- 1State Key Laboratory of Oral Diseases & National Clinical Research Center for Oral Diseases, West China School of Stomatology, Sichuan University, Chengdu, Sichuan, China
- 2Department of Pediatric Dentistry, West China Hospital of Stomatology, Sichuan University, Chengdu, Sichuan, China
- 3Department of Stomatology, Sichuan Provincial People’s Hospital, University of Electronic Science and Technology of China, Chengdu, China
Porphyromonas gingivalis (P. gingivalis) is a Gram-negative oral anaerobic bacterium that plays a key role in the pathogenesis of periodontitis. P. gingivalis expresses a variety of virulence factors that disrupt innate and adaptive immunity, allowing P. gingivalis to survive and multiply in the host and destroy periodontal tissue. In addition to periodontal disease, P.gingivalis is also associated with systemic diseases, of which insulin resistance is an important pathological basis. P. gingivalis causes a systemic inflammatory response, disrupts insulin signaling pathways, induces pancreatic β-cell hypofunction and reduced numbers, and causes decreased insulin sensitivity leading to insulin resistance (IR). In this paper, we systematically review the studies on the mechanism of insulin resistance induced by P. gingivalis, discuss the association between P. gingivalis and systemic diseases based on insulin resistance, and finally propose relevant therapeutic approaches. Overall, through a systematic review of the mechanisms related to systemic diseases caused by P. gingivalis through insulin resistance, we hope to provide new insights for future basic research and clinical interventions for related systemic diseases.
1 Introduction
P. gingivalis is a Gram-negative (G-) anaerobic bacterium that is an important component of the subgingival plaque biofilm and one of the red complexes that are thought to be the main pathogen causing chronic inflammation in periodontal disease (Kuboniwa et al., 2009; Coutinho Almeida-da-Silva et al., 2019; Asthana and Chhina, 2021; Lv et al., 2021). Periodontitis is a chronic inflammatory disease characterized by the pathology of the destruction of periodontal supporting tissues, including periodontal pockets, gums, and alveolar bone. As one of the most common oral diseases, periodontitis endangers the oral health of 70% of the world’s population. a survey by Eke, Paul et al. confirmed the high prevalence of periodontitis in adults over 30 years of age in the United States, with almost 50% of the population affected (Sutton et al., 2017). If periodontitis is left untreated, pockets can form between the gums and teeth, and the spread of inflammation can cause other complications. Periodontitis is a risk factor for diseases such as diabetes, atherosclerosis, Alzheimer’s disease, aspiration pneumonia and rheumatoid arthritis, and tumors (Buduneli et al., 2014; Chacon Arboleda et al., 2021).
Pseudomonas gingivalis has multiple virulence factors that may be associated with the severity of disease following mixed infections. P. gingivalis has multiple virulence factors, including envelope proteins, gingival proteases, bacterial hairs, hemagglutinin, lipopolysaccharide (LPS), hemolysin, iron uptake transport proteins, toxic outer membrane vesicles/vesicles, and DNA (Brunner et al., 2010; Singh et al., 2011; Gao et al., 2012; Ikai et al., 2015; Bozkurt et al., 2017; Nagano et al., 2017; Bregaint et al., 2022; Kim et al., 2022; Swarup et al., 2022). These virulence factors disrupt innate and adaptive immunity, allowing Porphyromonas gingivalis to survive and multiply in the host, causing an inflammatory response, inducing insulin resistance, and promoting the development of systemic disease (Zhao et al., 2007). Current research evidence suggests that elevated circulating inflammatory factors induce insulin resistance and that P. gingivalis is associated with systemic disease, of which insulin resistance has been considered to be one of the important pathological mechanisms. Insulin is an important endocrine hormone, mainly produced by pancreatic beta cells, that regulates blood glucose levels throughout the body (Kheirollahzadeh et al., 2022). The main signaling pathways through which insulin acts include insulin receptors, insulin receptor substrates, PI3K/Akt/mTOR and glucose transporter proteins (Liang et al., 2013; Petersen and Shulman, 2018). Insulin resistance (IR) is a pathophysiological phenomenon that refers to the inability of the body’s cells, tissues or body to respond adequately to normal levels of insulin and plays an important role in the development of metabolic syndrome (MS), cardiovascular disease (CVD), non-alcoholic fatty liver disease (NAFLD), and Alzheimer’s disease (AD) (Morris et al., 2014; Sasaki et al., 2020; Oh et al., 2021; PrayGod et al., 2021; Martin-Gonzalez et al., 2022).
Insulin resistance may develop through both genetic and acquired factors (Garbossa and Folli, 2017). Common genetic defects include mutations and polymorphisms in insulin receptors, glucose transporters, and signaling proteins involved in insulin signaling. Two of the 17 causes of acquired insulin resistance include obesity, lack of exercise, advanced glycosylation end products (AGE), excess free fatty acids (FFAs), psychological stress, smoking, alcohol intake, or certain drugs (Vlassara and Striker, 2011; Goodpaster and Sparks, 2017). All these factors are associated with a persistent state of low-grade inflammation. Multiple molecular and pathophysiological mechanisms are involved in insulin resistance (Khodabandehloo et al., 2016). Insulin resistance is the result of a combination of metabolic disorders, lipotoxicity, glucotoxicity and inflammation, as shown in Figure 1 (Khalid et al., 2021).
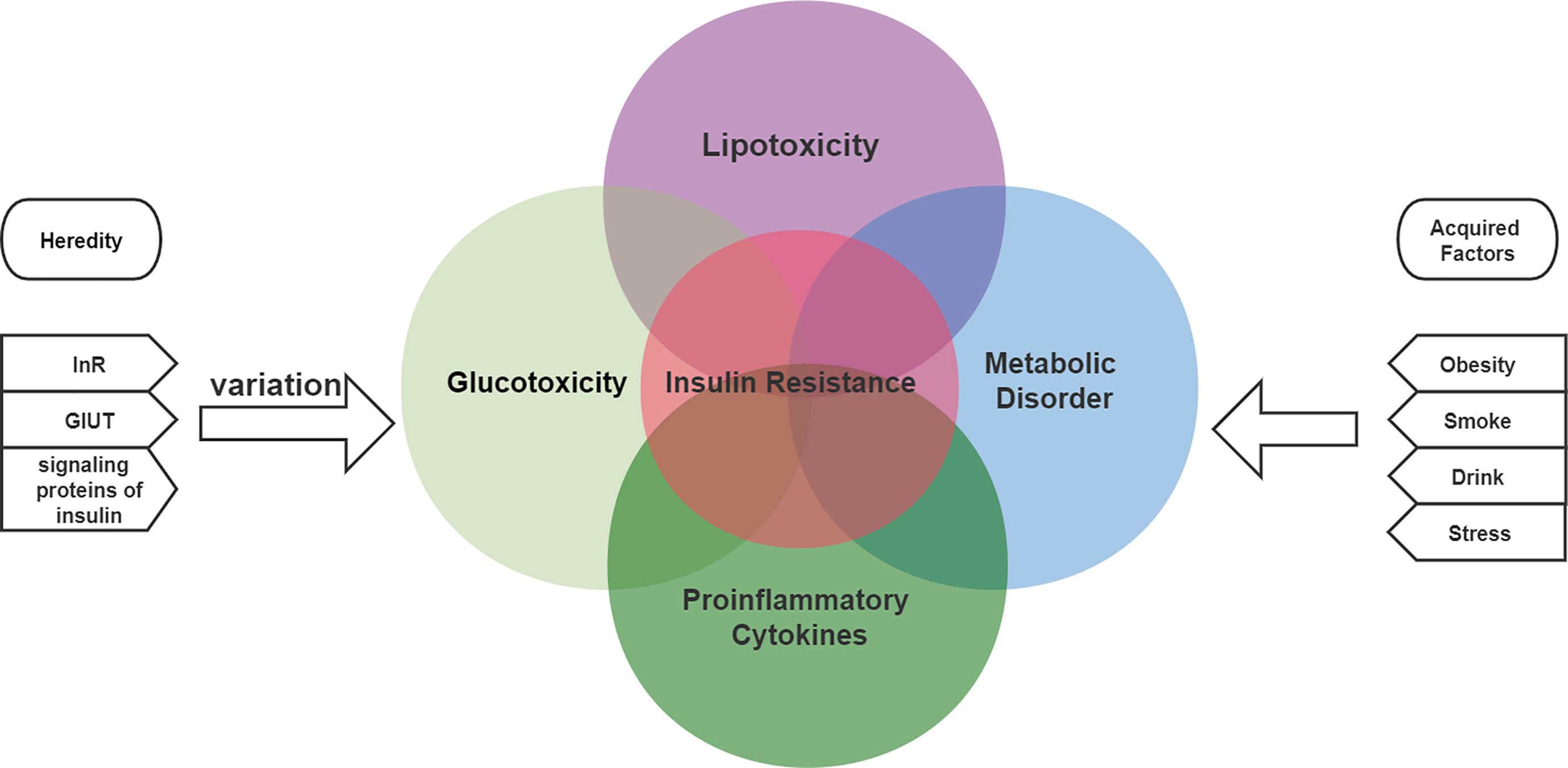
Figure 1 The etiology of insulin resistance includes genetic and acquired factors and is the result of a combination of metabolic disorders, lipotoxicity, glucotoxicity and inflammation.
This review inventories the pathogenicity of Pseudomonas gingivalis and insulin resistance by collecting the most recent studies on Pseudomonas gingivalis and insulin resistance and discusses the pathological mechanisms associated with insulin resistance. The impact of Pseudomonas gingivalis infection in different systems based on insulin resistance is explored, especially the association with diabetes, non-alcoholic fatty liver disease, Alzheimer’s disease, cardiovascular, and skeletal muscle.
Using the Web of science, PubMed, and Google Scholar databases, original research, case series, and review articles published in English through December 2022 were searched for the terms “Porphyromonas gingivalis”, “insulin resistance”, and potential related diseases or systemic disorders (i.e., “atherosclerosis,” “diabetes,” “cardiovascular disease,” “neurological disease “, “non-alcoholic fatty liver disease”, “skeletal muscle”). In addition, the reference lists of relevant articles were screened to reduce the risk of missing relevant information and to guide the search for potential associations. The search terms “oral bacteria” and “periodontitis” were also used as substitutes for “Porphyromonas gingivalis”, however, only articles that explicitly mentioned this bacterium and reported its potential activity in the organ of interest were retained. However, only articles that explicitly mention this bacterium and report its potential activity in the organ of interest were retained. The main points of interest are: (1) the pathological mechanism of insulin resistance caused by P. gingivalis; (2) the association of P.g with systemic diseases based on insulin resistance; and (3) treatment.
2 Pathogenesis of insulin resistance caused by P. gingivalis
2.1 Tissue colonization
P. gingivalis invades and survives in host tissues by disrupting the gingival epithelial barrier, internalizing into epithelial cells, and enhancing epithelial cell autophagy. Bacteremia may occur after oral surgery and can also lead to systemic transmission of P. gingivalis. Disruption of the complement system, degradation of antimicrobial peptides, and disruption of phagocytic function all contribute to the escape of P. gingivalis. P. gingivalis also suppresses adaptive immunity, allowing P. gingivalis to be present in host tissues and persistently cause inflammatory responses. Transit of oral microorganisms to distant organs is thought to play an important role in disease development. Many clinical reports have shown that P. gingivalis is the main pathogen of periodontal disease and is often detected in distal organs such as the liver, cardiovascular tissue, cerebrospinal fluid, and tubal-ovarian sites(Iida et al., 2004; Park et al., 2011; Tomas et al., 2012; Yoneda et al., 2012; Ishikawa et al., 2013; Eberhard, 2022).For example, these virulence factors are involved in the pathogenicity of bacteria in infected tissues and make possible their transmission. In vitro and clinical studies have shown that Pseudomonas gingivalis infiltrates and survives in non-oral human cells (e.g. coronary endothelial cells and placental cells) and may contribute to the local inflammatory response(Champaiboon et al., 2014; Weishan et al., 2016). Pseudomonas gingivalis can be detected in many cardiovascular diseases, such as atherosclerosis, myocardial infarction, stroke, aneurysm, pericarditis and pericardial tamponade(Pussinen et al., 2007; Patrakka et al., 2019). P. gingivalis DNA was detected in synovial tissue from RA patients, suggesting that the bacterium may be localized intracellularly (Moen et al., 2006; Martinez-Martinez et al., 2009; Ogrendik, 2012; Reichert et al., 2013; Totaro et al., 2013). Carrion, J et al. found that dendritic cells in the blood carry P. gingivalis in patients with acute coronary syndrome with chronic periodontitis and can transmit P. gingivalis from the oral mucosa to atherosclerotic plaques (Carrion et al., 2012). Furusho, H et al. performed P. gingivalis immunohistochemistry in liver biopsy specimens from patients with NASH and found the presence of P. gingivalis in the livers of patients with advanced fibrosis NASH(Furusho et al., 2013).
2.2 Decreased number and dysfunction of pancreatic islet beta cells
P. gingivalis disrupts the local and systemic immune system, causing a systemic inflammatory response that leads to insulin resistance in peripheral insulin-responsive tissues. there is a positive feedback regulatory mechanism between β-cells and insulin-sensitive tissues, where β-cells respond to the demands of liver, skeletal muscle and adipose tissue to increase insulin supply, and over-stimulation of β-cell insulin secretion leads to increased β-cell signaling and oxidative stress, which The resulting hyperinsulinemia leads to β-cell dysfunction and eventually β-cell apoptosis. In the insulin-resistant state, β-cell depletion to maintain normal blood glucose and compensate for insulin requirements is the key to the pathogenesis.
P. gingivalis disrupts the local and systemic immune system, causing a systemic inflammatory response that leads to insulin resistance in peripheral insulin-responsive tissues. there is a positive feedback regulatory mechanism between β-cells and insulin-sensitive tissues, where β-cells respond to the demands of liver, skeletal muscle and adipose tissue to increase insulin supply, and over-stimulation of β-cell insulin secretion leads to increased β-cell signaling and oxidative stress, which the resulting hyperinsulinemia leads to β-cell dysfunction and eventually β-cell apoptosis (Cerf, 2013; Wang et al., 2013; Mezza et al., 2019; Sharma et al., 2022). In the insulin-resistant state, β-cell depletion to maintain normal blood glucose and compensate for insulin requirements is the key to the pathogenesis (Ma et al., 2007).
In addition, P. gingivalis also migrates to the pancreas, causing inflammation and apoptosis of β-cells. ge Q-M et al. demonstrated that LPS increased the expression of Toll4 receptors on the membrane surface of pancreatic β-cells (Ge et al., 2011). Liza L. Ramenzoni et al. found that P.g can induce β-cell inflammation by activating the P13K/AKT signaling pathway through TLR4 to induce pro-inflammatory molecules, while stimulating insulin secretion and increasing β-cell compensatory responses(Ramenzoni et al., 2019). Ilievski, Vladimir et al. found that P. gingivalis metastasizes to the pancreas and that beta-cell apoptosis increases, leading to complex changes in islet morphology. serpinE1 appears to be involved in this process (Zhou et al., 2009).
Thus, the production of inflammatory factors, oxidative stress and the activation of signaling cascades associated with cytotoxicity lead to β-cell damage and degradation (Kouidrat et al., 2015; Abedini et al., 2018; Yaribeygi et al., 2019). As the number of beta cells decreases, the condition is further aggravated by the increased insulin requirements of the pancreas to overcome the body’s insulin-resistant state.
2.3 Inflammatory reaction
Studies have shown that continuous infusion of Pg can induce chronic systemic inflammation. P.g-LPS can be released in local and systemic organs, leading to endotoxemia, triggering regional and systemic inflammation, and promoting insulin resistance (Chiu and Lin, 2008; Baker et al., 2011; Huang et al., 2016).
The inflammatory mechanism by which P. gingivalis induces insulin resistance involves the activation of toll-like receptor (TLR) signaling pathway. The innate immune receptors TLR2 and TLR4 are pattern recognition receptors that are expressed in a variety of cell types, including macrophages, hepatocytes, and pancreatic beta cells (Hoshino et al., 1999; Jiang et al., 2000; Iwasaki and Medzhitov, 2004; Genco et al., 2005; Hemmi and Akira, 2005). Toll-like receptors play an important role in the mechanism of inflammation (Nishihara et al., 2009; Blasco-Baque et al., 2017). P. gingivalis LPS activates toll-like receptor (TLR)-related signaling pathways, which in turn trigger the release of cytokines and chemokines (Despres et al., 2008; Lee and Seong, 2009; Anhe et al., 2015; Le Sage et al., 2017). A previous study also reported that P.g-LPS could promote the production of interleukins and tumor necrosis factors (TNFs) by activating tlr4-related signaling pathways (Fujimoto et al., 2013). Lappin, DF et al. found elevated levels of Toll-like receptor 2 and 4 stimulators in saliva of patients with periodontitis compared to healthy subjects (Lappin et al., 2011). Watanabe, K et al. demonstrated a protective effect of TLR4 mutations on alveolar bone loss and improved glucose homeostasis in mice with periodontitis fed a high-fat diet (Watanabe et al., 2011).
The binding of LPS to TLR4 triggers a signaling cascade that leads to the activation of various pro-inflammatory pathways, such as the nuclear factor kappa B (NF-κB) pathway and the mitogen-activated protein kinase (MAPK) pathway. These pathways can induce the production of pro-inflammatory cytokines, such as tumor necrosis factor-a (TNF-a), interleukin-6,leptin, lipocalin, resistin, and acute phase proteins (Fernandez-Real and Ricart, 1999; Gonzalez et al., 2001; Evans et al., 2002). Advanced glycosylation end products (AGEs) are increased in diabetes and may also lead to inflammation and infection. Several molecules have been shown to induce insulin resistance, such as IL-6, TNF-a, resistin and free fatty acids (Rojo-Botello et al., 2012; Papale et al., 2018; Cattaneo et al., 2019; Lin et al., 2020; Wang et al., 2022b). IL-6 was one of the first cytokines to be considered a predictor or pathological marker of insulin resistance and cardiovascular disease (Mendiola et al., 2017). TNF-α has been shown to promote insulin resistance in different ways, such as TNF-α may increase insulin resistance by inhibiting the entry of glucose into smooth muscle cells (Savage et al., 2007; Rowan et al., 2018; Wang et al., 2022a). There is evidence that anti-tnf antibody treatment improves hepatic IR (Hu et al., 2022; Sinha and Haque, 2022). Resistin, an adipocyte-derived signaling polypeptide thought to have increased expression in inflammatory diseases and diabetes, named for its function in resisting insulin,is also a pro-inflammatory molecule (Hiroshima et al., 2012; Hrishi et al., 2016; Govindaraj et al., 2021). Palmitate, a nutritional free fatty acid (FFA) that activates TLR2, causes inflammation and induces insulin resistance (Hajishengallis, 2014; Ilievski et al., 2017).
Overall, the inflammatory mechanism of insulin resistance induced by P. gingivalis involves the activation of TLR signaling pathway and the production of pro-inflammatory cytokines. These findings suggest that periodontal disease may play a role in the development of insulin resistance and type 2 diabetes, and that treating periodontal disease may have beneficial effects on glycemic control in individuals with insulin resistance, as shown in Figure 2.
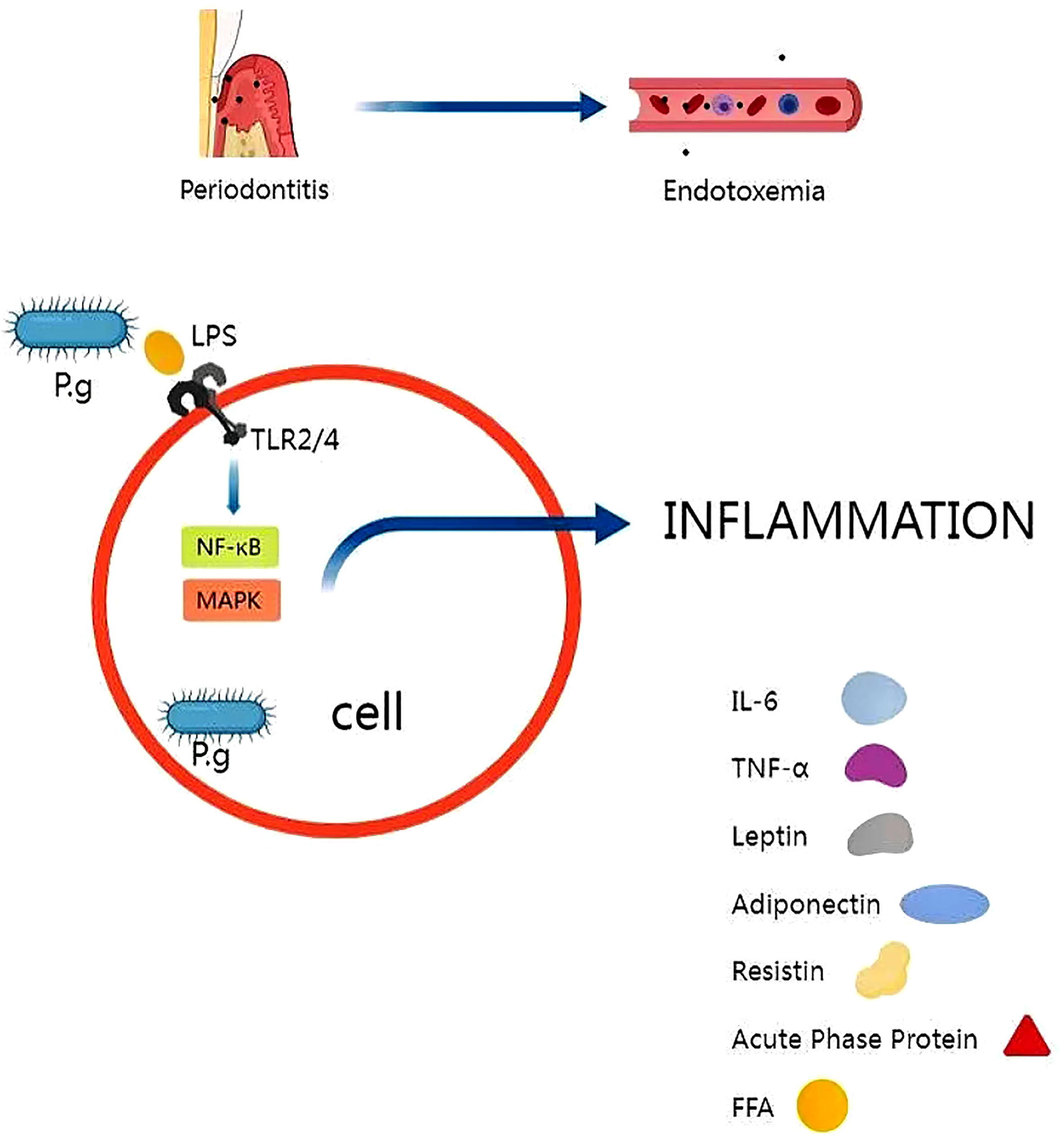
Figure 2 P.g is capable of disrupting the immune barrier, causing endotoxemia and triggering regional and systemic inflammation. The release of P.g-LPS activates toll-like receptor (TLR)-related signaling pathways, which in turn trigger the release of inflammatory factors. Several molecules have been shown to induce insulin resistance, such as IL-6, TNF-a, resistin and free fatty acids.
2.4 Immune escape
P. gingivalis can manipulate the host immune system by producing virulence factors that can interfere with immune cell signaling pathways or by inducing the production of anti-inflammatory cytokines, in order to maintain inflammation, obtain nutrients, and evade host immune killing. e, JZ et al. found that P. gingivalis was able to interfere with the immune system and thus induce insulin resistance (He et al., 2022).
The innate and adaptive immune systems are important components of the immune system. P. gingivalis interferes with the innate immune response in healthy gums:P. gingivalis disrupts the epithelial barrier, inhibits complement-mediated lysis, and achieves intracellular self-proliferation in epithelial cells, which initiates the first step in Pseudomonas gingivalis evasion of host immunity (Holmes et al., 2009). Escape of neutrophils and killing by destructive macrophages paves the way for the long-term persistence of Pseudomonas gingivalis. Then down-regulates the differentiation and development of adaptive immune cells: The ability to manipulate T-cell differentiation and immune responses provides for the survival of Pseudomonas gingivalis in the host (Soto et al., 2022). Low-grade inflammation induced by the innate immune system occurs under the control of the adaptive immune system, and if the immune system is compromised, the presence of bacteria at the site of infection may lead to systemic inflammation, thereby exacerbating insulin resistance (Harding et al., 2017; Ganz et al., 2022).
Huang, X et al. found that in obese individuals, macrophage infiltration and activation in periodontal tissue was significantly downregulated in patients with periodontitis, paralyzing the intrinsic immune response to periodontal disease(2017). Blasco-Baque et al., 2017 found that P. gingivalis infection affects antibody- P.g production, leading to an impaired specific immune system. P P. gingivalis, mediates not only local immune responses within periodontal pockets, but also systemic immune responses that enhance insulin resistance (Cao et al., 2019; Bonomo et al., 2020). He, JZ et al. found that P. gingivalis infection leading to dysbiosis of the oral microflora induced an immune response in the spleen of diabetic mice, characterized by a decrease in innate immune cells and an increase in adaptive immune cells, decreasing the levels of IL17-producing monocytes and ILC3 in the spleen, but increasing Th17 cells, which can lead to alveolar bone resorption. This is accompanied by an upregulation of the Th17/Tregs ratio and abnormalities in immune function, while an increase in TH17 cells promotes blocking inflammation and increases the risk of insulin resistance (Greggianin et al., 2023; Xiao et al., 2017).
Insulin plays a key role in the metabolism of immune cells, so insulin resistance caused by P. gingivalis infection can further damage the host’s immune function (DiAngelo et al., 2009; Hotamisligil, 2017; van Niekerk et al., 2020). Since glucose is pro-inflammatory, insulin mitigates the deleterious effects of hyperglycemia through metabolic regulation (Sun et al., 2014). In addition, studies in rodents have shown that insulin directly activates the phagocytic and bactericidal activity of immune cells and that islet deficiency can lead to an impaired immune response, which is one of the reasons why diabetic patients are susceptible to co-infections (Yano et al., 2012). Insulin regulates the metabolic reprogramming of T cells, thereby regulating adaptive immunity. In vitro, T cells lacking InsR have an attenuated response to antigen, and in vivo T cell-specific knockdown of InsR in mice results in reduced antigen-specific immunity to influenza virus infection(Tsai et al., 2018; Shinjyo and Kita, 2021).
2.5 Lipotoxicity
Studies have suggested that P. gingivalis can contribute to the development of insulin resistance, one proposed mechanism for this effect is through lipotoxicity. Insulin resistance (IR) is associated with lipotoxicity, and chronic inflammation may be a common denominator (Watanabe et al., 2011; Fleetwood et al., 2017; Ni et al., 2018; Pirih et al., 2021; Shaukat et al., 2022; Thouvenot et al., 2022; Yoshimoto et al., 2022). In mice, oral administration of P. gingivalis leads to systemic inflammation, increased adiposity and insulin resistance (Artese et al., 2015; Le Sage et al., 2017).
In hepatocytes, P. gingivalis can stimulate the production of free fatty acids(FFAs). Several studies have shown that FFAs can synergize with LPS to enhance the inflammatory response.TLR2 is an important receptor for macrophage recognition of P. gingivalis and mediates the destructive chronic inflammatory response(Nishimura et al., 2015; Marques et al., 2022). It was found that TLR2 can be activated by palmitate, a free fatty acid (FFA), leading to inflammation and induction of insulin resistance (Palmer et al., 2012; Furusho et al., 2013). lu, Z et al. found that FFAs can increase the expression of lps-triggered inflammatory molecules via CD36, leading to strong CD36-mediated inflammatory signaling and an intrinsic cellular immune response (Lu et al., 2017). In adipocytes, P. gingivalis can induce inflammation and the release of pro-inflammatory cytokines, which can also contribute to insulin resistance.
Excess free fatty acids (FFAs) in the body can form ectopic fat accumulation, and obesity or ectopic fat accumulation induces an innate immune response, followed by immune cell aggregation, which can interfere with insulin signaling and promote insulin resistance, as shown in Figure 3 (Peng et al., 2011; Shikama, 2018; Halade and Tourki, 2019; Jung et al., 2022).
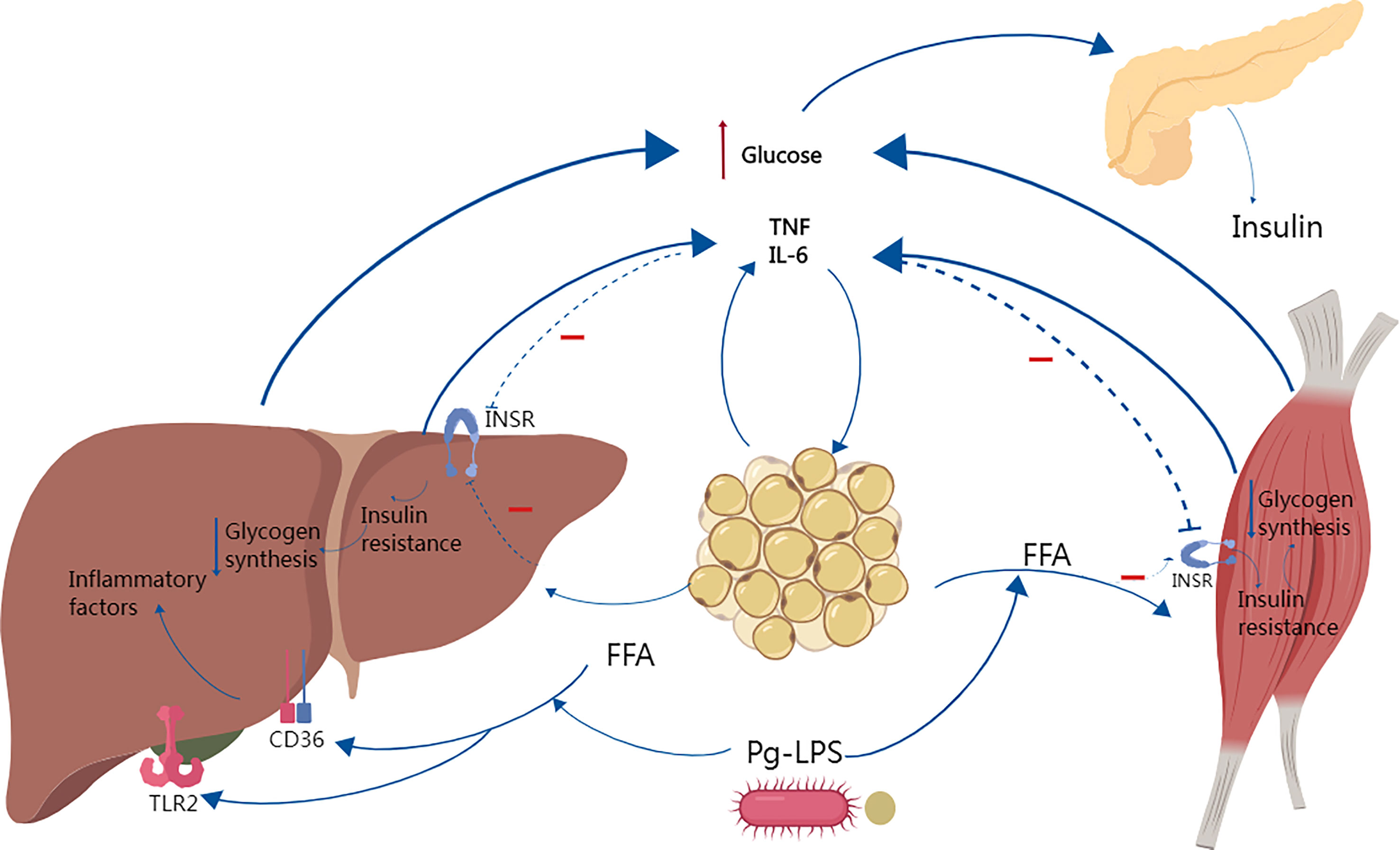
Figure 3 Studies have shown that Porphyromonas gingivalis causes systemic inflammation, increased adiposity and insulin resistance. In obese individuals, increased free fatty acid FFAs decrease insulin sensitivity by inhibiting insulin-mediated glucose uptake and reduce glycogen synthesis, and elevated circulating glucose increases insulin secretion, leading to hyperinsulinemia. Several studies have shown that FFAs can synergize with P.g-LPS to activate TLR2 and CD36, enhancing the inflammatory response and increasing the secretion of inflammatory factors such as IL-6 and TNF-α, which in turn further aggravate insulin resistance.
2.6 Dysbiosis of the gut flora
The gut microbiota is part of the host’s metabolic system and actively regulates energy balance (Wang et al., 2017). Dysbiosis of the gut flora is associated with a number of chronic inflammatory diseases such as diabetes and neurodegenerative diseases, including AD (Guo et al., 2017; Chen et al., 2021; Leblhuber et al., 2021; Rodriguez and Delzenne, 2021; Romanenko et al., 2021; Zawada et al., 2021).
There is some evidence to suggest that P. gingivalis can indirectly contribute to insulin resistance by altering the composition of the gut microbiome. Changes in the gut microbiome can lead to dysbiosis, which can promote inflammation and insulin resistance.
It was found that the microbial network of the intestinal microbiota changed significantly after P. gingivalis administration. Kashiwagi et al., 2021 found that oral administration of P. gingivalis caused changes in the intestinal flora and led to disturbances in entero-hepatic metabolism, which exacerbated hyperglycemia in an obese type 2 diabetic mouse model (Kashiwagi et al., 2021). In a 2014 study, Arimatsu, K et al. found significant changes in the intestinal microbiota of mice following oral administration of P. gingivalis a typical periodontal pathogen, with a significant increase in populations belonging to the family Bacillariophyceae, consistent with an increase in insulin resistance and systemic inflammation. The findings suggest that P. gingivalis can induce insulin resistance by altering the intestinal flora (Arimatsu et al., 2014a).
In obese individuals, P. gingivalis periodontal pathogens or E. coli LPS increase the production of pro-inflammatory factors,promote the downregulation of reactive oxygen species (ROS) and antioxidant defense systems, lead to oxidative stress, and trigger insulin resistance (de Faria and Fortunato, 2020; Zhou et al., 2021). In addition, the native flora plays a key role in host defense against infection by stimulating mucosal immune defenses (e.g., antimicrobial peptide and IgA release) and limiting invading microbes (Astapova and Leff, 2012; Lv et al., 2019). Thus, dysbiosis is associated with susceptibility to infection, which may further accelerate immunometabolic imbalance (Lazar et al., 2018).
3 Association of P. gingivalis with systemic diseases based on insulin resistance
3.1 Diabetes
Type 2 diabetes is a metabolic disease that is a major public health problem worldwide. The bidirectional association between periodontitis and diabetes has been widely accepted (Lalla and Papapanou, 2011).Results of cross-sectional studies have shown that patients with periodontitis have a higher incidence of insulin resistance (IR) (Bhat and Watanabe, 2015). Insulin resistance is an important cause of type 2 diabetes mellitus (T2DM) (Tian et al., 2020). P. gingivalis is the main causative agent of periodontitis, and several studies have shown that P. gingivalis can cause insulin resistance, which can lead to diabetes.
Ilievski, V et al. found in a 2020 study that oral administration of the periodontal pathogen P. gingivalis in mice led to insulin resistance, hyperinsulinemia and glucose intolerance. Sasaki, N et al. showed that in mice fed a high-fat diet, intravenous P. gingivalis led to impaired glucose tolerance, insulin resistance and hepatic steatosis (Sasaki et al., 2018). Ilievski, Vladimir et al. found that oral administration of P. gingivalis induced prediabetes (Ilievski et al., 2016). Seyama et al., 2020 study found that outer membrane vesicles released by P. gingivalis attenuated Akt/glycogen synthase kinase-3β (GSK-3β) signaling in hepatic HepG2 cells, inducing insulin resistance and allowing insulin-induced reduction in hepatic glycogen synthesis, thereby maintaining high blood glucose levels(Seyama et al., 2020). Bhat et al., 2014 demonstrated that P.g-LPS stimulates insulin secretion from the pancreatic β-cell lineage MIN cells.P.g-LPS may be important in the development of b-cell compensation and insulin resistance in patients with periodontitis in prediabetes (Bhat et al., 2014).Tian, J et al. an animal study showed that P. gingivalis periodontal infection significantly upregulated plasma branched-chain amino acid levels and exacerbated The plasma branched-chain amino acid biosynthetic pathway may provide a potential target for the link between periodontitis and T2DM (Kang et al., 2022).
Individuals with type 2 diabetes and periodontitis are severely impaired by the ability of one disease to exacerbate the other, and disease-associated inflammation is thought to be one mechanism that fuels this pathogenic cycle (Zhu et al., 2014). P. gingivalis can induce a sustained elevation of inflammatory factors associated with type 2 diabetes. Bacterial endotoxin/lipopolysaccharide (LPS) from infected periodontal sites readily invades the circulatory system and induces endotoxemia (Bhat et al., 2014). Thus, bacterial endotoxins may mediate inflammatory responses in distant organs, leading to increased levels of systemic inflammatory mediators, which exacerbate insulin resistance and promote the development of diabetes, as shown in Figure 4 (Ramenzoni et al., 2019).
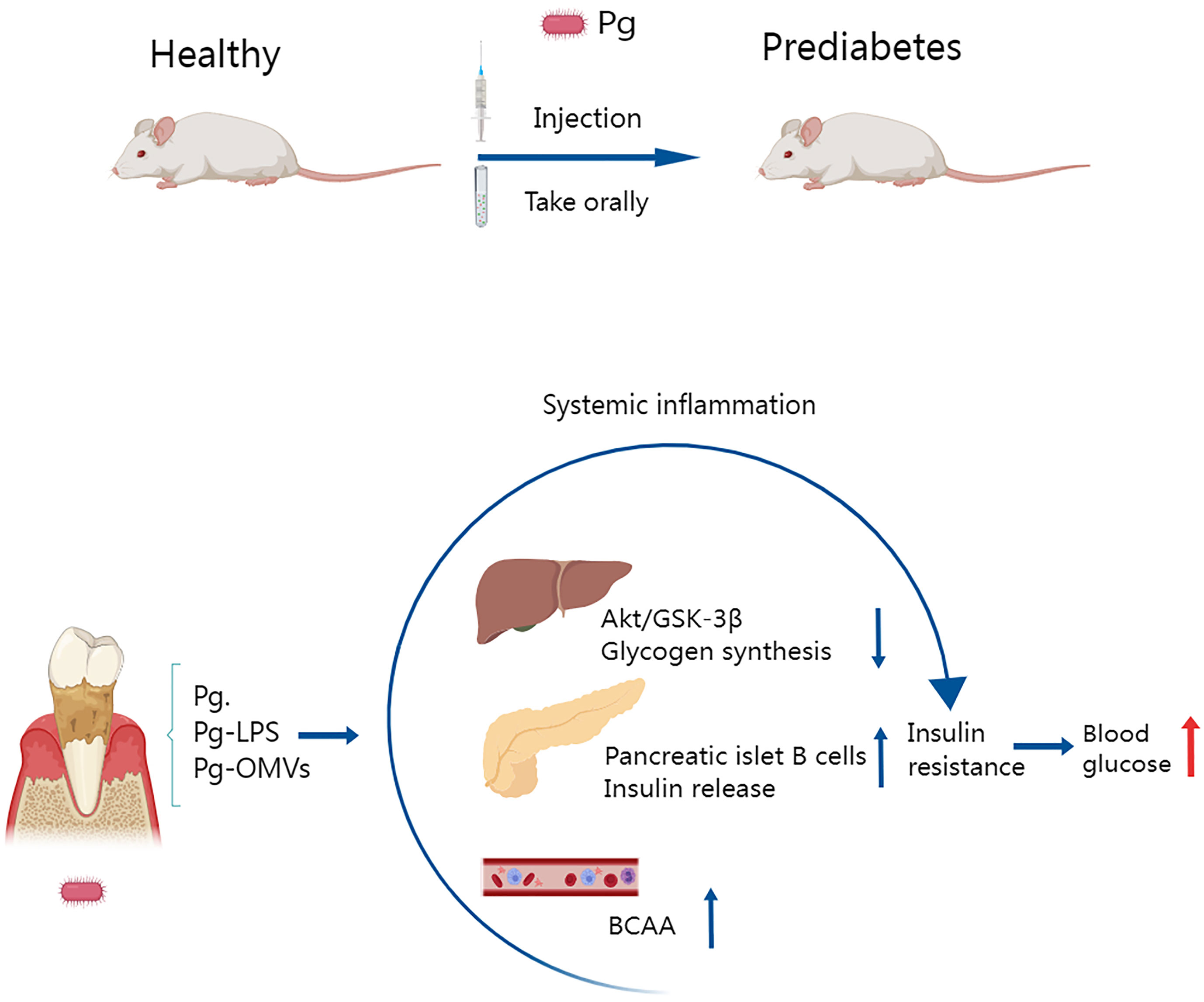
Figure 4 Several studies have shown that oral administration or injection of Porphyromonas gingivalis in mice leads to prediabetes and that insulin resistance is an important pathological mechanism involved. Porphyromonas gingivalis and the vesicles and LPS it releases disrupt hepatic glycogen synthase, cause pancreatic β-cell compensation, and elevate plasma branched-chain amino acids, which lead to decreased insulin function and reduced synthesis, inducing insulin resistance and leading to elevated blood glucose.
3.2 Non-alcoholic fatty liver disease
Non-alcoholic fatty liver disease (NAFLD) is a metabolic stress liver injury disease closely related to insulin resistance and genetic susceptibility. It is characterized by excessive invasion of triglycerides in hepatocytes independent of alcohol intake. Multiple risk factors are thought to contribute to the pathogenesis of this disease, including metabolic disorders, immune response(Thalla et al., 2022). Periodontitis is considered a risk factor for metabolic disorders, and periodontal disease bacteria can also exacerbate NAFLD (Nagao et al., 2021). Porphyromonas gingivalis-induced endotoxemia exacerbates NAFLD, increases insulin resistance, and inhibits glucose metabolism (Ezhilarasan, 2021).Studies in mice have shown that P. gingivalis can be localized in the autophagosomes and lysosomes of HepG2 cells (Souccar et al., 2010). Furusho, H et al. performed P. gingivalis immunohistochemistry in liver biopsy specimens from NAFLD patients and detected P. gingivalis in Kupffer cells and hepatocytes and found that P. gingivalis infection may upregulate the P.g-LPS-TLR2 pathway and activation of the inflammasome play an important role in the progression of NAFLD. P.g-LPS may also induce activation of the NF-κB signaling pathway, which plays a crucial role in inflammation and is associated with the development of obesity-induced insulin resistance, metabolic syndrome, and NAFLD (Nakahara et al., 2018).
Chronic low-grade inflammation induced by P. gingivalis infection induces insulin resistance, which affects hepatic glucolipid metabolism and consequently leads to lipid accumulation in hepatocytes. In addition to this, a study by Ahn et al., 2021 found that P. gingivalis induced the progression of nonalcoholic fatty liver disease in high-fat-fed mice by upregulating the CD36-PPARγ axis (Ahn et al., 2021). As one of the important metabolic enzymes in fatty acids, fatty acid translocase 36 (CD36) is widely expressed in various cells (myocytes, monocytes, macrophages, hepatocytes) (Thanakun et al., 2014). It not only mediates the uptake and transport of fatty acids directly, but also recognizes many endogenous metabolites of inflammation(Miquilena-Colina et al., 2011). Chronic low-grade inflammation caused by P. gingivalis has been reported to upregulate CD36 expression in hepatocytes and increase hepatic fat accumulation, which in turn leads to nonalcoholic fatty liver disease(Ni et al., 2018).
Recently, a growing body of evidence supports the association between NAFLD and dysbiosis of the oral and gut microbiota(Arimatsu et al., 2014a). Oral administration or injection of Porphyromonas gingivalis to mice has been reported to induce dysbiosis of the intestinal flora and promote the development of NAFLD with insulin resistance and systemic chronic inflammation(Bajaj et al., 2017). A significant increase in the abundance of Allobaculum spp. was found in mice receiving Porphyromonas gingivalis, which coincided with an increase in insulin resistance and systemic inflammation (Lee et al., 2015). However, Lactobacillus reuteri was reduced, which decreased insulin sensitivity (Morello et al., 2018).
3.3 Alzheimer’s disease
Alzheimer’s disease (AD), the most ordinary form of dementia, is a neurodegenerative disease characterized by cognitive decline due to the accumulation of β-amyloid (a β) plaques and neurofibrillary tangles in the brain.
Results from cross-sectional and longitudinal studies suggest that periodontitis is strongly associated with cognitive impairment (CI) and Alzheimer’s disease (AD).Stein, PS et al. found that periodontal disease may promote the onset/progression of AD and that P. gingivalis serum antibody levels are increased in patients with Alzheimer’s disease (AD) (Stein et al., 2012). Duan et al. 2022a study found that periodontitis may be a risk factor for exacerbation of cognitive dysfunction in patients with AD-like neurodegeneration, possibly through impairment of insulin signaling pathways, stimulation of glial proliferation and neuroinflammation(Duan et al., 2022a).
P. gingivalis is a gram-negative bacterium found in the oral cavity that causes periodontal disease. There is evidence of a strong association between P. gingivalis infection and Alzheimer’s disease AD, and it has been found in the brains of AD patients (Dominy et al., 2019; Bahar et al., 2021). Pseudomonas gingivalis infection can trigger inflammation of the peripheral and central nervous system in affected individuals, leading to cognitive decline. In mice, oral P. gingivalis infection leads to brain colonization and ad-like pathogenesis, including complement activation and β-amyloid formation, suggesting a potential mechanistic link between periodontal disease and AD (Poole et al., 2015). A systematic review of preclinical studies also found that P. gingivalis infection induces an inflammatory response and tissue degeneration in the brain, which is associated with cognitive impairment (Fernandes Costa et al., 2021). A 2018 study by Ilievski, V et al. found neurodegeneration and extracellular Aβ formation in young adult WT mice after repeated oral P. gingivalis administration. The neuropathological features observed in this study strongly suggest that low-grade chronic periodontal pathogen infection can lead to neuropathological development consistent with AD (Ilievski et al., 2018).
Several studies have shown that systemic infections caused by Porphyromonas gingivalis may affect the inflammatory state of the central nervous system (Fung et al., 2012). Inflammation is a major driver of insulin resistance and defective insulin signaling cascades. Dysregulation of brain IR and brain insulin signaling may play a key role in the pathogenesis of AD (Duan et al., 2022a). Bahar et al., 2021 study found neuroinflammation in the form of reactive microglia and astrocytes in P. gingivalis W83-infected db/db mice, and that key genes in the insulin signaling pathway (INSR, IGF1, IRS, IDE, PIK3R, SGK1, GYS, GSK3B, AKT1) mRNA abundance were upregulated, suggesting that P. gingivalis oral infection may exacerbate insulin resistance in the brain of db/db mice (Bahar et al., 2021). Insulin plays a key role in the regulation of immunometabolism in the central nervous system and periphery, and dysfunction of these signaling pathways has been associated with cognitive impairment.
3.4 Cardiovascular disease
Cardiovascular disease is the most common cause of death in industrialized countries. in 2014, cardiovascular disease accounted for 23.4% of deaths in the United States. Clinical and experimental findings suggest a strong link between periodontal disease and atherosclerosis (Wallet et al., 2018).
Kuroe et al., 2004 found that P. gingivalis infection was associated with atherosclerosis in non-obese Japanese patients with type 2 diabetes mellitus (Kuroe et al., 2004).Studies have shown that P. gingivalis infection accelerates atherosclerosis in hyperlipidemic animals and humans (Ikai et al., 2015; Nagaoka et al., 2017; Nakayama and Ohara, 2017; Naginyte et al., 2019). P. gingivalis and other oral bacteria are often detected in human atherosclerotic plaques and have been cultured from them(Manning-Tobin et al., 2009; Masters et al., 2010). Transient oral bacteremia occurs after activities such as routine dental treatment or daily oral hygiene (Mathur et al., 2018). This route of entry into the vascular system can serve as a direct pathway for bacteria to enter the endothelium (Mayer et al., 2017).Alternatively, oral bacteria may enter the vessel wall indirectly due to transport of intracellular bacteria by host immune cells to sites of atherosclerotic plaque formation or due to persistent inflammation in the oral cavity affecting the blood vessels. At the cellular level, P. gingivalis can invade human vascular cells, including human umbilical vein endothelial cells and coronary cells, and induce a range of cytokines and cell adhesion molecules that are characteristic of activated endothelial cells in atherosclerosis (Mehta et al., 2007; Mizoguchi et al., 2007).
P.g infection activates inflammatory pathways and increases the release of inflammatory factors, which is thought to be a novel mechanism by which periodontitis increases the risk of cardiovascular disease and insulin resistance (Lappin et al., 2011). Lappin, DF et al. found that experimental administration of TLR2 or TLR4 stimulants to mice resulted in insulin resistance as well as significantly accelerated atherosclerosis, and that deletion of the TLR2 or TLR4 genes prevented the development of these diseases. (Ioana et al., 2012).
Almudena Gómez-Hernández et al. found that severe hepatic insulin resistance is sufficient to cause cardiovascular insulin resistance and dysfunction. The different manifestations of insulin resistance, including dyslipidemia, hyperglycemia, inflammation, and obesity, may be mediators of insulin resistance co-producing endothelial dysfunction. Thus, hyperlipidemia, hyperglycemia and pro-inflammatory cytokines are known to selectively impair the PI3K/AKT/eNOS pathway, increase oxidative stress, and enhance ET-1 release via the intact or enhanced MAPK-ET1 pathway in endothelial cells. Insulin resistance states are associated with metabolic abnormalities, including glucotoxicity, lipotoxicity and inflammation, which can also lead to endothelial dysfunction(Gomez-Hernandez et al., 2021).
3.5 Skeletal muscle
Skeletal muscle is the most representative tissue of the body and is necessary for voluntary movement and body position (Baker et al., 2011). In addition, skeletal muscle plays an important role in nutritional homeostasis, thermoregulation, endocrine system regulation, energy metabolism and glucose uptake (Batsis and Villareal, 2018).About a quarter of the ingested glucose is stored in skeletal muscle as glycogen and used as a source of energy (Bolyen et al., 2019). The clinical consequences of insulin resistance and compensatory hyperinsulinemia have become a major public health problem. Myasthenia gravis is a progressive reduction in muscle mass with age. Sarcopenic obesity is characterized by an increase in body fat mass and a decrease in muscle mass (Zhu et al., 2013). The prevalence of skeletal muscle obesity is expected to increase as the elderly population increases (Zimowska et al., 2017).
Porphyromonas gingivalis infection leads to an increase in systemic inflammatory mediators, including TNF-α, and causes insulin resistance (Greggianin et al., 2023). Watanabe, K et al. found that P. gingivalis infection caused sarcopenic obesity and metabolic dysfunction associated with floundering muscle. The findings suggest that prevention and treatment of periodontal disease may contribute to the incidence of muscle-reducing obesity. Many studies have shown that periodontitis affects the diabetic state and P. gingivalis can increase insulin resistance by inhibiting glucose entry into smooth muscle cells using LPS and TNF-α (Sakalauskiene et al., 2014).
The findings suggest that P. gingivalis infection can trigger insulin resistance, a risk factor for metabolic syndrome and skeletal muscle metabolic dysfunction, one pathway of which is alterations in the gut microbiota. In a study by Watanabe et al., 2021, they found significant changes in the microbial network of the gut microbiota in mice taking P. gingivalis, compared to control flounder muscles that exhibited fat infiltration and low glucose uptake, higher TNF-α expression and lower insulin signaling, and TNF-α reduced glucose uptake in C2C12 myogenic cells in vitro,are explained in detail in Table 1. (Chandra and Shashikumarr, 2019; Watanabe et al., 2021).
4 Therapy
4.1 Periodontal treatment
Ni et al., 2018 study showed that periodontitis increases insulin resistance and that scaling and root planning improves insulin resistance (Ni et al., 2018). Laser treatment is an effective method to improve clinical and microbiological parameters in patients with diabetes mellitus with chronic pancreatitis. In addition, there is a better improvement in glycemic control. Therefore, patients with delayed wound healing, like DM2 with CP, can be effectively treated with laser as an adjunct to non-surgical periodontal treatment for better outcomes (Chandra and Shashikumarr, 2019).
4.2 Plant polyphenols
Polyphenol therapies have attracted a lot of attention in helping to counteract the harmful effects of periodontal bacteria and improve insulin resistance (Thouvenot et al., 2022). During periodontal disease, polyphenols exert antimicrobial effects and modulate the host inflammatory response. In addition, their anti-inflammatory activity may help improve adipose tissue function and insulin sensitivity during obesity (Gao et al., 2014). Notably, polyphenols are considered to be the most abundant antioxidants in fruits, vegetables and beverages of plant origin (Gurav and Jadhav, 2011; Furugen et al., 2013; Everard et al., 2014). Le Sage, F et al. demonstrated that polyphenols extracted from the medicinal plant Amaranthus longifolia (Antirhea borbonica), mentioned in the French Pharmacopoeia, were used for their antidiabetic effects and protected preadipocytes from pro-inflammatory agents (e.g. E. coli LPS) (Bedard and Krause, 2007). Plant polyphenols reduced the pro-inflammatory effect of LPS. At the same time, polyphenols increased the production of lipocalin and PPARγ, which are known to be key anti-inflammatory and insulin-sensitizing mediators, and to some extent reversed the oxidative stress effects on cells.
4.3 Immunotherapy
P.g - LPS is responsible for the metabolic damage caused by periodontitis, and it is important to reduce insulin resistance and type 2 diabetes through anti-inflammatory strategies that target the local immune system. P.g-lps-based suppressive therapies and antibiotics that directly target Porphyromonas can prevent the deleterious effects of periodontitis on glucose homeostasis in diabetic patients. In addition, vaccination against P.g reduces the effect of periodontitis on glucose metabolism. It was found that treatment with inactivated P.g prior to periodontal infection induced specific antibodies against P.g and protected mice from the metabolic impairment caused by periodontitis (Satoh and Ishihara, 2020). In addition, it was found that anti-TNF-a IgG inhibited the expression of TNF-a mRNA and IL-6 mRNA in the liver of diabetic mice after inoculation with P. gingivalis, are explained in detail in Table 2 (Nishihara et al., 2009).
5 Conclusion
P.g is the main causative agent of periodontitis, and P.g infection causes local and systemic inflammation while disrupting the immune system and further aggravating the inflammatory response, thereby inducing insulin resistance. In addition, P.g infection leads to β-cell dysfunction with reduced number, which makes insulin resistance more severe. P.g infection leads to intestinal flora disorder, which disrupts local immune defense and releases both P.g and LPS into blood. Based on insulin resistance, P.g can cause systemic diseases such as diabetes, non-alcoholic fatty liver disease, cardiovascular disease, Alzheimer’s disease, and sarcopenia. Based on this theory, the basic treatment of periodontal disease and the systemic therapy for P.g have received attention and become the hot topic nowadays. The association of Porphyromonas gingivalis with systemic diseases has been demonstrated, with insulin resistance being an important pathological mechanism, but the associated molecular mechanisms have not been elucidated and require further experimental confirmation. The effect of Porphyromonas gingivalis on skeletal muscle metabolism is a relatively weak part of the picture. In addition, the effect of Porphyromonas gingivalis on immune cells and how it further induces insulin resistance is a part that needs to be further explored by basic research.
Author contributions
SJ wrote the manuscript and prepared figures. XL and QD participated in the design, revision, and final approval of the manuscript. All authors contributed to the article and approved the submitted version.
Funding
This work was supported by the Applied Foundation in Science and Technology Office of Sichuan Province [2023NSFSC1998, to QD].
Conflict of interest
The authors declare that the research was conducted in the absence of any commercial or financial relationships that could be construed as a potential conflict of interest.
Publisher’s note
All claims expressed in this article are solely those of the authors and do not necessarily represent those of their affiliated organizations, or those of the publisher, the editors and the reviewers. Any product that may be evaluated in this article, or claim that may be made by its manufacturer, is not guaranteed or endorsed by the publisher.
References
Abedini, A., Cao, P., Plesner, A., Zhang, J., He, M., Derk, J., et al. (2018). RAGE binds preamyloid IAPP intermediates and mediates pancreatic beta cell proteotoxicity. J. Clin. Invest. 128 (2), 682–698. doi: 10.1172/jci85210
Ahn, J.-S., Yang, J. W., Oh, S.-J., Shin, Y. Y., Kang, M.-J., Park, H. R., et al. (2021). Porphyromonas gingivalis exacerbates the progression of fatty liver disease via CD36-PPAR gamma pathway. Bmb Rep. 54 (6), 323–328. doi: 10.5483/BMBRep.2021.54.6.050
Anhe, F. F., Roy, D., Pilon, G., Dudonne, S., Matamoros, S., Varin, T. V., et al. (2015). A polyphenol-rich cranberry extract protects from diet-induced obesity, insulin resistance and intestinal inflammation in association with increased akkermansia spp. population in the gut microbiota of mice. Gut 64 (6), 872–883. doi: 10.1136/gutjnl-2014-307142
Arimatsu, K., Yamada, H., Miyazawa, H., Minagawa, T., Nakajima, M., Ryder, M. I., et al. (2014a). Oral pathobiont induces systemic inflammation and metabolic changes associated with alteration of gut microbiota. Sci. Rep. 4, 4828. doi: 10.1038/srep04828
Artese, H. P. C., Foz, A. M., Rabelo, M. D., Gomes, G. H., Orlandi, M., Suvan, J., et al. (2015). Periodontal therapy and systemic inflammation in type 2 diabetes mellitus: a meta-analysis. PLoS One 10 (5), e0128344. doi: 10.1371/journal.pone.0128344
Astapova, O., Leff, T. (2012). “Adiponectin and PPAR gamma: cooperative and interdependent actions of two key regulators of metabolism,” in Adiponectin. Ed. Litwack, G. (New York: Elsevier), 143–162.
Asthana, G., Chhina, S. (2021). Interplay between periodontal disease and diabetes mellitus. Indian J. Med. Specialities 12 (3), 122–126. doi: 10.4103/injms.injms_132_20
Bahar, B., Kanagasingam, S., Tambuwala, M. M., Aljabali, A. A. A., Dillon, S. A., Doaei, S., et al. (2021). Porphyromonas gingivalis (W83) infection induces alzheimer's disease-like pathophysiology in obese and diabetic mice. J. Alzheimers Dis. 82 (3), 1259–1275. doi: 10.3233/jad-210465
Bajaj, J. S., Fagan, A., Sikaroodi, M., White, M. B., Sterling, R. K., Gilles, H., et al. (2017). Liver transplant modulates gut microbial dysbiosis and cognitive function in cirrhosis. Liver Transpl 23 (7), 907–914. doi: 10.1002/lt.24754
Baker, R. G., Hayden, M. S., Ghosh, S. (2011). NF-kappa b, inflammation, and metabolic disease. Cell Metab. 13 (1), 11–22. doi: 10.1016/j.cmet.2010.12.008
Batsis, J. A., Villareal, D. T. (2018). Sarcopenic obesity in older adults: aetiology, epidemiology and treatment strategies. Nat. Rev. Endocrinol. 14 (9), 513–537. doi: 10.1038/s41574-018-0062-9
Bedard, K., Krause, K. H. (2007). The NOX family of ROS-generating NADPH oxidases: physiology and pathophysiology. Physiol. Rev. 87 (1), 245–313. doi: 10.1152/physrev.00044.2005
Bhat, U. G., Ilievski, V., Unterman, T. G., Watanabe, K. (2014). Porphyromonas gingivalis lipopolysaccharide upregulates insulin secretion from pancreatic beta cell line MIN6. J. Periodontology 85 (11), 1629–1636. doi: 10.1902/jop.2014.140070
Bhat, U. G., Watanabe, K. (2015). Serpine1 mediates Porphyromonas gingivalis induced insulin secretion in the pancreatic beta cell line MIN6. J. Oral. Biol. (Northborough Mass.) 2 (2). doi: 10.13188/2377-987x.1000008
Blasco-Baque, V., Garidou, L., Pomie, C., Escoula, Q., Loubieres, P., Le Gall-David, S., et al. (2017). Periodontitis induced by Porphyromonas gingivalis drives periodontal microbiota dysbiosis and insulin resistance via an impaired adaptive immune response. Gut 66 (5), 872–885. doi: 10.1136/gutjnl-2015-309897
Bolyen, E., Rideout, J. R., Dillon, M. R., Bokulich, N., Abnet, C. C., Al-Ghalith, G. A., et al. (2019). Reproducible, interactive, scalable and extensible microbiome data science using QIIME 2. Nat. Biotechnol. 37 (8), 852–857. doi: 10.1038/s41587-019-0209-9
Bonomo, R. R., Cook, T. M., Gavini, C. K., White, C. R., Jones, J. R., Bovo, E., et al. (2020). Fecal transplantation and butyrate improve neuropathic pain, modify immune cell profile, and gene expression in the PNS of obese mice. Proc. Natl. Acad. Sci. U S A 117 (42), 26482–26493. doi: 10.1073/pnas.2006065117
Bozkurt, S. B., Hakki, S. S., Hakki, E. E., Durak, Y., Kantarci, A. (2017). Porphyromonas gingivalis lipopolysaccharide induces a pro-inflammatory human gingival fibroblast phenotype. Inflammation 40 (1), 144–153. doi: 10.1007/s10753-016-0463-7
Bregaint, S., Boyer, E., Fong, S. B., Meuric, V., Bonnaure-Mallet, M., Jolivet-Gougeon, A. (2022). Porphyromonas gingivalis outside the oral cavity. Odontology 110 (1), 1–19. doi: 10.1007/s10266-021-00647-8
Brunner, J., Scheres, N., El Idrissi, N. B., Deng, D. M., Laine, M. L., van Winkelhoff, A. J., et al. (2010). The capsule of Porphyromonas gingivalis reduces the immune response of human gingival fibroblasts. BMC Microbiol. 10, 1–11. doi: 10.1186/1471-2180-10-5
Buduneli, N., Biyikoglu, B., Ilgenli, T., Buduneli, E., Nalbantsoy, A., Sarac, F., et al. (2014). Is obesity a possible modifier of periodontal disease as a chronic inflammatory process? a case-control study. J. Periodontal Res. 49 (4), 465–471. doi: 10.1111/jre.12125
Cao, R. Y., Li, Q. L., Wu, Q. Q., Yao, M. F., Chen, Y., Zhou, H. B. (2019). Effect of non-surgical periodontal therapy on glycemic control of type 2 diabetes mellitus: a systematic review and Bayesian network meta-analysis. BMC Oral. Health 19 (1), 1–14. doi: 10.1186/s12903-019-0829-y
Carrion, J., Scisci, E., Miles, B., Sabino, G. J., Zeituni, A. E., Gu, Y., et al. (2012). Microbial carriage state of peripheral blood dendritic cells (DCs) in chronic periodontitis influences DC differentiation, atherogenic potential. J. Immunol. 189 (6), 3178–3187. doi: 10.4049/jimmunol.1201053
Cattaneo, C., Caramaschi, A., Uga, E., Braghin, M., Cosi, G., Peila, C., et al. (2019). Analysis of toll-like receptors in human milk: detection of membrane-bound and soluble forms. J. Immunol. Res. 2019. doi: 10.1155/2019/4078671
Cerf, M. E. (2013). Beta cell dysfunction and insulin resistance. Front. Endocrinol. 4. doi: 10.3389/fendo.2013.00037
Chacon Arboleda, P. T., Morales Velasquez, J. A., Echeverry Canas, C. E., Torres Henao, M. C., Olivares Duque, M. (2021). Periodontitis, overweight and obesity: a narrative review. Nutricion Clinica Y Dietetica Hospitalaria 41 (3), 130–140. doi: 10.12873/41chacon
Champaiboon, C., Poolgesorn, M., Wisitrasameewong, W., Sa-Ard-Iam, N., Rerkyen, P., Mahanonda, R. (2014). Differential inflammasome activation by Porphyromonas gingivalis and cholesterol crystals in human macrophages and coronary artery endothelial cells. Atherosclerosis 235 (1), 38–44. doi: 10.1016/j.atherosclerosis.2014.04.007
Chandra, S., Shashikumarr, P. (2019). Diode laser - a novel therapeutic approach in the treatment of chronic periodontitis in type 2 diabetes mellitus patients: a prospective randomized controlled clinical trial. J. Lasers Med. Sci. 10 (1), 56–63. doi: 10.15171/jlms.2019.09
Chen, Y. J., Xu, J. Y., Chen, Y. (2021). Regulation of neurotransmitters by the gut microbiota and effects on cognition in neurological disorders. Nutrients 13 (6), 2099. doi: 10.3390/nu13062099
Chiu, F.-L., Lin, J.-K. (2008). Tomatidine inhibits iNOS and COX-2 through suppression of NF-kappa b and JNK pathways in LPS-stimulated mouse macrophages. FEBS Lett. 582 (16), 2407–2412. doi: 10.1016/j.febslet.2008.05.049
Coutinho Almeida-da-Silva, C. L., Ramos-Junior, E. S., Morandini, A. C., Rocha, G. d. C., Marinho, Y., Tamura, A. S., et al. (2019). P2X7 receptor-mediated leukocyte recruitment and Porphyromonas gingivalis clearance requires IL-1 production and autocrine IL-1 beta receptor activation. Immunobiology 224 (1), 50–59. doi: 10.1016/j.imbio.2018.10.008
de Faria, C. C., Fortunato, R. S. (2020). The role of dual oxidases in physiology and cancer. Genet. Mol. Biol. 43. doi: 10.1590/1678-4685/gmb-2019-0096
Despres, J. P., Lemieux, I., Bergeron, J., Pibarot, P., Mathieu, P., Larose, E., et al. (2008). Abdominal obesity and the metabolic syndrome: contribution to global cardiometabolic risk. Arterioscler. Thromb. Vasc. Biol. 28 (6), 1039–1049. doi: 10.1161/atvbaha.107.159228
DiAngelo, J. R., Bland, M. L., Bambina, S., Cherry, S., Birnbaum, M. J. (2009). The immune response attenuates growth and nutrient storage in drosophila by reducing insulin signaling. Proc. Natl. Acad. Sci. United States America 106 (49), 20853–20858. doi: 10.1073/pnas.0906749106
Dominy, S. S., Lynch, C., Ermini, F., Benedyk, M., Marczyk, A., Konradi, A., et al. (2019). Porphyromonas gingivalis in alzheimer's disease brains: evidence for disease causation and treatment with small-molecule inhibitors. Sci. Adv. 5 (1), eaau3333. doi: 10.1126/sciadv.aau3333
Duan, L., Qian, X., Wang, Q., Huang, L., Ge, S. (2022a). Experimental periodontitis deteriorates cognitive function and impairs insulin signaling in a streptozotocin-induced alzheimer's disease rat model. J. Alzheimers Dis. 88 (1), 57–74. doi: 10.3233/jad-215720
Eberhard, J. (2022). The consistent detection of oral bacteria in atherosclerotic plaque does not qualify for dental treatment to reduce cardiovascular risk. J. Evidence-Based Dental Pract. 22 (2), 101718. doi: 10.1016/j.jebdp.2022.101718
Evans, J. L., Goldfine, I. D., Maddux, B. A., Grodsky, G. M. (2002). Oxidative stress and stress-activated signaling pathways: a unifying hypothesis of type 2 diabetes. Endocrine Rev. 23 (5), 599–622. doi: 10.1210/er.2001-0039
Everard, A., Geurts, L., Caesar, R., Van Hul, M., Matamoros, S., Duparc, T., et al. (2014). Intestinal epithelial MyD88 is a sensor switching host metabolism towards obesity according to nutritional status. Nat. Commun. 5, 5648. doi: 10.1038/ncomms6648
Ezhilarasan, D. (2021). Deciphering the toxicological role of Porphyromonas gingivalis derived endotoxins in liver diseases. Environ. Toxicol. Pharmacol. 88, 103755. doi: 10.1016/j.etap.2021.103755
Fernandes Costa, M. J., Torres de Araujo, I. D., Alves, L. D. R., da Silva, R. L., Calderon, P. D. S., Dutra Borges, B. C., et al. (2021). Relationship of Porphyromonas gingivalis and alzheimer's disease: a systematic review of pre-clinical studies. Clin. Oral. Investigations 25 (3), 797–806. doi: 10.1007/s00784-020-03764-w
Fernandez-Real, J. M., Ricart, W. (1999). Insulin resistance and inflammation in an evolutionary perspective: the contribution of cytokine genotype/phenotype to thriftiness. Diabetologia 42 (11), 1367–1374. doi: 10.1007/s001250051451
Fleetwood, A. J., Lee, M. K. S., Singleton, W., Achuthan, A., Lee, M. C., O'Brien-Simpson, N. M., et al. (2017). Metabolic remodeling, inflammasome activation, and pyroptosis in macrophages stimulated by Porphyromonas gingivalis and its outer membrane vesicles. Front. Cell. Infection Microbiol. 7. doi: 10.3389/fcimb.2017.00351
Fujimoto, Y., Shimoyama, A., Saeki, A., Kitayama, N., Kasamatsu, C., Tsutsui, H., et al. (2013). Innate immunomodulation by lipophilic termini of lipopolysaccharide; synthesis of lipid as from Porphyromonas gingivalis and other bacteria and their immunomodulative responses. Mol. Biosyst. 9 (5), 987–996. doi: 10.1039/c3mb25477a
Fung, A., Vizcaychipi, M., Lloyd, D., Wan, Y., Ma, D. (2012). Central nervous system inflammation in disease related conditions: mechanistic prospects. Brain Res. 1446, 144–155. doi: 10.1016/j.brainres.2012.01.061
Furugen, R., Hayashida, H., Saito, T. (2013). Porphyromonas gingivalis and escherichia coli lipopolysaccharide causes resistin release from neutrophils. Oral. Dis. 19 (5), 479–483. doi: 10.1111/odi.12027
Furusho, H., Miyauchi, M., Hyogo, H., Inubushi, T., Ao, M., Ouhara, K., et al. (2013). Dental infection of Porphyromonas gingivalis exacerbates high fat diet-induced steatohepatitis in mice. J. Gastroenterol. 48 (11), 1259–1270. doi: 10.1007/s00535-012-0738-1
Ganz, T., Fainstein, N., Ben-Hur, T. (2022). When the infectious environment meets the AD brain. Mol. Neurodegeneration 17 (1), 1–11. doi: 10.1186/s13024-022-00559-3
Gao, R., Fu, Y., Wei, Z., Zhou, E., Li, Y., Yao, M., et al. (2014). Chlorogenic acid attenuates lipopolysaccharide-induced mice mastitis by suppressing TLR4-mediated NF-kappa b signaling pathway. Eur. J. Pharmacol. 729, 54–58. doi: 10.1016/j.ejphar.2014.01.015
Gao, L., Miao, D., Meng, S., Zhao, L., Guo, S., Wu, Y. (2012). Heterogenic abilities in adhesion and invasion among clinical isolates of Porphyromonas gingivalis with type II fimbriae. Afr. J. Microbiol. Res. 6 (11), 2727–2734. doi: 10.5897/ajmr11.1283
Garbossa, S. G., Folli, F. (2017). Vitamin d, sub-inflammation and insulin resistance. a window on a potential role for the interaction between bone and glucose metabolism. Rev. Endocrine Metab. Disord. 18 (2), 243–258. doi: 10.1007/s11154-017-9423-2
Ge, Q. M., Du, S. C., Bian, F., Lin, N., Su, Q. (2011). Effects of lipopolysaccharides on TLR4 expression in INS-1 rat insulinoma cells. Cell. Mol. Biol. (Noisy-le-Grand France) 57 Suppl, OL1513–OL1519.
Genco, R. J., Grossi, S. G., Ho, A., Nishimura, F., Murayama, Y. (2005). A proposed model linking inflammation to obesity, diabetes, and periodontal infections. J. Periodontology 76 (11), 2075–2084. doi: 10.1902/jop.2005.76.11-S.2075
Gomez-Hernandez, A., de las Heras, N., Lopez-Pastor, A. R., Garcia-Gomez, G., Infante-Menendez, J., Gonzalez-Lopez, P., et al. (2021). Severe hepatic insulin resistance induces vascular dysfunction: improvement by liver-specific insulin receptor isoform a gene therapy in a murine diabetic model. Cells 10 (8), 2035. doi: 10.3390/cells10082035
Gonzalez, L., Anderson, I., Deane, D., Summers, C., Buxton, D. (2001). Detection of immune system cells in paraffin wax-embedded ovine tissues. J. Comp. Pathol. 125 (1), 41–47. doi: 10.1053/jcpa.2001.0475
Goodpaster, B. H., Sparks, L. M. (2017). Metabolic flexibility in health and disease. Cell Metab. 25 (5), 1027–1036. doi: 10.1016/j.cmet.2017.04.015
Govindaraj, K., Sudhakar, U., Bhuminathan, S., Govindaraj, J. (2021). Resistin levels in the gingival crevicular fluid among diabetic and non-diabetic chronic periodontitis patients. Bioinformation 17 (10), 899–902. doi: 10.6026/97320630017899
Greggianin, B. F., Marques, A. E. M., Amato, A. A., de Lima, C. L. (2023). Effect of periodontal therapy on insulin resistance in adults with dysglycemia and periodontitis: a systematic review and meta-analysis. Clin. Oral. Investig. 27 (4), 1329–1342. doi: 10.1007/s00784-023-04879-6
Guo, Z., Chen, Y., Mao, Y.-F., Zheng, T., Jiang, Y., Yan, Y., et al. (2017). Long-term treatment with intranasal insulin ameliorates cognitive impairment, tau hyperphosphorylation, and microglial activation in a streptozotocin-induced alzheimer's rat model. Sci. Rep. 7, 45971. doi: 10.1038/srep45971
Gurav, A., Jadhav, V. (2011). Periodontitis and risk of diabetes mellitus. J. Diabetes 3 (1), 21–28. doi: 10.1111/j.1753-0407.2010.00098.x
Hajishengallis, G. (2014). Immunomicrobial pathogenesis of periodontitis: keystones, pathobionts, and host response. Trends Immunol. 35 (1), 3–11. doi: 10.1016/j.it.2013.09.001
Halade, G. V., Tourki, B. (2019). “Specialized pro-resolving mediators directs cardiac healing and repair with activation of inflammation and resolution program in heart failure,” in Role of bioactive lipids in cancer, inflammation and related diseases. Eds. Honn, K. V., Zeldin, D. C. (West Berlin: Springer), 45–64.
Harding, A., Robinson, S., Crean, S. J., Singhrao, S. K. (2017). Can better management of periodontal disease delay the onset and progression of alzheimer's disease? J. Alzheimers Dis. 58 (2), 337–348. doi: 10.3233/jad-170046
He, J., Shen, X., Fu, D., Yang, Y., Xiong, K., Zhao, L., et al. (2022). Human periodontitis-associated salivary microbiome affects the immune response of diabetic mice. J. Oral. Microbiol. 14 (1), 2107814. doi: 10.1080/20002297.2022.2107814
Hemmi, H., Akira, S. (2005). TLR signalling and the function of dendritic cells. Chem. Immunol. Allergy 86, 120–135. doi: 10.1159/000086657
Hiroshima, Y., Bando, M., Inagaki, Y., Mihara, C., Kataoka, M., Murata, H., et al. (2012). Resistin in gingival crevicular fluid and induction of resistin release by Porphyromonas gingivalis lipopolysaccharide in human neutrophils. J. Periodontal Res. 47 (5), 554–562. doi: 10.1111/j.1600-0765.2011.01466.x
Holmes, C., Cunningham, C., Zotova, E., Woolford, J., Dean, C., Kerr, S., et al. (2009). Systemic inflammation and disease progression in Alzheimer disease. Neurology 73 (10), 768–774. doi: 10.1212/WNL.0b013e3181b6bb95
Hoshino, K., Takeuchi, O., Kawai, T., Sanjo, H., Ogawa, T., Takeda, Y., et al. (1999). Cutting edge: toll-like receptor 4 (TLR4)-deficient mice are hyporesponsive to lipopolysaccharide: evidence for TLR4 as the lps gene product. J. Immunol. 162 (7), 3749–3752.
Hotamisligil, G. S. (2017). Inflammation, metaflammation and immunometabolic disorders. Nature 542 (7640), 177–185. doi: 10.1038/nature21363
Hrishi, T. S., Kundapur, P. P., Naha, A., Thomas, B. S., Kamath, S., Bhat, G. S. (2016). Effect of adjunctive use of green tea dentifrice in periodontitis patients - a randomized controlled pilot study. Int. J. Dental Hygiene 14 (3), 178–183. doi: 10.1111/idh.12131
Hu, Z. Z., Zhou, J., Han, L., Li, X. X., Li, C., Wu, T. Y., et al. (2022). Acyclovir alleviates insulin resistance via activating PKM1 in diabetic mice. Life Sci. 304. doi: 10.1016/j.lfs.2022.120725
Huang, Y. L., Zeng, J., Chen, G. Q., Xie, X. D., Guo, W. H., Tian, W. D. (2016). Periodontitis contributes to adipose tissue inflammation through the NF-kappa b, JNK and ERK pathways to promote insulin resistance in a rat model. Microbes Infection 18 (12), 804–812. doi: 10.1016/j.micinf.2016.08.002
Huang, X., Yu, T., Ma, C., Wang, Y., Xie, B., Xuan, D., et al. (2017). Macrophages play a key role in the obesity-induced periodontal innate immune dysfunction via nucleotide-binding oligomerization domain-like receptor protein 3 pathway. J. periodontology 88 (6), 614. doi: 10.1902/jop.2017.176004
Iida, Y., Honda, K., Suzuki, T., Matsukawa, S., Kawai, T., Shimahara, T., et al. (2004). Brain abscess in which Porphyromonas gingivalis was detected in cerebrospinal fluid. Br. J. Oral. Maxillofac. Surg. 42 (2), 180–180. doi: 10.1016/s0266-4356(03)00190-6
Ikai, R., Hasegawa, Y., Izumigawa, M., Nagano, K., Yoshida, Y., Kitai, N., et al. (2015). Mfa4, an accessory protein of Mfa1 fimbriae, modulates fimbrial biogenesis, cell auto-aggregation, and biofilm formation in Porphyromonas gingivalis. PloS One 10 (10), e0139454. doi: 10.1371/journal.pone.0139454
Ilievski, V., Bhat, U. G., Suleiman-Ata, S., Bauer, B. A., Toth, P. T., Olson, S. T., et al. (2017). Oral application of a periodontal pathogen impacts SerpinE1 expression and pancreatic islet architecture in prediabetes. J. Periodontal Res. 52 (6), 1032–1041. doi: 10.1111/jre.12474
Ilievski, V., Kinchen, J. M., Prabhu, R., Rim, F., Leoni, L., Unterman, T. G., et al. (2016). Experimental periodontitis results in prediabetes and metabolic alterations in brain, liver and heart: global untargeted metabolomic analyses. J. Oral. Biol. (Northborough Mass.) 3 (1), e0204941. doi: 10.13188/2377-987x.1000020
Ilievski, V., Zuchowska, P. K., Green, S. J., Toth, P. T., Ragozzino, M. E., Le, K., et al. (2018). Chronic oral application of a periodontal pathogen results in brain inflammation, neurodegeneration and amyloid beta production in wild type mice. PLoS One 13 (10). doi: 10.1371/journal.pone.0204941
Ioana, M., Ferwerda, B., Plantinga, T. S., Stappers, M., Oosting, M., McCall, M., et al. (2012). Different patterns of toll-like receptor 2 polymorphisms in populations of various ethnic and geographic origins. Infection Immun. 80 (5), 1917–1922. doi: 10.1128/iai.00121-12
Ishikawa, M., Yoshida, K., Okamura, H., Ochiai, K., Takamura, H., Fujiwara, N., et al. (2013). Oral Porphyromonas gingivalis translocates to the liver and regulates hepatic glycogen synthesis through the Akt/GSK-3 beta signaling pathway. Biochim. Et Biophys. Acta-Molecular Basis Dis. 1832 (12), 2035–2043. doi: 10.1016/j.bbadis.2013.07.012
Iwasaki, A., Medzhitov, R. (2004). Toll-like receptor control of the adaptive immune responses. Nat. Immunol. 5 (10), 987–995. doi: 10.1038/ni1112
Jiang, Q. Q., Akashi, S., Miyake, K., Petty, H. R. (2000). Cutting edge: lipopolysaccharide induces physical proximity between CD14 and toll-like receptor 4 (TLR4) prior to nuclear translocation of NF-kappa b. J. Immunol. 165 (7), 3541–3544. doi: 10.4049/jimmunol.165.7.3541
Jung, T. W., Kim, H., Park, S. Y., Cho, W., Oh, H., Lee, H. J., et al. (2022). Stachydrine alleviates lipid-induced skeletal muscle insulin resistance via AMPK/HO-1-mediated suppression of inflammation and endoplasmic reticulum stress. J. Endocrinological Invest. 45 (11), 2181–2191. doi: 10.1007/s40618-022-01866-8
Kang, N., Zhang, Y., Xue, F., Duan, J. Y., Chen, F., Cai, Y., et al. (2022). Periodontitis induced by Porphyromonas gingivalis drives impaired glucose metabolism in mice. Front. Cell. Infection Microbiol. 12. doi: 10.3389/fcimb.2022.998600
Kashiwagi, Y., Aburaya, S., Sugiyama, N., Narukawa, Y., Sakamoto, Y., Takahashi, M., et al. (2021). Porphyromonas gingivalis induces entero-hepatic metabolic derangements with alteration of gut microbiota in a type 2 diabetes mouse model (vol 11, 18398, 2021). Sci. Rep. 11 (1), 18398. doi: 10.1038/s41598-021-99556-7
Khalid, M., Alkaabi, J., Khan, M. A. B., Adem, A. (2021). Insulin signal transduction perturbations in insulin resistance. Int. J. Mol. Sci. 22 (16), 8590. doi: 10.3390/ijms22168590
Kheirollahzadeh, F., Eftekhari, E., Ghollasi, M., Behzadi, P. (2022). Anti-hyperglycemic effects of eryngium billardierei f. delaroche extract on insulin-resistance HepG2 cells in vitro. Mol. Biol. Rep. 49 (5), 3401–3411. doi: 10.1007/s11033-022-07171-0
Khodabandehloo, H., Gorgani-Firuzjaee, S., Panahi, G., Meshkani, R. (2016). Molecular and cellular mechanisms linking inflammation to insulin resistance and beta-cell dysfunction. Trans. Res. 167 (1), 228–256. doi: 10.1016/j.trsl.2015.08.011
Kim, S., Bando, Y., Chang, C. Y., Kwon, J., Tarverti, B., Kim, D., et al. (2022). Topical application of Porphyromonas gingivalis into the gingival pocket in mice leads to chronic-active infection, periodontitis and systemic inflammation. Int. J. Mol. Med. 50 (2), 1–14. doi: 10.3892/ijmm.2022.5159
Kouidrat, Y., Amad, A., Arai, M., Miyashita, M., Lalau, J.-D., Loas, G., et al. (2015). Advanced glycation end products and schizophrenia: a systematic review. J. Psychiatr. Res. 66-67, 112–117. doi: 10.1016/j.jpsychires.2015.04.023
Kuboniwa, M., Amano, A., Hashino, E., Yamamoto, Y., Inaba, H., Hamada, N., et al. (2009). Distinct roles of long/short fimbriae and gingipains in homotypic biofilm development by Porphyromonas gingivalis. BMC Microbiol. 9, 1–13. doi: 10.1186/1471-2180-9-105
Kuroe, A., Taniguchi, A., Sekiguchi, A., Ogura, M., Murayama, Y., Nishimura, F., et al. (2004). Prevalence of periodontal bacterial infection in non-obese Japanese type 2 diabetic patients: relationship with c-reactive protein and albuminuria. Hormone Metab. Res. 36 (2), 116–118. doi: 10.1055/s-2004-814221
Lalla, E., Papapanou, P. N. (2011). Diabetes mellitus and periodontitis: a tale of two common interrelated diseases. Nat. Rev. Endocrinol. 7 (12), 738–748. doi: 10.1038/nrendo.2011.106
Lappin, D. F., Sherrabeh, S., Erridge, C. (2011). Stimulants of toll-like receptors 2 and 4 are elevated in saliva of periodontitis patients compared with healthy subjects. J. Clin. Periodontology 38 (4), 318–325. doi: 10.1111/j.1600-051X.2011.01702.x
Lazar, V., Ditu, L. M., Pircalabioru, G. G., Gheorghe, I., Curutiu, C., Holban, A. M., et al. (2018). Aspects of gut microbiota and immune system interactions in infectious diseases, immunopathology, and cancer. Front. Immunol. 9. doi: 10.3389/fimmu.2018.01830
Leblhuber, F., Ehrlich, D., Steiner, K., Geisler, S., Fuchs, D., Lanser, L., et al. (2021). The immunopathogenesis of alzheimer's disease is related to the composition of gut microbiota. Nutrients 13 (2), 361. doi: 10.3390/nu13020361
Lee, S. M., Han, H. W., Yim, S. Y. (2015). Beneficial effects of soy milk and fiber on high cholesterol diet-induced alteration of gut microbiota and inflammatory gene expression in rats. Food Funct. 6 (2), 492–500. doi: 10.1039/c4fo00731j
Lee, K. M., Seong, S. Y. (2009). Partial role of TLR4 as a receptor responding to damage-associated molecular pattern. Immunol. Lett. 125 (1), 31–39. doi: 10.1016/j.imlet.2009.05.006
Le Sage, F., Meilhac, O., Gonthier, M.-P. (2017). Anti-inflammatory and antioxidant effects of polyphenols extracted from antirhea borbonica medicinal plant on adipocytes exposed to Porphyromonas gingivalis and escherichia coli lipopolysaccharides. Pharmacol. Res. 119, 303–312. doi: 10.1016/j.phrs.2017.02.020
Liang, H., Tantiwong, P., Sriwijitkamol, A., Shanmugasundaram, K., Mohan, S., Espinoza, S., et al. (2013). Effect of a sustained reduction in plasma free fatty acid concentration on insulin signalling and inflammation in skeletal muscle from human subjects. J. Physiology-London 591 (11), 2897–2909. doi: 10.1113/jphysiol.2012.247510
Lin, X. T., Zhang, J. F., Wang, X. M., Lin, G. M., Chen, T. T. (2020). Pre-activation with TLR7 in combination with thioridazine and loratadine promotes tumoricidal T-cell activity in colorectal cancer. Anti-Cancer Drugs 31 (10), 989–996. doi: 10.1097/cad.0000000000000972
Lu, Z., Li, Y., Brinson, C. W., Kirkwood, K. L., Lopes-Virella, M. F., Huang, Y. (2017). CD36 is upregulated in mice with periodontitis and metabolic syndrome and involved in macrophage gene upregulation by palmitate. Oral. Dis. 23 (2), 210–218. doi: 10.1111/odi.12596
Lv, L. X., Jiang, H. Y., Yan, R., Li, L. J. (2019). Interactions between gut microbiota and hosts and their role in infectious diseases. Infect. Microbes Dis. 1 (1), 3–9. doi: 10.1097/im9.0000000000000001
Lv, Y.-T., Zeng, J.-J., Lu, J.-Y., Zhang, X.-Y., Xu, P.-P., Su, Y. (2021). Porphyromonas gingivalis lipopolysaccharide (Pg-LPS) influences adipocytes injuries through triggering XBP1 and activating mitochondria-mediated apoptosis. Adipocyte 10 (1), 28–37. doi: 10.1080/21623945.2020.1856527
Ma, D., Tao, B., Warashina, S., Kotani, S., Lu, L., Kaplamadzhiev, D. B., et al. (2007). Expression of free fatty acid receptor GPR40 in the central nervous system of adult monkeys. Neurosci. Res. 58 (4), 394–401. doi: 10.1016/j.neures.2007.05.001
Manning-Tobin, J. J., Moore, K. J., Seimon, T. A., Bell, S. A., Sharuk, M., Alvarez-Leite, J. I., et al. (2009). Loss of SR-a and CD36 activity reduces atherosclerotic lesion complexity without abrogating foam cell formation in hyperlipidemic mice. Arterioscler. Thromb. Vasc. Biol. 29 (1), 19–U51. doi: 10.1161/atvbaha.108.176644
Marques, E. S., Formato, E., Liang, W. L., Leonard, E., Timme-Laragy, A. R. (2022). Relationships between type 2 diabetes, cell dysfunction, and redox signaling: a meta-analysis of single-cell gene expression of human pancreatic alpha- and beta-cells. J. Diabetes 14 (1), 34–51. doi: 10.1111/1753-0407.13236
Martinez-Martinez, R. E., Abud-Mendoza, C., Patino-Marin, N., Rizo-Rodriguez, J. C., Little, J. W., Pablo Loyola-Rodriguez, J. (2009). Detection of periodontal bacterial DNA in serum and synovial fluid in refractory rheumatoid arthritis patients. J. Clin. Periodontology 36 (12), 1004–1010. doi: 10.1111/j.1600-051X.2009.01496.x
Martin-Gonzalez, C., Martin-Folgueras, T., Carlos Quevedo-Abeledo, J., De Vera-Gonzalez, A., Gonzalez-Delgado, A., De Armas-Rillo, L., et al. (2022). Apolipoprotein c-III is linked to the insulin resistance and beta-cell dysfunction that are present in rheumatoid arthritis. Arthritis Res. Ther. 24 (1), 126. doi: 10.1186/s13075-022-02822-w
Masters, S. L., Dunne, A., Subramanian, S. L., Hull, R. L., Tannahill, G. M., Sharp, F. A., et al. (2010). Activation of the NLRP3 inflammasome by islet amyloid polypeptide provides a mechanism for enhanced IL-1 beta in type 2 diabetes. Nat. Immunol. 11 (10), 897–U1501. doi: 10.1038/ni.1935
Mathur, A., Hayward, J. A., Man, S. M. (2018). Molecular mechanisms of inflammasome signaling. J. Leukocyte Biol. 103 (2), 233–257. doi: 10.1189/jlb.3MR0617-250R
Mayer, S., Raulf, M.-K., Lepenies, B. (2017). C-type lectins: their network and roles in pathogen recognition and immunity. Histochem. Cell Biol. 147 (2), 223–237. doi: 10.1007/s00418-016-1523-7
Mehta, J. L., Sanada, N., Hu, C. P., Chen, J., Dandapat, A., Sugawara, F., et al. (2007). Deletion of LOX-1 reduces atherogenesis in LDLR knockout mice fed high cholesterol diet. Circ. Res. 100 (11), 1634–1642. doi: 10.1161/circresaha.107.149724
Mendiola, A. S., Garza, R., Cardona, S. M., Mythen, S. A., Lira, S. A., Akassoglou, K., et al. (2017). Fractalkine signaling attenuates perivascular clustering of microglia and fibrinogen leakage druing systemic inflammation in mouse models of diabetic retionopathy. Front. Cell. Neurosci. 10. doi: 10.3389/fncel.2016.00303
Mezza, T., Cinti, F., Cefalo, C. M. A., Pontecorvi, A., Kulkarni, R. N., Giaccari, A. (2019). Beta-cell fate in human insulin resistance and type 2 diabetes: a perspective on islet plasticity. Diabetes 68 (6), 1121–1129. doi: 10.2337/db18-0856
Miquilena-Colina, M. E., Lima-Cabello, E., Sanchez-Campos, S., Garcia-Mediavilla, M. V., Fernandez-Bermejo, M., Lozano-Rodriguez, T., et al. (2011). Hepatic fatty acid translocase CD36 upregulation is associated with insulin resistance, hyperinsulinaemia and increased steatosis in non-alcoholic steatohepatitis and chronic hepatitis c. Gut 60 (10), 1394–1402. doi: 10.1136/gut.2010.222844
Mizoguchi, E., Orihara, K., Hamasaki, S., Ishida, S., Kataoka, T., Ogawa, M., et al. (2007). Association between toll-like receptors and the extent and severity of coronar, artery disease in patients with stable angina. Coronary Artery Dis. 18 (1), 31–38. doi: 10.1097/MCA.0b013e328010a474
Moen, K., Brun, J. G., Valen, M., Skartveit, L., Eribe, E. K. R., Olsen, I., et al. (2006). Synovial inflammation in active rheumatoid arthritis and psoriatic arthritis facilitates trapping of a variety of oral bacterial DNAs. Clin. Exp. Rheumatol. 24 (6), 656–663.
Monasterio, E., Fernandez, S., Castillo, B., Rojas, E., Cafferata, S., Rojas, C., et al. (2019). Capsular-defective Porphyromonas gingivalis mutant strains induce less alveolar bone resorption than W50 wild-type strain due to a decreased Th1/Th17 immune response and less osteoclast activity. J. Periodontol 90 (5), 522–543. doi: 10.1002/jper.18-0079
Morello, E., Sutti, S., Foglia, B., Novo, E., Cannito, S., Bocca, C., et al. (2018). Hypoxia-inducible factor 2α drives nonalcoholic fatty liver progression by triggering hepatocyte release of histidine-rich glycoprotein. Hepatology 67 (6), 2196–2214. doi: 10.1002/hep.29754
Morris, J. K., Vidoni, E. D., Honea, R. A., Burns, J. M., Alzheimers Dis Neuroimaging, I. (2014). Impaired glycemia increases disease progression in mild cognitive impairment. Neurobiol. Aging 35 (3), 585–589. doi: 10.1016/j.neurobiolaging.2013.09.033
Nagano, K., Hasegawa, Y., Yoshida, Y., Yoshimura, F. (2017). Novel fimbrilin PGN_1808 in Porphyromonas gingivalis. PLoS One 12 (3), e0173541. doi: 10.1371/journal.pone.0173541
Nagao, Y., Takahashi, H., Kawaguchi, A., Kitagaki, H. (2021). Effect of fermented rice drink "Amazake" on patients with nonalcoholic fatty liver disease and periodontal disease: a pilot study. Reports 4 (4), 36. doi: 10.3390/reports4040036
Nagaoka, K., Yanagihara, K., Harada, Y., Yamada, K., Migiyama, Y., Morinaga, Y., et al. (2017). Quantitative detection of periodontopathic bacteria in lower respiratory tract specimens by real-time PCR. J. Infection Chemotherapy 23 (2), 69–73. doi: 10.1016/j.jiac.2016.09.013
Naginyte, M., Thuy, D., Meade, J., Devine, D. A., Marsh, P. D. (2019). Enrichment of periodontal pathogens from the biofilms of healthy adults. Sci. Rep. 9, 5491. doi: 10.1038/s41598-019-41882-y
Nakahara, T., Hyogo, H., Ono, A., Nagaoki, Y., Kawaoka, T., Miki, D., et al. (2018). Involvement of Porphyromonas gingivalis in the progression of non-alcoholic fatty liver disease. J. Gastroenterol. 53 (2), 269–280. doi: 10.1007/s00535-017-1368-4
Nakayama, M., Ohara, N. (2017). Molecular mechanisms of Porphyromonas gingivalis-host cell interaction on periodontal diseases. Japanese Dental Sci. Rev. 53 (4), 134–140. doi: 10.1016/j.jdsr.2017.06.001
Ni, J., Chen, L., Zhong, S., Chai, Q., Zhang, L., Wang, D., et al. (2018). Influence of periodontitis and scaling and root planing on insulin resistance and hepatic CD36 in obese rats. J. Periodontology 89 (4), 476–485. doi: 10.1002/jper.17-0115
Nishihara, R., Sugano, N., Takano, M., Shimada, T., Tanaka, H., Oka, S., et al. (2009). The effect of Porphyromonas gingivalis infection on cytokine levels in type 2 diabetic mice. J. Periodontal Res. 44 (3), 305–310. doi: 10.1111/j.1600-0765.2008.01130.x
Nishimura, W., Oishi, H., FunahaShi, N., Fujiwara, T., Takahashi, S., Yasuda, K. (2015). Generation and characterization of MafA-kusabira orange mice. Endocrine J. 62 (1), 37–51. doi: 10.1507/endocrj.EJ14-0296
Ogrendik, M. (2012). Does periodontopathic bacterial infection contribute to the etiopathogenesis of the autoimmune disease rheumatoid arthritis? Discovery Med. 13 (72), 349–355.
Oh, B. K., Lee, S.-J., Kim, H., Choi, H.-I., Lee, J.-Y., Lee, S. H., et al. (2021). Relationship between alcohol consumption and insulin resistance measured using the homeostatic model assessment for insulin resistance: a retrospective cohort study of 280,194 people. Nutr. Metab. Cardiovasc. Dis. 31 (10), 2842–2850. doi: 10.1016/j.numecd.2021.06.023
Palmer, L. J., Chapple, I. L. C., Wright, H. J., Roberts, A., Cooper, P. R. (2012). Extracellular deoxyribonuclease production by periodontal bacteria. J. Periodontal Res. 47 (4), 439–445. doi: 10.1111/j.1600-0765.2011.01451.x
Papale, M., Ferretti, E., Battaglia, G., Bellavia, D., Mai, A., Tafani, M. (2018). EZH2, HIF-1, and their inhibitors: an overview on pediatric cancers. Front. Pediatr. 6. doi: 10.3389/fped.2018.00328
Park, S.-N., Park, J.-Y., Kook, J.-K. (2011). Development of porphyromonas gingivalis-specific quantitative real-time PCR primers based on the nucleotide sequence of rpoB. J. Microbiol. 49 (2), 315–319. doi: 10.1007/s12275-011-1028-y
Patrakka, O., Pienimaki, J., Tuomisto, S., Ollikainen, J., Lehtimaki, T., Karhunen, P. J., et al. (2019). Oral bacterial signatures in cerebral thrombi of patients with acute ischemic stroke treated with thrombectomy. J. Am. Heart Assoc. 8 (11), e012330. doi: 10.1161/jaha.119.012330
Peng, G., Li, L. H., Liu, Y. B., Pu, J., Zhang, S. Y., Yu, J. H., et al. (2011). Oleate blocks palmitate-induced abnormal lipid distribution, endoplasmic reticulum expansion and stress, and insulin resistance in skeletal muscle. Endocrinology 152 (6), 2206–2218. doi: 10.1210/en.2010-1369
Petersen, M. C., Shulman, G. I. (2018). Mechanisms of insulin action and insulin resistance. Physiol. Rev. 98 (4), 2133–2223. doi: 10.1152/physrev.00063.2017
Pirih, F. Q., Monajemzadeh, S., Singh, N., Sinacola, R. S., Shin, J. M., Chen, T., et al. (2021). Association between metabolic syndrome and periodontitis: the role of lipids, inflammatory cytokines, altered host response, and the microbiome. Periodontology 2000 87 (1), 50–75. doi: 10.1111/prd.12379
Poole, S., Singhrao, S. K., Chukkapalli, S., Rivera, M., Velsko, I., Kesavalu, L., et al. (2015). Active invasion of Porphyromonas gingivalis and infection-induced complement activation in ApoE(-/-) mice brains. J. Alzheimers Dis. 43 (1), 67–80. doi: 10.3233/jad-140315
PrayGod, G., Filteau, S., Range, N., Kitilya, B., Kavishe, B. B., Ramaiya, K., et al. (2021). Beta-cell dysfunction and insulin resistance in relation to pre-diabetes and diabetes among adults in north-western Tanzania: a cross-sectional study. Trop. Med. Int. Health 26 (4), 435–443. doi: 10.1111/tmi.13545
Pussinen, P. J., Alfthan, G., Jousilahti, P., Paju, S., Tuomilehto, J. (2007). Systemic exposure to Porphyromonas gingivalis predicts incident stroke. Atherosclerosis 193 (1), 222–228. doi: 10.1016/j.atherosclerosis.2006.06.027
Ramenzoni, L. L., Zuellig, R. A., Hussain, A., Lehmann, R., Heumann, C., Attin, T., et al. (2019). Bacterial supernatants elevate glucose-dependent insulin secretion in rat pancreatic INS-1 line and islet -cells via PI3K/AKT signaling. Mol. Cell. Biochem. 452 (1-2), 17–27. doi: 10.1007/s11010-018-3408-7
Reichert, S., Haffner, M., Keysser, G., Schaefer, C., Stein, J. M., Schaller, H.-G., et al. (2013). Detection of oral bacterial DNA in synovial fluid. J. Clin. Periodontology 40 (6), 591–598. doi: 10.1111/jcpe.12102
Rodriguez, J., Delzenne, N. M. (2021). Modulation of the gut microbiota-adipose tissue-muscle interactions by prebiotics. J. Endocrinol. 249 (1), R1–R23. doi: 10.1530/joe-20-0499
Rojo-Botello, N. R., Garcia-Hernandez, A. L., Moreno-Fierros, L. (2012). Expression of toll-like receptors 2, 4 and 9 is increased in gingival tissue from patients with type 2 diabetes and chronic periodontitis. J. Periodontal Res. 47 (1), 62–73. doi: 10.1111/j.1600-0765.2011.01405.x
Romanenko, M., Kholin, V., Koliada, A., Vaiserman, A. (2021). Nutrition, gut microbiota, and alzheimer's disease. Front. Psychiatry 12. doi: 10.3389/fpsyt.2021.712673
Rowan, S., Bejarano, E., Taylor, A. (2018). Mechanistic targeting of advanced glycation end-products in age-related diseases. Biochim. Et Biophys. Acta-Molecular Basis Dis. 1864 (12), 3631–3643. doi: 10.1016/j.bbadis.2018.08.036
Sakalauskiene, J., Kubilius, R., Gleiznys, A., Vitkauskiene, A., Ivanauskiene, E., Saferis, V. (2014). Relationship of clinical and microbiological variables in patients with type 1 diabetes mellitus and periodontitis. Med. Sci. Monitor 20, 1871. doi: 10.12659/MSM.890879
Sasaki, N., Katagiri, S., Komazaki, R., Watanabe, K., Maekawa, S., Shiba, T., et al. (2018). Endotoxemia by Porphyromonas gingivalis injection aggravates non-alcoholic fatty liver disease, disrupts Glucose/Lipid metabolism, and alters gut microbiota in mice. Front. Microbiol. 9, e015546. doi: 10.3389/fmicb.2018.02470
Sasaki, N., Ozono, R., Higashi, Y., Maeda, R., Kihara, Y. (2020). Association of insulin resistance, plasma glucose level, and serum insulin level with hypertension in a population with different stages of impaired glucose metabolism. J. Am. Heart Assoc. 9 (7). doi: 10.1161/jaha.119.015546
Satoh, Y., Ishihara, K. (2020). Investigation of the antimicrobial activity of bilberry (Vaccinium myrtillus l.) extract against periodontopathic bacteria. J. Oral. Biosci. 62 (2), 169–174. doi: 10.1016/j.job.2020.01.009
Savage, D. B., Petersen, K. F., Shulman, G. I. (2007). Disordered lipid metabolism and the pathogenesis of insulin resistance. Physiol. Rev. 87 (2), 507–520. doi: 10.1152/physrev.00024.2006
Seyama, M., Yoshida, K., Yoshida, K., Fujiwara, N., Ono, K., Eguchi, T., et al. (2020). Outer membrane vesicles of Porphyromonas gingivalis attenuate insulin sensitivity by delivering gingipains to the liver. Biochim. Et Biophys. Acta-Molecular Basis Dis. 1866 (6), 165731. doi: 10.1016/j.bbadis.2020.165731
Sharma, A., Lee-Odegard, S., Qvigstad, E., Sommer, C., Sattar, N., Gill, J. M. R., et al. (2022). Beta-cell function, hepatic insulin clearance, and insulin sensitivity in south Asian and Nordic women after gestational diabetes mellitus. Diabetes 71 (12), 2530–2538. doi: 10.2337/db22-0622
Shaukat, A., Hussain, G., Irfan, S., Ijaz, M. U., Anwar, H. (2022). Therapeutic potential of MgO and MnO nanoparticles within the context of thyroid profile and pancreatic histology in a diabetic rat model. Dose-Response 20 (3), 15593258221128743. doi: 10.1177/15593258221128743
Shikama, Y. (2018). Free fatty acids may be involved in the pathogenesis of oral-related and cardiovascular diseases. J. Oral. Biosci. 60 (3), 65–69. doi: 10.1016/j.job.2018.04.001
Shinjyo, N., Kita, K. (2021). Infection and immunometabolism in the central nervous system: a possible mechanistic link between metabolic imbalance and dementia. Front. Cell. Neurosci. 15. doi: 10.3389/fncel.2021.765217
Singh, A., Wyant, T., Anaya-Bergman, C., Aduse-Opoku, J., Brunner, J., Laine, M. L., et al. (2011). The capsule of Porphyromonas gingivalis leads to a reduction in the host inflammatory response, evasion of phagocytosis, and increase in virulence. Infection Immun. 79 (11), 4533–4542. doi: 10.1128/iai.05016-11
Sinha, S., Haque, M. (2022). Insulin resistance is cheerfully hitched with hypertension. Life-Basel 12 (4), 564. doi: 10.3390/life12040564
Soto, C., Rojas, V., Yanez, L., Hidalgo, A., Olivera, M., Pacheco, M., et al. (2022). Porphyromonas gingivalis-helicobacter pylori co-incubation enhances Porphyromonas gingivalis virulence and increases migration of infected human oral keratinocytes. J. Oral. Microbiol. 14 (1), 2107691. doi: 10.1080/20002297.2022.2107691
Souccar, N. M., Chakhtoura, M., Ghafari, J. G., Abdelnoor, A. M. (2010). Porphyromonas gingivalis in dental plaque and serum c-reactive protein levels in pregnancy. J. Infection Developing Countries 4 (6), 362–366. doi: 10.3855/jidc.1031
Stein, P. S., Steffen, M. J., Smith, C., Jicha, G., Ebersole, J. L., Abner, E., et al. (2012). Serum antibodies to periodontal pathogens are a risk factor for alzheimer's disease. Alzheimers Dementia 8 (3), 196–203. doi: 10.1016/j.jalz.2011.04.006
Sun, Q., Li, J., Gao, F. (2014). New insights into insulin: the anti-inflammatory effect and its clinical relevance. World J. Diabetes 5 (2), 89–96. doi: 10.4239/wjd.v5.i2.89
Sutton, J. D., Martinez, M. L. S., Gerkovich, M. M. (2017). Environmental tobacco smoke and periodontitis in united states non-smokers 2009 to 2012. J. Periodontology 88 (6), 565–574. doi: 10.1902/jop.2017.160725
Swarup, S., Sabharwal, P., Meena, M. K., Girdhar, A., Ganjoo, D., Khippal, J. (2022). Calprotectin and n-telopeptide of type I collagen (NTx) as gingival crevicular fluid (GCF) biomarker in peri-implantitis patients. Cureus J. Med. Sci. 14 (8). doi: 10.7759/cureus.28430
Thalla, S., Kamaraj, R., Kavitha, A. (2022). Increasing risk factors for non-alcoholic fatty liver disease; an insight into chronic periodontitis and insulin resistance. Endocrine Metab. Immune Disorders-Drug Targets 22 (8), 807–814. doi: 10.2174/1871530322666220104095534
Thanakun, S., Watanabe, H., Thaweboon, S., Izumi, Y. (2014). Association of untreated metabolic syndrome with moderate to severe periodontitis in Thai population. J. Periodontology 85 (11), 1502–1514. doi: 10.1902/jop.2014.140105
Thouvenot, K., Turpin, T., Taile, J., Clement, K., Meilhac, O., Gonthier, M.-P. (2022). Links between insulin resistance and periodontal bacteria: insights on molecular players and therapeutic potential of polyphenols. Biomolecules 12 (3), 378. doi: 10.3390/biom12030378
Tian, J., Liu, C., Zheng, X., Jia, X., Peng, X., Yang, R., et al. (2020). Porphyromonas gingivalis induces insulin resistance by increasing BCAA levels in mice. J. Dental Res. 99 (7), 839–846. doi: 10.1177/0022034520911037
Tomas, I., Diz, P., Tobias, A., Scully, C., Donos, N. (2012). Periodontal health status and bacteraemia from daily oral activities: systematic review/meta-analysis. J. Clin. Periodontology 39 (3), 213–228. doi: 10.1111/j.1600-051X.2011.01784.x
Totaro, M. C., Cattani, P., Ria, F., Tolusso, B., Gremese, E., Fedele, A. L., et al. (2013). Porphyromonas gingivalis and the pathogenesis of rheumatoid arthritis: analysis of various compartments including the synovial tissue. Arthritis Res. Ther. 15 (3), 1–8. doi: 10.1186/ar4243
Tsai, S., Clemente-Casares, X., Zhou, A. C., Lei, H., Ahn, J. J., Chan, Y. T., et al. (2018). Insulin receptor-mediated stimulation boosts T cell immunity during inflammation and infection. Cell Metab. 28 (6), 922–934 e4. doi: 10.1016/j.cmet.2018.08.003
van Niekerk, G., Christowitz, C., Conradie, D., Engelbrecht, A. M. (2020). Insulin as an immunomodulatory hormone. Cytokine Growth Factor Rev. 52, 34–44. doi: 10.1016/j.cytogfr.2019.11.006
Vlassara, H., Striker, G. E. (2011). AGE restriction in diabetes mellitus: a paradigm shift. Nat. Rev. Endocrinol. 7 (9), 526–539. doi: 10.1038/nrendo.2011.74
Wallet, S. M., Puri, V., Gibson, F. C., III (2018). Linkage of infection to adverse systemic complications: periodontal disease, toll-like receptors, and other pattern recognition systems. Vaccines 6 (2), 21. doi: 10.3390/vaccines6020021
Wang, Y. F., An, Z. Y., Lin, D. H., Jin, W. L. (2022b). Targeting cancer cachexia: molecular mechanisms and clinical study. Medcomm 3 (4), e164. doi: 10.1002/mco2.164
Wang, T., Ishikawa, T., Sasaki, M., Chiba, T. (2022a). Oral and gut microbial dysbiosis and non-alcoholic fatty liver disease: the central role of Porphyromonas gingivalis. Front. Med. 9. doi: 10.3389/fmed.2022.822190
Wang, Y. H., Kuang, Z., Yu, X. F., Ruhn, K. A., Kubo, M., Hooper, L. V. (2017). The intestinal microbiota regulates body composition through NFIL3 and the circadian clock. Science 357 (6354), 913–916. doi: 10.1126/science.aan0677
Wang, L., Zhang, J. J., Wang, B. K., Zhang, Y. W., Hong, J., Zhang, Y. F., et al. (2013). New evidence for an association between liver enzymes and pancreatic islet beta-cell dysfunction in young obese patients. Endocrine 44 (3), 688–695. doi: 10.1007/s12020-013-9937-7
Watanabe, K., Iizuka, T., Adeleke, A., Pham, L., Shlimon, A. E., Yasin, M., et al. (2011). Involvement of toll-like receptor 4 in alveolar bone loss and glucose homeostasis in experimental periodontitis. J. Periodontal Res. 46 (1), 21–30. doi: 10.1111/j.1600-0765.2010.01304.x
Watanabe, K., Katagiri, S., Takahashi, H., Sasaki, N., Maekawa, S., Komazaki, R., et al. (2021). Porphyromonas gingivalis impairs glucose uptake in skeletal muscle associated with altering gut microbiota. FASEB J. 35 (2), e21171. doi: 10.1096/fj.202001158R
Weishan, L., Dechao, L., Rongrong, Q. (2016). Effects of Porphyromonas gingivalis on interleukin-33 expression in rabbit vascular endothelium tissues. West China J. stomatology 34 (4), 354–357. doi: 10.7518/hxkq.2016.04.007
Xiao, E., Mattos, M., Vieira, G. H. A., Chen, S. S., Correa, J. D., Wu, Y. Y., et al. (2017). Diabetes enhances IL-17 expression and alters the oral microbiome to increase its pathogenicity. Cell Host Microbe 22 (1), 120–128. doi: 10.1016/j.chom.2017.06.014
Yano, H., Kinoshita, M., Fujino, K., Nakashima, M., Yamamoto, Y., Miyazaki, H., et al. (2012). Insulin treatment directly restores neutrophil phagocytosis and bactericidal activity in diabetic mice and thereby improves surgical site staphylococcus aureus infection. Infection Immun. 80 (12), 4409–4416. doi: 10.1128/iai.00787-12
Yaribeygi, H., Farrokhi, F. R., Butler, A. E., Sahebkar, A. (2019). Insulin resistance: review of the underlying molecular mechanisms. J. Cell. Physiol. 234 (6), 8152–8161. doi: 10.1002/jcp.27603
Yoneda, M., Naka, S., Nakano, K., Wada, K., Endo, H., Mawatari, H., et al. (2012). Involvement of a periodontal pathogen, Porphyromonas gingivalis on the pathogenesis of non-alcoholic fatty liver disease. BMC Gastroenterol. 12, 1–10. doi: 10.1186/1471-230x-12-16
Yoshimoto, T., Kittaka, M., Doan, A. A. P., Urata, R., Prideaux, M., Rojas, R. E., et al. (2022). Osteocytes directly regulate osteolysis via MYD88 signaling in bacterial bone infection. Nat. Commun. 13 (1), 6648. doi: 10.1038/s41467-022-34352-z
Zawada, A., Rychter, A. M., Ratajczak, A. E., Lisiecka-Masian, A., Dobrowolska, A., Krela-Kazmierczak, I. (2021). Does gut-microbiome interaction protect against obesity and obesity-associated metabolic disorders? Microorganisms 9 (1), 18. doi: 10.3390/microorganisms9010018
Zhao, L., Wu, Y. F., Meng, S., Yang, H., OuYang, Y. L., Zhou, X. D. (2007). Prevalence of fimA genotypes of Porphyromonas gingivalis and periodontal health status in Chinese adults. J. Periodontal Res. 42 (6), 511–517. doi: 10.1111/j.1600-0765.2007.00975.x
Zhou, Q., Leeman, S. E., Amar, S. (2009). Signaling mechanisms involved in altered function of macrophages from diet-induced obese mice affect immune responses. Proc. Natl. Acad. Sci. U S A 106 (26), 10740–10745. doi: 10.1073/pnas.0904412106
Zhou, Q. J., Nino, D. F., Yamaguchi, Y., Wang, S. X., Fulton, W. B., Jia, H. P., et al. (2021). Necrotizing enterocolitis induces T lymphocyte-mediated injury in the developing mammalian brain. Sci. Trans. Med. 13 (575), eaay6621. doi: 10.1126/scitranslmed.aay6621
Zhu, L., Baker, S. S., Gill, C., Liu, W., Alkhouri, R., Baker, R. D., et al. (2013). Characterization of gut microbiomes in nonalcoholic steatohepatitis (NASH) patients: a connection between endogenous alcohol and NASH. Hepatology 57 (2), 601–609. doi: 10.1002/hep.26093
Zhu, M., DeFuria, J., Carr, J., Belkina, A., Van Dyke, T., Gyurko, R., et al. (2014). B cells promote obesity-associated periodontitis and oral pathogen-associated inflammation. J. Immunol. 192, 59–4. doi: 10.1189/jlb.4A0214-095
Keywords: Porphyromonas gingivalis, insulin resistance, diabetes, Alzheimer’s disease, FFA
Citation: Jia S, Li X and Du Q (2023) Host insulin resistance caused by Porphyromonas gingivalis-review of recent progresses. Front. Cell. Infect. Microbiol. 13:1209381. doi: 10.3389/fcimb.2023.1209381
Received: 20 April 2023; Accepted: 27 June 2023;
Published: 13 July 2023.
Edited by:
Cheorl-Ho Kim, Sungkyunkwan University, Republic of KoreaReviewed by:
Michiyo Matsumoto-Nakano, Okayama University, JapanKeke Zhang, Wenzhou Medical University, China
Copyright © 2023 Jia, Li and Du. This is an open-access article distributed under the terms of the Creative Commons Attribution License (CC BY). The use, distribution or reproduction in other forums is permitted, provided the original author(s) and the copyright owner(s) are credited and that the original publication in this journal is cited, in accordance with accepted academic practice. No use, distribution or reproduction is permitted which does not comply with these terms.
*Correspondence: Xiaobing Li, OTA5OTg0OTYzQHFxLmNvbQ==; Qin Du, ZHVxaW5AbWVkLnVlc3RjLmVkdS5jbg==