- 1Department of Trauma Surgery, University Hospital Regensburg, Regensburg, Germany
- 2Department of Orthopaedic Surgery, University Hospital of Regensburg, Asklepios Klinikum Bad Abbach, Bad Abbach, Germany
- 3Nuffield Orthopaedic Centre, Oxford University Hospitals National Health Service (NHS) Foundation Trust, Oxford, United Kingdom
Background: Identifying novel biomarkers that are both specific and sensitive to periprosthetic joint infection (PJI) has the potential to improve diagnostic accuracy and ultimately enhance patient outcomes. Therefore, the aim of this systematic review is to identify and evaluate the effectiveness of novel biomarkers for the diagnosis of PJI.
Methods: We searched the MEDLINE, EMBASE, PubMed, and Cochrane Library databases from January 1, 2018, to September 30, 2022, using the search terms “periprosthetic joint infection,” “prosthetic joint infection,” or “periprosthetic infection” as the diagnosis of interest and the target index, combined with the term “marker.” We excluded articles that mentioned established biomarkers such as CRP, ESR, Interleukin 6, Alpha defensin, PCT (procalcitonin), and LC (leucocyte cell count). We used the MSIS, ICM, or EBJS criteria for PJI as the reference standard during quality assessment.
Results: We collected 19 studies that analyzed fourteen different novel biomarkers. Proteins were the most commonly analyzed biomarkers (nine studies), followed by molecules (three studies), exosomes (two studies), DNA (two studies), interleukins (one study), and lysosomes (one study). Calprotectin was a frequently analyzed and promising marker. In the scenario where the threshold was set at ≥50-mg/mL, the calprotectin point-of-care (POC) performance showed a high sensitivity of 98.1% and a specificity of 95.7%.
Conclusion: None of the analyzed biomarkers demonstrated outstanding performance compared to the established parameters used for standardized treatment based on established PJI definitions. Further studies are needed to determine the benefit and usefulness of implementing new biomarkers in diagnostic PJI settings.
1 Introduction
Periprosthetic joint infection (PJI) is a devastating complication that can occur after total joint arthroplasty (TJA). It often requires long-term antibiotic therapy and multiple revision surgeries, and even with optimal treatment, it can significantly decrease a patient’s quality of life. Additionally, the risk of mortality is high for those affected by PJI (Wildeman et al., 2021). In addition to the significant impact on individual patients, the socioeconomic burden of PJI is substantial and expected to increase in the future (Peel et al., 2013) The one-year and five-year risk of PJI after total hip arthroplasty (THA) is 0.7% and 1.1%, respectively, while for total knee arthroplasty (TKA), the corresponding figures are 0.7% and 1.4% (Kurtz et al., 2018). The overall one-year and five-year survival rates after PJI diagnosis were 88.7% and 67.2% for THA and 91.7% and 71.7% for TKA, respectively (Kurtz et al., 2018). In Germany, the total number of total joint arthroplasty (TJA) procedures is projected to increase by 45% for TKA and 23% for THA between now and 2040 (Rupp et al., 2020). Similarly, in the US, the incidence rate of primary TKAs is projected to increase by 43% from 2020 to 2050 (Klug et al., 2021). Among these revision surgeries, the biggest share is performed due to PJI (25%), followed by mechanical loosening (19%) as the second most common reason for TKA revisions (Bozic et al., 2015).
The treatment of infected and aseptic failures after TJA differs significantly and can impose a significant burden on patients (Andersson et al., 2010). Therefore, it is essential to avoid treating a non-infected joint as an infected one, and vice versa, as this can lead to increased morbidity, unnecessary costs, and avoidable surgical interventions (Moojen et al., 2014). Accurate diagnostics are thus of paramount importance in clinical practice to ensure appropriate treatment and avoid these adverse outcomes (Kurtz et al., 2022). In some cases, diagnosing PJI is straightforward, as clear clinical findings such as the presence of a sinus tract or pus around the implanted prosthesis are considered confirming diagnostic criteria (Parvizi et al., 2018; McNally et al., 2021). However, in many cases, these confirming criteria are not present, making PJI diagnostics challenging (Balato et al., 2020). Diagnosis typically relies on laboratory tests such as serology or synovial fluid analysis, microbiological analysis of tissue specimens or synovial fluid, as well as histological and radiographic findings. In recent years, efforts have been made to improve diagnostic accuracy. In 2011, the Musculoskeletal Infection Society (MSIS) published PJI criteria that classify “major” criteria, including the presence of a communicating sinus tract and two positive periprosthetic cultures, and “minor” criteria, such as elevated ESR/CRP, elevated synovial leukocyte count, elevated synovial polymorphonuclear (PMN)%, purulent material, isolated organism in one culture, and intraoperative frozen sections with histology (Parvizi et al., 2011). In 2013, the Infectious Diseases Society of America (IDSA) provided its own PJI diagnostic criteria with the aim of standardizing diagnostics (Osmon et al., 2013). Unlike the MSIS criteria, the IDSA criteria do not include elevated inflammatory markers but consider other factors such as the growth of a virulent organism from a single culture or the presence of acute inflammation from histopathology of the periprosthetic tissue. In 2013, the International Consensus Meeting (ICM) introduced a new minor criterion - the leukocyte esterase in synovial fluid measured by a urine strip test. Later, Parvizi et al. updated the ICM concept by introducing a scoring system based on the different sensitivity and specificity of the markers in 2018 (Parvizi et al., 2018). This updated system included promising new markers such as alpha-defensin in synovial fluid and D-dimer in serum. In 2021, the European Bone and Joint Infection Society (EBJIS) criteria were introduced, classifying cases as “unlikely,” “likely,” and “confirmed” All these criteria rely on various clinical, laboratory, microbiological, and histological analyses, as well as intraoperative findings, to establish a diagnosis (see Table 1).
Despite the improvements made in recent decades that have made correct diagnosis of PJI more likely through the introduction of different PJI criteria, the identification of a novel biomarker that is highly specific and sensitive for PJI could enable easier and more accurate diagnosis of this devastating disease, ultimately improving patient outcomes. Therefore, the objective of this systematic review is to identify and evaluate novel biomarkers for preoperative PJI diagnostics.
2 Materials and methods
2.1 Search strategy
This systematic review of the literature was performed according to the preferred Reporting Items for Systematic Reviews and Meta-Analyses guidelines (Page et al., 2021).We searched in the electronic databases MEDLINE, EMBASE, PubMed and Cochrane Library. The following search terms were used to screen literature that utilized new marker for PJI diagnosis: We used “periprosthetic joint infection” OR “prosthetic joint infection” OR “periprosthetic infection” as the diagnosis of interest and the target index applied AND “marker”. To focus on novel biomarkers already used biomarkers of the established PJI diagnostic criteria of MSIS, ICM and EBJIS were not included in the analysis. Therefore, the search terms included NOT “CRP”, NOT “ESR”, NOT “Interleukin 6”, NOT “Alpha defensin”, NOT “PCT” (Procalcitonin), NOT “LC” (leucocyte cell count). A second approach to only include novel biomarkers was setting the time frame for study inclusion from January 1, 2018 to September 30, 2022. After identification, all records were screened by two independent reviewers for the diagnostic markers either determined from blood samples or synovial fluid. All included articles had to be published in English. Animal studies, and studies investigating histological diagnostics were excluded from the analysis.
2.2 Data extraction and quality assessment
Two reviewers performed data extraction independently, and divergences was discussed with a third reviewer. Data were extracted from the eligible studies including the author names, year of publication, country, total number of participants (PJI/control group), mean age of the patients, level of evidence, study design, biomarker, sample type, sample part, sample collection, reference standard and sensitivity, specificity or cut-off of the new marker.
The Quality Assessment of Diagnostic Accuracy Studies-2 (QUADAS-2) tool was used to determine the potential risk of bias of each study following the full-text assessment (Whiting et al., 2011). MSIS, ICM or EBJS criteria for PJI were considered the reference standard during quality assessment.
An application to register this review in the International Prospective Register of Systematic Reviews (PROSPERO) was submitted but rejected because of study prioritization focusing on SARS- CoV-2 infections.
3 Results
3.1 Search results
The electronic database and bibliography search identified 149 studies, of which 130 were excluded after title/abstract and full text evaluation (see Figure 1 and Table 2). Therefore, 19 studies met the inclusion criteria. Of these, fifteen studies (79%) had prospective designs, and the remaining four (22%) were retrospective studies. Six studies (33%) focused solely on periprosthetic knee infections, while thirteen (67%) included both periprosthetic knee and hip infections. All studies provided diagnostic data for periprosthetic hip and knee infections based on the MSIS, EBJIS, or ICM criteria. The number of patients in the selected studies ranged from 23 to 224. Among the selected studies, 15 (79%) analyzed synovial fluid, three (16%) analyzed blood, and one (5%) analyzed urine (Table 3). The different biomarker analyses are shown in Tables 4 and 5.
The quality of all selected studies was evaluated using the QUADAS-2 tool, and the results are presented in Table 6.
Proteins were primarily analyzed as potential markers, with calprotectin being a frequently studied novel marker (Warren et al., 2021; Grassi et al., 2022; Warren et al., 2022) (Table 2). In one study, the calprotectin point-of-care (POC) performance showed a sensitivity of 98.1% and a specificity of 95.7% in a scenario with a threshold of ‡50-mg/mL (Figures 2, 3) (Warren et al., 2021).
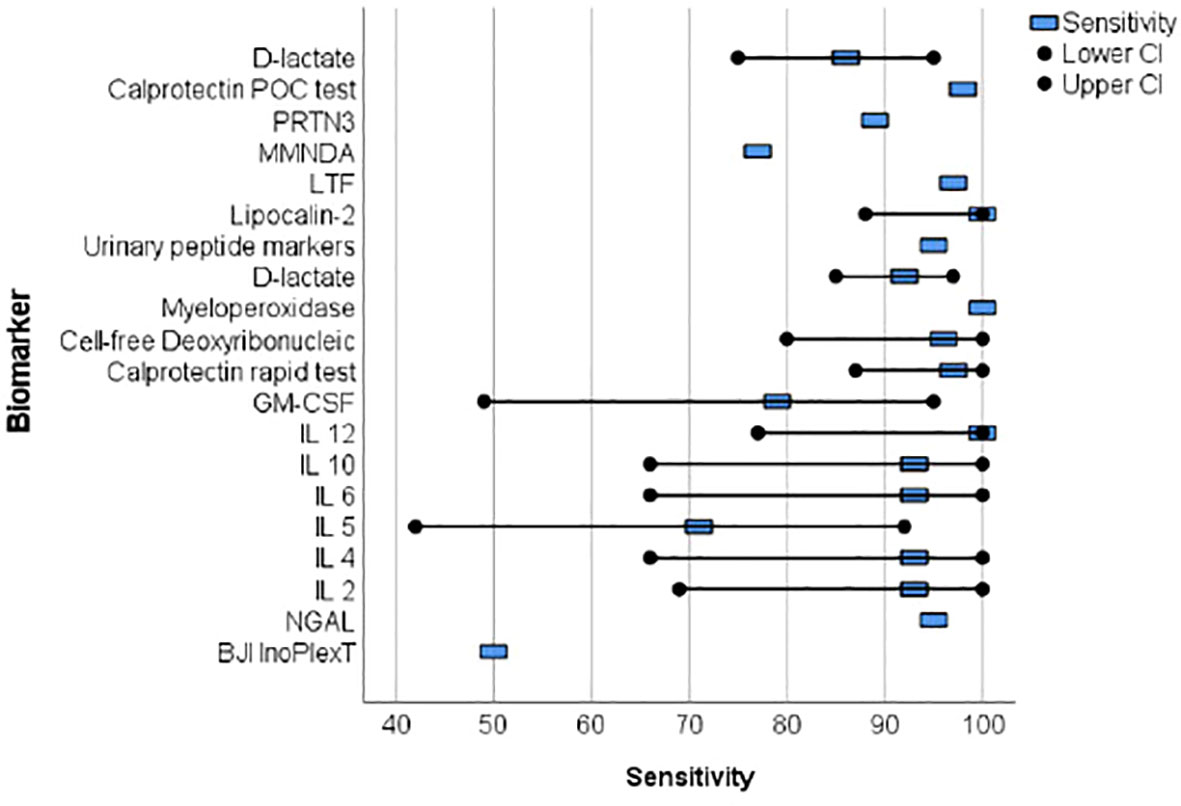
Figure 2 The forest plots of the sensitivity for novel diagnosis marker of periprosthetic joint infection.
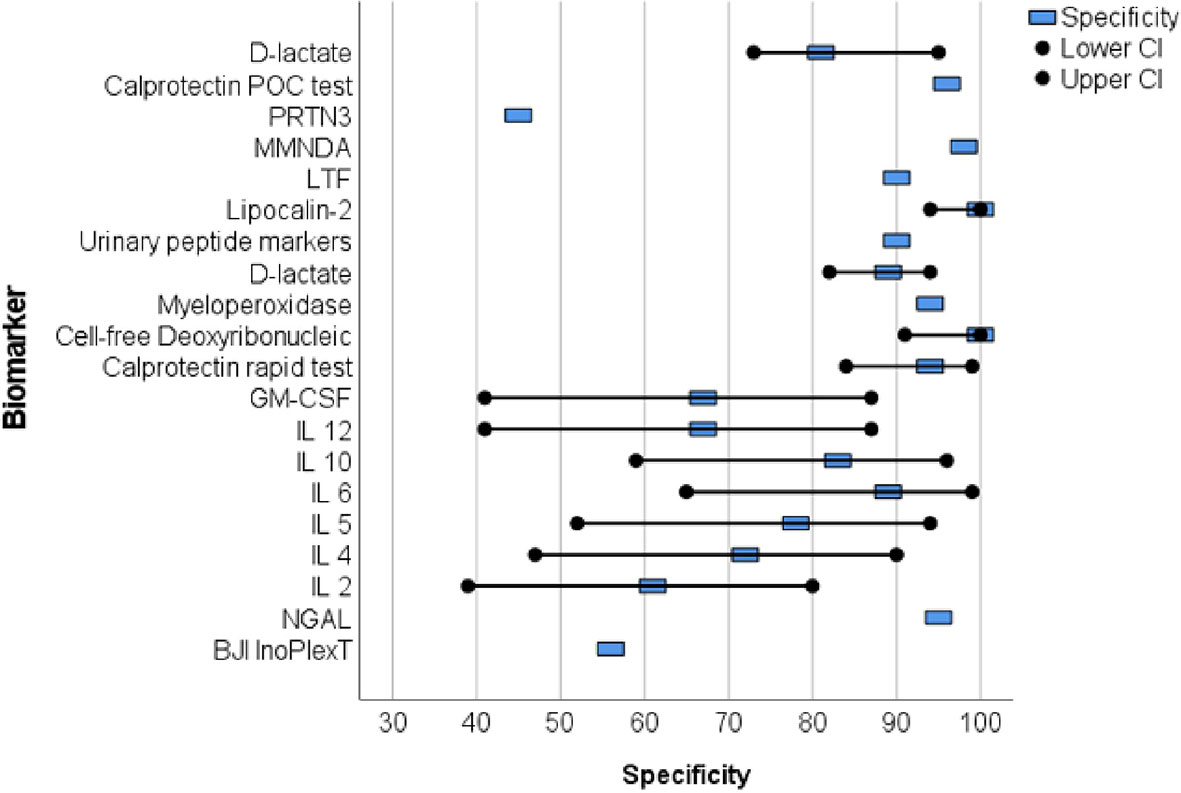
Figure 3 The forest plots of the specificity for novel diagnostic marker of periprosthetic joint infection.
Wang et al. collected 50 synovial fluid aspirates from hips and knees and verified the most promising proteins using ELISA (enzyme-linked immunosorbent assay) (Wang et al., 2019). The study identified that lactoferrin (LTF), myeloid nuclear differentiation antigen (MNDA), and polymorphonuclear leukocyte serine protease 3 (PRTN3) were sensitive, while LTF and MNDA were specific for diagnosing PJI. A retrospective cohort study analyzed only TKA synovial fluid and when applying the MSIS criteria, neutrophil gelatinase-associated lipocalin (NGAL) revealed 92% sensitivity and 83% specificity (Dijkman et al., 2020) (Figures 2, 3).
An additional novel approach to diagnose PJI involves analyzing the pattern of urinary peptide excretion. In a study analyzing urinary samples from 30 patients prior to surgery, a marker model consisting of 83 peptides demonstrated the best diagnostic performance with a sensitivity of 95% and specificity of 90% for diagnosing PJI (Omar et al., 2021) (Figures 2, 3). In a study by Vergara et al., synovial fluid was collected from 30.6% of patients with proven infections, 30.6% with aseptic implant failures, and 38.8% controls. Lipocalin-2 (LCN2) was found to discriminate nearly perfectly between controls and confirmed infections (Vergara et al., 2019) (Figure 2, 3). Soluble Pecam 1 (sPecam-1) is an immunologically reactive molecule that is removed from the surface of T cells upon activation by proinflammatory signals. Synovial samples were taken intraoperatively from 16 native knees, 20 aseptic knee revisions, and 22 knees with PJI. The amount of sPecam-1 was significantly greater in knees with PJI compared to aseptic TKA revision procedures (p ≤ 0.001) (Fuchs et al., 2021) (Table 5). In a prospective cohort study, Zonulin, soluble CD14 (sCD14), and lipopolysaccharide (LPS) were tested in blood samples before antibiotic administration. The study included 134 patients, of which 44 had PJI, 64 had aseptic failure, and 26 underwent primary TKA. Zonulin (7.642 ± 6.077 ng/mL vs 4.560 ± 3.833 ng/mL; p < 0.001) and sCD14 levels (555.721 ± 216.659 ng/mL vs 396.872 ± 247.920 ng/mL; p = 0.003) were significantly increased in PJI compared to non-infected cases (Chisari et al., 2022) (Table 5). Jubel et al. analyzed fourteen soluble immunoregulatory markers using bead-based multiplex assays and showed significant differences for nine markers when comparing PJI and control groups (Jubel et al., 2021) (Table 5). Extracellular vesicles (EVs) represent another group of the novel markers analyzed (Rüwald et al., 2020; Sallai et al., 2022). The concentration of EVs was significantly higher in the septic samples (p = 0.0105) and showed a different size pattern as compared to the aseptic ones (Table 4). Fröschen et al. evaluated a combination of six cytokines (IL-2, IL-4, IL-5, IL-6, lL-12 and GM-CSF) performed better in diagnosing chronic PJI than any cytokine alone. Regression analysis for this combination revealed a sensitivity of 100% and a specificity of 88.9% for a cut-of value of 0.41 (Fröschen et al., 2020). Myeloperoxidase (MPO) is a bactericidal enzyme that acts against pathogenic microorganisms, such as in PJI. In a small cohort study of 37 patients, MPO levels were significantly higher in the chronic PJI group than in the aseptic failure group (p < 0.001) (p < 0.001) (Ikeda et al., 2020) (Table 5). Another marker is cell-free deoxyribonucleic acid (cf-DNA) in synovial fluid and peripheral blood (Echeverria et al., 2021; Cobra et al., 2022). The sensitivity and specificity in synovial fluid were 96.2% and 100%, respectively (Figures 2, 3). BJI InoPlex is a multiplex ELISA that measures the immune response to certain bacterial species from Staphylococcus epidermidis, aureus and lugdunensis, Streptococcus B and Cutibacterium acnes. Dartus et al. included eleven hip and thirteen knee arthroplasty cases. The sensitivity for diagnosing a chronic PJI based on the 2018 ICM criteria was 50% and the specificity was 56% (Dartus et al., 2021) (Figure 2, 3).
D-lactate was studied in the largest cohort of patients (148 and 224) by Karbysheva et al. and Yermak et al. who used different PJI criteria. The sensitivity ranged from 86.4% to 94.3% and the specificity ranged from 78.4% to 80.8% with similar cutoffs (Yermak et al., 2019; Karbysheva et al., 2020) (Figures 2, 3).
4 Discussion
Over the last five years, 19 studies have reported on new biomarkers for PJI, with 15 of these studies specifically focused on parameters in the synovial fluid. Most of the studies analyzed proteins (nine studies), followed by molecules (three studies), exosomes (two studies), DNA (two studies), interleukins (one study), and lysosomes (one study). Calprotectin is a promising and frequently analyzed marker (Table 1) (Warren et al., 2021; Grassi et al., 2022; Warren et al., 2022). In scenarios with a threshold of ≥50 mg/mL, the calprotectin point-of-care performance showed a high sensitivity of 98.1% and specificity of 95.7% (Figures 2, 3). LCN2 is another hopeful marker that nearly perfectly discriminates between controls and confirmed infections in a small cohort of patients (72 patients/22 PJI) (Figures 2, 3) (Vergara et al., 2019). D-lactate, which has been analyzed in a large cohort of patients, is also noteworthy, revealing 94% sensitivity and 78% specificity (Figures 2, 3) (Yermak et al., 2019; Karbysheva et al., 2020). The sensitivity and specificity of these markers are comparable to those of established markers. A review by Sigmund et al. presented the performance of established and novel serum inflammatory biomarkers. The sensitivity and specificity of established markers such as erythrocyte sedimentation rate (ESR) or white blood cell count (WBC) demonstrated similar sensitivity and specificity in comparison to new markers. C-reactive protein (CRP) with a cut-off of 3-32mg/L showed a sensitivity of 62-100% and specificity of 64-96%, while procalcitonin demonstrated a maximum sensitivity of 90% and specificity of 100% (Sigmund et al., 2021).
More than two-thirds of the studies analyzed biomarkers from synovial fluid, but it’s important to note that diagnostic hip aspirations are unsuccessful in up to one-third of patients (Christensen et al., 2022). Five of the 19 studies analyzed only preoperative aspirates. Furthermore, there is a discordance of approximately 20% between preoperative aspirate culture and intraoperative synovial fluid culture, which makes relying solely on synovial fluid in the preoperative setting for diagnosing PJI challenging (Li et al., 2021). A meta-analysis of 14 studies that pooled preoperative aspiration culture data revealed an average sensitivity of 67.6% (range 28% to 100%) and an average specificity of 98.4% (range 96% to 100%) (Rodriguez-Merchan, 2018).
Inflammation triggers a series of signaling cascades, and different markers investigated in PJI are linked to these cascades, either up or down. For instance, calprotectin is secreted by neutrophils (Stríz and Trebichavský, 2004) which play a vital role in PJI diagnostics as PMN%. Likewise, the measurement of calprotectin in synovial fluid is significantly associated with PMN% and is an important marker for diagnosing PJI (Burri et al., 2013; Lisowska-Myjak et al., 2016; Honar et al., 2022). Similarly, D-lactate concentration is linked to microbial load. The concentration of D-lactate seems to depend on the number of bacteria, as higher levels of D-lactate were observed in culture-positive PJI compared to culture-negative PJI (Yermak et al., 2019). Given the interdependence of markers in the inflammation signaling cascades, it is not surprising that relying on a single new marker alone may not revolutionize PJI diagnostics.
Alpha defensin, which is a diagnostic marker included in the ICM 2018 criteria (Table 1), was initially hailed as a game-changing diagnostic marker. However, as it became known that alpha defensin is released by neutrophilic granulocytes and acts as part of the non-specific immune system, it was not surprising that the hoped-for diagnostic breakthrough was followed by disappointment. In the literature, the sensitivity of alpha-defensin for the diagnosis of PJI has been reported to range from 67% to 100%, and the specificity from 89% to 99% (Kasparek et al., 2016; Wyatt et al., 2016; Balato et al., 2020). Renz et al. calculated a sensitivity of 84% using the MSIS criteria, 67% using the IDSA criteria, and 54% using the PRO-IMPLANT/EBJIS criteria (Renz et al., 2018). Such variation according to the various criteria presents a challenge in clinical application. Therefore, the routine use of alpha-defensin testing is not recommended in the literature and should only be performed as an additional diagnostic test. Costs also have to be taken into account when using alpha-defensin for diagnostics. ELISA for alpha-defensin is much more expensive than the leukocyte esterase test strip (£0.11 [US$0.17] per test), costing around £500 [US$760] per test (Wyatt et al., 2016).
Besides cost, availability is a major concern for implementing new biomarkers in clinical practice. Established markers like CRP and synovial WBC are commonly used in medical centers, whereas newer markers like alpha defensin are rarely used for routine diagnosis of PJI. Alpha defensin is only collected in 19.4% of cases, while microbiological (97.7%), leukocyte count (74.8%), and PMN% (65.8%) are the most frequently measured parameters in diagnostic setting (Ahmad et al., 2016). Furthermore, storage of specimens poses a challenge as certain markers, such as cytokines and s-Pecam1, require specific temperatures during transportation, which can complicate logistics. As a result, introducing these markers in clinical practice can be difficult.
Modern genomic sequencing diagnostics may offer a solution to the challenges associated with biomarkers and conventional microbial diagnostics. While culture-based detection methods remain the gold standard, they are plagued by several limitations, including low sensitivity. Microbiological culture only detects the pathogen in 44-80% of cases (Malhotra and Morgan, 2004; Williams et al., 2004). One major factor that significantly affects the probability of detecting a pathogen through culture-based methods is the duration of the culture (Saleh et al., 2003; Schäfer et al., 2008). Additionally, contamination and resulting false positive findings can also be problematic (Yee et al., 2013). To overcome these limitations, culture-independent, molecular biology-based methods can be employed as an alternative diagnostic tool. In particular, plasmatic detection of circulating free DNA through Next Generation Sequencing (NGS) has shown promise as a diagnostic method for patients with bloodstream infections. Metagenomic NGS (mNGS) offers the ability to identify multiple organisms in a single sample (Gu et al., 2019). Early studies have suggested that NGS-based diagnostics are more effective than conventional culture-based methods for detecting bloodstream infections (Grumaz et al., 2016; Decker et al., 2017; Grumaz et al., 2019). In the case of PJI, Echeverria et al. identified the pathogen in 35 cases, including four cases that were deemed culture-negative (57%) (Echeverria et al., 2021). Having a pathogenic marker such as circulating free DNA could be beneficial as it specifically identifies present bacteria compared to nonspecific markers. Thus, NGS could be utilized to identify the pathogen in cases where culture-based methods are ineffective.
Several limitations of this systematic review must be acknowledged. First, the study compared three different PJI criteria, which are the most commonly used ones. The MSIS and ICM criteria were used in six studies. Sigmund et al. conducted a retrospective study of 206 PJI patients, of which 101 (49%) were diagnosed with PJI using the EBJIS definition, 99 (48%) with the IDSA definition, and 86 (42%) with the ICM definition. A total of 84 cases (41%) had an infection based on all three criteria. The novel EBJIS definition appears to be more sensitive for PJI diagnosis compared to the IDSA and ICM definitions. All infections classified by the IDSA or ICM criteria were identified by the EBJIS definition, indicating that the EBJIS definition is superior to the IDSA and ICM criteria for PJI diagnostics. However, only two studies in this systematic review used the EBJIS definition, which was introduced recently in 2021 (Sigmund et al., 2022). However, the present systematic review is limited by several factors. Secondly, only two studies in our review employed the recently introduced EBJIS definition, which limits the generalizability of our findings. Thirdly, the use of different cut-off values for biomarkers across studies makes comparison challenging. Finally, the limited availability of information on the time elapsed since the arthroplasty procedure may affect the accuracy of some biomarkers, as their diagnostic performance may vary in the early postoperative period (Yi et al., 2014).
5 Conclusion
Based on the current analysis, no novel biomarker investigated in the past five years for diagnosing PJI has been proven to outperform the already established diagnostic parameters. Further studies may demonstrate the usefulness of additional markers, such as calprotectin, in the established PJI diagnostic criteria.
Author contributions
SM, NW, and MR contributed to conception and design of the study. SM and NW organized the database. SM and NW performed the statistical analysis. SM wrote the first draft of the manuscript. NW and MR wrote sections of the manuscript. All authors contributed to the article and approved the submitted version.
Conflict of interest
The authors declare that the research was conducted in the absence of any commercial or financial relationships that could be construed as a potential conflict of interest.
Publisher’s note
All claims expressed in this article are solely those of the authors and do not necessarily represent those of their affiliated organizations, or those of the publisher, the editors and the reviewers. Any product that may be evaluated in this article, or claim that may be made by its manufacturer, is not guaranteed or endorsed by the publisher.
References
Ahmad, S. S., Becker, R., Chen, A. F., Kohl, S. (2016). EKA survey: diagnosis of prosthetic knee joint infection. Knee Surg. Sports Traumatol Arthrosc 24, 3050–3055. doi: 10.1007/s00167-016-4303-y
Andersson, A. E., Bergh, I., Karlsson, J., Nilsson, K. (2010). Patients' experiences of acquiring a deep surgical site infection: an interview study. Am. J. Infect. Control 38, 711–717. doi: 10.1016/j.ajic.2010.03.017
Balato, G., Matteo, V., Ascione, T., Di Donato, S.L., Franco, C., Smeraglia, F., et al. (2020). Laboratory-based versus qualitative assessment of α-defensin in periprosthetic hip and knee infections: a systematic review and meta-analysis. Arch. Orthop Trauma Surg. 140, 293–301. doi: 10.1007/s00402-019-03232-5
Bozic, K. J., Kamath, A. F., Ong, K., Lau, E., Kurtz, S., Chan, V., et al. (2015). Comparative epidemiology of revision arthroplasty: failed THA poses greater clinical and economic burdens than failed TKA. Clin. Orthop Relat. Res. 473, 2131–2138. doi: 10.1007/s11999-014-4078-8
Burri, E., Schulte, F., Muser, J., Meier, R., Beglinger, C. (2013). Measurement of calprotectin in ascitic fluid to identify elevated polymorphonuclear cell count. World J. Gastroenterol. 19, 2028–2036. doi: 10.3748/wjg.v19.i13.2028
Chisari, E., Cho, J., Wouthuyzen-Bakker, M., Parvizi, J. (2022). Gut permeability may be associated with periprosthetic joint infection after total hip and knee arthroplasty. Sci. Rep. 12, 15094. doi: 10.1038/s41598-022-19034-6
Christensen, T. H., Ong, J., Lin, D., Aggarwal, V. K., Schwarzkopf, R., Rozell, J. C., et al. (2022). How does a “Dry tap” impact the accuracy of preoperative aspiration results in predicting chronic periprosthetic joint infection? J. Arthroplasty 37, 925–929. doi: 10.1016/j.arth.2022.01.066
Cobra, H., Mozella, A. P., Da Palma, I. M., Salim, R., Leal, A. C. (2022). Cell-free deoxyribonucleic acid: a potential biomarker of chronic periprosthetic knee joint infection. J. Arthroplasty 37, 2455–2459. doi: 10.1016/j.arth.2022.07.002
Dartus, J., Martinot, P., Leclerc, J.-T., Senneville, E., Wallet, F., Putman, S., et al. (2021). Diagnostic accuracy of the BJI InoPlex™ (Diaxonhit) immunoassay on blood samples for periprosthetic joint infection in complex microbiological situations. preliminary results of 24 cases in a French reference center for complex bone and joint infection (CRIOAC). Orthop Traumatol Surg. Res. 107, 102909. doi: 10.1016/j.otsr.2021.102909
Decker, S. O., Sigl, A., Grumaz, C., Stevens, P., Vainshtein, Y., Zimmermann, S., et al. (2017). Immune-response patterns and next generation sequencing diagnostics for the detection of mycoses in patients with septic shock-results of a combined clinical and experimental investigation. Int. J. Mol. Sci. 8, 18. doi: 10.3390/ijms18081796
Dijkman, C., Thomas, A. R., Koenraadt, K. L. M., Ermens, A. A. M., van Geenen, R. C. I. (2020). Synovial neutrophilic gelatinase-associated lipocalin in the diagnosis of periprosthetic joint infection after total knee arthroplasty. Arch. Orthop Trauma Surg. 140, 941–947. doi: 10.1007/s00402-020-03427-1
Echeverria, A. P., Cohn, I. S., Danko, D. C., Shanaj, S., Blair, L., Hollemon, D., et al. (2021). Sequencing of circulating microbial cell-free DNA can identify pathogens in periprosthetic joint infections. J. Bone Joint Surg. Am. 103, 1705–1712. doi: 10.2106/JBJS.20.02229
Fröschen, F. S., Schell, S., Schildberg, F. A., Klausing, A., Kohlhof, H., Gravius, S., et al. (2020). Analysis of synovial biomarkers with a multiplex protein microarray in patients with PJI undergoing revision arthroplasty of the hip or knee joint. Arch. Orthop Trauma Surg. 140, 1883–1890. doi: 10.1007/s00402-020-03388-5
Fuchs, M., Trampuz, A., Kirschbaum, S., Winkler, T., Sass, F. A. (2021). Soluble pecam-1 as a biomarker in periprosthetic joint infection. J. Clin. Med. 10 (4), 612. doi: 10.3390/jcm10040612
Grassi, M., Salari, P., Farinelli, L., D'Anzeo, M., Onori, N., Gigante, A. (2022). Synovial biomarkers to detect chronic periprosthetic joint infection: a pilot study to compare calprotectin rapid test, calprotectin ELISA immunoassay and leukocyte esterase test. J. Arthroplasty 37, 781–786. doi: 10.1016/j.arth.2021.12.040
Grumaz, S., Grumaz, C., Vainshtein, Y., Stevens, P., Glanz, K., Decker, S. O., et al. (2019). Enhanced performance of next-generation sequencing diagnostics compared with standard of care microbiological diagnostics in patients suffering from septic shock. Crit. Care Med. 47, e394–e402. doi: 10.1097/CCM.0000000000003658
Grumaz, S., Stevens, P., Grumaz, C., Decker, S. O., Weigand, M. A., Hofer, S., et al. (2016). Next-generation sequencing diagnostics of bacteremia in septic patients. Genome Med. 8, 73. doi: 10.1186/s13073-016-0326-8
Gu, W., Miller, S., Chiu, C. Y. (2019). Clinical metagenomic next-generation sequencing for pathogen detection. Annu. Rev. Pathol. 14, 319–338. doi: 10.1146/annurev-pathmechdis-012418-012751
Honar, N., Nezamabadipour, N., Dehghani, S. M., Haghighat, M., Imanieh, M. H., Ataollahi, M., et al. (2022). An evaluation of ascitic calprotectin for diagnosis of ascitic fluid infection in children with cirrhosis. BMC Pediatr. 22, 382. doi: 10.1186/s12887-022-03433-9
Ikeda, S., Uchiyama, K., Minegishi, Y., Nakamura, M., Takaso, M. (2020). Evaluation of myeloperoxidase in synovial fluid as a biomarker for chronic periprosthetic joint infection. Int. Orthop 44, 1915–1920. doi: 10.1007/s00264-020-04753-0
Jubel, J. M., Randau, T. M., Becker-Gotot, J., Scheidt, S., Wimmer, M. D., Kohlhof, H. (2021). sCD28, sCD80, sCTLA-4, and sBTLA are promising markers in diagnostic and therapeutic approaches for aseptic loosening and periprosthetic joint infection. Front. Immunol. 12, 687065. doi: 10.3389/fimmu.2021.687065
Karbysheva, S., Yermak, K., Grigoricheva, L., Renz, N., Perka, C., Trampuz, A. (2020). Synovial fluid d-Lactate-A novel pathogen-specific biomarker for the diagnosis of periprosthetic joint infection. J. Arthroplasty 35, 2223–2229.e2. doi: 10.1016/j.arth.2020.03.016
Kasparek, M. F., Kasparek, M., Boettner, F., Faschingbauer, M., Hahne, J., Dominkus, M. (2016). Intraoperative diagnosis of periprosthetic joint infection using a novel alpha-defensin lateral flow assay. J. Arthroplasty 31, 2871–2874. doi: 10.1016/j.arth.2016.05.033
Klug, A., Gramlich, Y., Rudert, M., Drees, P., Hoffmann, R., Weißenberger, M., et al. (2021). The projected volume of primary and revision total knee arthroplasty will place an immense burden on future health care systems over the next 30 years. Knee Surg. Sports Traumatol Arthrosc 29, 3287–3298. doi: 10.1007/s00167-020-06154-7
Kurtz, S. M., Higgs, G. B., Lau, E., Iorio, R. R., Courtney, P. M., Parvizi, J. (2022). Hospital costs for unsuccessful two-stage revisions for periprosthetic joint infection. J. Arthroplasty 37, 205–212. doi: 10.1016/j.arth.2021.10.018
Kurtz, S. M., Lau, E. C., Son, M.-S., Chang, E. T., Zimmerli, W., Parvizi, J. (2018). Are we winning or losing the battle with periprosthetic joint infection: trends in periprosthetic joint infection and mortality risk for the Medicare population. J. Arthroplasty 33, 3238–3245. doi: 10.1016/j.arth.2018.05.042
Li, H., Xu, C., Hao, L., Chai, W., Jun, F., Chen, J. (2021). The concordance between preoperative aspiration and intraoperative synovial fluid culture results: intraoperative synovial fluid re-cultures are necessary whether the preoperative aspiration culture is positive or not. BMC Infect. Dis. 21, 1018. doi: 10.1186/s12879-021-06721-4
Lisowska-Myjak, B., Żytyńska-Daniluk, J., Skarżyńska, E. (2016). Concentrations of neutrophil-derived proteins in meconium and their correlations. biomark. Med. 10, 819–829. doi: 10.2217/bmm-2016-0034
Malhotra, R., Morgan, D. (2004). Role of core biopsy in diagnosing infection before revision hip arthroplasty. J. Arthroplasty 19, 78–87. doi: 10.1016/S0883-5403(03)00453-4
McNally, M., Sousa, R., Wouthuyzen-Bakker, M., Chen, A. F., Soriano, A., Vogely, H. C., et al. (2021). The EBJIS definition of periprosthetic joint infection. Bone Joint J. 103-B, 18–25. doi: 10.1302/0301-620X.103B1.BJJ-2020-1381.R1
Moojen, D. J. F., Zwiers, J. H., Scholtes, V. A. B., Verheyen, C. C. P. M., Poolman, R. W. (2014). Similar success rates for single and multiple debridement surgery for acute hip arthroplasty infection. Acta Orthop 85, 383–388. doi: 10.3109/17453674.2014.927729
Omar, M., Windhagen, H., Krettek, C., Ettinger, M. (2021). Noninvasive diagnostic of periprosthetic joint infection by urinary peptide markers: a preliminary study. J. Orthop Res. 39, 339–347. doi: 10.1002/jor.24913
Osmon, D. R., Berbari, E. F., Berendt, A. R., Lew, D., Zimmerli, W., Steckelberg, J. M., et al. (2013). Diagnosis and management of prosthetic joint infection: clinical practice guidelines by the infectious diseases society of America. Clin. Infect. Dis. 56, e1–e25. doi: 10.1093/cid/cis803
Page, M. J., McKenzie, J. E., Bossuyt, P. M., Boutron, I., Hoffmann, T. C., Mulrow, C. D., et al. (2021). The PRISMA 2020 statement: an updated guideline for reporting systematic reviews. Syst. Rev. 10, 89. doi: 10.1186/s13643-021-01626-4
Parvizi, J., Gehrke, T. (2014). Definition of periprosthetic joint infection. J. Arthroplasty 29, 1331. doi: 10.1016/j.arth.2014.03.009
Parvizi, J., Tan, T. L., Goswami, K., Higuera, C., Della Valle, C., Chen, A. F., et al. (2018). The 2018 definition of periprosthetic hip and knee infection: an evidence-based and validated criteria. J. Arthroplasty 33, 1309–1314.e2. doi: 10.1016/j.arth.2018.02.078
Parvizi, J., Zmistowski, B., Berbari, E. F., Bauer, T. W., Springer, B. D., Della Valle, C. J., et al. (2011). New definition for periprosthetic joint infection: from the workgroup of the musculoskeletal infection society. Clin. Orthop Relat. Res. 469, 2992–2994. doi: 10.1007/s11999-011-2102-9
Peel, T. N., Dowsey, M. M., Buising, K. L., Liew, D., Choong, P. F.M. (2013). Cost analysis of debridement and retention for management of prosthetic joint infection. Clin. Microbiol. Infect. 19, 181–186. doi: 10.1111/j.1469-0691.2011.03758.x
Renz, N., Yermak, K., Perka, C., Trampuz, A. (2018). Alpha defensin lateral flow test for diagnosis of periprosthetic joint infection: not a screening but a confirmatory test. J. Bone Joint Surg. Am. 100, 742–750. doi: 10.2106/JBJS.17.01005
Rodriguez-Merchan, E. C. (2018). Preoperative aspiration culture (PAC) for the diagnosis of infection in a prosthetic knee joint. Arch. Bone Jt Surg. 6, 342–345.
Rupp, M., Lau, E., Kurtz, S. M., Alt, V. (2020). Projections of primary TKA and THA in Germany from 2016 through 2040. Clin. Orthop Relat. Res. 478, 1622–1633. doi: 10.1097/CORR.0000000000001214
Rüwald, J. M., Randau, T. M., Hilgers, C., Masson, W., Irsen, S., Eymael, R. L., et al. (2020). Extracellular vesicle isolation and characterization from periprosthetic joint synovial fluid in revision total joint arthroplasty. J. Clin. Med. 9 (2), 516. doi: 10.3390/jcm9020516
Saleh, K. J., Clark, C. R., Sharkey, P. F., Goldberg, V. M., Rand, J. A., Brown, G. A., et al. (2003). Modes of failure and preoperative evaluation. J. Bone Joint Surg. Am. 85-A Suppl 1, S21–S25. doi: 10.2106/00004623-200300001-00006
Sallai, I., Marton, N., Szatmári, A., Kittel, Á., Nagy, G., Buzás, E. I., et al. (2022). Activated polymorphonuclear derived extracellular vesicles are potential biomarkers of periprosthetic joint infection. PloS One 17, e0268076. doi: 10.1371/journal.pone.0268076
Schäfer, P., Fink, B., Sandow, D., Margull, A., Berger, I., Frommelt, L., et al. (2008). Prolonged bacterial culture to identify late periprosthetic joint infection: a promising strategy. Clin. Infect. Dis. 47, 1403–1409. doi: 10.1086/592973
Sigmund, I. K., Luger, M., Windhager, R., McNally, M. A. (2022). Diagnosing periprosthetic joint infections : a comparison of infection definitions: EBJIS 2021, ICM 2018, and IDSA 2013. Bone Joint Res. 11, 608–618. doi: 10.1302/2046-3758.119.BJR-2022-0078.R1
Sigmund, I. K., Puchner, S. E., Windhager, R. (2021). Serum inflammatory biomarkers in the diagnosis of periprosthetic joint infections. Biomedicines 9, 1128. doi: 10.3390/biomedicines9091128
Stríz, I., Trebichavský, I. (2004). Calprotectin - a pleiotropic molecule in acute and chronic inflammation. Physiol. Res. 53, 245–253. doi: 10.33549/physiolres.930448
Vergara, A., Fernández-Pittol, M. J., Muñoz-Mahamud, E., Morata, L., Bosch, J., Vila, J., et al. (2019). Evaluation of lipocalin-2 as a biomarker of periprosthetic joint infection. J. Arthroplasty 34, 123–125. doi: 10.1016/j.arth.2018.09.047
Wang, C., Wang, Q., Li, R., Qin, J., Song, L., Zhang, Q., et al. (2019). LTF, PRTN3, and MNDA in synovial fluid as promising biomarkers for periprosthetic joint infection: identification by quadrupole orbital-trap mass spectrometry. J. Bone Joint Surg. Am. 101, 2226–2234. doi: 10.2106/JBJS.18.01483
Warren, J., Anis, H. K., Bowers, K., Pannu, T., Villa, J., Klika, A. K., et al. (2021). Diagnostic utility of a novel point-of-Care test of calprotectin for periprosthetic joint infection after total knee arthroplasty: a prospective cohort study. J. Bone Joint Surg. Am. 103, 1009–1015. doi: 10.2106/JBJS.20.01089
Warren, J. A., Klika, A. K., Bowers, K., Colon-Franco, J., Piuzzi, N. S., Higuera, C. A. (2022). Calprotectin lateral flow test: consistent across criteria for ruling out periprosthetic joint infection. J. Arthroplasty 37, 1153–1158. doi: 10.1016/j.arth.2022.01.082
Whiting, P. F., Rutjes, A. W. S., Westwood, M. E., Mallett, S., Deeks, J. J., Reitsma, J. B., et al. (2011). QUADAS-2: a revised tool for the quality assessment of diagnostic accuracy studies. Ann. Intern. Med. 155, 529–536. doi: 10.7326/0003-4819-155-8-201110180-00009
Wildeman, P., Rolfson, O., Söderquist, B., Wretenberg, P., Lindgren, V. (2021). What are the long-term outcomes of mortality, quality of life, and hip function after prosthetic joint infection of the hip? a 10-year follow-up from Sweden. Clin. Orthop Relat. Res. 479, 2203–2213. doi: 10.1097/CORR.0000000000001838
Williams, J. L., Norman, P., Stockley, I. (2004). The value of hip aspiration versus tissue biopsy in diagnosing infection before exchange hip arthroplasty surgery. J. Arthroplasty 19, 582–586. doi: 10.1016/j.arth.2003.11.011
Wyatt, M. C., Beswick, A. D., Kunutsor, S. K., Wilson, M. J., Whitehouse, M. R., Blom, A. W. (2016). The alpha-defensin immunoassay and leukocyte esterase colorimetric strip test for the diagnosis of periprosthetic infection: a systematic review and meta-analysis. J. Bone Joint Surg. Am. 98, 992–1000. doi: 10.2106/JBJS.15.01142
Yee, D. K. H., Chiu, K. Y., Yan, C. H., Ng, F. Y. (2013). Review article: joint aspiration for diagnosis of periprosthetic infection. J. Orthop Surg. (Hong Kong) 21, 236–240. doi: 10.1177/230949901302100225
Yermak, K., Karbysheva, S., Perka, C., Trampuz, A., Renz, N. (2019). Performance of synovial fluid d-lactate for the diagnosis of periprosthetic joint infection: a prospective observational study. J. Infect. 79, 123–129. doi: 10.1016/j.jinf.2019.05.015
Keywords: periprosthetic joint infection, PJI, diagnostic marker, diagnostics, synovial fluid
Citation: Schindler M, Walter N, Maderbacher G, Sigmund IK, Alt V and Rupp M (2023) Novel diagnostic markers for periprosthetic joint infection: a systematic review. Front. Cell. Infect. Microbiol. 13:1210345. doi: 10.3389/fcimb.2023.1210345
Received: 24 April 2023; Accepted: 23 June 2023;
Published: 17 July 2023.
Edited by:
Hongyi Shao, Beijing Jishuitan Hospital, ChinaReviewed by:
Vittorio Sambri, The Greater Romagna Hub Laboratory - DIMES Unibo, ItalyChristoph Biehl, University Medical Center Giessen, Germany
Marc Hanschen, Technical University of Munich, Germany
Copyright © 2023 Schindler, Walter, Maderbacher, Sigmund, Alt and Rupp. This is an open-access article distributed under the terms of the Creative Commons Attribution License (CC BY). The use, distribution or reproduction in other forums is permitted, provided the original author(s) and the copyright owner(s) are credited and that the original publication in this journal is cited, in accordance with accepted academic practice. No use, distribution or reproduction is permitted which does not comply with these terms.
*Correspondence: Markus Rupp, TWFya3VzLnJ1cHBAdWtyLmRl