- Sino-German Biomedical Center, National “111” Center for Cellular Regulation and Molecular Pharmaceutics, Cooperative Innovation Center of Industrial Fermentation (Ministry of Education & Hubei Province), Key Laboratory of Fermentation Engineering (Ministry of Education), Hubei University of Technology, Wuhan, China
Corona Virus Disease 2019 (COVID-19) continues to be a burden for human health since its outbreak in Wuhan, China in December 2019. Recently, the emergence of new variants of concerns (VOCs) is challenging for vaccines and drugs efficiency. In severe cases, SARS-CoV-2 provokes inappropriate hyperinflammatory immune responses leading to acute respiratory distress syndrome (ARDS) and even death. This process is regulated by inflammasomes which are activated after binding of the viral spike (S) protein to cellular angiotensin-converting enzyme 2 (ACE2) receptor and triggers innate immune responses. Therefore, the formation of “cytokines storm” leads to tissue damage and organ failure. NOD-like receptor family pyrin domain containing 3 (NLRP3) is the best studied inflammasome known to be activated during SARS-CoV-2 infection. However, some studies suggest that SARS-CoV-2 infection is associated with other inflammasomes as well; such as NLRP1, absent in melanoma-2 (AIM-2), caspase-4 and -8 which were mostly found during dsRNA virus or bacteria infection. Multiple inflammasome inhibitors that exist for other non-infectious diseases have the potential to be used to treat severe SARS-CoV-2 complications. Some of them have showed quite encouraging results during pre- and clinical trials. Nevertheless, further studies are in need for the understanding and targeting of SARS-Cov-2-induced inflammasomes; mostly an update of its role during the new VOCs infection is necessary. Hence, this review highlights all reported inflammasomes involved in SARS-CoV-2 infection and their potential inhibitors including NLRP3- and Gasdermin D (GSDMD)-inhibitors. Further strategies such as immunomodulators and siRNA are also discussed. As highly related to COVID-19 severe cases, developing inflammasome inhibitors holds a promise to treat severe COVID-19 syndrome effectively and reduce mortality.
Introduction
SARS-CoV-2 is a positive single-stranded RNA (ssRNA) virus belonging to Betacoronavirus genus, coronaviridae family. It has a large genome of 29.9kb within 13 open reading frames (ORFs). ORF1a and ORF1b permit the primary translation of a polyprotein to initiate infection (Kim et al., 2020). Subsequently, after some replications, the other ORFs assure the expression of the subgenomic mRNA encoding for all structural proteins (Sola et al., 2015). Until now, SARS-CoV-2 is provoking infection resulting in human’s hospitalization and death worldwide. The most people at risk are the ones with advanced age, hypertension, cardiovascular disease and diabetes (Wang et al., 2020). The main targets for antiviral development are RNA-dependent RNA-polymerase (RdRp), viral proteases or ACE-2 cell receptors (Kruse, 2020; Majumder and Minko, 2021). However, their progress in FDA approval is challenging due to their moderate effect and the rapid emergence of new virus variants (Robinson et al., 2022). Similarly, vaccines are being put into use by some commercial companies such as Jonhson & Johnson or Sinovac Biotech but their immune protection efficacy is far from satisfactory due to the fast evolution of SARS-CoV-2 (Hadj Hassine, 2022). COVID-19 symptoms are mild and self-limiting or in many cases asymptomatic. However, some of the infected people may develop severe symptoms which can lead to death (Huang et al., 2020). Indeed, it has infected 700 million people so far with 6.8 million deaths (COVID-19 Map - Johns Hopkins Coronavirus Resource Center (jhu.edu), access on 09.03.2023). Studies have proven that many severe cases are due to inappropriate hyperinflammatory responses of the immune system to the viral infection (Kaivola et al., 2021; Vora et al., 2021). In fact, immune system responses are the first line of defense against viral infection. Once the spike (S) protein of SARS-CoV-2 binds to the cell receptors, pattern-recognition receptors such as Nod-like receptor (NLR) or absent in melanoma-2 (AIM2) will assemble inflammasomes through inducing membrane pore formation and activation of proinflammatory cytokines in order to activate cell death and eliminate the viral infection (Figure 1). Indeed, during SARS-CoV-2 infection, those proteins are highly promoted. Therefore, the over-responses induce lung injuries leading to severe symptoms including acute respiratory distress syndrome (ARDS) and subsequent death (Lee et al., 2020). Thus, controlling this response of the immune system is essential and represents a valuable key for the fight against COVID-19 leading to severe symptoms (Yap et al., 2020; Rodrigues et al., 2021). This review highlights the involved inflammasomes during SARS-CoV-2 infection and summarizes their corresponding inhibitors. Consequently, the information presented would be helpful in the understanding of viral pathogenesis mechanism, the understanding of inflammasome roles during SARS-Cov-2 infection and development of novel therapeutic molecules against COVID-19. Moreover, it provides insights on future study direction of the virus as it is quite new and a lot remain unknown.
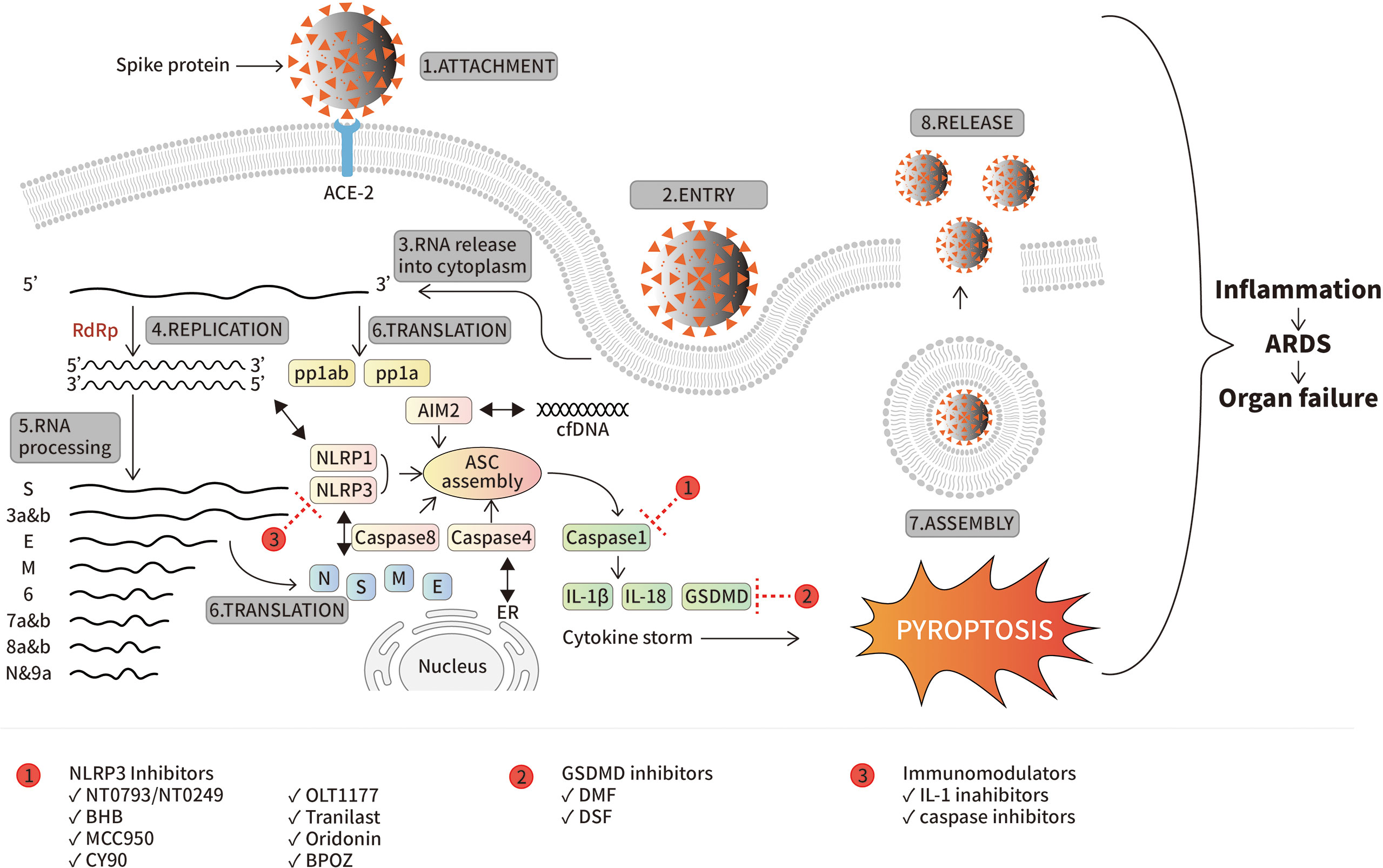
Figure 1 SARS-CoV-2 life cycle and inflammasomes activation. The attachment of the virus by its spike protein to ACE-2 is the first step inducing not only viral entry but also host immune responses. SARS-CoV-2 encodes for multiple ORFs (from S to 9a) by RNA-dependent RNA-polymerase (RdRp). While all structural and non-structural proteins are expressed, viral assembly occurs ending into release of new virions. Meanwhile, inflammasomes are highly activated by innate immune system in order to eliminate viral infection. Mechanistically, NLRP3 is activated by viral N and S protein, NLRP1 by the dsRNA formed during viral life cycle, AIM-2 by cfDNA and caspase-4 and -8. Activation of all those inflammasomes promotes ASC assembly and activation of caspase-1 following by expression of IL-1β, IL-18 and GSDMD (“cytokines storm”) resulting in pyroptosis which is mainly responsible of tissue damage leading to ARDS and organ failure during virus infection. The red circles containing the numbers 1, 2, and 3 represent three types of inflammasome inhibitors, and the positions of their intervening targets are indicated with a red dash line T, respectively.
Possible inflammasome targets
Excessive inflammatory responses induced upon SARS-CoV-2 infection are associated with severe symptoms of COVID-19. NLRP3 is the most studied inflammasome and the first known to be activated during SARS-CoV-2 infection (Freeman and Swartz, 2020). However, other inflammasomes such as NLRP1, AIM2, caspase-4 and -8 have a quite high possibility of involvement in activating cytokines that induce the over-responses of the immunity and the severity of the disease.
NOD-, LRR- and pyrin domain-containing protein 3 inflammasome
It is an essential component of the immune system and consists of a sensor (NLRP3), an adaptor (apoptosis-associated speck-like protein containing a caspase recruitment domain, ASC) and an effector (caspase-1). During harmful stimuli such as viral invasion, NLRP-3 induces ASC assembly which recruits caspase-1, resulting to the cleavage of pro-IL-1β and pro-IL-18 into their active forms IL-1β and IL-18. These latter are important cytokines that assure the inflammatory response of the immune system during the viral infection (Kaivola et al., 2021). However, in some cases, NLRP3 response is associated with serious diseases like autoinflammatory disease. Recently, it was proven to provoke severe symptoms in COVID-19 patients (Zhao et al., 2021). Furthermore, study in macrophages and dentritic cells as well as mice model revealed the promotion of mature cytokines by viral N protein which led to lung injuries. Mechanistically, N protein interacts directly with NLRP3 complex, promotes the binding of NLRP3 with ASC, and facilitates NLRP3 inflammasome assembly (Pan et al., 2021). Indeed, autopsy of COVID-19 patients revealed the presence of active NLRP3 in their peripheral blood mononuclear cells (PBMCs) and tissues (He et al., 2006). Moreover, in patients with severe symptoms, abundant of inflammasome-derived products, such as caspase-1, IL-6, IL-18 and LDH, were found in their sera (Rodrigues et al., 2021). The activation of NLRP3 during COVID-19 infection is via ACE-2 which is highly expressed at the monocyte of COVID-19 patients only (Junqueira et al., 2021). The ssRNA sequences of SARS-CoV-2 trigger activation of important immune molecules, such as TLRs or RIG-1, NF-KB promoting production of IL-1β, IL-18, LDH release, and mostly caspase-1 activation as the hallmark of inflammasome assembly which results in GSDMD activation followed by pyroptosis leading to tissue damage and ARDS (Campbell et al., 2021) (Figure 1). Furthermore, NLRP3 activation requires efflux of potassium and ASC (Xu et al., 2022). Thus, NLRP3 inflammasome represents an attractive target in the development of drugs for COVID-19 patients with severe manifestations.
NOD-, LRR- and pyrin domain-containing protein 1 inflammasome
Instead of NLRP3, this inflammasome pathway is initiated by NLRP1 as sensor. The mechanism involved seems to be the same as that for NLRP3 in which the cleavage of caspase-1 leads to IL-1β and IL-18 activation and pyroptosis (Bauernfried et al., 2021). Screening of different types of viruses for inflammasome responses in keratinocytes revealed that NLRP1 acts as a biosensor for double-stranded RNA (dsRNA) virus (Bauernfried et al., 2021). Indeed, a negative-strand RNA is synthesized by SARS-CoV-2 to serve as template for progeny viral genome production, this process is similar to other coronaviruses life cycle (Sola et al., 2015). Therefore, it is highly possible that viral dsRNA activates NLRP1 by binding to it through a leucine-rich repeat domain of NLRP1 and participates together with NLRP3 to the over-responses of the immune system leading to COVID-19 severe symptoms (Bauernfried et al., 2021). Moreover, viral proteases like EV-A71 3Cpro are able to activate NLRP1 in some cases through direct cleavage (Robinson et al., 2020). Therefore, SARS-CoV-2 proteases (PL2 and 3CLpro) are likely to be involved in the activation of NLRP1 inflammasome (Planès et al., 2022).
Absent in melanoma 2 inflammasome
AIM2 is the third sensor that is related to inflammasome activation during infection (Kaivola et al., 2021). It is associated predominantly to dsRNA virus or bacteria (Lugrin and Martinon, 2018). The mechanism is through ASC assembly and activation of caspase-1 resulting in cytokines expression and pyroptosis. Despite being a ssRNA virus, SARS-CoV-2 was associated with AIM-2 during its study in monocytes. Indeed, considerable levels of AIM-2 were detected in monocytes infected by SARS-CoV-2 (Junqueira et al., 2021). Furthermore, AIM-2 receptor activation was observed in peripheral blood mononuclear cells (PBMCs) from post-Covid-19 patients that presented lung injuries (Colarusso et al., 2022). It was speculated that the ability of SARS-CoV-2 to induce AIM2 inflammasome response is due to the presence of cell free DNA (cfDNA) in several tissues of COVID-19 patient including lungs. In fact, high level of cfDNA was found in COVID-19 patients that required intensive treatment or died during hospitalization (Andargie et al., 2021). Therefore, AIM2 represents a valuable marker and/or target for COVID-19 disease severity and corresponding treatment.
Caspase-4
Caspase-4 is a protein that participates to the inflammatory response during pathogen invasion (Viganò et al., 2015). Kaivola et al. speculated the involvement of the non-canonical inflammasome caspase-4 in COVID-19 inflammatory response due to both caspase-4 and SARS-CoV-2 localization on the endoplasmic reticulum (ER) membrane (Kaivola et al., 2021). Interestingly, recent studies confirmed that the caspase-4 and its homolog in mice, caspase 11, were associated with COVID-19 disease severity (Eltobgy et al., 2022). Nevertheless, the exact role of caspase-4 in inducing inflammation during viral infection remains unclear and needs further investigation.
Caspase-8
It is another caspase that induce inflammation during SARS-CoV-2 infection. What is known so far about its involvement in SARS-CoV-2 infection is its activation. In fact, SARS-CoV-2 triggered caspase-8 activation, expression of IL-1β and IL-18 resulting in apoptosis in lung epithelial cells (Li et al., 2020).
SARS-Cov-2 variants and inflammasomes
Multiple variants of SARS-Cov-2 have been emerging since its first apparition in Wuhan at the end of 2019. The virus has adapted quickly to humans and became highly mutated mostly at its spike protein sequence which is responsible of the attachment of the virus to ACE-2 cell surface receptor (Awadasseid et al., 2021; Harvey et al., 2021). To date, 5 of the existing variants are considered VOCs or variant of concerns by World Health Organization (WHO). VOCs have a high transmission rate compared to others: rapid spread and quick circulating dominance. In fact, most times, when a new variant emerges, the old one is no longer circulating. They are Alpha, Beta, Gamma and O’micron variants (Scovino et al., 2022). Their direct relation on how they handle immune responses including inflammasome is not yet elucidated. Through early studies on this new virus, inflammasome was positively correlated to lead the viral infection into severe disease by over-secreting cytokines and thus provoke tissue damages and reduce clinical outcomes (Figure 1) (Li et al., 2020; Junqueira et al., 2021; Kaivola et al., 2021; Vora et al., 2021). In fact, each variant has different transmission rate and pathogenicity (Kumar et al., 2022). It may be due to cross-protections acquired during past infection or for people that were vaccinated. Interestingly, studied showed that vaccinated people with the first strain Wuhan-hu have protection against Alpha, Beta and Gamma variant but not for O’micron which has more than 15 mutations at the spike receptor binding domain (RBD) plus multiple deletions and substitutions in the N-terminal (Cameroni et al., 2022; Zeng et al., 2022). Furthermore, a study in UK showed that there was a T cell strong memory in patients infected with SARS-Cov-2. Concerning the inflammasome response to SARS-Cov-2 new variants, deeper studies are in need to further confirm the statement of previous studies that obviously showed correlation of inflammasomes and severe COVID-19 (Peng et al., 2020). Hence, investigating the relationship between inflammasomes and the different VOCs will ultimately give more understanding on how the organism reacts towards the infection and how to limit occurrence of severe cases. Furthermore, NLRP3 inflammasome which is the most related to SARS-Cov-2 has different variants as well. Study proved that NLRP3 variants are also responsible of different responses and then clinical symptoms: severe or mild. Indeed, NLRP3 rs10157379 and rs10754558 are the ones positively related to severe COVID-19 (Maes et al., 2022).
Drugs developments and strategies targeting a certain type of inflammasomes
As a major class of anti-inflammatory molecules, series of compounds targeting a certain type of inflammasome are currently in the preclinical and clinical stages, and their indications include some non-infectious diseases such as atherosclerosis, Alzheimer, Parkinson, gout, rheumatoid arthritis and non-alcoholic steatohepatitis (Awadasseid et al., 2021; Harvey et al., 2021). For infectious diseases like SARS-CoV-2 infection, people may be worried whether the blockage of IL-1 increases the risk of infection. After all, cytokines are natural protein that the organism needs to protect against pathogens. However, the risk shall be reduced as there are multiple ways that innate immune response use to produce IL-1 during infection. Therefore, it makes NLRP3 the most attractive inflammasome to target (Awadasseid et al., 2021; Harvey et al., 2021). To date, there are no commercialized inflammasome inhibitors specifically applied for the treatment of SARS-CoV-2 infection (Declercq et al., 2022). Nevertheless, several existing ones for other diseases are being tested for COVID-19. The results seem encouraging, which brings a glimmer of hope for conquering COVID-19 induced ARDS. We summarize them herein after (Table 1).
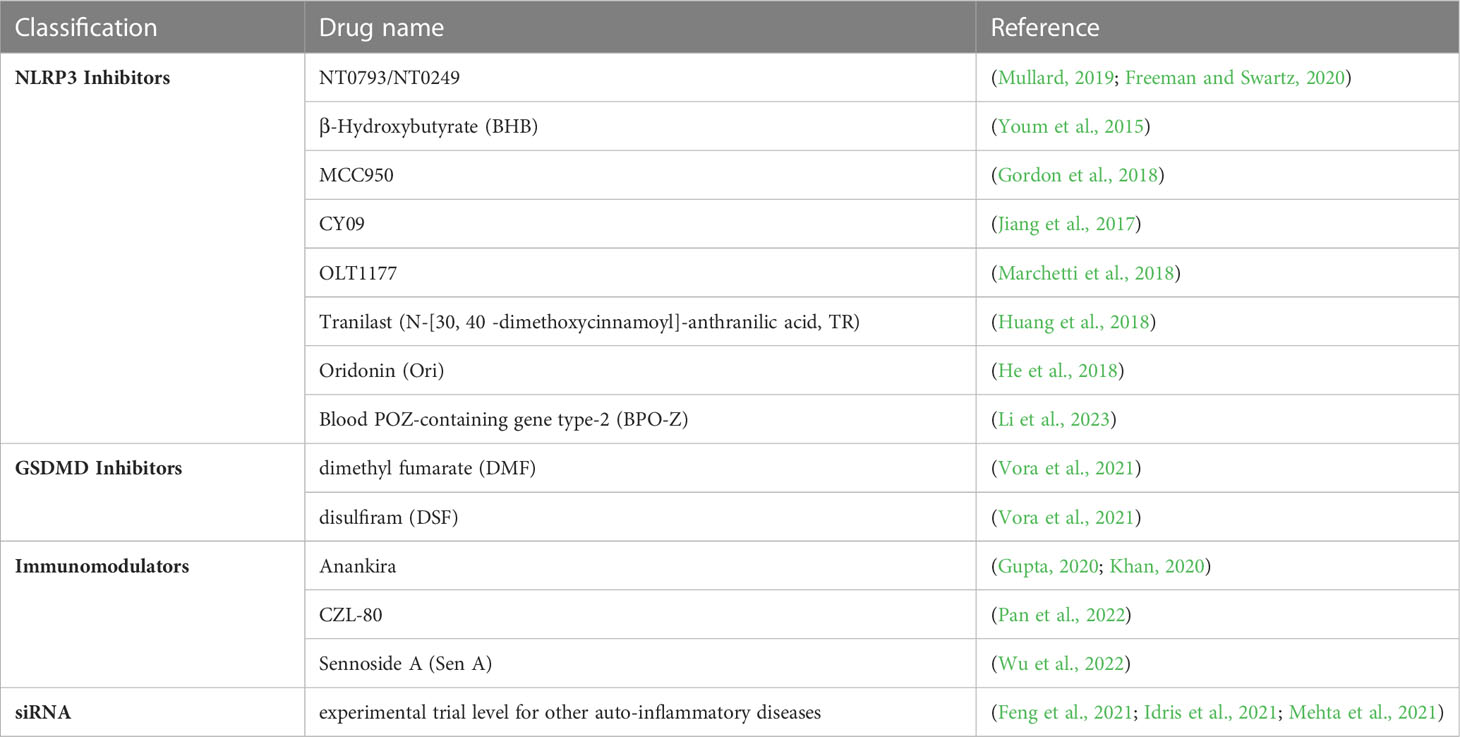
Table 1 A list of current developed anti-inflammasome inhibitors potentially applied for COVID-19 treatment.
NLRP3 inhibitors
NT0793/NT0249
Nodthera is a company specialized in development of molecule targeting NLRP3 inflammasome for treatment of multiple diseases such as Parkinson disease, Alzheimer disease (Pipeline - Nodthera, accessed on 17.04.2023). Currently, these molecules have passed preclinical trial and now in phase I (Mullard, 2019; Freeman and Swartz, 2020). Interestingly, it is likely to be used for COVID-19 patient with severe disease as well.
β-Hydroxybutyrate
β-Hydroxybutyrate is an endogenous ketone that is able to inhibit NLRP3 through reduction of potassium efflux ASC, and consequently reduce inflammation and IL-1β expression (Youm et al., 2015). It is therefore an optional treatment for COVID-19 leading to severe disease.
MCC950
MCC950 is known as NLRP3 inhibitor. It was able to reduce IL-1β production in vivo (C57BL/6 female mice) during study for Parkinson’s disease (Gordon et al., 2018).
CY09
CY09 a molecule, through binding to the NACHT domain of NLRP3 ATP-binding motif, is able to block inflammasome assembly and activation both in vitro and in vivo (Jiang et al., 2017).
OLT1177
OLT1177 is an active β-sulfonyl nitrile molecule that specifically targets NLRP3 inflammasome. In fact, LPS-stimulated human blood-derived macrophages and monocytes from patients with cryopyrin-associated periodic syndrome (CAPS) treated with OLT1177 presented a decreased level of IL-1β and IL-18. Its mechanism of action is known through inhibition of the binding between the sensor NLRP3 with its adaptor ASC and its interaction with caspase-1 effector (Marchetti et al., 2018).
Tranilast
Tranilast is a triptophan analog empirically used as anti-allergic. Later on, studies showed that it exhibits an inhibition activity against NLRP3 inflammasome. In fact, through direct binding to NLRP3 NACHT domain, TR prevents its assembly and oligomerization. The inhibition was efficient both in vivo (mouse models of NLRP3 inflammasome-related human diseases) and in vitro (mononuclear cells from patients with gout) (Huang et al., 2018).
Oridonin
Oridonin is an active compound of Rabdosia rubescens, a Chinese traditional medicinal herb. It has been used to treat inflammatory diseases. Currently, its mechanism of action was revealed as a NLRP3 inhibitor through binding to the NLRP3 NACHT domain at cysteine 279. Furthermore, its effects are both preventative and therapeutic during study in mouse models presenting peritonitis, gouty arthritis and type 2 diabetes disease (He et al., 2018).
Blood POZ-containing gene type-2
Blood POZ-containing gene type-2 is a cellular molecule involved in growth suppression. Recently it was identified as a negative regulator of NLRP3 inflammasome during SARS-CoV-2 infection. Consequently, it may represent a strategy to be used to inhibit NLRP3 activation which leads to severe COVID-19 syndrome (Li et al., 2023).
Impressively, the NLRP3 inhibitor MCC950 reduced caspase 1 activation and secretion of IL-1β in primary human monocytes infected with SARS-CoV-2 in vitro (Rodrigues et al., 2021). Furthermore, several phase II clinical trials are testing direct NLRP3 inhibition in patients with either mild or severe COVID-19, such as NCT04382053 by Novartis (https://clinicaltrials.gov/ct2/history/NCT04382053?V_34=View#StudyPageTop) and NCT04540120 by Olatec Therapeutics (https://www.sciencedirect.com/science/article/pii/B9780323918022000359). These data suggest that NLRP3 inhibition may be a superior strategy against COVID-19 severe symptom.
GSDMD inhibitors
Gasdermin D is a vital protein during the activation of cell death pathways such as pyroptosis (Burdette et al., 2021). It was able to be highly activated by certain inflammasome proteins (Liu et al., 2016). Therefore, it is an excellent target for the treatment of COVID-19 disease. Dimethyl fumarate (DMF) and disulfiram (DSF) are FDA approved drugs for the treatment of sclerosis and alcoholism, respectively. As they have been identified as GSDMD-inhibitors, their clinical trials are ongoing for the treatment of COVID-19 disease (Vora et al., 2021). In fact, COVID-19 patients under DMF treatment against sclerosis or under DSF against alcohol use disorder showed a self-limiting symptoms (Fillmore et al., 2021; Mantero et al., 2021). Therefore, it is being clinically tested against COVID-19 (trial number NCT04594343) with a treatment dose of 120 mg every 12 hours for 2 days or 240 mg every 12 hours for 8 days. Besides, DSF underwent two clinical trials. The first one was performed in 60 participants with mild disease with a dose of 1000 or 2000mg/day for 5 days (trial number NCT04485130). The second trial was with 200 participants hospitalized and treated with 500mg/day for 14 days (trial number NCT04594343) (Vora et al., 2021).
Immunomodulators
IL-1β is a part of the cytokine storm that is stimulated by inflammasomes (Martinon et al., 2002). In fact, IL-1 inhibitors have been considered as an excellent candidate for the treatment of SARS-CoV-2-related diseases (Mardi et al., 2021). Most importantly, several immunomodulators are actually commercially available. For instance, Anankira, an immunosuppressive drug used to treat rheumatoid arthritis, have shown considerable survival effects in COVID-19 patients. Its mechanism of action is to inhibit IL-1 by binding to it (Gupta, 2020; Khan, 2020).
Caspases are cysteine-dependent proteases involved in multiple cellular mechanisms including inflammasome activation during COVID-19 infection and thus represent a valuable target (Premeaux et al., 2022). In fact, caspase-1 as an effector of NLRP3 as well as caspase 4 and 8 could be targeted by caspases inhibitors which have been progressively in development (Lee et al., 2018). Indeed, CZL-80 is a caspase-1 inhibitor that had successfully treated mice developing progressive ischemic stroke (PIS) (Pan et al., 2022). Recently, Sennoside A (Sen A) was identified to inhibit caspase-1 and induced the suppression of both NLRP3 and AIM-2 inflammasomes (Wu et al., 2022).
siRNA
Small interference RNA has always been an attractive strategy to fight against multiple infections including viruses (Saw and Song, 2020). In development of anti-COVID-19 drugs, siRNA has been attempted to interfere with SARS-CoV-2 genomic ssRNA (Idris et al., 2021; Mehta et al., 2021). However, as we know now that the poor prognosis of COVID-19 is not due to the viral infection itself but the activation of inflammasomes leading to tissue damage and organ failure. Thus, targeting a specific type of inflammasome with siRNAs seems to represent a promising strategy. In fact, multiple inflammasomes have already been targeted by siRNA in several auto-inflammatory diseases (Feng et al., 2021).
Discussion and perspectives
Measures taken towards SARS-CoV-2 pandemic such as restrictions have been removed in almost all countries. However, until now, SARS-CoV-2 is continuing to provoke severe diseases and even death in the world (Chu et al., 2022; Fox et al., 2022). Indeed, finding a powerful and thorough treatment mostly for those leading to severe COVID-19 disease is still an ultimate goal that has to be reached as soon as possible. Moreover, the emergence of new variant of concerns are worrying as they become highly contagious and may lead to a new pandemic wave. Targeting inflammasome, an intracellular protein complex activated in innate immune response following antigen attacks, has been becoming more and more attractive with the increasing investment of many large pharmaceutical companies. Actually, series of inflammasome inhibitors are being tested in the pre- and clinical stages for the treatment of several non-infectious diseases such as CAPS, gouty arthritis, type 2 diabetes etc. For infectious disease like SARS-CoV-2 infection, the cause of cytokine storm is believed due to an excessive immune responses in the organism but not the virus itself (Kaivola et al., 2021). Multiple inflammasomes already exist within the organism and there are multiple alternative ways to produce IL-1 according to the pathogen (virus, bacteria…). Thus, blocking one inflammasome by its inhibitor does not increase the risk of infection and shall not affect the level of innate immune responses. Hence, it represents the best way to fight the infection. In fact, some NLRP3 inhibitors being evaluated under experimental studies have already showed quite satisfactory results (Freeman and Swartz, 2020; Declercq et al., 2022). Besides NLRP3, other types of inflammasomes that were initially known to interact with dsRNA or bacteria such as AIM-2, caspase-4 and -8 were found to play a role in the inflammation responses during COVID-19. Thus, this wide range of molecules represents a broad target spectrum that is likely to be used to develop therapeutics to fight SARS-CoV-2 induced acute lung injury. Therefore, the symptoms may be greatly alleviated through the application of a direct antiviral combined with an inflammasome inhibitor. Nevertheless, further clinical trials have to be carried out to confirm this statement. To ensure the safety of such anti-inflammatory drugs, other non-identified inflammasome that might exist and counteract with SARS-Cov-2 has to be taken in consideration. Moreover, NLRP3 inhibitors does not only suppresses IL-1 but IL-18 as well which is an important regulator for the innate system. This fact may produce an unexpected pathophysiological consequences through a complicated immune network. However, the development of inflammasome inhibitors still hold a promise to treat severe COVID-19 syndrome effectively and eventually reduce mortality.
Author contributions
KH, RD: conceptualization, literature review, critical and comparative analysis of available information. RD: original manuscript writing and figure preparation. KH: supervision, revision and funding acquisition. YW, DH: comments and suggestion. All authors contributed to the article and approved the submitted version.
Funding
This work was supported by the Key R&D Project (Social Development) of Hubei Province, China (2022BCA018) and by the Cooperative Innovation Center of Industrial Fermentation (Ministry of Education & Hubei Province), China (2022KF16) to KH.
Conflict of interest
The authors declare that the research was conducted in the absence of any commercial or financial relationships that could be construed as a potential conflict of interest.
Publisher’s note
All claims expressed in this article are solely those of the authors and do not necessarily represent those of their affiliated organizations, or those of the publisher, the editors and the reviewers. Any product that may be evaluated in this article, or claim that may be made by its manufacturer, is not guaranteed or endorsed by the publisher.
Abbreviations
ACE-2, cellular angiotensin-converting enzyme 2; AIM-2, absent in melanoma-2; ARDS, acute respiratory distress syndrome; ASC, apoptosis-associated speck-like protein containing a caspase recruitment domain; BHB, β-Hydroxybutyrate; BPOZ-2, Blood POZ-containing gene type-2; CAPS, cryopyrin-associated periodic syndrome; cfDNA, cell free DNA; COVID-19, Corona Virus Disease-19; DMF, dimethyl fumarate; DSF, disulfiram; ER, endoplasmic reticulum; EV-A71, enterovirus-A71; FDA, food and drug administration; GSDMD, Gasdermin D; IL-18, interleukin-18; IL-1β, interleukin-1 beta; LDH, lactate dehydrogenase; NF-KB, Nuclear factor kappa B; NLR, NOD-like receptor; NLRP3, NLR family pyrin domain containing 3; ORFs, open reading frames; PBMCs, peripheral blood mononuclear cells; RIG-I, retinoic acid-inducible gene I; RNAi, RNA interference; siRNA, small interference RNA; TLRs, toll-like receptors.
References
Andargie, T. E., Tsuji, N., Seifuddin, F., Jang, M. K., Yuen, P. S., Kong, H., et al. (2021). Cell-free DNA maps COVID-19 tissue injury and risk of death and can cause tissue injury. JCI Insight 6 (7), e147610. doi: 10.1172/jci.insight.147610
Awadasseid, A., Wu, Y., Tanaka, Y., Zhang, W. (2021). SARS-CoV-2 variants evolved during the early stage of the pandemic and effects of mutations on adaptation in wuhan populations. Int. J. Biol. Sci. 17, 97–106. doi: 10.7150/ijbs.47827
Bauernfried, S., Scherr, M. J., Pichlmair, A., Duderstadt, K. E., Hornung, V. (2021). Human NLRP1 is a sensor for double-stranded RNA. Sci. (New York N.Y.) 371(6528), eabd0811. doi: 10.1126/science.abd0811
Burdette, B. E., Esparza, A. N., Zhu, H., Wang, S. (2021). Gasdermin d in pyroptosis. Acta Pharm. Sinica. B 11, 2768–2782. doi: 10.1016/j.apsb.2021.02.006
Cameroni, E., Bowen, J. E., Rosen, L. E., Saliba, C., Zepeda, S. K., Culap, K., et al. (2022). Broadly neutralizing antibodies overcome SARS-CoV-2 omicron antigenic shift. Nature 602, 664–670. doi: 10.1038/s41586-021-04386-2
Campbell, G. R., To, R. K., Hanna, J., Spector, S. A. (2021). SARS-CoV-2, SARS-CoV-1, and HIV-1 derived ssRNA sequences activate the NLRP3 inflammasome in human macrophages through a non-classical pathway. iScience 24, 102295. doi: 10.1016/j.isci.2021.102295
Chu, D. T., Vu Ngoc, S. M., Vu Thi, H., Nguyen Thi, Y. V., Ho, T. T., Hoang, V. T., et al. (2022). COVID-19 in southeast Asia: current status and perspectives. Bioengineered 13, 3797–3809. doi: 10.1080/21655979.2022.2031417
Colarusso, C., Terlizzi, M., Maglio, A., Molino, A., Candia, C., Vitale, C., et al. (2022). Activation of the AIM2 receptor in circulating cells of post-COVID-19 patients with signs of lung fibrosis is associated with the release of IL-1α, IFN-α and TGF-β. Front. Immunol. 13. doi: 10.3389/fimmu.2022.934264
Declercq, J., De Leeuw, E., Lambrecht, B. N. (2022). Inflammasomes and IL-1 family cytokines in SARS-CoV-2 infection: from prognostic marker to therapeutic agent. Cytokine 157, 155934. doi: 10.1016/j.cyto.2022.155934
Eltobgy, M. M., Zani, A., Kenney, A. D., Estfanous, S., Kim, E., Badr, A., et al. (2022). Caspase-4/11 exacerbates disease severity in SARS-CoV-2 infection by promoting inflammation and immunothrombosis. Proc Natl Acad Sci U S A. 119 (21), e2202012119. doi: 10.1073/pnas.2202012119
Feng, N., Liang, L., Fan, M., Du, Y., Chen, C., Jiang, R., et al. (2021). Treating autoimmune inflammatory diseases with an siERN1-nanoprodrug that mediates macrophage polarization and blocks toll-like receptor signaling. ACS nano 15, 15874–15891. doi: 10.1021/acsnano.1c03726
Fillmore, N., Bell, S., Shen, C., Nguyen, V., La, J., Dubreuil, M., et al. (2021). Disulfiram use is associated with lower risk of COVID-19: a retrospective cohort study. PloS One 16, e0259061. doi: 10.1371/journal.pone.0259061
Fox, T., Geppert, J., Dinnes, J., Scandrett, K., Bigio, J., Sulis, G., et al. (2022). Antibody tests for identification of current and past infection with SARS-CoV-2. Cochrane Database systematic Rev. 11, CD013652. doi: 10.1002/14651858.CD013652.pub2
Freeman, T. L., Swartz, T. H. (2020). Targeting the NLRP3 inflammasome in severe COVID-19. Front. Immunol. 11. doi: 10.3389/fimmu.2020.01518
Gordon, R., Albornoz, E. A., Christie, D. C., Langley, M. R., Kumar, V., Mantovani, S., et al. (2018). Inflammasome inhibition prevents α-synuclein pathology and dopaminergic neurodegeneration in mice. Sci. Trans. Med. 10 (465), eaah4066. doi: 10.1126/scitranslmed.aah4066
Gupta, R. (2020). Anakinra: a silver lining in COVID-19? Crit. Care (London England) 24, 598. doi: 10.1186/s13054-020-03312-8
Hadj Hassine, I. (2022). Covid-19 vaccines and variants of concern: a review. Rev. Med. Virol. 32, e2313. doi: 10.1002/rmv.2313
Harvey, W. T., Carabelli, A. M., Jackson, B., Gupta, R. K., Thomson, E. C., Harrison, E. M., et al. (2021). SARS-CoV-2 variants, spike mutations and immune escape. Nat. Rev. Microbiol. 19, 409–424. doi: 10.1038/s41579-021-00573-0
He, L., Ding, Y., Zhang, Q., Che, X., He, Y., Shen, H., et al. (2006). Expression of elevated levels of pro-inflammatory cytokines in SARS-CoV-infected ACE2+ cells in SARS patients: relation to the acute lung injury and pathogenesis of SARS. J. Pathol. 210, 288–297. doi: 10.1002/path.2067
He, H., Jiang, H., Chen, Y., Ye, J., Wang, A., Wang, C., et al. (2018). Oridonin is a covalent NLRP3 inhibitor with strong anti-inflammasome activity. Nat. Commun. 9, 2550. doi: 10.1038/s41467-018-04947-6
Huang, Y., Jiang, H., Chen, Y., Wang, X., Yang, Y., Tao, J., et al. (2018). Tranilast directly targets NLRP3 to treat inflammasome-driven diseases. EMBO Mol. Med. 10 (4), e8689. doi: 10.15252/emmm.201708689
Huang, C., Wang, Y., Li, X., Ren, L., Zhao, J., Hu, Y., et al. (2020). Clinical features of patients infected with 2019 novel coronavirus in wuhan, China. Lancet (London England) 395, 497–506. doi: 10.1016/s0140-6736(20)30183-5
Idris, A., Davis, A., Supramaniam, A., Acharya, D., Kelly, G., Tayyar, Y., et al. (2021). A SARS-CoV-2 targeted siRNA-nanoparticle therapy for COVID-19. Mol. Ther. J. Am. Soc. Gene Ther. 29, 2219–2226. doi: 10.1016/j.ymthe.2021.05.004
Jiang, H., He, H., Chen, Y., Huang, W., Cheng, J., Ye, J., et al. (2017). Identification of a selective and direct NLRP3 inhibitor to treat inflammatory disorders. J. Exp. Med. 214, 3219–3238. doi: 10.1084/jem.20171419
Junqueira, C., Crespo, Â., Ranjbar, S., Lewandrowski, M., Ingber, J., de Lacerda, L. B., et al. (2021). SARS-CoV-2 infects blood monocytes to activate NLRP3 and AIM2 inflammasomes, pyroptosis and cytokine release. Res. Square. rs.3.rs–153628. doi: 10.21203/rs.3.rs-153628/v1
Kaivola, J., Nyman, T. A., Matikainen, S. (2021). Inflammasomes and SARS-CoV-2 infection. Viruses 13 (12), 2513. doi: 10.3390/v13122513
Khan, N. A. (2020). Anakinra for severe forms of COVID-19. Lancet Rheumatol. 2, e586–e587. doi: 10.1016/s2665-9913(20)30273-3
Kim, D., Lee, J. Y., Yang, J. S., Kim, J. W., Kim, V. N., Chang, H. (2020). The architecture of SARS-CoV-2 transcriptome. Cell 181, 914–921.e910. doi: 10.1016/j.cell.2020.04.011
Kruse, R. L. (2020). Therapeutic strategies in an outbreak scenario to treat the novel coronavirus originating in wuhan, China. F1000Research 9, 72. doi: 10.12688/f1000research.22211.2
Kumar, S., Thambiraja, T. S., Karuppanan, K., Subramaniam, G. (2022). Omicron and delta variant of SARS-CoV-2: a comparative computational study of spike protein. J. Med. Virol. 94, 1641–1649. doi: 10.1002/jmv.27526
Lee, S., Channappanavar, R., Kanneganti, T. D. (2020). Coronaviruses: innate immunity, inflammasome activation, inflammatory cell death, and cytokines. Trends Immunol. 41, 1083–1099. doi: 10.1016/j.it.2020.10.005
Lee, H., Shin, E. A., Lee, J. H., Ahn, D., Kim, C. G., Kim, J. H., et al. (2018). Caspase inhibitors: a review of recently patented compounds (2013-2015). Expert Opin. Ther. patents 28, 47–59. doi: 10.1080/13543776.2017.1378426
Li, J., Lin, H., Fan, T., Huang, L., Zhang, X., Tai, Y., et al. (2023). BPOZ-2 is a negative regulator of the NLPR3 inflammasome contributing to SARS-CoV-2-induced hyperinflammation. Front. Cell. infection Microbiol. 13. doi: 10.3389/fcimb.2023.1134511
Li, S., Zhang, Y., Guan, Z., Li, H., Ye, M., Chen, X., et al. (2020). SARS-CoV-2 triggers inflammatory responses and cell death through caspase-8 activation. Signal transduction targeted Ther. 5, 235. doi: 10.1038/s41392-020-00334-0
Liu, X., Zhang, Z., Ruan, J., Pan, Y., Magupalli, V. G., Wu, H., et al. (2016). Inflammasome-activated gasdermin d causes pyroptosis by forming membrane pores. Nature 535, 153–158. doi: 10.1038/nature18629
Lugrin, J., Martinon, F. (2018). The AIM2 inflammasome: sensor of pathogens and cellular perturbations. Immunol. Rev. 281, 99–114. doi: 10.1111/imr.12618
Maes, M., Tedesco Junior, W. L. D., Lozovoy, M. A. B., Mori, M. T. E., Danelli, T., Almeida, E. R. D., et al. (2022). In COVID-19, NLRP3 inflammasome genetic variants are associated with critical disease and these effects are partly mediated by the sickness symptom complex: a nomothetic network approach. Mol. Psychiatry 27, 1945–1955. doi: 10.1038/s41380-021-01431-4
Majumder, J., Minko, T. (2021). Recent developments on therapeutic and diagnostic approaches for COVID-19. AAPS J. 23, 14. doi: 10.1208/s12248-020-00532-2
Mantero, V., Abate, L., Basilico, P., Balgera, R., Salmaggi, A., Nourbakhsh, B., et al. (2021). COVID-19 in dimethyl fumarate-treated patients with multiple sclerosis. J. Neurol. 268, 2023–2025. doi: 10.1007/s00415-020-10015-1
Marchetti, C., Swartzwelter, B., Gamboni, F., Neff, C. P., Richter, K., Azam, T., et al. (2018). OLT1177, a β-sulfonyl nitrile compound, safe in humans, inhibits the NLRP3 inflammasome and reverses the metabolic cost of inflammation. Proc Natl Acad Sci U S A. 115 (7), E1530–E1539. doi: 10.1073/pnas.1716095115
Mardi, A., Meidaninikjeh, S., Nikfarjam, S., Majidi Zolbanin, N., Jafari, R. (2021). Interleukin-1 in COVID-19 infection: immunopathogenesis and possible therapeutic perspective. Viral Immunol. 34, 679–688. doi: 10.1089/vim.2021.0071
Martinon, F., Burns, K., Tschopp, J. (2002). The inflammasome: a molecular platform triggering activation of inflammatory caspases and processing of proIL-beta. Mol. Cell 10, 417–426. doi: 10.1016/s1097-2765(02)00599-3
Mehta, A., Michler, T., Merkel, O. M. (2021). siRNA therapeutics against respiratory viral infections-what have we learned for potential COVID-19 therapies? Advanced healthcare materials 10, e2001650. doi: 10.1002/adhm.202001650
Mullard, A. (2019). NLRP3 inhibitors stoke anti-inflammatory ambitions. Nat. Rev. Drug Discovery 18, 405–407. doi: 10.1038/d41573-019-00086-9
Pan, P., Shen, M., Yu, Z., Ge, W., Chen, K., Tian, M., et al. (2021). SARS-CoV-2 n protein promotes NLRP3 inflammasome activation to induce hyperinflammation. Nat. Commun. 12, 4664. doi: 10.1038/s41467-021-25015-6
Pan, L., Tang, W. D., Wang, K., Fang, Q. F., Liu, M. R., Wu, Z. X., et al. (2022). Novel caspase-1 inhibitor CZL80 improves neurological function in mice after progressive ischemic stroke within a long therapeutic time-window. Acta pharmacologica Sin. 43, 2817–2827. doi: 10.1038/s41401-022-00913-7
Peng, Y., Mentzer, A. J., Liu, G., Yao, X., Yin, Z., Dong, D., et al. (2020). Broad and strong memory CD4(+) and CD8(+) T cells induced by SARS-CoV-2 in UK convalescent individuals following COVID-19. Nat. Immunol. 21, 1336–1345. doi: 10.1038/s41590-020-0782-6
Planès, R., Pinilla, M., Santoni, K., Hessel, A., Passemar, C., Lay, K., et al. (2022). Human NLRP1 is a sensor of pathogenic coronavirus 3CL proteases in lung epithelial cells. Mol. Cell 82, 2385–2400.e2389. doi: 10.1016/j.molcel.2022.04.033
Premeaux, T. A., Yeung, S. T., Bukhari, Z., Bowler, S., Alpan, O., Gupta, R., et al. (2022). Emerging insights on caspases in COVID-19 pathogenesis, sequelae, and directed therapies. Front. Immunol. 13. doi: 10.3389/fimmu.2022.842740
Robinson, P. C., Liew, D. F. L., Tanner, H. L., Grainger, J. R., Dwek, R. A., Reisler, R. B., et al. (2022). COVID-19 therapeutics: challenges and directions for the future. Proc Natl Acad Sci U S A. 119 (15), e2119893119. doi: 10.1073/pnas.2119893119
Robinson, K. S., Teo, D. E. T., Tan, K. S., Toh, G. A., Ong, H. H., Lim, C. K., et al. (2020). Enteroviral 3C protease activates the human NLRP1 inflammasome in airway epithelia. Sci. (New York N.Y.) 370 (6521), eaay2002. doi: 10.1126/science.aay2002
Rodrigues, T. S., de Sá, K. S. G., Ishimoto, A. Y., Becerra, A., Oliveira, S., Almeida, L., et al. (2021). Inflammasomes are activated in response to SARS-CoV-2 infection and are associated with COVID-19 severity in patients. J. Exp. Med. 218 (3), e20201707. doi: 10.1084/jem.20201707
Saw, P. E., Song, E. W. (2020). siRNA therapeutics: a clinical reality. Sci. China. Life Sci. 63, 485–500. doi: 10.1007/s11427-018-9438-y
Scovino, A. M., Dahab, E. C., Vieira, G. F., Freire-de-Lima, L., Freire-de-Lima, C. G., Morrot, A. (2022). SARS-CoV-2's variants of concern: a brief characterization. Front. Immunol. 13. doi: 10.3389/fimmu.2022.834098
Sola, I., Almazán, F., Zúñiga, S., Enjuanes, L. (2015). Continuous and discontinuous RNA synthesis in coronaviruses. Annu. Rev. Virol. 2, 265–288. doi: 10.1146/annurev-virology-100114-055218
Viganò, E., Diamond, C. E., Spreafico, R., Balachander, A., Sobota, R. M., Mortellaro, A. (2015). Human caspase-4 and caspase-5 regulate the one-step non-canonical inflammasome activation in monocytes. Nat. Commun. 6, 8761. doi: 10.1038/ncomms9761
Vora, S. M., Lieberman, J., Wu, H. (2021). Inflammasome activation at the crux of severe COVID-19. Nat. Rev. Immunol. 21, 694–703. doi: 10.1038/s41577-021-00588-x
Wang, D., Hu, B., Hu, C., Zhu, F., Liu, X., Zhang, J., et al. (2020). Clinical characteristics of 138 hospitalized patients with 2019 novel coronavirus-infected pneumonia in wuhan, China. Jama 323, 1061–1069. doi: 10.1001/jama.2020.1585
Wu, J., Lan, Y., Shi, X., Huang, W., Li, S., Zhang, J., et al. (2022). Sennoside a is a novel inhibitor targeting caspase-1. Food Funct. 13, 9782–9795. doi: 10.1039/d2fo01730j
Xu, H., Akinyemi, I. A., Chitre, S. A., Loeb, J. C., Lednicky, J. A., McIntosh, M. T., et al. (2022). SARS-CoV-2 viroporin encoded by ORF3a triggers the NLRP3 inflammatory pathway. Virology 568, 13–22. doi: 10.1016/j.virol.2022.01.003
Yap, J. K. Y., Moriyama, M., Iwasaki, A. (2020). Inflammasomes and pyroptosis as therapeutic targets for COVID-19. J. Immunol. (Baltimore Md. 1950) 205, 307–312. doi: 10.4049/jimmunol.2000513
Youm, Y. H., Nguyen, K. Y., Grant, R. W., Goldberg, E. L., Bodogai, M., Kim, D., et al. (2015). The ketone metabolite β-hydroxybutyrate blocks NLRP3 inflammasome-mediated inflammatory disease. Nat. Med. 21, 263–269. doi: 10.1038/nm.3804
Zeng, B., Gao, L., Zhou, Q., Yu, K., Sun, F. (2022). Effectiveness of COVID-19 vaccines against SARS-CoV-2 variants of concern: a systematic review and meta-analysis. BMC Med. 20, 200. doi: 10.1186/s12916-022-02397-y
Keywords: SARS-CoV-2, inflammasome, inhibitors, innate immune response, NOD-like receptor family pyrin domain containing 3 (NLRP3)
Citation: Diarimalala RO, Wei Y, Hu D and Hu K (2023) Inflammasomes during SARS-CoV-2 infection and development of their corresponding inhibitors. Front. Cell. Infect. Microbiol. 13:1218039. doi: 10.3389/fcimb.2023.1218039
Received: 06 May 2023; Accepted: 26 May 2023;
Published: 09 June 2023.
Edited by:
Yongkui Li, Jinan University, ChinaCopyright © 2023 Diarimalala, Wei, Hu and Hu. This is an open-access article distributed under the terms of the Creative Commons Attribution License (CC BY). The use, distribution or reproduction in other forums is permitted, provided the original author(s) and the copyright owner(s) are credited and that the original publication in this journal is cited, in accordance with accepted academic practice. No use, distribution or reproduction is permitted which does not comply with these terms.
*Correspondence: Kanghong Hu, hukh@hbut.edu.cn