810719
Gómez-Gaviria, M., Vargas-Macías, A. P., García-Carnero, L. C., Martínez-Duncker, I., Mora-Montes, H. M. (2021). Role of protein glycosylation in interactions of medically relevant fungi with the host. J. Fungi 7, 875. doi: 10.3390/jof7100875
Gonçalves, B., Ferreira, C., Alves, C. T., Henriques, M., Azeredo, J., Silva, S. (2016). Vulvovaginal candidiasis: Epidemiology, microbiology and risk factors. Crit. Rev. Microbiol. 42, 905–927. doi: 10.3109/1040841X.2015.1091805
Grasso, P., Rozhavskaya, M., Reichert, L. E. (1998). In vivo effects of human follicle-stimulating hormone-related synthetic peptide hFSH-,β-(81–95) and its subdomain hFSH-β-(90–95) on the mouse estrous cycle. Biol. Reprod. 58, 821–825. doi: 10.1095/biolreprod58.3.821
Guimarães, T., Nucci, M., Mendonça, J. S., Martinez, R., Brito, L. R., Silva, N., et al. (2012). Epidemiology and predictors of a poor outcome in elderly patients with candidemia. Int. J. Infect. Dis. 16, e442–e447. doi: 10.1016/j.ijid.2012.02.005
Guinea, J. (2014). Global trends in the distribution of Candida species causing candidemia. Clin. Microbiol. Infect. 20, 5–10. doi: 10.1111/1469-0691.12539
Gürsoy, S., Koçkar, T., Atik, S. U., Önal, Z., Önal, H., Adal, E. (2018). Autoimmunity and intestinal colonization by Candida albicans in patients with type 1 diabetes at the time of the diagnosis. Korean J. Pediatr. 61, 217–220. doi: 10.3345/kjp.2018.61.7.217
Hager, C. L., Larkin, E. L., Long, L., Zohra Abidi, F., Shaw, K. J., Ghannoum, M. A. (2018). In Vitro and In Vivo Evaluation of the antifungal activity of apx001a/apx001 against candida auris. Antimicrob. Agents Chemother. 62. doi: 10.1128/AAC.02319-17
Hameed, A., Hussain1, S. A., Ijaz, M. U., Umer, M. (2020). Deletions of the Idh1, Eco1, Rom2, and Taf10 Genes Differently Control the Hyphal Growth, Drug Tolerance, and Virulence of Candida albicans. Folia Biologica (Praha). 66 91-103 66, 91–103.
Hani, U., Shivakumar, H. G., Osmani, R. A. M., Srivastava, A., Kumar Varma, N. S. (2016). Development of a curcumin bioadhesive monolithic tablet for treatment of vaginal candidiasis. Iran J. Pharm. Res. 15, 23–34.
Hanumantha Rao, K., Paul, S., Ghosh, S. (2021). N-acetylglucosamine signaling: Transcriptional dynamics of a novel sugar sensing cascade in a model pathogenic yeast, Candida albicans. J. Fungi 7, 65. doi: 10.3390/jof7010065
Herrero-de-Dios, C., Román, E., Pla, J., Alonso-Monge, R. (2020). Hog1 Controls Lipids Homeostasis Upon Osmotic Stress in Candida albicans. J. Fungi 6, 355. doi: 10.3390/jof6040355
Ho, J., Yang, X., Nikou, S. A., Kichik, N., Donkin, A., Ponde, N. O., et al. (2019). Candidalysin activates innate epithelial immune responses via epidermal growth factor receptor. Nat. Commun. 10, 2297. doi: 10.1038/s41467-019-09915-2
Hosseini, S. S., Ghaemi, E., Noroozi, A., Niknejad, F. (2019). Zinc oxide nanoparticles inhibition of initial adhesion and ALS1 and ALS3 gene expression in Candida albicans strains from urinary tract infections. Mycopathologia 184, 261–271. doi: 10.1007/s11046-019-00327-w
Hosseini Bafghi, M., Zarrinfar, H., Darroudi, M., Zargar, M., Nazari, R. (2022). Green synthesis of selenium nanoparticles and evaluate their effect on the expression of ERG3, ERG11 and FKS1 antifungal resistance genes in Candida albicans and Candida glabrata. Lett. Appl. Microbiol. 74, 809–819. doi: 10.1111/lam.13667
Hoyer, L. L., Cota, E. (2016). Candida albicans Agglutinin-Like Sequence (Als) family vignettes: A review of Als protein structure and function. Front. Microbiol. 7. doi: 10.3389/fmicb.2016.00280
Hussain, A., Ahsan, F. (2005). The vagina as a route for systemic drug delivery. J. Controlled Release 103, 301–313. doi: 10.1016/j.jconrel.2004.11.034
Ifrim, D. C., Bain, J. M., Reid, D. M., Oosting, M., Verschueren, I., Gow, N. A. R., et al. (2014). Role of Dectin-2 for host defense against systemic infection with Candida glabrata. Infect. Immun. 82, 1064–1073. doi: 10.1128/IAI.01189-13
Ivanov, M., Kannan, A., Stojković, D. S., Glamočlija, J., Calhelha, R. C., Ferreira, I. C. F. R., et al. (2020). Flavones, flavonols, and glycosylated derivatives—Impact on Candida albicans growth and virulence, Expression of CDR1 and ERG11, cytotoxicity. Pharmaceuticals 14, 27. doi: 10.3390/ph14010027
Jin, X., Luan, X., Xie, F., Chang, W., Lou, H. (2023). Erg6 acts as a downstream effector of the transcription factor Flo8 to regulate biofilm formation in Candida albicans. Microbiol. Spectr. doi: 10.1128/spectrum.00393-23
Kalimuthu, S., Alshanta, O. A., Krishnamoorthy, A. L., Pudipeddi, A., Solomon, A. P., McLean, W., et al. (2022). Small molecule based anti-virulence approaches against Candida albicans infections. Crit. Rev. Microbiol. 48, 743–769. doi: 10.1080/1040841X.2021.2025337
Karasulu, H. Y., Hilmioğlu, S., Metin, D. Y., Güneri, T. (2004). Efficacy of a new ketoconazole bioadhesive vaginal tablet on Candida albicans. Il Farmaco 59, 163–167. doi: 10.1016/j.farmac.2003.11.018
Kebaara, B. W., Langford, M. L., Navarathna, D. H. M. L. P., Dumitru, R., Nickerson, K. W., Atkin, A. L. (2008). Candida albicans Tup1 is involved in farnesol-mediated inhibition of filamentous-growth induction. Eukaryot Cell 7, 980–987. doi: 10.1128/EC.00357-07
Kenechukwu, F. C., Momoh, M. A., Nnamani, P. O., Umeyor, C. E., Uronnachi, E. M., Dias, M. L., et al. (2022). Dual-responsive micellar microgels matrixed with surface-engineered lipids: a new approach for controlled vaginal drug delivery. J. Pharm. Innov. 17, 821–839. doi: 10.1007/s12247-021-09546-5
Komalapriya, C., Kaloriti, D., Tillmann, A. T., Yin, Z., Herrero-de-Dios, C., Jacobsen, M. D., et al. (2015). Integrative model of oxidative stress adaptation in the fungal pathogen Candida albicans. PloS One 10, e0137750. doi: 10.1371/journal.pone.0137750
Khodavandi, A., Harmal, N. S., Alizadeh, F., Scully, O. J., Sidik, S. M., Othman, F., et al. (2011). Comparison between allicin and fluconazole in Candida albicans biofilm inhibition and in suppression of HWP1 gene expression. Phytomedicine 19, 56–63. doi: 10.1016/j.phymed.2011.08.060
Kim, J., Ha Quang Bao, T., Shin, Y.-K., Kim, K.-Y. (2018). Antifungal activity of magnoflorine against Candida strains. World J. Microbiol. Biotechnol. 34, 167. doi: 10.1007/s11274-018-2549-x
Ksiezopolska, E., Gabaldón, T. (2018). Evolutionary emergence of drug resistance in Candida opportunistic pathogens. Genes (Basel) 9, 461. doi: 10.3390/genes9090461
Kumwenda, P., Cottier, F., Hendry, A. C., Kneafsey, D., Keevan, B., Gallagher, H., et al. (2022). Estrogen promotes innate immune evasion of Candida albicans through inactivation of the alternative complement system. Cell Rep. 38, 110183. doi: 10.1016/j.celrep.2021.110183
Lee, Y., Puumala, E., Robbins, N., Cowen, L. E. (2021). Antifungal drug resistance: Molecular mechanisms in Candida albicans and beyond. Chem. Rev. 121, 3390–3411. doi: 10.1021/acs.chemrev.0c00199
Lesage, G., Bussey, H. (2006). Cell wall assembly in Saccharomyces cerevisiae. Microbiol. Mol. Biol. Rev. 70, 317–343. doi: 10.1128/MMBR.00038-05
Letscher-Bru, V. (2003). Caspofungin: the first representative of a new antifungal class. J. Antimicrobial Chemother. 51, 513–521. doi: 10.1093/jac/dkg117
Levy, M., Bassis, C. M., Kennedy, E., Yoest, K. E., Becker, J. B., Bell, J., et al. (2020). The rodent vaginal microbiome across the estrous cycle and the effect of genital nerve electrical stimulation. PloS One 15, e0230170. doi: 10.1371/journal.pone.0230170
Li, J., Coste, A. T., Liechti, M., Bachmann, D., Sanglard, D., Lamoth, F. (2021). Novel ERG11 and TAC1b mutations associated with azole resistance in Candida auris. Antimicrob. Agents Chemother. 65, 10–128. doi: 10.1128/AAC.02663-20
Li, W.-J., Liu, J.-Y., Shi, C., Zhao, Y., Meng, L., Wu, F., et al. (2019). FLO8 deletion leads to azole resistance by upregulating CDR1 and CDR2 in Candida albicans. Res. Microbiol. 170, 272–279. doi: 10.1016/j.resmic.2019.08.005
Li, F., Palecek, S. P. (2003). EAP1, a Candida albicans gene involved in binding human epithelial cells. Eukaryot Cell 2, 1266–1273. doi: 10.1128/EC.2.6.1266-1273.2003
Linden, J. R., De Paepe, M. E., Laforce-Nesbitt, S. S., Bliss, J. M. (2013). Galectin-3 plays an important role in protection against disseminated candidiasis. Med. Mycol 51, 641–651. doi: 10.3109/13693786.2013.770607
Lírio, J., Giraldo, P. C., Amaral, R. L., Sarmento, A. C. A., Costa, A. P. F., Gonçalves, A. K. (2019). Antifungal (oral and vaginal) therapy for recurrent vulvovaginal candidiasis: a systematic review protocol. BMJ Open 9, e027489. doi: 10.1136/bmjopen-2018-027489
Liu, J., Willems, H. M. E., Sansevere, E. A., Allert, S., Barker, K. S., Lowes, D. J., et al. (2021). A variant ECE1 allele contributes to reduced pathogenicity of Candida albicans during vulvovaginal candidiasis. PloS Pathog. 17, e1009884. doi: 10.1371/journal.ppat.1009884
Marc, G., Araniciu, C., Oniga, S., Vlase, L., Pîrnău, A., Duma, M., et al. (2018). New N-(oxazolylmethyl)-thiazolidinedione active against Candida albicans Biofilm: potential Als proteins inhibitors. Molecules 23, 2522. doi: 10.3390/molecules23102522
McLellan, C. A., Whitesell, L., King, O. D., Lancaster, A. K., Mazitschek, R., Lindquist, S. (2012). Inhibiting GPI anchor biosynthesis in fungi stresses the endoplasmic reticulum and enhances immunogenicity. ACS Chem. Biol. 7, 1520–1528. doi: 10.1021/cb300235m
Maras, B., Maggiore, A., Mignogna, G., D’Erme, M., Angiolella, L. (2021). Hyperexpression of CDRs and HWP1 genes negatively impacts on Candida albicans virulence. PloS One 16, e0252555. doi: 10.1371/journal.pone.0252555
Marrazzo, J. M., Dombrowski, J. C., Wierzbicki, M. R., Perlowski, C., Pontius, A., Dithmer, D., et al. (2019). Safety and efficacy of a novel vaginal anti-infective, TOL-463, in the treatment of bacterial vaginosis and vulvovaginal candidiasis: A randomized, single-blind, phase 2, controlled trial. Clin. Infect. Dis. 68, 803–809. doi: 10.1093/cid/ciy554
Martinez, A. I. (2004). Role of Pir1 in the construction of the Candida albicans cell wall. Microbiol. (N Y) 150, 3151–3161. doi: 10.1099/mic.0.27220-0
Mavrianos, J., Berkow, E. L., Desai, C., Pandey, A., Batish, M., Rabadi, M. J., et al. (2013). Mitochondrial two-component signaling systems in Candida albicans. Eukaryot Cell 12, 913–922. doi: 10.1128/EC.00048-13
Mayer, F. L., Wilson, D., Hube, B. (2013). Candida albicans pathogenicity mechanisms. Virulence 4, 119–128. doi: 10.4161/viru.22913
Mazu, K., Bricker, B., Flores-Rozas, H., Ablordeppey, S. (2016). The mechanistic targets of antifungal agents: An overview. Mini-Reviews Medicinal Chem. 16, 555–578. doi: 10.2174/1389557516666160118112103
McCall, A. D., Kumar, R., Edgerton, M. (2018). Candida albicans Sfl1/Sfl2 regulatory network drives the formation of pathogenic microcolonies. PloS Pathog. 14, e1007316. doi: 10.1371/journal.ppat.1007316
Mishra, S., Singh, S., Misra, K. (2017). Restraining pathogenicity in Candida albicans by taxifolin as an inhibitor of Ras1-pka pathway. Mycopathologia 182, 953–965. doi: 10.1007/s11046-017-0170-4
Moalli, P. A., Howden, N. S., Lowder, J. L., Navarro, J., Debes, K. M., Abramowitch, S. D., et al. (2005). A rat model to study the structural properties of the vagina and its supportive tissues. Am. J. Obstet Gynecol 192, 80–88. doi: 10.1016/j.ajog.2004.07.008
Mohammed, L., Jha, G., Malasevskaia, I., Goud, H. K., Hassan, A. (2021). The interplay between sugar and yeast infections: Do diabetics have a greater predisposition to develop oral and vulvovaginal candidiasis? Cureus. doi: 10.7759/cureus.13407
Moriyama, B., Gordon, L. A., McCarthy, M., Henning, S. A., Walsh, T. J., Penzak, S. R. (2014). Emerging drugs and vaccines for Candidemia. Mycoses 57, 718–733. doi: 10.1111/myc.12265
Mota Fernandes, C., Dasilva, D., Haranahalli, K., McCarthy, J. B., Mallamo, J., Ojima, I., et al. (2021). The future of antifungal drug therapy: Novel compounds and targets. Antimicrob. Agents Chemother. 65, 10–1128. doi: 10.1128/AAC.01719-20
Mottola, A., Ramírez-Zavala, B., Hünniger, K., Kurzai, O., Morschhäuser, J. (2021). The zinc cluster transcription factor Czf1 regulates cell wall architecture and integrity in Candida albicans. Mol. Microbiol. 116, 483–497. doi: 10.1111/mmi.14727
Moyes, D. L., Richardson, J. P., Naglik, J. R. (2015). Candida albicans- epithelial interactions and pathogenicity mechanisms: scratching the surface. Virulence 6, 338–346. doi: 10.1080/21505594.2015.1012981
Mukaremera, L., Lee, K. K., Mora-Montes, H. M., Gow, N. A. R. (2017). Candida albicans yeast, pseudohyphal, and hyphal morphogenesis differentially affects immune recognition. Front. Immunol. 8. doi: 10.3389/fimmu.2017.00629
Munro, C. A., Schofield, D. A., Gooday, G. W., Gow, N. A. R. (1998). Regulation of chitin synthesis during dimorphic growth of Candida albicans. Microbiol. (N Y) 144, 391–401. doi: 10.1099/00221287-144-2-391
Murphy, S. E., Bicanic, T. (2021). Drug resistance and novel therapeutic approaches in invasive candidiasis. Front. Cell Infect. Microbiol. 11. doi: 10.3389/fcimb.2021.759408
Naglik, J. R., Challacombe, S. J., Hube, B. (2003). Candida albicans secreted aspartyl proteinases in virulence and pathogenesis. Microbiol. Mol. Biol. Rev. 67, 400–428. doi: 10.1128/MMBR.67.3.400-428.2003
Naglik, J. R., Fidel, P. L., Odds, F. C. (2008). Animal models of mucosal Candida infection. FEMS Microbiol. Lett. 283, 129–139. doi: 10.1111/j.1574-6968.2008.01160.x
Nikoomanesh, F., Roudbarmohammadi, S., Khoobi, M., Haghighi, F., Roudbary, M. (2019). Design and synthesis of mucoadhesive nanogel containing farnesol: investigation of the effect on HWP1, SAP6 and Rim101 genes expression of Candida albicans in vitro. Artif. Cells Nanomed Biotechnol. 47, 64–72. doi: 10.1080/21691401.2018.1543193
Netea, M. G., Brown, G. D., Kullberg, B. J., Gow, N. A. R. (2008). An integrated model of the recognition of Candida albicans by the innate immune system. Nat. Rev. Microbiol. 6, 67–78. doi: 10.1038/nrmicro1815
Nie, X., Liu, X., Wang, H., Chen, J. (2010). Deletion of EFG1 promotes Candida albicans opaque formation responding to pH via Rim101. Acta Biochim. Biophys. Sin. (Shanghai) 42, 735–744. doi: 10.1093/abbs/gmq076
Nikoomanesh, F., Falahatinejad, M., Černáková, L., dos Santos, A. L. S., Mohammadi, S. R., Rafiee, M., et al. (2023). Combination of farnesol with common antifungal drugs: Inhibitory effect against Candida species isolated from women with RVVC. Medicina (B Aires) 59, 743. doi: 10.3390/medicina59040743
Nobile, C. J., Nett, J. E., Andes, D. R., Mitchell, A. P. (2006). Function of Candida albicans adhesin Hwp1 in biofilm formation. Eukaryot Cell 5, 1604–1610. doi: 10.1128/EC.00194-06
Obara, K., Yamamoto, H., Kihara, A. (2012). Membrane protein Rim21 plays a central role in sensing ambient pH in Saccharomyces cerevisiae. J. Biol. Chem. 287, 38473–38481. doi: 10.1074/jbc.M112.394205
Oberdorfer, G., Binter, A., Ginj, C., Macheroux, P., Gruber, K. (2012). Structural and Functional Characterization of NikO, an Enolpyruvyl Transferase Essential in Nikkomycin Biosynthesis. J. Biol. Chem. 287, 31427–31436. doi: 10.1074/jbc.M112.352096
O’Connor, L., Caplice, N., Coleman, D. C., Sullivan, D. J., Moran, G. P. (2010). Differential filamentation of Candida albicans and Candida dubliniensis is governed by nutrient regulation of UME6 expression. Eukaryot Cell 9, 1383–1397. doi: 10.1128/EC.00042-10
Oliver, J. D., Sibley, G. E. M., Beckmann, N., Dobb, K. S., Slater, M. J., McEntee, L., et al. (2016). F901318 represents a novel class of antifungal drug that inhibits dihydroorotate dehydrogenase. Proc. Natl. Acad. Sci. 113, 12809–12814. doi: 10.1073/pnas.1608304113
O’Meara, T. R., Robbins, N., Cowen, L. E. (2017). The Hsp90 chaperone network modulates Candida virulence traits. Trends Microbiol. 25, 809–819. doi: 10.1016/j.tim.2017.05.003
Orchard, M. G., Neuss, J. C., Galley, C. M. S., Carr, A., Porter, D. W., Smith, P., et al. (2004). Rhodanine-3-acetic acid derivatives as inhibitors of fungal protein mannosyl transferase 1 (PMT1). Bioorg Med. Chem. Lett. 14, 3975–3978. doi: 10.1016/j.bmcl.2004.05.050
Osmałek, T., Froelich, A., Jadach, B., Tatarek, A., Gadziński, P., Falana, A., et al. (2021). Recent advances in polymer-based vaginal drug delivery systems. Pharmaceutics 13, 884. doi: 10.3390/pharmaceutics13060884
Pandey, M., Choudhury, H., Abdul-Aziz, A., Bhattamisra, S. K., Gorain, B., Carine, T., et al. (2020). Promising drug delivery approaches to treat microbial infections in the vagina: A recent update. Polymers (Basel) 13, 26. doi: 10.3390/polym13010026
Pappas, P. G., Kauffman, C. A., Andes, D. R., Clancy, C. J., Marr, K. A., Ostrosky-Zeichner, L., et al. (2016). Executive summary: Clinical practice guideline for the management of candidiasis: 2016 update by the infectious diseases society of America. Clin. Infect. Dis. 62, 409–417. doi: 10.1093/cid/civ1194
Park, Y.-K., Shin, J., Lee, H.-Y., Kim, H.-D., Kim, J. (2021). Development of Carbazole Derivatives Compounds against Candida albicans: Candidates to Prevent Hyphal Formation via the Ras1-MAPK Pathway. J. Fungi 7, 688. doi: 10.3390/jof7090688
Parua, P. K., Ratnakumar, S., Braun, K. A., Dombek, K. M., Arms, E., Ryan, P. M., et al. (2010). 14-3-3 (Bmh) Proteins Inhibit Transcription Activation by Adr1 through Direct Binding to Its Regulatory Domain. Mol. Cell Biol. 30, 5273–5283. doi: 10.1128/MCB.00715-10
Patel, D. A., Gillespie, B., Sobel, J. D., Leaman, D., Nyirjesy, P., Weitz, M. V., et al. (2004). Risk factors for recurrent vulvovaginal candidiasis in women receiving maintenance antifungal therapy: Results of a prospective cohort study. Am. J. Obstet Gynecol 190, 644–653. doi: 10.1016/j.ajog.2003.11.027
Permana, A. D., Utomo, E., Pratama, M. R., Amir, M. N., Anjani, Q. K., Mardikasari, S. A., et al. (2021). Bioadhesive-thermosensitive in situ vaginal gel of the gel flake-solid dispersion of itraconazole for enhanced antifungal activity in the treatment of vaginal iscandidias. ACS Appl. Mater Interfaces 13, 18128–18141. doi: 10.1021/acsami.1c03422
Phillips, N. A., Rocktashel, M., Merjanian, L. (2023). Ibrexafungerp for the treatment of vulvovaginal candidiasis: Design, development and place in therapy. Drug Des. Devel Ther. Vol. 17, 363–367. doi: 10.2147/DDDT.S339349
Popolo, L., Degani, G., Camilloni, C., Fonzi, W. (2017). The PHR family: The role of extracellular transglycosylases in shaping Candida albicans cells. J. Fungi 3, 59. doi: 10.3390/jof3040059
Priya, A., Pandian, S. K. (2020). Piperine impedes biofilm formation and hyphal morphogenesis of Candida albicans. Front. Microbiol. 11. doi: 10.3389/fmicb.2020.00756
Rajasekar, V., Darne, P., Prabhune, A., Kao, R. Y. T., Solomon, A. P., Ramage, G., et al. (2021). A curcumin-sophorolipid nanocomplex inhibits Candida albicans filamentation and biofilm development. Colloids Surf B Biointerfaces 200, 111617. doi: 10.1016/j.colsurfb.2021.111617
Ray, D., Goswami, R., Banerjee, U., Dadhwal, V., Goswami, D., Mandal, P., et al. (2007). Prevalence of Candida glabrata and its response to boric acid vaginal suppositories in comparison with oral fluconazole in patients with diabetes and vulvovaginal candidiasis. Diabetes Care 30, 312–317. doi: 10.2337/dc06-1469
Rayner, C. R., Smith, P. F., Andes, D., Andrews, K., Derendorf, H., Friberg, L. E., et al. (2021). Model-informed drug development for anti-infectives: State of the art and future. Clin. Pharmacol. Ther. 109, 867–891. doi: 10.1002/cpt.2198
Reales-Calderón, J. A., Aguilera-Montilla, N., Corbí, Á.L., Molero, G., Gil, C. (2014). Proteomic characterization of human proinflammatory M1 and anti-inflammatory M2 macrophages and their response to Candida albicans. Proteomics 14, 1503–1518. doi: 10.1002/pmic.201300508
Revie, N. M., Iyer, K. R., Robbins, N., Cowen, L. E. (2018). Antifungal drug resistance: evolution, mechanisms and impact. Curr. Opin. Microbiol. 45, 70–76. doi: 10.1016/j.mib.2018.02.005
Richardson, J., Ho, J., Naglik, J. (2018). Candida–epithelial interactions. J. Fungi 4, 22. doi: 10.3390/jof4010022
Richardson, J. L., Illum, L. (1992). (D) Routes of delivery: Case studies. Adv. Drug Delivery Rev. 8, 341–366. doi: 10.1016/0169-409X(92)90008-E
Román, E., Prieto, D., Hidalgo-Vico, S., Alonso-Monge, R., Pla, J. (2023). The defective gut colonization of Candida albicans hog1 MAPK mutants is restored by overexpressing the transcriptional regulator of the white opaque transition WOR1. Virulence 14, 2174294. doi: 10.1080/21505594.2023.2174294
Roemer, T., Krysan, D. J. (2014). Antifungal Drug Development: Challenges, Unmet Clinical Needs, and New Approaches. Cold Spring Harb. Perspect. Med. 4, a019703–a019703. doi: 10.1101/cshperspect.a019703
Rutherford, J. C., Bahn, Y.-S., van den Berg, B., Heitman, J., Xue, C. (2019). Nutrient and stress sensing in pathogenic yeasts. Front. Microbiol. 10. doi: 10.3389/fmicb.2019.00442
Rybak, J. M., Doorley, L. A., Nishimoto, A. T., Barker, K. S., Palmer, G. E., Rogers, P. D. (2019). Abrogation of triazole resistance upon deletion of CDR1 in a clinical isolate of Candida auris. Antimicrob. Agents Chemother. 63, 10–1128. doi: 10.1128/AAC.00057-19
Sahni, N., Yi, S., Daniels, K. J., Huang, G., Srikantha, T., Soll, D. R. (2010). Tec1 mediates the pheromone response of the white phenotype of Candida albicans: Insights into the evolution of new signal transduction pathways. PloS Biol. 8, e1000363. doi: 10.1371/journal.pbio.1000363
Samot, J., Rouabhia, M. (2021). Effect of dermaseptin S4 on C. albicans growth and EAP1 and HWP1 gene expression. Probiotics Antimicrob. Proteins 13, 287–298. doi: 10.1007/s12602-020-09685-0
Schaller, M., Bein, M., Korting, H. C., Baur, S., Hamm, G., Monod, M., et al. (2003). The secreted aspartyl proteinases Sap1 and Sap2 cause tissue damage in an in vitro model of vaginal candidiasis based on reconstituted human vaginal epithelium. Infect. Immun. 71, 3227–3234. doi: 10.1128/IAI.71.6.3227-3234.2003
Seleem, D., Pardi, V., Murata, R. M. (2017). Review of flavonoids: A diverse group of natural compounds with anti-Candida albicans activity in vitro. Arch. Oral. Biol. 76, 76–83. doi: 10.1016/j.archoralbio.2016.08.030
Shao, T.-Y., Kakade, P., Witchley, J. N., Frazer, C., Murray, K. L., Ene, I. V., et al. (2022). Candida albicans oscillating UME6 expression during intestinal colonization primes systemic Th17 protective immunity. Cell Rep. 39, 110837. doi: 10.1016/j.celrep.2022.110837
Shareck, J., Nantel, A., Belhumeur, P. (2011). Conjugated linoleic acid inhibits hyphal growth in Candida albicans by modulating Ras1p cellular levels and downregulating TEC1 expression. Eukaryot Cell 10, 565–577. doi: 10.1128/EC.00305-10
She, X., Zhang, L., Peng, J., Zhang, J., Li, H., Zhang, P., et al. (2020). Mitochondrial complex I core protein regulates cAMP signaling via phosphodiesterase Pde2 and NAD homeostasis in Candida albicans. Front. Microbiol. 11. doi: 10.3389/fmicb.2020.559975
Sheppard, C. (2020). Treatment of vulvovaginitis. Aust. Prescr 43, 195–197. doi: 10.18773/austprescr.2020.055
Shi, Y., Tang, L., Bai, X., Du, K., Wang, H., Jia, X., et al. (2022). Heat stress altered the vaginal microbiome and metabolome in rabbits. Front. Microbiol. 13. doi: 10.3389/fmicb.2022.813622
Shibata, N., Suzuki, A., Kobayashi, H., Okawa, Y. (2007). Chemical structure of the cell-wall mannan of Candida albicans serotype A and its difference in yeast and hyphal forms. Biochem. J. 404, 365–372. doi: 10.1042/BJ20070081
Slutsky, B., Buffo, J., Soll, D. R. (1985). High-frequency switching of colony morphology in Candida albicans. Sci. (1979) 230, 666–669. doi: 10.1126/science.3901258
Sobel, J. D., Donders, G., Degenhardt, T., Person, K., Curelop, S., Ghannoum, M., et al. (2022). Efficacy and safety of oteseconazole in recurrent vulvovaginal candidiasis. NEJM Evidence 1. doi: 10.1056/EVIDoa2100055
Sood, G., Nyirjesy, P., Weitz, M. V., Chatwani, A. (2000). Terconazole cream for non-Candida albicans fungal vaginitis: Results of a retrospective analysis. Infect. Dis. Obstet Gynecol 8, 240–243. doi: 10.1002/1098-0997(2000)8
Spampinato, C., Leonardi, D. (2013). Candida infections, causes, targets, and resistance mechanisms: Traditional and alternative antifungal agents. BioMed. Res. Int. 2013, 1–13. doi: 10.1155/2013/204237
Staib, P., Kretschmar, M., Nichterlein, T., Kohler, G., Michel, S., Hof, H., et al. (1999). Host-induced, stage-specific virulence gene activation in Candida albicans during infection. Mol. Microbiol. 32, 533–546. doi: 10.1046/j.1365-2958.1999.01367.x
Steele, C., Ratterree, M., Fidel, J. P.L. (1999). Differential susceptibility of two species of macaques to experimental vaginal candidiasis. J. Infect. Dis. 180, 802–810. doi: 10.1086/314964
Sudbery, P., Gow, N., Berman, J. (2004). The distinct morphogenic states of Candida albicans. Trends Microbiol. 12, 317–324. doi: 10.1016/j.tim.2004.05.008
Sustr, V., Foessleitner, P., Kiss, H., Farr, A. (2020). Vulvovaginal candidiasis: Current concepts, challenges and perspectives. J. Fungi 6, 267. doi: 10.3390/jof6040267
Tan, J., Zhang, J., Chen, W., Sun, Y., Wan, Z., Li, R., et al. (2015). The A395T mutation in ERG11 gene confers fluconazole resistance in Candida tropicalis causing candidemia. Mycopathologia 179, 213–218. doi: 10.1007/s11046-014-9831-8
Thomas, D. A., Lee, J. V. K., Choo, S., Rao, H., Chong, P. P. (2022). Effects of fresh garlic extract on Candida albicans sessile cells, biofilms and biofilm associated genes, Flo8 and Ndt80. Life Sci. Med. Biomed. 6. doi: 10.28916/lsmb.6.1.2022.89
Tortorano, A. M., Prigitano, A., Morroni, G., Brescini, L., Barchiesi, F. (2021). Candidemia: evolution of drug resistance and novel therapeutic approaches. Infect. Drug Resist. Vol. 14, 5543–5553. doi: 10.2147/IDR.S274872
Umemura, M., Okamoto, M., Nakayama, K., Sagane, K., Tsukahara, K., Hata, K., et al. (2003). GWT1 gene is required for inositol acylation of glycosylphosphatidylinositol anchors in yeast. J. Biol. Chem. 278, 23639–23647. doi: 10.1074/jbc.M301044200
van Bruggen, R., Drewniak, A., Jansen, M., van Houdt, M., Roos, D., Chapel, H., et al. (2009). Complement receptor 3, not Dectin-1, is the major receptor on human neutrophils for β-glucan-bearing particles. Mol. Immunol. 47, 575–581. doi: 10.1016/j.molimm.2009.09.018
van der Graaf, C. A. A., Netea, M. G., Verschueren, I., van der Meer, J. W. M., Kullberg (2005). Differential cytokine production and Toll-Like Receptor signaling pathways by Candida albicans blastoconidia and hyphae. Infect. Immun. 73, 7458–7464. doi: 10.1128/IAI.73.11.7458-7464.2005
Vrbanac, A., Riestra, A. M., Coady, A., Knight, R., Nizet, V., Patras, K. A. (2018). The murine vaginal microbiota and its perturbation by the human pathogen group B Streptococcus. BMC Microbiol. 18, 197. doi: 10.1186/s12866-018-1341-2
Vylkova, S., Carman, A. J., Danhof, H. A., Collette, J. R., Zhou, H., Lorenz, M. C. (2011). The fungal pathogen Candida albicans autoinduces hyphal morphogenesis by raising extracellular pH. mBio 2, 10–1128. doi: 10.1128/mBio.00055-11
Wakade, R. S., Ristow, L. C., Wellington, M., Krysan, D. J. (2023). Intravital imaging-based genetic screen reveals the transcriptional network governing Candida albicans filamentation during mammalian infection. Elife 12. doi: 10.7554/eLife.85114
Walker, L. A., Gow, N. A. R., Munro, C. A. (2013). Elevated chitin content reduces the susceptibility of Candida species to caspofungin. Antimicrob. Agents Chemother. 57, 146–154. doi: 10.1128/AAC.01486-12
Wang, Y., Long, W., Zhang, F., Zhang, M., Zeng, K., Zhu, X. (2022). Hexyl-aminolevulinate ethosomes: a novel antibiofilm agent targeting zinc homeostasis in Candida albicans. Microbiol. Spectr. 10, 181–187. doi: 10.1128/spectrum.02438-22
Wang, L., Tang, X. (2008). A novel ketoconazole bioadhesive effervescent tablet for vaginal delivery: Design, in vitro and in vivo evaluation. Int. J. Pharm. 350, 181–187. doi: 10.1016/j.ijpharm.2007.08.042
Watanabe, N., Miyazaki, M., Horii, T., Sagane, K., Tsukahara, K., Hata, K. (2012). E1210, a new broad-spectrum antifungal, suppresses candida albicans hyphal growth through inhibition of glycosylphosphatidylinositol biosynthesis. Antimicrob. Agents Chemother. 56, 960–971. doi: 10.1128/AAC.00731-11
Whibley, N., Gaffen, S. L. (2014). Brothers in arms: Th17 and Treg responses in Candida albicans immunity. PloS Pathog. 10, e1004456. doi: 10.1371/journal.ppat.1004456
Whitney, P. G., Bär, E., Osorio, F., Rogers, N. C., Schraml, B. U., Deddouche, S., et al. (2014). Syk signaling in dendritic cells orchestrates innate resistance to systemic fungal infection. PloS Pathog. 10, e1004276. doi: 10.1371/journal.ppat.1004276
Willems, H. M. E., Ahmed, S. S., Liu, J., Xu, Z., Peters, B. M. (2020). Vulvovaginal candidiasis: A current understanding and burning questions. J. Fungi 6, 27. doi: 10.3390/jof6010027.s
Wu, Y., Hu, S., Wu, C., Gu, F., Yang, Y. (2022). Probiotics: potential novel therapeutics against fungal infections. Front. Cell Infect. Microbiol. 11. doi: 10.3389/fcimb.2021.793419
Wu, J., Wu, D., Zhao, Y., Si, Y., Mei, L., Shao, J., et al. (2020). Sodium new houttuyfonate inhibits Candida albicans biofilm formation by inhibiting the Ras1-cAMP-Efg1 pathway revealed by RNA-seq. Front. Microbiol. 11. doi: 10.3389/fmicb.2020.02075
Yan, H., Fang, T., Xu, H., Jiang, L. (2020). The pH-sensing Rim101 pathway positively regulates the transcriptional expression of the calcium pump gene PMR1 to affect calcium sensitivity in budding yeast. Biochem. Biophys. Res. Commun. 532, 453–458. doi: 10.1016/j.bbrc.2020.08.083
Yang, D., Hu, Y., Yin, Z., Gao, Q., Zhang, Y., Chan, F. Y., et al. (2020). Candida albicans ubiquitin and heat shock factor-type transcriptional factors are involved in 2-dodecenoic acid-mediated inhibition of hyphal growth. Microorganisms 8, 75. doi: 10.3390/microorganisms8010075
Yang, M., Cao, Y., Zhang, Z., Guo, J., Hu, C., Wang, Z., et al. (2023). Low intensity ultrasound-mediated drug-loaded nanoparticles intravaginal drug delivery: an effective synergistic therapy scheme for treatment of vulvovaginal candidiasis. J. Nanobiotechnol. 21, 53. doi: 10.1186/s12951-023-01800-x
Yong, J., Zu, R., Huang, X., Ge, Y., Li, Y. (2020). Synergistic effect of berberine hydrochloride and fluconazole against Candida albicans resistant isolates. Front. Microbiol. 11. doi: 10.3389/fmicb.2020.01498
Zameitat, E., Gojkovic, Z., Knecht, W., Piskur, J., Loffler, M. (2006). Biochemical characterization of recombinant dihydroorotate dehydrogenase from the opportunistic pathogenic yeast Candida albicans. FEBS J. 273, 3183–3191. doi: 10.1111/j.1742-4658.2006.05327.x
Zare-Bidaki, M., Maleki, A., Ghanbarzadeh, N., Nikoomanesh, F. (2022). Expression pattern of drug-resistance genes ERG11 and TAC1 in Candida albicans Clinical isolates. Mol. Biol. Rep. 49, 11625–11633. doi: 10.1007/s11033-022-07878-0
Zeise, K. D., Woods, R. J., Huffnagle, G. B. (2021). Interplay between Candida albicans and lactic acid bacteria in the gastrointestinal tract: Impact on colonization resistance, microbial carriage, opportunistic infection, and host immunity. Clin. Microbiol. Rev. 34. doi: 10.1128/CMR.00323-20
Zeng, Y., Rustchenko, E., Huang, X., Wu, T. T., Falsetta, M. L., Xiao, J. (2023). Dual transcriptome of Streptococcus mutans and Candida albicans interplay in biofilms. J. Oral. Microbiol. 15, 2144047. doi: 10.1080/20002297.2022.2144047
Zhu, L.-L., Zhao, X.-Q., Jiang, C., You, Y., Chen, X.-P., Jiang, Y.-Y., et al. (2013). C-type lectin receptors Dectin-3 and Dectin-2 form a heterodimeric pattern-recognition receptor for host defense against fungal infection. Immunity 39, 324–334. doi: 10.1016/j.immuni.2013.05.017
Zou, H., Fang, H.-M., Zhu, Y., Wang, Y. (2010). Candida albicans Cyr1, Cap1 and G-actin form a sensor/effector apparatus for activating cAMP synthesis in hyphal growth. Mol. Microbiol. 75, 579–591. doi: 10.1111/j.1365-2958.2009.06980.x
Keywords: C. albicans, vaginal candidiasis, predisposition factors, morphogenesis, multidrug resistance, possible drug targets
Citation: David H and Solomon AP (2023) Molecular association of Candida albicans and vulvovaginal candidiasis: focusing on a solution. Front. Cell. Infect. Microbiol. 13:1245808. doi: 10.3389/fcimb.2023.1245808
Received: 23 June 2023; Accepted: 21 September 2023;
Published: 13 October 2023.
Edited by:
Cheshta Sharma, The University of Texas Health Science Center at San Antonio, United StatesReviewed by:
Maryam Roudbary, Iran University of Medical Sciences, IranGulnaz Bashir, Sher-I-Kashmir Institute of Medical Sciences, India
Copyright © 2023 David and Solomon. This is an open-access article distributed under the terms of the Creative Commons Attribution License (CC BY). The use, distribution or reproduction in other forums is permitted, provided the original author(s) and the copyright owner(s) are credited and that the original publication in this journal is cited, in accordance with accepted academic practice. No use, distribution or reproduction is permitted which does not comply with these terms.
*Correspondence: Adline Princy Solomon, YWRsaW5lcHJpbnp5QHNhc3RyYS5hYy5pbg==